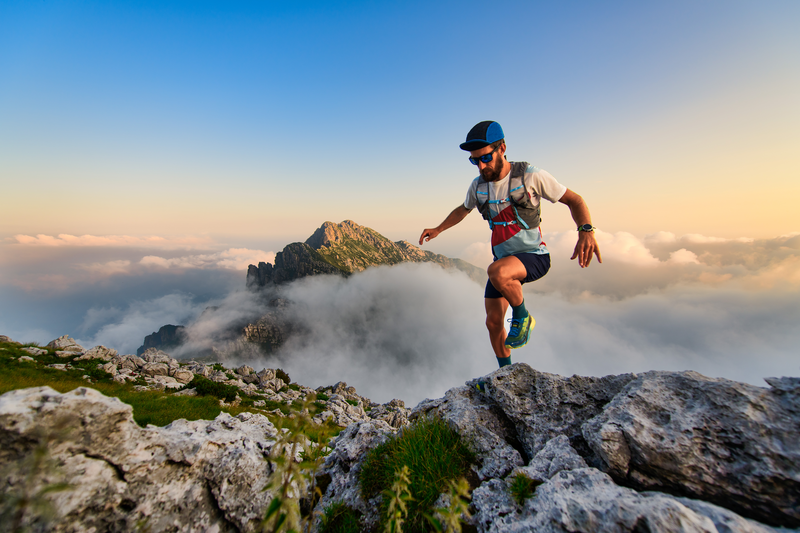
94% of researchers rate our articles as excellent or good
Learn more about the work of our research integrity team to safeguard the quality of each article we publish.
Find out more
REVIEW article
Front. Trop. Dis. , 22 December 2021
Sec. Vector Biology
Volume 2 - 2021 | https://doi.org/10.3389/fitd.2021.804066
This article is part of the Research Topic The Transition of Novel Genetic Control Strategies into Reality for Vector-Borne Tropical Disease Control and Prevention; Research Advances and next Steps View all 12 articles
Ingenious exploitation of transgenic approaches to produce malaria resistant or sterile mosquitoes, or hypervirulent mosquito pathogens, has produced many potential solutions to vector borne diseases. However, in spite of technological feasibility, it has not been determined how well these new methods will work, and how they should be tested and regulated. Some self-limiting transgenic fungal pathogens and mosquitoes are almost field ready, and may be easier to regulate than self-sustaining strategies. However, they require repeat sales and so must show business viability; low-cost mass production is just one of a number of technical constraints that are sometimes treated as an afterthought in technology deployment. No transgenic self-sustaining approach to anopheline control has ever been deployed because of unresolved ethical, social and regulatory issues. These overlapping issues include: 1) the transparency challenge, which requires public discourse, particularly in Africa where releases are proposed, to determine what society is willing to risk given the potential benefits; 2) the transboundary challenge, self-sustaining mosquitoes or pathogens are potentially capable of crossing national boundaries and irreversibly altering ecosystems, and 3) the risk assessment challenge. The polarized debate as to whether these technologies will ever be used to save lives is ongoing; they will founder without a political answer as to how do we interpret the precautionary principle, as exemplified in the Cartagena protocol, in the global context of technological changes.
“One strong and enduring lesson of the past four decades of biotechnology is how often great ideas fail even after good initial results”. Henry T. Greely (Director of the center for law and the biosciences at Stanford University) (1).
The five species of Plasmodium capable of causing human malaria are transmitted by approximately 100 species of anopheline mosquitoes (2). In 2019, an estimated 400,000 people, mostly young African children, died of malaria (3). Another 200 million people survived but many will have debilitating long term symptoms with reduced capacity to contribute to economic growth. Environmental change, pathogen and vector resistance to drugs and insecticides, increasing human population, slow progress in vaccine development, progressive urbanization and population movement are among the factors that have contributed to the high prevalence of vector-borne diseases (4, 5). The development of innovative approaches to reduce this burden are badly needed.
Currently, much attention is being focused on using genetic engineering (GE) to reduce, or possibly eliminate, populations of the three species of mosquito most responsible for malaria transmission — An. gambiae, An. coluzzii, and An. arabiensis — for example, by modifying males so all their offspring are infertile, or by engineering hypervirulent pathogens that selectively kill mosquitoes (6, 7). Another tactic introduces genetic cargos (e.g., Plasmodium-killing molecules) into mosquitoes or mosquito targeting pathogens so that the Plasmodium is targeted rather than the insect (6, 8). Many technical challenges have been overcome. For example, for sixty years, researchers have pondered how naturally occurring gene drives (genetic elements that bias inheritance in their favor) could be repurposed to combat mosquito-vectored diseases (9). The advent of new molecular techniques has made it much easier to engineer gene drives able to speed the spread of linked genetic modification (e.g., knockout of sex determination or pathogen-killing molecules) through a mosquito population (10). Currently, most attention is focused on CRISPR Cas9 technology, but many other naturally occurring site specific nucleases can also be used.
Genetics-based control strategies can be either self-sustaining or self-limiting (6, 7, 11) (Table 1). In self-limiting strategies, introduced transgenes are eliminated from the population over time. The first generation of hypervirulent transgenic pathogens are engineered to act like chemical insecticides achieving a quick knock down; they are not designed to recycle in mosquito populations (8). Self-limiting genetically modified (GM) insect strategies are seen as a technological advance of the sterile insect technique that has been used successfully since the 1950s (12). Wild females that mate with these males produce no viable offspring, thus temporarily suppressing the mosquito population. Long term control requires repeated releases to maintain a population in the environment, thus causing continuing operational costs (7). However, the biosafety implications for this approach maybe relatively manageable given the expectation that transgenes will only persist for one or a few generations after release. This has contributed to self-limiting strategies being much more advanced in terms of practical application than self-sustaining (propagating) strategies. In the literature, self-sustaining strategies are often associated with gene drive systems which can spread a fitness load or disease-refractory gene through a mosquito population. However, although they get much less attention, transgenes that provide a pathogen or a mosquito with a net benefit also have the potential to be highly invasive, and thus permanently alter wild populations (13). For example, genetically modified An. gambiae with enhanced immunity have a mating advantage over their wild type peers, potentially allowing the creation of Plasmodium-refractory GM mosquitoes that can outcompete wild-type mosquitoes (14). As with biocontrol agents, self-sustaining strategies promise low-cost interventions (7, 15) but could have far-reaching and hard to predict consequences to the natural environment (6). At a very basic level, it is not yet clear whether or how well gene drive methods or other self-sustaining strategies will work in nature. However, if successful, then a small number of GM mosquitoes with these constructs might be able to propagate transgenes over the entire range of a species.
Table 1 Self-sustaining and self-limiting strategies determine the potential of a transgene to persist or spread in the environment, either through some form of gene drive or through selective advantage, contributing to a risk profile for each product.
The WHO has expressed support for investigating genetically modified mosquitoes as a tool to fight mosquito-borne diseases. “More than 80 per cent of the global population lives in areas at risk of at least one major vector-borne disease. Taken together, these diseases exact an immense toll on economies and can impede both rural and urban development,” the agency said in an October 2020 statement (16). It, however, cautioned that the use of the technology “raises concerns and questions around ethics, safety, governance, affordability and cost–effectiveness that must be addressed”. Research into the technology “should be conducted through a step-wise approach and supported by clear governance mechanisms to evaluate any health, environmental and ecological implications”, it said (16). Preparing a Target Product Profile (TPP) is part of the process of gaining WHO approval. A TPP outlines the desired ‘profile’ or characteristics of a product that is aimed at a particular disease. It provides information for funders and developers on the performance and operational characteristics expected of products if they are to meet WHO’s needs. Figure 1 shows the elements considered in a TPP the author helped prepare for transgenic fungi. Although the details will vary, the same basic elements will be required for most GM microbe and mosquito products. All these elements are interrelated and need to be considered throughout research and development. Preparing a TPP is therefore a very useful exercise to ascertain the facts or characteristics that have to be true for a product to be successful. The premise being that the more accurately you can identify and test assumptions about key performance parameters, the more likely the new technology has a chance at success. A flaw in any one element could prove to be a fatal weakness.
