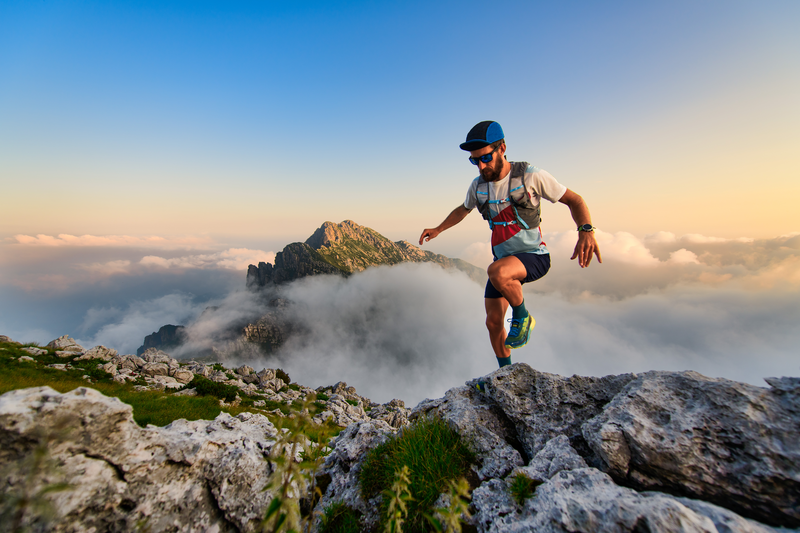
94% of researchers rate our articles as excellent or good
Learn more about the work of our research integrity team to safeguard the quality of each article we publish.
Find out more
MINI REVIEW article
Front. Trop. Dis. , 18 February 2022
Sec. Vector Biology
Volume 3 - 2022 | https://doi.org/10.3389/fitd.2022.828712
This article is part of the Research Topic Parasite Interactions with Insect Hosts in Tropical Diseases View all 5 articles
The Triatominae subfamily (Reduviidae) harbors some hematophagous insect species that have been firmly connected to the transmission of Trypanosoma cruzi, the causative agent of Chagas disease. Triatomines not only host and transmit trypanosomatids, but also coexist with a variety of symbiotic microorganisms that generally reside in the insect’s intestinal flora. The microbiome has profound effects on the physiology, immunity, fitness and survival of animals and plants. The interaction between triatomines and bacteria has been investigated to some extent and has revealed important bacteria symbionts. In contrast, the range of viral species that can infect triatomine insects is almost completely unknown. In some cases, genomic and metatranscriptomic approaches have uncovered sequences related to possible viral genomes, but, to date, only eight positive single-strand RNA viruses, namely Triatoma virus and Rhodnius prolixus viruses 1 - 7 have been investigated in more detail. Here, we review the literature available on triatomine viruses and the viruses-insect host relationship. The lack of broader metagenomic and metatranscriptomic studies in these medically relevant insects underscores the importance of expanding our knowledge of the triatomine virome both for surveillance purposes as well as to possibly harness their potential for insect vector population control strategies.
Hematophagous insect species belonging to the Triatominae (Reduviidae) sub-family are primary vectors of Trypanosoma cruzi, the etiologic agent of Chagas disease. This life-threatening parasitosis affects 6-7 million people in South and Central America (1) and is mainly transmitted by insect species belonging to three Triatomine genera: Rhodnius, Triatoma and Panstrongylus. However, more than 150 Triatomine species can support T. cruzi infections in laboratory settings or in the wild and therefore are potential vectors of the disease (2, 3). Triatomines are widely distributed in the Americas, from South Argentina to the South of USA causing concern in areas where Chagas disease is not endemic, but it is introduced by the increasing population mobility (2, 4, 5). Among triatomine species, Rhodnius prolixus has been extensively studied since the early 1900s proving an excellent model system to investigate several physiological and biochemical processes in insects (6–9). For instance, seminal studies by Sir V. B. Wigglesworth shed light on the hormonal control of oogenesis (10, 11) as well as the role of the juvenile hormone in metamorphosis (11). In recent years, the sequencing and partial assembly of Rhodnius genome has spun novel genetic and genomic studies, which have started to unveil the molecular mechanisms underlying triatomine development, reproduction, immunity and evolution (6, 12–18). Substantial progress has also been achieved in the characterization of the triatomine microbiome, where a special focus was placed on symbiotic bacteria. Triatomines are a reservoir of bacteria like Serratia marcescens, a Gram-negative bacteria that causes severe infections in humans and Rhodococcus rhodnii, the first symbiotic bacteria found in the triatomine gut (3, 19, 20). Because of space constraints, we refer the reader to excellent reviews on this topic as well as on the interaction between triatomines and T. cruzi (3, 13, 18, 21). Instead, here we focus on the virome of triatomine insects, a research area that is still in its infancy. Metagenomic approaches have helped uncover a wealth of viral species in arthropods especially in those of medical interest like the mosquito Aedes aegypti (22). Currently, 1,445 RNA viruses divided in more than 20 families clustered in 16 clades have been identified in insect tissues (23). Viral infections can prove harmless for the host or affect its survival and reproduction, while a few cases of beneficial virus-host interactions have been reported (24–27). Paradigmatic among the entomopathogenic class of viruses, the Slow Bee paralysis virus (SBPV) and related viral species (Deformed wing virus, Israeli acute paralysis virus, etc) are known to impair the development of bees (28), induce anterior leg paralysis (29) and malformations in wings and abdomen in the host (30, 31). SBPV is in fact responsible for the collapse of bee hives around the world causing large economic losses (32). Viruses however can represent beneficial symbionts in some cases (33). For instance, the plant virus Barley Yellow Dwarf Virus (BYDV) was shown to increase the fecundity and promote trophic facilitation of phytophagous vector species like the aphids Rhopalosiphum maidis and Rhopalosiphum padi (34). For the vast majority of the insect viruses however the characteristics of their interactions with the insect hosts are unknown. Despite the clinical relevance of some triatomine species, very little is known about their virome. Contigs related to viral genomes were independently identified in transcriptomic analyses of the salivary glands, fat bodies and testes of triatomine species like Rhodnius prolixus, Panstrongylus megistus and Panstrongylus lignarius (35–37). Viral sequences were also reported after sequencing the genome of Rhodnius prolixus (12). These observations however were not explored further and almost all that we know about triatomine viruses stems from the study of Triatoma virus (TrV) (38–40). TrV was discovered in a colony of field-collected Triatoma infestans (38, 40). Only recently, our group identified and characterized 7 novel viruses, namely Rhodnius prolixus virus 1-7 (RpV1-7), while investigating stage-specific de novo transcriptome assemblies in ovarian tissues of Rhodnius prolixus (16, 17). This review aims to describe the (limited) information available on triatomine viruses and underscore the importance of investing in this neglected area of scientific research.
