- 1International Centre of Insect Physiology and Ecology (icipe), Nairobi, Kenya
- 2Department of Biochemistry and Molecular Biology, Kilimanjaro Christian Medical University College (KCMUCo), Moshi, Tanzania
- 3Department of Infectious Diseases & Immunology, Emerging Pathogens Institute, College of Veterinary Medicine, University of Florida, Gainesville, FL, United States
Female Anopheles mosquitoes transmit Plasmodium parasites that cause human malaria. Currently, vector control is the most widely deployed approach to reduce mosquito population and hence disease transmission. This relies on use of insecticide-based interventions including Long-lasting Insecticide-treated Nets (LLINs) and Indoor Residual Spraying (IRS) where scale-up has contributed to a dramatic decline in malaria deaths and morbidity over the past decade. Challenges to their effective use include the emergence and spread of insecticide resistance by malaria vector populations coupled with the inability to curb outdoor transmission. Under these situations, use of larvicides through larval source management (LSM) can complement these existing measures. The need to minimize environmental impact and effect on non-target organisms has spurred interest in the development of eco-friendly larvicides of natural origin. Here, we review literature published in the last five years to highlight compounds of natural origin found to exhibit larvicidal activity against malaria mosquitoes. Specifically, the larvicidal activity of different classes of compounds is discussed including their effect on non-target organisms. Additionally, we provide suggestions for future research into mosquito larvicides including the use of chemical synthesis to improve the bioactivity of known natural compounds.
Introduction
Malaria is an infectious disease caused by Plasmodium parasites which are transmitted between humans by infected female Anopheles mosquito (1). In 2019, an estimated 229 million cases of malaria and 409,000 deaths were reported worldwide; of which >90% occurred in sub-Saharan African (SSA) (1). Of the four parasite species that cause human malaria (Plasmodium vivax, Plasmodium ovale, Plasmodium malariae and Plasmodium falciperum), P. falciparum is responsible for the most severe form of the disease and it accounts for ~99% of all cases in SSA (1).
To prevent and reduce malaria burden and transmission, control programs rely mainly on surveillance, prompt and accurate diagnosis, treatment of the disease and vector control (e.g. Insecticide Treated Nets (ITNs) and Indoor Residual Spraying (IRS)) (1, 2). According to the WHO, scale-up of insecticide-based approaches including LLINs and IRS, the mainstay of malaria vector control, has seen a dramatic decline in malaria deaths and morbidity over the past decade. Challenges to their use include the emergence and spread of insecticide resistance by malaria vector populations coupled to the inability to curb outdoor transmission. Under these scenarios, larviciding through larval source management (LSM) that target mosquito immature can be a useful method to complement existing measures.
Most larvicides currently employed in vector control are based on synthetic insecticides e.g. growth inhibitors such as difubenzuron and methoprene (3). But because of widespread resistance by mosquitoes and their negative effect on non-target organisms and environment, biolarvicides are now a preferred alternative (4). Some of the commonly sought sources of biolarvicides include plant, bacteria, algae, lichen and fungus (Figure 1).
This review discusses the bioactivity of larvicidal compounds isolated from these five natural sources in the last five years. Their potential effect on non-target organism is also highlighted and use of chemical synthesis to improve the effectiveness of larvicidal compounds is discussed.
Plant-Derived Compounds With Larvicidal Activity
A review of the literature in the last five years showed 52 compounds had been tested for mosquito larvicidal activity against malaria mosquitoes. Most of the studies were focused on plants (70%), followed by bacteria (17%) and then fungi (13%). There was no report of mosquito larvicidal compounds isolated from algae and lichen (Figure 2A). Within the plant Kingdom, most studies (24%) were conducted on the Asteraceae family, followed by Apiaceae (14%) (Figure 2B). Essential oil (EO) appears to be the primary focus of studies that were reviewed. Out of the 52 compounds of plant origin identified in the literature, 42 (81%) were EO (Figure 2C). Notable was the fact that more than 80% of the compounds were isolated from the leaves (43%) and seeds (39%) (Figure 2D). Only two studies (5, 6) investigated plant bark as a potential source of larvicidal compounds.
