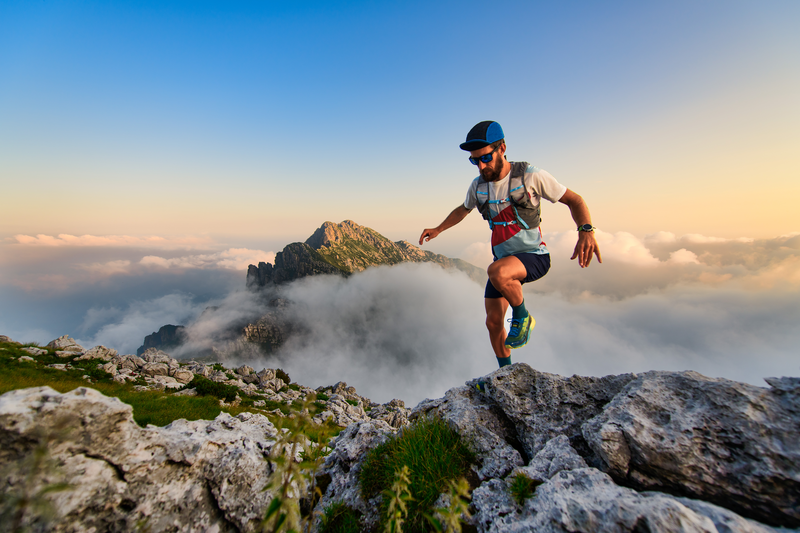
95% of researchers rate our articles as excellent or good
Learn more about the work of our research integrity team to safeguard the quality of each article we publish.
Find out more
REVIEW article
Front. Trop. Dis. , 31 August 2022
Sec. Vector Biology
Volume 3 - 2022 | https://doi.org/10.3389/fitd.2022.969299
This article is part of the Research Topic Mosquitoes and Their Role in Disease Transmission: Recent Advances and Innovations View all 9 articles
Presently, there is a need to develop effective and novel modes of control for mosquitoes, which remain a key driver of infectious disease transmission throughout the world. Control methods for these vectors have historically relied on a limited number of active ingredients (AIs) that have not experienced significant change in usage since the mid-20th century. The resulting development of widespread insecticide resistance has consequently increased the risk for future vector-borne disease outbreaks. Recently, metal nanoparticles have been explored for potential use in mosquito control due to their demonstrated toxicity against mosquitoes at all life stages. However, the majority of studies to date have focused on the larvicidal efficacy of metal nanoparticles with few studies examining their adulticidal potential. In this review, we analyze the current literature on green synthesized metal nanoparticles and their effect on adult mosquitoes.
Vector-borne disease remains a leading cause of death worldwide despite sustained eradication programs across Africa, Asia, and South America. Malaria and dengue have been identified as threats to global health by WHO accounting for a global 230 million and 96 million symptomatic cases in 2019 respectively (1). Unfortunately, vector-borne disease will continue to be of public health importance due to the confluence of changing climate, globalization and urbanization which together are likely to increase rates of transmission. Rising temperatures, in particular are predicted to expand the geographic range of many vector species, increase the transmission season length, and provide shorter incubation period for vector species and their associated pathogens (2). This rising incidence will largely be driven by mosquito-borne diseases (3) such as yellow fever, dengue, Zika, chikungunya, West Nile, eastern equine encephalitis and St. Louis encephalitis viruses for which most have no available vaccines. As such, mosquito control remains the most effective preventative strategy.
Current mosquito control strategies employ a combination of source reduction, larviciding and adulticiding. Of these three options, adult insecticides have historically provided control operations with an effective means to quickly target and suppress mosquito population outbreaks with ultra-low volume (ULV) spraying being the preferred delivery method (4). Unfortunately, sustained use and reliance on a small group of chemical classes of insecticides (i.e. pyrethroids, organophosphates and carbamates) has led to the development of widespread insecticide resistance (5–8). Continued use of these same active ingredients (AIs) overtime will lead to diminished effectiveness (9) and may lead to the resurgence of mosquito-borne diseases (10).
To mitigate further development of resistance and retain insecticide efficacy, new AIs and formulations are necessary. However, development can be prohibitively expensive, due in part to strict regulatory requirements encompassing toxicological and safety studies that promote more selective and less persistent insecticides (11). Registration of a new chemical AI costs an estimated 250 million USD and can take fifteen years of research and development to complete (12–14). This gap in effective control tools and insecticide resistance has led to increasing interest in alternative sources of insecticides.
