- Department of Entomology, University of Minnesota, St. Paul, MN, United States
The Japanese beetle, Popillia japonica Newman (Coleoptera: Scarabaeidae), an invasive species from northern Japan, was first detected in Minnesota in 1968. According to fruit growers and the Minnesota Department of Agriculture, population size and feeding damage has been an increasing concern since 2010. Based on trap-catch data, populations have recently exceeded 4,000 beetles/trap/week during July-August near raspberry fields, and can increase by an order of magnitude within 7-10 days. The primary goals of this study were to assess the spatial distribution of P. japonica adults in raspberry, and to develop and validate a practical fixed-precision sequential sampling plan for grower use. Taylor’s Power Law (TPL) regression was used to characterize the beetle’s spatial pattern in research plots and commercial fields, either with or without insecticide applications. We then used Green’s plan to develop an enumerative sequential sampling plan to estimate P. japonica density in primocane raspberry. Beetle population data were collected at two locations in southern Minnesota, including the Rosemount Research and Outreach Center, and a commercial field near Forest Lake. The TPL results, via slope comparisons, indicated no significant differences in P. japonica spatial pattern between insecticide treated plots versus untreated plots, or among 4 different insecticides (P>0.05). Utilizing all spatial pattern data, we characterized the distribution of P. japonica beetles to be highly aggregated in raspberry, with TPL slopes ranging from b = 1.38 to 1.55; all slopes were found to be >1.0. Although the slopes were not significantly different, we accounted for variability in spatial pattern by using 33 independent data sets, and the Resampling for Validation of Sampling Plans (RVSP) model to validate a sampling plan with a final average precision level of 0.25 (SEM/mean), recommended for integrated pest management (IPM) purposes. The final sampling plan required an average sample number of only 15, 1-m-row samples, while providing high relative net precision (RNP), and thus a cost-effective, efficient sample plan for growers.
Introduction
The Japanese beetle, Popillia japonica Newman (Coleoptera: Scarabaeidae), is native to northern Japan and highly invasive in the U.S. The beetle was first detected in New Jersey in 1916 (1, 2), and gradually spread west and southwest. Popillia japonica has now been documented in at least 31 states, and was first detected in Minnesota in 1968 (3). Since then, P. japonica populations remained relatively small and were primarily limited to urban areas within the 7-county metro area of Minneapolis & St. Paul (4). However, since 2010, P. japonica outbreaks have occurred more frequently in Minnesota, and have increasingly been a concern to growers of high-value fruit crops (5, 6). Popillia japonica adults are known to colonize over 300 wild and cultivated plant species across 79 families, resulting in a characteristic leaf “skeletonizing” feeding pattern (2). Adult feeding during July and August in the Midwest U.S. can quickly result in high defoliation rates on economically valuable hosts, particularly linden trees, roses, and numerous field and horticultural crops (2, 3, 6, 7). In addition to direct feeding damage, invasive arthropod species can often alter the feeding patterns of native pests (e.g., 8), and consequently disrupt existing Integrated Pest Management (IPM) programs (9, 10). Specifically, P. japonica has been shown to exacerbate feeding injury by a native insect, the Green June Beetle (Cotinis nitida), that is preferentially attracted to grapes damaged by P. japonica. In turn, yeast production and premature fermentation impacts fruit quality (8).
Among the most attractive fruit crops in the Midwest region, raspberries, apples and wine grapes are readily damaged (2, 5, 7, 11–13). In Minnesota, both summer (floricane) and fall (primocane) raspberries are significant sources of income to growers. DiGiacomo et al. (14) recently found that Driscoll Inc. (Watsonville, CA) documented high consumer demand for raspberries in Minnesota, where the Minneapolis-St. Paul market consumed 132% more fresh raspberries than the average U.S. household. High in vitamin C and K, with high antioxidant capacity, modern raspberry cultivars have been categorized as a “super food” (15). It was recently estimated that raspberry production in Minnesota yields 3.51 million pounds of marketable product ($8.42 lb/ac), valued annually at $70.6 million (14).
Because of the value of raspberries to the Minnesota fruit industry, many fruit growers utilize a “pick your own” production system, as the need for frequent harvests is labor intensive (14). However, scheduling both pest management activities, insecticide applications, as well as safe harvest dates can be challenging (6, 14). Raspberry is a perennial crop with one of two different harvest cycles; floricane is harvested throughout the summer (primarily July-August), and primocane harvested during autumn (August-October). Primocane raspberries are most commonly grown in Minnesota and are therefore at greater risk from insect pest feeding on foliage from the mid-late portion of the growing season (5, 7, 14). Popillia japonica prefer feeding on raspberry leaf tissue, even in the presence of other known host plants (16). Burkness et al. (11), in a 3-year study, found that the beetle attacked raspberry fruit ~20% of time. In addition to direct damage to fruit, feeding injury to foliage may also interfere with photosynthesis and late-season fruit production (17). Primocane raspberries also endure the most feeding pressure from P. japonica during the transition from vegetative growth to fruiting, as sucrose and nutrients are stored for berry production during July and August (17); this period also coincides with peak flights of P. japonica (7, 18).
Considerable Integrated Pest Management (IPM) research to date has focused on the P. japonica larval (white grub) stage of the insect’s life cycle (1, 3, 19). However, relatively little applied research in the Midwest U.S. has been directed toward adult P. japonica ecology or an understanding of the impact on fruit crops, including yield or quality of raspberries. With concerns about the beetle’s rapid colonization of fruit crops (e.g., 5, 6, 11), and that the highly visible impact of feeding damage may increase insecticide use, growers are currently in need of an effective monitoring tool to estimate the number of P. japonica adults present, and thus make objective IPM decisions. The primary control practice currently includes the use of foliar insecticides soon after finding a few P. japonica beetles, or high levels of defoliation. In addition, the process of sampling for beetles, or estimating defoliation is a challenge, depending on the experience of growers or consultants (e.g., 1). As part of our research to develop new IPM programs, monitoring systems are needed to track beetle population trends, such as the use of semiochemical-baited traps to understand regional pest pressure (5). In addition, for individual crops such as raspberries, statistically sound sampling methods are necessary for future use with economic or “action” thresholds, to better assess if and when insecticide applications are necessary (20).
Although time-saving, sequential sampling plans are available to assist with sampling plan design (21, 22), the challenge is that the “fixed precision level” proposed for enumerative plans (e.g., 23) is actually variable for any given sampling session (or bout) (24). The desired precision level is an expectation that assumes multiple sampling sessions. To overcome this concern and to assist with sampling plan validation, Naranjo and Hutchison (25) developed the Resampling for Validation of Sample Plans (RVSP) program to allow researchers to quantify how the variability in actual precision levels obtained based on actual sampling data sets. This approach has proven useful for a variety of arthropod species, and for the validation of both enumerative (25–27) and presence-absence (28–30) sampling plans.
Given the high value of raspberries in Minnesota (14), and the subsequent use of multiple insecticides for P. japonica, we were motivated to develop and validate a statistically sound sampling plan for grower use. To do so, we utilized the well-known Taylor’s Power Law regression (31) to characterize the spatial pattern of P. japonica adults, and the RVSP resampling approach to validate a cost-effective sequential sampling plan (23, 25). To provide additional efficiency, we examined the beetle’s spatial pattern within the raspberry canopy, to minimize the area sampled.
Materials and methods
Sample data
The primary data sets used for sampling P. japonica adults, without insecticide use, were collected during the summers of 2018, 2020, and 2021, using a primocane (fall bearing) variety, ‘Heritage.’ The primary research field site was located at the Rosemount Research & Outreach Center, University of Minnesota (ROC-North, 44.71520N, -93.09744W). Raspberries were maintained using standard production guidelines for fertility, and supplemental irrigation as needed (11, 32). This site (~ 0.1 ha) consisted of several 2-row research plots (4.6m long), with rows separated by 3.1 m of turf maintained between rows to minimize erosion. In 2020, 22 data sets were collected from 5 July to 3 September; in 2021, 34 data sets were collected from 5 July to 30 August. In addition, in 2018, four high-density samples were collected in commercial fields of primocane (fall bearing) raspberries, ‘Prelude’ and ‘Nova,’ at a site near Forest Lake, MN (45.22832N, -92.89175W). These samples were collected prior to insecticide use. The field size, including both varieties was ~0.2 ha, with row spacing at 3.1m, also separated by turf between rows. In total, 60 data sets from untreated research plots and the commercial field were available for analysis. For all samples, the same 1-m row, canopy sample unit was used. On each sample date, 16 to 60 randomly selected 1-m row samples were taken using a visual whole canopy inspection, to record adult P. japonica densities (e.g., 5). Each data set was then used to calculate the mean and variance for sampling plan development and validation.
