- 1Infectious Diseases Division, Department of Medicine, Uniformed Services University, Bethesda, MD, United States
- 2Vector Molecular Biology Section, Laboratory of Malaria and Vector Research, National Institute of Allergy and Infectious Diseases, Rockville, MD, United States
- 3Ministry of Health, Fundacão Oswaldo Cruz, Eusébio, Brazil
- 4Office of the Dean, School of Army Aviation Medicine, Fort Rucker, AL, United States
- 5Biostatistics Service, Department of Research Programs, Walter Reed National Military Medical Center, Bethesda, MD, United States
Leishmania major, transmitted in Iraq by the bite of a sand fly Phlebotomus papatasi, causes cutaneous leishmaniasis (CL). The sand fly saliva is immunogenic, with both systemic humoral and cellular human immune responses resulting from natural exposure. 248 Americans who developed L. major infection in Iraq were sex, race/ethnicity, year of Iraq deployment-matched to controls without CL. Using a case-control study design, we compared sand fly saliva-specific human IgG levels and recognized antigens between the two groups. Serologic responses to Ph. papatasi salivary gland homogenate were studied with ELISA and Western blot, using serial samples obtained from before travel, during CL treatment (CL) or at time of return to US (controls), as well as (for CL cases) six to 24 months after return to non-endemic US. The mean change in optical density (MCOD), reflecting the change in sand fly saliva-specific IgG before and after exposure in Iraq, was 0.296 (range -0.138 to 2.057) in cases and 0.151 (range -0.454 to1.085) in controls, p<0.001. Low levels of sand fly saliva specific antibody were noted in CL cases by 7-8 months after return to the US. The most frequently recognized Ph. papatasi salivary antigens were MW30 (PpSP32) and MW64, although other salivary proteins recognized were MW12/14, 15, 18, 28, 32, 36, 42, 44, 46, 52. Logistic regression suggested that MW15, 28 and 42 were associated with the largest effect on the MCOD. MW30 was the most frequently recognized antigen suggesting a role as biomarker for sand fly exposure and CL risk. Anti-Ph. papatasi saliva IgG waned within months of return to the US. We also discuss vector antigenic saliva proteins in the context of CL presentation and identify some salivary antigens that may correlate with less lesion area, ulcer versus papule/plaque, race among those with CL.
Introduction
Cutaneous leishmaniasis (CL) affects more than 4 million people worldwide and more than a billion individuals live in endemic regions and are potentially at risk of infection (1, 2). Leishmania (L.) major parasites, transmitted by the sand fly vector Phlebotomus papatasi, in North Africa, Middle East, and Southwest Asia countries cause CL in some areas of highest incidence including Iraq (2, 3). Of the sand fly fauna in Iraq, Ph. papatasi, Ph. sergenti and Ph. alexandri represent the only abundant anthropophilic species reported from the country (4, 5), where they are considered the primary vectors of L. major, L. tropica, and L. infantum, respectively (4, 6). Since 2001, more than 2400 U.S. soldiers have been diagnosed with CL; L. major represented the etiology in >99% CL cases from Iraq exposure (7–9). Introducing many Ph. papatasi-unexposed individuals into the circumstances of rudimentary shelter during the first year of combat in endemic Iraq, uniquely allows investigation of the role of human immune responses to vector saliva upon the risk and presentation of CL. With every bite, the sand fly probes and injects some of its saliva; a substance which has been found to be very immunogenic in human participants (10–14). Seminal observations (15, 16) have demonstrated that mice initially immunized with sand fly saliva (no parasite), as well as select molecules of saliva such as SP15 (17, 18), had subsequent clinical disease that was markedly ameliorated after challenge with Leishmania-infected sand fly bites.
Transcriptomic analysis of secreted Ph. papatasi saliva established that a limited number of secreted proteins are present, most with no known human homologue (14). Sand fly saliva has effects on immune responses, inflammation, and hemostasis which are critical to the successful blood feeding by the sand fly and to establishment of Leishmania infection in the skin (19). The L. major protective salivary protein(s) have not been completely identified, although Th1 like cellular immune responses seem essential for protection (14, 16, 20, 21). Sand fly protein SP32 (molecular weight~30 kDa) was found to be the immunodominant target of Ph. papatasi human antibody responses in Tunisia (12); this protein is similar to a silk-related protein found in moths. However, among 23 American Iraq-deployed soldiers infected with Leishmania (species was not stated), an immunodominant antibody response to salivary proteins MW14 kDa and 38 kDa was reported (13).
Our objective was to study the antibody levels to Ph. papatasi salivary antigens in a case control cohort using sera of U.S. soldiers with Iraq-acquired CL compared to their own serum antibody profile before deployment versus a matched race, sex, Iraq-deployed, no CL diagnosis, control group. We also sought to identify recognized salivary antigens associated with CL protection or severity. In addition, because of our referral leishmaniasis treatment center, detailed clinical and epidemiologic factors were available for correlation with immune responses to vector saliva in the CL cohort, as well as access to serial serum samples banked in the Department of Defense serum repository (DoDSR). This allows analysis of clinical outcomes and CL risk factors in comparison to the individuals’ immune response to Ph. papatasi salivary antigens and permits a novel longitudinal assessment of this immune response.
Materials and Methods
Study Design
This case-control study was designed to test serum samples from American soldiers who were deployed to Iraq from 2003-2004, and did (case) or did not (control) develop confirmed L major CL [by culture or polymerase chain reaction (PCR)] (Figure 1). Species of cultured parasites was determined using isoenzyme electrophoresis (22) and molecular testing for L. major was performed with the Smart-Leish PCR (23). Control sera were obtained from sex-, and race- matched soldiers who were deployed to Iraq during the same period, had no prior deployment to leishmaniasis endemic countries, and did not have a CL diagnosis listed in the Defense Medical Surveillance System. No additional information was available for the controls. The study received Institutional Review Board approval (WU01-19002) at Walter Reed Army Medical Center, the Walter Reed National Military Medical Center, and the Uniformed Services University. CL participants provided written informed consent and the matched control sera were provided in a de-identified fashion. CL cases were selected from participants with parasitologically confirmed L. major enrolled in a leishmaniasis treatment trial at Walter Reed Army Medical Center, Washington D.C. who completed a 30-question detailed leishmaniasis risk factor survey (template provided in Supplemental Materials), provided blood samples, and reported no lifelong prior travel to leishmaniasis endemic areas. The survey probed for demographic information including occupation, dates of deployment, night activities and attire, use of personal protective measures (repellent; military brand versus commercial, frequency, night application), permethrin treatment of uniforms, bednet, exposure to biting insects.
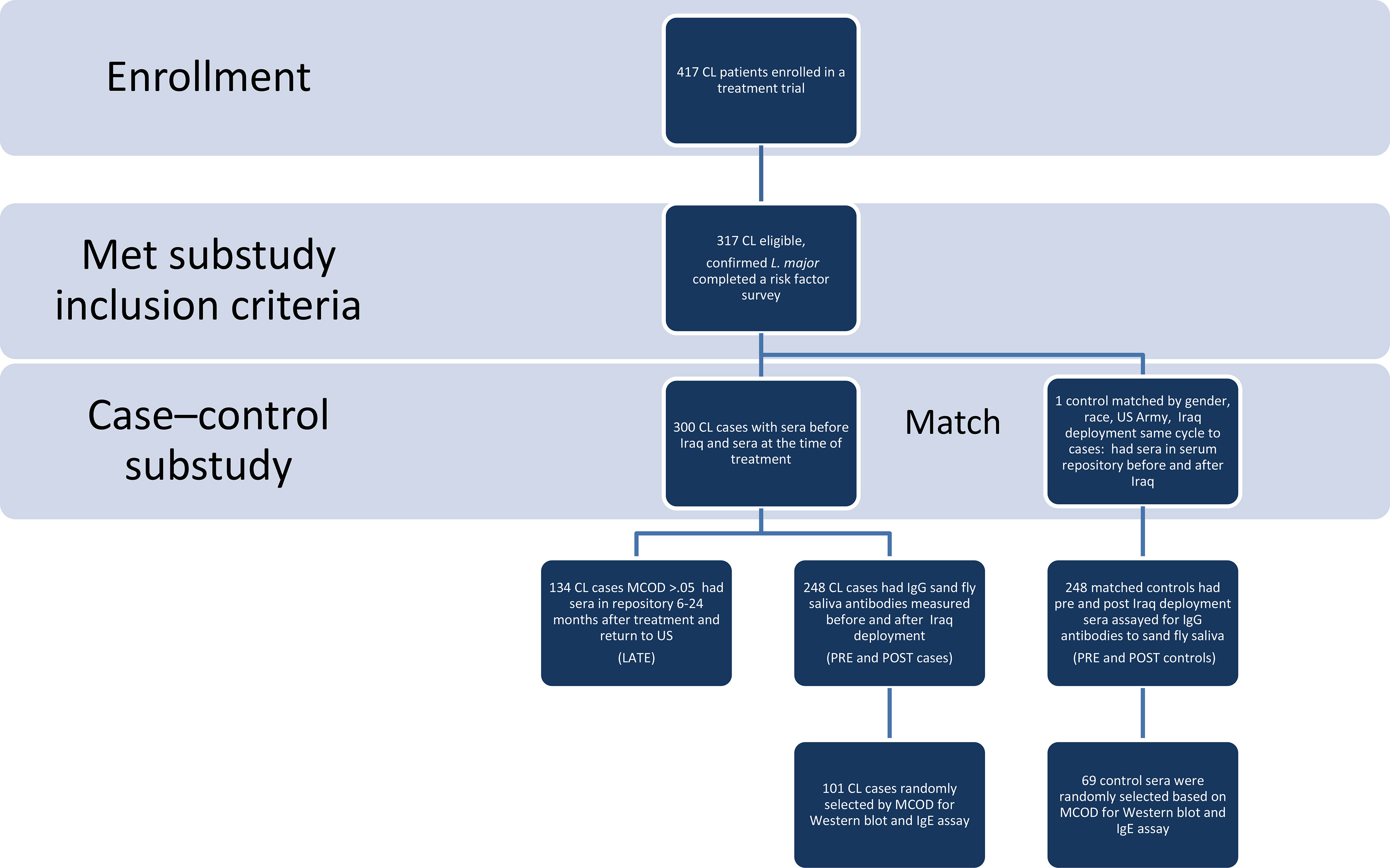
Figure 1 Study Flow Diagram. Using a study flow diagram, the eligibility and matching criteria used, the number of cases and controls at each phase of analysis are clearly delineated. CL, cutaneous leishmaniasis, MCOD is mean change in optical density of IgGs to Ph. papatasi saliva pre to post.
