- Department of Biology, York University, Toronto, ON, Canada
The insect ion transport peptide (ITP) and its alternatively spliced variant, ITP-like peptide (ITP-L), belong to the crustacean hyperglycemic hormone family of peptides and are widely conserved among insect species. While limited, studies have characterized the ITP/ITP-L signaling system within insects, and putative functions including regulation of ion and fluid transport, ovarian maturation, and thirst/excretion have been proposed. Herein, we aimed to molecularly investigate Itp and Itp-l expression profiles in the mosquito, Aedes aegypti, examine peptide immunolocalization and distribution within the adult central nervous system, and elucidate physiological roles for these neuropeptides. Transcript expression profiles of both AedaeItp and AedaeItp-l revealed distinct enrichment patterns in adults, with AedaeItp expressed in the brain and AedaeItp-l expression predominantly within the abdominal ganglia. Immunohistochemical analysis within the central nervous system revealed expression of AedaeITP peptide in a number of cells in the brain and in the terminal ganglion. Comparatively, AedaeITP-L peptide was localized solely within the pre-terminal abdominal ganglia of the central nervous system. Interestingly, prolonged desiccation stress caused upregulation of AedaeItp and AedaeItp-l levels in adult mosquitoes, suggesting possible functional roles in water conservation and feeding-related activities. RNAi-mediated knockdown of AedaeItp caused an increase in urine excretion, while knockdown of both AedaeItp and AedaeItp-l reduced blood feeding and egg-laying in females as well as hindered egg viability, suggesting roles in reproductive physiology and behavior. Altogether, this study identifies AedaeITP and AedaeITP-L as key pleiotropic hormones, regulating various critical physiological processes in the disease vector, A. aegypti.
Introduction
Neuropeptides comprise a large and diverse class of signaling molecules that, together with their receptors, play a significant role in controlling a myriad of behavioral and physiological processes, including reproduction, feeding, development, energy homeostasis, water and ion balance, and more (1–4). The crustacean hyperglycemic hormone (CHH) family of peptides are a large neuropeptide superfamily that includes structurally-related peptides composed of 72 to more than 80 amino acids (5) containing three highly conserved intramolecular disulfide bonds (6). Functional roles for the CHH family of peptides are linked to molting, stress responses, reproduction, and homeostatic regulation of energy metabolism (7). The insect ion transport peptide (ITP) and its alternatively spliced variant, ITP-like or ITP-long (ITP-L) belong to the CHH family of peptides (8, 9) and are widely conserved among insect species, including lepidopterans, such as the silkworm Bombyx mori (9, 10), and the tobacco hornworm Manduca sexta (11). While investigations examining the roles of ITP and ITP-L are limited, studies have suggested functions of both peptides as regulators of ion and fluid transport across the ileum of the desert locust Schistocerca gregaria (12), ecdysis in M. sexta (11), ovarian maturation in the red flower beetle Tribolium castaneum (13), and thirst/excretion regulation and clock neuron modulation in the fruit fly Drosophila melanogaster (14, 15).
The insect ITP was originally identified in S. gregaria (9, 12, 16), where it drives chloride-dependent movement of fluid across the ileum, hence suggesting a role as an anti-diuretic hormone (17). Subsequently, Meredith et al. identified the complete amino acid sequence of SchgrITP, with a 72-residue mature peptide sequence and six cysteine residues proposed to participate in disulfide bridge formation (18). The mature SchgrITPL peptide is only four amino acids longer than ITP, containing a unique carboxy-terminus (19, 20). Studies have revealed that both peptides share a common N-terminal sequence, whereas the C-terminal sequences diverge significantly, thus were predicted to arise from alternative splicing (18). Due to the shared N-terminus between the two peptide precursors, earlier studies proposed that the N-terminus permits the peptides to bind to its receptor (19). ITP is a potent stimulator of ileal short circuit current, whereas ITP-L is devoid of such activity, suggesting an antagonistic role of ITP-L on the putative ITP receptors in the locust hindgut (21).
Differential tissue immunolocalization of ITP and ITP-L in M. sexta and B. mori revealed ITP expression in bilaterally-paired neurosecretory cells in the brain with projections to the retrocerebral complex, whereas ITP-L expression was seen in peripheral neurosecretory cells and neurons of the ventral ganglia (9). Further investigations confirmed ITP localization exclusively to the central nervous system, and ITP-L to the central nervous system and peripheral tissues (18, 22, 23), suggesting differential functional roles for the alternatively spliced peptides. In 2007, Dai et al. were the first to identify a conserved ITP gene (Itp) in the mosquito, Aedes aegypti, which by alternative splicing, encodes for AedaeITP-L; a longer peptide isoform with an unblocked C-terminus, and AedaeITP; a shorter peptide with an amidated C-terminus. To date, the expression pattern, tissue distribution, and putative physiological function of either ITP or ITP-L has not been determined in A. aegypti. Herein, this study set out to characterize the tissue-specific expression and localization, as well as determine functional roles of AedaeITP and AedaeITP-L in the A. aegypti mosquito. Using a combination of molecular and physiological techniques, AedaeITP and AedaeITP-L was characterized in the adult stage, with expression and localization of AedaeITP in the brain and the terminal ganglion while AedaeITP-L was detected in the pre-terminal abdominal ganglia of the ventral nerve cord. Furthermore, using RNA interference (RNAi), the current results provide strong evidence that AedaeITP and AedaeITP-L play essential roles in osmotic and ionic regulation, reproductive physiology and mating behavior in the Aedes mosquito. Overall, these findings advance our understanding of ITP and ITP-L neuropeptides in mosquitoes and provide novel research directions for elucidating neuropeptidergic signaling in the disease-vector, A. aegypti.