Triatoma virus (TrV) is a non-enveloped virus that displays a positive single strand RNA (+) ssRNA) genome and was first identified in the abdominal contents of infected T. infestans as small spherical particles about 30 nm in diameter (38). Due to its unique capsid structure, TrV originated a new genus within the Dicistroviridae family, namely the Triatovirus genus (PDB ID: 3NAP and 1B35, respectively) (41–43) (Figure 1). The TrV genome is 9010 nt and harbors two open reading frames (ORFs) (44). ORF1 (549-5936 nt) encodes motifs characteristic of viral RNA-dependent RNA polymerases (RdRp), cysteine proteases and RNA helicases, while ORF2 encodes capsid proteins (Figure 1). The two ORFs are separated by 172 nt of non-coding RNA (44). Two putative internal ribosomal entry sites (IRESs) in the TrV genome were identified: the 5’UTR IRES of 548 nt and the intergenic region (IGR) IRES of 172 nt (45). The TrV capsid displays icosahedral symmetry with pseudo-triangulation number P = 3 and is formed by 60 protomers comprising the three major VP1, VP2 and VP3 and one minor (VP0) structural polypeptides (40, 41, 46, 47). The capsid shows spikes forming a crown commonly present in members of the Dicistroviridae family (48). The exposed amino acid residues in those projections were proposed to exert a role in the interaction with the host (47). Infections by TrV were shown to induce 97.6% mortality rates in blood-fed fifth instar nymphs, delay the molting process (39, 49) and impair oogenesis (49). In accordance, the oviposition rate in adult T. infestans females infected with TrV drops by ~80% compared to uninfected females (49). Fifteen triatomine species have been shown to support TrV infections in laboratory settings, where the virus was inoculated via intrahemocelic injection or artificial ingestion of purified viral particles (50). In an artificial TrV infection experiment, fecal suspensions parenterally inoculated into uninfected triatomines killed all insects (n= 515) within 36 h (51), whereas viral particles could be detected at fecal samples from 5th instar Triatoma infestans fed in an artificial feeder with TrV-contaminated human blood (52). T. infestans also becomes infected with TrV when feeding on contaminated chickens (51). Some triatomines infected with TrV were collected in the field. For instance, Triatoma sordida from the Chaco province (53), Psammolestes coreodes in nests in a region endemic for Chagas disease (54) and Triatoma breyeri in sylvatic habitats mainly in the arid Chaco and Monte ecoregions in Argentina (50). Field-collected Triatoma patagonica (55), Rhodnius neglectus, an autochthonous Brazilian species, and Meccus longipennis could also be artificially infected by TrV (56). However, only 10% of Triatoma infestans from sylvatic populations from Argentine were found to harbor TrV infections (39). The discrepancy between the infection rates in wild-caught and colony-raised insects mostly likely lies on the variety of viral transmission routes like cannibalism, coprophagy and transovarial transmissions and their specific contribution to the spread of the virus in the population (39). Rearing conditions in the laboratory are particularly favorable for fecal-oral transmission, because insects are artificially overcrowded during feeding (51). The presence of a pheromone in feces freshly deposited by nymphs (57) attracts unfed nymphs while arresting the locomotion of fed ones. Hence, this phenomenon might also favor the spread of the TrV in the insectaries (58).
Figure 1 Phylogenetic analysis and genome structure of the triatomine viruses. (A) Phylogenetic tree constructed from RdRp sequences of the (+) ssRNA viruses, TrV and RpV 1-7. Neighbor-Joining method with 1000 bootstrap replicates was used in the phylogenetic analysis. Bootstrap values are displayed in tree branches. Triatomine viruses belong to four different families: Dicistroviridae, Iflaviridae, Permutotetraviridae and Solemoviridae. (B) Genome organization of the TrV and RpVs displaying their structural and non-structural domains. CRPV_capsid, Cricket Paralysis Virus capsid protein, RdRp, RNA-dependent RNA Polymerase, RNA helicase, rhv-like, Picornavirus/Calicivirus coat protein, Peptidase domain, RT_like, Reverse Transcriptase_like. Adapted from Brito, et al. (16).
Unlike some arboviruses that are capable of infecting both invertebrates and vertebrates like Dengue Virus and Zika Virus among others, Insect-Specific Viruses (ISVs) display a host range restricted to insects (59). TrV inoculation in different insect cell lines such as Sf9, Sf21 (from Spodoptera frugiperda), High Five (H5) and C6/36 (Aedes albopictus) resulted in signs of infection or cytopathic effect (CPE) after 24-48 h (60). However, this phenotype disappeared after successive passages. These results were confirmed with three independent approaches: transfection, direct inoculation of the purified viral particles or inoculation of the purified viral particles with trypsin (61). Thus, TrV appears to be a triatomine-specific entomopathogenic virus. This characteristic led to propose TrV as a possible biological tool for the control of Chagas disease Triatomine vector populations (39, 40).