Clinical information, risk factor data, and demographics were obtained in the context of the CL treatment protocol. The same individual’s sera (controls and cases) banked prior to Iraq deployment (pre) were obtained from the Department of Defense Serum Repository, as were post-deployment sera for control samples (post), and later matched serum samples for CL cases at 6-24 months after deployment (late). The sera were tested for antibodies against salivary proteins of the main CL vector in Iraq, Ph. papatasi. Previous studies have demonstrated that salivary proteins of Ph. papatasi and Ph. duboscqi from different geographical areas are highly conserved (24–26). As such, we used a colonized Israeli strain of Ph. papatasi that originated from the Middle East to test the immune response in American soldiers exposed to vector bites in Iraq. All samples were tested in a blinded fashion at the Vector Molecular Biology section, National Institutes of Allergy and Infectious Disease; the laboratory was unaware of pre- and post-deployment or case/control status. For the Western blot analysis of specific parasite proteins, 170 samples were randomly selected from strata based on the mean change of optical density (MCOD) for both controls and cases: MCOD 0 – 0.25 (31 controls and 31 cases), >0.25 – 0.5 (22 controls and 19 cases), >0.5 -1.0 (14 controls and 20 cases) and greater than 1.0 (2 controls and 31 cases). Additionally, total serum IgE was measured in the Western blot subcohort, using ImmunoCAP IgE assay (Phadia U.S., Portage MI).
Sand Flies and Salivary Gland Homogenate (SGH)
Ph. papatasi Israeli strain sand flies were reared at the Walter Reed Army Institute of Research and at the Laboratory of Malaria and Vector Research, National Institutes of Allergy and Infectious Diseases (NIAID), as previously described (17). Salivary glands were dissected from 5 to 7-day-old females and stored in PBS at -70°C. Immediately before use, salivary glands were disrupted by ultrasonication in 1.5ml tubes, then centrifuged at 12,000x g for 3 min and the resultant supernatant (salivary gland homogenate - SGH) was used for different assays.
Analysis of Anti-Saliva Antibodies by ELISA and Western Blot
From pools of 50 pairs of sand fly salivary glands, we prepared a homogenate and measured the protein concentration by bicinchoninic acid method at 1.35 ug of protein/pair (Pierce™, BCA Protein Assay Kit, Thermofisher). ELISA microplates (Immunolon 4HB-Thermo) were coated equivalent to one pair of salivary glands per 1 mL of coating buffer (NaHC03 0.45 M, Na2C03 0.02 M, pH 9.6) and left overnight at 4°C. The concentration was 67.5ng/well in 50 microliters.
After a washing step with PBS-Tween 0.01%, wells were blocked with PBS-BSA 4% for 2 hours at room temperature (RT). Fifty microliters of sera (1:100 dilution) in PBS-BSA 4% was added to the wells and incubated for 1 hour at 37°C. After another washing cycle, wells were incubated with alkaline phosphatase conjugated anti-human IgG antibody (A-1543, Anti-human IgG [whole molecule] alkaline phosphatase conjugate, Sigma) (1:5000 dilution) in PBS-BSA 4%, for 1 hour at RT then sample wells were developed with p-nitrophenyl phosphate substrate (Sigma) for 45 minutes at RT. The absorbance was recorded at 405nm.
SDS-PAGE and Western blot analysis of Ph. papatasi SGH was performed as previously described (17). Briefly, SDS-PAGE was performed by electrophoresis of SGH equivalent to 10 pairs of Ph. papatasi (13.5 ug of protein) per Tris-Glycine gel (4-20%, 1.0 mm 2D well) (Invitrogen). The gel was transferred to a nitrocellulose membrane and blocked overnight with blocking buffer (TBS-Tween 0.05%-nonfat dry milk 5%), then incubated with each individual post-deployment serum (1:500 dilution) in blocking buffer for 3 hours at RT. After washes with TBS-Tween 0.05%, we incubated the membrane with 1:2000 dilution of alkaline phosphatase conjugated anti-human IgG antibody (A-1543, Anti-human IgG [whole molecule] alkaline phosphatase conjugate, Sigma) in blocking buffer for 45 minutes at RT. Bands were visualized by adding alkaline-phosphatase substrate (Promega). Analysis of digitalized membrane images allowed the identification of the reactive salivary proteins and their estimated molecular weights. The molecular weight used was SeeBlue™ Plus2 Pre-Stained Standard (Invitrogen) and the software was the Kodak 1D Image Analysis Software (Kodak) as part of the Kodak Electrophoresis Documentation and Analysis System 290.
Statistical Analysis
The primary outcome, mean change of optical density (MCOD), was calculated as the post deployment optical density minus the pre deployment optical density. This outcome was used in all statistical analyses in order to take into account the individual’s baseline value. In a clinical setting, it is most likely that only the post exposure optical density would be available, and the descriptive figures present this actual post deployment OD.
To explore the diagnostic accuracy of the post deployment OD, the 95th percentile value for controls was used as a cutoff: values greater than this threshold indicated disease and values lower than or equal to the threshold indicated no disease. The false positive rate was defined as the proportion of controls with values above the threshold, and the true positive rate was defined as the proportion of cases with values above the threshold.
Normality was examined using the Wilk-Shapiro test, and ordinal or continuous data which were not normally distributed were presented as medians with ranges. Groups were compared using the Wilcoxon rank sum test or Kruskal Wallis analysis of variance, while pre to post changes were examined using the Wilcoxon signed ranks test. The association of MCOD with continuous or ordinal data was described using Spearman’s rank correlation coefficient (rs).
Comparison of the IgG to recognized salivary proteins in cases and controls was examined using conditional logistic regression (procedure ‘clogit’ in STATA). Multivariate analysis comparing MCOD between cases and controls was examined using the same methodology; date of enrollment was also included as an independent variable in this model. Results from the conditional logistic regression analyses were presented using odds ratios (OR) together with 95% confidence intervals (95% CI). The association of the recognized salivary proteins was described using the kappa statistic (κ),
Factors associated with the total lesion area (summed areas of all lesions) were explored using linear regression analysis, using the natural log of the total lesion area as the dependent variable. Univariate comparisons between lesion area and each of the molecular weight antigens, MCOD, age, race, and date enrolled were initially examined, and variables with an association of p<0.20 were entered in the multivariate analysis. For each independent variable in the final model, standardized beta coefficients (β) are presented.
Data were described and analyzed using IBM SPSS Statistics for Windows (v 20.0, Armonk, NY: IBM Corp.). STATA (v.12, College Station, TX: StataCorp LP.), procedure ‘clogit’, was used only for the conditional logistic regression models described above.
Results
Study Population
The study population characteristics are described in Table 1, comprising a mainly young (median age 25.6 years in the CL and 26.1 years in the control group), predominantly White male cohort. As previously published (27), there were two areas in Iraq where CL was mostly acquired by this military population, Diyala and Ninewa governorates (specifically Tal Afar). Consistent with entry requirements for military service, these were healthy soldiers. The matched control soldier group had serum obtained a median of 2.5 months later than the cases who were returned to the U.S. for treatment prior to the end of their combat deployment. Post-deployment serum IgE levels were similar between CL cases and controls (Supplemental Figure 1). There was no leishmaniasis risk factor or clinical information available for the matched de-identified control group; among those with cutaneous leishmaniasis (CL) the median onset of skin lesions was six months after arrival in Iraq, the median duration of CL was 3 months (range 1-8), 60% had ulcerative disease, and the median number of skin lesions was four (range 1-32).