In addition to the ROC-North site, a second site, ROC-South (44.69104N, -93.07326W), was also established using ‘Heritage’ raspberry, where an insecticide study was conducted to assess efficacy and spatial pattern of P. japonica adults; this was ~4.5km south of ROC-North. ROC-south included 8 eight quadrants running east to west, where each quadrant contained 11, 3.1m rows of ‘Heritage’ raspberries separated by 3.1m turf alleys as previously described. As with ROC-north, the site was maintained using standard production guidelines for fertility, and supplemental irrigation as needed. The plots were assigned to 5 insecticide treatments, with 4 replications each, in a randomized complete block design (RCBD). The insecticide study in 2020 was conducted with sprays applied every two weeks from July 10 to August 28. Carbaryl (Sevin®, Monsanto, St. Louis MO), and spinosad (Entrust®, Corteva, Wilmington DE) were applied at rates of 32.0 and 6.0 oz of product/ac, respectively in a randomized complete block design. The zeta-cypermethrin (Mustang Maxx®, FMC, Philadelphia PA.) treatment, at a rate of 4.0 oz of product/ac, was applied weekly with the intent to maintain a “beetle free” zone. In 2021, the same trial was conducted at ROC-south, however, the spinosad treatment was replaced with acetamiprid (Assail®, United Phosphorous Inc., Bandra, East Mumbai), at a rate of 4.5oz of product/ac. In 2021, carbaryl and zeta-cypermethrin were applied at the same rates and intervals as in 2020. As with all other sampling data, the 1-m-row sample unit was used. Total data sets available for spatial pattern analysis ranged from 22-27, depending on the insecticide. In addition, a total of 16 data sets (4 data sets for each of 4 insecticides) were set aside prior to analysis, for sampling plan validation (25). Adult beetles from each study were identified by external anatomy and coloration unique to P. japonica (3, 19) and validated by comparisons to specimens previously deposited to the Insect Museum in the Department of Entomology, University of Minnesota.
Spatial pattern
We examined the spatial distribution of P. japonica adults using Taylor’s Power Law (TPL) which is based on a logarithmic relationship between the sample variance (s2) and the sample mean (m) (31), such that:
In practice, a and b are estimated by linear regression of log(s2) as a function of log(m), where b is the slope, and index of aggregation. In theory, the value of b is independent of mean density and is relatively “constant” for a species in a given environment (31). Among many aggregation indices, TPL has been found to provide robust estimates of spatial pattern (e.g., 22, 26, 28). During 2020-2021, a total of 60 data sets collected from raspberry plots not treated with insecticides were used to calculate the mean/variance estimates. The TPL regression was used to quantify the relationship between log variance and log mean, for 43 of the 60 population density samples. The remaining 17 samples were selected to reflect a density range from low-high, and set aside as independent data sets to be used for RVSP validation analysis (see below). The slope of the TPL regression line (b) is indicative of the spatial pattern of the population sampled; i.e., if b < 1, b = 1, or b > 1, the population can be characterized as having a uniform, random, or aggregated spatial distribution, respectively (22). In addition to the primary TPL regression (N=43), the spatial pattern for P. japonica adults was determined by comparing the slopes for the TPL regressions for the 4 insecticides mentioned previously, as well as the untreated plots. The total number of sample dates per insecticide ranged from 22-26, for each of the two years. All regressions were conducted using RStudio (33).
Enumerative sequential sampling
Development of the sampling plan was based on Green’s (23) “stop-line” model to estimate population density, after a successive number of samples are taken, using the formula:
where Tn is the cumulative number of individuals sampled for n samples, a is the antilog of a from the TPL regression, b is the slope from TPL, SE/m = D for precision level (e.g., D=0.10, 0.25). With this plan, one continues to take successive samples until the appropriate Tn is exceeded to estimate insect density, for the desired precision level.
Validation of Green’s plan was conducted by selecting 17 of the 60 sample data sets taken over two years at ROC-North prior to calculating TPL values, for use as independent data sets for validation (Naranjo & Hutchison 2007). The 17 data sets for validation were selected to represent a range of population densities from low to high. In addition, because of the potential variation in spatial pattern for insecticide treated plots, 16 sample dates were also selected from the insecticide study (4 samples for each of 4 insecticides), for a total of 33 validation data sets; for all validation data sets, the observed mean density ranged from 0.75 to 28.1 beetles per 1-m row. All validation data were then used with the TPL parameter estimates (a, b values) and Green’s plan to evaluate plan performance, via the Resampling for Validation of Sample Plans (RVSP) program (25). Using the RVSP package, during each sampling session (bout), the resampling method assumes that a unique set of random samples are taken to estimate density to mimic one taking samples at random in the field. Final plan performance is assessed after the sampling process has been repeated (simulated) 500 times for each data set. The outcomes for the actual precision levels achieved for each RVSP simulation, are compared to the desired precision, and subsequently changed, as an iterative process to achieve the final goals of D=0.10 and D=0.25, for research and IPM purposes, respectively (34). In addition, RVSP provides the predicted average population density, for comparison with actual population density, and average sample number (ASN) for each of the 500 simulation runs. Once the ASN was determined for each precision level, we also calculated Relative Net Precision (RNP), to compare the efficiency of each sampling plan based on the sampling cost and precision (27, 35),
where RV is relative variation (SE/mean) * 100 (34), and c is the total cost (in time) for collecting the selected sample, usually measured in person-hours.
Canopy strata
To further improve the efficiency of sampling, and reduce sampling cost via a more refined sample unit, we conducted a study in 2021 to determine how P. japonica beetles were distributed within the raspberry canopy. On 10 separate sample dates in 2021, untreated ‘Heritage’ raspberry plots at ROC-North were used to visually sample the top-third (0-15 cm), middle-third (16-30 cm), and bottom-third (31-45 cm) strata of the canopy, to assess the potential for differential beetle density among strata. Nondestructive random sampling, using the 1-m-row sample unit, was conducted on each sampling date (July-August), by selecting five plants at random, within the middle 2 rows/plot, in each of four quadrats. The insect strata data was transformed using a square-root transformation [√(x+0.5)], where x is the number of adults recorded per sample per date. The mean number of beetles per 1-m-row, and SEMs, and the proportion of beetles in each strata were calculated for analysis. Prior to analysis, the proportion data were arc-sine transformed. The strata density data were analyzed using ANOVA with RStudio (33), and Tukey’s HSD test for means comparison (P=0.05).
Results
Spatial pattern
Taylor’s Power Law regression analyses of log-variance as a function of the log-mean, for P. japonica adults indicated a strong positive relationship for beetle populations, as measured by the 1-m-row sample unit (Figure 1). The pattern was consistent regardless of the insecticide used or if the data were collected from untreated plots. For all treatment regressions, R2 estimates ranged from 0.79-0.89. Importantly, the TPL slope (b) values for all data sets, either from untreated or insecticide treated plots, were statistically greater than 1, (P<0.05, Table 1), indicating an aggregated spatial pattern for P. japonica adults in raspberry. We also did not find significant differences in slopes between the insecticide treated and untreated plots, nor differences among the four insecticides (P>0.05, Table 1).
