- 1Department of Biological Sciences, Loyola University New Orleans, New Orleans, LA, United States
- 2Químicas y Farmacia, University of San Carlos, Guatemala City, Guatemala
- 3Department of Biology, University of Vermont, Burlington, VT, United States
Despite successes in reducing transmission, Chagas disease (American trypanosomiasis) remains the greatest economic burden of any parasitic disease in Latin America afflicting mostly the poor and further contributing to poverty. We review a long-term (2001-2022), integrated Ecohealth approach that addresses sustainable development goals to reduce risk of Chagas transmission by the main native vector in Central America, Triatoma dimidiata, s.l. The basis of the Ecohealth intervention was the identification of the risk factors for house infestation, an understanding of and collaboration with local communities, and genetic and proteomic studies that revealed the epidemiology and mechanisms of the rapid reinfestation seen following insecticide application. We review the development of this approach from a pilot project in two Guatemalan villages, to an expanded initiative across three countries with vastly different ecology, cultures, and municipal organization, and finally development of a multi-institutional, large-scale project to develop a strategy to tackle the remaining hot spots in Central America. This integrated Ecohealth approach resulted in reduced risk of transmission as measured by a sustained decrease in house infestation without further use of insecticides, a reduction in vectors with human blood meals and the Chagas parasite, as well as other health and economic benefits. We discuss lessons learned and how this approach could be applied to other vector-borne diseases.
Introduction: Rationale for developing an integrated strategy for sustainable disease control
Most vector borne diseases disproportionately affect the poorest people and are associated with multiple, and often overlapping social, environmental, and biological factors. Initially control of Chagas and many other vector-borne diseases used solely indoor spraying of residual insecticides; an approach that proved inadequate in some localities largely due to reinfestation and insecticide resistance (1–4). Reframing control of vector-borne disease as an integrated strategy addressing these multiple factors can provide a broader range of health, social and economic benefits thus promoting sustainable development. The Future We Want (5) set the stage for the 17 Sustainable Development Goals (SDGs) developed by the United Nations as a “shared blueprint for peace and prosperity for people and the planet, now and into the future” (https://sdgs.un.org/goals). The SDGs highlight the interrelatedness of ecosystems, human societies, and global health, often referred to as Ecohealth or OneHealth, the former in this manuscript.
Here we describe how a Chagas Ecohealth program is driven by local communities and built around this interrelatedness and highlight several SDGs addressed by this approach, improving the lives of over 50,000 people. The program simultaneously addressed reducing Chagas disease by reducing human-vector contact and several health, social, ecological, and economic factors. As we developed the Ecohealth program we became aware that the approach aligns with SDG goals, and by presenting it in this framework, it becomes clear that it is an approach that can be broadly successful, not only for tackling a single disease, but to create the future we want (Figure 1). Of the 17 SDGs, we describe how the Ecohealth program addressed good health and well-being (house improvements that made houses refractory to Chagas vectors), explicitly included establishing partnerships and strengthening institutions (with Ministries of Health, NGOs, local government, etc.), building sustainable communities (use of local materials and forest restoration), and reducing inequalities (improving the highest risk houses, education, and attention to gender issues) while providing economic growth and better nutrition (domestic animal management, planting fruit trees) (Goals 3, 17, 16, 11, 13, 15, 10, 4, 5, 1, 8, 2, 12). An important component of the Chagas Ecohealth approach is that all aspects of the control effort included decision making by members of the communities where Chagas disease is endemic.
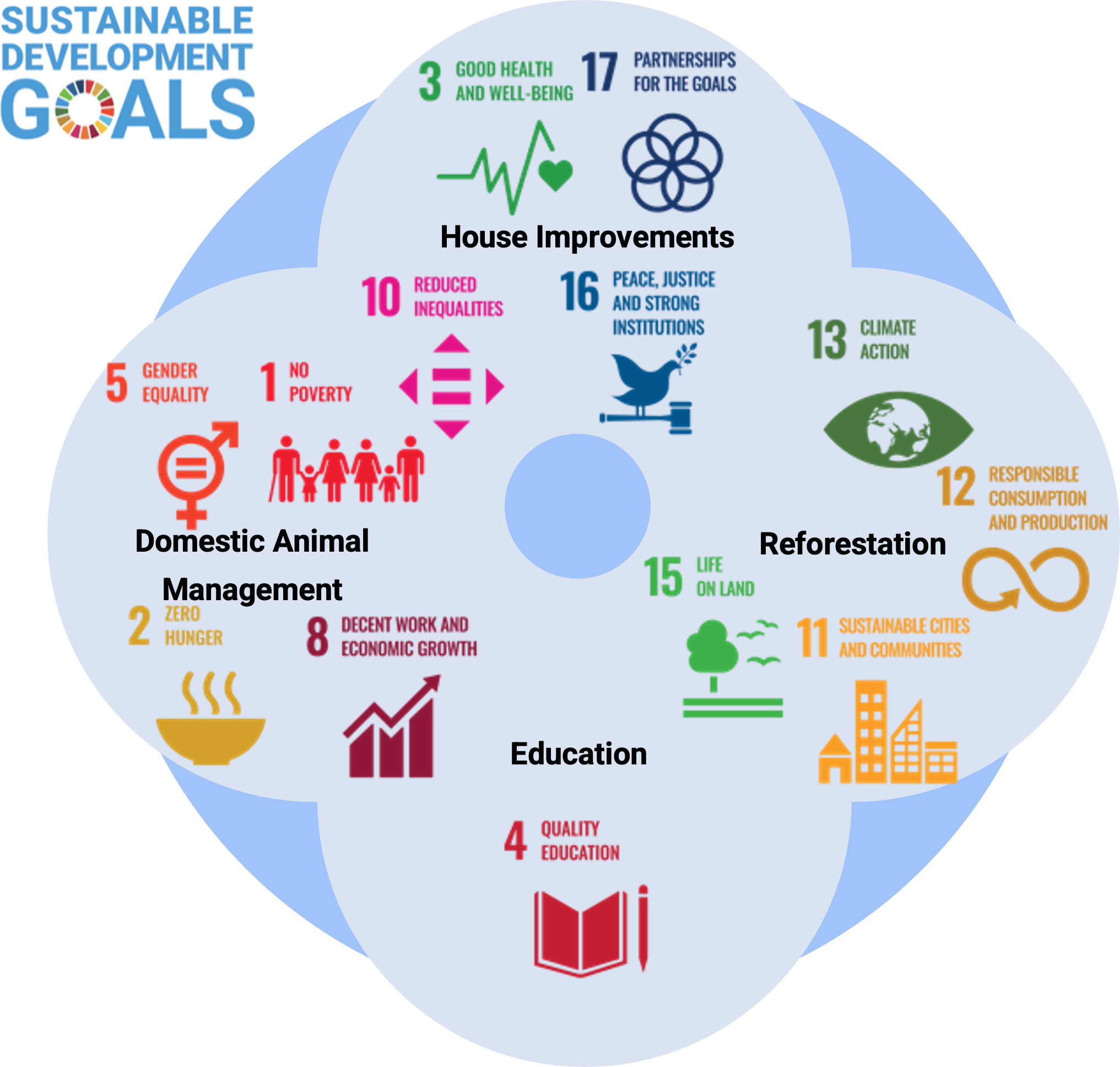
Figure 1 The four pillars of our Ecohealth program align with several of the United Nations Sustainable Development Goals. Early development included Goals 3, 17, 11, 10, and 16. After initial implementation we tackled Goals 1, 2, 8, 12, 13, 15, 4 and 5.
Chagas disease control in Central America: The problem and need for a new strategy
Chagas disease
The risk of Chagas disease is strongly linked with poverty. Traditional housing made of adobe, bajareque (mud and sticks), or vegetable material, common throughout rural Latin America, provides a perfect habitat for triatomines, the vectors of the Chagas parasite, Trypanosoma cruzi, if not maintained to prevent cracks in the walls (Figure 2). The insect vectors, all in the subfamily Triatominae (Hemiptera: Reduviidae), are blood feeders at each lifecycle stage and acquire the parasite from feeding on infected mammals. Triatomines hide during the day, coming out to feed at night. They take in a large quantity of blood necessitating simultaneous defecation, which can leave a liquid drop of T. cruzi-contaminated feces on the skin. A sleeping person may scratch the bite wound or rub the feces into the mucous membranes giving the parasite access to the bloodstream. Although fecal transmission is inefficient, the intense exposure to triatomine bugs for people who live in traditional housing puts them at serious risk of contracting the parasite. In southern Guatemala, 39% of Triatoma dimidiata carry the Chagas parasite (6). Early symptoms are mild and non-specific so often missed. However, ~30% of infected people will progress to a to life-threatening chronic Chagas disease (7) and approximately 5% of infected mothers will transmit the parasite congenitally (8). For diagnosis, at least two serologic tests are recommended (9, 10). Treatment is lengthy, using drugs developed in the 1960s that have limited efficacy in the chronic phase and come with very troublesome side effects (11); new treatments are actively being sought (12). Other less common modes of transmission include contaminated comestibles and solid organ transplant and blood transfusion (7). There is no vaccine available.