Figure 1 The principal requirements of a WHO Target product Profile (TPP) for vector control, based on a TPP prepared for a transgenic Metarhizium product (expressing the insect-specific neurotoxin Hybrid) trialed in Burkina Faso (17). A WHO TPP aims to provide information on the performance and operational characteristics expected of products if they are to meet WHO’s needs. It starts with a “product need” context and finding the right technology to solve the unmet need. In this case mosquito pathogens delivering an insect selective toxin to insecticide resistant mosquitoes. To produce a successful product requires identifying a pipeline of strategies and formalizing performance criteria. For example, the product needs to be shown to be safe, being at least comparable to approved (by EPA and other regulators) wild-type fungal products providing a highly favorable risk benefit profile. Hybrid (marketed by Vestaron) has also been approved by the EPA (for use on food commodities). Ideally the technology is patentable by the inventor, and rights are available at reasonable cost to the manufacturer. Metarhizium products have a proven track record of being efficiently manufactured and integrated with other technologies. Work in progress includes optimizing formulation, product stability and shelf life to increase acceptability and cost effectiveness. For marketing, the final product must of course be acceptable to the local population, easy to use, easy to maintain, etc. At a minimum it must not look or smell unattractive, create dust etc, and be tolerated by households such that they remain in-place for the full duration of use.
The first element that needs to be established is does the new technology work, and this is closely linked with the unmet needs it is meant to fulfill. The need in this case includes controlling insecticide resistant mosquitoes, and thus saving lives by reducing mosquito bites by an epidemiologically relevant extent. The new technology has to be able to do this at least as well, and preferably much better than older technologies, and preferably should also be compatible with them to allow integration. No gene drive has yet been released in the wild so it is not clear how they would work in nature. However, the technological feasibility of gene drives has developed very rapidly. For example, a gene drive to target a gene (doublesex) that regulates sexual development produces infertile female mosquitoes, but the males remain able to spread the debilitating gene to an ever-shrinking number of progeny. Large indoor cages of mosquitoes sustaining this gene drive were driven to extinction within one year (18). Importantly, this is the first gene drive study to use cages sufficiently large that they allowed some of the complex mosquito feeding and reproductive behaviors normally observed in the field. However, large cages cannot reproduce the complexities of nature, such as the genetic complexity of wild mosquitoes, nor physical features such as mountains and rivers that can split wild mosquitoes into reproductively isolated populations. Thus, while in theory a gene drive produced through self-sustaining strategies and its ecological consequences could be irreversible once released (6), many malaria researchers are more concerned that gene drives will not actually be powerful enough to affect mosquito numbers to an epidemiologically relevant degree. The Target Malaria research consortium (a non-profit seeking to use gene-drive mosquitoes in Africa) has produced a model of prospective release locations that considers mosquito populations at more than 40,000 settlements in Burkina Faso and surrounding countries. The model also considered the impact of physical objects (mountains, rivers etc.) to show that repeated introduction, rather than a single release, of modified mosquitoes over several years and numerous villages will be needed to reduce their abundance (19). Target Malaria do not expect gene drives to eliminate malaria, but only to deplete mosquitoes from an area so that the parasite-insect-human cycle collapses. Other gene drive initiatives that have a different approach, such as population modifications which render mosquitoes incapable of transmitting malaria, will likely have different ecological prospects (eradicating the disease instead of the vector species) and risks (potentially unlimited self-propagation), as well as economic consequences (if fewer releases necessary).
A different sort of risk is that early benefits will shrink because of the development of resistance. Anophelene mosquitoes have a track record of mutability and adaptability that has enabled them to out evolve many previous control attempts e.g., chemical insecticides. In the wild even a small genetic change can incur a fitness cost, and gene drives that can spread through the entire species could exert a strong selection for resistance. Prominent examples where gene drives do not spread unchecked have already been identified in proof-of-principal laboratory experiments (20, 21). However, there is likely to be an important difference in fitness cost and selection pressure between population suppression and population modification approaches if the latter only make the mosquitoes refractory to malaria and otherwise leave them unaltered. Such modification approaches could be quite resilient to drive resistance alleles (22, 23). Where resistance develops it will probably involve a change in the target sequence so that the nuclease can no longer cleave it, and this can be factored into the development of the gene drive. For example, designing the gene drive to target a region of its doublesex target gene that is highly conserved (18).
Recent advances have also been made in genetically engineering new types of highly effective mosquito-killing fungi that bring together genes from different organisms (8). Following WHO protocols established for genetically modified mosquitoes, though not yet implemented, Burkinabe and American scientists (including the author of this article) built a “MosquitoSphere” (a compartmentalized facility of mosquito netting) near the village of Soummossa. The trial showed that the mosquito pathogenic fungus, Metarhizium, genetically-engineered to produce a toxin (called Hybrid) found in spider venom, was as effective against insecticide resistant mosquitoes in a malaria endemic environment as anticipated from laboratory investigations (17). In laboratory studies, Metarhizium expressing multiple insect-specific toxins under control of a hemolymph-specific promoter increased fungal lethality to insecticide resistant mosquitoes at spore dosages as low as one conidium per mosquito (24). This suggests that transgenic pathogens can provide a breakthrough in the worldwide fight against malaria, subject to being able to scale up and deploy this new technology.
Genetic engineering has opened up a wide range of fungal targets for manipulation, all of which have produced substantial improvements in the desired traits. They have been used to express insect molecules that play critical roles in development, physiology, immune functioning and behavior, as well as human antibodies that target sporozoites as they travel through the hemolymph to the salivary glands (8, 25, 26). Fungi have also been engineered for improved resistance to all of the important environmental abiotic stresses (temperature, humidity, and exposure to UV-irradiation) that have historically reduced field efficacy of these fungi (26). These transgenes are likely to increase pathogen lifespan, host finding, sporulation, dispersal, and allow the exploitation of new ecological niches, potentially increasing pathogen spread in nature. Combining resistance to abiotic stress with increased targeted virulence should produce particularly effective strains, and along with an ability to engineer host range (27), facilitate production of designer self-sustaining pathogens able to recycle in wild mosquito populations, suppressing them in the long term. However, even fungi engineered to resist abiotic stresses are unlikely to be optimal in all environments, and longer-term factors like climate change will impact all aspects of host pathogen interactions in complicated but modellable ways (28).
As with gene drives, a potential downside to more effective transgenic Metarhizium is increased pressure on the mosquitoes to evolve resistance. Individual fruit flies vary a lot in their ability to suppress infectious fungi and bacteria, and resistance correlates with several unexpected phenotypes including sleep (29). Extensive genetic variation in resistance within the same population provides the raw material for selection to act upon with implications for the sustainability of wild type or transgenic fungi. However, infection with Metarhizium expressing hybrid quickly reduces insecticide resistance in wild caught malaria-vector mosquitoes, so that insecticides and biologicals could be applied together to maximize impact and to manage resistance issues to both chemicals and biologicals (30). Part of the unmet need fulfilled by transgenic Metarhizium strains would be to give old insecticides like pyrethroids a second chance, as well as managing the evolution of resistance to new insecticides. Here in particular, with their ability to reverse insecticide resistance, these fungi complement a capability gap, which is an important component of a TPP (Figure 1).
The same Burkinabe scientists involved in the semi-field trial of Metarhizium (led by Dr. Abdoulaye Diabaté) have also conducted a small-scale release of genetically modified sterile male mosquitoes in Bana, another village in Burkina Faso. However, scientists developing genetically manipulated anophelines agree that it will be at least 5 years before a product is ready for field testing, and only if allowed by regulatory bodies. Metarhizium is experimentally much more tractable than mosquitoes which has accelerated progress. Furthermore, Metarhizium provides an approach that is not necessarily specific to a reproductively isolated population of anophelines, leaving others to transmit disease. Consequentially, fungal products are placed to begin a series of open field trials to truly assess the efficacy, survivability, and environmental risk posed by GE pathogens. The final phase of scaling will be the deployment and monitoring. However, as with GM mosquitoes, this route to deployment is subject at each stage to decisions on efficacy and safety endpoints, regulatory approvals, and social acceptance. Progress therefore requires continuing positive overlap between these scientific, regulatory and social domains.
Use of gene drives and other transgenic approaches for pest and disease control may offer great benefits to society. However, as with any technology that can reduce populations, it may also pose risks to ecosystems. Eradication of malaria in Europe and America provides a historic example of this. Malaria was widespread in these continents and its control was achieved using chemical and sanitary measures to kill mosquitoes (31). However, the nature of these interventions, including drainage of wetlands and broad-spectrum pesticides like DTT, likely caused enormous unintended, and largely unexplored, environmental damage. There are several current definitions of risk, but the National Academies report ‘Preparing for Future Products of Biotechnology’, addresses the concept succinctly, stating risks ‘are comprised of undesirable outcomes (what), the possibility of occurrence (how likely), and state of reality (ways the risk occurs in pathways)’ (32). Students of risk assessment policies believe a well-designed policy should help manage the tension between a desire for caution regarding the risk of intervention and worry about the risks of non-intervention (33). Risks can be presented as the risk of not doing something. If the mosquito control technologies work then the price of non-intervention is between 1,200 and 2,000 people a day who die of malaria in Africa.
