- Department of Immunology, Duke University School of Medicine, Durham, NC, United States
Naegleria fowleri, or the “brain-eating amoeba,” is responsible for a rare, but lethal, infection known as primary amoebic meningoencephalitis (PAM). Confirmed PAM cases have seen both a rise in numbers, as well as expansion of geographic range over the past several decades. There is no effective therapy for PAM and the clinical prognosis remains grim with a mortality rate over 95%. The role of the immune response in disease prevention and disease severity remains unclear. In this review, we explore potential roles of inflammatory immune responses to N. fowleri in disease pathogenesis with a primary focus on pro-inflammatory cytokines IL-1, IL-6, and TNFα. We also discuss modulating proinflammatory cytokines as an additional immune therapy in PAM treatment.
Introduction
Naegleria fowleri, also known as the “brain-eating amoeba,” is a free-living amoeba ubiquitously found in sources of warm fresh water around the world. N. fowleri belongs to the phylum Percolozoa, and is one of only four free-living amoebae genera that cause human disease (Acanthamoeba spp., Balamuthia mandrillaris, Naegleria fowleri and Sappinia diploidea) (1). Substantial genetic diversity exists within N. fowleri as eight genetic variations, or “genotypes,” have been identified around the world in geographically restricted ranges. Notably, only four genotypes have been reported in human infections (types 1, 2, 3, and 5). Because N. fowleri is prevalent in fresh water, human environmental exposure is very common, yet typically non-pathogenic. However, amoeba contact with the nasal cavity, particular the olfactory regions, can result in infection and a rare, yet fatal, disease called primary amoebic meningoencephalitis (PAM), first described by Fowler and Carter in 1965 (2). Naegleria infections are commonly linked to recreational water activity, but can occur in any situation in which contaminated water comes into contact with the host nasal cavity (including ritual ablution) (3, 4). Early PAM symptoms, including fever, headache, nausea, and vomiting, are shared by many illnesses, making it difficult to establish an early PAM diagnosis. The later symptoms of PAM include altered mental status, nuchal rigidity, seizures, and coma. The disease progresses rapidly and is typically lethal; worldwide, there are only 7 documented survivors out of 182 cases from 1961 to 2021 (5). In this review, we focus on the roles that pro-inflammatory cytokines play in the immune response to N. fowleri infection and propose targeting cytokines as a therapeutic approach in PAM treatment.
Invasion of Naegleria fowleri
Once N. fowleri enters the nasal cavity, the amoeba must bypass several barriers within the turbinate structure and evade local immune surveillance to reach the olfactory bulb of the central nervous system (CNS). To start, the amoeba must reach the olfactory regions, located in the superior regions of the upper airway. At this point, the first line of defense is the mucus lying on the olfactory mucosa, secreted by Bowman’s glands (6). This mucus not only controls the ionic milieu of the olfactory sensory neurons (OSNs) but also provides a physical barrier to prevent direct pathogen attachment to the olfactory epithelium (7). Secretory IgA and IgM antibodies within the olfactory mucus could also impede pathogen movement in the olfactory tissues (8, 9). Anti-N. fowleri IgA antibodies have been reported in healthy human serum and saliva (10) at variable levels (11), however recent work from our group has indicated that serum antibody titers are poor prognostic indicators of olfactory protection (12). These data suggest antibody-based protection may, at best, be insufficient. Besides the pre-emptive defense potentially provided by antibodies within the nasal turbinates, an early cellular exudate in the mucus layer has been characterized in the mouse model of PAM. At 8h post-trophozoite inoculation, Rojas-Hernández and colleagues reported that immune cells and trophozoites were embedded in the mucus of the nasal cavity, suggesting an early cellular defensive mechanism to N. fowleri (13). However, the source of the immune cells and the mechanism of immune recognition of N. fowleri in the mucus layer remain poorly understood. Tight junction and adherens junctions lying between microvillar cells, supporting cells, and OSNs in the epithelium layers also establish a physical barrier to invasion within the nasal cavity (14). Nonetheless, N. fowleri trophozoites have been shown to destabilize the expression of tight junction proteins ZO-1, claudin-1, claudin-5, and occludin in MDCK cells and primary culture endothelial cells in vitro (15, 16). In the mouse model, N. fowleri trophozoites can invade the olfactory neuroepithelium without causing cell death or alarming the immune system at 24h post-infection (13). Notably, one recent in vitro study suggests that human mucoepithelial cells recognize N. fowleri trophozoites through TLR2/TLR4, and this further leads to pro-inflammatory cytokine production (17). Once the amoeba enters the lamina propria, it can readily gain access to OSN axon bundles. Olfactory sensory neuron axon bundles pass through the cribriform plate of the skull to enter the olfactory bulb within the CNS. The CNS has several effective barriers to prevent outside infiltration, however, these cribriform plate passages through the front of the skull, provide amoeba with a passageway into the brain that bypasses the blood brain barrier. It remains unclear how, or if, the amoeba selectively follows these axon bundles toward the brain. Some groups have suggested that neurotransmitters associated with olfactory neurons may act as a lure to attract N. fowleri. Indeed, there is evidence that a G-protein coupled receptor (GPCR) on N. fowleri surface has structural homology to the acetylcholine binding muscarinic acetylcholine receptor 1 (mAChR1) in humans (18). As N. fowleri invades deeper into the olfactory mucosa, entering the lamina propria and axon bundles (olfactory fascicles), it may encounter a unique population of extravascular neutrophils, that have been recently reported to uniquely surveil in the nasal epithelium and lamina propria under homeostatic condition (19, 20). Extravascular neutrophil encounter with amoeba could elicit a series of responses, including reactive oxygen or nitrogen species (ROS, NOS) production, and neutrophils extracellular trap (NET) formation within the olfactory tissues. Ultimately, in animal models, these effector functions can reduce the number of trophozoites invading the olfactory bulb of the CNS and slow fatal PAM development (21). But in animal models, amoeba invariably do reach the CNS and upon arrival glial cells have a minimal capacity to resist N. fowleri trophozoites. Microglia serve as the primary immune sentinel cell within the brain and these cells can respond to N. fowleri by activating NLRP3 inflammasome and MAPK signaling to secrete different pro-inflammatory cytokines (22). And while, published evidence suggests that microglia are capable of lysing and ingesting N. fowleri trophozoites, protease and extracellular vesicles secreted by N. fowleri can also lead to microglia cell death (23–25). As amoeba feed and divide, their increasing numbers result in damage that provokes an intense innate immune activation. Incomplete amoeba control initiates a feed forward loop of recruited blood-borne innate leukocytes and proinflammatory cytokine production that leads to a massively inflamed CNS environment with progressively increasing immune cell numbers (Figure 1). Innate immune cells are able to slow amoeba pathogenicity (21), and proinflammatory cytokines likely play important roles in their anti-amoebic pressure. Yet the excessive CNS immune response fails to control N. fowleri infection, and the collateral inflammatory damage leads to edema, hemorrhage, and elevated intracranial pressure that are critical factors in PAM pathogenicity. It’s unclear how individual proinflammatory cytokines are ultimately deleterious or beneficial to the PAM patients. Here we will further discuss the roles for proinflammatory cytokines, IL1 α/β, IL6 and TNFα in N. fowleri infection.