Recently, our group expanded the variety of viral species in triatomines by describing 7 unique viral genomes in Rhodnius prolixus (16). The RpVs are (+) single strand RNA viruses classified in three different families: Iflaviridae (RpV1 and RpV2), Permutotetraviridae (RpV3, RpV4 and RpV7) and Solemoviridae (RpV5 and RpV6) (16) and do not share any similarity with TrV (Figure 1A and Table 1).
RpV1 has the longest contig found (9.6 Kilobases) and it exhibits two main ORFs with amino acid sequence identity 36.99% and 40.88% with the proteins encoded by ORF1 and ORF2 of the Nesidiocoris tenuis virus 1 (NtV-1), respectively (16). NtV-1 has been classified in the Iflavirus genus, even though its genome revealed two different ORFs (62). ORF1 of RpV1 encodes putative structural capsid proteins related to Cricket Paralysis virus (CrPV) and Picornavirales order specific proteins such as RhV-like (Figure 1B). ORF2 instead encodes putative non-structural proteins such as the RdRp enzyme and a RNA helicase (16). ORF1 and ORF2 of RpV1 display a 1-nt overlap typical of the Iflavirus genus, which results in a -1nt frame-shift (Figure 1B). The RpV2 genome (contig ~8.9Kb) encodes a single polyprotein (2,890 amino acids) and it also seems to belong to the Iflavirus genus like RpV1 (16). The putative polyprotein of this virus shares ~70% amino acid sequence identity with the polyprotein of the Slow Bee Paralysis Virus (SBPV). For both viruses, the RhV-like and the CrPV structural capsid proteins are located at the N-terminal region, while the RdRp enzyme is at the C-terminal (16, 63). SBPV can be transmitted to Apis mellifera adult bees and pupae by the mite Varroa destructor (64). SBPV infections induce paralysis of the anterior legs and high mortality rates (29). Additionally, the bees that survived from one season to the next and were infected by Deformed Wing Virus (DWV) or SBPV showed a decrease in the infection rate by mite, suggesting equilibrium between entomopathogenic viruses and the mite (65). The large mite population can promote a starvation condition for the honeybee favoring condition-dependent SBPV virulence which was demonstrated in artificial infection assays in the Bombus terrestris pollinator (26). Since we first detected it in our ovarian RNA-seq datasets produced in 2014, RpV2 seems to have been lost to our colony (16). It is tempting to speculate that the pathogenicity of this virus might have eliminated the infected animals, eventually causing its disappearance from the colony. We cannot rule out however that RpV2 might still be circulating in the insectary, but at threshold viral levels below the sensitivity of our assays.
RpV3, RpV4 and RpV7 belong to Alphapermutotetravirus genus, Permutotetraviridae family and they exhibit a genome around 5Kb long. The putative RdRp enzymes of RpV3, RpV4 and RpV7 share ~42% to ~47% amino acid sequence identity with proteins from Shuangao and Hubei permutotetra-like viruses (16). Only two Alphapermutotetravirus are classified by Taxonomy in the International Committee on Taxonomy of Viruses (ICTV): Euprosterna elaeasa virus and Thosea asigna virus. In a large assay analyzing invertebrate RNA viruses from several metatranscriptome libraries, thirteen Hubei permutotetra-like viruses and one Shuangao permutotetra-like virus were found in different arthropods (23). The host range of Hubei permutotetra-like viruses seems to be broad, including different host species like Coleoptera sp., Aedes sp., Culex quinquefasciatus, Abraxas tenuisuffusa, Anopheles sinensis, Aedes albopictus, Aedes aegypti, Paracercion melanotum, Gryllidae sp., Neoscona nautica, Scutigeridae sp. and Spirurida sp1 (23, 66).
RpV5 and RpV6 (contigs ~2.8Kb for both) belong to the Sobemovirus genus and their encoded proteins share amino acid sequence identity ranging from 41% to 66%, respectively, when they were compared with Atrato sobemo-like virus. RpV5 and RpV6 also harbor two ORFs: ORF1 encodes Peptidase and ORF2 the RdRp enzyme (16) (Figure 1B). The Sobemovirus genus comprises some plant viruses whose natural host range is relatively narrow. Several sobemoviruses like the Rice Yellow Mottle Virus (RYMV) are of special interest as they infect crop plantations causing large economic losses (67). The main transmission route of sobemoviruses is through host plant wounds that can be caused by insects such as the beetle Ceratoma trifurcota (67, 68). Although sobemoviruses can infect invertebrates as beetles, aphids, mirid bugs, moths, grasshoppers and sucking bugs, the interaction of viruses with invertebrates has been poorly explored. It was recently shown that R. prolixus can feed on artificial sugar meals or even cherry tomatoes in laboratory settings, even though triatomines have been considered strictly hematophagous for over a century (69, 70). It is therefore possible that RpV5 and RpV6 infected the insects that originated our colony while they were feeding on the phloem of some plant.
Both RpVs and TrV are vertically transmitted to progeny, which likely contributes to establish persistent infections (16, 39). Vertical transmission is of special interest as it has been linked to the emergence of seasonal pandemics of some arboviroses like Dengue and Zika (71, 72). Furthermore, DWV and other viruses that infect honeybees were found in the queen’s ovaries and transmitted to the offspring including eggs and young larvae (24). Additional transmission routes have been demonstrated for TrV, but they remain still unexplored for the RpVs. For these viruses, also the host range restriction needs to be addressed in the future.