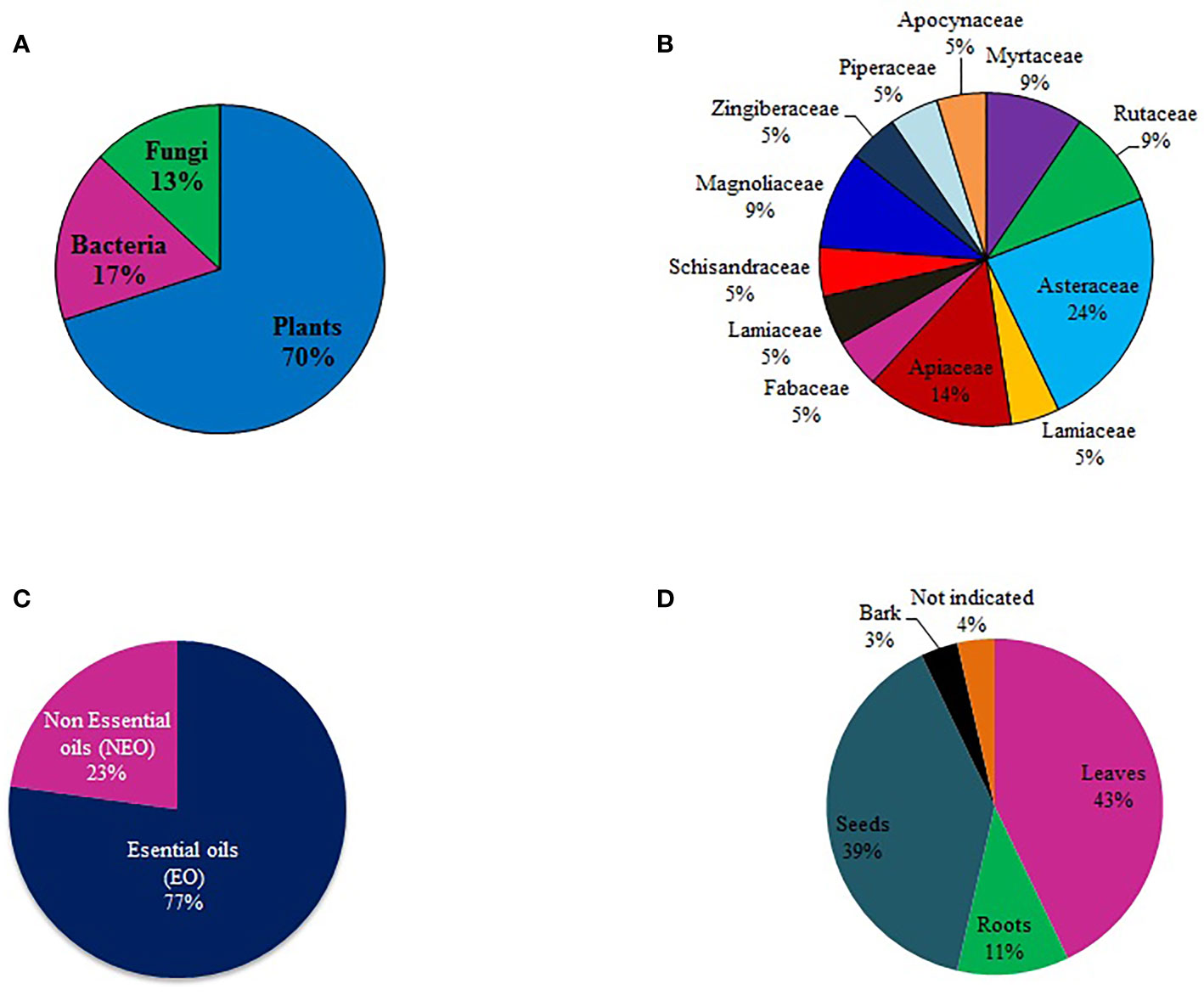
Figure 2 Proportion of larvicidal compounds (A) isolated from different natural sources, (B) categorized by plant families, (C) proportion of essential and non-essential oils, and (D) plant parts targeted in isolation of larvicidal compounds.
Mosquito larvicides are normally applied in an aquatic environment where conspecific and heterospecific mosquito larvae are present and thus, they need to have a broad spectrum of activity. The isolated compounds were screened for activity against larvae of 8 Anopheles species (Table 1). Only piperine, an alkaloid from Piper nigrum (Piperaceae), was tested for larvicidal activity against larvae of most Anopheles species: Anopheles arabiensis, An. coluzzii, An. gambiae, An. quadriannulatus and An. funestus (25). The rest of the compounds were evaluated for activity against larvae of one or two malaria vector species. Plant compounds evaluated for their larvicidal effect against different mosquito species are displayed in Table 1. Overall, An. stephensi and An. subpictus were the most common vectors studied (11 and 7 studies, respectively). Very few studies investigated the larvicidal effect of plant-derived compounds against dominant Afrotropical malaria vectors such as An. gambiae (20, 25), An. funestus and An. arabiensis (25).
Isolated compounds are normally preferred because they can be synthesized for commercial and large scale use. Furthermore, the pure compounds are generally expected to exhibit higher activity than crude samples. In the current review, some studies compared the activity of pure compounds to that of crude extracts and found the compounds to be more effective. For example, carvacrol, terpinen-4-ol, (Z)-γ-bisabolene, lavandulyl acetate, bicyclogermacrene, δ-cadinene, calarene and δ-4-carene were each found to be more effective than the crude EO (10, 15, 17, 21). However, reports of a reduction in larvicidal activity following fractionation and isolation of pure compounds are also not uncommon. For instance, eugenol and Z-ligustilide were found to be less potent than crude EO when tested for larvicidal activity against An. stephensi (8, 18). In yet another study, two compounds aurantio-obtusin and obtusin, isolated from Cassia tora L. (Fabaceae) seed pod were reported to be less potent than the crude seed extract when evaluated for larvicidal activity against An. gambiae (20). The observed potency of crude extracts/EO could be due to the synergistic/additive effect of other compounds in the extract. The potential interaction between components of whole plant extracts is demonstrated in a study by Wang et al. (22), where out of the 17 compounds from Magnolia denudata seeds (Magnoliaceae) tested for activity against An. sinensis larvae only 12 were found to be more potent than the crude EO.
Typically, the search for new larvicidal compounds of plant origin focuses on the use of organic solvent (Figure 3). The use of plant tissue culture is a rarely explored area in the search for new larvicidal agent of plant origin. Interestingly, Kumar et al. (6), isolated the secondary metabolite conessine from the callus culture derived from the bark of Holarrhena antidysenterica (Apocynaceae). Subsequent larvicidal bioassays conducted on the compound revealed a strong larvicidal activity against An. stephensi with lower doses (12μg/mL) causing a 100% mortality.