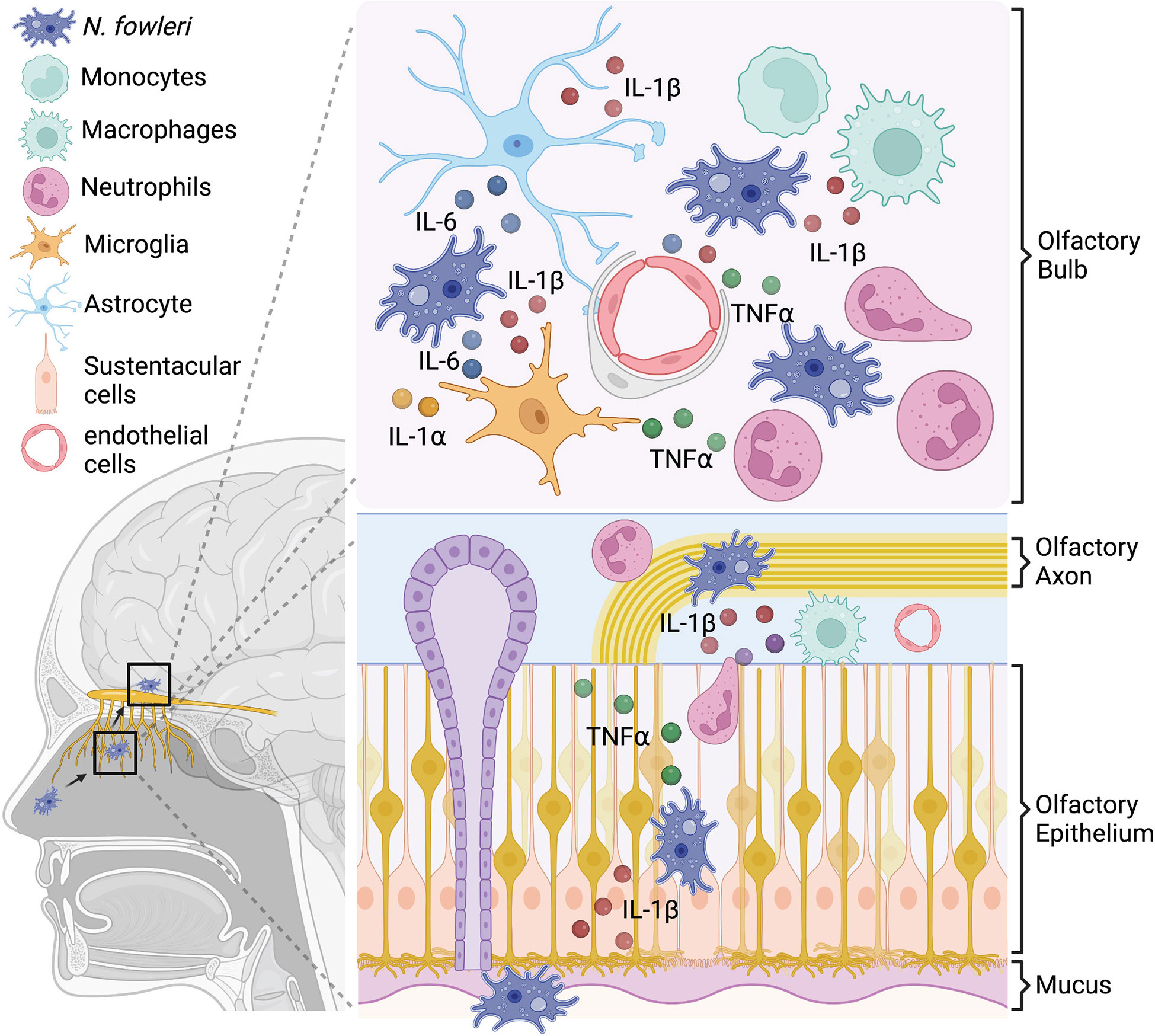
Figure 1 In vitro studies suggest a model for pro-inflammatory cytokine production during N. fowleri infection. N. fowleri attach to the olfactory epithelium and invade into the olfactory bulb through OSN axon tract. In the olfactory epithelium layer, resident macrophages and sustentacular cells may secrete pro-inflammatory cytokines in response to N. fowleri. In the olfactory bulb, microglia, astrocytes, endothelial cells, and macrophages may produce multiple pro- inflammatory cytokines after N. fowleri exposure. These cytokines likely play important anti- amoebic roles, but are also immunopathologic.