Regulation of transgenic microbes for pest control has precedents. Several transgenic microbial pest control agents have already been marketed without much fanfare (34). Hybrid, also known as Versitude, was recently approved by the U.S. Environmental Protection Agency (EPA) for control of pests. The EPA has already sanctioned small scale outdoor field trials of genetically engineered Metarhizium (35, 36). There are well established risk assessment models for wild type fungi, and Metarhizium has been approved for household use against cockroaches. Field release may be easier to regulate and implement with a self-limiting pathogen such as the Hybrid toxin expressing Metarhizium, which is not designed to recycle, and can integrate readily into existing control strategies. Even for such self-limiting strategies the timeline for release will likely be determined more by regulatory challenges than by the science, and it is generally assumed that the regulatory hurdles for any self-sustaining agents (e.g., a transgenic fungus or gene drive technology with the potential for continental impacts), will be higher. It is important to note that this assumption is in the absence of test cases. Risk assessments for all technologies will be on a case by case basis, so it is in fact difficult to predict how different transgenic approaches will be viewed by the public and regulators. Gene drives in particular may raise new challenges for regulation (37), but if the term “gene drives” develops forbiddingly negative connotations, it may still be possible to implement simpler strategies for replacing natural mosquito populations involving genes that can spread because they impart a selective advantage (13).
Currently, proponents of mosquito gene drive technologies predict that the first field trials could occur in 5–10 years in Uganda, Mali or Burkina Faso (38, 39). As gene drive mosquitoes dominate the gene-drive field, these trials could be the first test case for public acceptance of gene drive technology anywhere in the world. As such they need to start off well, with successful projects that gain substantial local support, or else the technology will likely become unusable for decades. This has focused attention on public opinion, scientific developments and risk governance in sub-Saharan Africa. Regulatory capacity in Africa has been greatly boosted by NEPAD (the New Partnership for Africa’s Development), establishing the African Biosafety Network of Expertise (ABNE) and the African Medicines Regulatory Harmonization (AMRH). These have assisted African union states to implement functional regulatory frameworks for both agriculture and health applications (40). Public concerns about genetically modified organisms (GMOs) and their own health will also influence this debate, and public discourse is needed about what society is willing to risk given the potential benefits. However, to date there have been very few efforts to actively engage African publics (41). This obviously needs to be rectified. Exceptions include consultations in Ghana, Kenya, Botswana, and Gabon (2016–2018) that were organized by the New Partnership for Africa’s Development. African stakeholders identified similar goals and risks to American stakeholders but also raised additional concerns about fresh water protection and mosquito behavior (42).
Only one transgenic mosquito approach, pursued by a company called Oxitec, has had regulatory approval and been subject to field tests (in Brazil, Panama, the Cayman Islands and Florida). Scudellari (39) attributes the success of GM insect technology to be largely due to the entrepreneurial engagement of Oxitec, with “its outstanding position in the field and pooled moral and exclusive technical authority to promote, produce and distribute GM insects. Oxitec puts GM insect technology into practice and demonstrates its workability”. The particular case of Oxitec mosquitos does not involve a gene drive, and should be self-limiting, but they share some common features and risks with gene drives. Oxitec genetically engineered Aedes aegypti (a carrier of yellow fever, dengue, and zika) to carry a ‘self-limiting’ gene that makes females dependent on an antibiotic —as they don’t get the antibiotic in nature, they die. But males survive and carry the gene into the next generation of females, which is expected to reduce the local female mosquito population. Oxitec is a for-profit company, and has not focused on independent peer reviewed publications. Their results though seem promising, showing a reduction of up to 95 per cent in mosquito populations and a drop of over 90 per cent in dengue cases. Despite this, many voices have been raised against the Oxitec trials. The company has been criticized for allegedly not being transparent about their and the US EPA’s review process, which Oxitec has rebutted. However, this criticism echoes those leveled against the agricultural company Monsanto and points to a crucial need to be seen to be transparent in order to build public confidence and reduce mistrust (43). There have also been concerns that releases may not turn out as expected, as happened in a trial at Brazil, which saw some hybridization and introgression of genetic elements from Oxitec mosquitoes into the wild population (44). Some of these concerns were also successfully challenged by Oxitec and the original manuscript (44) is now marked with an Editorial Expression of Concern (45). Of particular note, the introgressed genetic elements were reported to be from the transgenic mosquito genetic background rather than the transgenes for antibiotic dependence themselves. Therefore, if a particular effect from hybridization was observed it could not be attributed to genetic engineering.
GMOs are regulated in most countries, and are also covered by international agreements such as the WHO Guidance Framework for Testing of Genetically Modified Mosquitoes (46), and the Cartagena Protocol under the United Nations Convention on Biological Diversity (CBD) (47). This was amended (48) to emphasis specific issues that may arise from the environmental release of GM mosquitoes. Strict application of the Cartagena Protocol precludes use of systems where movements across national borders are hard to prevent, which will likely be the case for pathogens or mosquitoes which can spread their modified trait across a continent (49). Changed mosquitoes in countries that approve their release could end up being distributed world-wide and in places that rejected them. Mosquitoes spread through international trade and human travel — the Asian tiger mosquito, Aedes albopictus, may have been accidently imported from China to the USA and Europe on recycled tires and “lucky bamboo” plants (50). Regulation of GMOs requires an Environmental Risk Assessment (ERA) to be conducted before a GMO can be released into the environment. An ERA typically starts with developing hypotheses (or risk scenarios) of the potential harm the GMO could cause to the environment or people. Based on the Oxitec experience an ERA for gene drives would need to consider the potential for spillovers of gene-drive alleles to non-target populations. Due to the risks and complications of deploying gene drives, studies to date on spillover potential have relied on mathematical modeling. The models suggest that the extent of spillovers will be influenced by the configuration of the gene drive and migration rates (51), but uncertainty about malaria vector biology limits what modeling can do. Beyond the approximately 100 species of Anopheles mosquito, another 3,500-mosquito species do not carry malaria. The extent to which gene drives can pass between mosquito species is uncertain, as is whether a gene drive will work the same way in all malaria vectors, or if the populations of only some malaria vectors collapse, whether others will take their place? In addition to the direct risks of gene drives, there may also be broader consequences to altering or depleting a species. Anopheles mosquitoes are not known to be a classic keystone species that others depend on, but eliminating species of mosquitoes that contribute to pollination and provide food for some animals could have ecological consequences. These unknown potential consequences of successful gene drive applications will need to be evaluated by regulators. It is sometimes remarked that guidance on use of gene drives can derive from experience using natural (i.e., not engineered) enemy species as biological control, as this approach is widely accepted to be environmentally safe. This is broadly true, but engineered gene drives spilling over into non-target populations may present a higher scale of risk to stakeholders (51). There is considerable information on transgenic plants, some of which may be applicable, as gene drives represent only a subset of potentially invasive transgenes. Using the core principle of regulatory risk assessment “exposure” x “hazard” = “risk”, gene flow is the “exposure” component of the equation. Many cases of gene flow have been observed from transgenic plants but actual environmental “hazards” are very rare (52).
The type of experimental data and modeling etc. that needs to be collected prior to the release of a GMO will be determined by the risk scenarios generated during preparation of an ERA, and there may be challenging tradeoffs between reducing uncertainty and avoiding risk. As Redford et al. (37) pointed out, “seeking to reduce epistemic uncertainty by performing a risk assessment on emerging technologies may require research activities that themselves pose some risk.” Self-sustaining technologies exemplify this risk as there is currently little certainty about how they would behave in the environment. While modeling suggests that geographical features will isolate mosquito populations to limit the spread of gene drives (19), resolving uncertainty will still require reliable data on altered phenotype and population data, gene flow between target and non-target populations, modeling of impacts on food web structure, etc. (53). Conformity with WHO protocols require that an intentional field release not be conducted prior to evaluation in cage and semi-field trials. This step-by-step approach seems designed for GM systems such as crops that typically don’t spread much on their own, whereas gene drives are designed to spread. To date gene drives have been studied in a laboratory setting only. Field trials may not be possible with a potentially highly invasive self-sustaining technology as by their nature a positive result would lead to unauthorized international spread. At least in theory this could also occur after the accidental release of just a few individuals during testing.