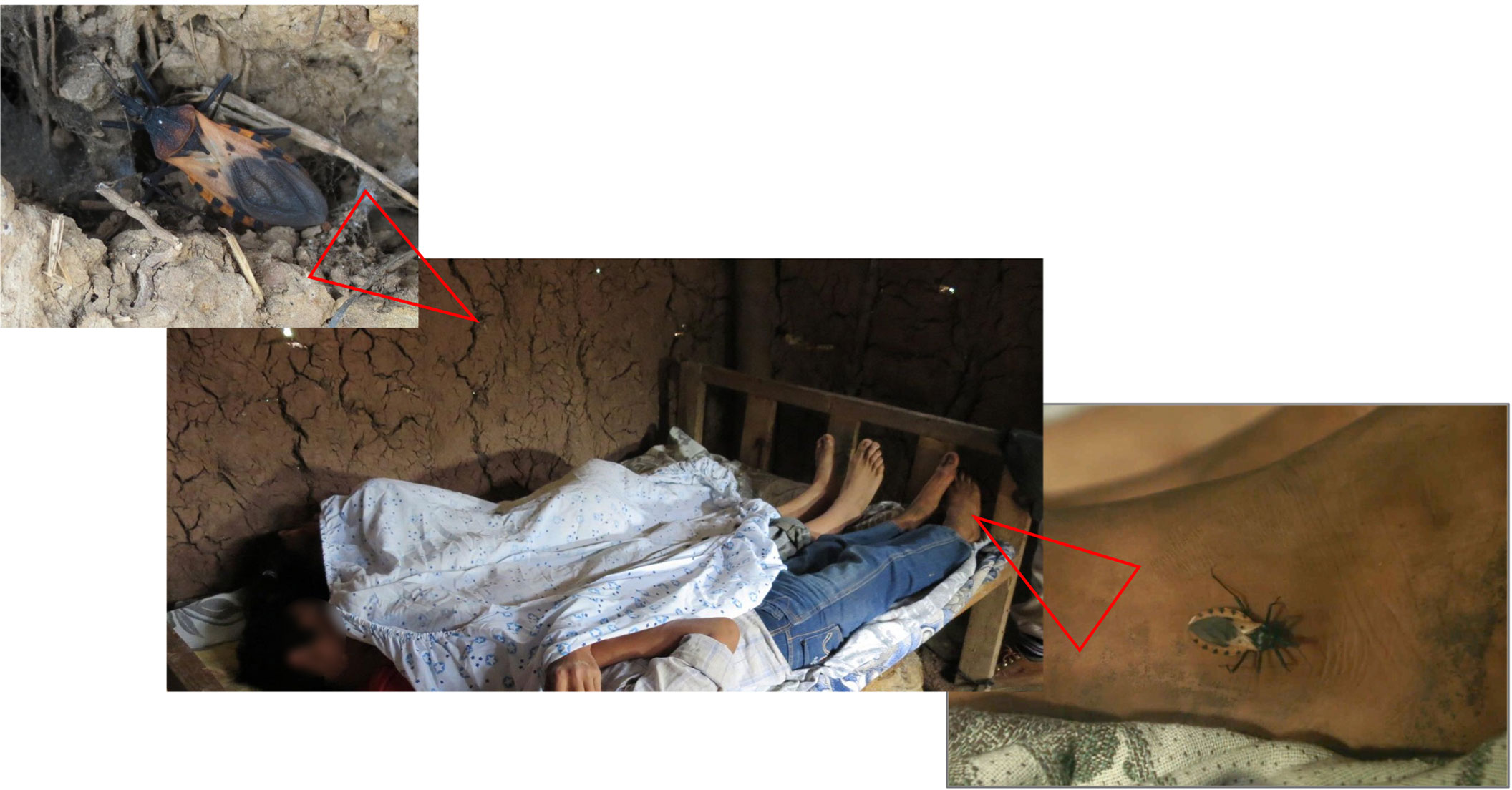
Figure 2 Thousands of people are tormented nightly by bites of the Chagas vectors (triatomine bugs) that live in the cracks and crevices of the adobe houses during the day and crawl down the walls to feed at night. Although transmission through bug feces is inefficient, living in this type of housing places people at risk of contracting Chagas disease due to the intense triatomine bug exposure.
The Chagas situation in Central America
In Central America, the highest prevalence of T. cruzi infection is in Guatemala, El Salvador and Honduras (13). Data is scarce and test kits, based on South American parasite strains, are known to miss many infections (10, 14), so the most recently published human T. cruzi-positive percentage (0.9 – 1.3%) (15) likely underestimates the true infection prevalence. Estimates suggest that 12% of the Central American population lives in conditions placing them at risk of Chagas, so it remains a serious public health issue (13).
Why Chagas control in Central America needs an integrated strategy
Rhodnius prolixus and Triatoma dimidiata sensu lato (s.l.) have been the most important Chagas vectors in Central America. R. prolixus, native to South America, was inadvertently introduced (16), but fortunately restricted to mainly to domestic ecotopes. The native T. dimidiata s.l. (a complex of at least three species (17),) is also present in sylvan ecotopes; the two species were found together in some localities in a country-wide survey (18). In 1999, the Central America Initiative for the control of Chagas disease of the Pan American Health Organization (IPCA-PAHO) recommended reducing human-vector contact and that vector control be focused on the domestic vector populations, with the goals of eliminating R. prolixus and reducing intradomicilary T. dimidiata s.l (19).. As a native species, the latter was not considered a candidate for elimination. In response, from 2000-2010 in Guatemala, all departments with domestic infestation were targeted, spraying houses with residual insecticides (20, 21). By 2008 it was certified that insecticides successfully eliminated transmission by the introduced species, R. prolixus (22). However, T. dimidiata s.l. frequently reinfested treated areas (1, 20, 23–25), requiring reapplication (26, 27). It was realized insecticide spraying would not control domestic T. dimidiata s.l. especially under conditions of low infestation (1). Repeated application is beyond the resources of many affected countries, insecticides can cause adverse environmental and human health effects, and resistance may emerge, as seen in the South American Chagas vector, T. infestans (3).
Control of T. dimidiata s.l. is challenging because its presence is dependent on interacting social, biological, and environmental factors. Deforestation, common in impoverished areas, removes vector habitat (e.g., animal nests) driving vectors into houses. Houses made of traditional materials, provide refugia, and animals, especially chickens, kept in houses when outdoor pens are not available, amplify vector populations by providing blood sources. Biologically, the T. dimidiata s.l. complex of species includes some in the process of domestication (17). The vector is also highly mobile among ecotopes and seasonally (4, 28), posing additional challenges to control. Insecticide application is only a provisional effort that does not prevent vectors from entering houses. Because of all these interrelated factors it was necessary to develop an integrated strategy centered around the communities that addresses the multiple risk factors.
To address this complicated zoonosis, the Ecohealth approach was focused on preventing human-vector contact with the goal of developing strong, sustainable communities (29–32). We developed interventions based on Ecohealth principles for the control of T. dimidiata s.l. beginning in 2000, with identification of risk factors for domestic infestation of T. dimidiata s.l (33, 34).. Below we describe the research that contributed to the development of this Ecohealth program.
Studies informing the integrated, Ecohealth approach
As a data-driven approach, we conducted genetic and ecological studies to distinguish vector taxa to target for intervention, understand the mechanism of rapid reinfestation including any regional differences, evaluate the success of the intervention and clarify the epidemiology. These genetic and ecological studies used both proteomic and DNA-based approaches and included reciprocal training of students across institutions and countries (35–41).
Identification of important vector taxa: Systematics
Vector taxonomic studies are important for identifying genetic lineages that vary in ecology which may impact epidemiological importance and require different intervention strategies. Different responses to insecticide treatment in different localities led us to investigate if there were differences among T. dimidiata s.l. populations at the systematic level related to epidemiological importance, for example, vectors that live year-round or visit houses seasonally or vary in T. cruzi infection prevalence. Many studies have shown that T. dimidiata s.l., the Triatominae species with the largest geographic range of any residing in contiguous continents (42), is a species complex of at least three independently evolving lineages (43). We have now formally described two of these lineages as new species, Triatoma mopan from the Rio Frio cave in Cayo, Belize (41) and Triatoma huehuetenanguensis from several communities in Huehuetenango, Guatemala (17). Although both species are infected with T. cruzi, and have evidence of human blood meals, as largely sylvan species they are less important for control of Chagas transmission to humans than is T. dimidiata s.l. This taxonomic work is ongoing, and it is likely that more species will be described within T. dimidiata s.l.
Vector movement: Population genetics and blood meal sources
Population genetic studies of vectors are important to assess movement among houses and villages and can explain reinfestation and epidemiological importance of particular populations. Several population genetic studies showed high mobility of the domestic and peridomestic populations of T. dimidiata s.l. in southern Guatemala. With low mobility one would expect high relatedness of individual vectors within a house and high genetic differentiation among houses and villages. However, Random Amplified Polymorphic DNA (RAPD) and microsatellite markers showed low relatedness of T. dimidiata s.l. within houses and low differentiation among houses and villages (39, 44). High mobility was also supported by low genetic differentiation among houses and villages 80 m–100 km apart (45, 46) and among six villages up to 10 km apart (39). Recent studies with over 2,000 SNPs across the genome support this high mobility, especially among nearby houses (4).
A time-course analysis of house infestation and reinfestation with the insect vectors focused on numbers of vectors found and their genetic relatedness in two nearby villages (4). In one village, houses were surveyed, treated with insecticide if infested and re-surveyed eight and 22 months later. After 22 months, 35% of houses were reinfested; genetic analysis indicated spraying was not fully effective and vectors were reinfesting from nearby houses. The second village functioned as an untreated control for seasonal dispersal and again high-infestation houses were sources for neighboring houses. These studies demonstrating high vector mobility showed the focus on individual houses was insufficient; a community level approach was important for sustained reduction of human-vector contact.