Bacteria Derived Compounds With Larvicidal Activity
Bacteria provide an unexploited yet promising reservoir of novel secondary metabolites which could be used as lead compounds for new larvicidal agents. So far, some compounds of bacterial origin have been isolated and tested for activity against mosquito larvae. For example, spinosyn A and spinosyn D from the bacterium Saccharopolyspora spinosa (Actinomycetales) were shown to have larvicidal activity against various mosquito species including Anopheles dirus, Anopheles minimus (26), An. gambiae and An. funestus (27).
Besides solid organic compounds, gases produced during fermentation by microbes have also been explored as larvicidal agents against malaria vectors. For instance, hydrogen cyanide (HCN) produced in larval water by Chromobacterium sp, Panama isolated from the midgut of Aedes aegypti mosquitoes, was found to be toxic to An. gambiae larvae (28). Notably, subsequent treatment of the larval water with a cyanide antidote hydroxocobalamin eliminated the larvicidal effect (28). HCN unlike spinosyns is very volatile and potentially toxic to non-target organisms and these characteristics may make it less ideal for use as a larvicide.
Some studies have also explored the use of polysaccharides of microbial origin as larvicidal agents. The marine bacterium Bacillus licheniformis Dahb1 strain isolated from shrimp intestine was found to produce an exopolysaccharide that displayed larvicidal activity against An. stephensi (29). The polysaccharide was extracted using methanol following 72 h fermentation at 37°C (29). The use of crystal proteins (Cry and Cyt toxins) produced during the sporulation phase of certain bacteria e.g. Bacillus thuringiensis var. israelensis has also been exploited for biological control of mosquito larvae (30, 31). Even though bacteria toxins are effective in controlling mosquito larvae, it is well known that with continued use, the disease vector will potentially develop resistance. Hence, there is need to identify new compounds, with possibly different modes of action, to be used in rotation with the bacteria toxins.
Fungal-Derived Compounds With Larvicidal Activity
Fungal toxin is yet another source of secondary metabolite that can be explored for new larvicidal agents against malaria vectors. Fungi are already used widely in agricultural fields as control agents against plant pathogens (32). Thus, they are more likely to be perceived as a source of environment-friendly compounds.
A review of the literature shows that, only a few fungal-derived metabolites have been isolated and tested for activity against malaria mosquito species. In a study by (33), a novel isoquinoline, 2-(4-((3E,5E)-14-aminotetradeca-3,5-dienyloxy)butyl)-1,2,3,4-tetrahydroisoquinolin-4-ol (ATDBTHIQN) was isolated and characterized from the ethyl acetate extract of a fungal strain Fusarium moniliforme KUMBF1201 sourced from paddy field soil. The compound was isolated after 14 days of fermentation at 28°C and found to exhibit potent larvicidal activity against the larvae of An. stephensi.
The focus in the last five years appear to have been on crude secondary metabolites rather than individual compounds. In a study by (34), crude secondary metabolites isolated from the fungus Metarhizium anisopliae showed a strong dose dependent larvicidal activity against larvae of An. stephensi mosquitoes. The fungus was isolated from infected dead Ae. aegypti mosquito larvae collected from natural traps and the compounds were obtained after 10 days of incubation at 26°C.
In a separate study, crude secondary metabolites from the entomopathogenic fungus Beauveria bassiana (Clavicipitaceae), isolated from an infected grasshopper (Melanoplus sanguinipes), were shown to have larvicidal activity against An. stephensi mosquitoes (35). Further analysis by coupled gas chromatography-mass spectrometry (GC-MS) led to the identification of 9,12-octadecadienoic acid (ZZ)– (63.2%) as one of the major compounds in the crude mycelium extract. Although larvicidal activity of the individual compound was not performed, it had previously been found to poses larvicidal activity against An. stephensi mosquitoes (36). Similarly, in the screen for novel larvicidal agents of fungal origin, crude secondary metabolites from Aspergillus terreus were found to have larvicidal activity against An. stephensi (37). Aspergillus terreus was isolated from soil and the compounds were obtained after 15 days of incubation at 24 ± 2°C.
Effect of Natural Product-Derived Compounds on Non-Target Organisms
Negative effect of insecticides on the environment and non-target organism has been a major concern worldwide for decades. Ecotoxicological effect of larvicidal agents is mainly evaluated on non-target aquatic organisms. This review revealed the number of isolated larvicidal compounds tested for safety against non-target organisms to be limited. Out of the 57 plant compounds identified, only 13 were evaluated for their toxicity against non-target aquatic organisms such as aquatic insects/water bugs (Anisops bouvieri, Chironomus circumdatus and Diplonychus indicus), aquatic crustaceans (Mesocyclops thermocyclopoides) and larvivorous fish (Gambusia affinis and Poecilia reticulate) (Table 2).
Overall, the compounds presented LC50 values that ranged between ~200 and 5000 ug/mL (Table 2). The most toxic was lavandulyl acetate from the EO of Heracleum sprengelianum (Apiaceae) which reported an LC50 that was two-fold higher than the concentration required to demonstrate larvicidal activity (100μg/mL) (38). The mechanism of action of this compound on the non-target organism was however, not investigated. The least toxic was (Z)-β-ocimene the major constituent of A. absinthium EO, which had an LC50 value of ~5000ug/mL and ~45-fold higher than the concentration required to demonstrate larvicidal activity (Table 2).