Because only one virus was known until recently, the investigation of antiviral systems in triatomines is also lagging behind. It has been suggested that haemocytes, phenoloxidase and nitric oxide might provide defense mechanisms against viral infections in these insect species (18), but this hypothesis has not been tested yet. However, the discovery of viral small interfering RNAs against the RpVs and the evolutionary conservation of key RNAi players in Rhodnius prolixus ovaries demonstrates that a RNAi-based antiviral system is active in triatomines and might keep the viral load below lethal levels (15–17). It will be interesting to explore these mechanisms especially in embryos, where the viral titers appear to be several orders of magnitudes lower than in other stages of Rhodnius prolixus early development. Recently, another class of small non-coding RNAs, namely the Piwi-interacting RNAs or piRNAs, was implicated in antiviral activity in Aedes mosquitoes (73, 74). Viral piRNAs can originate from viral sequences, known as Endogenous Viral Elements (EVEs), harbored in the genome of the insect (73). However, we did not identify either piRNAs or EVEs related to the RpVs in R. prolixus ovary (16). In the absence of similar studies in other Triatomines, it is therefore likely that the piRNA pathway does not contribute to antiviral defenses in ovarian tissues of Triatomine insects.
Triatomine insect species are of public health importance since they can vector the protozoan T. cruzi, the etiologic agent of Chagas Disease. Surprisingly, the characterization of the virome in these insects is still in the embryonic phase mostly due to the lack of broad unbiased metagenomic and metatranscriptomic studies. The metatranscriptomic studies from different Triatomine species including field-captured bugs together with functional assays for viral pathogenicity are some of the strategies which might be used to better understand the Triatomine virome diversity. This goal is of paramount importance and might be achieved by including the analysis of viral small interfering RNAs (vsiRNAs), which not only provide valuable information on the antiviral systems acting in the insect, but can also be employed for the assembly of the genome of new viruses (16, 75). If some triatomines are capable of transmitting T. cruzi to vertebrates and humans, we cannot rule out that they might also be competent vectors capable of transmitting pathogenic viruses (3). This hypothesis receives some support by the observation that most of the human sera samples from patients with Chagas disease in the Argentine, Bolivia and Mexico were positive for anti-TrV antibodies (76). The vectorial competence of triatomines has been tested with HIV and HBV, which were not able to replicate in the insect tissues or be transmitted to mammalian hosts (77, 78). To our knowledge however the interaction between triatomines and arboviruses like Dengue, Zika and Chikungynya viruses has not been tested yet. Conversely, some triatomine ISVs might be harnessed as biological tools for the control of insects of economic and medical relevance. When applied to triatomines, these strategies might lead to the control of triatomine vector populations in the wild and therefore, the reduction of Chagas disease diffusion. Finally, TrV Viral-Like Particles (TrV-VLPs) have been proposed to act as a vaccine adjuvant by enhancing the humoral immune response against T. cruzi chimeric antigens (79). A thorough characterization of the triatomine virome, its interactions with the insect host and host range restriction are therefore a challenge of paramount importance for the next future.
Writing – original draft: MC, TB, and AP. Writing – review & editing: MC, TB, IB, MB, VC, and AP. All authors contributed to the article and approved the submitted version.
This work was supported by the National Counsel of Technological and Scientific Development (CNPq) (428100/2018-0), the Research Support Foundation of the State of Rio de Janeiro (FAPERJ) E-26/210.912/2019, E-26/010.002720/2019 and E-26/010.001877/2015, Wellcome Trust grant 207486Z17Z, CAPES and INCT/Enem fundings.
The authors declare that the research was conducted in the absence of any commercial or financial relationships that could be construed as a potential conflict of interest.
All claims expressed in this article are solely those of the authors and do not necessarily represent those of their affiliated organizations, or those of the publisher, the editors and the reviewers. Any product that may be evaluated in this article, or claim that may be made by its manufacturer, is not guaranteed or endorsed by the publisher.
1. Chagas Disease (Also Known as American Trypanosomiasis). Available at: https://www.who.int/news-room/fact-sheets/detail/chagas-disease-(american-trypanosomiasis).