Assessment and epidemiology: Vector parasite presence and blood meal sources
Knowledge of the blood sources in the vectors provides an assessment of the effectiveness of the intervention in interrupting human-vector contact, e.g., a reduction in human blood meals following the Ecohealth intervention (37) and also reveals vector mobility and disease epidemiology, e.g., which vertebrates contribute to maintaining vector populations (47). Quantification of the parasite prevalence in the vectors is also important in the assessment of ongoing transmission risk and may reflect shifts to blood sources that do not support the parasite.
Vector feeding ecology studies were both DNA- and proteomics-based and indicate high mobility of vectors among ecotopes and houses. For example, in Anonito, Jutiapa, Guatemala 18-100% of the vectors had blood sources from animals (dogs, chicken, cats and cows) that were not present in the houses where the vectors were collected (40). Surprisingly, 50% of these vectors were nymphs, which are wingless, demonstrating mobility is not restricted to adults. Additional evidence of vector mobility is although 80% of vectors were collected inside houses, these vectors had evidence of feeding both inside (e.g., human) and outside (e.g., cow) of the houses.
Furthermore, studies of vector blood meal sources clarified the disease epidemiology and suggested interventions by indicating which animals were more important in different localities. For example, dog, human, and bird (most likely chicken) were the major blood sources in the highly domesticated populations of T. dimidiata s.l. in southern Guatemala (37, 40, 48, 49). In El Salvador, there was little feeding on dog and more feeding on mouse and pig; whereas in Honduras, there was less feeding on bird and more on mouse (48). These differences may have to do with the prevalence of the animal and whether they sleep in the house. The difference also suggests control measures, e.g., controlling dog populations may be more effective at limiting T. dimidiata s.l. proliferation in Guatemala but not El Salvador. Other technologies (e.g., mass spectroscopy and genomics) also proved useful in detecting blood sources (50, 51).
In summary, development and assessment of the impact of the Ecohealth intervention was realized by systematic, population genetic and molecular ecology studies. Discovering regional differences in vector taxa including behavior indicated the need to develop a location-specific approach. Vector population genetics and blood meal sources revealed the high mobility of both adults and nymphs, can explain the rapid reinfestation and supported a regional, rather than individual household intervention. Studies of blood meal sources supported the high migration and revealed regional differences in epidemiology. Reduced human blood sources along with decreased parasite prevalence in the vectors were early indicators of the success of the Ecohealth intervention. Sustainability of the changes was increased by partnerships (Goal 17) that played an important role in developing these assessment tools and training of students across countries and cultures, community members as well as Ministry of Health personnel including physicians, nurses, vector-control technicians, and home health workers increased overall education (Goal 4).
Determining the environmental and social risk factors for infestation: Community surveys
In addition to the biology, development of the integrated approach required an understanding of the environmental and social determinants that contribute to Chagas transmission and cultural practices that might influence these. We conducted extensive community surveys which included Knowledge, Attitude and Practice (KAP) questions alongside an assessment of house conditions and vector infestation and the data were used to develop models to understand the most important risk factors for T. dimidiata s.l. infestation. Early studies suggested that environmental conditions including dirt floor and poor hygiene conditions, such as clutter in and around houses, were risk factors for T. dimidiata infestation in Costa Rica (52, 53). These factors as well as deteriorated walls made of vegetable fiber, mud and sticks (bajareque) or adobe were also identified as risk factors in Guatemala (54). T. dimidiata s.l. was usually found in dark corners on the walls near beds and chicken nests in these traditionally built houses (54, 55). A statistical analysis of 17 possible risk factors for intradomicilary T. dimidiata s.l. infestation in Guatemala documented the link of low socioeconomic status with infestation along with: house age, house condition (including construction materials and condition of plaster), house hygiene, cement vs. dirt floor and animals in the houses (33). From this data three broad categories of houses were described: type A, lowest risk and most prosperous, built of cement block or completely plastered adobe; type B, intermediate with at least partially plastered adobe walls; and, type C, the highest risk with no plaster or cracked plaster on walls, animals in the house and clutter. These categories have been further refined to a quantitative scoring system of house types developed from a large-scale study (11 villages, >2,000 houses) in Guatemala, Honduras, and El Salvador to objectively classify houses with respect to their infestation risk (34). The model generated from this statistical analysis had moderate ability to predict infested houses and excellent ability to predict uninfested houses. Later work developed more sophisticated modeling methods to improve sampling and data-based interventions. One study modeled complex interactions of risk factors and identified mutually exclusive sets of risk-factors, for example, (chicken coops AND deteriorated bedroom walls) OR (accumulation of objects AND dirt floors AND many occupants AND over 3.5 years of electricity) (56). A later model, built on the threshold of 8% for human transmission (57), developed a sampling strategy that used less personnel and time by targeting houses with high risk of infestation and random sampling instead of the more traditional strictly random sampling (58).
The results of these community surveys on environmental and social risk factors of infestation provided key information reinforcing the need for an integrated Ecohealth approach. The most important SDGs at this stage were the realization that good health and wellbeing required reduction in poverty and inequalities (Goals 3, 1 and 10).
Below we describe three major collaborative studies developing and implementing the Ecohealth approach. First, development and piloting of the approach in the heavily infested department of Jutiapa, Guatemala; second, testing the broader applicability in areas that differ in ecology, culture, ethnicity, and social administrative structure in Guatemala, El Salvador, and Honduras; and third, a large-scale intervention leveraging the participation of many institutions to establish protocols to target remaining hot spots and eliminate Chagas across Central America.
Implementation and assessment
Data-driven development of integrated, community-engaged Ecohealth approach: Pilot project (2001-2008)
For our initial development of the integrated Ecohealth intervention, our transdisciplinary team engaged two highly endemic communities in southern Guatemala (La Brea and El Tule, Quesada, Jutiapa). These villages, despite regular insecticide spraying by the Ministry of Health, showed substantial reinfestation (38). Led by a medical entomologist we shared the data of household risk factors with the community (Figure 3A) and following an initial insecticide spray to knock down the numbers of vectors inside the houses, we jointly selected four interventions to maximally reduce T. dimidiata s.l. infestation and support development. These four interventions included (1): wall plastering to remove the cracks and crevices and make the houses refractory to the insect vectors, (2) cementing the floors to remove habitat for egg laying, and (3) building chicken coops and moving the chickens outside and away from the house thus removing a food source that amplifies the vector populations while simultaneously increasing nutrition and promoting economic development, and (4) reforestation with native trees to replace vector habitat and support economic development. In the first two years, with the help of anthropologists and sociologists, we studied community practices and gender roles to ensure that interventions were culturally appropriate and sustainable (30, 59, 60). We learned that wall plastering was the most common house improvement undertaken; traditionally, women, using their hands, plaster the walls to beautify the house (30). Customarily just mud was used, which rapidly cracks, recreating triatomine hiding places. To improve the plaster, community residents supplied locally - sourced materials, including sand, dirt, and volcanic ash. Architects and engineers evaluated the materials and designed a new formulation more resistant to cracking (Figure 3B), which is adapted to each village by first testing the adherence of plasters made with different ratios of sand to dirt. They also formulated a “cement-like” floor using volcanic ash and lime at approximately 1/10th the cost of commercial cement. Where river sand was far from the village, the Municipality assisted with transportation of the materials. We worked alongside the Vector Control Program personnel of the Ministry of Health while they conducted the entomological surveys and applied insecticide. Once optimized, instruction was provided to the larger community by two young men that we hired from the village (one shown instructing, Figure 3C), enabling community residents to make the improvements to their own houses (Figure 3D). The process was sequenced so once the walls were plastered, supplies were provided to complete the cement-like floors, then chicken wire was provided, and the householders constructed chicken coops and moved their chickens outside the house (Figure 4). Later fruit tree seedlings were provided, and a plant nursery established in the village. Throughout the process we included education on Chagas disease transmission and house and yard hygiene and provided training to the community residents and Ministry of Health personnel.
