- Department of Plant Medicine, National Pingtung University of Science and Technology, Neipu, Pingtung, Taiwan
The horizontal transmission of endosymbionts between hosts and parasitoids plays a crucial role in biological control, yet its mechanisms remain poorly understood. This study investigates the dynamics of horizontal transfer of Wolbachia (wCcep) from the rice moth, Corcyra cephalonica, to its parasitoid, Trichogramma chilonis. Through PCR detection and phylogenetic analysis, we demonstrated the presence of identical wCcep strains in both host and parasitoid populations, providing evidence for natural horizontal transmission. To investigate thoroughly, Wolbachia-free colonies were acquired through tetracycline treatment, and the initial density of wCcep in host eggs significantly influences transmission efficiency. High-density wCcep infections led to rapid transmission, with F1 parasitoid titers increasing by as much as 100-fold, while low-density infections exhibited more gradual increases. Additionally, without continuous exposure to infected hosts, wCcep density in T. chilonis diminished over generations. These findings enhance our understanding of Wolbachia’s transfer dynamics and have important implications for developing effective and sustainable biological control strategies using parasitoid wasps, particularly in managing Wolbachia-related pest populations in agricultural systems.
1 Introduction
Wolbachia, an extensively studied endosymbiotic bacterium present across diverse arthropod taxa, has emerged as a crucial focus in biological control research due to its capacity to manipulate host reproduction. This maternally inherited endosymbiont is estimated to infect over 50% of insect species (1). Although Wolbachia primarily spreads through vertical transmission from mother to offspring, accumulating evidence indicates that horizontal transfer—the acquisition of Wolbachia by a novel host from an unrelated donor—occurs with greater frequency than previously recognized across phylogenetically diverse insect taxa. (2).
Wolbachia was first discovered in the mosquito Culex pipiens (3). Within insect hosts, Wolbachia is primarily found in reproductive tissues but can also be present in somatic cells and tissues like salivary glands, hemolymph, and the gut (4). To increase its prevalence within host populations, Wolbachia manipulates host reproduction through mechanisms such as inducing parthenogenesis, feminization, male-killing, and cytoplasmic incompatibility (CI), which confers a reproductive advantage to infected females (5). Beyond reproductive manipulations, Wolbachia’s potential to enhance host fitness is a fascinating area of research, as it can protect against pathogens like dengue virus (6) and West Nile virus (7) and influence mitochondrial DNA variation (8), prompting interest in its use for vector-borne disease control (9, 10).
Horizontal transfer events have been documented in various insect orders, including Diptera (11), Hymenoptera (12–14), Lepidoptera (15, 16), Araneae (17), and Hemiptera (18). These events have been observed within and across different species, facilitated by various mechanisms such as parasitism, predation, and shared ecological niches (19). The potential for horizontal transfer is further supported by the discovery of highly similar Wolbachia strains in distantly related insect species, suggesting possible shifts between host lineages (15). Additionally, novel Wolbachia strains have been identified as inducing reproductive incompatibility in previously uninfected hosts, as observed in whiteflies (16). Furthermore, research on parasitoid wasps has demonstrated the horizontal transfer of parthenogenesis-inducing Wolbachia in laboratory settings (20), highlighting the adaptability and transferability of Wolbachia across different insect species.
Intriguingly, studies have reported highly similar Wolbachia strains (>95% sequence similarity) infecting phylogenetically distant butterfly species from the families Papilionidae and Nymphalidae (2). Likewise, ant species have been found harboring identical Wolbachia strains to their kleptoparasitic ant cricket hosts, indicating potential horizontal transfer events (19). Frydman et al. (21) demonstrated that Wolbachia could migrate from the hemolymph to reproductive tissues in Drosophila melanogaster following microinjection, reaching the oocytes within 15 days. These findings highlight the ability of Wolbachia to spread across species boundaries.
While horizontal transfer events have been documented, the factors governing the successful establishment and persistence of acquired Wolbachia strains within novel hosts remain poorly understood. Sanaei et al. (22) proposed a four-stage model for successful horizontal transfer: (1) contact and entry into the new host, (2) survival and practical replication within the new host, (3) efficient vertical transmission within the new host lineage, and (4) spread and maintenance within the new host population. Each stage presents unique challenges, including overcoming the host’s immune response, adapting to the new cellular environment, and ensuring vertical transmission and long-term persistence. Previous molecular evidence has shown that T. chilonis and other Trichogramma species collected in Taiwan harbor the same Wolbachia strain (wPip) as their factitious host, the rice moth (23, 24).
In this context, the current study explores the possibility of horizontal transfer of Wolbachia between the rice moth and its parasitoid, the Trichogramma wasp. To validate the dynamics of this potential horizontal transfer, rice moth eggs with different titers of wCcep were provided to T. chilonis. To assess the successful acquisition and persistence of wCcep in T. chilonis across generations. The current study contributes to the understanding of Wolbachia horizontal transfer dynamics, elucidating the factors that facilitate or impede the successful acquisition and maintenance of novel Wolbachia strains within new hosts.
2 Method
2.1 Collection of insect sources and rearing conditions
Egg masses of Ostrinia furnacalis (Asian corn borer) parasitized by Trichogramma wasps were collected from corn fields in Yanpu Township, Pingtung, Taiwan, in July-August 2020. The collected egg masses were placed in a growth chamber at 25°C. One day after adult emergence, individual female rice moths were isolated and reared on eggs of the factitious host, rice moth, Corcyra cephalonica, in 50 ml centrifuge tubes at 25°C, 20 ± 5% RH, and 12:12 h (light: dark) photoperiod to establish the laboratory population of the parasitoid, Trichogramma chilonis.