Nanotechnology has grown as a potential tool in insecticide application. Metal nanoparticles, in particular, are increasingly being incorporated into scientific and medical applications for the purposes of catalysis, imaging, diagnostics, drug delivery and pest management (15–20). Due to their wide array of applications, nanoparticles are currently one of the most active areas of research in the material sciences, including their use as an insecticide. Though there currently is no regulatory definition within the US as to what constitutes a nanoparticle insecticide, the term generally refers to anything that is 100 nm in size or smaller. The key feature that distinguishes a nanoinsecticide however, is that they demonstrate beneficial properties apart from the original material due to their small size. A number of studies have already demonstrated the toxic effects these metal nanoparticles possess against medically important mosquito vector species. However, the majority of studies have been limited to larvicidal efficacy and there has not yet been an in depth look into the use of metal nanoparticles as a potential adulticide. In this review, we summarize the current literature on the effectiveness of metal nanoparticles against adult mosquito vectors of medical importance. We also explore challenges for future research as well as potential pitfalls in evaluation methodology between existing studies.
Synthesizing a metal nanoparticle requires three separate components: a source of metal ions, a reducing agent and a capping or stabilizing agent (21). The combination of these three factors can greatly impact the physical characteristics, notably morphology and size, of the resulting nanoparticle (22). The objective of applying nanoparticle technology has been to enhance unique beneficial properties that many metals already demonstrate. For example, silver has been utilized as an antimicrobial treatment in medicine since the mid-twentieth century and low dose larval exposure to copper has been linked to longer larval development, lowered rates of eclosion and decreased larval fitness in mosquitoes (21, 23–26).
Increasingly, plants are being used in the production of metal nanoparticles due to their natural reducing and stabilizing potential. Plant-mediated synthesis, often referred to as green synthesis, is advantageous due to its simplicity as a single step reaction that does not require the use of intensive heat or chemical processing. Plant extracts are comprised of different phytochemicals, metabolites and volatile bioactive compounds, many of which have demonstrated toxic effects against mosquitoes acting as larvicides, pupicides, adulticides and repellents across a number of studies (27, 28). These compounds can disrupt receptor sites of the nervous system through different metabolic pathways. For example, alkaloids and monoterpenes act on Na+/K+ ion exchange while flavonoids target acetylcholinesterase (29). Additionally, plant extracts also demonstrate synergism with existing insecticides. Synergism screening has demonstrated enhanced adulticidal toxicity of permethrin when combined with Cyperus rotundus, Alpinia galanga and Cinnamomum verum essential oils (30). In 2013, Tong and Bloomquist identified six permethrin synergist essential oils whose mode of action was likely metabolic inhibition of enzymes cytochrome P450 monooxygenases and carboxylesterases (31). Plant-mediated metal nanoparticles, in theory, seek to incorporate the beneficial properties relevant for their use in insecticide application of both metal ions and plant extracts to improve control efficacy.
Metal nanoparticles are increasingly being explored for their use in mosquito control as a potential insecticide. Application of these particles against mosquitoes have proven to be quite effective across a wide range of studies (32, 33). An extensive review of more than 100 studies emphasizes the promising toxicity many biosynthesized metal nanoparticles demonstrate against the larvae and pupae of different mosquito species (32). Amongst these, selenium nanoparticles exhibited LC50 of 99.6, 104.13 and 240.7 µg/mL when administered to larvae of Anopheles stephensi (List.), Aedes aegypti (Linn.), and Culex quinquefasciatus (Say.) respectively while gold nanoparticles demonstrated slightly stronger toxicity with LC50 of 25.92 and 28.64 µg/mL against An. stephensi and Ae. aegypti respectively (34, 35). Similarly, silver nanoparticles (AgNP) synthesized via plant extracts have demonstrated a wide range of larvicidal efficacy against multiple species with LC50 values between 0.3 to 130.3 µg/mL (32, 33, 36–40).
While there have been many studies investigating the larvicidal efficacy of nanoparticles, few have investigated their effectiveness against adult mosquitoes. Table 1 summarizes findings of such studies conducted between 2011 and 2020. The majority of testing has focused on the adulticidal properties of AgNPs synthesized primarily via plant-mediated pathways against common mosquito vector species. Out of twenty-two identified studies, only two evaluated alternative metals (gold and zinc) and all but three used plant extracts as the reducing agents with the exception of fungal, bacterial and chemical synthesis pathways (Table 1). This disparity in metal choice is likely due to the low cost and availability of silver nitrate as alternative metal nanoparticles demonstrate comparable efficacy against adult mosquitoes. The gold nanoparticles evaluated against the malaria vector An. stephensi were demonstrated to have an LC50 at 11.23 µg/mL whereas zinc nanoparticles were shown to reduce longevity in the same mosquito species when exposed to concentration between 2-10 ppm (54, 61). All combinations of metal and reducing agent demonstrated toxic effects against mosquitoes. However, differences in material or reducing agent do not appear to produce noticeable differences in toxicity despite their role in determining physiochemical properties of the nanoparticle.
Table 1 Adulticidal toxicity of metal nanoparticles, their synthesis pathway and testing methodology against common mosquito vectors.