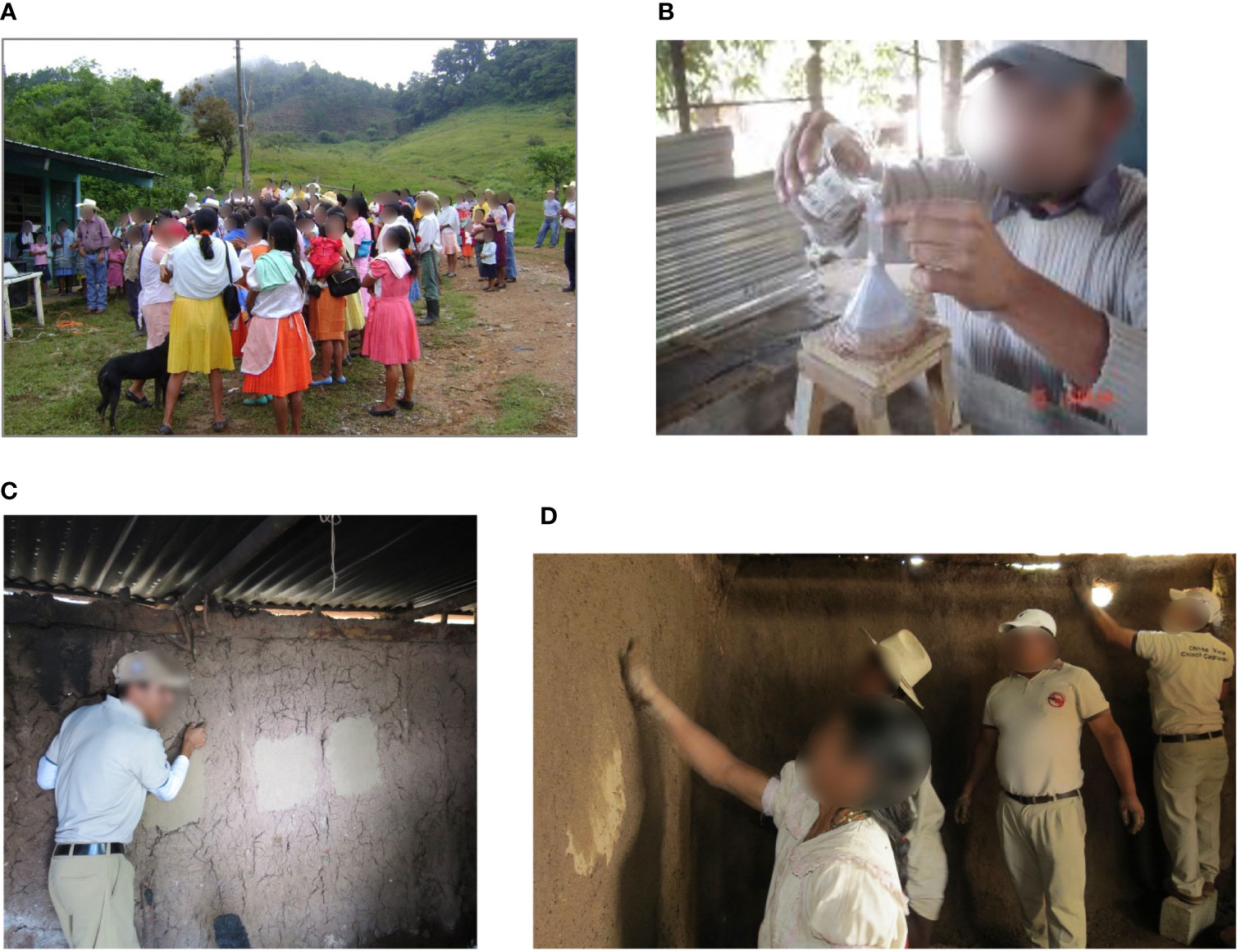
Figure 3 The Ecohealth approach to Chagas vector control engages the community (A) and uses local materials and cultural practices so it is sustainable and acceptable to the community. Architects and engineers, using local materials supplied by the community developed a new formulation that would keep the walls and floors refractory to the triatomine bugs for years (B), a technician we hired from the community (C), and ministry of health personnel (along with researchers, not pictured) teach and work alongside the community, using familiar cultural practices, to implement the approach (D).
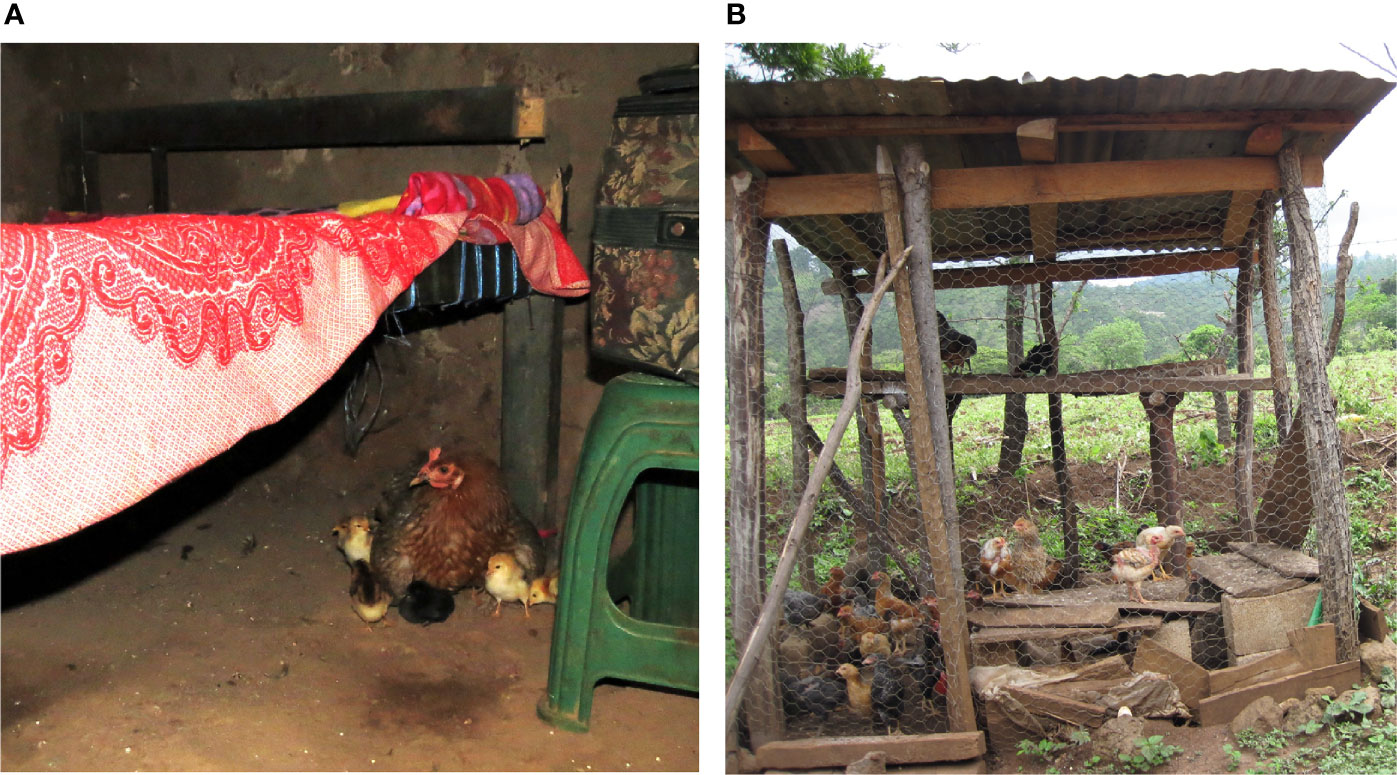
Figure 4 Chickens are traditionally kept near the bed for their protection (A), which amplifies the vector population and increases human-vector contact. As part of the Ecohealth intervention, chicken wire was supplied to community residents who constructed outdoor chicken coops (B) removing the vector blood source from the houses and increasing chicken production.
In choosing and implementing interventions, ecosystem effects were considered and mitigated. For example, we provided fruit trees that the community planted to replace trees harvested for chicken coops and to promote reforestation, improve nutrition and develop new economic opportunities, like selling the fruit.
Ecohealth includes pre- and post-intervention assessment, and we used multiple ways to evaluate the impact such as community participation, house condition, infestation, blood meal sources and community satisfaction. Assessments and interventions were conducted over several years in two villages, La Brea (2001-2020) (38) (Pereira et al., submitted), and El Tule (2004-2011) (37). There was strong community participation, 87% or 90% of community residents improving their houses, La Brea and El Tule, respectively, which correlated with a reduction in “at risk” houses from 62% to 15% (La Brea, 2002-2009) and 39% to 18% (El Tule, 2004-2009). Annual household infestation measures along with post-intervention socioeconomic surveys 4–5 years out documented a statistically significant and sustained decrease in infestation in both villages receiving the Ecohealth interventions (from 9.3 to 1.5% and 25 to 4.6%) concomitant with a significant improvement in the quality of the houses. This sustained reduction in infestation is dramatically different to the reinfestation that previously occurred (1, 27, 38). Indeed, two years prior to the intervention, 42% of homesteads were infested in one village despite insecticide spraying all the known infested houses one year prior (38). Importantly, in both villages infestation remained below the 8% threshold of infested houses where transmission is unlikely (57). We also studied blood meal sources in El Tule and following wall plastering the blood meals of the insect vectors had shifted away from human blood sources (almost 40% before interventions to less than 10% after and none in the five T. dimidiata s.l. collected at the final survey), indicating reduced human-vector contact and that changes in blood sources can be used as an early marker to test the effectiveness of the interventions (37). A decrease in T. cruzi infection in the vectors (~50% in 2004, < 20% in 2009 and 0 in 2011) correlated with this shift in blood sources (37), which may be due to greater feeding on chickens, as the parasite does not replicate in birds. There was also a higher number of houses with chicken coops following the intervention and community residents reported eating and selling more chickens and eggs. The survey in 2020 in La Brea indicated unanimous satisfaction with the Ecohealth intervention among community residents that performed the intervention. They noted the low cost of the intervention, the ease of maintenance and an improvement in their social status (Figure 5) (Pereira, et al., submitted).