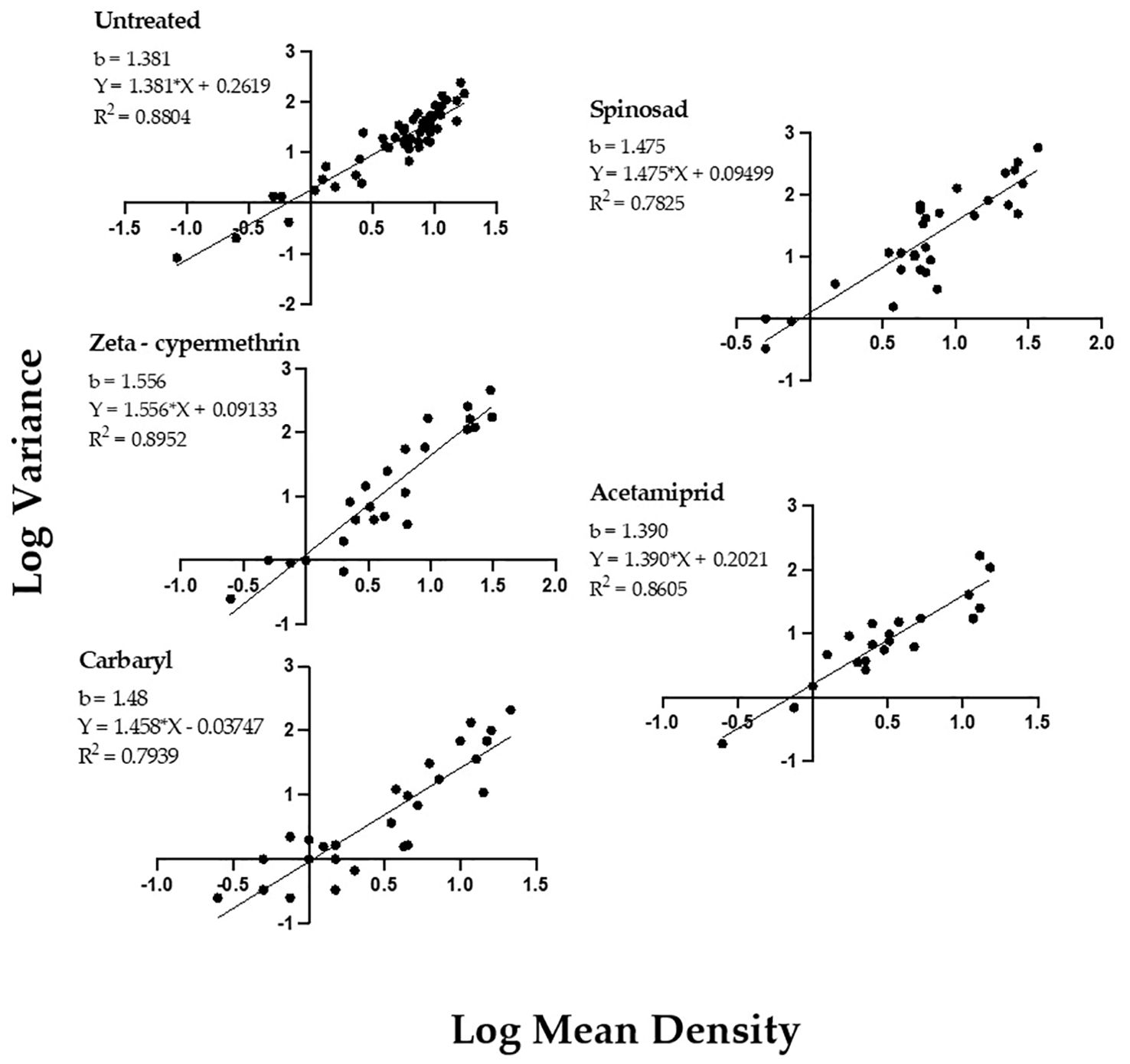
Figure 1. Taylor’s Power Law for P. japonica adult density and variance (log transformed), collected on primocane (‘Heritage’) raspberry, Rosemount MN, Forest Lake MN 2020, and Rosemount MN 2021 (see also Table 1).
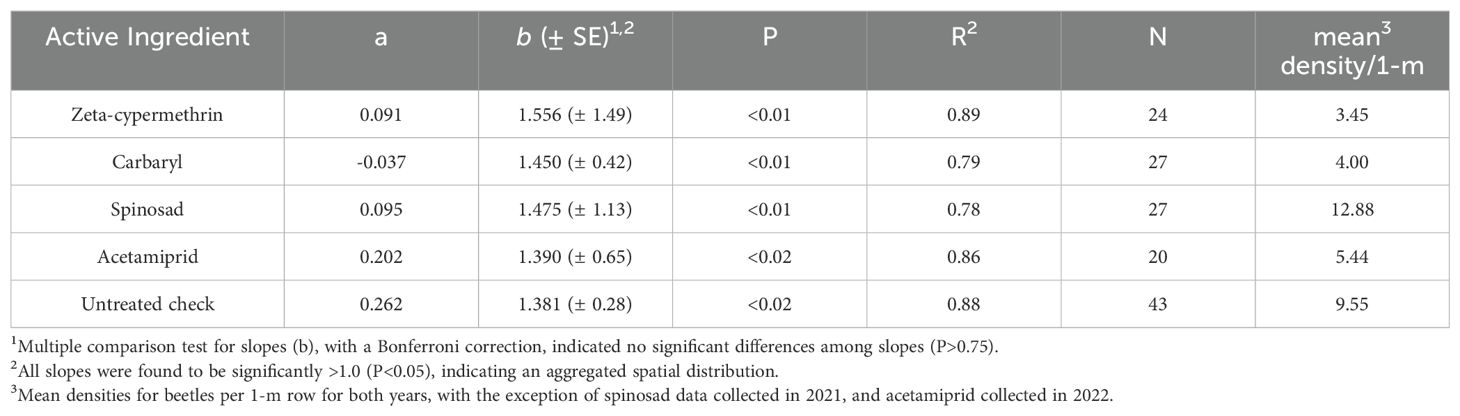
Table 1. Taylor’s Power Law slope comparisons and mean density for P. japonica adults in raspberry, where sampling was conducted in untreated plots, and those treated with foliar insecticides, Rosemount, MN, 2021-2022.
Enumerative sequential sampling and validation
The development and validation of Green’s sequential sampling plan, via RVSP, suggested that to achieve an observed average precision level (D) of ~0.10, a high average sample size of 106 sample units are required (Table 2). By contrast, to achieve an average precision level (D) of ~0.25 for IPM decision-making, an average sample size of only 15, 1-m-row samples are required (Table 3). The initial ‘desired precision’ levels specified in the RVSP validation were higher than expected, and were therefore decreased to 0.21 and 0.08, to achieve the desired actual precision levels of 0.25 and 0.10, respectively. This option with RVSP is often necessary to adjust (fine-tune) the precision levels to determine optimum final sample size (26, 27). Based on RVSP analysis, average maximum and minimum sample sizes were 23 and 15 for a precision levels of D = 0.25. By contrast, average maximum and minimum sample sizes were 123 and 106 were necessary for a precision level of D = 0.10. The full range of expected, average sample size requirements are illustrated in Figure 2 (see also Supplementary Table S1). Finally, the results for sampling efficiency, based on RNP, which includes the time to take samples for a given ASN at each precision level are shown in Table 4. As expected, the RNP was much higher, and most cost-effective (21.40) for the IPM-based precision level of D = 0.25.