This raises the seemingly impossible paradox of how to mitigate risks and conduct field trials in a system that could permanently alter a species? Conducting field trials on isolated islands first and/or molecular confinement measures such as self-eliminating transgenes (‘biodegradable transgenes”) have been suggested as means to generate data before the introduction into borderless/expansive environments (54–56). The US Defense Advanced Research Projects Agency (DARPA) Safe Genes program is spending US$65 million across several US research teams developing gene drives with built-in controls and/or external overrides so that gene-drive technologies can be reversed or contained. The involvement of DARPA, part of the United States military, has raised concerns in the media and among anti-GM activists about the militarization of gene drives. However, DARPA themselves have claimed their purpose is to develop tools to circumvent gene drives if something goes wrong, whether nefariously or by accident (57). With DARPA funding the Target Malaria team recently reported that a single release of male mosquitoes expressing an anti-CRISPR protein (AcrIIA4), could stop the doublesex drive spreading in a caged population (58), though how they will test this countermeasure is unclear as DARPA Safe Genes contracts expressly forbid conducting a field trail. Nevertheless, this approach provides a possible intervention in the case of unintended releases, particularly for self-sustaining strategies with high potential of spread. DARPA funding also contributed to the development of “daisy-chain drives”. These are a potentially self-exhausting form of CRISPR-based gene drive that consists of a series of genetic elements that are sequentially lost with each generation until the drive is no longer functional (59). Constraining generational and geographic spread will reduce the impact of daisy-chain drives on the diseases vectored by widely dispersed mosquito species, but conversely, they could facilitate safe field testing, and allow local communities to decide on deployment with minimal risk of spread across political borders (59).
Another potentially key aspect of an ERA is the nature of the genetic insertions (“the cargo’ as it is sometimes called) that will be dispersed through the population. Regulators may consider that a gene drive which prevents the mosquito transmitting disease would be less likely to cause harm than one designed to spread sterility. This also applies to the different strategies proposed with entomopathogenic fungi. For example, GM Metarhizium expressing insecticidal toxins to quickly reduce An. gambiae populations, and GM Metarhizium expressing antibodies targeting sporozoites to produce mosquitoes with a lower level of malaria infection (8, 60). The population suppression strategy should have more rapid impact, but the disease reduction strategy should bypass some concerns regarding the emergence of resistance, and employing a transgenic fungus that differs from wild type only in expression of a specific anti-plasmodium gene seems inherently less likely to raise concern. Most Metarhizium strains persist as clonal populations, with no sign of horizontal gene transfers on a human historical scale (61, 62). There are also a variety of mitigating strategies if required by regulators, including engineering poor or blocked conidiation, low persistence, and/or autolytic programs (63), or using site-specific recombination systems that eliminate the transgenes from the fungus after it has killed the insect so that it returns to wild-type virulence (8, 64).
The core precautionary prospective of the Cartagena protocol is shared by many research councils, scientific societies and regulatory agencies, and considered in a wide range of environmental laws, treaties, and protocols. In November 2018, the Conference of the Parties to the Convention on Biological Diversity considered imposing a moratorium on gene drives, but ultimately approved a cautious approach to moving forward. Researchers would have to show that GE insects posed no threat, and any field test would have to be preceded by the free, prior and informed consent of people who live in affected areas (1, 60). How to demarcate “affected” in this context is not clear, but could be interpreted as the area that the gene drive could disperse. The very laudable aim of the precautionary principle is to prevent risky and untested products. However, no human endeavor is risk free. According to the WHO, a strict interpretation of the precautionary principle could mean that before granting regulatory approvals all possible safety issues would need to be resolved, regardless of societal needs and potential benefits (46). As Matt Ridley, author of “The Rational Optimist”, states; ‘At its worst the principle does huge harm because it says banish potential hazards without considering the benefits of an innovation while ignoring the hazards or an existing technology. Don’t do anything new.” (65).
Ridley and others argue that new policies should be examined for the impact they could have on innovation, and if harmful to innovation they be reconsidered. The WHO believe their guidelines already have embedded mechanisms that balance risk and benefit evaluation, and avoid creating barriers, high costs and extended indecision on regulatory approvals (46). They propose conducting economic evaluations to compare alternative courses of action as a basis for making sound decisions. However, the major risk assessment challenge is the need for a political answer as to how to interpret the precautionary principle in the global context of rapid technological changes capable of altering entire ecosystems (43, 66). There is a body of opinion that a new neutral globally harmonized regulatory system is required which would need to be trusted, transparent and enforceable, with punitive liabilities. The United Nations and other organizations have working groups to study the issues. Disagreements derive from defining what a “neutral” system looks like. Anti-GMO groups take that as meaning not biased towards professional expertise, which presumes a steep learning curve by lay people worldwide. This curve must allow decision makers to address what constitutes “informed consent” in the context of biological and ecological uncertainties, and it is unclear how this regulatory system could be empowered to override the concerns of the most risk adverse countries. Many countries will be unwilling to give up sovereignty in this way-the USA for example has not ratified the Cartagena protocol-and their rights have to be respected, yet, requiring worldwide consensus before proceeding in any one place seems a recipe for stalemate.
Historically, social acceptability issues relating to GE have focused on food products and have usually been driven by perceptions of benefit and risk, whether there were non-GE alternatives, whether the products were sold by large multinational corporations, and the nature and timeliness of the benefit to the public (66, 67). Although some of these points may also apply to mosquito control, actual public responses to deploying transgenic products for health purposes are difficult to anticipate. In contrast to GE food products, mosquito control work is mostly funded by public health organizations or philanthropies, and often conducted at universities or non-profit organizations. This could raise different public concerns if a large funder such as the Gates foundation in a resource-poor environment was considered to have undue influence and control of the narrative. In any event, it is totally clear that science based regulatory decisions will not be enough for any emerging biotechnology proposed for field release. Social acceptability and values-based concerns will have to be addressed for all the transgenic vector control technologies under consideration. An element of the current anti-GMO activist attack is that there is a lack of transparency, no matter how much information is provided. However, historical lack of transparency and public consultation, sometimes simply due to naivety, has helped conspiracy theorists with this line of attack, dominating the political narrative and spreading disinformation on risks for decades.
Of crucial importance today is public participation and capacity building in countries like Burkina Faso, where releases of self-sustaining pathogens or gene drives may be made. If these stakeholders reject these technologies they will not progress. As Fredros Okumu (Director of Science of the Ifakara Health Institute in Tanzania) told The Washington Post (68) many people in Africa view gene drives as “foreign technology”. “The key to winning approval of local communities to this kind of malaria eradication program is to fund research institutions in Africa and train African scientists who can then explain the benefits of gene drives to their fellow citizens”, he said. Burkina Faso is progressing in this direction with one of Africa’s highest-profile malaria research laboratories [the Institut de Recherche en Sciences de la Santé (IRSS)], which has funding from many external sources, including the World Bank, and is part of the Target Malaria consortium. IRRS has an ethics committee and Burkina Faso a well-established National Biosafety Agency with experience at creating staged approval processes.
Kuzma (69) argues that before progressing with gene drive projects, “external experts, stakeholders, and citizens with specialized and local knowledge” should be consulted in a transparent way. The Target Malaria project in Burkina Faso provides potential examples of how this might be done. In 2019 they conducted a small-scale release of sterile male mosquitoes in the village of Bana. These did not have a gene drive mechanism but where intended to prepare for potential future releases of gene drive mosquitoes. For several years preceding the trial the consortium focused on characterizing local mosquitoes and their behavior and ecology. Of crucial importance, they also engaged the local community to explain the project and to agree a set of principles – transparency, inclusiveness, openness to different perspectives –to help garner community participation in project activities. The community itself designed its own acceptance model, choosing to establish a representative panel of citizens that was a conduit between the institute and the community. This was a very small-scale trial, with a self-limiting mosquito strategy, and a larger trial over a wide geographical area, particularly if it involved self-sustaining transgenics, would require a broader notion of “community”. A recent Target Malaria sponsored workshop that focused on what it means to get community agreement for gene drive research identified this as an area requiring further discussion (70).
Many advocates of transgenic vector control technologies, as well as other emerging fields with great potential, fear they will be susceptible to subversion by anti-technology activists inducing unfounded fears in a fearful public; this fear of fear has been called ‘synbiophobia-phobia’ (71). Western Anti-GMO advocates have recently stepped up attempts to influence African public opinion. However, the experience of many groups helping to introduce new technologies is that African communities tend to form their own opinions on their benefits after experiencing them. Thus, mobile banking (Orange money, Nana-Express, Telecel-Flash) conducted using cell phones has been adopted by people irrespective of their level of literacy. This was also the case with Burkinabe cotton-farmers, who quickly accepted GM Bt-cotton which produced the expected beneficial results (72). However, owing to technical issues with the fiber length produced by GMO Bt cotton the seeds were withdrawn in 2015, after they had been in use for eight years. This displeased farmers who report suffering significant crop loss, despite using more pesticides (73). According to Dr. Umar Traore of the National Biosafety Agency in Burkina Faso referring to Bt cotton in the context of GMO approaches to mosquito control, “what we learned is that we should avoid mistakes when it comes to biosafety matters. Because if it was a biosafety issue, the whole technology would have collapsed here. We are happy that it’s not a biosafety issue.” (74).