2. Coura JR, Viñas PA. Chagas Disease: A New Worldwide Challenge. Nature (2010) 465(7301):S6–7. doi: 10.1038/nature09221
3. Vieira CB, Praça YR, Bentes KL da S, Santiago PB, Silva SMM, Silva GDS, et al. Triatomines: Trypanosomatids, Bacteria, and Viruses Potential Vectors? Front Cell Infect Microbiol (2018) 8:405. doi: 10.3389/fcimb.2018.00405
4. Hernández L, Abrahan L, Moreno M, Gorla D, Catalá S. Phenotypic Variability Associated to Genomic Changes in the Main Vector of Chagas Disease in the Southern Cone of South America. Acta Trop (2008) 106(1):60–7. doi: 10.1016/j.actatropica.2008.01.006
5. de la Vega GJ, Medone P, Ceccarelli S, Rabinovich J, Schilman PE. Geographical Distribution, Climatic Variability and Thermo-Tolerance of Chagas Disease Vectors. Ecography (2015) 38:851–60. doi: 10.1111/ecog.01028
6. Nunes-da-Fonseca R, Berni M, Tobias-Santos V, Pane A, Araujo HM. Rhodnius Prolixus: From Classical Physiology to Modern Developmental Biology. Genesis (2017) 55(5):1–11. doi: 10.1002/dvg.22995
7. Henriques BS, Gomes B, Oliveira PL, de Garcia ES, Azambuja P, Genta FA. Characterization of the Temporal Pattern of Blood Protein Digestion in : First Description of Early and Late Gut Cathepsins. Front Physiol (2020) 11:509310. doi: 10.3389/fphys.2020.509310
8. Gandara ACP, Dias FA, de Lemos PC, Stiebler R, Bombaça ACS, Menna-Barreto R, et al. Urate and NOX5 Control Blood Digestion in the Hematophagous Insect Rhodnius Prolixus. Front Physiol (2021) 12:org/10.3389/fphys.2021.633093. doi: 10.3389/fphys.2021.633093
9. Sterkel M, Ons S, Oliveira PL. DOPA Decarboxylase is Essential for Cuticle Tanning in Rhodnius Prolixus (Hemiptera: Reduviidae), Affecting Ecdysis, Survival and Reproduction. Insect Biochem Mol Biol (2019) 108:24–31. doi: 10.1016/j.ibmb.2019.03.006
10. Wigglesworth VB. Memoirs: The Function of the Corpus Allatum in the Growth and Reproduction of Rhodnius Prolixus (Hemiptera). J Cell Sci (1936) s2-79:91–121. doi: 10.1242/jcs.s2-79.313.91
11. Wigglesworth VB. The Determination of Characters at Metamorphosis in Rhodnius Prolixus (Hemiptera). J Exp Biol (1940) 17:201–23. doi: 10.1242/jeb.17.2.201
12. Mesquita RD, Vionette-Amaral RJ, Lowenberger C, Rivera-Pomar R, Monteiro FA, Minx P, et al. Genome of Rhodnius Prolixus, an Insect Vector of Chagas Disease, Reveals Unique Adaptations to Hematophagy and Parasite Infection. Proc Natl Acad Sci USA (2015) 112(48):14936–41. doi: 10.1073/pnas.1506226112
13. Carmona-Peña SP, Contreras-Garduño J, Castro DP, Manjarrez J, Vázquez-Chagoyán JC. The Innate Immune Response of Triatomines Against Trypanosoma Cruzi and Trypanosoma Rangeli With an Unresolved Question: Do Triatomines Have Immune Memory? Acta Trop (2021) 224:106108. doi: 10.1016/j.actatropica.2021.106108
14. Berni M, Fontenele MR, Tobias-Santos V, Caceres-Rodrigues A, Mury FB, Vionette-do-Amaral R, et al. Toll Signals Regulate Dorsal-Ventral Patterning and Anterior-Posterior Placement of the Embryo in the Hemipteran Rhodnius Prolixus. Evodevo (2014) 5:38. doi: 10.1186/2041-9139-5-38
15. Brito T, Julio A, Berni M, de Castro Poncio L, Bernardes ES, Araujo H, et al. Transcriptomic and Functional Analyses of the piRNA Pathway in the Chagas Disease Vector Rhodnius Prolixus. PloS Negl Trop Dis (2018) 12(10):e0006760. doi: 10.1371/journal.pntd.0006760
16. de Brito TF, Coelho VL, Cardoso MA, de A Brito IA, Berni MA, Zenk FL, et al. Transovarial Transmission of a Core Virome in the Chagas Disease Vector Rhodnius Prolixus. PloS Pathog (2021) 17(8):e1009780. doi: 10.1371/journal.ppat.1009780
17. Coelho VL, de Brito TF, de Abreu Brito IA, Cardoso MA, Berni MA, Araujo HMM, et al. Analysis of Ovarian Transcriptomes Reveals Thousands of Novel Genes in the Insect Vector Rhodnius Prolixus. Sci Rep (2021) 11(1):1918. doi: 10.1038/s41598-021-81387-1
18. Flores-Villegas AL, Salazar-Schettino PM, Córdoba-Aguilar A, Gutiérrez-Cabrera AE, Rojas-Wastavino GE, Bucio-Torres MI, et al. Immune Defence Mechanisms of Triatomines Against Bacteria, Viruses, Fungi and Parasites. Bull Entomol Res (2015) 105(5):523–32. doi: 10.1017/S0007485315000504
19. Azambuja P, Feder D, Garcia ES. Isolation of Serratia Marcescens in the Midgut of Rhodnius Prolixus: Impact on the Establishment of the Parasite Trypanosoma Cruzi in the Vector. Exp Parasitol (2004) 107(1-2):89–96. doi: 10.1016/j.exppara.2004.04.007