Pro-inflammatory cytokines in N. fowleri infection
IL-1α/β
The interleukin-1 (IL-1) cytokine family is a mainstay of innate immunity and inflammation. IL-1 signaling is a key feature of “inflammasome” activation and commonly viewed as a core pro-inflammatory cytokine. Indeed, IL-1 inhibitors have been clinically successful in treating autoimmune, as well as variety of inherited and acquired, inflammatory disorders. Two IL-1 family cytokines, IL-1α and IL-1β, are both reportedly produced by multiple cell types during N. fowleri infection (Table 1). While these IL-1 family members do not have direct effects on N. fowleri (31), they play critical roles in alerting or enhancing the immune reaction to N. fowleri infection [26].
Within the airway, nasal epithelial cells are one of first cell types to contact N. fowleri and in vitro studies suggest that they may respond with IL-1β secretion. A study of human mucoepidermal cell line found that IL-1β was secreted within 3h of co-culture with N. fowleri trophozoites. Interestingly, this study also found that trophozoites could induce mucin production from human epithelial cells (29). IL-1β secretion from monocytes or macrophages requires both priming (signal 1) and activation (signal 2) to produce activated cleaved IL-1β. Briefly, danger associated molecular pattern signals (DAMPs) activate the transcription factor NfκB (signal 1) leading to increased pro-IL-1β expression. Pro-IL-1β is an inactive precursor and requires cleavage by caspase-1 to form the active IL-1β molecule. Perturbation of ionic concentration (Ca2+ and K+ efflux) or further DAMP stimulation (32, 33) can lead to caspase-1 activation through the multi-protein inflammasome complex (inflammasome) (signal 2). In a non-contact co-culture system, macrophage-like cells (THP-1 cells) secrete cleaved IL-1β 3h post co-culture with N. fowleri trophozoites (22). Further analysis showed activation of caspase-1 and the ASC/NLRP3 inflammasome in THP-1 cells co-cultured with N. fowleri (22). Interestingly, another study found that N. fowleri-derived extracellular vesicles did not elicit IL-1α or IL-1β production from THP-1 cells (28). This finding implies that extracellular vesicles alone are minimally stimulatory, and damage caused by factors secreted by live amoeba is required to trigger IL-1 production. Overall, these in vitro data suggest that myeloid cells, especially, tissue-resident macrophages within the olfactory mucosa could respond to N. fowleri infection by secreting pro-inflammatory cytokines.
In vitro studies of multiple CNS cell types including microglia, astrocytes, and endothelial cells, have reported their secretion of IL-1α and IL-1β in response to N. fowleri infection. In one recent study, Lee et al. demonstrated that purified excretory and secretory N. fowleri proteins (NfESP) induced IL-1α and TNFα expression in BV-2 microglial cells (27). The same group showed that treatment of BV-2 microglial cells with a N. fowleri derived cathepsin B led to increased expression of IL-1α, IL-1β, IL-6, and TNF, and these effects are dependent on the NF-κB, AP-1 and MAPK signaling (26). Similarly, primary rat astrocytes also secrete IL-1β and IL-6 after being cultured with N. fowleri lysates and this secretion is dependent on ERK, JNK, and MAPKs activation (30). In addition to CNS glial cells, microvascular endothelial cells can express pro-inflammatory cytokines after contact with N. fowleri in an in vitro model; co-culture of N. fowleri with rat brain endothelial cells not only reduces endothelial tight junction protein expression but also increases expression of IL-6, IL-1β, and TNF (16). In the CNS, endothelial cells, astrocytes, and neurons, but not microglia, have been shown to homeostatically express IL-1R1 (34). During N. fowleri infection, abundant IL-1 release from different cell types may stimulate endothelial CXCL2 production that amplifies peripheral neutrophil recruitment and CNS immunopathology (34). Moreover, the elevated IL-1 may be responsible for “sickness behaviors” through endothelial IL-1R cell during N. fowleri infection (34). Unfortunately, current evidence is almost entirely based on data from in vitro systems and cell lines, and it remains unclear whether IL-1 signaling is essential to control N. fowleri infection in vivo and whether IL-1 signaling is ultimately beneficial or detrimental to the host.
IL-6
IL-6 is a pleiotropic cytokine that is involved in the acute immune response and inflammation to infection. Experiments have demonstrated that IL-6 is produced by multiple cell types, including microglia, astrocytes, and endothelium cells after exposing to N. fowleri in vitro (Table 1). One critical role for IL-6 in infection is driving the host fever response through brain endothelium cells (35). The fever response is believed to benefit the host by generating a less hospitable environment for pathogens that thrive at or below body temperature. However, this is not the case for N. fowleri, which is a thermophilic organism that readily grows at temperatures above 40-42C. While IL-6 driven fever may not be an appropriate response to N. fowleri, IL-6R is highly expressed on neutrophils and monocytes (36), that are critical innate immune effectors that infiltrate the CNS during N. fowleri infection. Both monocytes and neutrophils can shed membrane-bound IL-6R from their cell surface to provide soluble IL-6R (sIL-6R) into the local environment (37, 38). Soluble IL-6R binds IL-6 to form a sIL-6R/IL-6 complex, that potently binds to GP-130 on endothelial cells, activating STAT3-dependent signaling, and inducing monocyte recruiting chemokine CCL2 expression (38, 39). Despite the known pro-inflammatory roles for IL-6 it is still unclear whether IL-6 can stimulate anti-amoebic function during N. fowleri infection or if the secondary effects contribute to tissue damage. More studies are needed to understand how IL-6 may impact N. fowleri pathogenesis.