Public debate in the west surrounding GMOs has relied heavily on emotional discourse and conspiracy theories. Generic anti-GMO attitudes have been fanned by pressure groups that believe public opinion is more readily influenced by appeals to emotion than by objective facts (75). A particularly effective example of framing was the use of “Frankenfoods” to indicate things inappropriately brought together that escape constraints. There have already been attempts to frame discussions of gene drive organisms as ‘genetic extinction technologies’ (76), which exaggerates their power, while implying that all gene drives are intended to be global mosquito killers, whereas many are intended to be local and/or target the Plasmodium rather than the mosquito. This raises the question as to whether people can be reasoned out of positions that are based on emotion? Jonathan Swift (1667-1745) thought not “It is useless to attempt to reason a man out of a thing he was never reasoned into”. Furthermore, attributing public concerns to scientific ignorance ignores evidence that people can become more polarized when they are given more information for confirmation bias to work with (77). Notwithstanding this, as the covid pandemic made clear, scientists need to be able to communicate their complicated and highly technical areas of research, and make their intentions known, as early as possible. There is a particular need for scientists who can interact with decision makers in government and elsewhere. Advice for science policy training programs usually includes paring down extensive explanations into jargon-free digestible bites, and the need to adopt a clear and confident tone; studies suggest that the tentative tones adopted by many scientists are an obstacle to communication (78).
One way to ensure costs that would be appropriate to developing World use would be use gene drive mosquitoes or a mosquito pathogen that could be released in small numbers, and that will reproduce and proliferate. Ideally, this would cause a population collapse or modification of a large part of the mosquito population so that they are refractory to malaria. Such a product would not require repeat sales and thus could be developed by government and philanthropic agencies without thought for profits. For profit use of biotechnology is often used by anti-GMO pressure groups to spark public fears of misuse by corporations. The historical context of these fears was the early dominance of Monsanto in producing genetically engineered foods. Monsanto’s seed patenting model was conflated with the science, tainting all GMO research. Gene drives may be more favorably viewed by the public if people don’t fear that profits have priority over public health. Likewise, it should be possible to engineer free-living self-sustaining microbes that will reproduce under field conditions at a level that would provide effective control. However, as with gene drive mosquitoes, such agents would have no respect for national borders and hence could fall foul of the Cartagena protocol.
The cost effectiveness of a control strategy based on a self-limiting pathogen or GM mosquito will always be compared with any available alternatives, including chemical insecticides. The most cost-effective control strategies and agents will be used in the short or long term, unless more expensive control programs are subsidized by governments or international agencies (79). An example of the latter is the use of Metarhizium acridum for locust control in Africa (79). Oxitec’s current business model suggests that without continuing government or philanthropic subsidies, customers will eventually make ongoing payments for repeated releases of GM mosquitoes to avoid resurgences in mosquito populations. In the meantime, the company has received significant venture capital investment, public subsidy, and grants from the Gates Foundation and the Welcome foundation. In 2015, the biotechnology company Intrexon Corporation (USA), purchased Oxitec (80), ensuring Oxitec has the financial assets to move GM insect technology forward (81). Self-limiting gene drives would seem to be a requirement for a private commercial gene drive industry to develop. However, the Target Malaria model suggests that repeated releases of engineered self-propagating mosquitoes over several years and numerous villages will be needed to reduce the abundance of wild type mosquitoes (19). Other gene drive initiatives, such as daisy chain drives, are designed to produce limited spread and persistence, and will therefore require to show business viability, or like M. acridum they will need to be subsidized. There is potential for commercial use of gene drives, at least for agricultural pests, and if successful could build public confidence in the technology (82). For example, the California Cherry Board has funded research on a gene drive that could potentially eradicate the spotted-wing Drosophila, an invasive fruit fly that ruins soft fruits (83). Commercial usage will require low-cost mass production, and overcoming a number of other technical constraints that are sometimes treated as an afterthought in technology deployment and commercialization. In particular, any gene drive technology is going to be highly regulated, providing an obstacle to commercialization that explains why most gene-drive funding has come from either philanthropies, such as the Gates Foundation, or the military. Likewise, regulatory costs are a major factor explaining why commercialization of transgenic crops has been largely left to large corporations (82).
There are many commercial microbial pest control products, and even a few that are GE (34). The GE products are deployed in the USA but most biological control products are used in developing countries and with crops where the slow speed of kill of most wild type pathogens is not uneconomical. Cost effectiveness can vary with government regulations affecting registration and use, and as well as these, the costs of development, production and use of biocontrol agents is typically much lower in developing countries. One of the world’s most successful biological control programs involves treating two million hectares of Brazilian sugar cane with Metarhizium anisopliae to control spittlebugs (84). The fungus is produced on a large scale by sugar mills. Chinese factories also produce thousands of tons of Metarhizium spp yearly, principally to combat locusts. For smaller-scale production serving local needs, fermentation in plastic bags containing low-cost agricultural materials such as rice may be practical (85). Africa already has this technology (86). Fortunately, high quality research over several decades have already provided entomopathogenic fungi with an outstanding record of safety to human users (87), so manufacture, storage, distribution, delivery, and application should pose no risk to the workforce.
Manufacturing processes for GM insects or microbes will also need to meet any requirements of local, regional or other appropriate regulatory authority. Parameters indicative of consistent manufacturing will need to be defined and specified, and this might be easier at a large factory scale. However, deploying Metarhizium as a community-based “cottage industry” provides a small-scale decentralized solution that will likely be less expensive than industrial production and more likely to be embraced by the communities. Locally produced fungus could bypass regulations governing transport of transgenic products across international borders, although trans-border movement by humans would be virtually certain, irrespective of regulations, at least if the product seems to work. Keeping costs low will also be contingent on ultimate formulation (spores provided as granules, suspended in oil or as an impregnated rolled sheet in a vacuum bag) and application (as sprays, in bait stations, applied to surfaces), and hence duration of efficacy, as well as the ability to distribute the product through existing delivery channels. This exemplifies how all of the elements of a TPP are interconnected and contingent on each other (Figure 1).
This review covers the significant progress in the field of transgenic based mosquito control during the last few years. There is every reason to believe that, if given the opportunity, various methodologies founded on diverse strategies for effective and safe anopheline control, have the potential to be transformative for public health. However, to date only a self-limiting transgenic fungus is close to field ready (having been tested in a “semi-field’ MosquitoSphere). Testing a very broad array of engineering strategies is consistent with the highly exploratory approach required for optimizing mosquito control potential, and also with a need to be flexible in light of regulatory constraints that may permanently stymie some approaches. It is also unlikely that there will be a single strategy that is optimal in all conditions, and the collective goal of scientists working in the field of mosquito control is to provide a wide range of options and resources that different communities can exploit according to their circumstances.
The biggest challenge raised in this review, and many other forums, is how do we give mosquito control strategies the appropriate regulation required for safe use, and deploy them in ways that will be acceptable to the world? The possibility that self-sustaining technologies could soon be tested outside the laboratory has increased political sensitivity, and the fate of the technology is in balance. A movement for a full ban on gene drives gained momentum at the run up to the 2018 Conference of the Parties to the Convention on Biological Diversity. Representatives of a number of African nations, concerned about their citizens health, joined with scientists to say that a moratorium would be wrong. Opposition may grow again if a gene-drive experiment, or perhaps any other transgenic technology, is perceived to have gone wrong. However, while gene drive mosquitoes will be the likely test case for public acceptance of gene drive technology, mosquito research does not operate in a vacuum. The whole field of synthetic biology, with its aspiration to revolutionize the way we work with biology, is addressing the same social and political issues (88). The issues being the same, so are the proposed solutions: scientists to engage with the public, listen to alternative viewpoints, adapt their ideas and be as transparent as possible about their work and intentions (88). The view is that everyone on Earth is a stakeholder, and so all views are valid and should be considered. This is a populist credo but the devil is in the details for how to make it work in practice e.g., will extra credence in decision making be given to professional expertise? Also, of course, some scientists are not well adapted to public engagement, and decades of social science has shown that knowledge level accounts for a very small amount of variance in public attitude toward new technology. It is however probable that public opinion in most of Africa is not yet set on the issue of GE organisms, and their acceptance, if it occurs, will depend at least in part on the “trustworthiness” of the information source. Local scientists, activist groups and governments may be trusted for different reasons. The WHO (2014) stated that “decision-making bodies approving biosafety testing should have the capacity to formulate the risk problem, define appropriate end points for risk, interpret the character of risk components and understand the efficacy and uncertainty related to proposed measures” (16). For science to be embedded in society requires that these decision-making bodies provide a ‘pluralistic accountability system for world politics’ (89). As seen during the covid pandemic it is not easy to take complicated scientific issues into governmental institutions, democratic or otherwise, and get the best solutions to the world’s problems. Lay people from all parts of the world serving on a decision-making body for new technologies would need to become experts to interpret information intelligently, to question power holders, and to accommodate disagreement and uncertainty. Helping to inspire such a global accountability system would be a remarkable acknowledgement of the impact of mosquitoes and their associated transgenic technologies, but we are clearly not there yet.