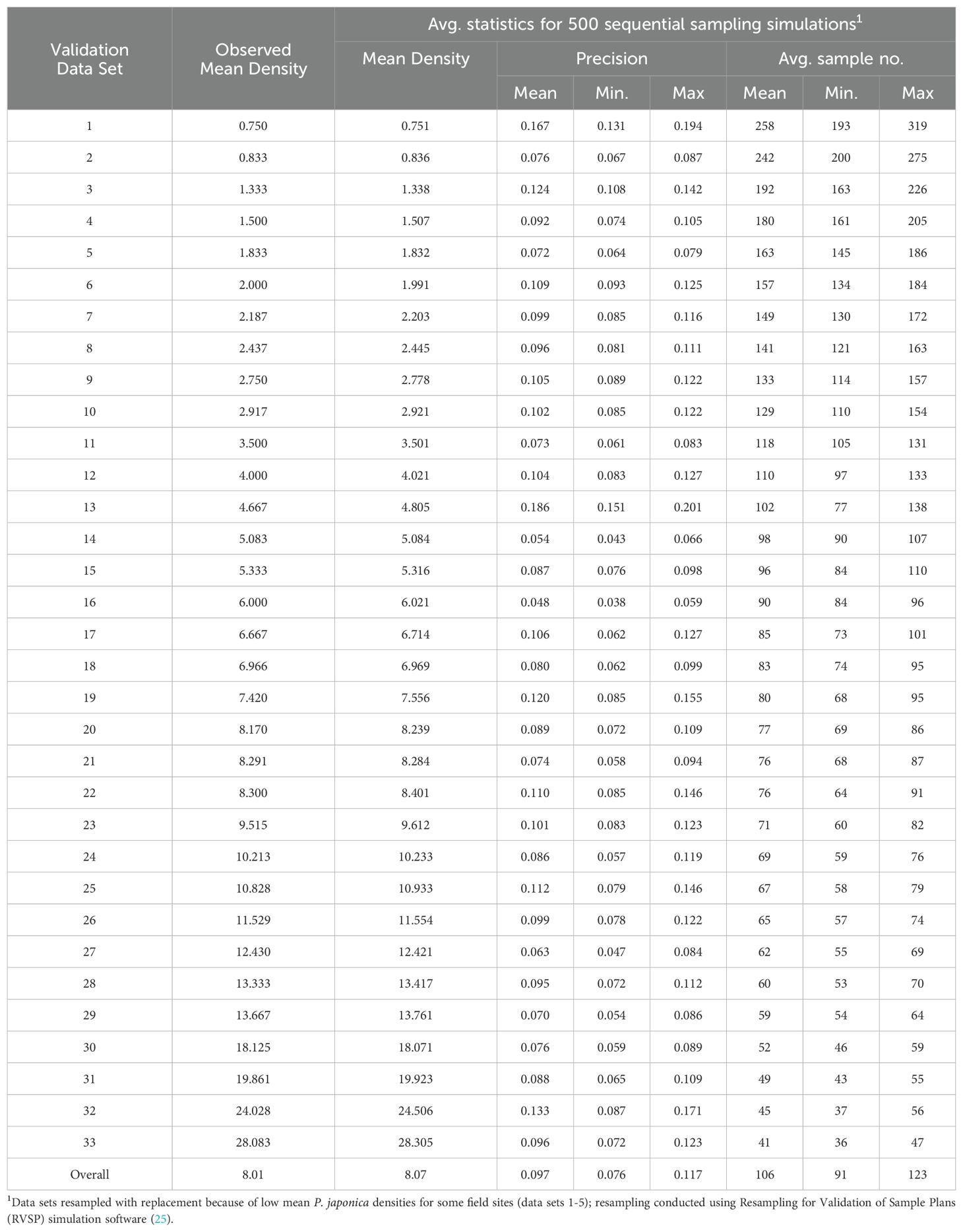
Table 2. Resampling simulations used to validate a fixed precision, sequential sampling plan (23), for P. japonica adult density (1-m-row), by using a pre-set precision level of 0.08 (desired 0.10), via Taylor’s Power Law (a = 1.83, and b = 1.38).
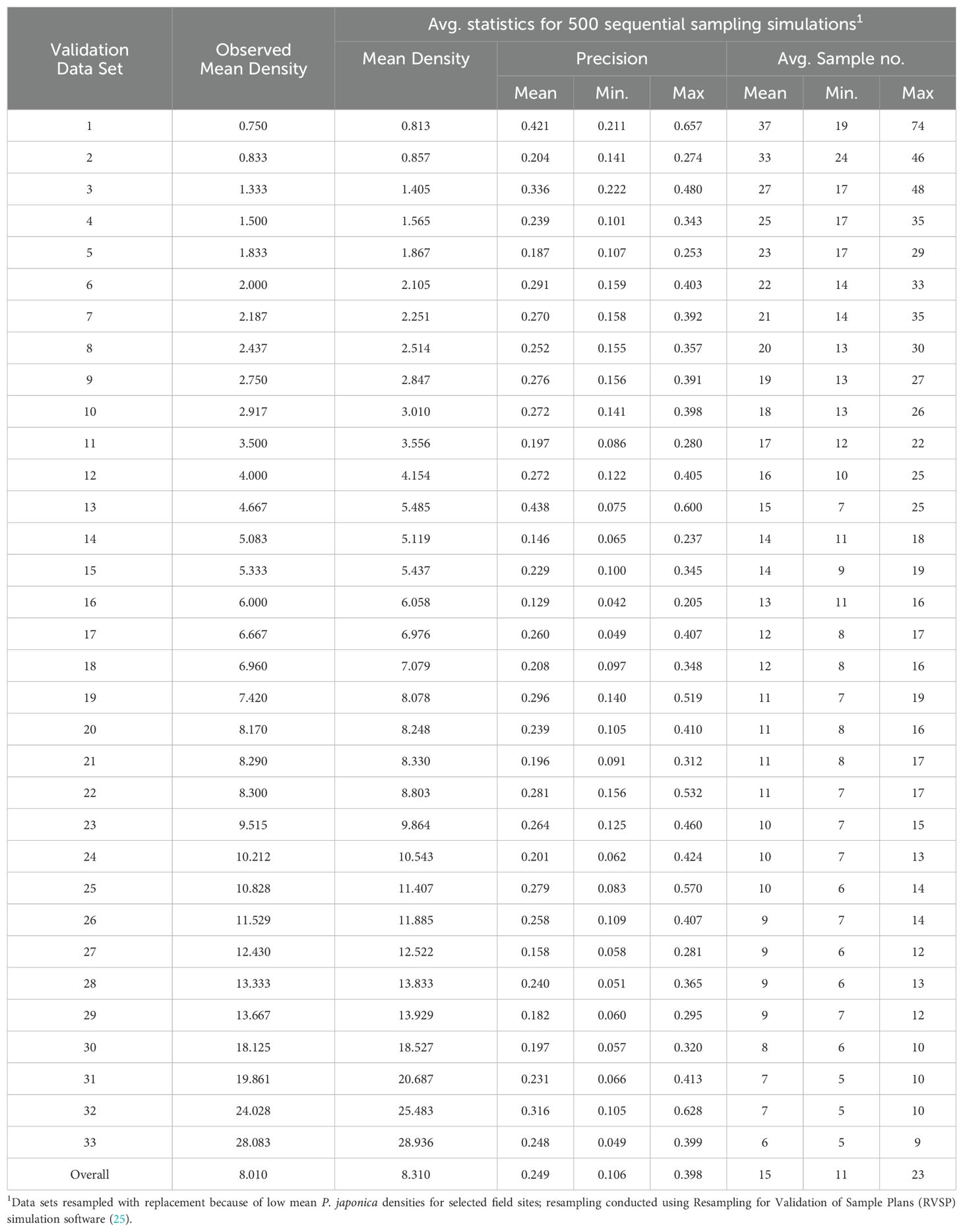
Table 3. Resampling simulations used to validate a fixed precision, sequential sampling plan (23), for P. japonica adult density (1-m-row), by using a pre-set precision level of 0.22 (desired 0.25), via Taylor’s Power Law (a = 1.83, and b = 1.38).