Materials and methods
Animals
Aedes aegypti eggs (Liverpool strain) were collected from an established laboratory colony as described previously (24, 25) and hatched in double-distilled water in an incubator at 26°C on a 12:12 hour light: dark cycle. Larvae were fed a solution of 2% (w/v) brewer’s yeast and 2% (w/v) Argentine beef liver powder (NOW foods, Bloomingdale, IL, USA). For colony upkeep, female mosquitoes were fed sheep’s blood in Alsever’s solution (Cedarlane Laboratories Ltd., Burlington, ON, Canada) using an artificial feeding system (24). Adults were provided with 10% sucrose solution ad libidum.
Tissue/organ dissections, RNA extraction, and cDNA synthesis
One- and four-day old adult female (n=30) and male (n=40) A. aegypti were briefly anaesthetized with CO2 and submerged in Dulbecco’s phosphate buffered saline (DPBS; Wisent Corporation, St. Bruno, QC, Canada), and the following body segments and tissues/organs were dissected and isolated: head, thorax, midgut, Malpighian tubules (MTs), hindgut, reproductive tissues (ovaries, testes, and accessory reproductive tissues) and carcass (remaining cuticle, musculature, fat body, and abdominal ganglia). For the central nervous system expression profile, the brain, thoracic ganglia, and abdominal ganglia were collected. Whole adult RNA was obtained by collecting fourth instar larvae (n=10), early- and late-stage pupae (n=10), and one- and four-day old adult female (n=10) and male (n=11) mosquitoes. For the starvation and desiccation assay, whole adult male (n=6-7) and females (n=5-6) were isolated 24 h or 48 h post treatment. To confirm knockdown efficiency following double-stranded RNA (dsRNA) treatment, whole adult male (n=5-6) and female (n=5) were isolated four-, six-, and eight-days post injection. Whole adult and organ samples were stored in 1x RNA protection buffer at -20°C until further processing. Samples were then thawed at room temperature and total RNA was isolated using the Monarch Total RNA Miniprep Kit following manufacturers protocol with an on-column DNase treatment to remove genomic DNA (New England Biolabs, Whitby, ON, Canada). Purified total RNA samples were subsequently aliquoted onto a Take3 micro-volume plate and quantified on a Synergy Multi-Mode Microplate Reader (BioTek, Winooski, VT, USA). To determine AedaeItp and AedaeItp-l transcript levels, cDNA was synthesized from 500 ng (developmental expression profile), 80 ng (spatial expression profile), and 250 ng (starvation assay and dsRNA injections) total RNA using the iScript™ Reverse Transcription Supermix for RT-qPCR (Bio-Rad, Mississauga, ON, Canada) following manufacturers protocol, including a ten-fold dilution of cDNA following synthesis.
RT- quantitative PCR
To measure expression profiles for AedaeItp and AedaeItp-l, transcript abundance was quantified on a StepOnePlus™ Real Time PCR system (Applied Biosystems, Carlsbad, CA, USA) using PowerUP™ SYBR® Green Master Mix (Applied Biosystems, Carlsbad, CA, USA). Cycling conditions were as follows: 1) uracil-DNA glycosylase (UDG) activation 50°C for 2 min, 2) 95°C for 2 min, and 3) 40 cycles of i) 95°C for 15 seconds and ii) 60°C for 1 minute. Gene-specific primers for AedaeItp and AedaeItp-l were designed over multiple exons (see Supplementary Table S1 for list of primers) based on a previously reported mRNA sequence (Genbank Accession Numbers: (Itp) AY950503 and (Itp-l) AY950506) (9). To ensure specificity for each individual peptide-specific transcript, reverse primers for AedaeItp (nucleotides 418-438) and AedaeItp-l (nucleotides 418-428) were designed over transcript-specific exon-exon boundaries that, in the case of AedaeItp-l, includes exon 3 since this exon is absent in AedaeItp (Supplementary Figure S1). Relative expression levels were determined using the CT method (26) and normalized to the geometric mean of rp49 and rps18 reference genes, which were previously determined as optimal endogenous controls (27). Developmental expression profiles consisted of an average of 5-6 biological replicates that each included triplicate technical replicates for each target gene. Spatial expression profiles, starvation assay and dsRNA knockdown experiments consisted of 3-4 biological replicates. Primer specificity for target mRNA was assessed by conducting no reverse-transcriptase and no-template controls along with performing standard curves to calculate primer efficiencies.