2.2 PCR detection
To confirm the infection status, ten female T. chilonis from each iso-female line were collected, and their genomic DNA was extracted using the ALS Tissue Genomic DNA Extraction Kit. PCR amplification of Wolbachia wsp and ftsZ genes was performed using wsp81F/691R and FtsZBf/Br primers, respectively. The primers amplify the Trichogramma COI gene as an internal reference (5, 25, 26). The PCR reaction mixture consisted of 2 μL of template DNA, 0.5 μL of each forward and reverse primer, 4 μL of FIREPol® MasterMix, and 13 μL of ddH2O. The PCR reactions were performed under the following conditions: initial denaturation at 95°C for 2 minutes, followed by 35 cycles of 95°C for 30 seconds, 55°C for 1 minute, and 72°C for 1 minute, with a final extension at 72°C for 7 minutes. The same protocols were also used to determine the Wolbachia infection rate of the laboratory population of C. cephalonica.
2.3 Phylogenetic analysis of Wolbachia, wCcep
The wsp and ftsZ gene sequences of Wolbachia from the iso-female lines and the laboratory population of C. cephalonica were analyzed using maximum likelihood estimation (MLE) with 1,000 bootstrap replicates (33), and compared with Wolbachia strains in the NCBI database.
2.4 Establishing Wolbachia, wCcep-free (wCcep-) C. cephalonica and T. chilonis populations
C. cephalonica was reared on rice bran treated with 4.8 mg/g tetracycline under conditions of 30°C, 20 ± 5% RH, and 12L:12D photoperiod. After the fifth generation, Wolbachia absence in the C. cephalonica population was confirmed by qPCR. T. chilonis were reared on C. cephalonica eggs free of wCcep. Their Wolbachia status was monitored by qPCR each generation to establish a wCcep- T. chilonis population. To minimize the potential effects of tetracycline residues, the antibiotic-treated C. cephalonica population was reared on untreated rice bran for at least one generation before being used in subsequent experiments.
2.5 Evaluation of horizontal transfer of Wolbachia, wCcep in T. chilonis
2.5.1 Quantity of Wolbachia, wCcep in T. chilonis
With the 49th generation of T. chilonis reared on wCcep- hosts, wCcep density in parasitoids was monitored by qPCR for 15 generations to determine if wCcep could persist without infected hosts. Each generation was tested, and each sample was run in triplicate. T. chilonis individuals with wCcep were used as the control group. The qPCR reaction mixture consisted of 5 μL iQ SYBR Green Supermix (Bio-Rad), 0.25 μL of each primer, 2.5 μL ddH2O, and 2 μL extracted DNA, for a total volume of 10 μL. The target gene, wsp, was detected using the qWspcc5R and qWspcc5F primers, while the reference gene, COI, was detected using the qTcCOI5R and qTcCOI5F primers (Supplementary Table 1). The qPCR conditions were 95°C for 3 minutes, followed by 40 cycles of 95°C for 10 seconds and 59.5°C for 30 seconds.
The density of Wolbachia, wCcep, was determined using the ΔΔCt method. First, by subtracting the Ct value of the reference gene from the Ct value of the target Wolbachia, wCcep gene, the ΔCt value was calculated for each sample. Then, the ΔΔCt value was calculated by subtracting the ΔCt of the control group from the ΔCt of the treatment group. Finally, the fold change in wCcep density was determined using the formula: Fold gene expression = 2-(ΔΔCt).
2.5.2 Effect of Wolbachia, wCcep density on horizontal transmission efficiency
After 15 generations of rearing T. chilonis on wCcep- hosts, they were then provided with either (1) wCcep-infected C. cephalonica eggs (high Wolbachia titer treatment) or (2) tetracycline-treated (2.4 mg/g) C. cephalonica eggs (low Wolbachia titer treatment). To compare the differences, qPCR at each generation monitored the density and replication rate of wCcep in the parasitoids.
2.5.3 DNA extraction and quantification of Wolbachia, wCcep density in C. cephalonica
Eggs were collected from two populations of C. cephalonica: one infected with wCcep and one treated with antibiotics for five generations. To extract DNA from C. cephalonica eggs, they were crushed with a pestle in 1.5 mL microcentrifuge tubes. Then 50 μL of 5% Chelex solution and 1 μL of Proteinase K solution were added, and the samples were incubated at 56°C for 40 minutes, followed by incubation at 95°C for 10 minutes.
Each generation was tested with twenty samples, each run in triplicate. The qPCR reaction mixture was composed of 5 μL of iQ SYBR Green Supermix (Bio-Rad), 0.25 μL of each primer, 2.5 μL of ddH2O, and 2 μL of extracted DNA, for a total volume of 10 μL. The primers qWspcc5R and qWspcc5F were utilized to detect the target wCcep product, while the qCcCOI1R and qCcCoI1F primers were used for the reference gene (refer to Supplementary Table 1). Statistical analyses were performed using R software (version 4.3.0; 27). Kruskal-Wallis tests followed by post-hoc comparisons using the Benjamini-Hochberg correction to control for multiple testing and maintain the false discovery rate at 0.05.