The risk factor survey (CL cases only) showed multiple opportunities for exposure to sand fly bites. Half responded that they mainly slept outdoors, 20% inside a tent, and 23% in buildings. Of note, 45% of study respondents noted difficult or impossible accessibility to a military insect repellent during deployment and 25% responded that they didn’t use repellent because it was unavailable. Of those that used repellents, 54% reported using military issue DEET and 43% a commercially purchased repellent, and 65% limited the application of repellent to exposed skin.
Antibody Response to Sand Fly Salivary Proteins
Results of measurement of human serum antibodies to Ph. papatasi saliva are reported as optical density read at 405nm. The outcome variable of interest, the mean change of optical density pre–post Iraq deployment (MCOD), for cutaneous L. major infection cases and uninfected controls was 0.296 (range -.0.138 to 2.057) and 0.151 (range -0.454 to 1.085) p<0.001 respectively (Supplemental Figure 2). MCOD and post-deployment OD were highly correlated in both controls (rs=0.91, p<0.001) and cases (rs=0.98, p<0.001). Figure 2A provides OD405nm for CL versus control samples at pre-, post-deployment and (for CL only) late timepoints.
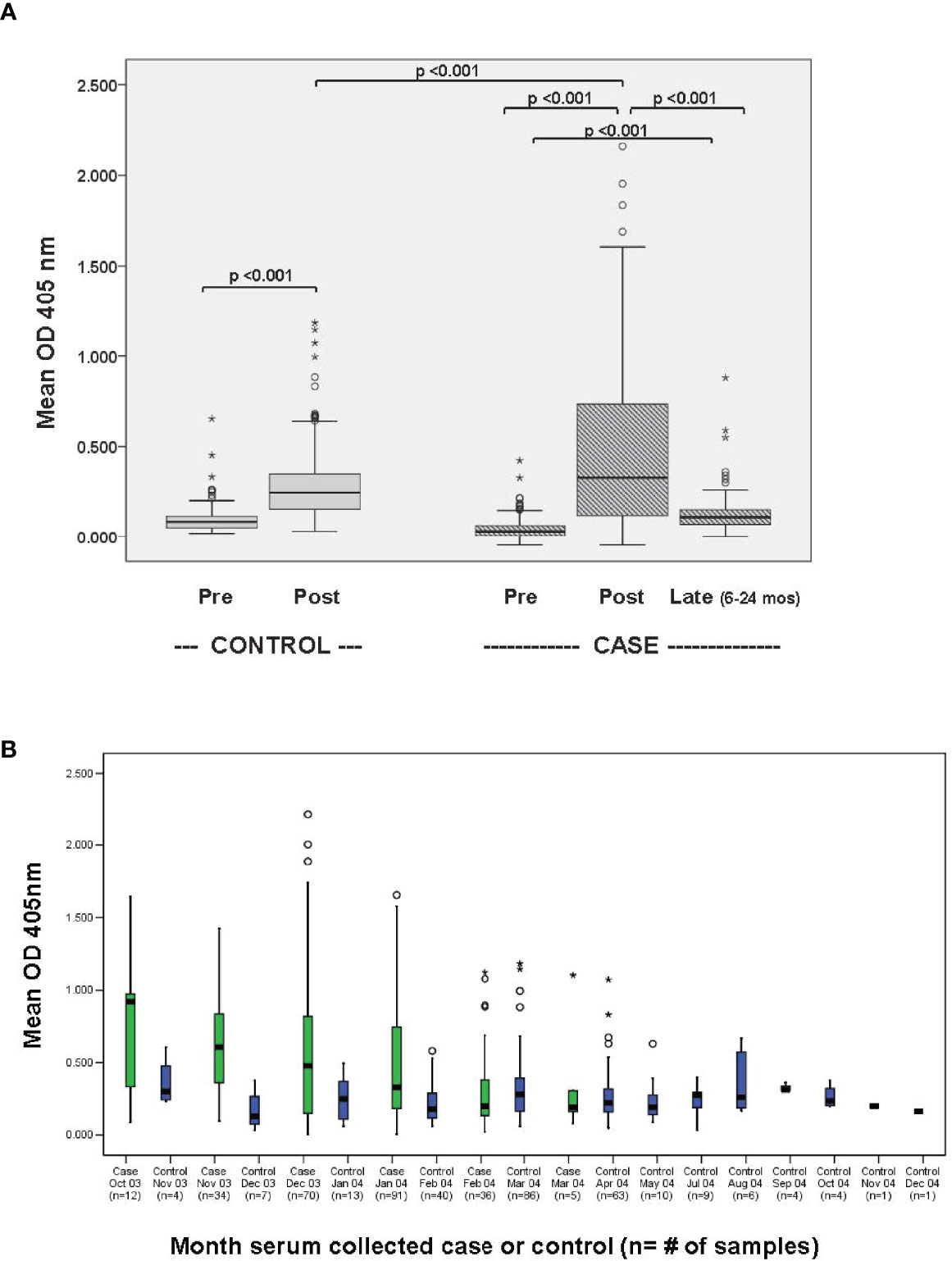
Figure 2 (A) Human antibody responses to Ph. papatasi salivary gland homogenate before and after deployment to Iraq. 248 sera from matched CL cases and deployed controls (2003-2004, first Iraq deployment cycle) were evaluated for IgG response to Ph. papatasi salivary gland homogenate by ELISA both before travel to Iraq (pre) and with the diagnosis of cutaneous leishmaniasis (CL, cases) or after re-deployment to the U.S (controls, deployed no CL). Additionally for those with CL, late (6-24 months) available banked sera, obtained after return to U.S., was analyzed to assess durability. (B) Mean post-deployment serum antibody levels to Ph. papatasi salivary gland homogenate: case versus control by month of blood draw. Month of obtained blood sample is shown in 189 people who arrived in Iraq February-April 2003 (pre-transmission season). CL “post” blood is drawn at the time of CL treatment and control “post” sera is obtained once the soldier rotated back to the U.S. Symbols: ○ is considered a potential outlier, the value being more than 1.5 but less than 3 interquartile range (IQR) above quartile 3, * is an extreme outlier, a value more than 3 above quartile 3.
Anti-Ph. papatasi Saliva IgG Increased in Iraq-Deployed Cases and Controls
Figure 2A demonstrates that the post-deployment sera show higher levels of antibody to sand fly saliva than the pre-deployment sera in both cases (p<0.001) and controls (p<0.001), though post-deployment levels are higher in the CL cases than controls (p<0.001), and that levels of antibody to Ph. papatasi saliva in a subset of 134 CL cases decreases within 6-24 months after return to the U.S.(p<0.001), an area not endemic for Ph. papatasi. From this data, we can define a cut-off value to identify people with Ph. papatasi saliva exposure in the near term. The 95th percentile upper limit for the OD405 in the 248 post-deployment control sera was 0.66: this cut off with an odds ratio of 9.7 (95% CI 5.0-18.8) yields a false positive rate of 4.4% and true positive rate of 31.0% for a case versus control predictive antibody response. The 95th percentile upper limit for the OD405 in the pre-deployment sera was 0.19 for the controls and for the cases.
Kinetics of anti-Ph. papatasi Saliva IgG Levels in Iraq-Deployed Cases and Controls
We limited our data set to the 189 soldiers arriving in Iraq during the pretransmission season (February through April) and we compared case versus control post-deployment antibody response by month of blood collection (Figure 2B). While case sera antibody levels dropped with time (rs= -0.31, p<0.001), the control sera antibody levels were lower and generally constant over the time period studied (rs=0.09, p=0.157). Case sera antibody levels were not significantly correlated with the length of the time period between arrival and enrollment (rs=-0.09, p=0.155).
Anti-Ph. papatasi Saliva IgG Levels Are Short-Lived
The distribution of reported CL lesion onset by month is consistent with an estimated 1-2 month incubation period after sand fly inoculation (Figure 3A). The arrival date in Iraq included 10% in February, 45% in March, 23% in April, and 9% in May 2003. The kinetic decline of antibody to sand fly salivary homogenate was explored in the 134 cases where late (6-24 month post-deployment) sera was available (Figure 3B). Estimating that sand fly transmission peaks in late summer (28), this figure shows a decline in serum antibody over time of blood collection after the transmission season (rs= -0.28, p<0.001) with low levels by 7-8 months.