Immunohistochemistry
To examine AedaeITP and AedaeITP-L immunoreactivity in the central nervous system, whole one- and four-day old adult male and female A. aegypti were collected and incubated in freshly prepared 4% paraformaldehyde (PFA) fixative overnight at 4°C. The following day, tissues/organs dissections of the central nervous system consisting of the brain, thoracic ganglia, and the abdominal ganglia, were performed in DPBS. Tissues/organs were then permeabilized by incubating on a rocker for 1 h at RT in 4% Triton X-100 (Sigma Aldrich, Oakville, ON, Canada), 10% normal sheep serum (NSS) (v/v) and 2% BSA (w/v) prepared in DPBS, followed by three 15 min washes in DPBS. After the last wash, the DPBS was removed and substituted with a 1:1000 dilution of primary antiserum solution (0.4% Triton-X- 100, 2% NSS (v/v), and 2% BSA (w/v)) in DPBS (prepared the day before use and incubated at 4°C to reduce non-specific binding) on a rocker for 96 h at 4°C. The custom primary antiserum solution was raised in rabbit against a synthetic peptide (SSFFDIECKGQFNKA) antigen corresponding to a 15-amino acid region of AedaeITP and AedaeITP-L (nucleotides 154-198 on common exon 2, amino acids 1-15 of the shared N-terminal sequence, Supplementary Figure S1), thus targeting both AedaeITP and AedaeITP-L (Biomatik, Kitchener, ON, Canada). Following incubation, tissues/organs were washed with DPBS four times on a rocker over the course of an hour and subsequently incubated overnight at 4°C with a goat anti-rabbit Alexa Fluor® 568 IgG (H+L) secondary antibody (Molecular Probes, Life Technologies, Eugene, OR, USA) diluted 1:200 in 10% NSS made up in DPBS and protected from light. The following day, tissues/organs were washed three times with DPBS for 15 min each. As a negative control, the anti-AedaeITP/ITP-L primary antiserum was preincubated with 10 μM antigen (SSFFDIECKGQFNKA) overnight prior to use. Additionally, tissues/organs were also incubated with a no-primary control (0.4% Triton-X-100, 2% NSS (v/v), and 2% BSA (w/v) prepared in DPBS). Tissues/organs were mounted on cover slips with mounting media comprised of DPBS with 50% glycerol containing 4 μg/mL 4’6-diamidino-2-phenylindole dihydrochloride (DAPI) and were visualized on a Zeiss LSM 800 confocal laser microscope (Carl Zeiss, Jena, Germany) and processed with the Zeiss LSM Image Browser software or visualized on a Lumen Dynamics XCite™ 120Q Nikon fluorescence microscope (Nikon, Mississauga, ON, Canada).
Starvation and blood feeding assay
To examine the potential roles of AedaeITP and AedaeITP-L in mosquito feeding/starvation, adult males and females were isolated post-emergence and sorted into three treatment conditions: desiccated (no food or water provided), fed (10% sucrose ad-libitum), and starved (only water provided). The adults were collected after 24 h or 48 h, and mRNA transcript levels of AedaeItp and AedaeItp-l were examined by RT-qPCR (as described above). To investigate whether a protein-rich meal influences the transcript abundance of AedaeItp and AedaeItp-l, adult female mosquitoes (four-six day old) were given 20 min to blood feed on sheep’s blood in Alsever’s solution, and all blood fed females were subsequently isolated after 1, 6, 12 and 24 h post-bloodmeal. Blood fed females were compared to control, similarly aged (five-six day old) females that were provided sucrose ad libitum. Post isolation, mRNA transcript levels of AedaeItp and AedaeItp-l were examined by RT-qPCR (as described above).
Preparation and microinjection of AedaeItp and AedaeItp-l dsRNA
Gene-specific primers were designed to amplify a region of the AedaeItp and AedaeItp-l transcripts as a template for dsRNA synthesis (ensuring no overlap with RT-qPCR primers, Supplementary Table S1, Supplementary Figure S2). Similar to the gene-specific probes, dsAedaeItp primers were designed over common exons 1 and 2, that targets and knocks down both AedaeItp and AedaeItp-l mRNA, whereas AedaeItp-l primers were designed over the unique exon 3 targeting only AedaeItp-l transcript (Supplementary Figure S1). The AedaeItp and AedaeItp-l target regions were amplified and cloned into pGEM-T-Easy vector and subsequently subcloned into the L4440 vector, which possesses two T7 promoters, each flanking either side of the multiple cloning site. L4440 was a gift from Andrew Fire (Addgene plasmid#1654; http://n2t.net/addgene:1654; RRID : Addgene_1654). The AedaeItp and AedaeItp-l targets were screened with a M13 forward (5’-TGTAAAACGACGCCAGT-3’) and L4440 reverse primer (5’-AGCGAGTCAGTCAGTGAGCGAG-3’) and reamplified with a T7 primer serving as a forward and reverse primer. Double stranded RNA was synthesized by in vitro transcription using the HiScribe T7 High Yield RNA Synthesis Kit (New England Biolabs, Whitby, ON, Canada) following manufacturers recommendations. Following synthesis, the dsRNA was incubated at 75°C for 5 min for denaturation and left at RT for 15 min to allow rehybridization, followed by RNA purification using a Monarch RNA Cleanup Kit following the manufacturer’s protocol (New England Biolabs, Whitby, ON, Canada). One-day old male and female adult mosquitoes were briefly anesthetized using CO2 and injected in the thorax with 1 μg of AedaeItp, AedaeItp-l or control Egfp (enhanced green fluorescent protein) dsRNA dissolved in nuclease-free H2O in a total volume of 200nL using a Nanoject III Programmable Nanoliter Injector (Drummond Scientific, Broomall, PA, USA).