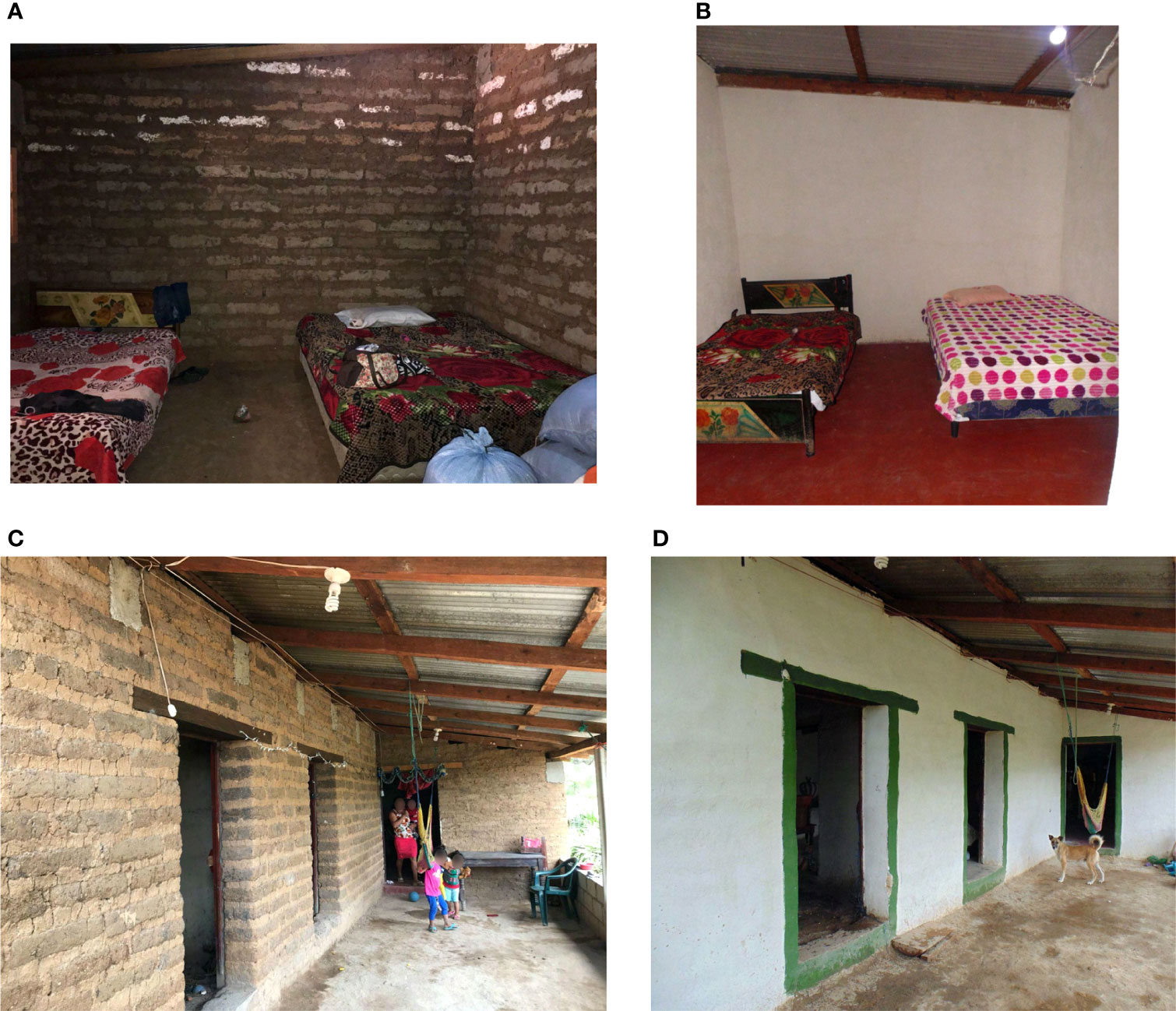
Figure 5 The same house before (A, C) and after (B, D) Ecohealth improvements inside and outside, respectively. Once the houses are improved the finished walls and floors and brighter interior encourages residents to keep it clean, which removes bug refugia and further reduces infestation.
In addition to the assessment of movement and blood meal sources, spatial analysis of the distribution of vectors revealed no evidence of clustering of the 31 T. dimidiata s.l.-infested homesteads in one village (38). The village did contain five “hot spots’’, each of which was centered on a single homestead having an exceptionally high vector count and which together accounted for 52% of all vectors collected. High numbers of nymphs were found in these houses indicating ongoing reproduction. These hot spots may explain results of recent studies using markers across the genome documenting “reinfestation” by insecticide survivors and migrants from nearby houses (4). Spatial analysis failed to implicate sylvan or passive transport as sources for reinfestation (38); there was no concentration of infested houses at the periphery of the village or evidence that infested homesteads were concentrated along the main road. The geospatial evaluation reinforced that insecticide spraying focusing on individual houses is not an effective control strategy, rather an integrated approach including house improvements making houses refractory to vectors, in addition to improving the quality of life, provide for sustainable vector control. Even with the hot spots, overall household infestation remained low (58).
In summary, the Ecohealth approach resulted in a sustained reduction in house infestation at a level below 8% where Chagas transmission is unlikely (57), a shift away from human blood sources indicating reduced human-vector contact, and a reduction of T. cruzi infection in the insect vectors, further supporting this approach for the long-term reduction of risk of Chagas (37, 38). In addition, due to an increase in the number of chicken coops, nearly threefold in one village (37), chicken and egg production increased dramatically, which provided more protein and improved the community residents’ economic status. A reduction in prevalence of soil transmitted intestinal parasites in schoolchildren is also expected with the floor improvements and the trees planted provide additional nutritional and economic benefit. Indeed, trees planted in La Brea, Guatemala are producing fruits that are eaten, used in wine making and sold commercially.
Testing the Ecohealth approach in diverse settings: Three countries (2011-2014)
After demonstrating the effectiveness of the integrated Ecohealth approach in the two communities described above, we investigated applying this approach in settings that differ in ecology, cultures and ethnicities, predominant house materials and municipal organization (34). We chose villages in Guatemala (cloud forest to tropical forest), El Salvador (deciduous forest), and Honduras (mountainous pine/oak forest) from the areas of highest Chagas endemicity in Central America and determined the risk factors for infestation with T. dimidiata s.l. to see if the Ecohealth approach would be likely to work in these different settings. Teams from each country, which included Ministry of Health personnel, and in some countries, researchers, conducted the surveys, which again covered family and socioeconomic issues, household practices and care of domestic animals, knowledge of Chagas disease, entomological parameters and house condition. We also used this opportunity to train additional personnel in these countries.
Results of these surveys showed differences in many of these parameters. For example, most houses were made of adobe or cement block in El Salvador, bajareque and adobe in Guatemala, and adobe in Honduras (34). In the villages studied in El Salvador walls had less deteriorated plaster and fewer houses had dirt floors than those in Guatemala or Honduras and residents had generally higher levels of education. Assessment of >2,000 houses across 11 villages showed considerable variation in infestation; the highest was in Guatemala and most of the insects collected were in cracks in walls made of bajareque or adobe. Multimodel inference used to identify predictors of house infestation in these 11 villages found that of the 25 factors assessed, house wall materials and condition, and signs of infestation, (e.g., dead triatomines, exuviae, eggs and characteristic vector fecal streaks) were among the most important predictors. Considerable geographic heterogeneity is indicated by improved predictive power of the model when random village effects are included.
In a later publication, the role of sylvan, synanthropic, and domestic animals in the Chagas transmission cycle was assessed by comparing infestation, blood meal sources and T. cruzi prevalence in vectors collected during the surveys. The villages in Guatemala showed significantly higher infestation and colonization indices (number of houses with nymphs/number of houses surveyed) than El Salvador or Honduras (48). There were also significant differences in blood sources in vectors among the three countries, for example, opossum in Guatemala might be linking sylvatic and domestic transmission cycles whereas in Honduras, dog plays a major role in maintaining domestic transmission and bird, followed by human, were the most prevalent blood sources in El Salvador. T. cruzi infection in vectors was lowest in Guatemala and similar in El Salvador and Honduras.
Despite the differences, risk factors in the three settings reveal that house improvements and domestic animal management are a viable strategy in all of these localities for interrupting Chagas transmission (34, 41); however, the particular interventions most likely to reduce human-vector contact needed to be decided at a local scale. The expansion demonstrated that the approach could be applicable in different ecosystems and cultures in Mesoamerica.
Developing a strategy with multiple institutions to target the remaining hot spots: Partnerships (2018-2022)
Next we undertook a large-scale, multi-disciplinary and inter-agency initiative to engage many communities in a highly endemic area of Guatemala. The goals were to refine the necessary tools, methods, and technology transfer, developing a strategy to address the remaining Chagas hotspots in Central America (32). This large-scale initiative called the “Alliances for the Elimination of Chagas in Central America,” called just “Alliances” below, brought together the Guatemalan Ministry of Public Health and Social Assistance, national academic institutions and international non-governmental institutions (NGOs) including the Pan American Health Organization (PAHO), and for profit companies, each entering into a formal agreement and contributing their expertise in collaboration with the members of the local communities. The initiative included 32 villages in Comapa, and 19 in Jutiapa municipality, both in Jutiapa department, Guatemala. Complete survey data was gathered in 11 villages, including 6,096 people in 1,572 households. This area is known for many of the highest risk factors (rural population, majority living in poverty, high infestation and rapid vector reinfestation). Field work (2018-2021) included socioeconomic and household surveys, vector surveillance and control, house improvements, domestic animal management, reforestation, diagnosis and treatment of individuals, health promotion, training and identification of barriers to diagnosis and treatment. The latter involved workshops held jointly with all the civic organizations involved in Chagas care from the national, to departmental, to community leaders, universities, Chagas patients and international NGO partners to encourage better coordination. Engagement and training of the leaders and community residents of the 51 villages was also provided. The purpose of this large-scale control effort is to demonstrate that investing the resources of several institutions more broadly across a highly endemic area, could result in a geographical area where the vectorial transmission is interrupted by infestation below the threshold for likely transmission and where Chagas patients could be easily diagnosed and treated. After 4-5 years of training, program development and Ecohealth interventions, the management could be guided by the Ministry of Health, with community residents empowered with knowledge about Chagas disease and how to interrupt its transmission and with the satisfaction of achievement of development goals.