The author confirms being the sole contributor of this work and has approved it for publication.
This material is based upon work jointly supported by the Plant Biotic Program of the National Science Foundation and the USDA National Institute of Food and Agriculture under grant no. DEB 1 911 777, Hatch project accession no. 1015969 from the USDA National Institute of Food and Agriculture, as well as grants from the National Institutes of Health (grant number RO3-AI3575 and grant number RO1-AI106998).
The author declares that the research was conducted in the absence of any commercial or financial relationships that could be construed as a potential conflict of interest.
All claims expressed in this article are solely those of the authors and do not necessarily represent those of their affiliated organizations, or those of the publisher, the editors and the reviewers. Any product that may be evaluated in this article, or claim that may be made by its manufacturer, is not guaranteed or endorsed by the publisher.
1. Greely. Combating Malaria by Modifying Mosquitoes Could Save Thousands of Lives. It’s Also Risky (2019). Washington Post. Available at: https://www.washingtonpost.com/outlook/2019/02/05/combating-malaria-by-modifying-mosquitoes-could-save-thousands-lives-its-also-risky/ (Accessed 10/18/2021).
2. Raghavendra K, Barik TK, Reddy BP, Sharma P, Dash AP. Malaria Vector Control: From Past to Future. Parasitol Res (2011) 108:757–79. doi: 10.1007/s00436-010-2232-0
3. WHO. World Malaria Report (2020). Available at: https://www.who.int/publications/i/item/9789240015791.
4. Enayati A, Hemingway J. Malaria Management: Past, Present, and Future. Annu Rev Entomol (2010) 55:569–91. doi: 10.1146/annurev-ento-112408-085423
5. Trape JF, Tall A, Diagne N, Ndiath O, Ly AB, Faye J, et al. Malaria Morbidity and Pyrethroid Resistance After the Introduction of Insecticide-Treated Bednets and Artemisinin-Based Combination Therapies: A Longitudinal Study. Lancet Infect Dis (2011) 11:925–32. doi: 10.1016/S1473-3099(11)70194-3
6. Leftwich PT, Bolton M, Chapman T. Evolutionary Biology and Genetic Techniques for Insect Control. Evolutionary Appl (2015) 9(1):212–30. doi: 10.1111/eva.12280
7. Mumford JD, Carrasco LR. Economics of Transgenic Insects for Field Release. In: Benedict MQ, editor. Transgenic Insects. Wallingford, UK: CABI (2014). p. 306–18.
8. Lovett B, Bilgo E, Diabate A, St. Leger RJ. A Review of Progress Toward Field Application of Transgenic Mosquitocidal Entomopathogenic Fungi. Pest Manage Science (2019) 75:2316–24. doi: 10.1002/ps.5385
9. Craig GB, Hickey WA, VandeHey RC. An Inherited Male-Producing Factor in Aedes Aegypti. Science (1960) 132(3443):1887–9. doi: 10.1126/science.132.3443.1887
10. Eckhoff PA, Wenger EA, Godfray HCJ, Burt A. Impact of Mosquito Gene Drive on Malaria Elimination in a Computational Model With Explicit Spatial and Temporal Dynamics. Proc Natl Acad Sci (2017) 114(2):E255–64. doi: 10.1073/pnas.1611064114
11. Alphey LS, Crisanti A, Randazzo F, Akbari OS. Opinion: Standardizing the Definition of Gene Drive. Proc Natl Acad Sci. (2020) 117(49):30864–7. doi: 10.1073/pnas.2020417117
12. Reeves GR, Phillipson M. Mass Releases of Genetically Modified Insects in Area-Wide Pest Control Programs and Their Impact on Organic Farmers. Sustainability (2017) 9(1):59. doi: 10.3390/su9010059
13. Adelman ZN. “Demystifying the Risk Assessment Process for Laboratory-Based Experiments Utilizing Invasive Genetic Elements: It Is More Than Gene Drive”. In: Applied Biosafety. (2021) vol. 26. pp. 154–63. doi: 10.1089/apb.20.0074.
14. Pike A, Dong Y, Dizaji NB, Gacita A, Mongodin EF, Dimopoulos G. Changes in the Microbiota Cause Genetically Modified Anopheles to Spread in a Population. Science (2017) 357(6358):1396–9. doi: 10.1126/science.aak9691
15. Prasad R. GM Mosquito Trials to Control Dengue, Chikungunya Launched. Chennai, Tamil Nadu, India: The Hindu (2017). Available at: http://www.thehindu.com/sci-tech/health/GM-mosquito-trials-to-control-dengue-chikungunya-launched/article17093840.ece.
16. WHO. WHO Takes a Position on Genetically Modified Mosquitoes (2020). Available at: https://www.who.int/news/item/14-10-2020-who-takes-a-position-on-genetically-modified-mosquitoes (Accessed October 27, 2021).
17. Lovett B, Bilgo E, Millogo S, Ouattarra AK, Sare I, Gnambani E, et al. Transgenic Metarhizium Rapidly Kills Mosquitoes in a Malaria-Endemic Region of Burkina Faso. Science (2019) 364:894–7. doi: 10.1126/science.aaw8737
18. Hammond A, Pollegioni P, Persampieri T, North A, Minuz R, Trusso A, et al. Gene-Drive Suppression of Mosquito Populations in Large Cages as a Bridge Between Lab and Field. Nat Commun (2021) 12:4589. doi: 10.1038/s41467-021-24790-6
19. North AR, Burt A, Godfray HCJ. Modelling the Potential of Genetic Control of Malaria Mosquitoes at National Scale. BMC Biol (2019) 17:26. doi: 10.1186/s12915-019-0645-5
20. KaramiNejadRanjbar M, Eckermann KN, Ahmed HMM, Sánchez CHM, Dippel S, Marshall JM, et al. Consequences of Resistance Evolution in a Cas9-Based Sex-Conversion Suppression Gene Drive for Insect Pest Management. Proc Natl Acad Sci USA (2018) 115:6189–94. doi: 10.1073/pnas.1713825115
21. de Jong T. Gene Drives do Not Always Increase in Frequency: From Genetic Models to Risk Assessment. J Consum Prot Food Saf (2017) 12:299. doi: 10.1007/s00003-017-1131-z
22. Schmidt H, Collier TC, Hanemaaijer MJ, Houston PD, Lee Y, Lanzaro GC. Abundance of Conserved CRISPR-Cas9 Target Sites Within the Highly Polymorphic Genomes of Anopheles and Aedes Mosquitoes. Nat Commun (2020) 11:1425. doi: 10.1038/s41467-020-15204-0
23. Lanzaro GC, Sánchez C HM, Collier TC, Marshall JM, James AA. Population Modification Strategies for Malaria Vector Control are Uniquely Resilient to Observed Levels of Gene Drive Resistance Alleles. BioEssays (2021) 20:2000282. doi: 10.1002/bies.202000282
24. Bilgo E, Lovett B, Fang W, Bende N, King GF, Diabate D, et al. Improved Efficacy of an Arthropod Toxin Expressing Fungus Against Insecticide-Resistant Malaria-Vector Mosquitoes. Sci Rep (2017) 7:3433. doi: 10.1038/s41598-017-03399-0
25. Fang W, Vega-Rodriguez J, Ghosh AK, Jacobs-Lorena M, Khang A, St. Leger RJ. Development of Transgenic Fungi That Kill Human Malaria Parasites in Mosquitoes. Science (2011) 331:1074–7. doi: 10.1126/science.1199115
26. Ortiz-Urquiza A, Zhibing L, Keyhani NO. Improving Mycoinsecticides for Insect Biological Control. Appl Microbiol Biotechnol (2015) 99:1057–68. doi: 10.1007/s00253-014-6270-x
27. Wang S, Fang W, Wang C, St. Leger RJ. Insertion of an Esterase Gene Into a Specific Locust Pathogen (Metarhizium Acridum) Enables it to Infect Caterpillars. PloS Pathog (2011) 7(6):e1002097. doi: 10.1371/journal.ppat.1002097
28. St. Leger RJ. Insects and Their Pathogens in a Changing Climate. J Invertebrate Pathol (2021) 184. doi: 10.1016/j.jip.2021.107644
29. Wang JB, Lu HL, St. Leger RJ. The Genetic Basis for Variation in Resistance to Infection in the Drosophila Melanogaster Genetic Reference Panel. PloS Pathog (2017) 13(3):e1006260. doi: 10.1371/journal.ppat.1006260
30. Bilgo E, Lovett B, Bayili K, Millogo AS, Saré I, Dabiré RK, et al. Transgenic Metarhizium Pingshaense Synergistically Ameliorates Pyrethroid-Resistance in Wild-Caught, Malaria-Vector Mosquitoes. PloS One (2018) 13(9):e0203529. doi: 10.1371/journal.pone.0203529
31. WHO. History of Malaria Elimination in the European Region – Factsheet (2016). Available at: http://www.euro.who.int/data/assets/pdf_file/0003/307272/Facsheet-malaria-elimination.pdf (Accessed October 19, 2021).