Feronia elephantum was used to synthesize AgNP which were evaluated against Ae. aegypti, An. stephensi and Cx. quinquefasciatus using the WHO susceptibility bioassay demonstrating LC50 values of 20.40, 18.04 and 21.80 µg/mL at 24h respectively (45). Similarly, nanoparticles synthesized from three other plant species, Chomelia asiatica, Zeuxine gracilis and Heliotropium indicum, were tested using the same methodology producing a range of LC50 between 8.48-32.23 µg/mL (46–48). The LC50 of Chenopodium ambrosioides synthesized AgNP tested against Ae. albopictus was determined to be 14.29 µg/mL (49). A series of studies conducted by Azarudeen et al. also confirmed comparable results using Hedyotis puberula, Ventilago maderaspatana and Naregamia alata to synthesize AgNPs (50–52). Of the studies conducted using the WHO tube bioassay, the lowest to highest LC50 achieved ranged from 6.68 to 48.94 µg/mL from AgNPs synthesized using Phyllanthus niruri and to V. maderaspatana respectively.
Although the majority of adulticide efficacy was evaluated using the WHO tube bioassay, several studies opted for alternative test methodologies. One study tested silver nanoparticles following WHO spatial spray guidelines against Cx. quinquefasciatus utilizing a fungal mediated synthesis yielding LC50 at 22h of 0.4 µl/cm2 (41). Bacteria mediated synthesis using Listeria monocytogenes, Bacillus subtilius and Streptomyces anulatus produced lower LC50 values at 0.16, 0.08 and 0.06 µl/cm2 respectively (43). Two additional studies evaluating AgNPs as a spatial spray also demonstrated comparable toxicity with an LC50 of Ficus religiosa synthesized particles at 0.12 µl/cm2 and Azadirachta indica at 0.53 µl/cm2 (42, 44). A modified CDC bottle bioassay study determined sodium citrate synthesized AgNPs induced 100% mortality at 4h post-treatment when applied to the bottles at 90 ppm (59). During the same study, nanoparticles conjugated with deltamethrin did not improve the treatment efficacy over a deltamethrin only control. Two separate studies conducted against Ae. aegypti and An. stephensi also confirmed sub-lethal exposure to AgNPs reduced adult longevity in both male and female mosquitoes.
Preliminary studies on metal nanoparticle adulticides have shown promising results which warrant further research and discussion on how to potentially integrate this technology as a mosquito control tool. This is necessary because adulticides are the primary tool used to curb active transmission of mosquito-borne disease, yet there are so few available insecticidal AIs when compared to larviciding. Increasing levels of insecticide resistance across mosquito populations globally have also diminished the potency of current insecticides and new AIs are needed to maintain effectiveness of mosquito control operations. However, our review of the current literature on metal nanoparticle adulticides exposes several key issues that should be addressed in future testing. These include inconsistent testing methodology, an unknown mode of action, absence of field efficacy trials and unexplored interactions with existing insecticides.
First, there is no consistency in methodology for evaluating the toxicity of nanoparticles. According to WHO guidelines, the effectiveness of a novel insecticide must be assessed across three phases of testing: (1) inherent toxicity evaluations, (2) small-scale studies and outdoor semi-field applications and (3) operational trials against field populations with before and after measurements of mosquito population abundance (63). Due to their novelty in mosquito control research, metal nanoparticles have so far been limited to the first phase of testing.
For laboratory trials, the standard measure of intrinsic toxicity is through the direct topical application of a given insecticide to the pronotum across a range of concentrations to determine the lethal dose. This is typically supplemented with additional testing to determine its insecticidal activity as a spatial spray. Here, an insecticide is atomized to produce droplets of a known size into a wind tunnel and again, administered to test mosquitoes across a range of concentrations to calculate lethal concentration.
In the current literature however, a majority of researchers have opted to use the WHO tube bioassay and the CDC bottle bioassay as alternative methodologies. Briefly, the WHO tube bioassay employs a tarsal contact test scheme. In this evaluation, filter paper is impregnated with a known concentration of an insecticide and placed into a tube. Mosquitoes are then introduced into the tube where they are exposed to the impregnated filter paper for one hour, after which they are transferred into a separate clean holding tube where they can be observed for mortality. Similarly, the CDC bottle bioassay also employs the same tarsal contact scheme using glass bottles whose interior surface are treated with a known concentration of insecticide dissolved into a solvent such as acetone. After the bottles have dried, mosquitoes can be introduced and assessed for mortality.
