- 1Entomology Department, Aggeu Magalhães Institute, Oswaldo Cruz Foundation, Recife, Brazil
- 2Department of Molecular and Cellular Biology, University of California, Davis, Davis, CA, United States
Culex quinquefasciatus is responsible for the transmission of filarial worms and several arboviruses. Olfaction plays a crucial role in disease transmission as it influences behaviors that are essential for the survival and reproduction of the mosquito, such as the host-seeking behavior, courtship, and oviposition. Understanding the molecular events that coordinate how mosquitoes find their host may lead to alternative methods to reduce diseases transmission. Our aim was to investigate the differential expression profile of odorant receptor (ORs) and odorant-binding proteins (OBPs) genes in Cx. quinquefasciatus field females compared with CqSLab laboratory mosquito colony. Seventeen genes of interest were evaluated for their qualitative and specific expression by RT-PCR on RNAs extracted from female antennae, female legs, complete male bodies, incomplete female bodies (no head and no legs), and L4 larvae. The general expression mapping of olfactory genes revealed that all analyzed genes were expressed in antennae. Some genes showed different qualitative expression profiles, such as CquiOR2, CquiOR64, CquiOR93, CquiOBP11, and CquiOBP16, which were expressed exclusively in female antennae. On the other hand, CquiOR37, CquiOBP2, and CquiOBP43 are expressed in all sample types, and CquiOBP10 was expressed in female antennae and legs and in the complete male bodies. The expression of CquiOBP5 was detected in the female’s antennae and body, but it was absent in the legs. The quantitative differential expression analysis of six of the 17 genes by RT-qPCR was performed from RNA samples from antenna pools collected in three physiological states, post-emergence, post-mating, and post-blood feeding of the field females and CqSLab. A total of 3,600 antennae were analyzed, in pools containing 100 pairs. Most genes screened showed a higher expression level in field mosquitoes when compared with the laboratory strain CqSLab. The expression of CquiOBP5 and CquiOBP10 genes was significantly different between the post-mating and post blood-meal samples of laboratory females (p < 0.05). Our results suggest specialization of the function of the genes studied and divergence in the expression pattern of field mosquitoes compared with laboratory mosquitoes, and therefore, caution should be exercised in the interpretation of data from laboratory mosquito studies.
Introduction
The southern house mosquito Culex quinquefasciatus mosquito is one of the most prevalent vectors of lymphatic filariasis, and females of the species have a hematophagic feeding behavior to complete their gonadotropic cycle, the main characteristic that involves them in the pathogens transmission (1, 2). For insects, the perception of different contexts in the environment depends on their sense of smell, which is considered essential for their survival and reproduction (3). Behavioral aspects such as the search for food, search for a mate, search for oviposition sites, and group communication, whether intraspecific or interspecific, are linked to the olfactory perception of insects (4–6). In mosquitoes, blood supply is a physiological requirement for egg maturation, and it is directly related to olfaction. The main organs directly linked to olfactory perception are the antennae and adaptive evolution resulted not only in morphological variations of this organ but also in functional specialization (3, 7).
In contact with biologically active chemical stimuli, insects respond variably in accordance with biotic or abiotic factors, experience from previous situations, and physiological state (5, 8). The olfactory nervous system is highly conserved among insects and is composed of olfactory receptor neurons (ORNs) and chemosensory proteins responsible for detecting peripheral odor molecules, including odorant receptors (ORs) and odorant-binding proteins (OBPs), which are located inside the sensilla in the antennae (5, 8, 9). The plasticity of the insect’s sensory system response occurs through changes in the expression of olfactory genes, activity, or neural structures (5). The response mechanism to odor stimuli occurs when odor molecules penetrate through the sensilla cuticle pores and reach the lumen filled by sensilla lymph, carried by OBPs to the ORN membrane, where these molecules interact with ORs, generating action potentials. From there, the stimulus travels throughout the insect’s nervous system to finally culminate in a behavioral response (8, 10).
OR and OBP proteins are responsible for modulating the peripheral olfactory sensory system (3, 8, 10). The ORs are integral membrane proteins that form heterodimers, giving rise to a ligand-dependent ion channel, activated by one or more types of odor molecules (4, 6, 11, 12). When odor molecules bind to ORs, ionic pore opens, providing the depolarization of the ORN and consequent propagation of an action potential (4). In Cx. quinquefasciatus, there is a large repertoire of ORs (13). The OBPs are small, soluble, low–molecular weight proteins with excellent stability to temperature variations, proteolysis, and against denaturing agents (14–16). They are found in large quantities in the lymph of insect sensilla and are classified on the basis of the number of amino acid residues found in their primary structure. They are further classified into subfamilies in terms of amino acid sequence and tissue expression. There are currently 109 OBPs annotated in the Cx. quinquefasciatus genome (13). These proteins play the important role of carrying hydrophobic odorant molecules through the lymph of the sensilla to the membrane of the ORNs. Previous studies indicate that insect OBPs have a modulating effect on olfactory physiology and on behavior linked to specific odorants (10). To characterize the functions of individual olfactory genes, recent studies have been used several techniques to silence these genes and identify their roles in insect behavior (17, 18).
A mosquito’s behavioral responses are directly related to gene expression within its cells. The fluctuation in gene expression through different physiological states is responsible for commanding what behavior will be performed. The example of behavior permutation can be seen after the blood feeding of a female mosquito, and the satiety state will make the female no longer look for hosts to feed on blood but will start the detection of odorants related to the search for good places for oviposition (19). There is a functional correlation between the behavioral and sensory response and the increased expression of chemosensory genes, which suggests a close relationship between the transcription of OR and OBP genes, and the function of their gene products, which translates into behaviors regulated by the condition physiological effect of each individual within a population of mosquitoes (19).