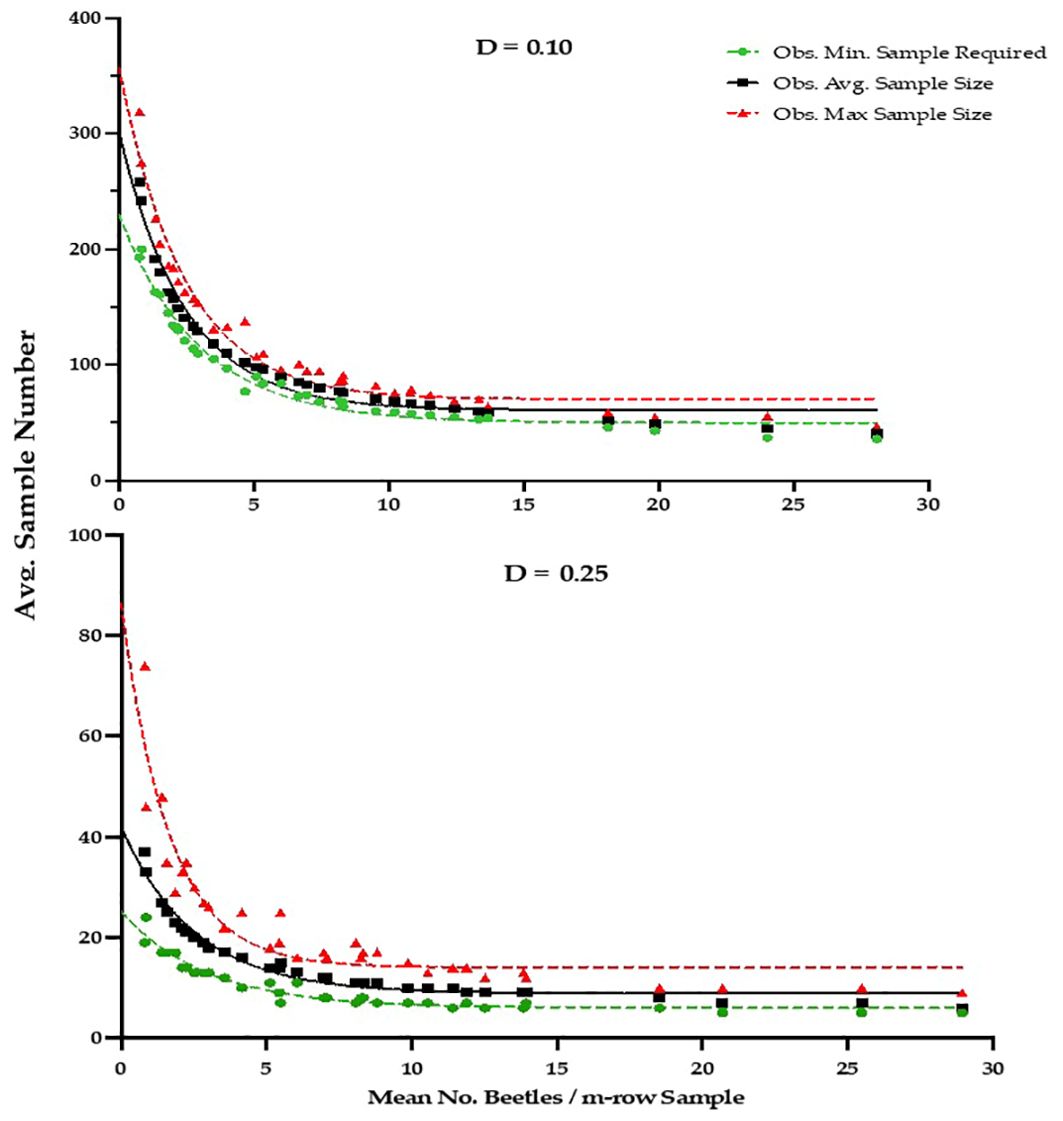
Figure 2. Observed Average, Minimum and Maximum Sample size results from RSVP Validation analysis for desired precision levels of D=0.10 and D=0.25, based on Green’s Sequential Sampling Plan for P. japonica adults, using a 1-m-row sampling unit in raspberry, Rosemount MN, 2020-2021 (see Supplementary Table S1 for fitted equations for each precision level).
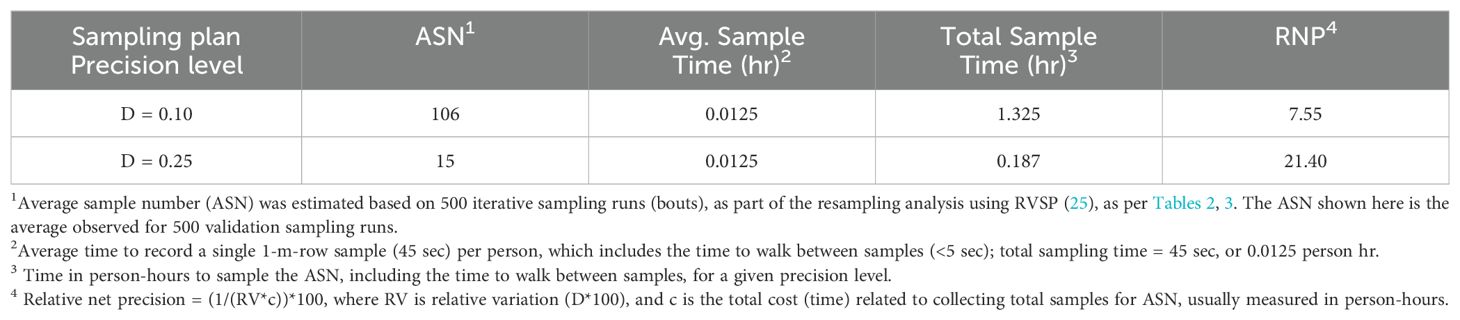
Table 4. Efficiency of two fixed-precision sequential sampling plans for P. japonica adults in raspberry, as measured by relative net precision (RNP).
Canopy strata
The canopy strata distribution study, where the canopy was equally partitioned vertically across three equally spaced strata (15 cm intervals), indicated significant differences in P. japonica beetle density (P<0.05, Table 5). Over the course of 10 sample dates and using the 1-m-row sample unit, a significantly higher number of beetles were observed in the top 1/3 of the canopy (P<0.05); there were also significant differences between the mid- and bottom 1/3 strata. Likewise, the mean proportion of beetles found varied significantly by strata (P<0.05, Table 5), with 78.8% of the P. japonica adults found in the top 1/3 of the canopy. These results suggest that additional savings in sampling time could be reduced by focusing the sampling effort on the top 1/3 of the canopy.
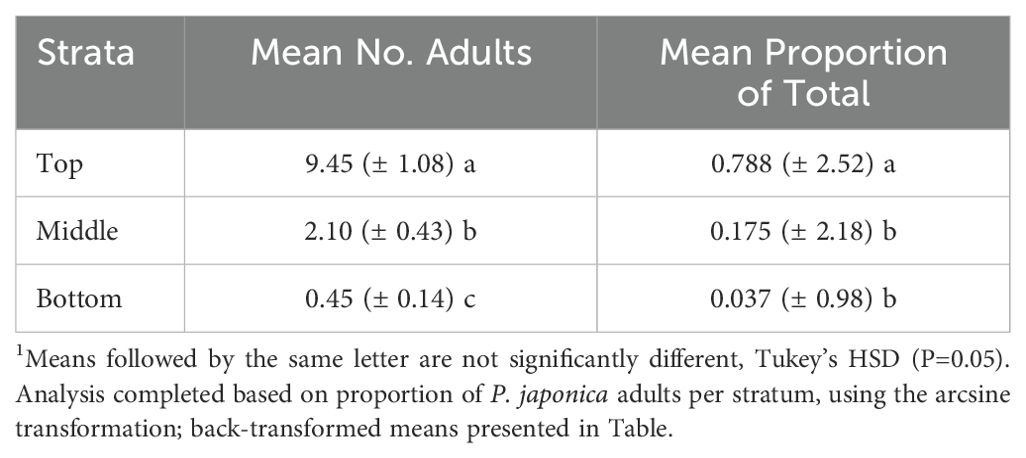
Table 5. Mean (+/-SEM)1 number of P. japonica adults per m-row for three strata (15-cm intervals) within a raspberry (‘Heritage’) canopy (no insecticide sprays), Rosemount MN, 2021.