More than half of the twenty-two studies reviewed in this article were conducted using the WHO tube bioassay. Of the remaining studies, four tested the metal nanoparticles as a spatial spray, one using the CDC bottle bioassay and one using fabrics impregnated with silver nanoparticles. In the latter study, mosquitoes were exposed to the impregnated fabrics using the same contact and holding methodology as the WHO tube bioassay. This diverse assortment of studies is problematic because the results cannot be directly compared. Furthermore, the WHO tube bioassay and CDC bottle bioassay are not representative of how mosquitoes would interact with the nanoparticles in real world applications. Adulticide treatments are primarily delivered as a spatial spray where individual droplets must come into physical contact with a mosquito. Prolonged tarsal contact schemes are more suited for testing residual insecticides but are not an appropriate proxy for adulticide sprays. Data generated in these studies would therefore not comply with registration requirements for novel insecticides. For these reasons, lethal dose and lethal concentration as determined by direct topical applications and spatial spray testing following WHO guidelines would provide optimal metrics by which to judge the efficacy of any new insecticide. As further research into metal nanoparticle insecticides progress, testing needs to be standardized to ensure any obtained data can be compared between studies.
Second, although metallic nanoparticles have demonstrated strong efficacy to control mosquitoes at all life stages, their mode of action has yet to be definitively determined. One hypothesis is that metal nanoparticles act on cellular membranes and detoxification enzymes which leads to loss of cellular function and eventual death (32, 64). However, the mechanism by which nanoparticles induce mortality has yet to be fully explored and further research is needed. Third, testing should be expanded to include field collected populations and insecticide resistant colonies to better validate the potential operational efficacy of metal nanoparticle adulticides amidst the rising prevalence of insecticide resistance. Fourth, the scope of research should be broadened not only to stand-alone effects but also how the metal nanoparticles interact with existing insecticides. This could conceivably include their role as an additive insecticidal agents but also as nanocarrier delivery systems for conventional insecticides (65). In one such study, deltamethrin was successfully conjugated with silver nanoparticles (59). Although this did not improve treatment efficacy above the deltamethrin control, investigating potential synergism presents a promising avenue of research.
In summary, preliminary studies on metal nanoparticle adulticides have shown promising results but are hindered by inconsistent testing methodology, preventing meaningful comparisons between studies. Additionally, the mode of action, field study results and synergism with existing insecticides remains largely unexplored. Future research efforts should seek to address these areas. Plants possess bioactive compounds including alkaloids, ketones, tannins and essential oils which have demonstrated insecticidal effects, but many of these same compounds can also act as synergists with existing insecticides (66, 67). By synthesizing metal nanoparticles in conjunction with these bioactive compounds, researchers aim to confer beneficial properties to enhance insecticide formulations, thus providing potential new tool in managing resistance in mosquito populations.
KB was responsible for conceptualizing the topic being review and for drafting the manuscript. All authors contributed to revision of the manuscript and have approved the submitted version.
Publication fees were provided by grant funding through Florida Department of Agriculture and Consumer Services, AWD11192: Evaluation of a nanoparticle encapsulated permethrin formulation against three species of adult mosquitoes: Aedes aegypti, Culex quinquefasciatus and Anopheles quadrimaculatus.
The authors declare that the research was conducted in the absence of any commercial or financial relationships that could be construed as a potential conflict of interest.
All claims expressed in this article are solely those of the authors and do not necessarily represent those of their affiliated organizations, or those of the publisher, the editors and the reviewers. Any product that may be evaluated in this article, or claim that may be made by its manufacturer, is not guaranteed or endorsed by the publisher.
1. World Health O. World Malaria Report. 20 years of global progress and challenges. Geneva: World Health Organization (2020).
2. Colón-González FJ, Sewe M, Tompkins A, Sjödin H, Casallas García A, Rocklöv J, et al. Projecting the risk of mosquito-borne diseases in a warmer and more populated world - a multi-model, multi-scenario intercomparison modelling study. Lancet Planet Health (2021) 5:e404–e14. doi: 10.1016/S2542-5196(21)00132-7
3. Rosenberg R, Lindsey NP, Fischer M, Gregory CJ, Hinckley AF, Mead PS, et al. Vital signs: trends in reported vectorborne disease cases - United States and territories, 2004-2016. MMWR Morb Mortal Wkly Rep (2018) 67(17):496–501. doi: 10.15585/mmwr.mm6717e1
4. Tabachnick WJ. Research contributing to improvements in controlling Florida’s mosquitoes and mosquito-borne diseases. Insects (2016) 7(4):50. doi: 10.3390/insects7040050
5. Marcombe S, Farajollahi A, Healy SP, Clark GG, Fonseca DM. Insecticide resistance status of United States populations of Aedes albopictus and mechanisms involved. PloS One (2014) 9(7):e101992. doi: 10.1371/journal.pone.0101992
6. McInnis SJ, Goddard J, Deerman JH, Nations T, Varnado WC. Insecticide resistance testing of Culex quinquefasciatus and Aedes albopictus from Mississippi. J Am Mosq Control Assoc (2019) 35(2):147–50. doi: 10.2987/18-6795.1
7. Mundis SJ, Estep AS, Waits CM, Ryan SJ. Spatial variation in the frequency of knockdown resistance genotypes in Florida Aedes aegypti populations. Parasites Vectors (2020) 13(1):241. doi: 10.1186/s13071-020-04112-3
8. Shin D, Smartt CT. Assessment of esterase gene expression as a risk marker for insecticide resistance in Florida Culex nigripalpus (Diptera: Culicidae). J Vector Ecol (2016) 41(1):63–71. doi: 10.1111/jvec.12195
9. Baleta A. Insecticide resistance threatens malaria control in Africa. Lancet (2009) 374(9701):1581–2. doi: 10.1016/S0140-6736(09)61933-4
10. Cohen JM, Smith DL, Cotter C, Ward A, Yamey G, Sabot OJ, et al. Malaria resurgence: a systematic review and assessment of its causes. Malar J (2012) 11:122. doi: 10.1186/1475-2875-11-122
11. Sparks TC. Insecticide discovery: an evaluation and analysis. Pestic Biochem Physiol (2013) 107(1):8–17. doi: 10.1016/j.pestbp.2013.05.012