Considering the large diversification of the two gene families, in the present study, we aimed to describe the expression patterns of OR and OBP genes in a tropical Cx. quinquefasciatus population, compared with a laboratory colony, to identify putative subfuncionalization among these gene members. We focused on genes among the most abundantly expressed in the Cx. quinquefasciatus antenna described in Leal et al. (13). Our hypothesis here is that these genes may play different roles in crucial biological process for mosquitos’ adaptation and survival, and gene expression differentiation could be the first step to observe this. Here, the expression of OR and OBP genes in different tissues and in three physiological states of Cx. quinquefasciatus females was investigated using reverse transcriptase PCR (RT-PCR) and real-time quantitative PCR (RT-qPCR) assays. The differential expression of OR and OBP genes were screened in field mosquito antennae compared with laboratory mosquitoes antenna. Our results showed that four olfactory genes (CquiOBP2, CquiOBP10, CquiOR2, and CquiOR93) are significantly more expressed in field populations when compared with the laboratory colony at the post-mating stage. This allow us to speculate that these genes may play a role related to the detection of odorant stimuli in female field mosquitoes after mating, and they could be a target for the development of new methodologies and biotechnological products, which may help reduce disease transmission by interfering with the vector’s host-seeking behavior.
Material and methods
Mosquito colonies
Samples of Cx. quinquefasciatus were obtained from two colonies: CqSLab, a reference colony from the city of Recife, maintained for more than 10 years under laboratory conditions; and a field population, established from eggs rafts of the mosquito Cx. quinquefasciatus collected from two municipalities of Recife (S 8°03′36.6″, W 34°53′21.1″), Mustardinha and Cidade Universitária, both belonging to the Metropolitan region of Recife (MRR) and with high rates of infestation and proliferation by mosquitoes of the species Cx. quinquefasciatus. Only the F1 generation from the field population was used in the present study. All mosquitoes were kept in the insectary of the entomology department under controlled conditions of temperature (26 ± 2°C), humidity (70% ± 20%), and a photoperiod of 12-h light/12-h darkness (20).
OR and OBP genes selected
Seventeen genes were selected from two families of chemosensory genes, among those reported in Leal et al. (13) as the most abundant in antenna samples: OR (seven ORs genes) and OBP (10 OBPs genes). Specific primers for each gene were designed using PrimerSelect and EditSeq softwares, DNASTAR 7.0.0 package (Supplementary Table 1) according to the VectorBase code, published by 13. The 18S ribosomal gene was used as the endogenous gene (21).
Qualitative tissue-specific gene expression assays of ORs and OBPs by RT-PCR
For the RT-PCR assays, five types of tissue samples were produced in pools: 1) 100 pairs of female antennae; 2) 60 female legs; 3) five complete bodies of male mosquitoes; 4) five incomplete bodies of females (headless and legless); and 5) five fourth instar larvae from the CqSLab colony, to map the tissue-specific expression of target genes through the presence of transcripts. RNA extractions were conducted using TRIzol™ (Invitrogen) following the manufacturer’s protocol. Samples were treated with DNase™ (Ambion-Invitrogen), quantified in NanoDrop 2000c spectrophotometer (Thermo Scientific), and then were converted to cDNA with SuperScript™ II RT (Invitrogen) following the manufacturer’s protocol and quantified. Finally, reactions were performed with the Taq PCR Master Mix Kit (Qiagen) in Mastercycler® nexus X2 gradient thermal cycler (Eppendorf). The amplified products were analyzed by electrophoresis in 2% agarose gel in 0.5× TBE(Tris-Borate-EDTA running buffer pH 8.0) and stained with ethidium bromide (10 mg/ml).
Tissue collection for RT-qPCR
Experiments to obtain female mosquitoes in three specific physiological states (post-emergence, post-mating, and post-blood feeding) were optimized.
Post-emergence: The experiment consisted of six small cages (20 cm × 15.2 cm × 16 cm), three with field mosquitoes and three with CqSLab mosquitoes, containing 100 female pupae each cage, to obtain newly emerged adult females. The aspiration of the cages was performed with an automatic manual aspirator; the females collected were frozen at −80°C, after 20 min, and transferred to petri dishes to avoid losing the antennae. The antennae were dissected using entomological tweezers and placed in 1.5-ml microtubes, to produce homogenates containing 100 antennae pairs. The samples were macerated with a plastic pestle, and finally, the homogenates were stored at −80°C.
Post-mating: The experiment consisted of six small cages, three cages with field mosquitoes and three cages with CqSLab mosquitoes, containing 100 females and 200 males each, in the adult phase. After 7 days of contact, mosquitoes were captured with an automatic manual aspirator, and only females were selected and frozen at −80°C. The insemination status was verified through the dissection and analysis of the spermatheca, fixed and stained with Giemsa in a ZEISS West Germany Axiophot optical microscope, present at the Nucleus of Technological Platforms (NPT-IAM) under 20× to 40× objectives. Only the antennae of inseminated females (positive sperm) were dissected to produce homogenates, composed of 100 antennae pairs of inseminated females and 100 µl of ultra-pure water. Lastly, the homogenates were stored at −80°C.
Post blood feeding: The experiment consisted of six small cages, three cages containing field mosquitoes and three cages containing CqSLab mosquitoes, each one with 100 females and 200 males. After 7 days of contact, they received blood meal and defibrinated rabbit blood (Oryctolagus cuniculus) in an artificial apparatus. The engorged females were captured 2 h after the blood meal with an automatic manual aspirator and frozen at −80°C. The antennae were dissected with entomological forceps, and the homogenates were prepared with 100 pairs of antennae for each cage used and 100 µl of ultra-pure water.