Discussion
In this study we found that P. japonica adults were highly aggregated on primocane (fall-bearing) raspberries regardless of whether insecticides had been applied. Although the insecticides included in this study can reduce beetle densities differentially (36), our results indicate that the sampling plan presented is robust and applicable to commercial field situations for IPM decision-making. Aggregation behavior of P. japonica adults can be attributed to several factors related to the beetle’s ecology. First, mated females usually oviposit in moist or irrigated soil supporting turf grass or nearby pastures (1, 19), where subsequent grub (larval) populations are also known to exhibit an aggregated spatial pattern (37, 38). Secondly, P. japonica adults emerging from these source populations each summer are often aggregated as well. Sara et al. (39) observed an aggregated distribution of P. japonica adults in soybean, where beetle density was most pronounced along field edges. More recently, a similar trend was observed for P. japonica adults in commercial vineyards (13). Thirdly, a primary mechanism responsible for initial beetle aggregations on several plant species is attributed to the release of volatile organic compounds (VOCs) in response to beetle feeding. For example, Loughrin et al. (40, 41) quantified the attraction of P. japonica adult aggregations to several VOCs emanating from crab apple and wine grapes, including floral kairomones (e.g., pbenethanol, linalool) or fruit-2like volatiles [e.g., (Z)-3-hexenyl butyrate, (Z)-3-hexenyl benzoate)]. The VOCs are released soon after P. japonica adults initiate feeding. Although VOCs have not been documented for raspberry, a similar phenomenon could be responsible for the aggregation phenomenon observed on raspberry (see also 11). Finally, once high numbers of adult P. japonica adults have colonized host plants, the release of the female sex pheromone also attracts additional males to the same feeding sites (1).
An extensive entomological literature has shown that the use of Taylor’s Power Law regression provides a reliable approach to characterizing spatial pattern across a diversity of arthropod taxa (21, 24, 25, 31). Moreover, with the development of Green’s (23) sequential sampling plan for estimating population density, the slope of the TPL regression was found to be useful for developing practical sampling plans, with designated average precision levels (21, 26). However, despite the reliability of the TPL regression, and other measures of spatial aggregation, additional research affirmed that such measures serve only as initial estimates of spatial pattern, reflecting a continuum from random to aggregated, rather than a fixed index (24). Like other ecological parameters spatial pattern estimates are dependent on sample size, host crops, or external variables such as insecticide use. Importantly, an additional key factor affecting the final performance of a sampling plan is the stochastic nature of the sequential sampling process itself; i.e., each time a plan is implemented (sampling bout), a different set of plants are sampled and different arthropod densities are encountered, all of which yields a slightly different estimate of pest density and the final precision level obtained (21, 24, 25).
These findings prompted the development of a bootstrap, or resampling approach to develop and validate sequential sampling plans that would incorporate both sources of variation (24). As illustrated by Naranjo and Hutchison (25), the model RVSP was developed to provide a validation process for sequential sampling plans using actual insect sampling data sets, versus a theoretical distribution such as the negative binomial. The resampling approach is a form of bootstrap sampling, where independent data sets for a given species are used to assess the actual precision of a sampling plan, allowing for more flexibility in building sampling plans based on realistic spatial patterns (22, 25, 26). In addition, as an iterative process it is used to modify the pre-set precision levels, to eventually achieve the desired precision and reasonable ASN. This is particularly useful for IPM applications. By contrast, traditional plans that are not validated can lead to unnecessarily high sample sizes, that are too time-consuming (25). The simulations can also be processed in a matter of seconds. Thus, the initial pre-set precision can and should be adjusted as needed to reach the desired observed precision levels for the sampling plan to be effective. For P. japonica we therefore adjusted the precision levels to 0.08 and 0.21 in the final RVSP simulations to achieve the desired actual precision of D=0.10 and 0.25, respectively. The results of Green’s sequential sampling plan also indicate that as the density of P. japonica increases, fewer samples are required to determine adult density (Tables 2, 3, Figure 2).
The strata study indicated that the majority of P. japonica adults are found in the top third of the canopy (Table 5). Feeding by P. japonica in the upper strata of crop canopies has also been documented in wine grapes (5) and soybean (42). Feeding in the upper strata of various crops has been attributed to their attraction to sunlight (UV) or the nutritional value of feeding on younger leaves (1, 11). This information will be helpful for growers and crop consultants as they can focus their crop inspections more efficiently within the upper canopy strata. Finally, although a formal time-of-day study was not conducted, we found that beetle activity was most noticeable between 11am to 5pm, and that beetle counts too early in the morning could lead to underestimates of the actual infestation levels.
To our knowledge, this is the first study to document the spatial pattern of P. japonica adults in raspberry. However, previous work with P. japonica has shown that the adults also exhibit aggregated distributions, when beetles were sampled in wine grapes where a strong “edge effect” was noted (13), or when trapping beetles in semiochemical-baited traps (5). It is also notable that the strong linear TPL relationship for P. japonica adults is similar to the TPL results found for other beetle species (e.g., 26). Regardless of the biological basis for aggregation pattern in the field, it is well known that the subsequent sampling plans for such species, often necessitates higher sample sizes and costs, compared to sampling plans for species characterized by random spatial patterns (25).
Our sequential sampling plan indicates that, on average, only 15 samples are necessary to estimate adult P. japonica population density in raspberry, when using the IPM based precision level (D) of 0.25 (Table 3). With a sample time of 30-45 sec, and the time to walk between samples averaging 5 sec, we assumed a final conservative estimate of 45 sec for total time to take a 1-m-row sample. Thus a sample size of 15 would equate to ~11 minutes per field site, which is a reasonable time frame for growers and crop consultants to make control decisions (Table 4). When beetle densities are relatively moderate to high, the sampling time will be much less (Figure 2). The combination of a brief sampling time and a validated precision level (0.25 for IPM), suggests a high level of efficiency in sampling adults. Popillia japonica often overwinter in loam-clay soils and prefer moist turf (1, 43), which is commonly grown between raspberry rows. This should inform growers that once a population is established, there will continue to be moderate to high beetle pressure in the foreseeable future making an effective sampling plan imperative. Recent studies in Minnesota suggest that P. japonica adult emergence begins during late June (6, 7); therefore growers and consultants in the upper Midwest region should begin sampling by mid-July to catch peak beetle activity, and begin sampling efforts.
Although the pattern for aggregation of P. japonica adults was consistent among all insecticides tested (b >1.0), the differences observed in population density and corresponding ASNs (Tables 2, 3) was not surprising given the known differences in efficacy of insecticides for this species (36); there may also be sub-lethal or behavioral effects on the beetles in treated plots, that may also differentially affect spatial pattern. For example, Burkness et al. (36) found that zeta-cypermethrin (Mustang Maxx) consistently reduces beetle populations to <5 beetles/m-row, for up to 2 weeks following an application, resulting in several sample dates with low densities. As shown for other insect species, low densities may lead to a more random spatial pattern. Moreover, the change from aggregated to random is more of a continuum versus an abrupt change of the TPL slope from b=1.0 to b>1.0 (22).
The primary production practice for raspberries in Minnesota is the use of fall bearing varieties (14) because they yield well, and are less labor-intensive than summer raspberries that require intense labor for pruning each season. In recent years, because of the establishment of another invasive, spotted-wing Drosophila (Drosophila suzukii), more growers are beginning to transition toward summer bearing raspberries (14). Although our research was conducted primarily with the fall bearing ‘Heritage’ variety, we believe the proposed sampling method should be applicable to summer bearing raspberries as well. Fall and summer bearing raspberries share similar canopy growth patterns, with ample foliage produced during summer-fall growth periods, when P. japonica adults are most active in the Midwest region (7). However, because multiple varieties are grown in the region, further research is needed to determine the degree to which P. japonica adults show similar aggregation behavior, and thus changes in spatial aggregation that could affect sampling plan recommendations. In addition, more work should be done with other varieties to evaluate the degree of fruit feeding by P. japonica beetles, as this may require more targeted sampling on fruit and flowers versus foliage late in the season. As P. japonica continues to colonize crops in Europe (44–48), and global climate change continues to facilitate invasive pest expansion (49, 50), it will be critical for researchers to develop innovative monitoring tools for both surveillance and IPM applications to minimize excess insecticide use and respond to grower challenges.