20. Erikson D. The Pathogenic Aerobic Organisms of the Actinomyces Group. London: H. M. Stat. Off. (1935). p. 61.
21. Garcia ES, Castro DP, Figueiredo MB, Azambuja P. Immune Homeostasis to Microorganisms in the Guts of Triatomines (Reduviidae): A Review. Mem Inst Oswaldo Cruz (2010) 105:605–10. doi: 10.1590/s0074-02762010000500001
22. Parry R, James ME, Asgari S. Uncovering the Worldwide Diversity and Evolution of the Virome of the Mosquitoes Aedes Aegypti and Aedes Albopictus. Microorganisms (2021) 9:1653. doi: 10.3390/microorganisms9081653
23. Shi M, Lin X-D, Tian J-H, Chen L-J, Chen X, Li C-X, et al. Redefining the Invertebrate RNA Virosphere. Nature (2016) 540(7634):539–43. doi: 10.1038/nature20167
24. Chen YP, Pettis JS, Collins A, Feldlaufer MF. Prevalence and Transmission of Honeybee Viruses. Appl Environ Microbiol (2006) 72(1):606–11. doi: 10.1128/AEM.72.1.606-611.2006
25. Fullaondo A, Lee SY. Regulation of Drosophila-Virus Interaction. Dev Comp Immunol (2012) 36(2):262–6. doi: 10.1016/j.dci.2011.08.007
26. Manley R, Boots M, Wilfert L. Condition-Dependent Virulence of Slow Bee Paralysis Virus in Bombus Terrestris: Are the Impacts of Honeybee Viruses in Wild Pollinators Underestimated? Oecologia (2017) 184(2):305–15. doi: 10.1007/s00442-017-3851-2
27. Bonning BC, Allen Miller W. Dicistroviruses. Annu Rev Entomol (2010) 55:129–50. doi: 10.1146/annurev-ento-112408-085457
28. Bowen-Walker PL, Martin SJ, Gunn A. The Transmission of Deformed Wing Virus Between Honeybees (Apis Mellifera L.) by the Ectoparasitic Mite Varroa Jacobsoni Oud. J Invertebr Pathol (1999) 73(1):101–6. doi: 10.1006/jipa.1998.4807
29. Bailey L, Woods RD. Three Previously Undescribed Viruses From the Honey Bee. J Gen Virol (1974) 25(2):175–86. doi: 10.1099/0022-1317-25-2-175
30. Jong DD, De Jong D, Morse RA, Eickwort GC. MITE PESTS OF HONEY BEES. Annu Rev Entomol (1982) 27:229–52. doi: 10.1146/annurev.en.27.010182.001305
31. Schatton-Gadelmayer K, Engels W. Hemolymph Proteins and Body Weight in Newly Emerged Worker Honey Bees According to Different Rates of Parasitation by Brood Mites (Hymenoptera: Apidae: Apis Mellifera / Acarina: Varroidae: Varroa Jacobsoni. Entomol Gen (1988) 14:93–101. doi: 10.1127/entom.gen/14/1988/093
32. Epa US, OCSPP. Colony Collapse Disorder (2013). Available at: https://www.epa.gov/pollinator-protection/colony-collapse-disorder.
33. Roossinck MJ. The Good Viruses: Viral Mutualistic Symbioses. Nat Rev Microbiol (2011) 9(2):99–108. doi: 10.1038/nrmicro2491
34. Porras M, De Moraes CM, Mescher MC, Rajotte EG, Carlo TA. A Plant Virus (BYDV) Promotes Trophic Facilitation in Aphids on Wheat. Sci Rep (2018) 8(1):11709. doi: 10.1038/s41598-018-30023-6
35. Schwarz A, Medrano-Mercado N, Schaub GA, Struchiner CJ, Bargues MD, Levy MZ, et al. An Updated Insight Into the Sialotranscriptome of Triatoma Infestans: Developmental Stage and Geographic Variations. PloS Negl Trop Dis (2014) 8(12):e3372. doi: 10.1371/journal.pntd.0003372
36. Ribeiro JMC, Schwarz A, Francischetti IMB. A Deep Insight Into the Sialotranscriptome of the Chagas Disease Vector, Panstrongylus Megistus (Hemiptera: Heteroptera). J Med Entomol (2015) 52(3):351–8. doi: 10.1093/jme/tjv023
37. Nevoa JC, Mendes MT, da Silva MV, Soares SC, Oliveira CJF, Ribeiro JMC. An Insight Into the Salivary Gland and Fat Body Transcriptome of Panstrongylus Lignarius (Hemiptera: Heteroptera), the Main Vector of Chagas Disease in Peru. PloS Negl Trop Dis (2018) 12(2):e0006243. doi: 10.1371/journal.pntd.0006243
38. Muscio OA, LaTorre JL, Scodeller EA. Small Nonoccluded Viruses From Triatomine Bug Triatoma Infestans (Hemiptera: Reduviidae). J Invertebr Pathol (1987) 49(2):218–20. doi: 10.1016/0022-2011(87)90163-7
39. Muscio OA, La Torre JL, Bonder MA, Scodeller EA. Triatoma Virus Pathogenicity in Laboratory Colonies of Triatoma Infestans (Hemiptera: Reduviidae). J Med Entomol (1997) 34:253–6. doi: 10.1093/jmedent/34.3.253
40. Muscio OA, La Torre JL, Scodeller EA. Characterization of Triatoma Virus, a Picorna-Like Virus Isolated From the Triatomine Bug Triatoma Infestans. J Gen Virol (1988) 69(Pt 11):2929–34. doi: 10.1099/0022-1317-69-11-2929
41. Agirre J, Aloria K, Arizmendi JM, Iloro I, Elortza F, Sánchez-Eugenia R, et al. Capsid Protein Identification and Analysis of Mature Triatoma Virus (TrV) Virions and Naturally Occurring Empty Particles. Virology (2011) 409(1):91–101. doi: 10.1016/j.virol.2010.09.034
42. Genus: Triatovirus - Dicistroviridae - Positive-Sense RNA Viruses. ICTV. (2021). Available at: https://talk.ictvonline.org/ictv-reports/ictv_online_report/positive-sense-rna-viruses/w/dicistroviridae/561/genus-triatovirus.