Evaluation of the OR and OBP genes differentially expressed in three physiological states through RT-qPCR
After RT-PCR assays, nine genes (OR2, OR93, OBP16, OR64, OBP11, OBP2, OBP5, OBP10, and OR151) were tested by real-time quantitative reverse transcription PCR (RT-qPCR) using absolute quantification. These genes were selected among the 17 genes analyzed by RT-PCR based on tissue specificity; OR2, OR64, OR93, OBP11, and OBP16 were exclusively expressed in antenna; and the remaining displayed a diverse qualitative expression profile (OBP2, OBP5, OBP10, and OR151). From these nine genes, only a subset of six genes (OBP2, OBP5, OBP10, OBP11, OR2, and OR93) resulted in good PCR efficiency to be used in real-time qPCR assays according to the Minimum Information for Publication of Quantitative Real-Time PCR Experiments (MIQE) guidelines (22) and was selected for one-step quantitative RT-PCR, using relative quantification. These genes have been shown to play roles in the physiological states progression in insects such as reproduction, pheromone perception, oviposition, and others (23–28). The evaluation of the differential expression of the target genes was conducted between three physiological states of Cx. quinquefasciatus females. Total RNAs were extracted from pools of 100 pairs of antennae of female mosquitoes from the CqSLab colony and from the field colony, with TRIzol™ (Invitrogen), according to the manufacturer’s instructions. RNA samples were treated with DNase™ (Ambion-Invitrogen) followed by quantification in Qubit™ 3.0 Fluorometer (Thermo Scientific). Samples were normalized at concentration of 10 ng. The 18S ribosomal gene was used as the endogenous control of reactions. The RT-qPCR experiments were carried out in a ABI 7500 real-time PCR system (Applied Biosystems), samples were tested in biological triplicate with technical replicates, and relative quantification analyses were performed by the 2−ΔΔCT method (29), with post-emergence CqSLab used as a calibrator sample in analyses, through the 7500 software version 2.0.4 (Applied Biosystems). Statistically significant differences in gene expression by RT-qPCR were evaluated with unpaired t-test, using the GraphPad Prism 8 software.
Results
RT-PCR
Transcripts of all the 17 genes evaluated were detected by RT-PCR, producing PCR products with the expected size. All tested genes were expressed in antenna, but five of them showed a tissue-specific transcription being exclusive to antennae, and they were CquiOR2 (CPIJ002479), CquiOR64 (CPIJ006216), CquiOR93 (CPIJ039866), CquiOBP11 (CPIJ006551), and CquiOBP16 (CPIJ012715). Three genes—CquiOR37 (CPIJ004163), CquiOBP2 (CPIJ007617), and CquiOBP43 (CPIJ017326)—were ubiquitously expressed and displayed expression in all samples analyzed (female antennae, female legs, incomplete female bodies, whole males, and L4 larvae), and the remaining genes—CquiOBP3 (CPIJ007611), CquiOBP5 (CPIJ007608), CquiOBP7 (CPIJ001365), CquiOBP10 (CPIJ013976), CquiOBP23 (CPIJ001876), CquiOBP46 (CPIJ010782), CquiOR121 (CPIJ014392), CquiOR125 (CPIJ015178), and CquiOR151 (CPIJ017911)—produced transcripts in at least two tissues. Seven genes (CquiOR37, CquiOR151, CquiOBP2, CquiOBP3, CquiOBP7, CquiOBP10, and CquiOBP43) were expressed in both males and females (Figure 1).
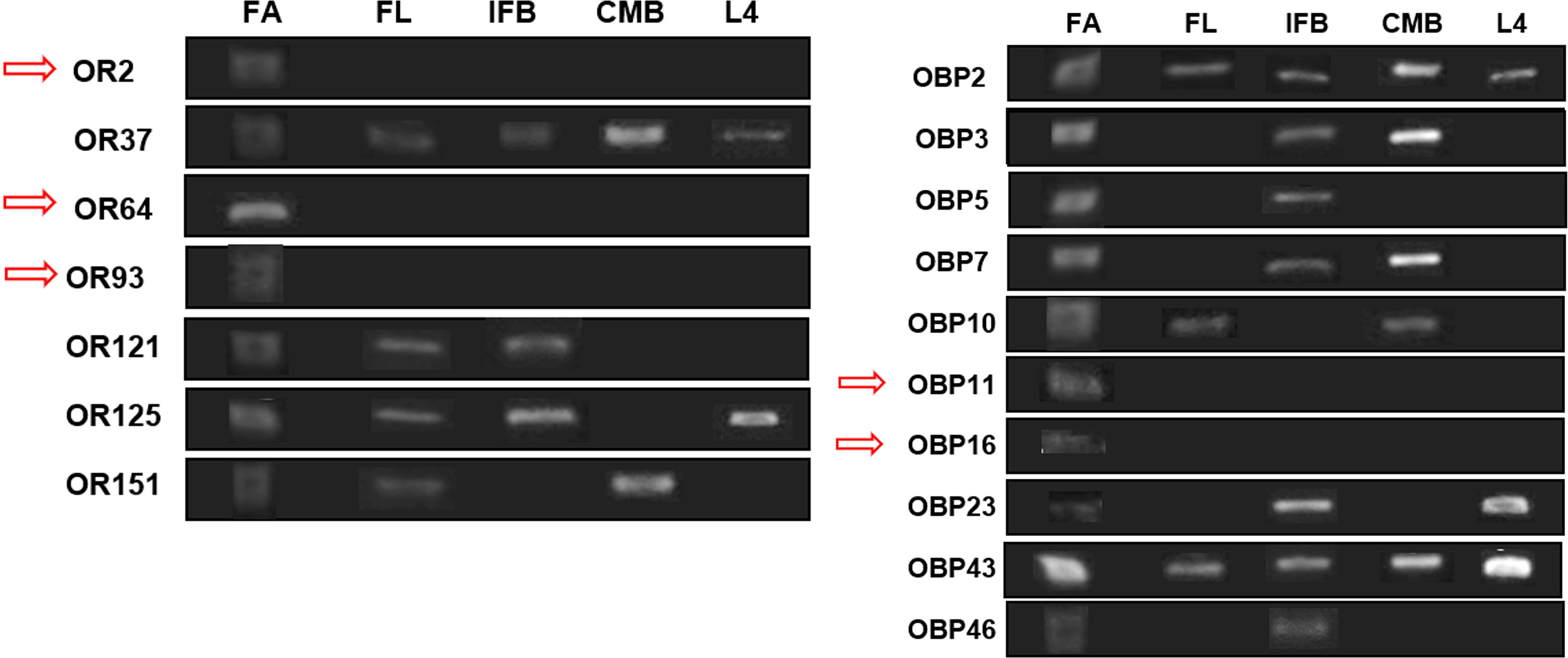
Figure 1 Overview of OR and OBP genes transcripts, observed through RT-PCR. The image consists of the clipping of all agarose gels performed, showing the bands related to the amplified genes in each tissue sample evaluated. FA, female body; FL, female leg; IFB, incomplete female body; CMB, complete male body; L4, fourth instar larvae. The red arrows indicate the genes with transcripts exclusively in the antennae of female Cx. quinquefasciatus.
