- 1Department of Biological Sciences, Quinnipiac University, Hamden, CT, United States
- 2The Wellcome Trust Research Laboratory, Division of Gastrointestinal Sciences, Christian Medical College, Vellore, India
- 3Departments of Global Health, Medicine (Infectious Diseases), Pediatrics and Epidemiology, University of Washington, Seattle, WA, United States
Mass drug administration programs for the control of soil-transmitted helminths (STH) in humans most commonly utilize a single class of drugs; the benzimidazoles. Most such programs focus on the treatment of pre-school and school aged children attending schools, although there is increasing interest in the potential utility of community-wide MDA to reduce infection intensity within communities and possibly to interrupt STH transmission. In animals, mass treatment with benzimidazoles leads to the rapid selection of parasites containing resistance-encoding single nucleotide polymorphisms (SNP) and the potential emergence of resistance in parasite species that infect humans is of major potential public health concern. As programs scale up delivery of anthelmintics and consider expanding treated populations, monitoring of drug efficacy and the potential emergence of anthelmintic resistance with sensitive diagnostic tools is critical to ensure the continued success of STH control programs. In particular, as programs consider the adoption of community-wide deworming, there is concern that such a strategy may increase the risk of drug resistance by limiting the number of untreated individuals which serve as a refugia of unexposed worm populations. We review the literature for evidence of drug resistance in human STH infections and explore risks and mitigation strategies for emergence of drug resistance in the context of community-wide deworming.
Introduction
Soil transmitted helminths (STH) are among the most widespread neglected tropical diseases (NTD), affecting ~1.5 billion people globally (1, 2). STH disproportionately impact the poorest and most vulnerable communities. STH include hookworms (Necator americanus and Ancylostoma duodenale), Ascaris lumbricoides and Trichuris trichiura. Infection occurs through ingestion of eggs of A. lumbricoides and T. trichiura or larval penetration of the skin by hookworm larvae present in contaminated soil (3, 4). Moderate‐ and heavy‐intensity (MHI) hookworm infections are associated with lower hemoglobin levels and anemia, which may be particularly detrimental to pregnant women and young children who often have low baseline iron stores (5–8). Children with STH infections also experience malnutrition and poor cognitive development, further impacting school attendance and performance and future economic productivity (9–11). The current World Health Organization (WHO) strategy is focused on eliminating morbidity through the targeted deworming of at-risk populations with anthelminthic medications, including pre-school-age children (PSAC), school-age children (SAC), and women of reproductive age (WRA).
Large-scale deworming programs have led to a significant decline in the prevalence, intensity, and associated morbidity of STH (12). However, until substantial economic development occurs, including significant improvements in access to clean water, sanitation and hygiene (WASH), reservoirs among untreated adults will continue to lead to contamination and persistence in the environment (ranging from weeks for hookworm to years for Ascaris) (13). This leads to rapid re-infection in at-risk populations unless deworming is repeated frequently over time. Evidence from mathematical models and field trials indicate that it may be possible to interrupt the transmission of STH in some geographic settings by adopting a community-wide mass drug administration (MDA) strategy in which all individuals, including adults, are treated (14–16). A transmission-interruption strategy could allow for the discontinuation of long-term deworming programs and substantially reduce the need for drug donation upon which deworming programs are highly dependent (17).
The two most commonly used drugs in existing STH programs (and available as part of drug donation programs) are albendazole and mebendazole. Both of these drugs belong to a single class of benzimidazoles that act by inhibiting tubulin polymerization, leading to worm paralysis and death (18). A 2017 meta-analysis demonstrated that while both drugs were highly efficacious against A. lumbricoides, albendazole had higher efficacy against hookworm infections, with a cure rate (CR) of 80% and an egg reduction rate (ERR) of 90%. In contrast, mebendazole appeared most effective against T. trichiura, resulting in a CR of 42.1% and an ERR of 66% (19). The continued use of drugs with less than optimal efficacy suggests that the likelihood of resistance emerging in human populations may be high and, while global estimates continue to show that morbidity due to STH is declining, some studies in areas with a long history of deworming suggest a decline in efficacy of these drugs (20, 21). In fact, a 2018 review of this subject suggested that the efficacy of both albendazole and mebendazole for the treatment of hookworm infection may have decreased by as much as 15% during a two-decade period from 1995 until 2015 (22). This same review suggests that reduced efficacy is even more pronounced for the treatment of T. trichiura, with efficacy reductions at or near 30%. Whether these potential declines in efficacy are a result of the emergence of drug resistance is not clear (23) but such trends provide circumstantial evidence in support of this possibility.