12. Holm RE, Baron JJ. Evolution of the crop protection industry. Pesticides Agric Environ (2002), 295–326.
13. Hunter P. Challenges and options for disease vector control: the outbreak of Zika virus in South America and increasing insecticide resistance among mosquitoes have rekindled efforts for controlling disease vectors. EMBO Rep (2016) 17(10):1370–3. doi: 10.15252/embr.201643233
14. National Research Council. The future role of pesticides in US agriculture. Washington DC, United States: National Academies Press (2000).
15. Ali S, Shafique O, Mahmood T, Hanif MA, Ahmed I, Khan BA. A review about perspectives of nanotechnology in agriculture. Pakistan J Agric Res (2018) 30(2):116–21. doi: 10.17582/journal.pjar/2018/31.2.116.121
16. Lang C, Mission EG, Ahmad Fuaad AA-H, Shaalan M. Nanoparticle tools to improve and advance precision practices in the agrifoods sector towards sustainability - a review. J Cleaner Prod (2021) 293:126063. doi: 10.1016/j.jclepro.2021.126063
17. Mittal D, Kaur G, Singh P, Yadav K, Ali S. Nanoparticle-based sustainable agriculture and food science: recent advances and future outlook. Front Nanotechnol (2020) 2:579954. doi: 10.3389/fnano
18. Muhammad F, Nguyen TDT, Raza A, Akhtar B, Aryal S. A review on nanoparticle-based technologies for biodetoxification. Drug Chem Toxicol (2017) 40(4):489–97. doi: 10.1080/01480545.2016.1277736
19. Rajendran NK, Kumar SSD, Houreld NN, Abrahamse H. A review on nanoparticle based treatment for wound healing. J Drug Delivery Sci Technol (2018) 44:421–30. doi: 10.1016/j.jddst.2018.01.009
20. Raura N, Garg A, Arora A, Roma M. Nanoparticle technology and its implications in endodontics: A review. Biomater Res (2020) 24(1):21. doi: 10.1186/s40824-020-00198-z
21. Reidy B, Haase A, Luch A, Dawson KA, Lynch I. Mechanisms of silver nanoparticle release, transformation and toxicity: A critical review of current knowledge and recommendations for future studies and applications. Mater (Basel) (2013) 6(6):2295–350. doi: 10.3390/ma6062295
22. Tolaymat TM, El Badawy AM, Genaidy A, Scheckel KG, Luxton TP, Suidan M. An evidence-based environmental perspective of manufactured silver nanoparticle in syntheses and applications: A systematic review and critical appraisal of peer-reviewed scientific papers. Sci Total Environ (2010) 408(5):999–1006. doi: 10.1016/j.scitotenv.2009.11.003
23. Perez MH, Noriega FG. Aedes aegypti pharate 1st instar quiescence affects larval fitness and metal tolerance. J Insect Physiol (2012) 58(6):824–9. doi: 10.1016/j.jinsphys.2012.03.005
24. Perez MH, Noriega FG. Sub-lethal metal stress response of larvae of. Aedes Aegypti Physiol Entomol (2014) 39(2):111–9. doi: 10.1111/phen.12054
25. Rayms-Keller A, Olson KE, McGaw M, Oray C, Carlson JO, Beaty BJ. Effect of heavy metals on Aedes aegypti (Diptera: Culicidae) larvae. Ecotoxicol Environ Saf (1998) 39(1):41–7. doi: 10.1006/eesa.1997.1605
26. Reza M, Ilmiawati C, Matsuoka H. Application of copper-based ovitraps in local houses in West Sumatra, Indonesia: a field test of a simple and affordable larvicide for mosquito control. Trop Med Health (2016) 44:11. doi: 10.1186/s41182-016-0007-8
27. Piplani M, Bhagwat DP, Singhvi G, Sankaranarayanan M, Balana-Fouce R, Vats T, et al. Plant-based larvicidal agents: an overview from 2000 to 2018. Exp Parasitol (2019) 199:92–103. doi: 10.1016/j.exppara.2019.02.014
28. Shehata AZ, El-Sheikh TM, Shaapan RM, Abdel-Shafy S, Alanazi AD. Ovicidal and latent effects of Pulicaria jaubertii (asteraceae) leaf extracts on Aedes aegypti. J Am Mosq Control Assoc (2020) 36(3):161–6. doi: 10.2987/20-6952.1