In vivo urine production assay
To determine if AedaeITP and/or AedaeITP-L influences urine output, adult female mosquitoes were injected with 500 nL of a HEPES buffered saline (HBS), consisting of 11.9 mM HEPES, 137 mM NaCl, and 2.7 mM KCl, titrated to a pH of 7.45 and filter sterilized before use. Based on an established protocol (28), four-day old females were injected and placed into a graduated, packed-cell volume tube (MidSci, St. Louis, MO, USA) for two hours at 28°C with three mosquitoes per tube and excretion volumes were measured. Specifically, following the incubation period, mosquitoes were removed from the tube, which was then centrifuged at 16,000xg for 30 s to allow for the excreted volume to be measured visually under a dissecting microscope, via the graduated column at the bottom of the tube. Treatment females were either four-days old dsEgfp, dsAedaeItp, dsAedaeItp-l females (injected at one-day old), or non-dsRNA injected four-days old female mosquitoes that served as controls. Mosquito images were captured using a Zeiss Stemi 508 microscope with an Axiocam 208 color camera (Carl Zeiss, Jena, Germany).
Mating and egg-laying assay
A. aegypti mosquitoes were separated at the pupal stage and individually placed into a 24-well plate to allow adults to emerge. One-day old non-mated male and female adults were then isolated and injected as follows: 1) dsAedaeItp or dsAedaeItp-l knockdown females mated with virgin males, 2) dsAedaeItp or dsAedaeItp-l knockdown males mated with virgin females, and 3) dsAedaeItp or dsAedaeItp-l knockdown females mated with dsAedaeItp or dsAedaeItp-l knockdown males. For 1), dsAedaeItp or dsAedaeItp-l females were grouped with one-day old virgin males at a 1:2 ratio of female: male per insect box (BugDorm-5 insect box, MegaView Science Co. Taiwan) between 18 and 24 h after dsRNA injection. After four-five days post-mating, knockdown females were provided a bloodmeal. For 2), dsAedaeItp or dsAedaeItp-l males were mated with two-three-day old virgin females, and females were provided a bloodmeal 48 h post-mating. Lastly, for 3), dsAedaeItp or dsAedaeItp-l males and females were mated, and females were provided a bloodmeal four-five days post injection. For all blood feeding assays, females were given 20 min to blood feed, and blood fed females were subsequently isolated and weighed individually before being placed in an inverted 25 cm2 cell culture flask (Corning) lined with filter paper containing 3 mL of distilled water (dH2O) from larvae rearing containers to promote egg laying. Laid eggs were collected after 4 days and were semi-desiccated for 72 h and counted. Females were then removed and weighed before spermathecae were dissected and viewed under a microscope to confirm insemination. Eggs were placed in 40 mL of dH2O with 1 mL larval food (1:1 ratio of 2% brewer’s yeast and 2% liver powder), and hatched larvae (if any) were counted after 48 h.
Sperm quantification
Sperm quantification in the paired seminal vesicles (male sperm storage organs) and testes of male A. aegypti, along with the spermathecae (sperm storage organs) of female A. aegypti 4 days post dsRNA injection was performed following previously published protocols (29, 30). The seminal vesicles and testes from male along with spermathecae from female mosquitoes (9-11 mosquitoes per dsRNA mating treatment from 3-4 mating replicates) were placed in a 96-well plate with 100 μL PBS, and gently torn open using ultrafine forceps to release spermatozoa. An additional 10 μL PBS was used to rinse the forceps and the PBS with spermatozoa was mixed thoroughly using a P100 pipette. Five 1 μL droplets of the PBS/spermatozoa mixture were spotted onto a microscope slide (previously treated with poly-L-lysine to promote sperm attachment), allowed to air dry completely, and subsequently fixed with 70% ethanol. Slides were mounted using mounting media comprised of DPBS with 50% glycerol containing 4 μg/mL DAPI, and the nuclei of spermatozoa within each 1 μL droplet was imaged under 4X magnification using an Olympus IX81 inverted microscope (Olympus Canada, Richmond Hill, ON, Canada). The nuclei of spermatozoa were counted in each 1 μL droplet, averaged across all five droplets for each animal, and multiplied by the dilution factor to determine total spermatozoa numbers within the seminal vesicle, testes, and spermatheca.
Statistical analyses
All graphs were created and statistical analyses performed using GraphPad Prism v9.1 (GraphPad Software, San Diego, CA, USA). Data was analyzed accordingly using an unpaired t-test or one-way or two-way ANOVA with the appropriate post-hoc test as indicated in each figure caption, with differences between treatments considered significant if p<0.05.