3 Results
3.1 Phylogenetic analysis of Wolbachia, wCcep
After discovering stable Wolbachia infection in T. chilonis from Yanpu, Pingtung, phylogenetic analyses confirmed that the Wolbachia strain detected in our T. chilonis iso-female lines belongs to supergroup B, specifically the wCcep strain, identical to the strain found in their laboratory host, C. cephalonica. This suggests that the wCcep detected in T. chilonis was likely transferred from the laboratory-maintained C. cephalonica population. When comparing the wsp gene fragments, there were no differences between the wPip infection recorded in 2016 and wCcep, with only a 1% divergence detected in the ftsZ gene fragment (Figure 1).
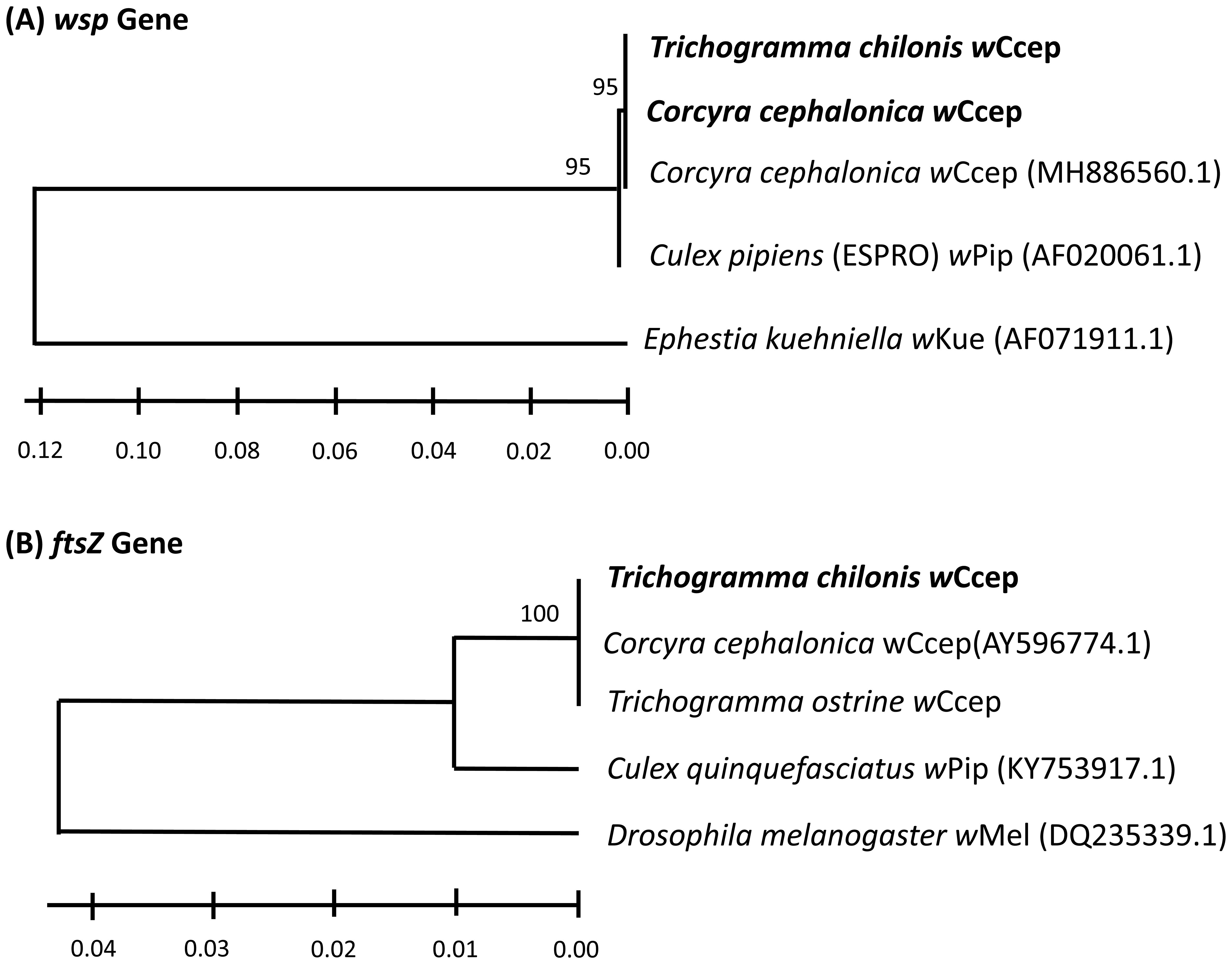
Figure 1. Neighbor-joining tree for Wolbachia strains based on partial sequences of (A) wsp gene and (B) ftsZ gene. Distances were calculated using the Maximum Likelihood Estimation in MEGA X (33). Bootstrap support values (1000 replicates). Bold text indicates the laboratory populations tested in this study. Wolbachia strains are identified by the host species from which they were isolated, followed by an NCBI accession number.
3.2 Establishing Wolbachia, wCcep-free (wCcep-) C. cephalonica and T. chilonis populations
Analysis revealed significant variation in wCcep density across five generations of C. cephalonica maintained on tetracycline-supplemented artificial diet (4.8 mg/g) (Kruskal-Wallis chi-squared = 63.613, d.f = 5, p = 2.173 × 1012). As treatment generations increased, Wolbachia density consistently decreased compared to the infected population. A significant decline in wCcep density was observed from the first generation and continued to decrease in subsequent generations. By the fifth generation, wCcep was no longer detectable, indicating that continuous tetracycline treatment for five generations effectively eradicated wCcep infection in C. cephalonica. This procedure successfully established a Wolbachia-free (wCcep-) strain of C. cephalonica (Figure 2).