Differential gene expression by RT-qPCR at female adult life stages
In general, the RT-qPCR results showed that most genes, apart from OR93 at post-blood feeding, were predominantly more expressed in the field samples when compared with the laboratory colony CqSLab (Figure 2A–C). This result was statistically significant (p < 0.05) for four genes (OBP2, OBP10, OR2, and OR93) expressed at higher levels in female antennae from the post-mating stage in field samples, one gene at post-emergence (OBP11), and one gene (OBP10) at post-blood feeding (Figure 2). There was no significant difference of expression among the three physiological stages for both laboratory and field samples, with the exception of two genes (OBP5 and OBP10), which were expressed at significantly higher levels (p < 0.05) in female antennae at the post-blood feeding stage, compared with the post-mating stage in laboratory colony (Figure 3C). In addition, when comparing CqSLab collected after emergence with after mating, OBP10 was down regulated, and OBP5 was upregulated after blood meal (Figures 3A, B, respectively).
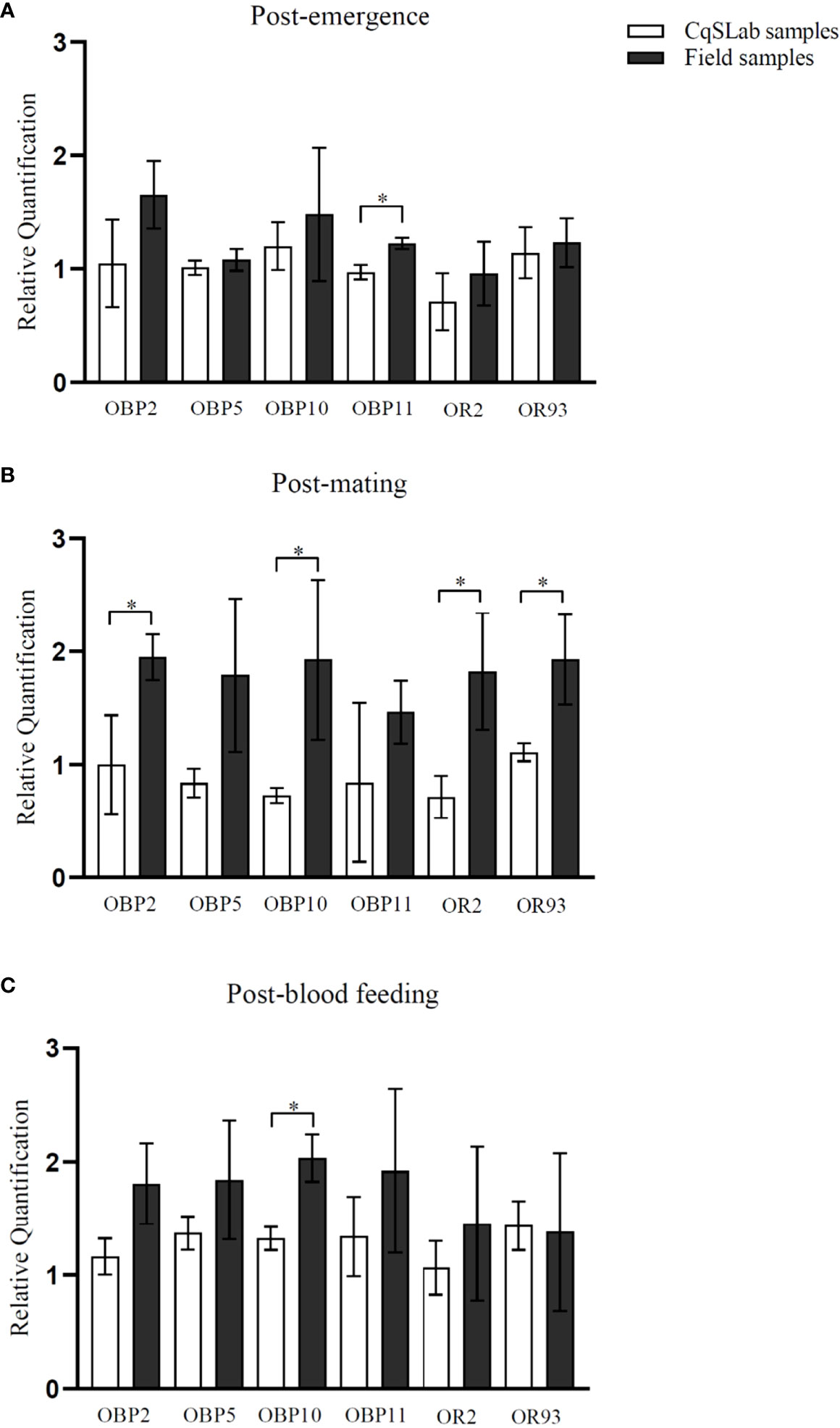
Figure 2 Graphs per physiological state generated from RT-qPCR assays with genes CquiOBP2, CquiOBP5, CquiOBP10, CquiOBP11, CquiOR2, and CquiOR93: (A) Post-emergence; (B) Post-mating; (C) Post-blood feeding. Increased expression is noted in most genes. Five genes showed a significant increase in expression (p < 0.05) in antennae samples from field females compared with laboratory samples, four (CquiOBP2, CquiOBP10, CquiOR2, and CquiOR93) in the post-mating physiological state, and one (CquiOBP11) in the post-emergence and OBP10 again in the post-blood feeding. Bars indicate standard deviation. (*) Statistically significant (p < 0.05).