43. Valles SM, Chen Y, Firth AE, Guérin DMA, Hashimoto Y, Herrero S, et al. ICTV Virus Taxonomy Profile: Dicistroviridae. J Gen Virol (2017) 98(3):355–6. doi: 10.1099/jgv.0.000756
44. Czibener C, La Torre JL, Muscio OA, Ugalde RA, Scodeller EA. Nucleotide Sequence Analysis of Triatoma Virus Shows That It Is a Member of a Novel Group of Insect RNA Viruses. J Gen Virol (2000) 81(Pt 4):1149–54. doi: 10.1099/0022-1317-81-4-1149
45. Czibener C, Alvarez D, Scodeller E, Gamarnik AV. Characterization of Internal Ribosomal Entry Sites of Triatoma Virus. J Gen Virol (2005) 86(Pt 8):2275–80. doi: 10.1099/vir.0.80842-0
46. Rozas-Dennis GS, Squires G, Pous J, Costabel MD, Lepault J, Navaza J, et al. Purification, Crystallization and Preliminary X-Ray Analysis of Triatoma Virus (TrV) From Triatoma Infestans. Acta Crystallogr D Biol Crystallogr (2004) 60(Pt 9):1647–50. doi: 10.1107/S0907444904015185
47. Squires G, Pous J, Agirre J, Rozas-Dennis GS, Costabel MD, Marti GA, et al. Structure of the Triatoma Virus Capsid. Acta Crystallogr D Biol Crystallogr (2013) 69(Pt 6):1026–37. doi: 10.1107/S0907444913004617
48. Estrozi LF, Neumann E, Squires G, Rozas-Dennis G, Costabel M, Rey FA, et al. Phasing of the Triatoma Virus Diffraction Data Using a Cryo-Electron Microscopy Reconstruction. Virology (2008) 375(1):85–93. doi: 10.1016/j.virol.2007.12.025
49. Rozas-Dennis GS, Cazzaniga NJ. Effects of Triatoma Virus (TrV) on the Fecundity and Moulting of Triatoma Infestans (Hemiptera: Reduviidae). Ann Trop Med Parasitol (2000) 94(6):633–41. doi: 10.1080/00034983.2000.11813586
50. Ceccarelli S, Balsalobre A, Susevich ML, Echeverria MG, Gorla DE, Marti GA. Modelling the Potential Geographic Distribution of Triatomines Infected by Triatoma Virus in the Southern Cone of South America. Parasit Vectors (2015) 8:153. doi: 10.1186/s13071-015-0761-1
51. Muscio O, Bonder MA, La Torre JL, Scodeller EA. Horizontal Transmission of Triatoma Virus Through the Fecal-Oral Route in Triatoma Infestans (Hemiptera: Triatomidae). J Med Entomol (2000) 37(2):271–5. doi: 10.1603/0022-2585-37.2.271
52. Marti GA, Balsalobre A, Susevich ML, Rabinovich JE, Echeverría MG. Detection of Triatomine Infection by Triatoma Virus and Horizontal Transmission: Protecting Insectaries and Prospects for Biological Control. J Invertebr Pathol (2015) 124:57–60. doi: 10.1016/j.jip.2014.10.008
53. Marti GA, Echeverria MG, Susevich ML, Becnel JJ, Pelizza SA, García JJ. Prevalence and Distribution of Parasites and Pathogens of Triatominae From Argentina, With Emphasis on Triatoma Infestans and Triatoma Virus TrV. J Invertebr Pathol (2009) 102(3):233–7. doi: 10.1016/j.jip.2009.06.010
54. Susevich ML, Marti GA, Serena MS, Echeverría MG. New Triatoma Virus Hosts in Wild Habitats of Argentina. J Invertebr Pathol (2012) 110(3):405–7. doi: 10.1016/j.jip.2012.03.023
55. Rozas-Dennis GS, Cazzaniga NJ, Guerin DMA. Triatoma Patagonica (Hemiptera, Reduviidae), a New Host for Triatoma Virus. Mem Inst Oswaldo Cruz (2002) 97(3):427–9. doi: 10.1590/S0074-02762002000300028
56. Marti GA, Echeverría MG, Susevich ML, Ceccarelli S, Balsalobre A, Rabinovich JE, et al. Exploration for Triatoma Virus (TrV) Infection in Laboratory-Reared Triatomines of Latin America: A Collaborative Study*. Int J Trop Insect Sci (2013) 33:294–304. doi: 10.1017/s1742758413000337
57. Schofield CJ, Patterson JW. Assembly Pheromone of Triatoma Infestans and Rhodnius Prolixus Nymphs (Hemiptera: Reduviidae). J Med Entomol (1977) 13(6):727–34. doi: 10.1093/jmedent/13.6.727
58. Schofield CJ. The Behaviour of Triatominae (Hemiptera: Reduviidae): A Review. Bull Entomol Res (1979) 69:363–79. doi: 10.1017/s0007485300018897
59. Roundy CM, Azar SR, Rossi SL, Weaver SC, Vasilakis N. Insect-Specific Viruses: A Historical Overview and Recent Developments. Adv Virus Res (2017) 98:119–46. doi: 10.1016/bs.aivir.2016.10.001
60. Susevich ML, Marti GA, Echeverría MG. First Description of Hemagglutination by a Virus Belonging to the Family Dicistroviridae. Arch Virol (2014) 159(3):581–4. doi: 10.1007/s00705-013-1865-6
61. Susevich ML, Marti GA, Metz GE, Echeverría MG. First Study of Different Insect Cells to Triatoma Virus Infection. Curr Microbiol (2015) 70(4):470–5. doi: 10.1007/s00284-014-0746-x
62. Dong Y, Chao J, Liu J, Rice A, Holdbrook R, Liu Y, et al. Characterization of a Novel RNA Virus From Nesidiocoris Tenuis Related to Members of the Genus Iflavirus. Arch Virol (2018) 163(2):571–4. doi: 10.1007/s00705-017-3622-8
63. de Miranda JR, Dainat B, Locke B, Cordoni G, Berthoud H, Gauthier L, et al. Genetic Characterization of Slow Bee Paralysis Virus of the Honeybee (Apis Mellifera L.). J Gen Virol (2010) 91(Pt 10):2524–30. doi: 10.1099/vir.0.022434-0
64. Santillán-Galicia MT, Teresa Santillán-Galicia M, Ball BV, Clark SJ, Alderson PG. Transmission of Deformed Wing Virus and Slow Paralysis Virus to Adult Bees (Apis melliferaL.) Byvarroa Destructor. J Apic Res (2010) 49:141–8. doi: 10.3896/ibra.1.49.2.01
65. Carreck NL, Ball BV, Martin SJ. Honey Bee Colony Collapse and Changes in Viral Prevalence Associated With Varroa Destructor. J Apic Res (2010) 49:93–4. doi: 10.3896/ibra.1.49.1.13
66. Ribeiro G de O, de Oliveira Ribeiro G, Morais VS, Monteiro FJC, Ribeiro ESD, da S Rego MO, et al. Aedes Aegypti From Amazon Basin Harbor High Diversity of Novel Viral Species. Viruses (2020) 12:866. doi: 10.3390/v12080866
67. Sõmera M, Sarmiento C, Truve E. Overview on Sobemoviruses and a Proposal for the Creation of the Family Sobemoviridae. Viruses (2015) 7:3076–115. doi: 10.3390/v7062761
68. Walters HJ. Bean Leaf Beetle as a Vector of the Cowpea Strain of Southern Bean Mosaic Virus. Phytopathology (1970) 60:177. doi: 10.1094/phyto-60-177
69. Díaz-Albiter HM, Ferreira TN, Costa SG, Rivas GB, Gumiel M, Cavalcante DR, et al. Everybody Loves Sugar: First Report of Plant Feeding in Triatomines. Parasit Vectors (2016) 9:114. doi: 10.1186/s13071-016-1401-0
70. Jurberg J, Galvão C, Weirauch C, Moreira FFF. Hematophagous Bugs (Reduviidae, Triatominae). In: True Bugs (Heteroptera) of the Neotropics. Dordrecht: Springer Netherlands (2015). p. 353–93.