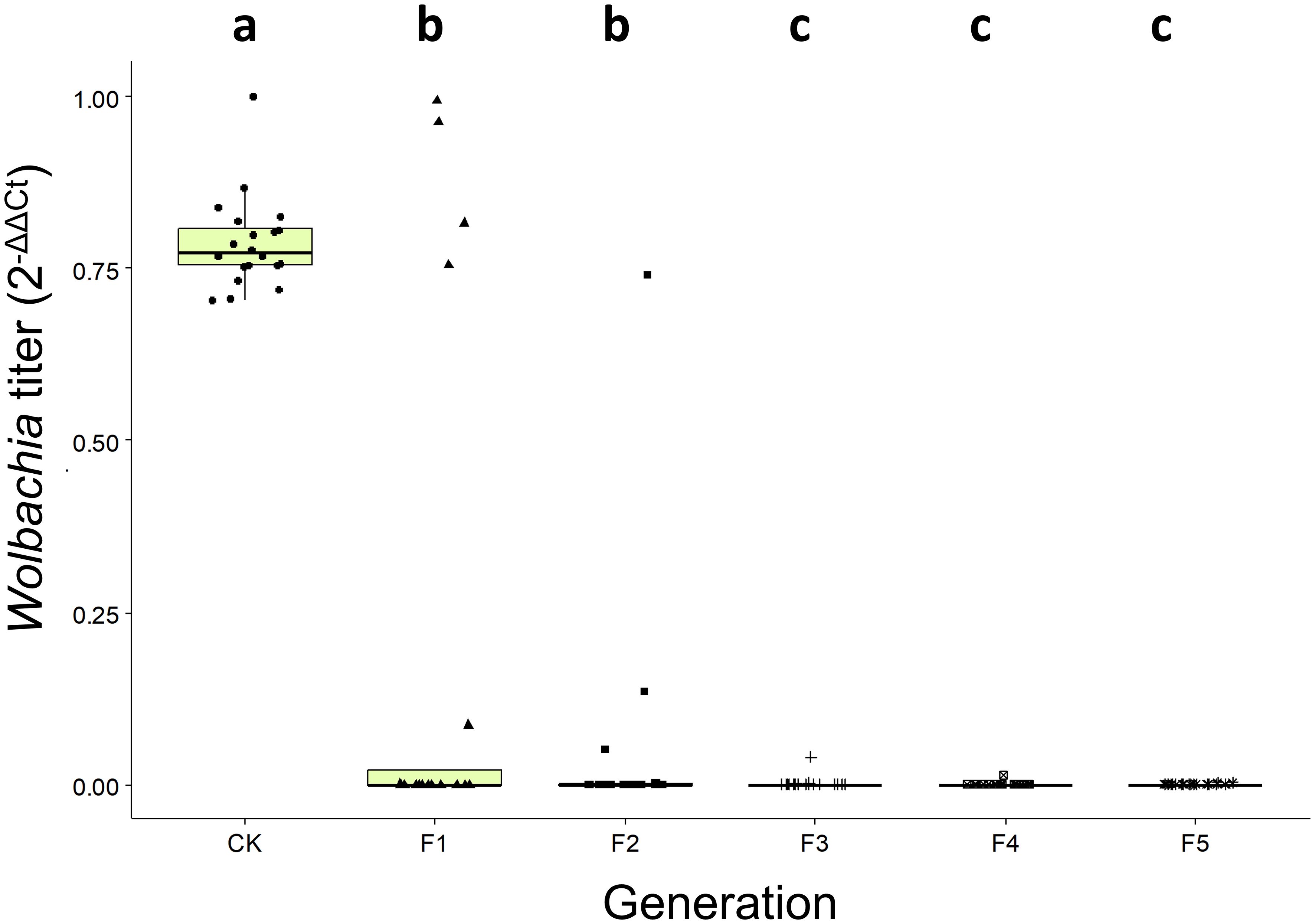
Figure 2. Wolbachia (wCcep) titer on C. cephalonica after 1 to 5 generations of tetracycline treatment. Different letters indicate significant differences (p < 0.05; Kruskal-Wallis test with post-hoc Benjamini-Hochberg test).
Further, qPCR of wCcep titers in T. chilonis iso-female lines revealed significant variations among different iso-female strains; male individuals exhibited significantly higher wCcep titers than females (Figures 3A, B). In a laboratory-maintained population of T. chilonis reared for 49 generations, parasitizing wCcep-free C. cephalonica eggs led to a gradual decrease in wCcep density as parasitism generations increased (F1-F15) (Kruskal-Wallis chi-squared = 32.908, d.f = 4, p = 1.248 × 10-6). The decline in wCcep density within T. chilonis was observed starting from the first generation. These findings suggest that without additional supplementation of wCcep, the bacterial density in T. chilonis progressively diminishes, indicating the necessity of continuous parasitism of C. cephalonica to maintain wCcep levels (Figure 3C).