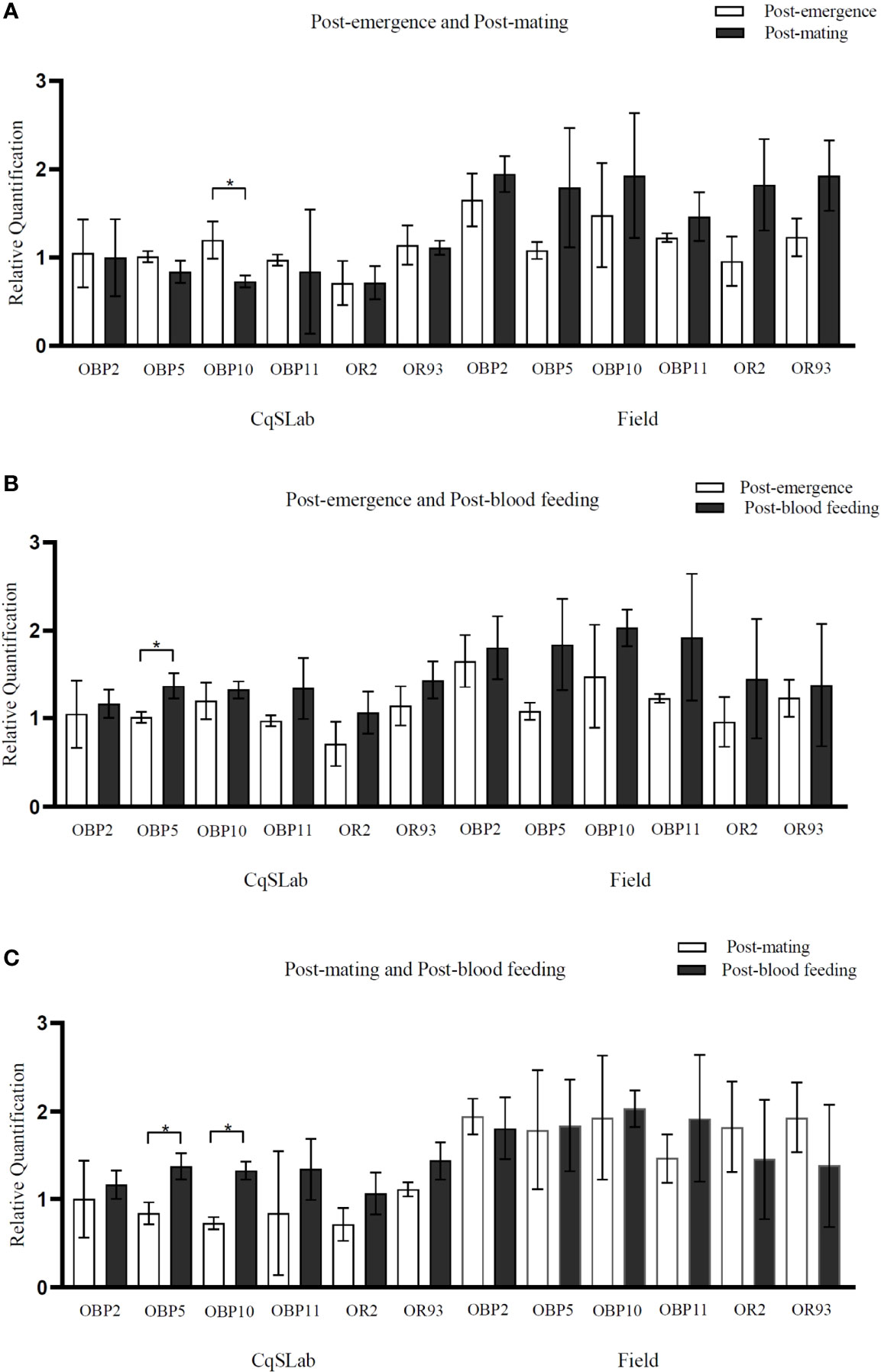
Figure 3 Graphs generated from RT-qPCR assays with genes CquiOBP2, CquiOBP5, CquiOBP10, CquiOBP11, CquiOR2, and CquiOR93 comparing the physiological stages post-emergence and post-mating (A), post-emergence and post-blood feeding (B), and post-mating and post-blood feeding (C) in laboratory colony and field population.The genes OBP5 and OBP10 were expressed at significantly higher levels (p < 0.05) in CqSLab female antennae at the post blood feeding stage, in comparison with the post-mating stage (C). The gene OBP10 was expressed at significantly lower level (p < 0.05) in CqSLab female antennae at the post-mating stage in comparison with the post-emergence stage (A). The differential expression of genes analysed comparing the physiological states in the field colony was not statistically significant. Bars indicate standard deviation. (*) Statistically significant (p < 0.05).
Discussion
In the present study, we investigated a subset of olfactory genes in Cx. quinquefasciatus that may be associated with important traits such as host-seeking behavior. Our results showed that, in general, genes were differentially expressed in field caught mosquitoes compared with the laboratory colony in at least one of the physiological states studied, except for the OBP5 gene, showing that the colonization process when founding a colony in the insectary impacted on the mechanisms regulating its expression.
The results of RT-PCR showed a great variety of expression pattern for the 17 genes, with only three being ubiquitously expressed in all tissues analyzed. All the remaining genes were specific to few tissues, but all of them were expressed in antennae, corroborating with Leal et al. (13) results, although they have used a colony of Cx. quinquefasciatus collected in the USA and colonized in a laboratory in 1950. They have observed differential expression of olfactive genes using RNA-seq in this same mosquito species, with great expression in antennae samples. These data highlight the specialization of function in the members of this important gene family.
Like other mosquito species, Cx. quinquefasciatus females acquire a blood meal after mating, and this behavior is directly related to the variation in the gene expression of their olfactory system, between one physiological state and another, stimulating the behavior of searching for a host to complete its gonadotropic cycle (1, 5, 30). In this study, in addition to observe the expression of OR and OBP genes at different tissues, we have investigated the expression of six genes by RT-qPCR at three different moments of adult female’s life. Here, we were able to detect variation in gene expression among the different life stages for OBP5 and OBP10 genes in the laboratory colony, providing information for future studies, which will be required for demonstrating the ecological significance of such variation in transcript levels. Studying the differences of gene expression profile along life development and over adult life is important to better understand vectorial capacity in mosquito populations. Analysis of microarray studies in Anopheles gambiae revealed that specific genes are differentially expressed, displaying an age-dependent transcriptional level, which could be used to determine the age of mosquitoes (31). These changes in mosquito females’ adult life could interfere in the blood-feeding pattern or the interaction with repellent products. Indeed, we have previously demonstrated that the age of Aedes aegypti females affect mosquito responses to DEET repellent (32). A previous study showed that OBP5 was involved with transportation of a human-derived attractant nonanal and the insect repellent picaridin, suggesting the link of this OBP with the detection of blood source. There was also affinity between OBP5 and the oviposition pheromone MOP (24).