29. Senthil-Nathan S. A review of resistance mechanisms of synthetic insecticides and botanicals, phytochemicals, and essential oils as alternative larvicidal agents against mosquitoes. Front Physiol (2020) 10:1591. doi: 10.3389/fphys.2019.01591
30. Chansang A, Champakaew D, Junkum A, Jitpakdi A, Amornlerdpison D, Aldred AK, et al. Synergy in the adulticidal efficacy of essential oils for the improvement of permethrin toxicity against Aedes aegypti L. (Diptera: Culicidae) Parasit Vectors (2018) 11(1):417. doi: 10.1186/s13071-018-3001-7
31. Tong F, Bloomquist JR. Plant essential oils affect the toxicities of carbaryl and permethrin against Aedes aegypti (Diptera: Culicidae). J Med Entomol (2013) 50(4):826–32. doi: 10.1603/me13002
32. Benelli G. Plant-mediated biosynthesis of nanoparticles as an emerging tool against mosquitoes of medical and veterinary importance: a review. Parasitol Res (2016) 115(1):23–34. doi: 10.1007/s00436-015-4800-9
33. Benelli G, Caselli A, Canale A. Nanoparticles for mosquito control: challenges and constraints. J King Saud Univ - Sci (2017) 29(4):424–35. doi: 10.1016/j.jksus.2016.08.006
34. Murugan K, Benelli G, Panneerselvam C, Subramaniam J, Jeyalalitha T, Dinesh D, et al. Cymbopogon citratus-synthesized gold nanoparticles boost the predation efficiency of copepod Mesocyclops aspericornis against malaria and dengue mosquitoes. Exp Parasitol (2015) 153:129–38. doi: 10.1016/j.exppara.2015.03.017
35. Sowndarya P, Ramkumar G, Shivakumar MS. Green synthesis of selenium nanoparticles conjugated Clausena dentata plant leaf extract and their insecticidal potential against mosquito vectors. Artif Cells Nanomed Biotechnol (2017) 45(8):1490–5. doi: 10.1080/21691401.2016.1252383
36. Barik TK, Kamaraju R, Gowswami A. Silica nanoparticle: A potential new insecticide for mosquito vector control. Parasitol Res (2012) 111(3):1075–83. doi: 10.1007/s00436-012-2934-6
37. Doshi M, Bosak A, Neal CJ, Isis N, Kumar U, Jeyaranjan A, et al. Exposure to nanoceria impacts larval survival, life history traits and fecundity of Aedes aegypti. PloS Negl Trop Dis (2020) 14(9):e0008654. doi: 10.1371/journal.pntd.0008654
38. Elumalai D, Kaleena PK, Ashok K, Suresh A, Hemavathi M. Green synthesis of silver nanoparticle using Achyranthes aspera and its larvicidal activity against three major mosquito vectors. Eng Agricult Environ Food (2016) 9(1):1–8. doi: 10.1016/j.eaef.2015.08.002
39. Murugan K, Panneerselvam C, Samidoss CM, Madhiyazhagan P, Suresh U, Roni M, et al. In vivo and in vitro effectiveness of azadirachta indica-synthesized silver nanocrystals against Plasmodium berghei and Plasmodium falciparum, and their potential against malaria mosquitoes. Res Vet Sci (2016) 106:14–22. doi: 10.1016/j.rvsc.2016.03.001
40. Priya S, Murugan K, Priya AS, Dinesh D, Panneerselvam C, Devi GD, et al. Green synthesis of silver nanoparticles using Calotropis gigantea and their potential mosquito larvicidal property. Int J Pure Appl Zool (2014) 2:0.
41. Soni N, Prakash S. Fungal-mediated nano silver: An effective adulticide against mosquito. Parasitol Res (2012) 111(5):2091–8. doi: 10.1007/s00436-012-3056-x
42. Soni N, Prakash S. Silver nanoparticles: a possibility for malarial and filarial vector control technology. Parasitol Res (2014) 113(11):4015–22. doi: 10.1007/s00436-014-4069-4
43. Soni N, Prakash S. Antimicrobial and mosquitocidal activity of microbial synthesized silver nanoparticles. Parasitol Res (2015) 114(3):1023–30. doi: 10.1007/s00436-014-4268-z
44. Soni N, Prakash S eds. Different geometrical agnps for vector control and their added value of antibacterial activity J Parasitol Res Photon (2015) 105:232–43.