Results
Tissue/organ-specific expression profile of AedaeItp and AedaeItp-l transcripts
Post-embryonic stages and selected tissues/organs of mosquitoes were examined for AedaeItp and AedaeItp-l transcript expression and compared between males and females. Developmental expression profiling revealed enrichment of AedaeItp transcript abundance in adult stage mosquitoes (Figure 1A), with greatest and significant enrichment in one- and four-day old adult males compared to fourth instar larvae. In contrast, AedaeItp-l transcript was significantly enriched in late pupal and adult stage mosquitoes, including one-day old males and females (Figure 1B). Transcript abundance of AedaeItp was significantly higher compared to AedaeItp-l abundance in adult-stage mosquitoes, with over a three-fold higher abundance of AedaeItp transcript compared to AedaeItp-l (Figure 1C). Additionally, AedaeItp transcript abundance was exclusively and significantly enriched in the head (Figures 2A, C) and brain (Figures 2B, D) in both adult male and female mosquitoes. Comparatively, expression of AedaeItp-l transcript was significantly enriched in the carcass (Figures 2E, G) and abdominal ganglia (Figures 2F, H) in adult mosquitoes.
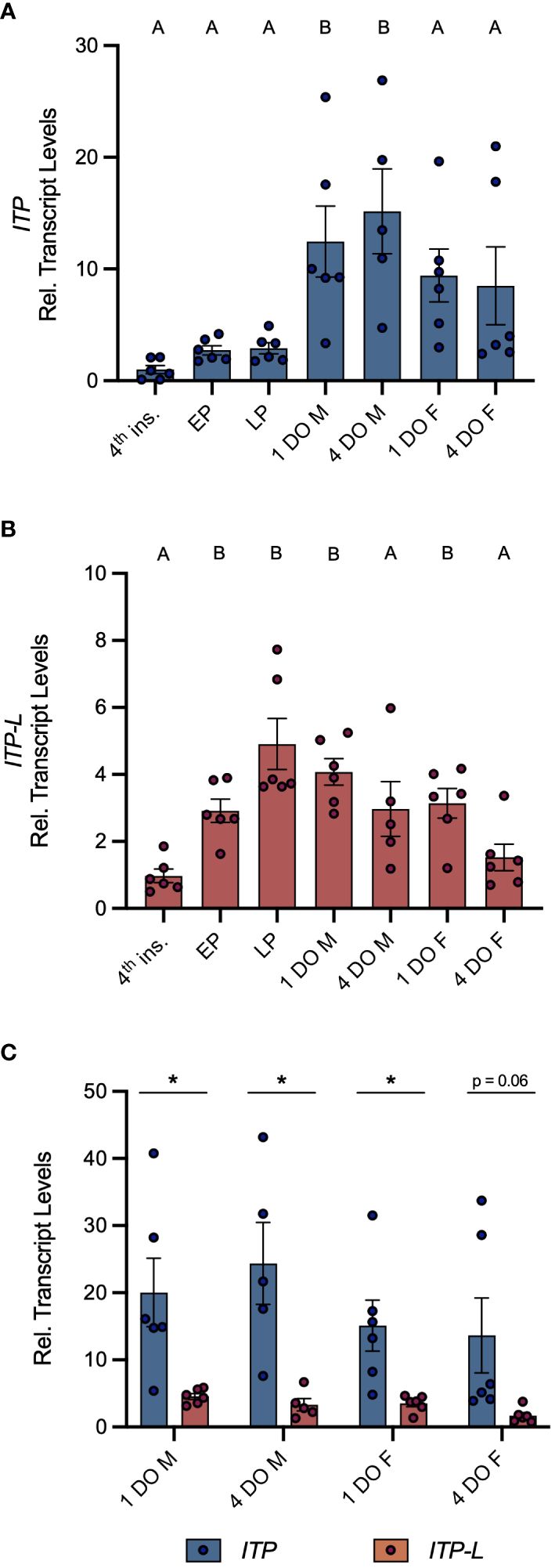
Figure 1 Developmental transcript expression profile of AedaeItp and AedaeItp-l in A. aegypti. Expression of AedaeItp (A) and AedaeItp-l (B) transcript was analyzed in post-embryonic stages of the mosquito shown relative to transcript levels in fourth instar larvae. Data labeled with different letters are significantly different from fourth instar larvae (mean ± SEM; one-way ANOVA with Dunnett’s multiple comparison, p<0.05, n=5–6 biological replicates). (C) Comparison expression profile of AedaeItp and AedaeItp-l transcript levels in one and four day old adult male and female mosquitoes. Significant differences between AedaeItp and AedaeItp-l transcript levels are denoted by an asterisk (*) (mean ± SEM; unpaired t-tests, p<0.05, n=5–6 biological replicates). 4th ins, 4th instar larvae; EP, early pupa; LP, late pupa; M, male; F, female; DO, day old.