There was high household participation in the initial entomological and house condition surveys, 72-98% depending on the village (32). Many of the houses initially belonged to B (medium) or C (high) risk categories; these combined ranged from a high of 79% in a particular village to a low of 47%. Five of the villages had very high infestation (>20% homesteads infested), whereas three had low infestation (<8%). The absence of Rhodnius prolixus and the high prevalence of T. dimidiata s.l. inside the houses in the area were confirmed. All participating houses were treated with insecticide whether or not they were infested and community residents were instructed in house improvements. Participation in improvements varied among villages from a high of 79% of houses improved to a low of 21%. Chagas serological surveys were conducted in the five high infestation villages (2,531 serum samples) with diagnosis offered to the entire population. With high participation (72-73%) the seroprevalence of T. cruzi antibodies was 4-17%, depending on the village (average 11%), and was higher in women than men. Importantly, no child under five years old was found T. cruzi positive, indicating that the transmission was interrupted at least five years previous; there was some infection in 6-19-year-olds, which is likely due to T. dimidiata s.l., as this was after the elimination of R. prolixus. Most Chagas patients are 20-40 years old, likely reflecting infection by R. prolixus.
Barriers to diagnosis and treatment revealed by the Alliances project included centralized diagnosis facilities and a lack of training of healthcare personnel. Development activities included decentralization by the establishment of diagnostic facilities in the departments of Jutiapa, Chiquimula and Jalapa, and a new option of patients providing a sample at their local Health Center for analysis at a more regional facility. This greatly reduced the distance needed to travel for the patients and the time needed to obtain the results. The laboratory in Jutiapa is now fully functional in Chagas diagnosis, Chiquimula and Jalapa laboratories are still being established. Training was provided to people of the Ministry of Health and healthcare providers and there is a new Chagas Clinic in the Comapa Health Center in Jutiapa, with personnel trained in Chagas diagnosis and treatment including managing the challenging side effects of the treatment.
For ongoing vector surveillance, a community-based system was implemented in 32 villages with 80 boxes placed throughout the villages that the community residents could use to deposit any vectors found and so report to the Ministry of Health the reappearance of the triatomines.
By establishing partnerships and strengthening institutions (Goals 17 and 16), this approach demonstrated leveraging diverse expertise from international to national and local levels in a cooperative approach, infestation in a highly endemic area can be reduced below the level where Chagas transmission is likely to occur. The Alliances project supported work in 32 villages in Comapa implementing the already established house improvements, management of domestic animals, reforestation, and education with the addition of a community vector surveillance system to monitor and ensure maintenance of infestation below the level of likely transmission. In addition, the Ecohealth control strategy was extended to include locally available diagnosis and treatment. This multi-institutional approach of implementing the Ecohealth strategy can be applied to remaining hot spots of Chagas transmission in Mesoamerica to work towards eliminating Chagas disease as well as other vector borne diseases.
Conclusions: Data-driven, integrated Ecohealth approach leads to innovative, sustainable vector control strategies
The integrated Ecohealth vector control approach, initially based on reducing the risk factors for infestation of native Chagas disease vectors, proved to be successful in sustained reduction of vector transmission and promoted sustainable development (31, 37, 38)(Pereira et al., submitted). Beginning by demonstrating success in two villages over 20 years ago, it was expanded to three countries in Central America validating the approach for different ecologies, cultures, and social systems. More recently, the Alliances project, through partnerships, extended the strategy adding local diagnosis and treatment to tackle remaining hot spots on a large scale. Taken together, the Ecohealth intervention has improved the health and well-being of more than 50,000 people through integrated social transformations that eventually improved family health, household infrastructure and economics (31)(Pereira et al., submitted).
Ecohealth requires integrated actions among stakeholders (the community, universities, NGOs, municipalities, and Ministries of Health); this requires more organization and coordination than simply spraying insecticides. The Alliances project goal was to develop a strategy that could be overseen by the Ministry of Health to implement the required house improvements, relocation of domestic animals, reforestation and education along with developing the infrastructure and training for local diagnosis and treatment. In this process, interdisciplinary and intersectional participation is essential requiring more planning, negotiation, and coordination among groups. The Chagas Ecohealth approach especially required facilitating the development of relationships among people of the Ministry of Health and other organizations; strong partnerships are required to successfully implement the approach. With this approach, developing countries can be free of dependence on imported insecticides, avoid their adverse ecological effects, and can further sustainable development.
Full partnership with the community residents is key to acceptance and sustainability over the long term. Community residents have essential knowledge; they also perform the work and make the modifications according to their preferences. In building relationships with the communities, hiring community residents and developing their leadership skills was important. Their leadership, respect and identity within the communities was fundamental to the adoption of the strategy, as these individuals became examples as they were often the first to implement house improvements. Close, long-term collaboration with local Ministry of Health workers encouraged an open dialogue among the partners, establishing trust and empathy (31), enabling us to address gender issues. We acknowledged the power of women to influence people’s behaviors, both with respect to encouraging reduced human-vector contact, and to discovering economic development opportunities arising from movement of domestic animals and reforestation (59, 60). Surveillance is essential to long-term vector control. In addition to the benefits we describe above, an analysis of studies conducted across Latin America showed that community participation is more effective than active searches by trained personnel (61). They recommend community participation as a strategic component for long-term Chagas vector surveillance (61).
Our study across three countries in Central America acknowledged the cultural diversity of this region (indigenous Maya, Chorti, Xinca, Lencas as well as Ladino, etc.) and emphasized that Chagas control be adapted to local communities’ identity. Each village has unique cultural factors defining identity, requiring that development and implementation of the control strategies be culturally and gender appropriate (59). Community engagement varies among villages; although remarkably high, some have very high community participation (98%) and others lower (72%) (32). In some isolated villages where the transportation of the materials was done by the municipality, this involvement motivated the interventions. Affordability of the local materials is an important issue for sustainability (Pereira et al., submitted), the closer the proximity of the materials, the easier to maintain the house improvements. The attitudes of community leaders (formal and informal), access to education, the mentoring of local managers and the expectation of better living conditions are among the factors that influenced the adoption of the house improvements and other aspects of the Ecohealth interventions (59, 60) (Pereira et al., submitted).
Future challenges are to use these sustainable development goals to address additional diversity influencing Chagas transmission including other vector species. During our extensive work in Guatemala, we reported two new species within the T. dimidiata complex and have evidence of additional species yet to be described. In Mexico, there are 17 different vector species of which four live in houses year-round. Additional challenges are vectors that only enter houses seasonally. We have learned from working across three countries that the approach can and needs to be adapted to local situations including local vector species. The recently published “TriatoScore” assigning an epidemiological-risk score for Chagas disease vector control and surveillance looks to be a useful tool to adapt the effort to local conditions (62).
Although reducing human-vector contact is the strongest way to reduce Chagas and other vector borne diseases, currently, there is little interest in funding vector control strategies; however, there is increasing interest in sustainable development. Our development of the Ecohealth strategy for Chagas control demonstrates the opportunity of improved health in the context of the sustainable development goals. It is a stronger way forward to the future we want.
Author contributions
PD, MM, and LS conceived the review, reviewed the literature, drafted, reviewed, edited and approved the manuscript. All authors contributed to the article and approved the submitted version.
Funding
Funding was provided by the IDRC (grants 101812, 106531–001 and 108651-001), the National Institute of Allergy and Infectious Diseases (NIAID) at the U.S. National Institutes of Health (NIH) (grant R03AI26268/1–2) and the National Science Foundation (NSF) (grant BCS-1216193) as part of the joint NSFNIH-USDA (United States Department of Agriculture) Ecology and Evolution of Infectious Diseases program. The funders had no role in the study design, data collection and analysis, decision to publish, or preparation of the manuscript.
Acknowledgments
We are grateful for the collaboration of the residents, the Ministries of Health, for fieldwork and logistical support of Belter Alcantara and Antonieta Rodas and work of students from University of San Carlos, Guatemala, University of Vermont, USA and Loyola University New Orleans, USA.
Conflict of interest
The authors declare that the research was conducted in the absence of any commercial or financial relationships that could be construed as a potential conflict of interest.
Publisher’s note
All claims expressed in this article are solely those of the authors and do not necessarily represent those of their affiliated organizations, or those of the publisher, the editors and the reviewers. Any product that may be evaluated in this article, or claim that may be made by its manufacturer, is not guaranteed or endorsed by the publisher.
Author disclaimer
Any opinions, findings, and conclusions or recommendations expressed in this material are those of the author(s) and do not necessarily reflect the views of the funding organizations.
Abbreviations
SDGs, Sustainable Development Goals; PAHO, Pan American Health Organization; IPCA, Central America Initiative for the Control of Chagas disease; NGOs, Non-Governmental Organizations; SNPs, Single Nucleotide Polymorphisms.
References
1. Yoshioka K, Nakamura J, Perez B, Tercero D, Perez L, Tabaru Y. Effectiveness of Large-scale chagas disease vector control program in Nicaragua by residual insecticide spraying against. Triatoma dimidiata Am J Trop Med Hyg (2015) 93(6):1231–9. doi: 10.4269/ajtmh.15-0403
2. Dumonteil E, Escobedo-Ortegon J, Reyes-Rodriguez N, Arjona-Torres A, Ramirez-Sierra MJ. Immunotherapy of Trypanosoma cruzi infection with DNA vaccines in mice. Infect Immun (2004) 72(1):46–53. doi: 10.1128/IAI.72.1.46-53.2004
3. Mougabure-Cueto G, Picollo MI. Insecticide resistance in vector chagas disease: Evolution, mechanisms and management. Acta Trop (2015) 149:70–85. doi: 10.1016/j.actatropica.2015.05.014
4. Cahan SH, Orantes LC, Wallin KF, Hanley JP, Rizzo DM, Stevens L, et al. Residual survival and local dispersal drive reinfestation by Triatoma dimidiata following insecticide application in Guatemala. Infect Genet Evol (2019) 74:104000. doi: 10.1016/j.meegid.2019.104000
5. General assembly UN. the future we want, a/Res/66/288 (2012). Available at: https://www.un.org/en/development/desa/population/migration/generalassembly/docs/globalcom pact/A_RES_66_288.pdf.