Anthelminthic drug resistance
In the veterinary world, routine mass treatment of livestock has led to widespread drug resistance, with well documented single nucleotide polymorphisms (SNPs) that have resulted in the entire class of benzimidazole drugs being rendered ineffective. Isothermal loop-mediated isothermal amplification (LAMP) assays, PCR, Sanger sequencing, pyrosequencing and next generation sequencing (NGS) based methods have identified SNPs associated with benzimidazole resistance in a variety of human and veterinary nematodes at one of three codons – 167, 198 and 200 – in the gene encoding the β-tubulin isotype 1 (24, 25) (more appropriately named the β-tubulin A locus to prevent false implications of orthology) (26). (Human examples detailed in Table 1). These studies used nucleic acid derived from a variety of sources, including adult worms (from expulsion studies), dissected or concentrated eggs, or stool samples (Table 2). Some studies have recorded treatment history and collected samples both before and after treatment in order to document a temporal association between treatment and the emergence of putative genotypic determinants of resistance. In addition to presence or absence, pyrosequencing and NGS also allow for estimates on the proportion of the worm population in a sample with SNPs and would be more useful in longitudinal follow up studies with samples collected before and after administration of treatment.
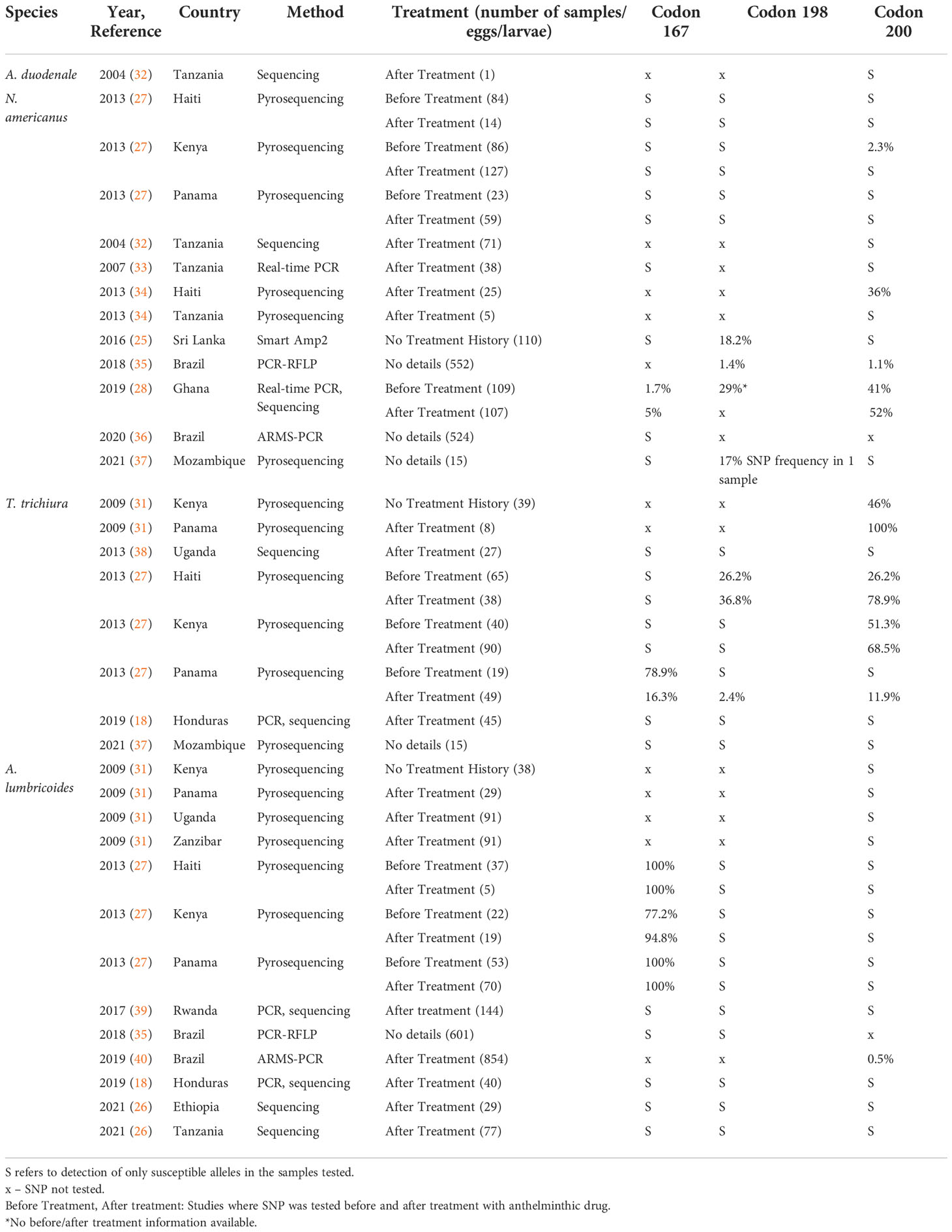
Table 2 Review of rates of β-tubulin A locus single nucleotide polymorphisms reported in human STH samples.