In summary, our study shows that P. japonica adults exhibit an aggregated distribution on fall bearing ‘Heritage’ raspberries in Minnesota. Despite a high level of aggregation, the validation analysis, when using Green’s sequential sampling plan, requires an average of only 15 1-m-row samples to estimate the population density, at an average precision level (D) of 0.25, recommended for IPM decision-making (25). As with other sequential sampling plans, more samples are necessary at low densities (e.g., < 5 beetles/sample). For the high density range of 10 to 25 beetles/sample unit, the ASN continued to decline, especially for D=0.25, but ranged from 8-15 samples, respectively (Table 3, Figure 2). The sequential sampling plan for P. japonica on raspberry should be useful to growers and crop consultants, by providing statistically sound estimates of population density, with a reasonable sample size and cost. Finally, research is underway in Minnesota to determine economic injury levels, and a practical economic threshold for P. japonica in raspberry. The sampling plan can then be used in tandem with an effective economic threshold for P. japonica adults, to further build an IPM program for fall bearing raspberry in the Midwest U.S.
Data availability statement
The original contributions presented in the study are included in the article/Supplementary Material. Further inquiries can be directed to the corresponding author.
Ethics statement
The manuscript presents research on animals that do not require ethical approval for their study.
Author contributions
AT: Data curation, Formal analysis, Investigation, Methodology, Validation, Writing – original draft, Writing – review & editing. EB: Conceptualization, Data curation, Methodology, Supervision, Validation, Writing – review & editing. WH: Conceptualization, Data curation, Formal analysis, Funding acquisition, Investigation, Methodology, Project administration, Resources, Supervision, Validation, Writing – review & editing.
Funding
The author(s) declare financial support was received for the research, authorship, and/or publication of this article. The study was funded by support from the AES (Agric. Expt. Station) Rapid Agric. Response Fund (award, 081823) and USDA-Minn. Dept. of Agric. Specialty Crops Block grants (awards: 0070811, 0092341), and the University of Minnesota, AES, St. Paul, MN.
Acknowledgments
We thank Oliver McEllistrem, Sally GA Nelson, and Dr. Dominique Ebbenga (UMN Fruit & Vegetable IPM Lab) for assistance with the field research, and acknowledge Drs. Robert Koch and Mary Rogers (UMN) for providing helpful reviews for a previous version of the manuscript.
Conflict of interest
The authors declare that the research was conducted in the absence of any commercial or financial relationships that could be construed as a potential conflict of interest.
The author WH declared that they were an editorial board member of Frontiers, at the time of submission. This had no impact on the peer review process and the final decision.
Publisher’s note
All claims expressed in this article are solely those of the authors and do not necessarily represent those of their affiliated organizations, or those of the publisher, the editors and the reviewers. Any product that may be evaluated in this article, or claim that may be made by its manufacturer, is not guaranteed or endorsed by the publisher.
Supplementary material
The Supplementary Material for this article can be found online at: https://www.frontiersin.org/articles/10.3389/finsc.2024.1465829/full#supplementary-material
References
1. Potter DA, Held DW. Biology and management of the Japanese beetle. Annu Rev Entomol. (2002) 47:175–205. doi: 10.1146/annurev.ento.47.091201.145153
2. Althoff EM, Rice KB. Japanese beetle (Coleoptera: Scarabaeidae) invasion of North America: history, ecology, and management. J Integ Pest Manage. (2022) 13:1–11. doi: 10.1093/jipm/pmab043
3. Shanovich HN, Dean AN, Koch RL, Hodgson E. Biology and management of Japanese Beetle (Coleoptera: Scarabaeidae) in corn and soybean. J Integr Pest Manage. (2019) 10:9. doi: 10.1093/jipm/pmz009
4. Wawrzynski RP, Ascerno ME. Mass trapping for Japanese beetle (Coleoptera: Scarabaeidae) suppression in isolated areas. J Arboricult. (1998) . 24:303–7. doi: 10.48044/jauf.1998.038
5. Ebbenga DN, Burkness EC, Clark MD, Hutchison WD. Impact of adult Popillia japonica (Coleoptera: Scarabaeidae) foliar feeding injury on fruit yield and quality of a temperate, cold-hardy wine grape, ‘Frontenac’. Front Insect Sci. (2022) 2:887659. doi: 10.3389/finsc.2022.887659
6. Ebbenga DN, Wold-Burkness SJ, Burkness EC, Hutchison WD. Japanese beetle as an Emerging Fruit Pest, in: Fruit Edge (2023). St. Paul, MN: University of Minnesota, Extension. Available online at: https://fruitedge.umn.edu/Japanese-beetle/Japanese-beetle-emerging-pest-fruit-crops (Accessed June 15, 2024).
7. Ebbenga DN, Burkness EC, Hutchison WD. Optimizing the use of semiochemical-based traps for efficient monitoring of Popillia japonica (Coleoptera: Scarabaeidae): Validation of a volumetric approach. J Econ Entomol. (2022) 115:869–76. doi: 10.1093/jee/toac049
8. Hammons DL, Kurtural SK, Newman MC, Potter DA. Invasive Japanese beetles facilitate aggregation and injury by a native scarab pest of ripening fruits. Proc Nat Acad Sci. (2009) 106:3686–91. doi: 10.1073/pnas.0811097106
9. Leskey TC, Nielsen AL. Impact of the invasive Brown Marmorated Stink Bug in North America and Europe: History, biology, ecology, and management. Annu Rev Entomol. (2018) 63:599–618. doi: 10.1146/annurev-ento-020117-043226
10. Venette RC, Hutchison WD. Invasive insect species: Grand challenges, strategies and opportunities. Front Insect Sci. (2021) 1:650520. doi: 10.3389/finsc.2021.650520
11. Burkness EC, Ebbenga DN, Toninato AG, Hutchison WD. Exclusion and repulsion of Popillia japonica (Coleoptera: Scarabaeidae) using various coverings on high tunnel structures for primocane raspberry. Insects. (2022) . 13:771. doi: 10.3390/insects13090771
12. Henden J, Guédot C. Effect of surrounding landscape on Popillia japonica abundance and their spatial pattern within Wisconsin vineyards. Front Insect Sci. (2022) 2:961437. doi: 10.3389/finsc.2022.961437
13. Lannan MC, Guédot C. Attract-and-kill for managing Popillia japonica (Coleoptera: Scarabaeidae) abundance and leaf injury in commercial vineyards. J Econ Entomol. (2024) 117:508–15. doi: 10.1093/jee/toae031
14. DiGiacomo G, Hadrich J, Hutchison WD, Peterson H, Rogers M. Economic impact of spotted wing drosophila (Diptera: Drosophilidae) yield loss on Minnesota raspberry farms: a grower survey. J Integr Pest Manage. (2019) 10:11. doi: 10.1093/jipm/pmz006
15. Moyer RA, Hummer KE, Finn CE, Frei B, Wrolstad RE. Anthocyanins, phenolics, and antioxidant capacity in diverse small fruits: Vaccinium, Rubus, and Ribes. J Agric Food Chem. (2002) 50:519–25. doi: 10.1021/jf011062r
16. Ladd TL Jr. Japanese beetle (Coleoptera: Scarabaeidae): Influence of favored food plants on feeding response. J Econ Entomol. (1987) 80:1014–7. doi: 10.1093/jee/80.5.1014
17. Pehluvan M, Güleryüz M. Research on the adaptation of some raspberry (Rubus ideaus L.) cultivars in Oltu (Erzurum) ecological conditions. Alinteri Zirai Bilimler Dergisi. (2010) 18:7–13.
18. Ebbenga DN, Hanson AA, Burkness EC, Hutchison WD. A degree-day model for forecasting adult phenology of Popillia japonica (Coleoptera: Scarabaeidae) in a temperate climate. Front Insect Sci. (2022) 2:1075807. doi: 10.3389/finsc.2022.1075807
19. Fleming WE. Biology of the Japanese Beetle. Technical Bulletin No. 1449. Washington, DC: USDA (1972).
20. Radcliffe EB, Hutchison WD, Cancelado R eds. Integrated Pest Management: Concepts, Tactics, Strategies and Case Studies. Cambridge UK: Cambridge Univ. Press. UK (2009). 450 pp.
21. Binns MR, Nyrop JP. Sampling insect populations for the purpose of IPM decision making. Annu Rev Entomol. (1992) 37:427–53. doi: 10.1146/annurev.en.37.010192.002235
22. Hutchison WD. Sequential sampling to determine population density. In: Pedigo L, Buntin D, editors. Handbook of sampling methods for arthropods in agriculture. CRC, Boca Raton, FL (1994). p. 207–44.