Tumor necrosis factor-alpha (TNFα)
TNFα has been frequently linked with anti-amoeba activities. In 1984, Ferrante and colleagues observed that conditioned medium from mononuclear leukocytes could activate human neutrophils to increase N. fowleri killing functions (40). TNFα has been shown to be the major player augmenting the neutrophil respiratory burst and lysosomal enzyme release in response to N. fowleri (41, 42). Myeloid cells express both TNFR1 and TNFR2, which bind soluble TNFα and membrane-bound TNFα, respectively. Several studies have reported TNFα “licensing” neutrophils to enhance or perform effector functions. TNFR1 signaling was shown to license mouse neutrophils and increase TLR-dependent cytokine production in a peritonitis model (43). Similarly, TNFα increased neutrophil elastase (ELANE) expression and induced NET formation via TNFR2 in human neutrophils (44), and NETs are reported to damage IgG opsonized N. fowleri trophozoites (45). In addition to myeloid cell activation, TNFα is known to potently increase endothelial cell adhesiveness (46), which in turn facilitates immune cell extravasation at inflamed sites. In vitro experiments have described TNFα production from microglia, endothelial cells, and epithelium cells upon N. fowleri stimulation. Notably, several in vitro studies have shown that TNFα release by microglia peaked 3h after N. fowleri exposure- earlier than observed IL-1β or IL-6 secretion (Table 1), suggesting that TNFα may contribute to the earliest immune activity in response to N. fowleri (24, 25, 27). TNFα clearly plays a key role in innate immune function, particularly through catalyzing subsequent inflammatory cascades, but more in vivo and mechanistic studies must be conducted to understand how TNFα signaling in different cell types and anatomical locations contributes to N. fowleri immunity.
Targeting pro-inflammatory cytokines in PAM treatment
Initial symptoms of N. fowleri infection (headache and fever) belie disease seriousness. Even when meningitis is eventually suspected, delay in initiating treatments likely contributes to the fatality rate. Current clinical PAM treatment includes supportive care and broad-spectrum antifungals and antibiotics, including amphotericin B, fluconazole, rifampin, miltefosine, and azithromycin (47). External ventricular drain (EVD), hyperosmolar therapy, hyperventilation, and induced hypothermia have also been used to resolve cerebral edema and intracranial pressure in recent cases (5, 48). Nonetheless, the mortality of PAM remains over 95% and additional therapeutic approaches are desperately needed.
N. fowleri is not an obligate pathogen, and indeed infection is best described as opportunistic, but accidental (49). Mammalian immune systems are poorly adapted to handle the size and speed of N. fowleri, nevertheless, immune pressure does impede pathogen growth (21). Innate immune activation and inflammatory processes that arise within the CNS during amoeba infection are especially immunopathologic in the context of the confined CNS space. While the complex physiology of the CNS is impossible to mimic in vitro, a recent in vitro study indicated that leukocytes could enhance N. fowleri-induced cell death of human microvascular endothelial cells in co-culture experiments (50). This study reinforces the clinical use of anti-inflammatories for PAM patients and indeed all North American PAM survivors received dexamethasone, a corticosteroid with broad anti-inflammatory activities (5). The clinical utility of dexamethasone during PAM further suggests that the overwhelming brain inflammatory immune response is a critically pathologic component of disease. While the cerebrospinal fluid (CSF) from PAM patients has unfortunately not been analyzed for inflammatory mediators, many clinical studies have reported significant pro-inflammatory cytokine elevations during meningitis and encephalitis (51–55). These studies of CNS infections strongly suggest that PAM patient CSF contains high levels of pro-inflammatory cytokines. Targeted blockade of specific cytokines may therefore allow novel therapeutic approaches that alleviate detrimental inflammatory effects while preserving key immune-enhancing effects in PAM patients. Tocilizumab is a humanized monoclonal antibody targeting both membrane and soluble IL-6R, that is FDA approved by for severe cytokine release syndrome (CRS) (56) and has been issued severe COVID adults and pediatric patients (57). Tocilizumab delivery into the CSF space achieves significant in vivo concentrations in rhesus macaques, making it a candidate for controlling IL-6 signaling in PAM patients (58). IL-1α and β can be inhibited by recombinant human IL-1 receptor antagonist (Anakinra). Anakinra blocks IL-1α and IL-1β activities and is FDA approved for different inflammatory diseases, including rheumatoid arthritis, and cryopyrin-associated periodic syndromes (59, 60). Notably, the intravenous injection of Anakinra achieved effective concentrations in the CNS of subarachnoid hemorrhage patients, indicating blood-brain-barrier penetration (61).
TNFα blockade has been used clinically for over 20 years and five FDA approved drugs are currently available to block TNFα signaling (infliximab, etanercept, adalimumab, certolizumab pegol, and golimumab) for treatment of rheumatoid arthritis, psoriatic arthritis, and many other chronic inflammation diseases. During N. fowleri infection, excess TNFα within the CNS environments could make TNFα an appealing target for reducing inflammation. Indeed, perispinal TNFα blocking with etanercept improved stroke and traumatic brain injury (TBI) clinical outcomes in an observational study (62).
TNFα receptors (TNFR1 and TNFR2) have strikingly different downstream signaling that can drive seemingly contradictory CNS phenotypes. In neurons, TNFR1 activation is linked with neuroinflammation; while TNFR2 signaling is neuroprotective (63–65). The relative importance of TNFα signaling through either TNFR1 or TNFR2 during N. fowleri infection has not been fully elucidated. It will be necessary to dissect the in vivo TNFR1 and TNFR2 function during N. fowleri infection to determine if there may be clinical utility in TNFα blockade for PAM patients.