While monitoring for these known SNPs of importance in the veterinary world is a logical starting place for resistance monitoring efforts, a focus on the presence/absence of veterinary markers may not be sufficient to determine whether resistance is emerging in human parasite populations. Resistance development within human-infecting parasite populations may develop through the convergent evolution of similar SNPs but could just as easily occur as the result of genotypic changes in other positions or on other β-tubulin genes. As such, broad surveillance efforts employing less targeted approaches are required and monitoring should not be limited to those SNPs having known associations with veterinary resistance. Rather, effective surveillance will require the use of tools such as RNA sequencing or amplicon sequencing to broadly surveil transcriptional products for the presence of both identical and novel SNPs and SNP locations.
Definitive evidence of clinically relevant benzimidazole resistance has not been clearly documented in humans. However, in some regions, where continued human transmission occurs despite repeated rounds of targeted deworming, benzimidazole resistance may have emerged among different human STH species (27, 31, 41). In such settings, detection of resistance-encoding SNPs may be indicative of resistance in circulating worm populations and if resistance development mirrors patterns seen in the veterinary community, these SNPs might rapidly and irreversibly become dominant due to drug selection pressure (42). This is of concern, as prolonged MDA campaigns at suboptimal coverage could impact helminth populations and result in expansion of anthelmintic resistant worm populations (41). While it is important to remember that SNPs at the aforementioned loci have not been definitively shown to be relevant for resistance development in human-infecting species, clinically relevant resistance, brought about by intervention-mediated selective pressures, may arise by multiple other mechanisms (43). Additional research using sequencing-based approaches at the species level with a wide geographic representation is urgently needed to identify resistance determinants in the human population (should they exist) or to monitor for their development and emergence (43–45).
While generally seen with only limited or modest prevalence in human-infecting STH species, the presence of SNPs associated with benzimidazole resistance in the veterinary world remains a cause of concern among the STH community. For human hookworm infection, most studies documenting the presence of veterinary resistance markers pertain to N. americanus (Table 2). SNPs at codon 167 have been identified in Ghana (28), at codon 198 in Ghana (28), Brazil (35) and Sri Lanka (25) and at codon 200 in Ghana (28), Kenya (27), Haiti (34) and Brazil (35). Looking at samples collected before and after treatment, the study from Ghana showed a very modest (1.7% to 5%), but significant increase in detection among samples collected after treatment with albendazole (28). For Trichuris infection, where the efficacy of benzimidazoles is intrinsically lower, a study from Panama showed a decrease in resistant SNPs after treatment at codon 167 (27). However, for codon 198 and 200, studies in Haiti, Kenya and Panama showed an increase in SNP proportion with both heterozygous and homozygous populations seen (27, 31). In human Ascaris infections, most resistant SNPs reported have been in codon 167, and these have been reported in Haiti, Kenya and Panama (27). While studies documenting the appearance of such SNPs are important and cautionary, at present, their relevance and relationship to resistance development remains unclear.
Mitigation strategies and considerations
If community-wide deworming is adopted to interrupt transmission of STH, drug pressure within the targeted population would be dramatically increased and the proportion of the population left untreated (the refugia) would be minimized. This would decrease the pool of wild-type parasites in the community (42). As such, strategies to mitigate the potential emergence of drug resistance will be needed (44, 46). In the veterinary community, it has historically been suggested that maintaining an untreated sub-population of as little as 20% of a flock (who would harbor drug susceptible helminth populations) can significantly delay emergence and spread of resistance. Delayed resistance, resulting from these relatively low levels of untreated individuals, has been suggested as a possible reason for the lack of confirmed resistance in the human population, where non-adherence alone oftentimes ensures the existence of such a sub-population of untreated individuals (42). However, while the maintenance of an untreated sub-population may delay resistance, it is also counterproductive to efforts aimed at transmission interruption. Balancing such concerns will be critical for the success of any community-wide intervention program.