23. Green RH. On fixed precision sequential sampling. Res Popul. Ecol. (1970) 12:249–51. doi: 10.1007/BF02511568
24. Hutchison WD, Hogg DB, Poswall MA, Berberet RC, Cuperus GW. Implications of the stochastic nature of Kuno’s and Green’s fixed-precision stop-lines: Sampling plans for the pea aphid (Homoptera: Aphididae) in alfalfa as an example. J Econ Entomol. (1988) 81:749–58. doi: 10.1093/jee/81.3.749
25. Naranjo SE, Hutchison WD. Validation of arthropod sampling plans using a resampling approach: software and analysis. Am Entomol. (1997) 43:48–57. https://www.ars.usda.gov/research/software/download/?softwareid=129&modecode=20-20-05-05. (Accessed September 15, 2024).
26. Galvan TL, Burkness EC, Hutchison WD. Enumerative and binomial sequential sampling plans for the multicolored Asian lady beetle (Coleoptera: Coccinellidae) in wine grapes. J Econ Entomol. (2007) 100:1000–10. doi: 10.1093/jee/100.3.1000
27. Ribeiro AV, Aita RC, Pezzini DT, DiFonzo CD, Hunt TE, Knodel JJ, et al. Optimization of sample unit size for sampling stink bugs (Hemiptera: Pentatomidae) in soybean. Crop Prot. (2022) 157:105986. doi: 10.1016/j.cropro.2022.105986
28. Burkness EC, Hutchison WD. Development and validation of a binomial sequential sampling plan for striped cucumber beetle (Coleoptera: Chrysomelidae) in cucurbits. J Econ Entomol. (1997) 90:1590–4. doi: 10.1093/jee/90.6.1590
29. Hodgson EW, Burkness EC, Hutchison WD, Ragsdale DW. Enumerative and binomial sequential sampling plans for soybean aphid (Homoptera: Aphididae) in soybean. J Econ Entomol. (2004) 97:2127–36. doi: 10.1093/jee/97.6.2127
30. de Araújo TA, Pezzini DT, Ramos RS, Picanço MC, Bastos CS, Hunt TE, et al. Development and validation of sampling plans for Frankliniella schultzei on tomato. Crop Prot. (2020) 134:105163. doi: 10.1016/j.cropro.2020.105163
32. Beckerman J, Bessin R, Welty C, Athey K, Wahle E, Lewis D, et al. Midwest Fruit Management Guide. West Lafayette, IN: Purdue Extension, Purdue University (2021). Available at: https://ag.purdue.edu/hla/hort/pages/sfg_sprayguide.aspx.
33. R Studio Team. RStudio, Integrated Development for R. RStudio. Boston, MA: PBC (2020). Available at: http://www.rstudio.com/.
35. Pedigo LP, Rice ME, Krell RK. Entomology and Pest Management. 7th ed. Long Grove IL: Waveland Press Inc. (2021). 584 pp.
36. Burkness EC, Ebbenga DN, Hutchison WD. Evaluation of foliar insecticide control of adult Japanese beetle in raspberry 2019. Arthropod. Manage Tests. (2020) 45 (1), 1–1. doi: 10.1093/amt/tsaa009
37. Ng YS, Trout RJ, Ahmad S. Spatial distribution of the larval populations of the Japanese Beetle (Coleoptera: Scarabaeidae) in turfgrass. J Econ Entomol. (1983) 76:26–32. doi: 10.1093/jee/76.1.26
38. Ng YS, Trout RJ, Ahmad S. Sequential sampling plans for larval populations of the Japanese beetle (Coleoptera: Scarabaeidae) in turfgrass. J Econ Entomol. (1983) 76:251–3. doi: 10.1093/jee/76.2.251
39. Sara SA, McCallen EB, Switzer PV. The spatial distribution of the Japanese beetle, Popillia japonica, in soybean fields. J Insect Sci. (2013) 13:36. doi: 10.1673/031.013.3601
40. Loughrin JH, Potter DA, Hamilton-Kemp TR. Volatile compounds induced by herbivory act as aggregation kairomones for the Japanese beetle (Popillia japonica Newman). J Chem Ecol. (1995) 21:1457–67. doi: 10.1007/BF02035145
41. Loughrin JH, Potter DA, Hamilton-Kemp TR, Byers ME. Role of feeding–induced plant volatiles in aggregative behavior of the Japanese Beetle (Coleoptera: Scarabaeidae). Environ Entomol. (1996) 25:1188–91. doi: 10.1093/ee/25.5.1188
42. Ribeiro AV, Cira TM, MacRae IV, Koch RL. Effects of feeding injury from Popillia japonica (Coleoptera: Scarabaeidae) on soybean spectral reflectance and yield. Front Insect Sci. (2022) 2:1006092. doi: 10.3389/finsc.2022.1006092
43. Gould GE. Japanese beetle damage to soybeans and corn. J Econ Entomol. (1963) 56:776–81. doi: 10.1093/jee/56.6.776
44. Santoiemma G, Battisti A, Gusella G, Cortese G, Tosi L, Gilioli G, et al. Chemical control of Popillia japonica adults on high-value crops and landscape plants of northern Italy. Crop Prot. (2021) 150:105808. doi: 10.1016/j.cropro.2021.105808
45. Poggi S, Desneux N, Jactel H, Tayeh C and Verheggen F. A nationwide pest risk analysis in the context of the ongoing Japanese beetle invasion in Continental Europe: The case of metropolitan France. Front Insect Sci. (2022) 2:1079756. doi: 10.3389/finsc.2022.1079756
46. Gotta P, Ciampitti M, Cavagna B, Bosio G, Gilioli G, Alma A, et al. Roversi PF and Marianelli L. Popillia japonica – Italian outbreak management. Front Insect Sci. (2023) 3:1175138. doi: 10.3389/finsc.2023.1175138
47. Graf T, Scheibler F, Niklaus PA, Grabenweger G. From lab to field: biological control of the Japanese beetle with entomopathogenic fungi. Front Insect Sci. (2023) 3:1138427. doi: 10.3389/finsc.2023.1138427
48. Strangi A, Paoli F, Nardi F, Shimizu K, Kimoto T, Iovinella I, et al. Tracing the dispersal route of the invasive Japanese beetle. Popillia japonica. J Pest Sci. (2024) 97:613–29. doi: 10.1007/s10340-023-01653-1
49. Kistner-Thomas EJ. The potential global distribution and voltinism of the Japanese beetle (Coleoptera: Scarabaeidae) under current and future climates. J Insect Sci. (2019) 9:1–13. doi: 10.1093/jisesa/iez023
Keywords: IPM decision-making, Japanese beetle, Taylor’s Power Law, resampling, IPM, sequential sampling
Citation: Toninato AG, Burkness EC and Hutchison WD (2024) Spatial distribution and fixed-precision sequential sampling plans for Popillia japonica (Coleoptera: Scarabaeidae) adults in primocane raspberry: influence of foliar insecticides. Front. Insect Sci. 4:1465829. doi: 10.3389/finsc.2024.1465829
Received: 16 July 2024; Accepted: 16 September 2024;
Published: 02 October 2024.
Edited by:
Deguang Liu, Northwest A&F University, ChinaReviewed by:
Eustachio Tarasco, University of Bari Aldo Moro, ItalyDexian Li, Northwest A&F University, China
Copyright © 2024 Toninato, Burkness and Hutchison. This is an open-access article distributed under the terms of the Creative Commons Attribution License (CC BY). The use, distribution or reproduction in other forums is permitted, provided the original author(s) and the copyright owner(s) are credited and that the original publication in this journal is cited, in accordance with accepted academic practice. No use, distribution or reproduction is permitted which does not comply with these terms.
*Correspondence: William D. Hutchison, aHV0Y2gwMDJAdW1uLmVkdQ==
†Present address: Adam G. Toninato, Mitsui Chemicals America, Rye Brook, NY, United States
Eric C. Burkness, Minnesota Department of Agriculture, St. Paul, MN, United States