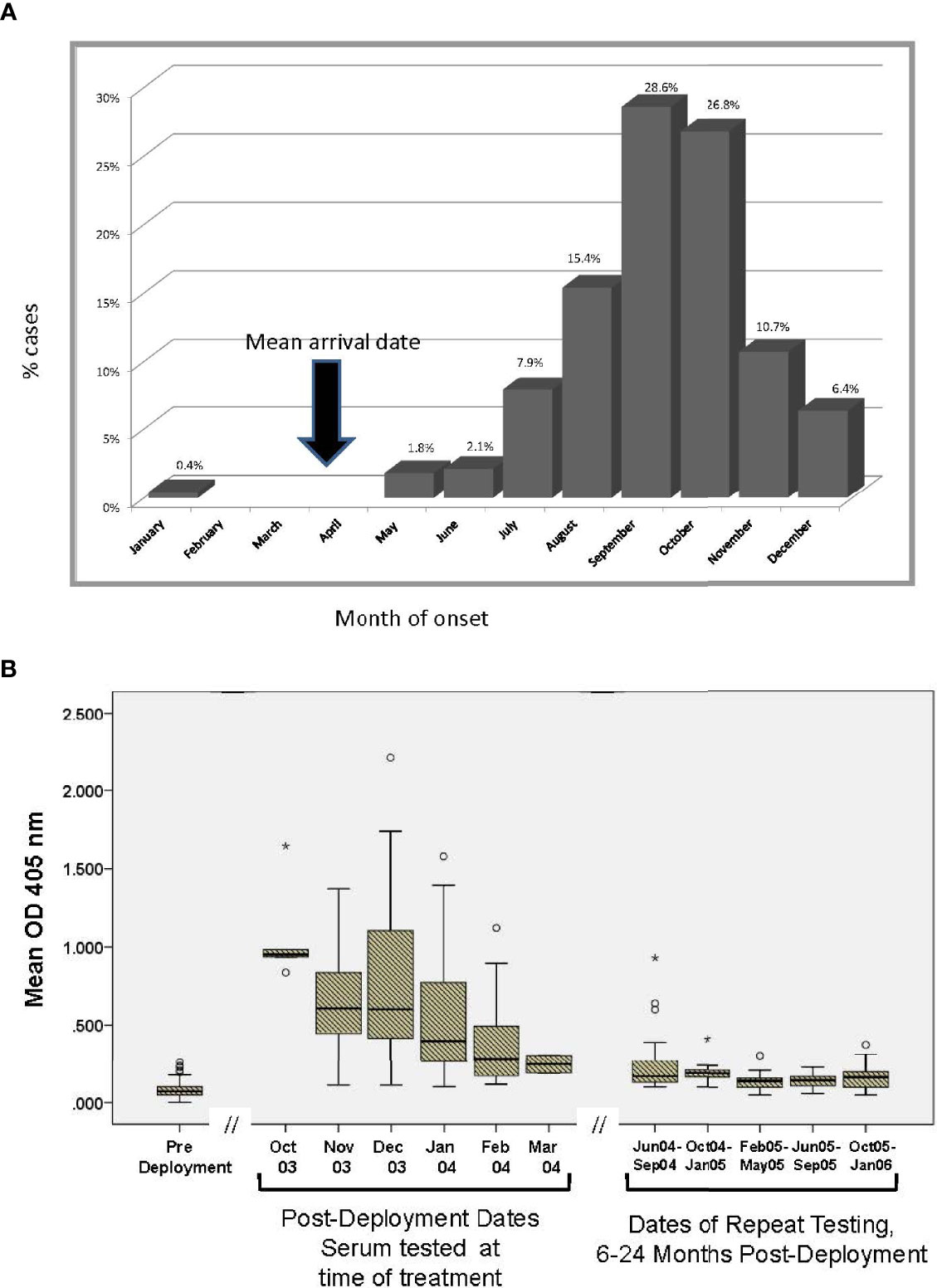
Figure 3 (A) Reported month of lesion onset among CL cases. As Ph. papatasi biting is seasonal in Iraq, vector salivary antibody responses can be interpreted in this context. On average, post sera in CL group was obtained 3.7 months after the lesion onset. The arrow represents the time most units arrived in Iraq during 2003. (B) Kinetics of antibody recognizing Ph. papatasi salivary antigens in 134 CL cases with serial blood samples. Subset of 134 CL where availability of banked sera at pre, post and late timepoints permitted assessment over time. Symbols: ○ is considered a potential outlier, the value being more than 1.5 but less than 3 interquartile range (IQR) above quartile 3, * is an extreme outlier, a value more than 3 above quartile 3.
Assessment of IgG MCOD, Demographic and Clinical Factors Among CL Cases
As seen in Supplemental Figure 2, a higher MCOD was associated with CL, p<0.001. Among CL cases, there was no statistically significant influence of length of potential exposure in Iraq or serum IgE levels on the MCOD but there was a modest effect of higher age associated with lower MCOD (rs=-0.13, p=0.04), and White race was associated with a higher MCOD compared to other race/ethnicity groups (p=0.032). Supplemental Table 1 shows the association of MCOD with some demographic and clinical factors in case versus control groups. In a conditional logistic regression model, MCOD was significantly different between groups (OR = 12.5, 95% CI: 5.8 to 26.9, p<0.001). Further, despite controlling for the observed effect of proximity of time of blood draw to transmission season in the conditional logistic regression model, the antibody responses among cases were still higher than controls (OR = 4.3, 95% CI: 1.3 to 14.5, p=0.019) and the observed effect of time of enrollment (MCOD decreasing over time) was significant (OR = 0.2, 95% CI: 0.1 to 0.3, p<0.001). Age was not significant in the model (OR=1.0, 95% CI: 0.9 to 1.0, p=0.897).
Clinical features among cases were explored to determine any association between IgG antibodies to saliva of Ph. papatasi, the vector of L. major in Iraq, and CL in this cohort. There was no statistically significant association of MCOD on the number, maximal size, ulceration, duration, or total area of CL skin lesions. It is noted that the serum was acquired at the time of enrollment into a CL treatment protocol and not prior to diagnosis, with the median time from reported onset to enrollment of 3.7 months.
Association of Use of Personal Protective Measures and MCOD in CL Cases
Correlating MCOD to information provided by the CL cases in the leishmaniasis risk factor survey shows some consistent patterns (Table 2). The MCOD was higher in those reporting use of any repellent (median MCOD: 0.343 vs. 0.168, p=0.029) as well as those using repellent at night (0.397 vs. -0.159, p=0.003). However, the MCOD was not significantly influenced when evaluated for the frequency of applying repellent once, twice, thrice, four times or more per day (rs=0.03, p=0.72), or when related to the recall of the frequency of insect bites per day (infrequent 0-49/day, frequent 50-99 bites/day, very frequent >100 times/day) (rs=0.11, p=0.095). The association of repellent and MCOD is still significant when examined using multivariate model to control for date of enrollment and age. Treating uniforms with permethrin (0.435 vs 0.263, p= 0.103), or using permethrin treated bednets (0.487 vs. 0.268, p=0.060) was not associated with MCOD and was not commonly done. Sleeping in the combat uniform (0.433 vs. 0.244, p=0.027) was associated with greater MCOD (Table 2); notably only 42 cases reported using permethrin treated uniforms.
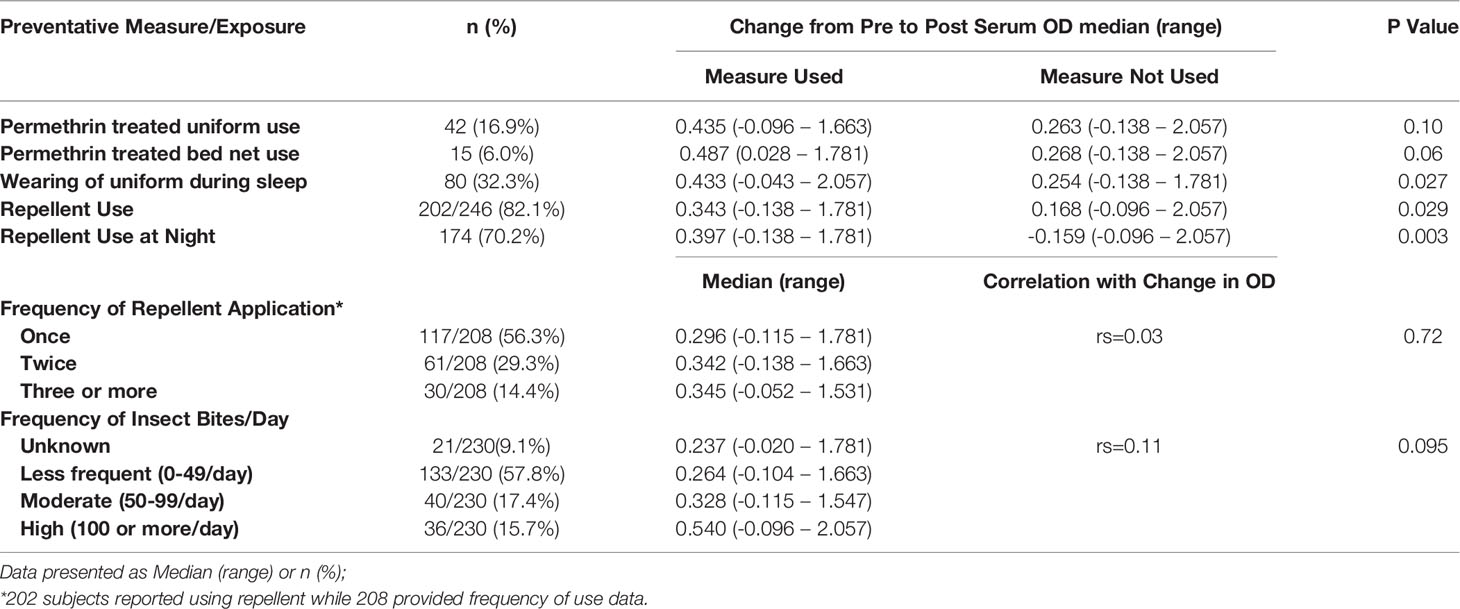
Table 2 Cases only: Association of preventative measures and exposure with change in antibody levels against sand fly salivary gland homogenate.