Among the non-target organisms, fish, G. affinis and P. reticulate, appeared to be the least sensitive to the compounds reporting, on average, very high LC50 values (1881.52 and 1862.07μg/mL, respectively). According to Govindarajan et al. (7), exposure to some of the compounds such as α-humulene and β-elemene does not affect survival and swimming activity of G. affinis. Among the water bugs, A. bouvier was the least sensitive (average LC50 of 1390.05) followed by C. circumdatus (average LC50 = 888.02μg/mL) and finally D. indicus (average LC50 = 879.01μg/mL) (Table 2). Notably, all the compounds were evaluated for toxicity against G. affinis making it the most popular model for ecotoxicological assays. Chironomus circumdatus and P. reticulate were the least used models with only three compounds evaluated against them (Table 2).
Interestingly, a review of the literature revealed that pure or isolated compounds of microbial origin were not evaluated for toxicity against non-target organisms. Also noted in the literature was that all the ecotoxicological assessments were based on laboratory tests. It is well known that in the laboratory, fluctuations in climatic conditions such as temperature which may affect efficacy of the compounds tend to be minimized (39). Thus, it is possible for laboratory results to underestimate the potential effect of the compounds on non-target organism. This perhaps may explain why all the compounds reviewed in this study were found to be generally safer to the non-target organism (LC50 >100μg/mL). Moreover, assessments of side effects of the compounds on non-target organisms were based mainly on acute mortality evaluations. However, for appropriate evaluation, there is need to assess the sub-lethal effects of the compounds on organisms surviving exposure.
Structure Activity Relationship
The existing literature reveals an enormous potential for use of natural compounds in the control of mosquitoes. So far, several secondary metabolites belonging to different classes such as alkaloids, terpenes and flavonoids have been isolated and tested against larvae of various mosquito species. These compounds displayed mixed results, with some showing high toxicity against mosquito larvae, while others showed moderate to low activity (Table 3). Most compounds evaluated for larvicidal activity were terpenes and activity varied across different classes of compounds.
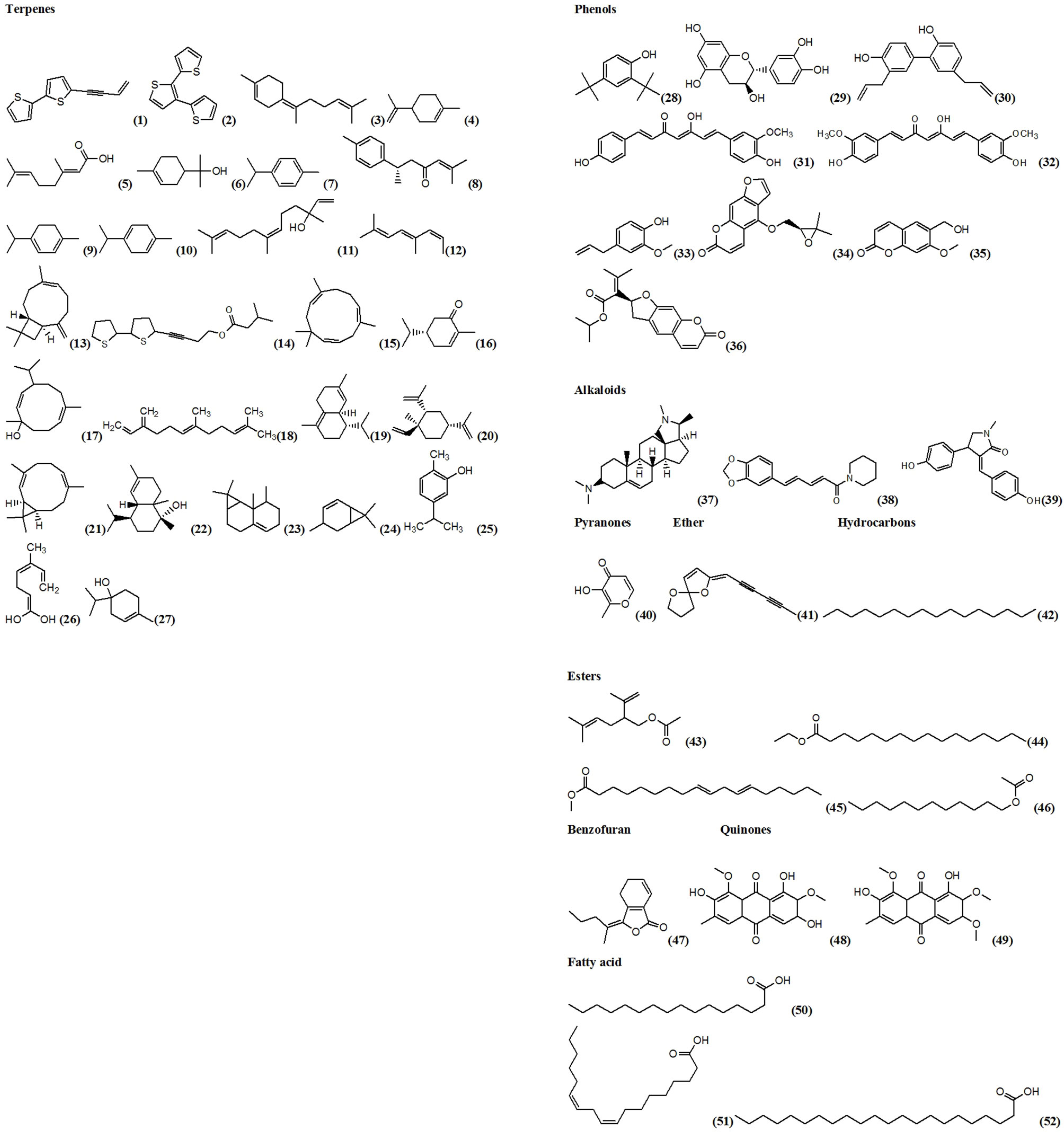
Figure 4 Structures of compounds of natural origin with larvicidal activity against malaria mosquitoes.