Concluding remarks
While early identification of PAM remains a key clinical parameter, basic understanding of the immune response during in vivo N. fowleri infection is critical to improving current clinical outcomes. Here we have described what is known about pro-inflammatory cytokine secretion by different cell types in the context of N. fowleri. Billions of dollars have been spent to develop exquisitely targeted therapeutics against pro-inflammatory mediators that are now in widespread clinical use. These drugs offer opportunities to expand clinical treatment beyond the broad immunosuppression of corticosteroids, and selectively target detrimental components of the immunopathologic landscape during N. fowleri infection. However, additional in vivo mechanistic studies are needed to understand the specific beneficial and detrimental roles of the cytokine response in the CNS. In vivo studies of genetically deficient animals or with in vivo antibody blockade will be crucial to dissecting each cytokine’s role in the innate immune response and immunopathology during N. fowleri infection. Combining these in vivo studies with models of clinically relevant treatment approaches will support new therapeutic approaches to PAM.
Author contributions
All authors listed have made a substantial, direct, and intellectual contribution to the work, and approved it for publication.
Funding
EAM is partially supported by 1R01NS121067, 1R21AG074324, and by the Duke School of Medicine Whitehead Family Scholarship.
Conflict of interest
The authors declare that the research was conducted in the absence of any commercial or financial relationships that could be construed as a potential conflict of interest.
Publisher’s note
All claims expressed in this article are solely those of the authors and do not necessarily represent those of their affiliated organizations, or those of the publisher, the editors and the reviewers. Any product that may be evaluated in this article, or claim that may be made by its manufacturer, is not guaranteed or endorsed by the publisher.
References
1. Visvesvara GS, Moura H, Schuster FL. Pathogenic and opportunistic free-living amoebae: Acanthamoeba spp., balamuthia mandrillaris, naegleria fowleri, and sappinia diploidea. FEMS Immunol Med Microbiol (2007) 50(1):1–26. doi: 10.1111/j.1574-695X.2007.00232.x
2. Fowler M, Carter RF. Acute pyogenic meningitis probably due to acanthamoeba sp.: A preliminary report. Br Med J (1965) 2(5464):740–2. doi: 10.1136/bmj.2.5464.734-a
3. Centers for Disease C. Prevention. notes from the field: Primary amebic meningoencephalitis associated with ritual nasal rinsing–st. Thomas, U.S. virgin islands, 2012. MMWR Morb Mortal Wkly Rep (2013) 62(45):903.
4. Ghanchi NK, Khan E, Khan A, Muhammad W, Malik FR, Zafar A. Naegleria fowleri meningoencephalitis associated with public water supply, Pakistan, 2014. Emerg Infect Dis (2016) 22(10):1835–7. doi: 10.3201/eid2210.151236
5. Gharpure R, Bliton J, Goodman A, Ali IKM, Yoder J, Cope JR. Epidemiology and clinical characteristics of primary amebic meningoencephalitis caused by naegleria fowleri: A global review. Clin Infect Dis (2021) 73(1):e19–27. doi: 10.1093/cid/ciaa520
6. Getchell ML, Getchell TV. Fine structural aspects of secretion and extrinsic innervation in the olfactory mucosa. Microsc Res Tech. (1992) 23(2):111–27. doi: 10.1002/jemt.1070230203
7. Bryche B, Baly C, Meunier N. Modulation of olfactory signal detection in the olfactory epithelium: Focus on the internal and external environment, and the emerging role of the immune system. Cell Tissue Res (2021) 384(3):589–605. doi: 10.1007/s00441-021-03467-y
8. Yu YY, Kong W, Yin YX, Dong F, Huang ZY, Yin GM, et al. Mucosal immunoglobulins protect the olfactory organ of teleost fish against parasitic infection. PloS Pathog (2018) 14(11):e1007251. doi: 10.1371/journal.ppat.1007251
9. Linden SK, Sutton P, Karlsson NG, Korolik V, McGuckin MA. Mucins in the mucosal barrier to infection. Mucosal Immunol (2008) 1(3):183–97. doi: 10.1038/mi.2008.5
10. Rivera V, Hernandez D, Rojas S, Oliver G, Serrano J, Shibayama M, et al. IgA and IgM anti-naegleria fowleri antibodies in human serum and saliva. Can J Microbiol (2001) 47(5):464–6. doi: 10.1139/w01-004
11. Rivera-Aguilar V, Hernandez-Martinez D, Rojas-Hernandez S, Oliver-Aguillon G, Tsutsumi V, Herrera-Gonzalez N, et al. Immunoblot analysis of IgA antibodies to naegleria fowleri in human saliva and serum. Parasitol Res (2000) 86(9):775–80. doi: 10.1007/s004360000243
12. Wellford SA, Moseman AP, Dao K, Wright KE, Chen A, Plevin JE, et al. Mucosal plasma cells are required to protect the upper airway and brain from infection. Immunity (2022) 55(11):2118–34. doi: 10.1016/j.immuni.2022.08.017