Sand Fly Salivary Antigens Recognized by Human Anti-Ph. papatasi Saliva Antibodies
Sand fly saliva is a complex antigenic/immunogenic substance. The molecular weights of the most abundant sand fly salivary proteins from the CL vector Ph. papatasi have been previously identified (14, 17, 29). We compared human serologic IgG responses to antigens in Ph. papatasi salivary gland homogenate using Western blot analysis in a stratified randomly selected subset of 101 cases and 69 controls (see Materials and Methods, Supplementary Material). This difference in sample size between case and control sera assayed with Western blotting is because the MCOD is not normally distributed in both cases and controls with most high MCODs found in the cases (see Supplemental Figure 2).
Ph. papatasi Salivary Antigens Recognized by CL and Control Sera
In almost all cases, in this stratified randomly-selected subcohort, the frequency of recognized salivary antigens was higher in sera from people with CL as compared to control sera. Table 3 shows that the most frequently recognized sand fly salivary proteins were MW30 and MW64 in both case and control sera. Other SGH antigens recognized were MW12/14, 15, 18, 28, 32, 36, 42, 44, 46, 52 and several “non-traditional” salivary proteins (see Supplemental Table 2). Controlling for the MCOD, recognition of MW32 correlated more often with control than case sera (odds ratio 4.1, 95% CI 1.4 to11.7). Except for MW32, all salivary proteins had a statistically significant correlation with higher MCOD. Logistic regression analysis found that MW15, 28 and 42 were associated with the largest effect on case versus control MCOD differences in this cohort. There were pairs of salivary proteins that were generally recognized together by Western blot; MW30 and 64 (κ=0.72, p<0.001), MW44 and 46 (κ=0.78, p<0.001). Lower antibody levels were associated with older participants for MW30 (median age [range] with protein absent versus present: (26.7 years [20.2 to 56.9 years] versus 25.5 years [19.8 to 52.6], p=0.050), MW42 (26.6 [20.2 to 56.9] versus 24.5 [19.8 to 52.6], p=0.043) and MW46 (26.5[20.4 to 56.9] versus 23.4 [19.8 to 37.6], p=0.007).
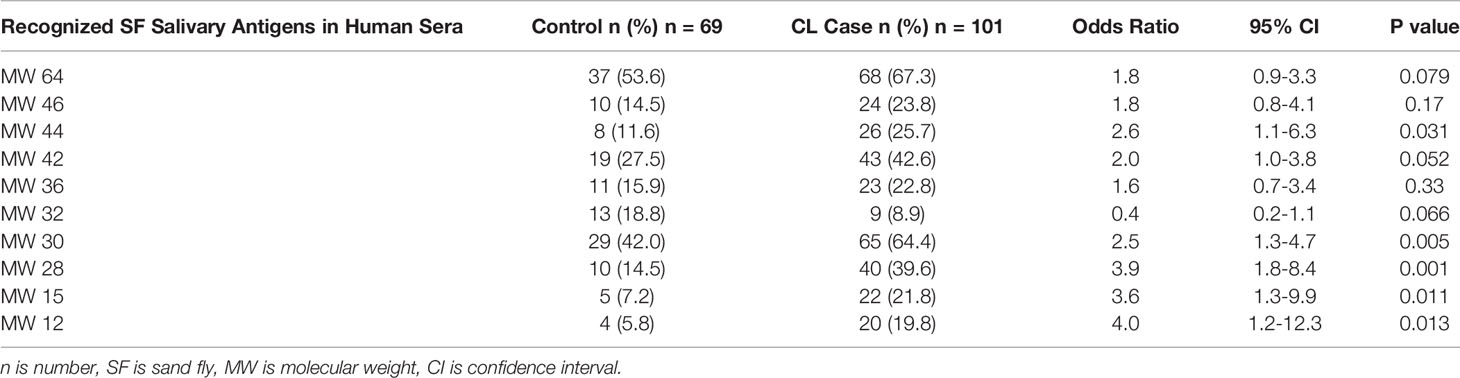
Table 3 Comparison of Ph. papatasi salivary antigens recognized in sera of CL case versus deployed control group.
Recognition of Salivary Antigens in CL Cases and Clinical Correlation
Restricting analysis to the CL cases with Western blot data, we found that absence of a response to MW36 and 46 correlated with longer duration of CL (specifically time from lesion onset to enrollment). Total lesion area, used as a proxy for more severe disease, was associated with MW32 (n=9), as was larger maximum area. MW32 antibody was more likely present in Asian, African-American, and Hispanic CL cases as compared to White (p=0.033). Ulceration was seen if MW15 or 30 were negative (p=0.04, 0.009 respectively). Multivariate analysis found increasing age (β=0.28, p =0.003), later date of enrollment (β=0.21, p=0.026), absence of MW44 (β=-0.22, p=0.021), and presence of MW32 (β=0.33, p=0.001) were associated with larger total lesion area (more severity). Among the analysis of vector risk factors MW36 was reported in those with the highest category of biting exposure, >100 bites/day. No other statistically significant associations were noted with all vector salivary proteins and IgE serum level, other clinical parameters, or personal protective measures collected from the risk factor survey.
Discussion
The investigation of human immune response to bites of sand flies demonstrated that both humoral and cellular systemic immunity develops (9, 10, 12, 18, 30–33). The IgG antibody subtype most associated with this human serologic response was IgG4 and no correlation with specific IgE was found (11). Here we report the kinetics, frequency, duration of-, and the Ph. papatasi saliva antigens recognized by- anti-Ph. papatasi IgG antibodies in a cohort of 496 deployed U.S. military members (coming from a nonendemic region) who traveled to Iraq. We evaluate these vector saliva antibody responses in the context of cutaneous leishmaniasis (due to L. major) clinical presentation (among 248 cases) and personal protection measures utilized. Uniquely, our research was supported by the resource of longitudinally available, matched, banked sera for each participant prior to travel (34) as well as sera from after return to the nonendemic U.S. This permitted each person to serve as their own control, which we present as mean change in optical density pre-exposure to post (CL diagnosis [cases] or return to U.S. [matched deployed controls without CL]).
Our results show that the level of antibody to Ph. papatasi salivary gland homogenate was significantly higher in exposed (traveled to Iraq) participants as compared to pre-deployment. This finding is consistent with reports that antibody to Ph. papatasi salivary proteins may serve as a biomarker for exposure to vector (10, 11, 13, 35, 36). Antibody to Ph. papatasi salivary gland homogenate was also significantly higher in those with parasitologically confirmed L. major cutaneous infection (case, Figure 2A) versus no CL diagnosis suggesting that it also serves as a biomarker for the risk of acquiring leishmaniasis. This finding supports other reports of this observation (10, 11, 13, 35, 37).
Some slight cross reactivity between human anti-Ph. sergenti and Ph. papatasi SGH responses was reported previously (10). In mice (36), Drahota et al. found that blood from mice bitten by Ph. sergenti tested positively using Ph. papatasi SGH, although with lower levels than Ph. sergenti (38). We did not test this in our cohort, but given that 98% human CL in American soldiers deployed to Iraq was caused by L. major and in sand fly surveillance at Talil Air Base in southern Iraq, 1% captured female sand files were Ph. sergenti versus 24% were Ph. papatasi (4) we assess that contact with Ph. sergenti was not high in this reported cohort. In the U.S. there are Lutzomyia species, especially across the southern regions where many large military bases are located. We conjecture that the small elevations of anti-Ph. papatasi saliva antibody seen in the pre-deployment samples might reflect some cross reactivity to Lutzomyia species. Alternatively, a sand fly unrelated antigen may have elicited a response in pre-deployment sera that coincidentally cross reacted with Ph. papatasi saliva. In the control samples, we had very limited information in terms of prior travel to leishmaniasis endemic areas (only that they never deployed [versus traveled] there during military service) since these de-identified sera were provided from the serum repository.
Our data also reveal that among those with CL, levels of antibody to Ph. papatasi salivary gland homogenate decreased significantly by 6-24 months after return from Iraq to nonendemic U.S. This feature (short duration IgG response) potentiates the value of anti-SGH as a biomarker of seasonal vector exposure. There is limited data available about the durability of the humoral immune responses to Ph. papatasi as most work has been cross sectional prevalence studies or follow up during sand fly season. In a pediatric cohort in endemic Tunisia followed over two L. major transmission seasons, only 3/200 children remained Ph. papatasi saliva antibody negative after transmission season in October (11), whereas among people exposed to vectors of visceral leishmaniasis, Ph. argentipes and Ph. kandelakii IgG to vector salivary proteins waned between exposure seasons (31, 39). Human antibodies to Ph. sergenti saliva waned between October-March in Iran (32). In this study, a multivariable analysis found that in addition to CL, White race was associated with higher levels of Ph. papatasi anti-SGH IgG and older age was associated with modestly lower levels. We speculate that older age may be confounded by less outside exposure at night as age correlates with advanced military rank/leadership positions. Additionally, we have consistently observed that White race is over-represented for risk of cutaneous leishmaniasis in the U.S. military (27, 40, 41). Given prior murine and non-human primate data (15–17, 30, 31) showing that pre-exposure immune responses to uninfected sand fly saliva may mitigate the severity of subsequent clinical leishmaniasis, we explored clinical factors but found no statistically significant association of MCOD on number, maximal size, ulceration, or duration of skin lesion. Among CL cases there was no influence of length of potential exposure (time in Iraq), sex or IgE levels on MCOD.