In addition to maintaining adequate refugia, there are many other lessons to be learned from the veterinary community. The need for sensitive diagnostic tools able to screen for resistance markers at the point-of-collection will be critical to managing and mitigating risk of widespread resistance development, particularly under an expanded treatment landscape. However, while technologies such as LAMP and recombinase polymerase amplification continue to increase the feasibility of field-friendly testing, adequate tools are difficult to envision until a clear definition of resistance markers in the human-infecting parasite community has been defined. Exploring the use of novel deworming agents, repurposing older drugs, the use of combination drug therapies, and a focus on the avoidance of underdosing will also play important roles in future efforts to avoid the development of widespread resistance within the human-parasite community. Innovative strategies to reduce environmental contamination will also be critical (23, 47) as will integration of community-directed approaches and engagement to affect behavioral change to improve equitable coverage and adherence with MDA and WASH uptake.
Adequate consideration of animal reservoirs of infection and parasite hybridization events are also likely to prove critical if resistance development is to be delayed/avoided. Evidence of the interbreeding of Ascaris suum and A. lumbricoides presents a cautionary tale of the need to take a One-Health approach to intervention strategies (48). While zoonotic reservoirs and/or interbreeding parasite populations present significant risk for the development of and lateral transfer of resistance, it is also possible that in some instances animal reservoirs could serve as an untreated sub-population, providing beneficial refugia. Consideration of such factors may prove critical for both the success of future intervention efforts and to avoid the development of resistance in STH species affecting humans.
Author contributions
SA, NP and JW conceptualized the review, MM carried out the literature review, SA and NP drafted the manuscript which was reviewed and finalized by all authors. All authors contributed to the article and approved the submitted version.
Funding
Funding for the DeWorm3 trial was provided by the Bill & Melinda Gates Foundation (grant number: INV-022149).
Acknowledgments
We thank Midhun Sasikumar for help in formatting the manuscript and references.
Conflict of interest
The authors declare that the research was conducted in the absence of any commercial or financial relationships that could be construed as a potential conflict of interest.
Publisher’s note
All claims expressed in this article are solely those of the authors and do not necessarily represent those of their affiliated organizations, or those of the publisher, the editors and the reviewers. Any product that may be evaluated in this article, or claim that may be made by its manufacturer, is not guaranteed or endorsed by the publisher.
References
1. de Silva NR, Brooker S, Hotez PJ, Montresor A, Engels D, Savioli L. Soil-transmitted helminth infections: updating the global picture. Trends Parasitol (2003) 19(12):547–51. doi: 10.1016/j.pt.2003.10.002
2. Pullan RL, Smith JL, Jasrasaria R, Brooker SJ. Global numbers of infection and disease burden of soil transmitted helminth infections in 2010. Parasites Vectors (2014) 7(1):37. doi: 10.1186/1756-3305-7-37
3. Bethony J, Brooker S, Albonico M, Geiger SM, Loukas A, Diemert D, et al. Soil-transmitted helminth infections: ascariasis, trichuriasis, and hookworm. Lancet (2006) 367(9521):1521–32. doi: 10.1016/S0140-6736(06)68653-4
4. Zawawi A, Else KJ. Soil-transmitted helminth vaccines: Are we getting closer? Front Immunol (2020) 11:576748. doi: 10.3389/fimmu.2020.576748
5. Brooker S, Hotez PJ, Bundy DAP. Hookworm-related anaemia among pregnant women: a systematic review. PloS Negl Trop Dis (2008) 2(9):e291. doi: 10.1371/journal.pntd.0000291