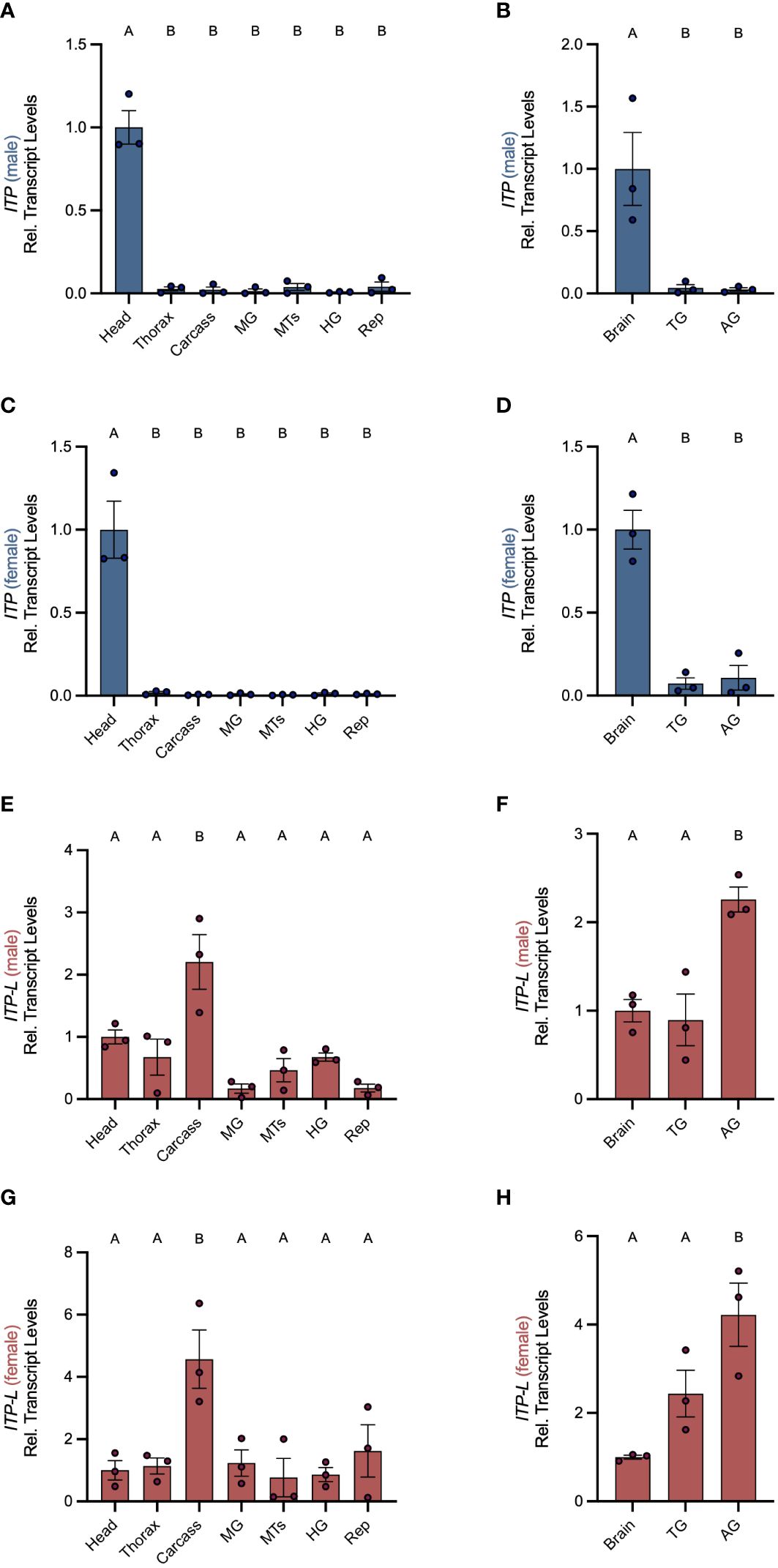
Figure 2 Spatial transcript expression profile of AedaeItp and AedaeItp-l in A. aegypti. Expression of AedaeItp (A–D) and AedaeItp-l (E–H) transcript levels were analyzed in various tissues/organs from one-day old adult males (A, B, E, F) and females (C, D, G, H) shown relative to transcript levels in the mosquito head/brain. MTs, Malpighian tubules; MG, midgut; HG, hindgut; Rep, reproductive tissues/organs; TG, thoracic ganglia; AG, abdominal ganglia. Bars labeled with different letters are significantly different from head/brain (mean ± SEM; one-way ANOVA with Dunnett’s multiple comparison, p<0.05, n=3 biological replicates).
AedaeITP- and AedaeITP-L-like immunoreactivity in the central nervous system
Using whole mount immunohistochemistry, the central nervous system from one-day old male and female mosquitoes revealed AedaeITP- and AedaeITP-L-like immunostaining in three pairs of lateral neurosecretory cells in the medial anterior region of each brain hemisphere with axonal processes projecting anteriorly near an additional single pair of lateral neurosecretory cells (Figures 3A–E). A number of varicosities and blebs can be seen peripherally near the axonal projections, suggestive of release sites of these neuropeptides (Figures 3A–E). In abdominal ganglia 2-6, AedaeITP- and AedaeITP-L-like staining was observed in a single pair of neurosecretory cells, positioned laterally on either side of each ganglia (Figures 3F, G). The terminal ganglion, which is a fusion of abdominal ganglia 7 and 8, revealed AedaeITP- and AedaeITP-L-like immunostaining in a single pair of neurosecretory cells located in the anterior region of the ganglion (corresponding to abdominal ganglia 7) (Figure 3H). Staining in these cells and projections were absent in control treatments where either the antiserum was preabsorbed with the ITP antigen or the omission of the primary antiserum (Supplementary Figure S1).