13. Rojas-Hernandez S, Jarillo-Luna A, Rodriguez-Monroy M, Moreno-Fierros L, Campos-Rodriguez R. Immunohistochemical characterization of the initial stages of naegleria fowleri meningoencephalitis in mice. Parasitol Res (2004) 94(1):31–6. doi: 10.1007/s00436-004-1177-6
14. Steinke A, Meier-Stiegen S, Drenckhahn D, Asan E. Molecular composition of tight and adherens junctions in the rat olfactory epithelium and fila. Histochem Cell Biol (2008) 130(2):339–61. doi: 10.1007/s00418-008-0441-8
15. Shibayama M, Martinez-Castillo M, Silva-Olivares A, Galindo-Gomez S, Navarro-Garcia F, Escobar-Herrera J, et al. Disruption of MDCK cell tight junctions by the free-living amoeba naegleria fowleri. Microbiol (Reading) (2013) 159(Pt 2):392–401. doi: 10.1099/mic.0.063255-0
16. Coronado-Velazquez D, Betanzos A, Serrano-Luna J, Shibayama M. An In vitro model of the blood-brain barrier: Naegleria fowleri affects the tight junction proteins and activates the microvascular endothelial cells. J Eukaryot Microbiol (2018) 65(6):804–19. doi: 10.1111/jeu.12522
17. Martinez-Castillo M, Santos-Argumedo L, Galvan-Moroyoqui JM, Serrano-Luna J, Shibayama M. Toll-like receptors participate in naegleria fowleri recognition. Parasitol Res (2018) 117(1):75–87. doi: 10.1007/s00436-017-5666-9
18. Baig AM. Primary amoebic meningoencephalitis: Neurochemotaxis and neurotropic preferences of naegleria fowleri. ACS Chem Neurosci (2016) 7(8):1026–9. doi: 10.1021/acschemneuro.6b00197
19. Palominos MF, Calfun C, Nardocci G, Candia D, Torres-Paz J, Whitlock KE. The olfactory organ is a unique site for neutrophils in the brain. Front Immunol (2022) 13:881702. doi: 10.3389/fimmu.2022.881702
20. Gonzalez RJ, Alvarez D, Mazo IB, Matysyak C, Garg R, Helble J, et al. Constitutive immune surveillance of the nasal mucosa by neutrophils. Am Assoc Immnol (2021) 206.
21. Ferrante A, Carter RF, Lopez AF, Rowan-Kelly B, Hill NL, Vadas MA. Depression of immunity to naegleria fowleri in mice by selective depletion of neutrophils with a monoclonal antibody. Infect Immun (1988) 56(9):2286–91. doi: 10.1128/iai.56.9.2286-2291.1988
22. Kim JH, Sohn HJ, Yoo JK, Kang H, Seong GS, Chwae YJ, et al. NLRP3 inflammasome activation in THP-1 target cells triggered by pathogenic naegleria fowleri. Infect Immun (2016) 84(9):2422–8. doi: 10.1128/IAI.00275-16
23. Thai TL, Kang JM, Le HG, Lee J, Yoo WG, Shin HJ, et al. Fowlerstefin, a cysteine protease inhibitor of naegleria fowleri, induces inflammatory responses in BV-2 microglial cells in vitro. Parasit Vectors (2020) 13(1):41. doi: 10.1186/s13071-020-3909-6
24. Oh YH, Jeong SR, Kim JH, Song KJ, Kim K, Park S, et al. Cytopathic changes and pro-inflammatory cytokines induced by naegleria fowleri trophozoites in rat microglial cells and protective effects of an anti-Nfa1 antibody. Parasite Immunol (2005) 27(12):453–9. doi: 10.1111/j.1365-3024.2005.00799.x
25. Lee YJ, Park CE, Kim JH, Sohn HJ, Lee J, Jung SY, et al. Naegleria fowleri lysate induces strong cytopathic effects and pro-inflammatory cytokine release in rat microglial cells. Korean J Parasitol (2011) 49(3):285–90. doi: 10.3347/kjp.2011.49.3.285
26. Le HG, Kang JM, Vo TC, Na BK. Naegleria fowleri cathepsin b induces a pro-inflammatory immune response in BV-2 microglial cells via NF-kappaB and AP-1 dependent-MAPK signaling pathway. Int J Mol Sci (2022) 23(15):8388. doi: 10.3390/ijms23158388
27. Lee J, Kang JM, Kim TI, Kim JH, Sohn HJ, Na BK, et al. Excretory and secretory proteins of naegleria fowleri induce inflammatory responses in BV-2 microglial cells. J Eukaryot Microbiol (2017) 64(2):183–92. doi: 10.1111/jeu.12350
28. Lertjuthaporn S, Somkird J, Lekmanee K, Atipimonpat A, Sukapirom K, Sawasdipokin H, et al. Extracellular vesicles from naegleria fowleri induce IL-8 response in THP-1 macrophage. Pathogens (2022) 11(6):632. doi: 10.3390/pathogens11060632
29. Cervantes-Sandoval I, Serrano-Luna JJ, Meza-Cervantez P, Arroyo R, Tsutsumi V, Shibayama M. Naegleria fowleri induces MUC5AC and pro-inflammatory cytokines in human epithelial cells via ROS production and EGFR activation. Microbiol (Reading). (2009) 155(Pt 11):3739–47. doi: 10.1099/mic.0.030635-0
30. Kim JH, Song AR, Sohn HJ, Lee J, Yoo JK, Kwon D, et al. IL-1beta and IL-6 activate inflammatory responses of astrocytes against naegleria fowleri infection via the modulation of MAPKs and AP-1. Parasite Immunol (2013) 35(3-4):120–8. doi: 10.1111/pim.12021
31. Fischer-Stenger K, Marciano-Cabral F. The arginine-dependent cytolytic mechanism plays a role in destruction of naegleria fowleri amoebae by activated macrophages. Infect Immun (1992) 60(12):5126–31. doi: 10.1128/iai.60.12.5126-5131.1992
32. Lopez-Castejon G, Brough D. Understanding the mechanism of IL-1beta secretion. Cytokine Growth Factor Rev (2011) 22(4):189–95. doi: 10.1016/j.cytogfr.2011.10.001