6. Smith JL, Brooker S. Impact of hookworm infection and deworming on anaemia in non-pregnant populations: a systematic review. Trop Med Int Health (2010) 15(7):776–95. doi: 10.1111/j.1365-3156.2010.02542.x
7. Taghipour A, Ghodsian S, Jabbari M, Olfatifar M, Abdoli A, Ghaffarifar F. Global prevalence of intestinal parasitic infections and associated risk factors in pregnant women: a systematic review and meta-analysis. Trans R Soc Trop Med Hyg (2021) 115(5):457–70. doi: 10.1093/trstmh/traa101
8. Mazibuko XI, Chimbari M. The effect of schistosomiasis and soil-transmitted helminths on expressive language skills among African preschool children. BMC Infect Dis (2022) 22(1):264. doi: 10.1186/s12879-022-07260-2
9. Crompton DW. The public health importance of hookworm disease. Parasitology (2000) 121 Suppl:S39–50. doi: 10.1017/S0031182000006454
10. Degarege A, Erko B. Association between intestinal helminth infections and underweight among school children in tikur wuha elementary school, northwestern Ethiopia. J Infect Public Health (2013) 6(2):125–33. doi: 10.1016/j.jiph.2012.11.008
11. Mireku MO, Boivin MJ, Davidson LL, Ouédraogo S, Koura GK, Alao MJ, et al. Impact of helminth infection during pregnancy on cognitive and motor functions of one-Year-Old children. PloS Negl Trop Dis (2015) 9(3):e0003463. doi: 10.1371/journal.pntd.0003463
12. King CH. Helminthiasis epidemiology and control: Scoring successes and meeting the remaining challenges. Adv Parasitol (2019) 103:11–30. doi: 10.1016/bs.apar.2018.08.001
13. Senecal J, Nordin A, Vinnerås B. Fate of ascaris at various pH, temperature and moisture levels. J Water Health (2020) 18(3):375–82. doi: 10.2166/wh.2020.264
14. Truscott JE, Turner HC, Farrell SH, Anderson RM. Soil-transmitted helminths: Mathematical models of transmission, the impact of mass drug administration and transmission elimination criteria. Adv Parasitol (2016) 94:133–98. doi: 10.1016/bs.apar.2016.08.002
15. Coffeng LE, Truscott JE, Farrell SH, Turner HC, Sarkar R, Kang G, et al. Comparison and validation of two mathematical models for the impact of mass drug administration on ascaris lumbricoides and hookworm infection. Epidemics (2017) 18:38–47. doi: 10.1016/j.epidem.2017.02.001
16. Pullan RL, Halliday KE, Oswald WE, Mcharo C, Beaumont E, Kepha S, et al. Effects, equity, and cost of school-based and community-wide treatment strategies for soil-transmitted helminths in Kenya: a cluster-randomised controlled trial. Lancet (2019) 393(10185):2039–50. doi: 10.1016/S0140-6736(18)32591-1
17. Anderson R, Farrell S, Turner H, Walson J, Donnelly CA, Truscott J. Assessing the interruption of the transmission of human helminths with mass drug administration alone: optimizing the design of cluster randomized trials. Parasites Vectors (2017) 10(1):93. doi: 10.1186/s13071-017-1979-x
18. Matamoros G, Rueda MM, Rodríguez C, Gabrie JA, Canales M, Fontecha G, et al. High endemicity of soil-transmitted helminths in a population frequently exposed to albendazole but no evidence of antiparasitic resistance. Trop Med Infect Dis (2019) 4(2):2. doi: 10.3390/tropicalmed4020073
19. Moser W, Schindler C, Keiser J. Efficacy of recommended drugs against soil transmitted helminths: systematic review and network meta-analysis. BMJ (2017) 358:j4307. doi: 10.1136/bmj.j4307
20. Vlaminck J, Cools P, Albonico M, Ame S, Ayana M, Cringoli G, et al. Therapeutic efficacy of albendazole against soil-transmitted helminthiasis in children measured by five diagnostic methods. PloS Negl Trop Dis (2019) 13(8):e0007471. doi: 10.1371/journal.pntd.0007471
21. Walker M, Cools P, Albonico M, Ame SM, Ayana M, Dana D, et al. Individual responses to a single oral dose of albendazole indicate reduced efficacy against soil-transmitted helminths in an area with high drug pressure. PloS Negl Trop Dis (2021) 15(10):e0009888. doi: 10.1371/journal.pntd.0009888