32. National Academies of Sciences, Engineering, and Medicine. Preparing for Future Products of Biotechnology. Washington (DC: The National Academies Press (2017). doi: 10.17226/24605
33. Wareham C, Nardini C. Policy on Synthetic Biology: Deliberation, Probability, and the Precautionary Paradox. Bioethics (2015) 29:118–25. doi: 10.1111/bioe.12068
34. Wozniak CA, McClung G, Gagliardi J, Segal M, Matthews K. Regulation of Genetically Engineered Microorganisms Under FIFRA, FFDCA and TSCA. In: Wozniak CA, McHughen A (eds). Regulation of Agricultural Biotechnology. The United States and Canada. Dordrecht: Springer (2012). p. 57–94. doi: 10.1007/978-94-007-2156-2_4
35. Hu G, St. Leger RJ. Field Studies of a Recombinant Mycoinsecticide (Metarhizium Anisopliae) Reveal That it is Rhizosphere Competent. Appl Environ Microbiol (2002) 68:6383–7. doi: 10.1128/AEM.68.12.6383-6387.2002
36. Liao X, O’Brien T, Fang W, St. Leger RJ. The Plant Beneficial Effects of Metarhizium Species Correlate With Their Association With Roots. Appl Microbiol Biotechnol (2014) 98:7089–96. doi: 10.1007/s00253-014-5788-2
37. Redford KH, Brooks TM, Macfarlane NBW, Adams JS. (eds). Genetic Frontiers for Conservation: An Assessment of Synthetic Biology and Biodiversity Conservation. In: Technical Assessment. Gland, Switzerland: IUCN (2019).
38. EFSA Panel on Genetically Modified Organisms GMO, Naegeli H, Bresson JL, Dalmay T, Dewhurst IC, Epstein MM, et al. Adequacy and Sufficiency Evaluation of Existing EFSA Guidelines for the Molecular Characterization, Environmental Risk Assessment and Post-Market Environmental Monitoring of Genetically Modified Insects Containing Engineered Gene Drives. EFSA J (2020) 18:e06297. doi: 10.2903/j.efsa.2020.6297
39. Scudellari M. Self-Destructing Mosquitoes and Sterilized Rodents: The Promise of Gene Drives. Nature; (2019) 571:160–2. doi: 10.1038/d41586-019-02087-5
40. Glover B, Akinbo O, Savadogo M, Timpo S, Lemgo G, Sinebo W, et al. Strengthening Regulatory Capacity for Gene Drives in Africa: Leveraging NEPAD’s Experience in Establishing Regulatory Systems for Medicines and GM Crops in Africa. BMC Proc (2018) 12:11. doi: 10.1186/s12919-018-0108-y
41. Hartley S, Smith RDJ, Kokotovich A, Opesen C, Habtewold T, Ledingham K, et al. Ugandan Stakeholder Hopes and Concerns About Gene Drive Mosquitoes for Malaria Control: New Directions for Gene Drive Risk Governance. Malar J (2021) 20:149. doi: 10.1186/s12936-021-03682-6
42. Teem JL, Ambali A, Glover B, Ouedraogo J, Makinde D, Roberts A. Problem Formulation for Gene Drive Mosquitoes Designed to Reduce Malaria Transmission in Africa: Results From Four Regional Consultations 2016–2018. Malar J (2019) 18:347. doi: 10.1186/s12936-019-2978-5
43. Schwindenhammer S. The Rise, Regulation and Risks of Genetically Modified Insect Technology in Global Agriculture. Science Technol Soc (2020) 25:124–41. doi: 10.1177/0971721819890042
44. Evans BR, Kotsakiozi P, Costa-da-Silva AL, Ioshino RS, Garziera L, Pedrosa MC, et al. Transgenic Aedes Aegypti Mosquitoes Transfer Genes Into a Natural Population. Sci Rep (2019) 9:13047. doi: 10.1038/s41598-019-49660-6
45. Evans BR, Kotsakiozi P, Costa-da-Silva AL, Ioshino RS, Garziera L, Pedrosa MC, et al. Editorial Expression of Concern: Transgenic Aedes Aegypti Mosquitoes Transfer Genes Into a Natural Population. Sci Rep (2020) 10(1):5524. doi: 10.1038/s41598-020-62398-w
46. WHO. Guidance Framework for Testing of Genetically Modified Mosquitoes Vol. 41. . Geneva, Switzerland: WHO (2014).
47. CBD. Cartagena Protocol on Biosafety to the Convention on Biological Diversity: Text and Annexes. Montreal, Canada: CBD (2000).
48. CBD. Guidance on Risk Assessment of Living Modified Organisms and Monitoring in the Context of Risk Assessment. UNEP/CBD/BS/COP-MOP/8/8/Add.1. Montreal, Canada: CBD (2016).
49. Marshall J. The Cartagena Protocol and Genetically Modified Mosquitoes. Nat Biotechnol (2010) 28:896–7. doi: 10.1038/nbt0910-896
50. Medlock JM, Hansford KM, Schaffner F, Versteirt V, Hendrickx G, Zeller H, et al. A Review of the Invasive Mosquitoes in Europe: Ecology, Public Health Risks, and Control Options. Vector Borne Zoonotic Dis (2012) 12:435–47. doi: 10.1089/vbz.2011.0814
51. Greenbaum G, Feldman MW, Rosenberg NA, Kim J. Designing Gene Drives to Limit Spillover to non-Target Populations. PloS Genet (2021) 17(2):e1009278. doi: 10.1371/journal.pgen.1009278
52. Ellstrand N. “Born to Run”? Not Necessarily: Species and Trait Bias in Persistent Free-Living Transgenic Plants. Front Bioeng Biotechnol (2018) 6:88. doi: 10.3389/fbioe.2018.00088
53. Moro D, Byrne M, Kennedy M, Campbell S, Tizard M. Identifying Knowledge Gaps for Gene Drive Research to Control Invasive Animal Species: The Next CRISPR Step. Glob Ecol Conserv (2018) 13:e00363. doi: 10.1016/j.gecco.2017.e00363
54. ZaplEtal J, Najmitabrizi N, Erraguntla M, Lawley MA, Myles KM, Adelman ZN. Making Gene Drive Biodegradable. Philos Trans R Soc Lond B Biol Sci (2021) 376(1818):20190804. doi: 10.1098/rstb.2019.0804
55. Lukindu M, Bergey CM, Wiltshire RM, Small ST, Bourke BP, Kayondo JK, et al. Spatio-Temporal Genetic Structure of Anopheles Gambiae in the Northwestern Lake Victoria Basin, Uganda: Implications for Genetic Control Trials in Malaria Endemic Regions. Parasites Vectors (2018) 11:246. doi: 10.1186/s13071-018-2826-4
56. Lanzaro GC, Campos M, Crepeau M, Cornel A, Estrada A, Gripkey H, et al. Selection of Sites for Field Trials of Genetically Engineered Mosquitoes With Gene Drive. Evolutionary Appl (2021) 142147–:2161. doi: 10.1111/eva.13283
57. Brown KV. Why DARPA is Investing Big in Gene Drives (2017). Gizmodo. Available at: https://gizmodo.com/why-darpa-is-investing-big-in-gene-drives-1821028638 (Accessed November 3, 2021).