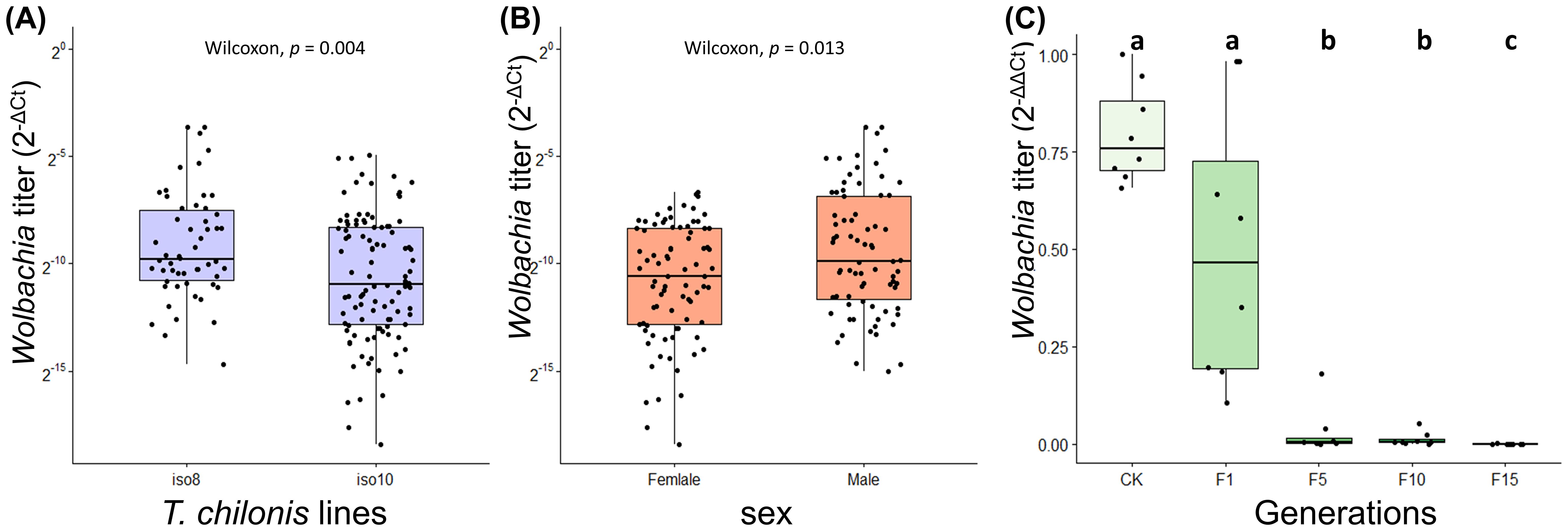
Figure 3. Wolbachia (wCcep) titer on (A) T. chilonis iso-female lines, (B) male and female T. chilonis, and (C) T. chilonis parasitizing Wolbachia-free C. cephalonica across 15 generations. Different letters indicate significant differences (p < 0.05; Kruskal-Wallis test with post-hoc Benjamini-Hochberg correction).
Our investigation into wCcep transmission dynamics revealed significant titer variations depending on the initial infection levels in host eggs. The F15 generation of T. chilonis, parasitizing high-density wCcep eggs (2-ΔΔCt = 1), showed dramatic titer increases, with some F1 individuals exhibiting nearly 100-fold higher densities compared to controls (Kruskal-Wallis chi-squared = 36.43, d.f = 7, p = 6.013 × 10-6). In contrast, parasitization of tetracycline-treated, low-density wCcep eggs (2-ΔΔCt = 0.014) resulted in a slower, more gradual increase in wCcep titers (Kruskal-Wallis chi-squared = 22.757, d.f = 7, p = 1.879 × 10-3). Notably, both scenarios demonstrated significant titer fluctuations across generations F1 to F10, with the high-density treatment showing more pronounced variability, notably a marked decrease after F1 followed by a resurgence around F7 (Figures 4A, B).
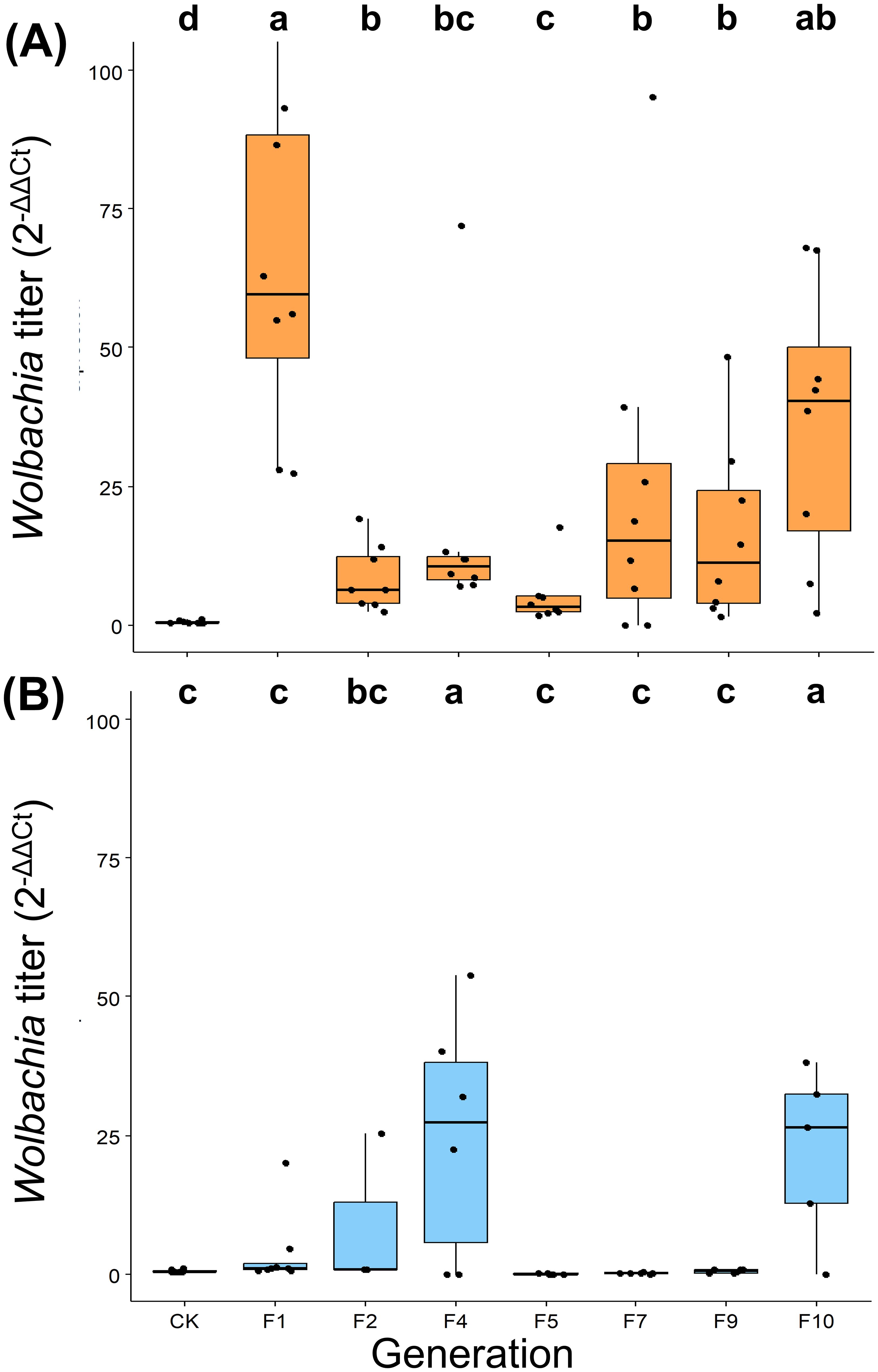
Figure 4. Trichogramma chilonis re-infected with Wolbachia (wCcep) by parasitizing C. cephalonica with (A) high wCcep titer (2-ΔΔCt = 1) and (B) low wCcep titer (2-ΔΔCt = 0.014). The letters indicate significant differences (p<0.05; Kruskal-Wallis test with post-hoc Benjamini and Hochberg test).
4 Discussion
This study demonstrated that Wolbachia strain wCcepundergoes horizontal transmition from C. cephalonica to uninfected T. chilonis through host-parasitoid interactions. This transmission occurred and led to successful proliferation within the new host. The 100% sequence similarity in the ftsZ gene between wCcep strains from field-collected C. cephalonica and T. chilonis in southwestern Taiwan. The comparison of existing wsp and ftsZ sequences in the PubMLST database also showed that the Wolbachia strain isolated from T. chilonis is closely related to the wCcep strain within the STC-41 clonal complex, which is usually found in Lepidoptera hosts (2) (Supplementary Table 2). These results are consistent with previous studies that have shown Wolbachia horizontal transmission in Trichogramma species, such as intraspecific transfer in T. kaykai through superparasitism (28) and interspecific transmission of wDen from T. dendrolimi to T. evanescens through microinjection (29).