An interesting observation in our study was that higher levels of antibody to Ph. papatasi saliva were found in soldiers reporting any use of insect repellent or using repellent at night, although there was no association with reported frequency of repellent application or recalled frequency of bites per day. This higher level of antibody to vector saliva associated with reported use of personal protection measures may suggest that those experiencing more bites were more likely to use repellent. Other considerations include inconsistent use of repellent in the combat environment, repellent wasn’t applied to all exposed areas, repellent may have degraded in the heat, or sand flies were resistant to DEET. Additionally, due to limited available military brand repellent, service members may have been using aerosol DEET or less active preparations sent from home and not applying it frequently enough or completely. Lastly, self reporting by soldiers with vector borne infection (CL) might over-report proper use of repellent due to perceived risk of repercussion if they did not follow military personal protective measure procedures.
Specific Ph. papatasi salivary proteins have been associated with human immunodominant serological responses (10–13, 35, 36, 42). PpSP32, a 30 kDa molecular weight protein similar to a silk-related protein from Nephila calvipes but exclusive to sand flies (14), has consistently been identified as the most prominent IgG response to Ph. papatasi saliva in humans (10–13, 35, 36, 42). The most frequently recognized antigenic salivary proteins in our study were MW30 and MW64. MW30 had a 2.5 odds ratio noted in L. major CL cases compared to controls. MW30 (presumably PpSP32) was recognized in 42% Iraq-deployed controls and 64.4% CL cases. PpSP32 has been reported as an immunodominant response in humans exposed to Ph. papatasi bites in Turkey, Tunisia, Jordan, Egypt and Saudi Arabia (10–13, 34, 36). Other SGH proteins recognized by human sera in this study were MW12/14, 15, 18, 28, 32, 36, 42, 44, 46, 52. Logistic regression analysis suggested that MW15, 28 and 42 had the largest effect on the case versus control MCOD differences, highlighting them as potential biomarkers for CL. A prior report of 40 U.S. soldiers who deployed to Iraq (23 CL, 17 described as “bitten”) found MW38 and 14 kDa (mass spectrometry suggesting the 14kDa protein was PpSP15, and the 38 kDa a salivary apyrase) to be immunodominant (13). The small number of participants in this sub-analysis (n=40) may have caused skewing of the results of U.S. soldiers as in this same report, people living in endemic Egypt and Jordan showed broader immunodominant antibody responses at MW12, 26, 30, 38, 44 kDa (13). Among sera from 114 Jordanian people, Western blots of Ph. papatasi SGH identified bands at MW14, 15, 28, 30, 32, 36, 42, 44, 46, 52, 64 kDa with the most immunodominant being PpSP28,32,36 (36). Presence of humoral immune responses to many vector salivary antigens inversely correlated with strong cell mediated immune responses (36). In the Ph. papatasi sialome, PpSP28 is the most abundant transcript (a D7 secreted protein, odorant binding protein) (14, 43, 44). Other proteins include salivary apyrase (PpSP36, 36 kDa) and yellow related proteins PpSP42, PpSP44 which have a biogenic amine-binding function (14, 44, 45). Small members of the odorant binding family, SP12, 14, 15 are similar to D7 proteins, perhaps ancestrally derived from them (14). The observation that some salivary proteins are reactive in pairs, may be due to the formation of complexes such as dimers, as we speculate for the recognition of MW30 and MW64, or to crossreactivity of protein homologues that are similar, as we postulate for the recognition of MW44 and MW46—both belonging to the yellow family of proteins.
Some interesting and novel clinically-associated findings from our analysis of specific salivary proteins in this cohort of Iraq-deployed soldiers were identified. These are relevant outcomes that are rarely assessed in studies of vector saliva humoral immunity. While the numbers of participants in both control and case arms are low, MW32 kDa antibody responses were more likely in the control group than the CL arm, albeit not statistically significant (p=0.066). This is intriguing and merits further study as to whether this antibody may have a protective role against L. major infection. Among those with L. major CL, absence of antibody to Ph. papatasi MW36 and MW46 was associated with a longer time from lesion onset to enrollment in study, possibly suggesting that these responses are early to wane. Counterintuitively, presence of MW32 kDa band was associated with our proxies for more severe CL, specifically larger maximum area (single lesion) and total lesion area (summed areas of all lesions). MW32 was also more likely recognized in people of color with CL compared to Whites (p=0.033). In our study, logistic regression identified absence of MW 44 and presence of MW32 antibody responses as associated with more severity (larger total lesion area). Ulcer presentation was associated with negative MW15 or MW30 (presumed PpSP32). In a Saudi Arabia cohort, higher levels of antibody to PpSP32 correlated with a nodular presentation of L. major (35). MW36 antibody was associated with those reporting more than 100 insect bites per day. Notably others have reported that PpSP44 triggered the highest amount of interferon gamma production after lymphocyte stimulation (46), and while cellular and humoral immune responses are often discordant for Ph. papatasi salivary proteins, it is interesting that absence of humoral response to MW44 (presumed PpSP44) in this study is associated with more CL disease burden. Lastly, PpSP44 and PpSP15 transcripts were shown to be upregulated in blood fed sand flies (wild populations, Iran) as well as during summer months and low in gravid flies (34). PpSP15 transcript was higher in uninfected sand flies compared to those with L. major (34). The highest protein levels (SGH) were noted in unfed sand flies and in the summer (35). Whether these characteristics might impact the type of human immune exposure to vector saliva in wild populations of sand flies in Iraq is only conjecture.
Our population of healthy, young men with little exposure to phlebotomine sand fly saliva previous to their deployment to a country of high CL risk (2) is generalizable to the North American adventure traveler but perhaps not to endemic populations in regions of greatest risk of CL. Our study used salivary gland homogenate and did not characterize the noted bands beyond MW, so they cannot be definitively linked to various salivary protein characterizations. There is the potential of skew in our data set for specific protein responses as we randomly selected populations based on strata of MCOD, but there were few high level MCOD in the control arms leading to less people in that group. This would have persisted if we had used all 496 available sera, as the number of control sera with OD >1 is very limited (two). The study design did not permit us to study cellular immune responses as banked cells and sufficient control participants (deployed but no CL) were not available for real time blood collection. The longer exposure period of the control population is not expected to meaningfully affect the results of our analysis; higher antibody levels in the control arm would only decrease the differential observations and the sand flies in Iraq were unlikely to be active transmitters in the winter/early spring that represented the additional time in country for the control group.
In summary, we successfully assessed the antigenic profiles after natural bite exposures to Ph. papatasi saliva among nearly 500 nonimmune American soldiers deployed to Iraq; 248 of whom had parasitologically confirmed L. major cutaneous infection. Using a case control study design, complemented by serial blood samples (so each participant could also be compared to their pre-deployment antibody levels) we were able to identify biomarkers for both vector exposure as well as CL infection. Relatively short duration of these antibody responses was demonstrated. Uniquely we were able to correlate sand fly exposure reported risk factors to levels of antibody to Ph. papatasi salivary proteins. In addition, we report some clinical associations with specific Ph. papatasi proteins such as clinical severity, ulcer development, and race/ethnicity. Further work should corroborate whether MW32 salivary protein antibody responses can be protective against L. major infection since this could have a role in prevention measures such as vaccination.
Data Availability Statement
The raw data supporting the conclusions of this article will be made available by the authors, without undue reservation.
Ethics Statement
The studies involving human participants were reviewed and approved by Walter Reed Army Medical Center. The patients/participants provided their written informed consent to participate in this study.
Author Contributions
Contributions by authors as follows: NA, SK, and JV designed study and coordinated work. WP collected risk factor data. NA collected clinical data and samples. FO and RG performed laboratory testing. NA wrote paper, all authors contributed to revisions. RH performed statistical analysis and developed figures and tables. All authors contributed to the article and approved the submitted version.
Funding
Funding support was provided from Walter Reed Army Medical Center Global War on Terror Program (NA) and by the Intramural Research Program of the NIH, National Institute of Allergy and Infectious Diseases (FO, SK, JV).The funders had no role in study design, data collection and analysis, decision to publish, or preparation of the manuscript. Funding for open access publication was provided from the Henry Jackson Foundation project “Infectious Diseases Research and Education Support Funds”, award 3022233-1.0-61916.
Author Disclaimer
The views expressed in this manuscript are those of the authors and do not reflect the official policy of the Uniformed Services University, the Department of the Army/Navy/Air Force, the Department of Defense, the Department of Health and Human Services, or the United States Government.