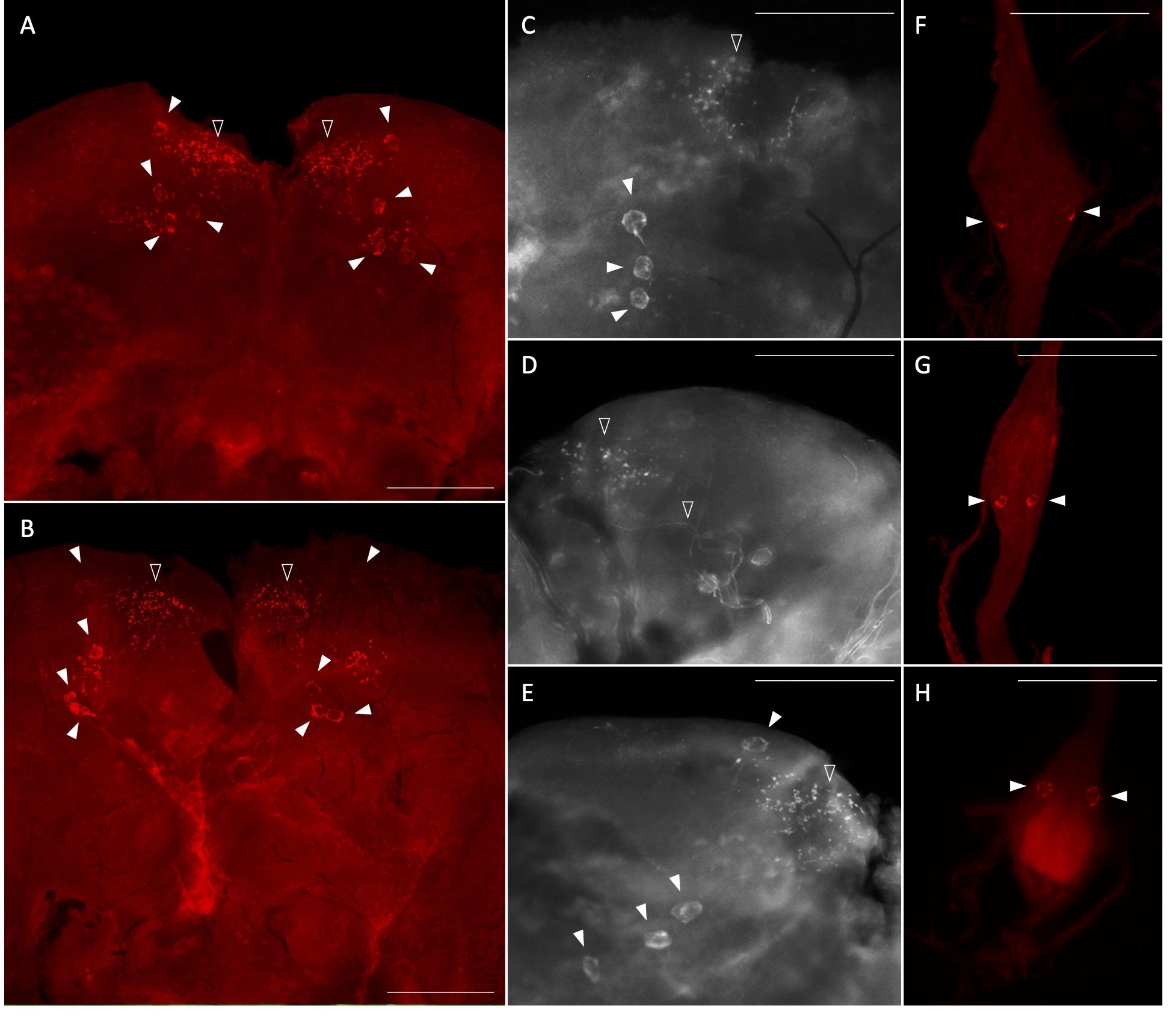
Figure 3 Immunolocalization of AedaeITP and AedaeITP-L in the central nervous system of the A. aegypti mosquito. AedaeITP- and AedaeITP-L-like immunoreactivity was examined in (A) male and (B) female brains, in four pairs of neurosecretory cells (indicated by white arrowheads), with axonal processes projecting anteriorly (C–E), towards varicosities and blebs on the periphery of the brain (indicated by empty arrowheads). (F, G) Ventral view of abdominal ganglia showing a single pair of lateral neurosecretory cells and (H) an anterior pair observed in the terminal ganglion. Scale bars: (A, B) 200 μM; (C–H) 100 μM.