The most potent compound was 3-hydroxy-2-methyl-4H-pyran-4-one isolated from the methanol root extract of Senecio laetus (Asteraceae) and evaluated for activity against the larvae of An. stephensi. The compound showed 100% mortality at 20 ppm and the LC50 was 1.22μg/mL after 24 h of exposure period (14). The second most potent compound was 5-(3-buten-1-yn-1-yl)-2,2’-bithiophene isolated from the roots of E. grijsii (LC50 = 1.4μg/mL) (11). The third was n-hexadecane from M. denudata seeds (Magnoliaceae) which was tested for activity against An. sinensis larvae (LC50 = 1.8μg/mL) (22). n-hexadecane however is a non-polar compound and thus its use will require formulation with adjuvants to increase its water solubility and bioavailability to larvae.
Conessine isolated from callus culture derived from the bark of Holarrhena antidysenterica (Apocynaceae) also revealed a strong larvicidal activity against An. stephensi with an LC50 value of 1.93μg/mL (6). This further reveals the potential of this method in isolation of compounds with larvicidal activity. Three coumarins, prantschimgin, oxypeucedanin and 6-hydroxymethylherniarin from root extracts of Ferulago trifida (Apiaceae), reported an activity greater that 100μg/mL and thus were considered non-potent against mosquito larvae (19).
Synergistic or antagonistic interaction is a common occurrence between compounds, yet very few studies evaluate larvicidal activity of binary or tertiary mixtures of pure compounds. For instance, in the reviewed studies, none of the compounds tested for larvicidal activity was further investigated for possible interaction between compounds of the same or different classes. Combining metabolites of biological origin has previously been shown to improve efficacy through synergistic interactions. For example, combination of two protein toxins Cyt1Aa and BinA isolated from B. thuringiensis and Lysinibacillus sphaericus were shown to increase toxicity against Ae. aegypti, despite the mosquito lacking receptors for bin proteins (40). Besides increasing potency, synergism of compounds that display different modes of action can help delay resistance.
Future Prospects and Recommendation
As highlighted in this review, biological sources such as micro-organisms and plants contain considerable amounts of mosquito larvicidal metabolites which could be promising alternatives to synthetic insecticides. In the last five years, not much has been done towards screening secondary metabolites of microbial origin for their larvicidal activity. This could be due to the lack of established protocols for microbial culture or a narrow scope of potential sources of microbes. Future efforts can also focus on mosquito-associated microbiota as an alternative source of new larvicidal compounds. Even though microbiota are needed for the healthy development of the mosquitoes some microbes may produce pathogenic compounds which may be exploited in the development of new larvicidal agents (41). Additionally, mosquito breeding habitats can be screened for bacteria species that produce secondary metabolites that are toxic to mosquito larvae (42). Another alternative approach would involve screening existing fungi and bacterial natural product libraries for potential larvicidal compounds. Most compounds of microbial origin have been isolated and screened for various activities including antimicrobial, anti-inflammatory and antioxidant. This approach could reveal the larvicidal activities of existing compounds. In addition, reverse chemical ecology can be used to prepare molecules that specifically block larval receptors that detect food finding sources or inhibit enzyme function associated with receptor-chemical interactions.
There is still an urgent need to reduce mosquito populations to the lowest level and thus prevent malaria transmission. A major shortcoming of larvicides from natural sources is that they are short lasting requiring multiple rounds of application to produce desired effect. Thus, many a time control programs are forced to rely on synthetic chemicals. Performing, structural modification on existing natural product compounds can help identify novel compounds with different modes of action against mosquitoes. For example, recently, two derivatives of the sesquiterpene lactone parthenin, isolated from the invasive weed Parthenium hysterophorus (Asteraceae) in East Africa, including an ethylene glycol derivative and 2α‐azidocoronopilin, were found to be more potent as mosquito larvicides than parthenin against the malaria vector An. gambiae (43). This shows that structural transformation of natural product compounds may be a promising approach to obtain novel compounds that are effective against mosquitoes.
Conclusion
The review reveals most compounds evaluated for larvicidal activity so far to belong to classes of terpenes and phenols. Nonetheless, new compounds are required to help augment the current level of larviciding and to help reduce vector abundance and consequently disease risk. Improving the efficiency of secondary metabolites of biological origin through structural modification is one way of expanding the larviciding agent tool box, while avoiding impacting negatively on non-target organisms.
Author Contributions
TM contributed to manuscript conception and design, literature review, manuscript preparation. DT contributed to manuscript conception and design, manuscript preparation. BT contributed to manuscript conception and design, manuscript preparation. RD contributed to manuscript preparation. RK contributed to manuscript preparation. All authors contributed to the article and approved the submitted version.
Funding
The authors acknowledge financial support by the following organizations and agencies: DELTAS Africa (grant # DEL-15-011 to THRiVE-2), UK’s Foreign, Commonwealth & Development Office (FCDO); the Swedish International Development Cooperation Agency (Sida); the Swiss Agency for Development and Cooperation (SDC); the Federal Democratic Republic of Ethiopia; and the Government of the Republic of Kenya.