Conflict of Interest
The authors declare that the research was conducted in the absence of any commercial or financial relationships that could be construed as a potential conflict of interest.
The handling editor declared a shared affiliation, though no other collaboration, with one of the authors NA at the time of the review.
Publisher’s Note
All claims expressed in this article are solely those of the authors and do not necessarily represent those of their affiliated organizations, or those of the publisher, the editors and the reviewers. Any product that may be evaluated in this article, or claim that may be made by its manufacturer, is not guaranteed or endorsed by the publisher.
Acknowledgments
We would like to thank Dr. Angelia Cost, Armed Forces Health Surveillance Division, for identifying matched controls, conducting the matching to the Department of Defense Serum Repository samples and making sample arrangements. These data have been presented in part in poster presentation at the Military Health System Research Symposium 2015. References to non-Federal entities or products do not constitute or imply a Department of Defense or Uniformed Services University of the Health Sciences endorsement. This work was prepared by a military or civilian employees of the U.S. Government as part of the individual’s official duties and therefore is in the public domain and does not possess copyright protection (public domain information may be freely distributed and copied; however, as a courtesy it is requested that the Uniformed Services University and the author be given an appropriate acknowledgement).
Supplementary Material
The Supplementary Material for this article can be found online at: https://www.frontiersin.org/articles/10.3389/fitd.2021.766273/full#supplementary-material
Supplementary Material | Risk factor survey.
Supplementary Material | Ph. papatasi salivary gland homogenate Western blots using case and control sera.
References
1. World Health Organization. WHO Fact Sheet: Leishmaniasis (2020). Available at: https://www.who.int/news-room/fact-sheets/detail/leishmaniasis.
2. Scheufele CJ, Giesey RL, Delost GR. The Global, Regional, and National Burden of Leishmaniasis: An Ecologic Analysis From the Global Burden of Disease Study 1990-2017. J Am Acad Dermatol (2021) 84(4):1203–5. doi: 10.1016/j.jaad.2020.08.043
3. Alvar J, Velez ID, Bern C, Herrero M, Desjeux P, Cano J, et al. Leishmaniasis Worldwide and Global Estimates of Its Incidence. PloS One (2012) 7(5):e35671. doi: 10.1371/journal.pone.0035671
4. Coleman RE, Burkett DA, Sherwood V, Caci J, Spradling S, Jennings BT, et al. Impact of Phlebotomine Sand Flies on U.S. Military Operations at Tallil Air Base, Iraq: 2. Temporal and Geographic Distribution of Sand Flies. J Med Entomol (2007) 44(1):29–41. doi: 10.1603/0022-2585(2007)44[29:iopsfo]2.0.co;2
5. Al-Bajalan MMM, Niranji SS, Al-Jaf SM, Kato H. First Molecular Identification of Leishmania Major in Phlebotomus Papatasi in an Outbreak Cutaneous Leishmaniasis Area in Iraq. Acta Trop (2021) 215:105807. doi: 10.1016/j.actatropica.2020.105807
6. Coleman RE, Hochberg LP, Swanson KI, Lee JS, McAvin JC, Moulton JK, et al. Impact of Phlebotomine Sand Flies on U.S. Military Operations at Tallil Air Base, Iraq: 4. Detection and Identification of Leishmania Parasites in Sand Flies. J Med Entomol (2009) 46(3):649–63. doi: 10.1603/033.046.0383
7. Centers for Disease C, Prevention. Cutaneous Leishmaniasis in U.S. Military Personnel–Southwest/Central Asia, 2002-2003. MMWR Morb Mortal Wkly Rep (2003) 52(42):1009–12.
8. Centers for Disease C, Prevention. Update: Cutaneous Leishmaniasis in U.S. Military Personnel–Southwest/Central Asia, 2002-2004. MMWR Morb Mortal Wkly Rep (2004) 53(12):264–5.
9. Stahlman S, Williams VF, Taubman SB. Incident Diagnoses of Leishmaniasis, Active and Reserve Components, U.S. Armed Forces, 2001-2016. MSMR (2017) 24(2):2001–162–7.
10. Rohousova I, Ozensoy S, Ozbel Y, Volf P. Detection of Species-Specific Antibody Response of Humans and Mice Bitten by Sand Flies. Parasitology (2005) 130(Pt 5):493–9. doi: 10.1017/S003118200400681X
11. Marzouki S, Ben Ahmed M, Boussoffara T, Abdeladhim M, Ben Aleya-Bouafif N, Namane A, et al. Characterization of the Antibody Response to the Saliva of Phlebotomus Papatasi in People Living in Endemic Areas of Cutaneous Leishmaniasis. Am J Trop Med Hyg (2011) 84(5):653–61. doi: 10.4269/ajtmh.2011.10-0598
12. Marzouki S, Abdeladhim M, Abdessalem CB, Oliveira F, Ferjani B, Gilmore D, et al. Salivary Antigen SP32 Is the Immunodominant Target of the Antibody Response to Phlebotomus Papatasi Bites in Humans. PloS Negl Trop Dis (2012) 6(11):e1911. doi: 10.1371/journal.pntd.0001911
13. Geraci NS, Mukbel RM, Kemp MT, Wadsworth MN, Lesho E, Stayback GM, et al. Profiling of Human Acquired Immunity Against the Salivary Proteins of Phlebotomus Papatasi Reveals Clusters of Differential Immunoreactivity. Am J Trop Med Hyg (2014) 90(5):923–38. doi: 10.4269/ajtmh.13-0130
14. Abdeladhim M, Jochim RC, Ben Ahmed M, Zhioua E, Chelbi I, Cherni S, et al. Updating the Salivary Gland Transcriptome of Phlebotomus Papatasi (Tunisian Strain): The Search for Sand Fly-Secreted Immunogenic Proteins for Humans. PloS One (2012) 7(11):e47347. doi: 10.1371/journal.pone.0047347
15. Belkaid Y, Kamhawi S, Modi G, Valenzuela J, Noben-Trauth N, Rowton E, et al. Development of a Natural Model of Cutaneous Leishmaniasis: Powerful Effects of Vector Saliva and Saliva Preexposure on the Long-Term Outcome of Leishmania Major Infection in the Mouse Ear Dermis. J Exp Med (1998) 188(10):1941–53. doi: 10.1084/jem.188.10.1941
16. Kamhawi S, Belkaid Y, Modi G, Rowton E, Sacks D. Protection Against Cutaneous Leishmaniasis Resulting From Bites of Uninfected Sand Flies. Science (2000) 290(5495):1351–4. doi: 10.1126/science.290.5495.1351
17. Valenzuela JG, Belkaid Y, Garfield MK, Mendez S, Kamhawi S, Rowton ED, et al. Toward a Defined Anti-Leishmania Vaccine Targeting Vector Antigens: Characterization of a Protective Salivary Protein. J Exp Med (2001) 194(3):331–42. doi: 10.1084/jem.194.3.331
18. Oliveira F, Rowton E, Aslan H, Gomes R, Castrovinci PA, Alvarenga PH, et al. A Sand Fly Salivary Protein Vaccine Shows Efficacy Against Vector-Transmitted Cutaneous Leishmaniasis in Nonhuman Primates. Sci Transl Med (2015) 7(290):290ra90. doi: 10.1126/scitranslmed.aaa3043
19. Abdeladhim M, Kamhawi S, Valenzuela JG. What's Behind a Sand Fly Bite? The Profound Effect of Sand Fly Saliva on Host Hemostasis, Inflammation and Immunity. Infect Genet Evol (2014) 28:691–703. doi: 10.1016/j.meegid.2014.07.028
20. Gomes R, Oliveira F, Teixeira C, Meneses C, Gilmore DC, Elnaiem DE, et al. Immunity to Sand Fly Salivary Protein LJM11 Modulates Host Response to Vector-Transmitted Leishmania Conferring Ulcer-Free Protection. J Invest Dermatol (2012) 132(12):2735–43. doi: 10.1038/jid.2012.205
21. Abdeladhim M, Ben Ahmed M, Marzouki S, Belhadj Hmida N, Boussoffara T, Belhaj Hamida N, et al. Human Cellular Immune Response to the Saliva of Phlebotomus Papatasi Is Mediated by IL-10-Producing CD8+ T Cells and Th1-Polarized CD4+ Lymphocytes. PloS Negl Trop Dis (2011) 5(10):e1345. doi: 10.1371/journal.pntd.0001345
22. Kreutzer RD, Christensen HA. Characterization of Leishmania Spp. By Isozyme Electrophoresis. Am J Trop Med Hyg (1980) 29(2):199–208. doi: 10.4269/ajtmh.1980.29.199
23. US Army Medical Research and Materiel Command. Smart Leish (2011). Available at: https://www.accessdata.fda.gov/cdrh_docs/pdf8/K081868.pdf (Accessed October 16, 2021).