6. Dorn PL, Engelke D, Rodas A, Rosales R, Melgar S, Brahney B, et al. Utility of the polymerase chain reaction in detection of Trypanosoma cruzi in Guatemalan chagas' disease vectors. Am J Trop Med Hyg (1999) 60(5):740–5. doi: 10.4269/ajtmh.1999.60.740
7. World Health Organization. Chagas disease (American trypanosomiasis) (2018) [November, 28, 2018] . Available at: https://www.who.int/en/news-room/factsheets/detail/chagas-disease-(american-trypanosomiasis).
8. Howard EJ, Xiong X, Carlier Y, Sosa-Estani S, Buekens P. Frequency of the congenital transmission of Trypanosoma cruzi: A systematic review and meta-analysis. BJOG (2014) 121(1):22–33. doi: 10.1111/1471-0528.12396
9. Organización Panamericana de la Salud. Guía Para El Diagnóstico Y El Tratamiento de la Enfermedad De Chagas (2018). Available from: http://iris.paho.org/xmlui/handle/123456789/49653 .
10. Hochberg NS, Wheelock A, Hamer DH, Marcus R, Nolan MS, Meymandi S, et al. Chagas disease in the united states: A perspective on diagnostic testing limitations and next steps. Am J Trop Med Hyg (2021) 104(3):800–804. doi: 10.4269/ajtmh.19-0871
11. Sales Junior PA, Molina I, Fonseca Murta SM, Sanchez-Montalva A, Salvador F, Correa-Oliveira R, et al. Experimental and clinical treatment of chagas disease: A review. Am J Trop Med Hyg (2017) 97(5):1289–303. doi: 10.4269/ajtmh.16-0761
12. García-Huertas P, Cardona-Castro N. Advances in the treatment of chagas disease: Promising new drugs, plants and targets. BioMed Pharmacother (2021) 142:112020. doi: 10.1016/j.biopha.2021.112020
13. Peterson JK, Yoshioka K, Hashimoto K, Caranci A, Gottdenker N, Monroy C, et al. Chagas disease epidemiology in central America: An update. Curr Trop Med Rep (2019) 6(2):62–105. doi: 10.1007/s40475-019-00176-z
14. Truyens C, Dumonteil E, Alger J, Cafferata ML, Ciganda A, Gibbons L, et al. Geographic variations in test reactivity for the serological diagnosis of Trypanosoma cruzi infection. J Clin Microbiol (2021) 59(12):e0106221. doi: 10.1128/jcm.01062-21
15. Guhl F. Geographical distribution of chagas disease. In: Telleria J, Tibayrenc M, editors. American Trypanosomiasis Chagas disease: One hundred years of research, Elsevier, vol. . p . Amsterdam, Netherlands (2017). p. 89–112.
16. Dujardin JP, Munoz M, Chavez T, Ponce C, Moreno J, Schofield CJ, et al. The origin of Rhodnius prolixus in central America. Med Vet Entomol (1998) 12:113–5. doi: 10.1046/j.1365-2915.1998.00092.x
17. Lima-Cordón RA, Monroy MC, Stevens L, Rodas A, Rodas GA, Dorn PL, et al. Description of Triatoma huehuetenanguensis sp. n., a potential chagas disease vector (Hemiptera, reduviidae, triatominae). ZooKeys (2019) 820:51–70. doi: 10.3897/zookeys.820.27258
18. Tabaru Y, Monroy C, Rodas A, Mejia M, Rosales R. The geographical distribution of vectors of chagas' disease and populations at risk of infection in Guatemala. Med Ent Zool (1999) 50(1):9–17. doi: 10.7601/mez.50.9_1
19. Organización Panamericana de la Salud. Primera Reunion de la Comisión Intergubernamental de la Iniciativa De Centroamericana Y Belice Para La Interrupción de la Transmision Vectorial de la Enfermedad De Chagas Por Rhodnius prolixus, Disminución de la Infestación Domicilaria Por Triatoma dimidiata Y La Eliminación de la Transmisión Transfusional Del Trypanosoma cruzi. Documento Ops/Hcp/Hct/145/99. Washington, DC: OPS
20. Hashimoto K, Cordon-Rosales C, Trampe R, Kawabata M. Impact of single and multiple residual sprayings of pyrethroid insecticides against Triatoma dimidiata (Reduviiade; triatominae), the principal vector of chagas disease in jutiapa, Guatemala. Am J Trop Med Hyg (2006) 75(2):226–30. doi: 75/2/226 [pii] doi: 10.4269/ajtmh.2006.75.2.0750226
21. Hashimoto K, Alvarez H, Nakagawa J, Juarez J, Monroy C, Cordon-Rosales C, et al. Vector control intervention towards interruption of transmission of chagas disease by Rhodnius prolixus, main vector in Guatemala. Memorias Do Instituto Oswaldo Cruz (2012) 107(7):877–87. doi: 10.1590/s0074-02762012000700007
22. Hashimoto K, Schofield CJ. Elimination of Rhodnius prolixus in central America. Parasites Vectors (2012) 5:45. doi: 10.1186/1756-3305-5-45
23. Dorn PL, Monroy C, Curtis A. Triatoma dimidiata (Latreille, 1811): A review of its diversity across its geographic range and the relationship among populations. Infect Genet Evol (2007) 7(2):343–52. doi: 10.1016/j.meegid.2006.10.001
24. Dumonteil E, Gourbiere S, Barrera-Perez M, Rodriguez-Felix E, Ruiz-Pina H, Banos-Lopez O, et al. Geographic distribution of Triatoma dimidiata and transmission dynamics of Trypanosoma cruzi in the Yucatan peninsula of Mexico. Am J Trop Med Hyg (2002) 67:176–83. doi: 10.4269/ajtmh.2002.67.176
25. Dumonteil E, Ruiz-Pina H, Rodriguez-Felix E, Barrera-Perez M, Ramirez-Sierra MJ, Rabinovich JE, et al. Re-infestation of houses by Triatoma dimidiata after intra-domicile insecticide application in the Yucatan peninsula, Mexico. Mem Inst Oswaldo Cruz (2004) 99(3):253–6. doi: 10.1590/S0074-02762004000300002
26. Manne J, Nakagawa J, Yamagata Y, Goehler A, Brownstein JS, Castro MC. Triatomine infestation in Guatemala: Spatial assessment after two rounds of vector control. Am J Trop Med Hyg (2012) 86(3):446–54. doi: 10.4269/ajtmh.2012.11-0052
27. Nakagawa J, Hashimoto K, Cordon-Rosales C, Abraham Juarez J, Trampe R, Marroquin Marroquin L. The impact of vector control on Triatoma dimidiata in the Guatemalan department of jutiapa. Ann Trop Med Parasitol (2003) 97(3):288–97. doi: 10.1179/000349803235001895
28. Monroy MC, Bustamante DM, Rodas AG, Enriquez ME, Rosales RG. Habitats, dispersion and invasion of sylvatic Triatoma dimidiata (Hemiptera: Reduviidae: Triatominae) in peten, Guatemala. J Med Entomol (2003) 40(6):800–6. doi: 10.1603/0022-2585-40.6.800
29. Monroy C, Rodas A, Mejia M, Tabaru Y. Wall plastering and paints as methods to control vectors of chagas disease in Guatemala. Med Entomol Zool (1998) 49(3):187–93. doi: 10.7601/mez.49.187
30. Monroy C, Bustamante DM, Pineda S, Rodas A, Castro X, Ayala V, et al. House improvements and community participation in the control of Triatoma dimidiata re-infestation in jutiapa, Guatemala. Cad Saude Publica (2009) 25 Suppl 1:S168–78. doi: 10.1590/s0102-311x2009001300016
31. Castro-Arroyave D, Monroy MC, Irurita MI. Integrated vector control of chagas disease in Guatemala: A case of social innovation in health. Infect Dis Poverty (2020) 9(1):25. doi: 10.1186/s40249-020-00639-w
32. Monroy MC, Penados D, Pineda J, Ruiz EL, Agreda EO, Alcantara B, et al. A multidisciplinary, collaborative, inter-agency and comprehensive approach for the control of chagas disease as a public health problem in Guatemala. Acta Trop (2022) 225:106157. doi: 10.1016/j.actatropica.2021.106157
33. Bustamante DM, Monroy C, Pineda S, Rodas A, Castro X, Ayala V, et al. Risk factors for intradomiciliary infestation by the chagas disease vector Triatoma dimidiata in jutiapa, Guatemala. Cad Saude Publica (2009) 25 Suppl 1:S83–92. doi: 10.1590/s0102-311x2009001300008
34. Bustamante Zamora DM, Hernandez MM, Torres N, Zuniga C, Sosa W, de Abrego V, et al. Information to act: Household characteristics are predictors of domestic infestation with the chagas vector Triatoma dimidiata in central America. Am J Trop Med Hyg (2015) 93(1):97–107. doi: 10.4269/ajtmh.14-0596
35. de la Rua NM, Bustamante DM, Menes M, Stevens L, Monroy C, Kilpatrick CW, et al. Towards a phylogenetic approach to the composition of species complexes in the north and central American Triatoma, vectors of chagas disease. Infect Genet Evol (2014) 24:157–66. doi: 10.1016/j.meegid.2014.03.019
36. Richards B, la Rua NM, Monroy C, Stevens L, Dorn PL. Novel polymerase chain reaction-restriction fragment length polymorphism assay to determine internal transcribed spacer-2 group in the chagas disease vector, Triatoma dimidiata (Latreille, 1811). Mem Inst Oswaldo Cruz (2013) 108(4):395–8. doi: 10.1590/S0074-0276108042013001
37. Pellecer MJ, Dorn PL, Bustamante DM, Rodas A, Monroy MC. Vector blood meals are an early indicator of the effectiveness of the ecohealth approach in halting chagas transmission in Guatemala. Am J Trop Med Hyg (2013) 88(4):638–44. doi: 10.4269/ajtmh.12-0458
38. Lucero DE, Morrissey LA, Rizzo DM, Rodas A, Garnica R, Stevens L, et al. Ecohealth interventions limit triatomine reinfestation following insecticide spraying in la brea, Guatemala. Am J Trop Med Hyg (2013) 88(4):630–7. doi: 10.4269/ajtmh.12-0448
39. Stevens L, Monroy MC, Rodas AG, Hicks RM, Lucero DE, Lyons LA, et al. Migration and gene glow among domestic populations of the chagas insect vector Triatoma dimidiata (Hemiptera: Reduviidae) detected by microsatellite loci. J Med Entomol (2015) 52(3):419–28. doi: 10.1093/jme/tjv002
40. Penados D, Pineda JP, Laparra-Ruiz E, Galvan MF, Schmoker AM, Ballif BA, et al. Assessing risk of vector transmission of chagas disease through blood source analysis using lc-Ms/Ms for hemoglobin sequence identification. PloS One (2022) 17(1):e0262552. doi: 10.1371/journal.pone.0262552
41. Dorn PL, Justi SA, Dale C, Stevens L, Galvao C, Lima-Cordon R, et al. Description of Triatoma mopan sp. n. from a cave in Belize (Hemiptera, reduviidae, triatominae). ZooKeys (2018) 775):69–95. doi: 10.3897/zookeys.775.22553
42. Lent H, Wygodzinsky P. Revision of the triatominae (Hemiptera, reduviidae) and their significance as vectors of chagas disease. Bull Am Mus Nat Hist (1979) 163:123–520.