58. Taxiarchi C, Beaghton A, Don NI, Kyrou K, Gribble M, Shittu D, et al. A Genetically Encoded Anti-CRISPR Protein Constrains Gene Drive Spread and Prevents Population Suppression. Nat Commun (2021) 12:3977. doi: 10.1038/s41467-021-24214-5
59. Noble C, Min J, Olejarz J, Buchthal J, Chavez A, Smidler AL, et al. Daisy-Chain Gene Drives for the Alteration of Local Populations. Proc Natl Acad Sci (2019) 116(17):8275–82. doi: 10.1073/pnas.1716358116
60. Fang W, Vega-Rodriguez J, Ghosh AK, Jacobs-Lorena M, Khang A, St. Leger RJ. Development of Transgenic Fungi That Kill Human Malaria Parasites in Mosquitoes. Science (2011) 331:1074–7. doi: 10.1126/science.1199115
61. Wang S, O’Brien T, Pava-Ripoll M, St. Leger RJ. Local Adaptation of an Introduced Transgenic Insect Fungal Pathogen Due to New Beneficial Mutations. Proc Natl Acad Sci USA (2011) 108:20449–54. doi: 10.1073/pnas.1113824108
62. Zhang Q, Chen X, Guo N, Meng Y, St. Leger RJ, Fang W. Horizontal Gene Transfer Allowed the Emergence of Broad Host Range Entomopathogens. Proc Natl Acad Sci (2019) 116:7982–9. doi: 10.1073/pnas.1816430116
63. Fang W, St. Leger RJ. Enhanced UV Resistance and Improved Killing of Malaria Mosquitoes by Photolyase Transgenic Entomopathogenic Fungi. PloS One (2012) 7(8):e43069. doi: 10.1371/journal.pone.0043069
64. Zhang DH, Lu HL, Liao X, St. Leger RJ, Nuss DL. Simple and Efficient Recycling of Fungal Selectable Marker Genes With the Cre-loxP Recombination System via Anastomosis. Fungal Genet Biol (2013) 61:S1087–1845(13)00153-9. doi: 10.1016/j.fgb.2013.08.013
65. Ridley M. Britain Should Adopt the Innovation Principal (2017). Available at: https://www.rationaloptimist.com/blog/the-innovation-principle/ (Accessed 9/14/2021).
66. Galaz V. Global Environmental Governance, Technology and Politics. In: The Anthropocene Gap. Cheltenham, UK: Edward Elgar (2014).
67. Rudenko L, Palmer MJ, Oye K. Considerations for the Governance of Gene Drive Organisms. Pathog Global Health (2018) 112:162–81. doi: 10.1080/20477724.2018.1478776
68. Achenbach J. “Gene Drive” Research to Fight Diseases can Proceed Cautiously, U.N. Group Decides (2018). The Washington Post. Available at: https://www.washingtonpost.com/science/2018/11/30/gene-drive-research-fight-diseases-can-proceed-cautiously-un-group-decides/ (Accessed 10/18/2021).
69. Kuzma J. Procedurally Robust Risk Assessment Framework for Novel Genetically Engineered Organisms and Gene Drives. Regul Governance (2021) 15:1144–65. doi: 10.1111/rego.12245
70. Thizy D, Pare Toe L, Mbogo C, Matoke-Muhia D, Alibu VP, Kathleen Barnhill-Dilling S, et al. Proceedings of an Expert Workshop on Community Agreement for Gene Drive Research in Africa - Co-Organised by KEMRI, PAMCA and Target Malaria [Version 2; Peer Review: 2 Approved]. Gates Open Res (2021) 5:19. doi: 10.12688/gatesopenres.13221.2
71. Marris C. The Construction of Imaginaries of the Public as a Threat to Synthetic Biology. Sci Culture (2015) 24(1):83–98. doi: 10.1080/09505431.2014.986320
72. Vitale JD, Vognan G, Ouattarra M, Traore O. The Commercial Application of GMP Crops in Africa: Burkina Faso’s Decade of Experience With Bt Cotton. AgBioForum (2010) 13:320–32.
73. Gakpo O. Burkina Faso Cotton Industry Wants to Bring Back GMO Seeds (2018). Available at: https://allianceforscience.cornell.edu/blog/2018/02/burkina-faso-cotton-industry-wants-bring-back-gmo-seeds/ (Accessed 10/19/2021).
74. Gakpo O. Burkina Faso is Moving Forward With GMP Research (2018). Available at: https://allianceforscience.cornell.edu/blog/2018/01/burkina-faso-is-moving-forward-with-gmo-research/ (Accessed 10/18/2021).
75. Blancke S, van Breusegem F, De Jaeger G, Braeckman J, Van Montagu M. Fatal Attraction: The Intuitive Appeal of GMO Opposition. Trends Plant Sci (2015) 20:414–8. doi: 10.1016/j.tplants.2015.03.011
76. Available at: http://www.etcgroup.org/content/gene-drive-files (Accessed 10/19/2021). Press release dated December 4, 2017.
77. Kahan DM, Peters E, Wittlin M, Slovic P, Ouellette LL, Braman D, et al. The Polarizing Impact of Science Literacy and Numeracy on Perceived Climate Change Risks. Nat Climate Change (2012) 2(10):p.732. doi: 10.1038/nclimate1547
78. Kornberg M. It Isn't Just What You Say About Science; It's Also How You Say it (2021). Scientific American. Available at: https://www.scientificamerican.com/article/it-isnt-just-what-you-say-about-science-its-also-how-you-say-it/ (Accessed 10/19/2021). Opinion Peace September 17.
79. Federici BA. Bacteria as Biological Control Agents for Insects: Economics, Engineering and Environmental Safety. In: Vurro M, Gressel J, editors. Novel Biotechnologies for Biocontrol Agent Enhancement and Management. Berlin: Springer (2007). p. 25–52.
80. Intrexon. Oxitec – A Pioneer in Biological Insect Control Solutions (2017). Available at: https://www.dna.com/Company/Subsidiaries/Oxitec (Accessed 10/19/2021).
81. Oxitec. Paper Provided to the House of Lords Select Committee on Science and Technology Call for Evidence on Genetically Modified Insects. Written Evidence (GMI0016) (2015). Available at: http://www.parliament.uk/documents/lords-committees/science-technology/GMInsects/GMInsectsevidence.pdf (Accessed 10/19/2021).
82. Mitchell PD, Brown Z, McRoberts N. Economic Issues to Consider for Gene Drives. J Responsible Innovation (2018) 5:sup1:S180–202. doi: 10.1080/23299460.2017.1407914
83. Regalado A. Farmers Seek to Deploy Powerful Gene Drive. MIT Technology Review (2017). Available at: https://www.technologyreview.com/s/609619/farmers-seek-to-deploy-powerful-gene-drive/ (Accessed November 3, 2021).
84. Iwanicki NS, Pereira AA, Botelho ABRZ, Rezende JM, Moral RDA, Zucchi MA, et al. Monitoring of the Field Application of Metarhizium Anisopliae in Brazil Revealed High Molecular Diversity of Metarhizium Spp in Insects, Soil and Sugarcane Roots. Sci Rep (2019) 9:4443. doi: 10.1038/s41598-019-38594-8
85. Jaronski S, Mascarin GM. Mass Production of Fungal Entomopathogens. In: Lacey LA, editor. Microbial Control of Insect and Mite Pests From Theory to Practice. Yakima, WA: IP Consulting International (2016). p. 141–55. doi: 10.1016/B978-0-12-803527-6.00009-3
86. Bateman R. Production Processes for Anamorphic Fungi. IPARC, Silwood Park: Ascot, Berks (2007). Available at: http://www.dropdata.net/mycoharvester/Mass_production.PDF.
87. Zimmermann G. Review on Safety of the Entomopathogenic Fungus Metarhizium Anisopliae. Biocontrol Sci Technol (2007) 17:879–920. 11–15. doi: 10.1080/09583150701593963
88. Gallup O, Ming H, Ellis T. Ten Future Challenges for Synthetic Biology. Eng Biol (2021) 5:1–9. doi: 10.1049/enb2.12011
Keywords: mosquito control, malaria, disease vector, gene drive technology, cartagena protocol, risk assessment, field trials, targeted product profile
Citation: St. Leger RJ (2021) From the Lab to the Last Mile: Deploying Transgenic Approaches Against Mosquitoes. Front. Trop. Dis 2:804066. doi: 10.3389/fitd.2021.804066
Received: 28 October 2021; Accepted: 02 December 2021;
Published: 22 December 2021.
Edited by:
Adam E. Vorsino, United States Fish and Wildlife Service, United StatesReviewed by:
Joshua Fisher, United States Fish and Wildlife Service, United StatesCopyright © 2021 St. Leger. This is an open-access article distributed under the terms of the Creative Commons Attribution License (CC BY). The use, distribution or reproduction in other forums is permitted, provided the original author(s) and the copyright owner(s) are credited and that the original publication in this journal is cited, in accordance with accepted academic practice. No use, distribution or reproduction is permitted which does not comply with these terms.
*Correspondence: Raymond J. St. Leger, c3RsZWdlckB1bWQuZWR1
Disclaimer: All claims expressed in this article are solely those of the authors and do not necessarily represent those of their affiliated organizations, or those of the publisher, the editors and the reviewers. Any product that may be evaluated in this article or claim that may be made by its manufacturer is not guaranteed or endorsed by the publisher.
Research integrity at Frontiers
Learn more about the work of our research integrity team to safeguard the quality of each article we publish.