Most studies with the OR and OBP genes in mosquito species are limited to identification and genomic characterization (33–36). More recently, some studies are now focused on characterizing its function in mosquito biology. Taparia etal. (1) have demonstrated that members of the OR8 gene subfamily are regulated in response to blood-feeding and are possibly involved with host-seeking behavior in the Cx. quinquefasciatus species. Likewise, Wu et al. (2) found a positive correlation between the expression of the two genes CquiOR114/117 and the blood-feeding behavior. They found variation of the expression level of both genes at different days after emergence of mosquitoes and the correlation was demonstrated by silencing the genes using the RNA interference technique. Similarly, a study using CRISPR/Cas9-mediated silencing of two genes in Ae. aegypti (OBP10 and OBP22) showed its involvement in the host-seeking behavior (26). Interestingly, the authors also demonstrated that those OBPs have a pleiotropic effect on several processes important for mosquito vectorial capacity, such as reproduction and arbovirus transmission.
Recently, the higher expression of an OBP gene in a Culex quinquefasciatus resistant colony was, for the first time, associated to insecticide resistance (37). This suggests a new mechanism of insecticide resistance, and therefore, more studies should be conducted to identify OBP role in the development of insecticide resistance. In the present study, we have identified eight OBPs that are expressed in the legs or female bodies and could be further investigated regarding its possible interaction with insecticides.
Culex quinquefasciatus has 109 OBP genes and 180 OR genes annotated (13, 35), which represent an expansion in comparison with the 131 OR in the other domestic mosquito Ae. aegypti (38). Regarding the OBP genes, 111 were found in Ae. aegypti; however, only 19 direct orthologs were observed between these two species (35). Although both species are domestic mosquitoes, Cx. quinquefasciatus has special features that are probably associated to its great diversity of olfactory genes, such as egg laying in a wide variety of polluted and non-polluted larval habitats and the ability to feed on diverse animal species, including human and birds (39). Considering its great vectorial capacity for transmitting several pathogens such as nematodes, virus, and protozoan and that it is a cosmopolitan species, in contrast to Ae. aegypti distribution (present only in tropical and sub-tropical areas), studying the functional characterization of olfactory genes is a big challenge. Divergence of gene expression pattern for olfactory genes has been observed even for subspecies. In a very recent paper, Gu et al. (18) identified genes (OR5 and OR65) that were expressed in a subspecies-specific way and demonstrated through RNA interference that two olfactory genes could inhibit the blood-feeding behavior of Cx. quinquefasciatus. Our data show that studies for the functional characterization of olfactory genes in mosquitoes using laboratory strains should analyze the data with caution, as the results for natural populations may differ considerably. Furthermore, the expression pattern of the OR and OBP gene repertoire may vary according to each ecological niche.
We are all witnessing the impact of global warming on the environment and on the lives of different species of organisms, particularly on mosquitoes; it will probably increase its density and distribution (40–42). The current mosquito control measures have not been successful enough to reduce the number of cases of vector-borne diseases in endemic areas; in this sense, personal protection measures emerge as an important tool to prevent the increase in disease transmission. The present study opens up new research possibilities to better understand the genetic basis of mosquito olfaction.
Data availability statement
The raw data supporting the conclusions of this article will be made available by the authors, without undue reservation.
Author contributions
SS: conception of the study, data acquisition, analysis of data, and manuscript writing; TR: conception of the study, experimental execution support, data analyses, and manuscript writing and revision; EH: acquisition of data, experimental execution support, and data analyses; RB: experimental execution support; WL: data analysis, manuscript revision, and intellectual contributions. CJ: intellectual contributions, manuscript writing and revision, and financial support. All authors reviewed the paper and agreed with the final version.
Funding
This work was supported by Conselho Nacional de Desenvolvimento Científico e Tecnológico (CNPq Brazil, CNPq/PAPES VI Fiocruz 407475/2012-5) and FACEPE-FIOCRUZ (grant APQ-0085-2.13/16 and APQ-1630-2.01/15).
Acknowledgments
The authors thank the team of the Insectarium and of the Animals Facilities at IAM-FIOCRUZ-PE for technical support; the Program for Technological Development in Tools for Health-PDTIS-FIOCRUZ for use of its automatic real-time PCR facilities; the Coordenação de Aperfeiçoamento de Pessoal de Nível Superior (CAPES – Finance code 001) for financial support; and the Fundação Oswaldo Cruz/Vice-Presidência de Pesquisa e Laboratórios de Referência/Serviço de Referência para Controle de Culicídeos Vetores/Instituto Aggeu Magalhães-PE (FIOCRUZ/VPPLR/SRCCV/IAM-PE).
Conflict of interest
The authors declare that the research was conducted in the absence of any commercial or financial relationships that could be construed as a potential conflict of interest.
Publisher’s note
All claims expressed in this article are solely those of the authors and do not necessarily represent those of their affiliated organizations, or those of the publisher, the editors and the reviewers. Any product that may be evaluated in this article, or claim that may be made by its manufacturer, is not guaranteed or endorsed by the publisher.