24. Kato H, Anderson JM, Kamhawi S, Oliveira F, Lawyer PG, Pham VM, et al. High Degree of Conservancy Among Secreted Salivary Gland Proteins From Two Geographically Distant Phlebotomus Duboscqi Sandflies Populations (Mali and Kenya). BMC Genomics (2006) 7:226. doi: 10.1186/1471-2164-7-226
25. Ramalho-Ortigao M, Coutinho-Abreu IV, Balbino VQ, Figueiredo CA Jr., Mukbel R, Dayem H, et al. Phlebotomus Papatasi SP15: mRNA Expression Variability and Amino Acid Sequence Polymorphisms of Field Populations. Parasit Vectors (2015) 8:298. doi: 10.1186/s13071-015-0914-2
26. Flanley CM, Ramalho-Ortigao M, Coutinho-Abreu IV, Mukbel R, Hanafi HA, El-Hossary SS, et al. Phlebotomus Papatasi Sand Fly Predicted Salivary Protein Diversity and Immune Response Potential Based on In Silico Prediction in Egypt and Jordan Populations. PloS Negl Trop Dis (2020) 14(7):e0007489. doi: 10.1371/journal.pntd.0007489
27. Aronson NE, Wortmann GW, Johnson SC, Jackson JE, Gasser RA Jr., Magill AJ, et al. Safety and Efficacy of Intravenous Sodium Stibogluconate in the Treatment of Leishmaniasis: Recent U.S. Military Experience. Clin Infect Dis (1998) 27(6):1457–64. doi: 10.1086/515027
28. Coleman RE, Burkett DA, Putnam JL, Sherwood V, Caci JB, Jennings BT, et al. Impact of Phlebotomine Sand Flies on U.S. Military Operations at Tallil Air Base, Iraq: 1. Background, Military Situation, and Development of a "Leishmaniasis Control Program". J Med Entomol (2006) 43(4):647–62. doi: 10.1603/0022-2585(2006)43[647:iopsfo]2.0.co;2
29. Lestinova T, Rohousova I, Sima M, de Oliveira CI, Volf P. Insights Into the Sand Fly Saliva: Blood-Feeding and Immune Interactions Between Sand Flies, Hosts, and Leishmania. PloS Negl Trop Dis (2017) 11(7):e0005600. doi: 10.1371/journal.pntd.0005600
30. Oliveira F, Lawyer PG, Kamhawi S, Valenzuela JG. Immunity to Distinct Sand Fly Salivary Proteins Primes the Anti-Leishmania Immune Response Towards Protection or Exacerbation of Disease. PloS Negl Trop Dis (2008) 2(4):e226. doi: 10.1371/journal.pntd.0000226
31. Oliveira F, Giorgobiani E, Guimaraes-Costa AB, Abdeladhim M, Oristian J, Tskhvaradze L, et al. Immunity to Vector Saliva Is Compromised by Short Sand Fly Seasons in Endemic Regions With Temperate Climates. Sci Rep (2020) 10(1):7990. doi: 10.1038/s41598-020-64820-9
32. Veysi A, Mahmoudi AR, Yaghoobi-Ershadi MR, Jeddi-Tehrani M, Rassi Y, Zahraei-Ramazani A, et al. Human Immune Response to Phlebotomus Sergenti Salivary Gland Antigens in a Leishmaniasis-Endemic Focus in Iran. Pathog Glob Health (2020) 114(6):323–32. doi: 10.1080/20477724.2020.1789399
33. Veysi A, Mahmoudi A, Yaghoobi-Ershadi M, Jeddi-Tehrani M, Rassi Y, Zahraei-Ramazani A, et al. Salivary Gland Antigens of Laboratory-Bred Phlebotomus Sergenti and Their Immunogenicity in Human Volunteers in Laboratory Condition. Asian Pacific J Trop Med (2020) 13(1):17–23.
34. Rubertone MV, Brundage JF. The Defense Medical Surveillance System and the Department of Defense Serum Repository: Glimpses of the Future of Public Health Surveillance. Am J Public Health (2002) 92(12):1900–4. doi: 10.2105/AJPH.92.12.1900
35. Mondragon-Shem K, Al-Salem WS, Kelly-Hope L, Abdeladhim M, Al-Zahrani MH, Valenzuela JG, et al. Correction: Severity of Old World Cutaneous Leishmaniasis Is Influenced by Previous Exposure to Sandfly Bites in Saudi Arabia. PloS Negl Trop Dis (2015) 9(12):e0004294. doi: 10.1371/journal.pntd.0004294
36. Mukbel RM, Khasharmeh RH, Hijjawi NS, Khalifeh MS, Hatmal MM, McDowell MA. Human Immune Response to Salivary Proteins of Wild-Caught Phlebotomus Papatasi. Parasitol Res (2016) 115(9):3345–55. doi: 10.1007/s00436-016-5094-2
37. Kammoun-Rebai W, Bahi-Jaber N, Naouar I, Toumi A, Ben Salah A, Louzir H, et al. Human Cellular and Humoral Immune Responses to Phlebotomus Papatasi Salivary Gland Antigens in Endemic Areas Differing in Prevalence of Leishmania Major Infection. PloS Negl Trop Dis (2017) 11(10):e0005905. doi: 10.1371/journal.pntd.0005905
38. Drahota J, Lipoldova M, Volf P, Rohousova I. Specificity of Anti-Saliva Immune Response in Mice Repeatedly Bitten by Phlebotomus Sergenti. Parasite Immunol (2009) 31(12):766–70. doi: 10.1111/j.1365-3024.2009.01155.x
39. Clements MF, Gidwani K, Kumar R, Hostomska J, Dinesh DS, Kumar V, et al. Measurement of Recent Exposure to Phlebotomus Argentipes, the Vector of Indian Visceral Leishmaniasis, by Using Human Antibody Responses to Sand Fly Saliva. Am J Trop Med Hyg (2010) 82(5):801–7. doi: 10.4269/ajtmh.2010.09-0336
40. Aronson NE. Leishmaniasis in American Soldiers: Parasites From the Front. In: Emerging Infections 7. Washington DC: ASM Press (2006). p. 325–42.
41. Aronson NE, Wortmann GW, Byrne WR, Howard RS, Bernstein WB, Marovich MA, et al. A Randomized Controlled Trial of Local Heat Therapy Versus Intravenous Sodium Stibogluconate for the Treatment of Cutaneous Leishmania Major Infection. PloS Negl Trop Dis (2010) 4(3):e628. doi: 10.1371/journal.pntd.0000628
42. Marzouki S, Kammoun-Rebai W, Bettaieb J, Abdeladhim M, Hadj Kacem S, Abdelkader R, et al. Validation of Recombinant Salivary Protein PpSP32 as a Suitable Marker of Human Exposure to Phlebotomus Papatasi, the Vector of Leishmania Major in Tunisia. PloS Negl Trop Dis (2015) 9(9):e0003991. doi: 10.1371/journal.pntd.0003991
43. Valenzuela JG, Charlab R, Gonzalez EC, de Miranda-Santos IK, Marinotti O, Francischetti IM, et al. The D7 Family of Salivary Proteins in Blood Sucking Diptera. Insect Mol Biol (2002) 11(2):149–55. doi: 10.1046/j.1365-2583.2002.00319.x
44. Valenzuela JG, Belkaid Y, Rowton E, Ribeiro JM. The Salivary Apyrase of the Blood-Sucking Sand Fly Phlebotomus Papatasi Belongs to the Novel Cimex Family of Apyrases. J Exp Biol (2001) 204(Pt 2):229–37. doi: 10.1242/jeb.204.2.229
45. Sumova P, Sima M, Kalouskova B, Polanska N, Vanek O, Oliveira F, et al. Amine-Binding Properties of Salivary Yellow-Related Proteins in Phlebotomine Sand Flies. Insect Biochem Mol Biol (2019) 115:103245. doi: 10.1016/j.ibmb.2019.103245
Keywords: sand fly, salivary proteins, antibody, cutaneous leishmaniasis, Phlebotomus papatasi
Citation: Aronson NE, Oliveira F, Gomes R, Porter WD, Howard RS, Kamhawi S and Valenzuela JG (2022) Antibody Responses to Phlebotomus papatasi Saliva in American Soldiers With Cutaneous Leishmaniasis Versus Controls. Front. Trop. Dis 2:766273. doi: 10.3389/fitd.2021.766273
Received: 28 August 2021; Accepted: 25 November 2021;
Published: 03 January 2022.
Edited by:
Marcelo Ramalho-Ortigao, Uniformed Services University of the Health Sciences, United StatesReviewed by:
Anne Poinsignon, Institut de Recherche Pour le Développement (IRD), FranceIva Kolarova, Charles University, Czechia
Copyright © 2022 Aronson, Oliveira, Gomes, Porter, Howard, Kamhawi and Valenzuela. This is an open-access article distributed under the terms of the Creative Commons Attribution License (CC BY). The use, distribution or reproduction in other forums is permitted, provided the original author(s) and the copyright owner(s) are credited and that the original publication in this journal is cited, in accordance with accepted academic practice. No use, distribution or reproduction is permitted which does not comply with these terms.
*Correspondence: Naomi E. Aronson, bmFvbWkuYXJvbnNvbkB1c3Vocy5lZHU=
†Retired