22. Schulz JD, Moser W, Hürlimann E, Keiser J. Preventive chemotherapy in the fight against soil-transmitted helminthiasis: Achievements and limitations. Trends Parasitol (2018) 34(7):590–602. doi: 10.1016/j.pt.2018.04.008
23. Moser W, Schindler C, Keiser J. Drug combinations against soil-transmitted helminth infections. Adv Parasitol (2019) 103:91–115. doi: 10.1016/bs.apar.2018.08.002
24. Avramenko RW, Redman EM, Windeyer C, Gilleard JS. Assessing anthelmintic resistance risk in the post-genomic era: a proof-of-concept study assessing the potential for widespread benzimidazole-resistant gastrointestinal nematodes in north American cattle and bison. Parasitology (2020) 147(8):897–906. doi: 10.1017/S0031182020000426
25. Rashwan N, Bourguinat C, Keller K, Gunawardena NK, Silva N, Prichard R. Isothermal diagnostic assays for monitoring single nucleotide polymorphisms in necator americanus associated with benzimidazole drug resistance. PloS Negl Trop Dis (2016) 10(12):e0005113. doi: 10.1371/journal.pntd.0005113
26. Roose S, Avramenko RW, Pollo SMJ, Wasmuth JD, Ame S, Ayana M, et al. Characterization of the β-tubulin gene family in ascaris lumbricoides and ascaris suum and its implication for the molecular detection of benzimidazole resistance. PloS Negl Trop Dis (2021) 15(9):e0009777. doi: 10.1371/journal.pntd.0009777
27. Diawara A, Halpenny CM, Churcher TS, Mwandawiro C, Kihara J, Kaplan RM, et al. Association between response to albendazole treatment and β-tubulin genotype frequencies in soil-transmitted helminths. PloS Negl Trop Dis (2013) 7(5):e2247. doi: 10.1371/journal.pntd.0002247
28. Orr AR, Quagraine JE, Suwondo P, George S, Harrison LM, Dornas FP, et al. Genetic markers of benzimidazole resistance among human hookworms (Necator americanus) in kintampo north municipality, Ghana. Am J Trop Med Hyg (2019) 100(2):351–6. doi: 10.4269/ajtmh.18-0727
29. Jimenez Castro PD, Howell SB, Schaefer JJ, Avramenko RW, Gilleard JS, Kaplan RM. Multiple drug resistance in the canine hookworm ancylostoma caninum: an emerging threat? Parasit Vectors (2019) 12(1):576. doi: 10.1186/s13071-019-3828-6
30. Furtado LFV, Bello ACP de P, dos Santos HA, Carvalho MRS, Rabelo ÉML. First identification of the F200Y SNP in the β-tubulin gene linked to benzimidazole resistance in ancylostoma caninum. Vet Parasitol (2014) 206(3–4):313–6. doi: 10.1016/j.vetpar.2014.10.021
31. Diawara A, Drake LJ, Suswillo RR, Kihara J, Bundy DAP, Scott ME, et al. Assays to detect beta-tubulin codon 200 polymorphism in trichuris trichiura and ascaris lumbricoides. PloS Negl Trop Dis (2009) 3(3):e397. doi: 10.1371/journal.pntd.0000397
32. Albonico M, Wright V, Bickle Q. Molecular analysis of the beta-tubulin gene of human hookworms as a basis for possible benzimidazole resistance on pemba island. Mol Biochem Parasitol (2004) 134(2):281–4. doi: 10.1016/j.molbiopara.2003.12.008
33. Schwenkenbecher JM, Albonico M, Bickle Q, Kaplan RM. Characterization of beta-tubulin genes in hookworms and investigation of resistance-associated mutations using real-time PCR. Mol Biochem Parasitol (2007) 156(2):167–74. doi: 10.1016/j.molbiopara.2007.07.019
34. Diawara A, Schwenkenbecher JM, Kaplan RM, Prichard RK. Molecular and biological diagnostic tests for monitoring benzimidazole resistance in human soil-transmitted helminths. Am J Trop Med Hyg (2013) 88(6):1052–61. doi: 10.4269/ajtmh.12-0484
35. Zuccherato LW, Furtado LF, Medeiros C da S, Pinheiro C da S, Rabelo ÉM. PCR-RFLP screening of polymorphisms associated with benzimidazole resistance in necator americanus and ascaris lumbricoides from different geographical regions in Brazil. PloS Negl Trop Dis (2018) 12(9):e0006766. doi: 10.1371/journal.pntd.0006766
36. Furtado LFV, Dos Santos TR, de Oliveira VNGM, Rabelo ÉML. Genotypic profile of benzimidazole resistance associated with SNP F167Y in the beta-tubulin gene of necator americanus helminths obtained from Brazilian populations. Infect Genet Evol (2020) 86:104594. doi: 10.1016/j.meegid.2020.104594