Supplementary material
The Supplementary Material for this article can be found online at: https://www.frontiersin.org/articles/10.3389/fitd.2022.874727/full#supplementary-material
References
1. Taparia T, Ignell R, Hill SR. Blood meal induced regulation of the chemosensory gene repertoire in the southern house mosquito. BMC Genomics (2017) 18:1–9. doi: 10.1186/s12864-017-3779-2
2. Wu Q, Li C, Liu Q, Guo X, Shi Q, Zhang H, et al. RNA Interference of odorant receptor CquiOR114/117 affects blood-feeding behavior in Culex quinquefasciatus. Acta Trop (2020) 204:105343. doi: 10.1016/j.actatropica.2020.105343
3. Fan J, Francis F, Liu Y, Chen JL, Cheng DF. An overview of odorant-binding protein functions in insect peripheral olfactory reception. Genet Mol Res (2011) 10:3056–69. doi: 10.4238/2011.December.8.2
4. Carraher C, Dalziel J, Jordan MD, Christie DL, Newcomb RD, Kralicek AV. Towards an understanding of the structural basis for insect olfaction by odorant receptors. Insect Biochem Mol Biol (2015) 66:31–41. doi: 10.1016/j.ibmb.2015.09.010
5. Gadenne C, Barrozo RB, Anton S. Plasticity in insect olfaction: To smell or not to smell? Annu Rev Entomol (2016) 61:317–33. doi: 10.1146/annurev-ento-010715-023523
6. Hughes DT, Pelletier J, Rahman S, Chen S, Leal WS, Luetje CW. Functional and nonfunctional forms of CquiOR91, an odorant selectivity subunit of culex quinquefasciatus. Chem Senses (2017) 42:333–41. doi: 10.1093/chemse/bjx011
7. Sim S, Ramirez JL, Dimopoulos G. Dengue virus infection of the aedes aegypti salivary gland and chemosensory apparatus induces genes that modulate infection and blood-feeding behavior. PLoS Pathog (2012) 8:e1002631. doi: 10.1371/journal.ppat.1002631
8. Wolff GH, Riffell JA. Olfaction, experience and neural mechanisms underlying mosquito host preference. J Exp Biol (2018) 221:jeb157131. doi: 10.1242/JEB.157131
9. Guidobaldi F, May-Concha IJ, Guerenstein PG. Morphology and physiology of the olfactory system of blood-feeding insects. J Physiol Paris (2014) 108:96–111. doi: 10.1016/j.jphysparis.2014.04.006
10. Leal WS. Odorant reception in insects: Roles of receptors, binding proteins, and degrading enzymes. Annu Rev Entomol (2013) 58:373–91. doi: 10.1146/annurev-ento-120811-153635
11. Carey AF, Wang G, Su CY, Zwiebel LJ, Carlson JR. Odorant reception in the malaria mosquito Anopheles gambiae. Nature (2010) 464:66–71. doi: 10.1038/nature08834
12. Li Q, Liberles SD. Aversion and attraction through olfaction. Curr Biol (2015) 25:120–9. doi: 10.1016/j.cub.2014.11.044.Aversion
13. Leal WS, Choo Y-M, Xu P, da Silva CSB, Ueira-Vieira C. Differential expression of olfactory genes in the southern house mosquito and insights into unique odorant receptor gene isoforms. Proc Natl Acad Sci (2013) 110:18704–9. doi: 10.1073/pnas.1316059110
14. Brito NF, Moreira MF, Melo ACA. A look inside odorant-binding proteins in insect chemoreception. J Insect Physiol (2016) 95:51–65. doi: 10.1016/j.jinsphys.2016.09.008
15. De T, Das Thomas T, Verma S, Singla D, Chauhan C, Srivastava V, et al. A synergistic transcriptional regulation of olfactory genes drives blood-feeding associated complex behavioral responses in the mosquito Anopheles culicifacies. Front Physiol (2018) 9:577. doi: 10.3389/fphys.2018.00577
16. Pelosi P, Iovinella I, Zhu J, Wang G, Dani FR. Beyond chemoreception: diverse tasks of soluble olfactory proteins in insects. Biol Rev (2018) 93:184–200. doi: 10.1111/brv.12339
17. Diallo S, Shahbaaz M, Makwatta JO, Muema JM, Masiga D, Christofells A, et al. Antennal enriched odorant binding proteins are required for odor communication in Glossina f. fuscipes. Biomolecules (2021) 11:1–21. doi: 10.3390/biom11040541
18. Gu ZY, Gao HT, Yang QJ, Ni M, Li MJ, Xing D, et al. Screening of olfactory genes related to blood-feeding behaviors in culex pipiens quinquefasciatus and Culex pipiens molestus by transcriptome analysis. PLoS Negl Trop Dis (2022) 16(2):e0010204. doi: 10.1371/journal.pntd.0010204
19. Omondi AB, Ghaninia M, Dawit M, Svensson T, Ignell R. Age-dependent regulation of host seeking in Anopheles coluzzii. Sci Rep (2019) 9:1–9. doi: 10.1038/s41598-019-46220-w
20. Amorim LB, Helvecio E, de Oliveira CMF, Ayres CFJ. Susceptibility status of Culex quinquefasciatus (Diptera: Culicidae) populations to the chemical insecticide temephos in pernambuco, Brazil. Pest Manage Sci (2013) 69:1307–14. doi: 10.1002/ps.3502
21. Liu N, Li T, Reid WR, Yang T, Zhang L. Multiple cytochrome P450 genes: Their constitutive overexpression and permethrin induction in insecticide resistant mosquitoes, culex quinquefasciatus. PLoS One (2011) 6:e23403. doi: 10.1371/journal.pone.0023403
22. Bustin SA, Benes V, Garson JA, Hellemans J, Huggett J, Kubista M, et al. The MIQE guidelines: minimum information for publication of quantitative real-time PCR experiments. Clin Chem (2009) 55(4):6–1. doi: 10.1373/clinchem.2008.112797
23. Scialò F, Hansson BS, Giordano E, Polito CL, Digilio FA. Molecular and functional characterization of the odorant Receptor2 (OR2) in the tiger mosquito Aedes albopictus. PLoS One (2012) 7:e36538. doi: 10.1371/journal.pone.0036538
24. Yin J, Choo YM, Duan H, Leal W.S. Selectivity of odorant-binding proteins from the southern house mosquito tested against physiologically relevant ligands. Front Physiol (2015) 6:56. doi: 10.3389/fphys.2015.00056