33. Rossol M, Pierer M, Raulien N, Quandt D, Meusch U, Rothe K, et al. Extracellular Ca2+ is a danger signal activating the NLRP3 inflammasome through G protein-coupled calcium sensing receptors. Nat Commun (2012) 3:1329. doi: 10.1038/ncomms2339
34. Liu X, Nemeth DP, McKim DB, Zhu L, DiSabato DJ, Berdysz O, et al. Cell-Type-Specific interleukin 1 receptor 1 signaling in the brain regulates distinct neuroimmune activities. Immunity (2019) 50(2):317–33 e6. doi: 10.1016/j.immuni.2018.12.012
35. Eskilsson A, Mirrasekhian E, Dufour S, Schwaninger M, Engblom D, Blomqvist A. Immune-induced fever is mediated by IL-6 receptors on brain endothelial cells coupled to STAT3-dependent induction of brain endothelial prostaglandin synthesis. J Neurosci (2014) 34(48):15957–61. doi: 10.1523/JNEUROSCI.3520-14.2014
36. Farahi N, Paige E, Balla J, Prudence E, Ferreira RC, Southwood M, et al. Neutrophil-mediated IL-6 receptor trans-signaling and the risk of chronic obstructive pulmonary disease and asthma. Hum Mol Genet (2017) 26(8):1584–96. doi: 10.1093/hmg/ddx053
37. Schumacher N, Meyer D, Mauermann A, von der Heyde J, Wolf J, Schwarz J, et al. Shedding of endogenous interleukin-6 receptor (IL-6R) is governed by a disintegrin and metalloproteinase (ADAM) proteases while a full-length IL-6R isoform localizes to circulating microvesicles. J Biol Chem (2015) 290(43):26059–71. doi: 10.1074/jbc.M115.649509
38. Chalaris A, Rabe B, Paliga K, Lange H, Laskay T, Fielding CA, et al. Apoptosis is a natural stimulus of IL6R shedding and contributes to the proinflammatory trans-signaling function of neutrophils. Blood (2007) 110(6):1748–55. doi: 10.1182/blood-2007-01-067918
39. Rose-John S. IL-6 trans-signaling via the soluble IL-6 receptor: Importance for the pro-inflammatory activities of IL-6. Int J Biol Sci (2012) 8(9):1237–47. doi: 10.7150/ijbs.4989
40. Ferrante A, Mocatta TJ. Human neutrophils require activation by mononuclear leucocyte conditioned medium to kill the pathogenic free-living amoeba, naegleria fowleri. Clin Exp Immunol (1984) 56(3):559–66.
41. Michelson MK, Henderson WR Jr., Chi EY, Fritsche TR, Klebanoff SJ. Ultrastructural studies on the effect of tumor necrosis factor on the interaction of neutrophils and naegleria fowleri. Am J Trop Med Hyg (1990) 42(3):225–33. doi: 10.4269/ajtmh.1990.42.225
42. Ferrante A. Augmentation of the neutrophil response to naegleria fowleri by tumor necrosis factor alpha. Infect Immun (1989) 57(10):3110–5. doi: 10.1128/iai.57.10.3110-3115.1989
43. Deguine J, Wei J, Barbalat R, Gronert K, Barton GM. Local TNFR1 signaling licenses murine neutrophils for increased TLR-dependent cytokine and eicosanoid production. J Immunol (2017) 198(7):2865–75. doi: 10.4049/jimmunol.1601465
44. Neuenfeldt F, Schumacher JC, Grieshaber-Bouyer R, Habicht J, Schroder-Braunstein J, Gauss A, et al. Inflammation induces pro-NETotic neutrophils via TNFR2 signaling. Cell Rep (2022) 39(3):110710. doi: 10.1016/j.celrep.2022.110710
45. Contis-Montes de Oca A, Carrasco-Yepez M, Campos-Rodriguez R, Pacheco-Yepez J, Bonilla-Lemus P, Perez-Lopez J, et al. Neutrophils extracellular traps damage naegleria fowleri trophozoites opsonized with human IgG. Parasite Immunol (2016) 38(8):481–95. doi: 10.1111/pim.12337
46. Min JK, Kim YM, Kim SW, Kwon MC, Kong YY, Hwang IK, et al. TNF-related activation-induced cytokine enhances leukocyte adhesiveness: induction of ICAM-1 and VCAM-1 via TNF receptor-associated factor and protein kinase c-dependent NF-kappaB activation in endothelial cells. J Immunol (2005) 175(1):531–40. doi: 10.4049/jimmunol.175.1.531
47. Cope JR, Conrad DA, Cohen N, Cotilla M, DaSilva A, Jackson J, et al. Use of the novel therapeutic agent miltefosine for the treatment of primary amebic meningoencephalitis: Report of 1 fatal and 1 surviving case. Clin Infect Dis (2016) 62(6):774–6. doi: 10.1093/cid/civ1021
48. Linam WM, Ahmed M, Cope JR, Chu C, Visvesvara GS, da Silva AJ, et al. Successful treatment of an adolescent with naegleria fowleri primary amebic meningoencephalitis. Pediatrics (2015) 135(3):e744–8. doi: 10.1542/peds.2014-2292
49. Casadevall A, Pirofski LA. Accidental virulence, cryptic pathogenesis, martians, lost hosts, and the pathogenicity of environmental microbes. Eukaryot Cell (2007) 6(12):2169–74. doi: 10.1128/EC.00308-07
50. Baig AM. Pathogenesis of amoebic encephalitis: Are the amoebae being credited to an 'inside job' done by the host immune response? Acta Trop (2015) 148:72–6. doi: 10.1016/j.actatropica.2015.04.022
51. Ye Q, Shao WX, Shang SQ, Shen HQ, Chen XJ, Tang YM, et al. Clinical value of assessing cytokine levels for the differential diagnosis of bacterial meningitis in a pediatric population. Med (Baltimore). (2016) 95(13):e3222. doi: 10.1097/MD.0000000000003222