37. Gandasegui J, Grau-Pujol B, Cambra-Pelleja M, Escola V, Demontis MA, Cossa A, et al. Improving stool sample processing and pyrosequencing for quantifying benzimidazole resistance alleles in trichuris trichiura and necator americanus pooled eggs. Parasit Vectors (2021) 14(1):490. doi: 10.1186/s13071-021-04941-w
38. Hansen TVA, Thamsborg SM, Olsen A, Prichard RK, Nejsum P. Genetic variations in the beta-tubulin gene and the internal transcribed spacer 2 region of trichuris species from man and baboons. Parasit Vectors (2013) 6:236. doi: 10.1186/1756-3305-6-236
39. Krücken J, Fraundorfer K, Mugisha JC, Ramünke S, Sifft KC, Geus D, et al. Reduced efficacy of albendazole against ascaris lumbricoides in Rwandan schoolchildren. Int J Parasitol Drugs Drug Resist (2017) 7(3):262–71. doi: 10.1016/j.ijpddr.2017.06.001
40. Furtado LFV, Medeiros C da S, Zuccherato LW, Alves WP, de Oliveira VNGM, da Silva VJ, et al. First identification of the benzimidazole resistance-associated F200Y SNP in the beta-tubulin gene in ascaris lumbricoides. PloS One (2019) 14(10):e0224108. doi: 10.1371/journal.pone.0224108
41. Albonico M, Engels D, Savioli L. Monitoring drug efficacy and early detection of drug resistance in human soil-transmitted nematodes: a pressing public health agenda for helminth control. Int J Parasitol (2004) 34(11):1205–10. doi: 10.1016/j.ijpara.2004.08.001
42. Geerts S, Gryseels B. Drug resistance in human helminths: current situation and lessons from livestock. Clin Microbiol Rev (2000) 13(2):207–22. doi: 10.1128/CMR.13.2.207
43. Kotze AC, Gilleard JS, Doyle SR, Prichard RK. Challenges and opportunities for the adoption of molecular diagnostics for anthelmintic resistance. Int J Parasitol Drugs Drug Resist (2020) 14:264–73. doi: 10.1016/j.ijpddr.2020.11.005
44. Doyle SR, Cotton JA. Genome-wide approaches to investigate anthelmintic resistance. Trends Parasitol (2019) 35(4):289–301. doi: 10.1016/j.pt.2019.01.004
45. Melville LA, Redman E, Morrison AA, Rebecca Chen PC, Avramenko R, Mitchell S, et al. Large Scale screening for benzimidazole resistance mutations in nematodirus battus, using both pyrosequence genotyping and deep amplicon sequencing, indicates the early emergence of resistance on UK sheep farms. Int J Parasitol Drugs Drug Resist (2020) 12:68–76. doi: 10.1016/j.ijpddr.2020.03.001
46. Keiser J, Utzinger J. Community-wide soil-transmitted helminth treatment is equity-effective. Lancet (2019) 393(10185). doi: 10.1016/S0140-6736(18)32981-7
47. Boehme C, Carmona S, Nogaro S, Malecela M. Diagnostic testing holds the key to NTD elimination. PloS Negl Trop Dis (2021) 15(8):e0009626. doi: 10.1371/journal.pntd.0009626
Keywords: soil transmitted helminth, mass drug administration (MDA), drug resistance, neglected tropical disease, benzimidazole
Citation: Pilotte N, Manuel M, Walson JL and Ajjampur SSR (2022) Community-wide mass drug administration for soil-transmitted helminths – risk of drug resistance and mitigation strategies. Front. Trop. Dis 3:897155. doi: 10.3389/fitd.2022.897155
Received: 15 March 2022; Accepted: 31 October 2022;
Published: 16 November 2022.
Edited by:
Stephen R Doyle, Wellcome Sanger Institute (WT), United KingdomReviewed by:
Javier Gandasegui, Instituto Salud Global Barcelona (ISGlobal), SpainCopyright © 2022 Pilotte, Manuel, Walson and Ajjampur. This is an open-access article distributed under the terms of the Creative Commons Attribution License (CC BY). The use, distribution or reproduction in other forums is permitted, provided the original author(s) and the copyright owner(s) are credited and that the original publication in this journal is cited, in accordance with accepted academic practice. No use, distribution or reproduction is permitted which does not comply with these terms.
*Correspondence: Judd L. Walson, walson@uw.edu