25. Ruel DM, Yakir E, Bohbot JD. Supersensitive odorant receptor underscores pleiotropic roles of indoles in mosquito ecology. Front Cell Neurosci (2019) 12:533. doi: 10.3389/fncel.2018.00533
26. Dong S, Ye Z, Tikhe CV, Tu ZJ, Zwiebel LJ, Dimopoulos G. Pleiotropic odorant-binding proteins promote Aedes aegypti reproduction and flavivirus transmission. MBio (2021) 12:1–18. doi: 10.1128/mBio.02531-21
27. Hu L, Chen B, Liu K, Yu G, Chen Y, Dai J, et al. OBP2 in the midlegs of the Male bactrocera dorsalis is involved in the perception of the female-biased sex pheromone 4-Allyl-2,6-dimethoxyphenol. J Agric Food Chem (2021) 69:126–34. doi: 10.1021/acs.jafc.0c05945
28. Silva D, Ceballos R, Arismendi N, Dalmon A, Vargas M. Variant a of the deformed wings virus alters the olfactory sensitivity and the expression of odorant binding proteins on antennae of Apis mellifera. Insects (2021) 12:895. doi: 10.3390/insects12100895
29. Livak KJ, Schmittgen TD. Analysis of relative gene expression data using real-time quantitative PCR and the 2-ΔΔCT method. Methods (2001) 25:402–8. doi: 10.1006/meth.2001.1262
30. Camargo C, Ahmed-Braimah YH, Amaro IA, Harrington LC, Wolfner MF, Avila FW. Mating and blood-feeding induce transcriptome changes in the spermathecae of the yellow fever mosquito Aedes aegypti. Sci Rep (2020) 10:1–13. doi: 10.1038/s41598-020-71904-z
31. Cook PE, Sinkins SP. Transcriptional profiling of Anopheles gambiae mosquitoes for adult age estimation. Insect Mol Biol (2010) 19:745–51. doi: 10.1111/j.1365-2583.2010.01034.x
32. Leal WS, Barbosa RMR, Zeng F, Faierstein GB, Tan K, Paiva MHS, et al. Does zika virus infection affect mosquito response to repellents? Sci Rep (2017) 7:1–9. doi: 10.1038/srep42826
33. Zhou JJ, He XL, Pickett JA, Field LM. Identification of odorant-binding proteins of the yellow fever mosquito Aedes aegypti: genome annotation and comparative analyses. Insect Mol Biol (2008) 17:147–63. doi: 10.1111/j.1365-2583.2007.00789.x
34. Pitts RJ, Rinker DC, Jones PL, Rokas A, Zwiebel LJ. Transcriptome profiling of chemosensory appendages in the malaria vector anopheles gambiae reveals tissue- and sex-specific signatures of odor coding. BMC (2011) 12:271. doi: 10.1186/1471-2164-12-271
35. Manoharan M, Chong MNF, Vaïtinadapoulé A, Frumence E, Sowdhamini R, Offmann B. Comparative genomics of odorant binding proteins in anopheles gambiae, aedes aegypti, and culex quinquefasciatus. Genome Biol Evol (2013) 5:163–80. doi: 10.1093/gbe/evs131
36. Matthews BJ, McBride CS, DeGennaro M, Despo O, Vosshall LB. The neurotranscriptome of the Aedes aegypti mosquito. BMC Genomics (2016) 17:1–20. doi: 10.1186/s12864-015-2239-0
37. Shen RX, Wang YT, Wu JH, Zhang N, Zhang HD, Xing D, et al. Deltamethrin interacts with Culex quinquefasciatus odorant-binding protein: a novel potential resistance mechanism. Parasit Vectors (2022) 15(1):2. doi: 10.1186/s13071-021-05041-5
38. Nene V, Wortman JR, Lawson D, Haas B, Kodira C, Tu Z, et al. Genome sequence of Aedes aegypti, a major arbovirus vector. Sci (80- ) (2007) 316:1718–23. doi: 10.1126/science.1138878
39. Reddy BP, Labbé P, Corbel V. Culex genome is not just another genome for comparative genomics. Parasit Vectors (2012) 5:63. doi: 10.1186/1756-3305-5-63
40. Ramasamy R, Surendran SN. Global climate change and its potential impact on disease transmission by salinity-tolerant mosquito vectors in coastal zones. Front Physiol (2012) 3:198. doi: 10.3389/fphys.2012.00198
41. Samy AM, Elaagip AH, Kenawy MA, Ayres CF, Peterson AT, Soliman DE. Climate change influences on the global potential distribution of the mosquito Culex quinquefasciatus, vector of West Nile virus and lymphatic filariasis. PLoS One (2016) 11(10):e0163863. doi: 10.1371/journal.pone.0163863
Keywords: differential gene expression, RT-qPCR, olfactory genes, mosquito vector, host seeking behavior
Citation: Santos SA, Romão TP, Helvecio E, Barbosa RMR, Leal WS and Ayres CFJ (2022) Elevated expression of odorant receptors and odorant-binding proteins genes detected in antennae of Culex quinquefasciatus field females. Front. Trop. Dis 3:874727. doi: 10.3389/fitd.2022.874727
Received: 12 February 2022; Accepted: 24 June 2022;
Published: 26 July 2022.
Edited by:
Kounbobr Roch Dabiré, Research Institute for Health Science-Direction Régionale de l’Ouest (IRSS-DRO), Burkina FasoReviewed by:
Merid Negash Getahun, International Centre of Insect Physiology and Ecology (ICIPE), KenyaRajnikant Dixit, National Institute of Malaria Research (ICMR), India
Copyright © 2022 Santos, Romão, Helvecio, Barbosa, Leal and Ayres. This is an open-access article distributed under the terms of the Creative Commons Attribution License (CC BY). The use, distribution or reproduction in other forums is permitted, provided the original author(s) and the copyright owner(s) are credited and that the original publication in this journal is cited, in accordance with accepted academic practice. No use, distribution or reproduction is permitted which does not comply with these terms.
*Correspondence: Constância Flávia Junqueira Ayres, Y29uc3RhbmNpYS5heXJlc0BmaW9jcnV6LmJy; Tatiany Patricia Romão, dGF0aWFueS5yb21hb0BmaW9jcnV6LmJy
†These authors have contributed equally to this work