45. Veerakumar K, Govindarajan M. Adulticidal properties of synthesized silver nanoparticles using leaf extracts of Feronia elephantum (Rutaceae) against filariasis, malaria, and dengue vector mosquitoes. Parasitol Res (2014) 113(11):4085–96. doi: 10.1007/s00436-014-4077-4
46. Veerakumar K, Govindarajan M, Hoti SL. Evaluation of plant-mediated synthesized silver nanoparticles against vector mosquitoes. Parasitol Res (2014) 113(12):4567–77. doi: 10.1007/s00436-014-4147-7
47. Muthukumaran U, Govindarajan M, Rajeswary M, Veerakumar K, Amsath A, Muthukumaravel K. Adulticidal activity of synthesized silver nanoparticles using Chomelia asiatica Linn.(Family: Rubiaceae) against Anopheles stephensi, Aedes aegypti and Culex quinquefasciatus (Diptera: Culicidae). Int J Zool Appl Biosci (2016) 1:118–29. doi: 10.1007/s00436-014-4265-2
48. Kovendan K, Chandramohan B, Govindarajan M, Jebanesan A, Kamalakannan S, Vincent S, et al. Orchids as sources of novel nanoinsecticides? efficacy of Bacillus sphaericus and Zeuxine gracilis-fabricated silver nanoparticles against dengue, malaria and filariasis mosquito vectors. J Cluster Sci (2018) 29(2):345–57. doi: 10.1007/s10876-018-1331-4
49. Subramaniam J, Murugan K, Jebanesan A, Pontheckan P, Dinesh D, Nicoletti M, et al. Do Chenopodium ambrosioides-synthesized silver nanoparticles impact Oryzias melastigma predation against Aedes albopictus larvae? J Cluster Sci (2017) 28(1):413–36. doi: 10.1007/s10876-016-1113-9
50. Azarudeen R, Govindarajan M, Amsath A, Km S, Alharbi N, Vijayan P, et al. Size-controlled fabrication of silver nanoparticles using the: Hedyotis puberula leaf extract: toxicity on mosquito vectors and impact on biological control agents. RSC Adv (2016) 6:96573–83. doi: 10.1039/c6ra23208f
51. Azarudeen RMST, Govindarajan M, AlShebly MM, AlQahtani FS, Amsath A, Benelli G. One pot green synthesis of colloidal silver nanocrystals using the Ventilago maderaspatana leaf extract: acute toxicity on malaria, Zika virus and filariasis mosquito vectors. J Cluster Sci (2017) 28(1):369–92. doi: 10.1007/s10876-016-1103-y
52. Azarudeen RMST, Govindarajan M, Amsath A, Muthukumaran U, Benelli G. Single-step biofabrication of silver nanocrystals using Naregamia alata: a cost effective and eco-friendly control tool in the fight against malaria, Zika virus and St. Louis encephalitis mosquito vectors. J Cluster Sci (2017) 28(1):179–203. doi: 10.1007/s10876-016-1067-y
53. AlQahtani FS, AlShebly MM, Govindarajan M, Senthilmurugan S, Vijayan P, Benelli G. Green and facile biosynthesis of silver nanocomposites using the aqueous extract of Rubus ellipticus leaves: toxicity and oviposition deterrent activity against Zika virus, malaria and filariasis mosquito vectors. J Asia-Pac Entomol (2017) 20(1):157–64. doi: 10.1016/j.aspen.2016.12.004
54. Subramaniam J, Murugan K, Panneerselvam C, Kovendan K, Madhiyazhagan P, Dinesh D, et al. Multipurpose effectiveness of Couroupita guianensis-synthesized gold nanoparticles: high antiplasmodial potential, field efficacy against malaria vectors and synergy with Aplocheilus lineatus predators. Environ Sci pollut Res Int (2016) 23(8):7543–58. doi: 10.1007/s11356-015-6007-0
55. Subramaniam J, Murugan K, Panneerselvam C, Kovendan K, Madhiyazhagan P, Kumar PM, et al. Eco-friendly control of malaria and arbovirus vectors using the mosquitofish Gambusia affinis and ultra-low dosages of Mimusops elengi-synthesized silver nanoparticles: towards an integrative approach? Environ Sci pollut Res Int (2015) 22(24):20067–83. doi: 10.1007/s11356-015-5253-5
56. Suresh U, Murugan K, Benelli G, Nicoletti M, Barnard DR, Panneerselvam C, et al. Tackling the growing threat of dengue: Phyllanthus niruri-mediated synthesis of silver nanoparticles and their mosquitocidal properties against the dengue vector Aedes aegypti (Diptera: Culicidae). Parasitol Res (2015) 114(4):1551–62. doi: 10.1007/s00436-015-4339-9