Importantly, our study demonstrates that the initial density of wCcep in host eggs affects the timing and efficiency of horizontal transmission. Higher wCcep densities facilitated more rapid transmission, aligning with other findings. For example, Liu et al. (30) showed that increased Wolbachia inoculation frequency in Drosophila melanogaster led to higher infection densities. Meanwhile, Toomey et al. (31) identified high Wolbachia density as a critical factor for horizontal transmission in D. melanogaster. These collective findings suggest that the initial encounter density of Wolbachia is crucial not only for successful horizontal transmission but also for subsequent proliferation and vertical transmission to offspring in new hosts.
Notably, following the parasitization of wCcep-infected C. cephalonica eggs by uninfected T. chilonis, we observed a rapid initial increase in wCcep density in the offspring generation. However, this density did not consistently increase over subsequent generations; it exhibited significant fluctuations. We hypothesize that these generational density fluctuations may be attributed to the host’s innate immune response to the newly acquired Wolbachia, leading to unstable wCcep densities in the novel host (32).
Hu and Li (16) reported a case where wCcep successfully induced reproductive incompatibility in whiteflies after infection via microinjection. wCcep-infected C. cephalonica populations exhibited similar characteristics, suggesting its potential to induce cytoplasmic incompatibility in T. chilonis (unpublished data). Additionally, Wolbachia infection may affect reproduction and fitness, which will be further investigated in future studies.
5 Conclusions
The current study provides evidence through molecular analysis and re-infection trials demonstrating that Wolbachia (wCcep) can be transmitted from C. cephalonica to T. chilonis. We established a wCcep-free C. cephalonica colony over five generations using tetracycline. The transmission timing depends on population density, and wCcep can persist in T. chilonis for one to two generations. These findings are important for biological control programs using T. chilonis and managing Wolbachia infections in mass-rearing systems.
Data availability statement
The datasets presented in this study can be found in online repositories. The names of the repository/repositories and accession number(s) can be found in the article/Supplementary Material.
Ethics statement
The manuscript presents research on animals that do not require ethical approval for their study.
Author contributions
CL: Conceptualization, Methodology, Project administration, Writing – original draft. YH: Conceptualization, Methodology, Project administration, Writing – review & editing. LW: Data curation, Resources, Writing – review & editing.
Funding
The author(s) declare financial support was received for the research, authorship, and/or publication of this article. This research was funded by the Ministry of Science and Technology, Taiwan (MOST 111-2313-B-020-003-MY3) and by the Animal and Plant Health Inspection Agency (APHIA), Ministry of Agriculture (MOA) (112AS-5.5.3-BQ-B1).