Author Disclaimer
The views expressed herein do not necessarily reflect the official opinion of the donors.
Conflict of Interest
The authors declare that the research was conducted in the absence of any commercial or financial relationships that could be construed as a potential conflict of interest.
Publisher’s Note
All claims expressed in this article are solely those of the authors and do not necessarily represent those of their affiliated organizations, or those of the publisher, the editors and the reviewers. Any product that may be evaluated in this article, or claim that may be made by its manufacturer, is not guaranteed or endorsed by the publisher.
Acknowledgments
TM, DT, and BT acknowledge support from Behavioural and Chemical Ecology Unit, ICIPE. TM acknowledges support from Technical University of Kenya. The images in Figures 1, 3 were created with (biorender.com).
References
1. World Health Organization. Fact Sheet About Malaria. (2020). Available at: https://www.who.int/news-room/fact-sheets/detail/malaria.
2. World Health Organization. World Malaria Report 2018. (2018). Available at: https://www.who.int/malaria/publications/world-malaria-report-2018/en/.
3. da Silva MR, Kyrylchuk A, Grafova I, Kliukovskyi D, Bezdudnyy A, Rozhenko A, et al. Synthesis, Molecular Docking Studies, and Larvicidal Activity Evaluation of New Fluorinated Neonicotinoids Against Anopheles Darlingi Larvae. PloS One (2020) 15(2):e0227811. doi: 10.1371/journal.pone.0227811
4. Shen D, Nyawira KT, Xia A. New Discoveries and Applications of Mosquito Fungal Pathogens. Curr Opin Insect Sci (2020) 1(40):111–6. doi: 10.1016/j.cois.2020.05.003
5. Liu XC, Lai D, Liu QZ, Zhou L, Liu Q, Liu ZL. Bioactivities of a New Pyrrolidine Alkaloid From the Root Barks of Orixa Japonica. Molecules (2016) 21(12):1–8. doi: 10.3390/molecules21121665
6. Kumar D, Kumar G, Das R, Kumar R, Agrawal V. In Vitro Elicitation, Isolation, and Characterization of Conessine Biomolecule From Holarrhena Antidysenterica (L.) Wall. Callus and its Larvicidal Activity Against Malaria Vector, Anopheles Stephensi Liston. Environ Sci Pollut Res Int (2018) 25(7):6783–96. doi: 10.1007/s11356-017-1038-3
7. Govindarajan M, Benelli G. α-Humulene and β-Elemene From Syzygium Zeylanicum (Myrtaceae) Essential Oil: Highly Effective and Eco-Friendly Larvicides Against Anopheles Subpictus, Aedes Albopictus and Culex Tritaeniorhynchus (Diptera: Culicidae). Parasitol Res (2016) 115(7):2771–8. doi: 10.1007/s00436-016-5025-2
8. Osanloo M, Sedaghat MM, Esmaeili F, Amani A. Larvicidal Activity of Essential Oil of Syzygium Aromaticum (Clove) in Comparison With Its Major Constituent, Eugenol, Against Anopheles Stephensi. J Arthropod Borne Dis (2018) 12(4):361–9. doi: 10.18502/jad.v12i4.354
9. Pavela R, Govindarajan M. The Essential Oil From Zanthoxylum Monophyllum a Potential Mosquito Larvicide With Low Toxicity to the non-Target Fish Gambusia Affinis. J Pest Sci (2017) 90(1):369–78. doi: 10.1007/s10340-016-0763-6
10. Govindarajan M, Vaseeharan B, Alharbi NS, Kadaikunnan S, Khaled JM, Al-Anbr MN, et al. High Efficacy of (Z)-γ-Bisabolene From the Essential Oil of Galinsoga Parviflora (Asteraceae) as Larvicide and Oviposition Deterrent Against Six Mosquito Vectors. Environ Sci Pollut Res Int (2018) 25(11):10555–66. doi: 10.1007/s11356-018-1203-3
11. Zhao MP, Liu QZ, Liu Q, Liu ZL. Identification of Larvicidal Constituents of the Essential Oil of Echinops Grijsii Roots Against the Three Species of Mosquitoes. Molecules (2017) 22(2):1–11. doi: 10.3390/molecules22020205
12. Benelli G, Govindarajan M, Rajeswary M, Senthilmurugan S, Vijayan P, Alharbi NS, et al. Larvicidal Activity of Blumea Eriantha Essential Oil and its Components Against Six Mosquito Species, Including Zika Virus Vectors: The Promising Potential of (4E,6Z)-Allo-Ocimene, Carvotanacetone and Dodecyl Acetate. Parasitol Res (2017) 116(4):1175–88. doi: 10.1007/s00436-017-5395-0
13. Govindarajan M, Benelli G. Artemisia Absinthium-Borne Compounds as Novel Larvicides: Effectiveness Against Six Mosquito Vectors and Acute Toxicity on non-Target Aquatic Organisms. Parasitol Res (2016) 115(12):4649–61. doi: 10.1007/s00436-016-5257-1
14. Ali SI, Venugopalan V. Mosquito Larvicidal Potential of Hydroxy-2-Methyl-4h-Pyran-4-One (MALTOL) Isolated From the Methanol Root Extract of Senecio Laetus Edgew and Its In-Silico Study. Nat Prod Res (2019) 7:1–5. doi: 10.1080/14786419.2019.1634712
15. Govindarajan M, Rajeswary M, Hoti SL, Benelli G. Larvicidal Potential of Carvacrol and Terpinen-4-Ol From the Essential Oil of Origanum Vulgare (Lamiaceae) Against Anopheles Stephensi, Anopheles Subpictus, Culex Quinquefasciatus and Culex Tritaeniorhynchus (Diptera: Culicidae). Res Vet Sci (2016) 104:77–82. doi: 10.1016/j.rvsc.2015.11.011
16. Elumalai D, Hemavathi M, Hemalatha P, Deepaa CV, Kaleena PK. Larvicidal Activity of Catechin Isolated From Leucas Aspera Against Aedes Aegypti, Anopheles Stephensi and Culex Quinquefasciatus (Diptera: Culicidae). Parasitol Res (2016) 115(3):1203–12. doi: 10.1007/s00436-015-4856-6
17. Govindarajan M, Benelli G. Eco-Friendly Larvicides From Indian Plants: Effectiveness of Lavandulyl Acetate and Bicyclogermacrene on Malaria, Dengue and Japanese Encephalitis Mosquito Vectors. Ecotoxicol Environ Saf (2016) 133:395–402. doi: 10.1016/j.ecoenv.2016.07.035
18. Osanloo M, Amani A, Sereshti H, Shayeghi M, Sedaghat MM. Extraction and Chemical Composition Essential Oil of Kelussia Odoratissima and Comparison its Larvicidal Activity With Z-Ligustilide (Major Constituent) Against Anopheles Stephensi. J Entomol Zool Stud (2017) 5(4):611–6.