71. Ferreira-de-Lima VH, Lima-Camara TN. Natural Vertical Transmission of Dengue Virus in Aedes Aegypti and Aedes Albopictus: A Systematic Review. Parasit Vectors (2018) 11(1):77. doi: 10.1186/s13071-018-2643-9
72. Comeau G, Zinna RA, Scott T, Ernst K, Walker K, Carrière Y, et al. Vertical Transmission of Zika Virus in Produces Potentially Infectious Progeny. Am J Trop Med Hyg (2020) 103(2):876–83. doi: 10.4269/ajtmh.19-0698
73. Tassetto M, Kunitomi M, Whitfield ZJ, Dolan PT, Sánchez-Vargas I, Garcia-Knight M, et al. Control of RNA Viruses in Mosquito Cells Through the Acquisition of vDNA and Endogenous Viral Elements. Elife (2019) 8:1–29. doi: 10.7554/eLife.41244
74. Suzuki Y, Baidaliuk A, Miesen P, Frangeul L, Crist AB, Merkling SH, et al. Non-Retroviral Endogenous Viral Element Limits Cognate Virus Replication in Aedes Aegypti Ovaries. Curr Biol (2020) 30(18):3495–506.e6. doi: 10.1016/j.cub.2020.06.057
75. Olmo RP, Martins NE, Aguiar ERGR, Marques JT, Imler J-L. The Insect Reservoir of Biodiversity for Viruses and for Antiviral Mechanisms. Acad Bras Cienc (2019) 91(Suppl 3):e20190122. doi: 10.1590/0001-3765201920190122
76. Querido JFB, Echeverría MG, Marti GA, Costa RM, Susevich ML, Rabinovich JE, et al. Seroprevalence of Triatoma Virus (Dicistroviridae: Cripaviridae) Antibodies in Chagas Disease Patients. Parasit Vectors (2015) 8:29. doi: 10.1186/s13071-015-0632-9
77. Nuzzo SF, Neto VA, Braz LMA, Silva MFS, de Oliveira ML, Castilho MAL, et al. Evaluation of Presence of Protein 24 From HIV in Feces of Triatoma Infestans Fed Blood From HIV Positive Patients. Rev Saúde Pública (1998) 32(5):464–6. doi: 10.1590/S0034-89101998000500009
78. Granato CF, de Mendonça JS, Pinto PL, Amato Neto V, Veronesi R, Tolezano E. Papel De Triatomíneos Na Transmissão De Infecção Pelo Vírus Da Hepatite Tipo B, Em Diferentes Formas Clínicas Da Doença [Role of Triatomidae in the Transmission of Infection by Hepatitis Virus Type B, in Different Clinical Forms of the Disease]. Rev Hosp Clin Fac Med São Paulo (1987) 42(4):173–5.
Keywords: Triatomine, virus, microbiome, metatranscriptome, Chagas disease
Citation: Cardoso MA, Brito TF, Brito IAdA, Berni MA, Coelho VL and Pane A (2022) The Neglected Virome of Triatomine Insects. Front. Trop. Dis 3:828712. doi: 10.3389/fitd.2022.828712
Received: 03 December 2021; Accepted: 13 January 2022;
Published: 18 February 2022.
Edited by:
Ana Beatriz Barletta Ferreira, National Institutes of Health Clinical Center (NIH), United StatesReviewed by:
Carla Nunes De Araújo, University of Brasilia, BrazilCopyright © 2022 Cardoso, Brito, Brito, Berni, Coelho and Pane. This is an open-access article distributed under the terms of the Creative Commons Attribution License (CC BY). The use, distribution or reproduction in other forums is permitted, provided the original author(s) and the copyright owner(s) are credited and that the original publication in this journal is cited, in accordance with accepted academic practice. No use, distribution or reproduction is permitted which does not comply with these terms.
*Correspondence: Attilio Pane, YXBhbmVAaWNiLnVmcmouYnI=
Disclaimer: All claims expressed in this article are solely those of the authors and do not necessarily represent those of their affiliated organizations, or those of the publisher, the editors and the reviewers. Any product that may be evaluated in this article or claim that may be made by its manufacturer is not guaranteed or endorsed by the publisher.
Research integrity at Frontiers
Learn more about the work of our research integrity team to safeguard the quality of each article we publish.