Acknowledgments
We are grateful to the scientists who conducted and published their primary studies, the three editors, and the reviewers for their constructive comments on the manuscript.
Conflict of interest
The authors declare that the research was conducted in the absence of any commercial or financial relationships that could be construed as a potential conflict of interest.
Generative AI statement
The authors declare that no Generative AI was used in the creation of this manuscript.
Publisher’s note
All claims expressed in this article are solely those of the authors and do not necessarily represent those of their affiliated organizations, or those of the publisher, the editors and the reviewers. Any product that may be evaluated in this article, or claim that may be made by its manufacturer, is not guaranteed or endorsed by the publisher.
Supplementary material
The Supplementary Material for this article can be found online at: https://www.frontiersin.org/articles/10.3389/finsc.2024.1519986/full#supplementary-material
References
1. Weinert LA, Araujo-Jnr EV, Ahmed MZ, Welch JJ. The incidence of bacterial endosymbionts in terrestrial arthropods. Proc R Soc B. (2015) 282:20150249. doi: 10.1098/rspb.2015.0249
2. Ahmed MZ, Breinholt WJ, Kawahara YA. Evidence for common horizontal transmission of Wolbachia among butterflies and moths. BMC Evol Biol. (2016) 16:118. doi: 10.1186/s12862-016-0660-x
3. Kaur R, Shropshire JD, Cross KL, Leigh B, Mansueto AJ, Stewart V, et al. Living in the endosymbiotic world of Wolbachia: a centennial review. Cell Host Microbe. (2021) 29:879–93. doi: 10.1016/j.chom.2021.03.006
4. Pietri JE, Debruhl H, Sullivan W. The rich somatic life of. Wolbachia Microbiologyopen. (2016) 5:923–36. doi: 10.1002/mbo3.390
5. Werren JH, Zhang W, Guo LR. Evolution and phylogeny of Wolbachia: reproductive parasites of arthropods. Proc Biol Sci. (1995) 261:55–63. doi: 10.1098/rspb.1995.0117
6. Moreira LA, Iturbe-Ormaetxe I, Jeffery JA, Lu G, Pyke AT, Hedges LM, et al. A Wolbachia symbiont in Aedes aEgypti limits infection with Dengue, Chikungunya, and Plasmodium. Cell. (2009) 139:1268–78. doi: 10.1016/j.cell.2009.11.042
7. Micieli MV, Glaser RL. Somatic Wolbachia (Rickettsiales: Rickettsiaceae) levels in Culex quinquefasciatus and Culex pipiens (Diptera: Culicidae) and resistance to West Nile virus infection. J Med Entomol. (2014) 51:189–99. doi: 10.1603/me13152
8. Whitworth TL, Dawson RD, Magalon H, Baudry E. DNA barcoding cannot reliably identify species of the blowfly genus Protocalliphora (Diptera: Calliphoridae). Proc R Soc B. (2007) 274:1731–9. doi: 10.1098/rspb.2007.0062
9. Hoffmann AA, Montgomery BL, Popovici J, Iturbe-Ormaetxe I, Johnson PH, Muzzi F, et al. Successful establishment of Wolbachia in Aedes populations to suppress dengue transmission. Nature. (2011) 476:454–7. doi: 10.1038/nature10356
10. Zheng B, Chen LH, Sun QW. Analyzing the control of dengue by releasing Wolbachia-infected male mosquitoes through a delay differential equation model. Math Biosci Eng. (2019) 16:5531–50. doi: 10.3934/mbe.2019275
11. Towett-Kirui S, Morrow JL, Close S, Royer JE, Riegler M. Host–endoparasitoid–endosymbiont relationships: concealed Strepsiptera provide new twist to Wolbachia in Australian tephritid fruit flies. Environ Microbiol. (2021) 23:5587–604. doi: 10.1111/1462-2920.15715
12. Batista PD, Keddie BA, Dosdall LM, Harris HL. Phylogenetic placement and evidence for horizontal transfer of Wolbachia in Plutella xylostella (Lepidoptera: Plutellidae) and its parasitoid, Diadegma insulare (Hymenoptera: Ichneumonidae). Can Entomol. (2010) 142:57–64. doi: 10.4039/n09-801
13. Huigens ME, de Almeida RP, Boons PAH, Luck RF, Stouthamer R. Natural interspecific and intraspecific horizontal transfer of parthenogenesis-inducing Wolbachia in Trichogramma wasps. Proc R Soc Lond B Biol Sci. (2004) 271:509–15. doi: 10.1098/rspb.2003.2640
14. Wang N, Jia S, Xu H, Liu Y, Huang D. Multiple horizontal transfers of bacteriophage WO and host Wolbachia in fig wasps in a closed community. Front Microbiol. (2016) 7:136. doi: 10.3389/fmicb.2016.00136
15. Gao S, Ren Y, Su C, Zhu D. High levels of multiple phage WO infections and its evolutionary dynamics associated with Wolbachia-infected butterflies. Front Microbiol. (2022) 13:865227. doi: 10.3389/fmicb.2022.865227
16. Hu H, Li Z. A novel Wolbachia strain from the rice moth Corcyra cephalonica induces reproductive incompatibility in the whitefly Bemisia tabaci: sequence typing combined with phenotypic evidence. Environ Microbiol Rep. (2015) 7:508–15. doi: 10.1111/1758-2229.12279