19. Goodarzi S, Tavakoli S, Abai MR, Amini Z, Vatandoost H, Yassa N, et al. Strong Insecticidal Potential of Methanol Extract of Ferulago Trifida Fruits Against Anopheles Stephensi as Malaria Vector. Environ Sci Pollut Res Int (2019) 26(8):7711–7. doi: 10.1007/s11356-019-04149-7
20. Mbatchou VC, Tchouassi DP, Dickson RA, Annan K, Mensah AY, Amponsah IK, et al. Mosquito Larvicidal Activity of Cassia Tora Seed Extract and its Key Anthraquinones Aurantio-Obtusin and Obtusin. Parasit Vectors (2017) 10:562. doi: 10.1186/s13071-017-2512-y
21. Govindarajan M, Rajeswary M, Benelli G. δ-Cadinene, Calarene and .δ-4-Carene From Kadsura Heteroclita Essential Oil as Novel Larvicides Against Malaria, Dengue and Filariasis Mosquitoes. Comb Chem High Throughput Screen (2016) 19(7):565–71. doi: 10.2174/1386207319666160506123520
22. Wang Z, Perumalsamy H, Wang M, Shu S, Ahn Y-J. Larvicidal Activity of Magnolia Denudata Seed Hydrodistillate Constituents and Related Compounds and Liquid Formulations Towards Two Susceptible and Two Wild Mosquito Species. Pest Manage Sci (2016) 72(5):897–906. doi: 10.1002/ps.4064
23. Wang Z, Perumalsamy H, Wang X, Ahn Y-J. Toxicity and Possible Mechanisms of Action of Honokiol From Magnolia Denudata Seeds Against Four Mosquito Species. Sci Rep (2019) 9(1):411. doi: 10.1038/s41598-018-36558-y
24. Ali A, Wang Y-H, Khan IA. Larvicidal and Biting Deterrent Activity of Essential Oils of Curcuma Longa, Ar-Turmerone, and Curcuminoids Against Aedes Aegypti and Anopheles Quadrimaculatus (Culicidae: Diptera). J Med Entomol (2015) 52(5):979–86. doi: 10.1093/jme/tjv072
25. Samuel M, Oliver SV, Coetzee M, Brooke BD. The Larvicidal Effects of Black Pepper (Piper Nigrum L.) and Piperine Against Insecticide Resistant and Susceptible Strains of Anopheles Malaria Vector Mosquitoes. Parasites Vectors (2016) 9(1):238. doi: 10.1186/s13071-016-1521-6
26. Britch SC, Linthicum KJ, Aldridge RL, Golden FV, Pongsiri A, Khongtak P, et al. Ultra-Low Volume Application of Spinosad (Natular 2ec) Larvicide as a Residual in a Tropical Environment Against Aedes and Anopheles Species. J Am Mosquito Control Assoc (2018) 34(1):58–62. doi: 10.2987/17-6692.1
27. Gimnig JE, Ombok M, Bayoh N, Mathias D, Ochomo E, Jany W, et al. Efficacy of Extended Release Formulations of NatularTM (Spinosad) Against Larvae and Adults of Anopheles Mosquitoes in Western Kenya. Malar J (2020) 19(1):436. doi: 10.1186/s12936-020-03507-y
28. Short SM, van Tol S, MacLeod HJ, Dimopoulos G. Hydrogen Cyanide Produced by the Soil Bacterium Chromobacterium Sp. Panama Contributes to Mortality in Anopheles Gambiae Mosquito Larvae. Sci Rep (2018) 8(1):8358. doi: 10.1038/s41598-018-26680-2
29. Abinaya M, Vaseeharan B, Divya M, Vijayakumar S, Govindarajan M, Alharbi NS, et al. Structural Characterization of Bacillus Licheniformis Dahb1 Exopolysaccharide—Antimicrobial Potential and Larvicidal Activity on Malaria and Zika Virus Mosquito Vectors. Environ Sci Pollut Res (2018) 25(19):18604–19. doi: 10.1007/s11356-018-2002-6
30. Derua YA, Kahindi SC, Mosha FW, Kweka EJ, Atieli HE, Zhou G, et al. Susceptibility of Anopheles Gambiae Complex Mosquitoes to Microbial Larvicides in Diverse Ecological Settings in Western Kenya. Med Vet Entomol (2019) 33(2):220–7. doi: 10.1111/mve.12353
31. Zhang Q, Hua G, Adang MJ. Effects and Mechanisms of Bacillus Thuringiensis Crystal Toxins for Mosquito Larvae. Insect Sci (2017) 24(5):714–29. doi: 10.1111/1744-7917.12401
32. Thambugala KM, Daranagama DA, Phillips AJL, Kannangara SD, Promputtha I. Fungi vs. Fungi in Biocontrol: An Overview of Fungal Antagonists Applied Against Fungal Plant Pathogens. Front Cell Infect Microbiol (2020) 10:604923. doi: 10.3389/fcimb.2020.604923