57. Pavithra Bharathi V, Ragavendran C, Murugan N, Natarajan D. Ipomoea batatas (Convolvulaceae)-mediated synthesis of silver nanoparticles for controlling mosquito vectors of Aedes albopictus, Anopheles stephensi, and Culex quinquefasciatus (Diptera : Culicidae). Artif Cells Nanomed Biotechnol (2017) 45(8):1568–80. doi: 10.1080/21691401.2016.1261873
58. Benelli G, Kadaikunnan S, Alharbi NS, Govindarajan M. Biophysical characterization of Acacia caesia-fabricated silver nanoparticles: effectiveness on mosquito vectors of public health relevance and impact on non-target aquatic biocontrol agents. Environ Sci pollut Res Int (2018) 25(11):10228–42. doi: 10.1007/s11356-017-8482-y
59. Sooresh A, Kwon H, Taylor R, Pietrantonio P, Pine M, Sayes CM. Surface functionalization of silver nanoparticles: novel applications for insect vector control. ACS Appl Mater Interfaces (2011) 3(10):3779–87. doi: 10.1021/am201167v
60. Roni M, Murugan K, Panneerselvam C, Subramaniam J, Nicoletti M, Madhiyazhagan P, et al. Characterization and biotoxicity of Hypnea musciformis-synthesized silver nanoparticles as potential eco-friendly control tool against Aedes aegypti and Plutella xylostella. Ecotoxicol Environ Saf (2015) 121:31–8. doi: 10.1016/j.ecoenv.2015.07.005
61. Murugan K, Roni M, Panneerselvam C, Aziz AT, Suresh U, Rajaganesh R, et al. Sargassum wightii-synthesized ZNO nanoparticles reduce the fitness and reproduction of the malaria vector Anopheles stephensi and cotton bollworm Helicoverpa armigera. Physiol Mol Plant Pathol (2018) 101:202–13. doi: 10.1016/j.pmpp.2017.02.004
62. El-Sayed AA, Amr A, Kamel OMHM, El-Saidi MMT, Abdelhamid AE. Eco-friendly fabric modification based on AgNPs at Moringa for mosquito repellent applications. Cellulose (2020) 27(14):8429–42. doi: 10.1007/s10570-020-03355-8
63. World Health O. Guidelines for laboratory and field testing of mosquito larvicides. Geneva: World Health Organization (2005).
64. Foldbjerg R, Jiang X, Miclaus T, Chen C, Autrup H, Beer C. Silver nanoparticles - wolves in sheep’s clothing? Toxicol Res (2015) 4:563–75. doi: 10.1039/C4TX00110A
65. Athanassiou CG, Kavallieratos NG, Benelli G, Losic D, Usha Rani P, Desneux N. Nanoparticles for pest control: current status and future perspectives. J Pest Sci (2018) 91(1):1–15. doi: 10.1007/s10340-017-0898-0
66. Norris EJ, Bloomquist JR. Co-Toxicity factor analysis reveals numerous plant essential oils are synergists of natural pyrethrins against aedes aegypti mosquitoes. Insects (2021) 12(2):154. doi: 10.3390/insects12020154
Keywords: silver nanoparticle, metal nanoparticle, adulticide, AgNP, green synthesis, nanoinsecticide, nanotechnology
Citation: Blore K, Baldwin R, Batich CD, Koehler P, Pereira R, Jack CJ, Qualls WA and Xue RD (2022) Efficacy of metal nanoparticles as a control tool against adult mosquito vectors: A review. Front. Trop. Dis 3:969299. doi: 10.3389/fitd.2022.969299
Received: 14 June 2022; Accepted: 08 August 2022;
Published: 31 August 2022.
Edited by:
Indra Vythilingam, University of Malaya, MalaysiaReviewed by:
Luc Salako Djogbénou, Université d’Abomey-Calavi, BeninCopyright © 2022 Blore, Baldwin, Batich, Koehler, Pereira, Jack, Qualls and Xue. This is an open-access article distributed under the terms of the Creative Commons Attribution License (CC BY). The use, distribution or reproduction in other forums is permitted, provided the original author(s) and the copyright owner(s) are credited and that the original publication in this journal is cited, in accordance with accepted academic practice. No use, distribution or reproduction is permitted which does not comply with these terms.
*Correspondence: Kai Blore, a2Jsb3JlQGFtY2RmbC5vcmc=
Disclaimer: All claims expressed in this article are solely those of the authors and do not necessarily represent those of their affiliated organizations, or those of the publisher, the editors and the reviewers. Any product that may be evaluated in this article or claim that may be made by its manufacturer is not guaranteed or endorsed by the publisher.
Research integrity at Frontiers
Learn more about the work of our research integrity team to safeguard the quality of each article we publish.