17. Baldo L, Ayoub NA, Hayashi CY, Russell JA, Stahlhut JK, Werren JH. Insight into the routes of Wolbachia invasion: high levels of horizontal transfer in the spider genus Agelenopsis revealed by Wolbachia strain and mitochondrial DNA diversity. Mol Ecol. (2007) 17:557–69. doi: 10.1111/j.1365-294x.2007.03608.x
18. Zhang K, Han X, Hong X. Various infection status and molecular evidence for horizontal transmission and recombination of Wolbachia and Cardinium among rice planthoppers and related species. Insect Sci. (2012) 20:329–44. doi: 10.1111/j.1744-7917.2012.01537.x
19. Tseng S, Hsu P, Lee C, Wetterer JK, Hugel S, Wu LH, et al. Evidence for common horizontal transmission of Wolbachia among ants and ant crickets: kleptoparasitism added to the list. Microorganisms. (2020) 8:805. doi: 10.3390/microorganisms8060805
20. Lindsey AR, Stouthamer R. The effects of outbreeding on a parasitoid wasp fixed for infection with a parthenogenesis-inducing Wolbachia symbiont. Heredity. (2017) 119:411–7. doi: 10.1038/hdy.2017.53
21. Frydman HM, Li JM, Robson DN, Wieschaus E. Somatic stem cell niche tropism in. Wolbachia Nature. (2006) 441:509–12. doi: 10.1038/nature04756
22. Sanaei E, Charlat S, Engelstädter J. Wolbachia host shifts: routes, mechanisms, constraints and evolutionary consequences. Biol Rev. (2020) 96:433–53. doi: 10.1111/brv.12663
23. Wu LH, Hoffmann AA, Thomson LJ. Trichogramma parasitoids for control of Lepidopteran borers in Taiwan: species, life-history traits and. Wolbachia Infect J Appl Entomol. (2016) 140:353–63. doi: 10.1111/jen.12263
24. Wu LH, Hoffmann AA, Thomson LJ. Taiwanese Trichogramma of Asian corn borer: morphology, ITS-2 rDNA characterization, and natural Wolbachia infection. J Insect Sci. (2016) 16:22. doi: 10.1093/jisesa/iew006
25. Folmer O, Black MB, Hoeh WR, Lutz RA, Vrijenhoek RC. DNA primers for amplification of mitochondrial cytochrome c oxidase subunit I from diverse metazoan invertebrates. Mar Biotechnol. (1994) 3:294–9. https://www.mbari.org/wp-content/uploads/2016/01/Folmer_94MMBB.pdf.
26. Zhou W, Zhou W, Rousset F, O’Neill SL. Phylogeny and PCR–based classification of Wolbachia strains using wsp gene sequences. Proc R Soc B. (1998) 265:509–15. doi: 10.1098/RSPB.1998.0324
27. R Core Team. R: A language and environment for statistical computing. Vienna, Austria: R Foundation for Statistical Computing (2023). doi: 10.5281/zenodo.7931724
28. Huigens ME, Luck R, Klaassen RHG, R Maas MFPM, Timmermans MJTN, Stouthamer R. Infectious parthenogenesis. Nature. (2000) 405:178–9. doi: 10.1038/35012066
29. Watanabe M, Kageyama D, Miura K. Transfer of a parthenogenesis-inducing Wolbachia endosymbiont derived from Trichogramma dendrolimi into Trichogramma evanescens. J Invertebrate Pathol. (2013) 112:83–7. doi: 10.1016/J.JIP.2012.09.006
30. Liu XC, Li YR, Dong B, Li ZX. The intruding Wolbachia strain from the moth fails to establish itself in the fruit fly due to immune and exclusion reactions. Curr Microbiol. (2020) 77:2441–8. doi: 10.1007/S00284-020-02067-3
31. Toomey ME, Panaram K, Fast EM, Beatty C, Frydman HM. Evolutionarily conserved Wolbachia-encoded factors control pattern of stem-cell niche tropism in Drosophila ovaries and favor infection. P Natl Acad Sci USA. (2013) 110:10788–93. doi: 10.1073/PNAS.1301524110
32. Madhav M, Brown G, Morgan JA, Asgari S, McGraw EA, Munderloh UG, et al. Wolbachia successfully replicate in a newly established horn fly, Haematobia irritans irritans (L.) (Diptera: Muscidae) cell line. Pest Manag Sci. (2020) 76:2441–52. doi: 10.1002/ps.5785
Keywords: Wolbachia acquisition, symbiosis, Wolbachia persistence, biological control, climate impact
Citation: Lai CT, Hsiao YT and Wu L-H (2024) Evidence of horizontal transmission of Wolbachia wCcep in rice moths parasitized by Trichogramma chilonis and its persistence across generations. Front. Insect Sci. 4:1519986. doi: 10.3389/finsc.2024.1519986
Received: 30 October 2024; Accepted: 22 November 2024;
Published: 09 December 2024.
Edited by:
Xiaofeng Xia, Fujian Agriculture and Forestry University, ChinaReviewed by:
Yunqiu Yang, Anhui Agricultural University, ChinaShuzhong Li, Yangzhou University, China
Copyright © 2024 Lai, Hsiao and Wu. This is an open-access article distributed under the terms of the Creative Commons Attribution License (CC BY). The use, distribution or reproduction in other forums is permitted, provided the original author(s) and the copyright owner(s) are credited and that the original publication in this journal is cited, in accordance with accepted academic practice. No use, distribution or reproduction is permitted which does not comply with these terms.
*Correspondence: Li-Hsin Wu, bGloc2lud3V1QGdtYWlsLmNvbQ==
†These authors have contributed equally to this work and share first authorship
‡ORCID: Li-Hsin Wu, orcid.org/0000-0001-8176-3533