43. Justi SA, Cahan S, Stevens L, Monroy C, Lima-Cordón R, Dorn PL. Vectors of diversity: Genome wide diversity across the geographic range of the chagas disease vector Triatoma dimidiata sensu lato (Hemiptera: Reduviidae). Mol Phylogenet Evol (2018) 120:144–50. doi: 10.1016/j.ympev.2017.12.016
44. Melgar S, Chávez JJ, Landaverde P, Herrera F, Rodas A, Enriquez E, et al. The number of families of Triatoma dimidiata in a Guatemalan house. Mem Inst Oswaldo Cruz (2007) 102(2):221–3. doi: 10.1590/s0074-02762007005000001
45. Calderón C, Dorn P, Melgar S, Chávez J, Rodas A, Rosales R, et al. A preliminary assessment of genetic differentiation of Triatoma dimidiata, (Hemiptera: Reduviidae) in Guatemala by rapd-pcr. J Med Entomol (2004) 41:882–7. doi: 10.1603/0022-2585-41.5.882
46. Dorn PL, Melgar S, Rouzier V, Gutierrez A, Combe C, Rosales R, et al. The chagas vector, Triatoma dimidiata (Hemiptera: Reduviidae), is panmictic within and among adjacent villages in Guatemala. J Med Entomol (2003) 40(4):436–40. doi: 10.1603/0022-2585-40.4.436
47. Zeledon R, Solano G, Zuniga A, Swartzwelder JC. Biology and ethology of Triatoma dimidiata (Latreille, 1811). 3. habitat and blood sources. J Med Entomol (1973) 10(4):363–70. doi: 10.1093/jmedent/10.4.363
48. Lima-Cordón RA, Stevens L, Solorzano Ortiz E, Rodas GA, Castellanos S, Rodas A, et al. Implementation science: Epidemiology and feeding profiles of the chagas vector Triatoma dimidiata prior to ecohealth intervention for three locations in central America. PloS Negl Trop Dis (2018) 12(11):e0006952. doi: 10.1371/journal.pntd.0006952
49. Keller JI, Lima-Cordon R, Monroy MC, Schmoker AM, Zhang F, Howard A, et al. Protein mass spectrometry detects multiple bloodmeals for enhanced chagas disease vector ecology. Infect Genet Evol (2019) 74:103998. doi: 10.1016/j.meegid.2019.103998
50. Keller JI, Ballif BA, St Clair RM, Vincent JJ, Monroy MC, Stevens L. Chagas disease vector blood meal sources identified by protein mass spectrometry. PloS One (2017) 12(12):e0189647. doi: 10.1371/journal.pone.0189647
51. Kieran TJ, Gottdenker NL, Varian CP, Saldana A, Means N, Owens D, et al. Blood meal source characterization using illumina sequencing in the chagas disease vector Rhodnius pallescens (Hemiptera: Reduviidae) in Panama. J Med Entomol (2017) 54(6):1786–9. doi: 10.1093/jme/tjx170
52. Zeledon R, Vargas LG. The role of dirt floors and of firewood in rural dwellings in the epidemiology of chagas' disease in Costa Rica. Am J Trop Med Hyg (1984) 33(2):232–5. doi: 10.4269/ajtmh.1984.33.232
53. Starr MD, Rojas JC, Zeledon R, Hird DW, Carpenter TE. Chagas' disease: Risk factors for house infestation by Triatoma dimidiata, the major vector of Trypanosoma cruzi in Costa Rica. Am J Epidemiol (1991) 133(7):740–7. doi: 10.1093/oxfordjournals.aje.a115949
54. Tabaru Y, Monroy C, Rodas A, Mejia M. Chagas' disease surveillance in various residences in Santa maria ixhuatan, department of Santa Rosa, Guatemala. Med Entomol Zool (1999) 50(1):19–25. doi: 10.7601/mez.50.19
55. Monroy C, Mejía M, Rodas A, Rosales R, Horio M, Tabaru Y. Comparison of indoor searches with whole house demolition collections of the vectors of chagas disease and their indoor distribution. Med Entomol Zool (1998) 49(3):195–200. doi: 10.7601/mez.49.195
56. Hanley JP, Rizzo DM, Stevens L, Helms Cahan S, Dorn PL, Morrissey LA, et al. Novel evolutionary algorithm identifies interactions driving infestation of Triatoma dimidiata, a chagas disease vector. Am J Trop Med Hyg (2020) 103(2):735–44. doi: 10.4269/ajtmh.18-0733
57. Aiga H, Sasagawa E, Hashimoto K, Nakamura J, Zuniga C, Chevez JE, et al. Chagas disease: Assessing the existence of a threshold for bug infestation rate. Am J Trop Med Hyg (2012) 86(6):972–9. doi: 10.4269/ajtmh.2012.11-0652
58. Case BKM, Young JG, Penados D, Monroy C, Hebert-Dufresne L, Stevens L. Spatial epidemiology and adaptive targeted sampling to manage the chagas disease vector Triatoma dimidiata. PloS Negl Trop Dis (2022) 16(6):e0010436. doi: 10.1371/journal.pntd.0010436
59. Triana DRR, Mertens F, Zúniga CV, Mendoza Y, Nakano EY, Monroy MC. The role of gender in chagas disease prevention and control in Honduras: An analysis of communication and collaboration networks. EcoHealth (2016) 13:535–48. doi: 10.1007/s10393-016-1141-9
60. Soto López JD, Monroy MC, Dorn P, Castellanos S, Lima R, Rodas A. Effect of community education in an integrate control for Triatoma dimidiata (Hemiptera:Reduviidae). Rev Cubana Medicina Trop (2019) 71(4):e380. http://revmedtropical.sld.cu/index.php/medtropical/article/view/380
61. Abad-Franch F, Vega MC, Rolon MS, Santos WS, Rojas de Arias A. Community participation in chagas disease vector surveillance: Systematic review. PloS Negl Trop Dis (2011) 5(6):e1207. doi: 10.1371/journal.pntd.0001207
Keywords: sustainable development goals - SDGs, vector control, Triatoma dimidiata, Central America, Chagas disease, Trypanosoma cruzi
Citation: Dorn PL, Monroy MC and Stevens L (2022) Sustainable, integrated control of native vectors: The case of Chagas disease in Central America. Front. Trop. Dis 3:971000. doi: 10.3389/fitd.2022.971000
Received: 16 June 2022; Accepted: 19 July 2022;
Published: 21 September 2022.
Edited by:
Kaio Cesar Chaboli Alevi, Sao Paulo State Universty, BrazilReviewed by:
Rodrigo Gurgel-Gonçalves, University of Brasilia, BrazilHélcio Reinaldo Gil-Santana, Cruz Institute, Oswaldo Cruz Foundation (Fiocruz), Brazil
Jose Alejandro Martinez-Ibarra, Universidad de Guadalajara, Mexico
Copyright © 2022 Dorn, Monroy and Stevens. This is an open-access article distributed under the terms of the Creative Commons Attribution License (CC BY). The use, distribution or reproduction in other forums is permitted, provided the original author(s) and the copyright owner(s) are credited and that the original publication in this journal is cited, in accordance with accepted academic practice. No use, distribution or reproduction is permitted which does not comply with these terms.
*Correspondence: Patricia L. Dorn, dorn@loyno.edu
†These authors have contributed equally to this work
‡ORCID: Patricia L. Dorn, https://orcid.org/0000-0001-8555-7008
Lori Stevens, https://orcid.org/0000-0003-3847-5979