52. Mastroianni CM, Lancella L, Mengoni F, Lichtner M, Santopadre P, D'Agostino C, et al. Chemokine profiles in the cerebrospinal fluid (CSF) during the course of pyogenic and tuberculous meningitis. Clin Exp Immunol (1998) 114(2):210–4. doi: 10.1046/j.1365-2249.1998.00698.x
53. Keller LS, Drozdowicz CK, Rice L, Bowman TA, Lang CM. An evaluation of three anaesthetic regimes in the gray short-tailed opossum (Monodelphis domestica). Lab Anim. (1988) 22(3):269–75. doi: 10.1258/002367788780746449
54. Kothur K, Wienholt L, Mohammad SS, Tantsis EM, Pillai S, Britton PN, et al. Utility of CSF Cytokine/Chemokines as markers of active intrathecal inflammation: Comparison of demyelinating, anti-NMDAR and enteroviral encephalitis. PloS One (2016) 11(8):e0161656. doi: 10.1371/journal.pone.0161656
55. Michael BD, Griffiths MJ, Granerod J, Brown D, Keir G, Wnek M, et al. The interleukin-1 balance during encephalitis is associated with clinical severity, blood-brain barrier permeability, neuroimaging changes, and disease outcome. J Infect Dis (2016) 213(10):1651–60. doi: 10.1093/infdis/jiv771
56. Le RQ, Li L, Yuan W, Shord SS, Nie L, Habtemariam BA, et al. FDA Approval summary: Tocilizumab for treatment of chimeric antigen receptor T cell-induced severe or life-threatening cytokine release syndrome. Oncologist (2018) 23(8):943–7. doi: 10.1634/theoncologist.2018-0028
57. Rosas IO, Brau N, Waters M, Go RC, Hunter BD, Bhagani S, et al. Tocilizumab in hospitalized patients with severe covid-19 pneumonia. N Engl J Med (2021) 384(16):1503–16. doi: 10.1056/NEJMoa2028700
58. Nellan A, McCully CML, Cruz Garcia R, Jayaprakash N, Widemann BC, Lee DW, et al. Improved CNS exposure to tocilizumab after cerebrospinal fluid compared to intravenous administration in rhesus macaques. Blood (2018) 132(6):662–6. doi: 10.1182/blood-2018-05-846428
59. Mertens M, Singh JA. Anakinra for rheumatoid arthritis. Cochrane Database Syst Rev (2009) 1):CD005121. doi: 10.1002/14651858.CD005121.pub3
60. Kullenberg T, Lofqvist M, Leinonen M, Goldbach-Mansky R, Olivecrona H. Long-term safety profile of anakinra in patients with severe cryopyrin-associated periodic syndromes. Rheumatol (Oxford). (2016) 55(8):1499–506. doi: 10.1093/rheumatology/kew208
61. Galea J, Ogungbenro K, Hulme S, Greenhalgh A, Aarons L, Scarth S, et al. Intravenous anakinra can achieve experimentally effective concentrations in the central nervous system within a therapeutic time window: Results of a dose-ranging study. J Cereb Blood Flow Metab (2011) 31(2):439–47. doi: 10.1038/jcbfm.2010.103
62. Tobinick E, Kim NM, Reyzin G, Rodriguez-Romanacce H, DePuy V. Selective TNF inhibition for chronic stroke and traumatic brain injury: An observational study involving 629 consecutive patients treated with perispinal etanercept. CNS Drugs (2012) 26(12):1051–70. doi: 10.1007/s40263-012-0013-2
63. Marchetti L, Klein M, Schlett K, Pfizenmaier K, Eisel UL. Tumor necrosis factor (TNF)-mediated neuroprotection against glutamate-induced excitotoxicity is enhanced by n-methyl-D-aspartate receptor activation. essential role of a TNF receptor 2-mediated phosphatidylinositol 3-kinase-dependent NF-kappa b pathway. J Biol Chem (2004) 279(31):32869–81. doi: 10.1074/jbc.M311766200
64. Zou JY, Crews FT. TNF alpha potentiates glutamate neurotoxicity by inhibiting glutamate uptake in organotypic brain slice cultures: Neuroprotection by NF kappa b inhibition. Brain Res (2005) 1034(1-2):11–24. doi: 10.1016/j.brainres.2004.11.014
Keywords: Naegleria fowleri, immune response to Naegleria, primary amoebic meningoencephalitis, CNS infection, olfactory infection, neuroinvasion, eukaryotic pathogen, immunopathology
Citation: Chen CW and Moseman EA (2023) Pro-inflammatory cytokine responses to Naegleria fowleri infection. Front. Trop. Dis 3:1082334. doi: 10.3389/fitd.2022.1082334
Received: 28 October 2022; Accepted: 22 December 2022;
Published: 18 January 2023.
Edited by:
Melissa Jamerson, Virginia Commonwealth University, United StatesReviewed by:
Gladis Fragoso, Institute of Biomedical Research, National Autonomous University of Mexico, MexicoCopyright © 2023 Chen and Moseman. This is an open-access article distributed under the terms of the Creative Commons Attribution License (CC BY). The use, distribution or reproduction in other forums is permitted, provided the original author(s) and the copyright owner(s) are credited and that the original publication in this journal is cited, in accordance with accepted academic practice. No use, distribution or reproduction is permitted which does not comply with these terms.
*Correspondence: E. Ashley Moseman, ashley.moseman@duke.edu