33. Pradeep FS, Palaniswamy M, Ravi S, Thangamani A, Pradeep BV. Larvicidal Activity of a Novel Isoquinoline Type Pigment From Fusarium Moniliforme KUMBF1201 Against Aedes Aegypti and Anopheles Stephensi. Process Biochem (2015) 50(9):1479–86. doi: 10.1016/j.procbio.2015.05.022
34. Vivekanandhan P, Swathy K, Kalaimurugan D, Ramachandran M, Yuvaraj A, Kumar AN, et al. Larvicidal Toxicity of Metarhizium Anisopliae Metabolites Against Three Mosquito Species and non-Targeting Organisms. PloS One (2020) 15(5):e0232172. doi: 10.1371/journal.pone.0232172
35. Ragavendran C, Kishore Dubey N, Natarajan D. Beauveria Bassiana (Clavicipitaceae): A Potent Fungal Agent for Controlling Mosquito Vectors of Anopheles Stephensi, Culex Quinquefasciatus and Aedes Aegypti (Diptera: Culicidae). RSC Adv (2017) 7(7):3838–51. doi: 10.1039/C6RA25859J
36. Rahuman AA, Gopalakrishnan G, Ghouse BS, Arumugam S, Himalayan B. Effect of Feronia Limonia on Mosquito Larvae. Fitoterapia (2000) 71(5):553–5. doi: 10.1016/S0367-326X(00)00164-7
37. Ragavendran C, Natarajan D. Insecticidal Potency of Aspergillus Terreus Against Larvae and Pupae of Three Mosquito Species Anopheles Stephensi, Culex Quinquefasciatus and Aedes Aegypti. Environ Sci Pollut Res Int (2015) 22(21):17224–37. doi: 10.1007/s11356-015-4961-1
38. Luz TRSA, de Mesquita LSS, do Amaral FMM, Coutinho DF. Essential Oils and Their Chemical Constituents Against Aedes Aegypti L. (Diptera: Culicidae) Larvae Acta Trop (2020) 212:105705. doi: 10.1016/j.actatropica.2020.105705
39. Abbes K, Biondi A, Kurtulus A, Ricupero M, Russo A, Siscaro G, et al. Combined Non-Target Effects of Insecticide and High Temperature on the Parasitoid Bracon Nigricans. PloS One (2015) 10(9):e0138411. doi: 10.1371/journal.pone.0138411
40. Bideshi DK, Park H-W, Hice RH, Wirth MC, Federici BA. Highly Effective Broad Spectrum Chimeric Larvicide That Targets Vector Mosquitoes Using a Lipophilic Protein. Sci Rep (2017) 7(1):11282. doi: 10.1038/s41598-017-11717-9
41. Jupatanakul N, Pengon J, Selisana SMG, Choksawangkarn W, Jaito N, Saeung A, et al. Serratia Marcescens Secretes Proteases and Chitinases With Larvicidal Activity Against Anopheles Dirus. Acta Trop (2020) 212:105686. doi: 10.1016/j.actatropica.2020.105686
42. Ranasinghe HAK, Amarasinghe LD. Naturally Occurring Microbiota Associated With Mosquito Breeding Habitats and Their Effects on Mosquito Larvae. BioMed Res Int (2020) 2020:4065315. doi: 10.1155/2020/4065315
Keywords: malaria, larvicides, mosquitoes, natural products, Anopheles
Citation: Milugo TK, Tchouassi DP, Kavishe RA, Dinglasan RR and Torto B (2021) Naturally Occurring Compounds With Larvicidal Activity Against Malaria Mosquitoes. Front. Trop. Dis 2:718804. doi: 10.3389/fitd.2021.718804
Received: 01 June 2021; Accepted: 21 July 2021;
Published: 11 August 2021.
Edited by:
Paul O. Mireji, Kenya Agricultural and Livestock Research Organization, KenyaReviewed by:
Eliningaya J. Kweka, Tropical Pesticides Research Institute, TanzaniaPaulo Eduardo Martins Ribolla, São Paulo State University, Brazil
Copyright © 2021 Milugo, Tchouassi, Kavishe, Dinglasan and Torto. This is an open-access article distributed under the terms of the Creative Commons Attribution License (CC BY). The use, distribution or reproduction in other forums is permitted, provided the original author(s) and the copyright owner(s) are credited and that the original publication in this journal is cited, in accordance with accepted academic practice. No use, distribution or reproduction is permitted which does not comply with these terms.
*Correspondence: Trizah K. Milugo, dG1pbHVnb0BpY2lwZS5vcmc=