Roles of AedaeITP and AedaeITP-L in desiccation and starvation stress and blood feeding
Post emergence, one-day old adult male and female mosquitoes were placed in either a fed (provided a 10% sucrose meal), desiccated (no food or water provided), or starved (provided a water meal, no sucrose) condition and isolated after 24 h or 48 h to examine a potential role of AedaeITP and AedaeITP-L in desiccation and starvation stress (Figure 4A). When adult male and female mosquitoes were placed in the starved or desiccated treatment for 24 h, there was no difference in mRNA abundance of either AedaeItp or AedaeItp-l compared to control fed conditions (Figures 4B, D, F, H). However, when the mosquitoes were subjected to these treatments for 48 h, there was a significant enrichment of both AedaeItp and AedaeItp-l transcript levels in the desiccated condition (ranging between ~1.75 and ~3.5-fold) compared to control fed animals (Figures 4C, E, G, I) while no change to the transcript levels in animals that were starved but provided with water. Next, to determine if AedaeITP and/or AedaeITP-L may play a role in relation to blood feeding, four- to six-day old adult female mosquitoes were provided a bloodmeal to examine whether a protein-rich meal influences the transcript abundance of AedaeItp and AedaeItp-l (Figure 5A). AedaeItp mRNA abundance did not change significantly compared to control, sucrose-fed females over any of the measured timepoints between 1 and 24 h post-blood feeding (Figure 5B) although abundance trended lower at the 1, 6 and 12 hr post-blood feeding timepoints. Similarly, AedaeItp-l mRNA abundance did not change significantly at any of the post blood-feeding timepoints in comparison to control, sucrose-fed females (Figure 5C).
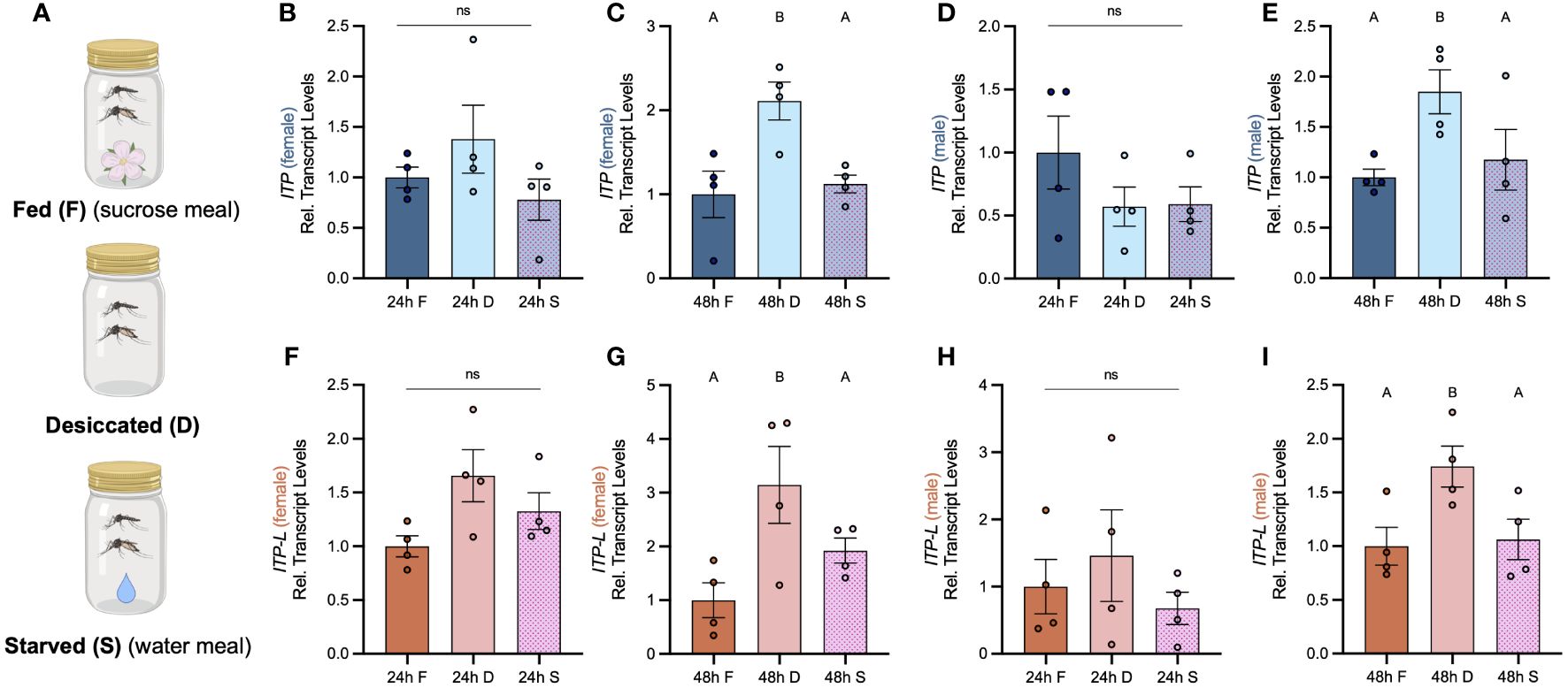
Figure 4 Effect of desiccation and starvation stress on transcript levels of AedaeItp and AedaeItp-l in whole body samples of adult A. aegypti. (A) Post-emergence, adult males were placed in a fed (sucrose meal provided), desiccated (no food or water provided), and starved (only water provided) condition for 24 h and 48 h, and abundance of (B–E) AedaeItp and (F–I) AedaeItp-l transcript were analyzed, shown relative to transcript levels in the control F, fed; D, desiccated; S, starved. Bars labeled with different letters are significantly different from the 24 h fed adult controls (mean ± SEM; one-way ANOVA with Bonferroni multiple comparison, p<0.05, n=4 biological replicates) and ns denotes no statistical significance.
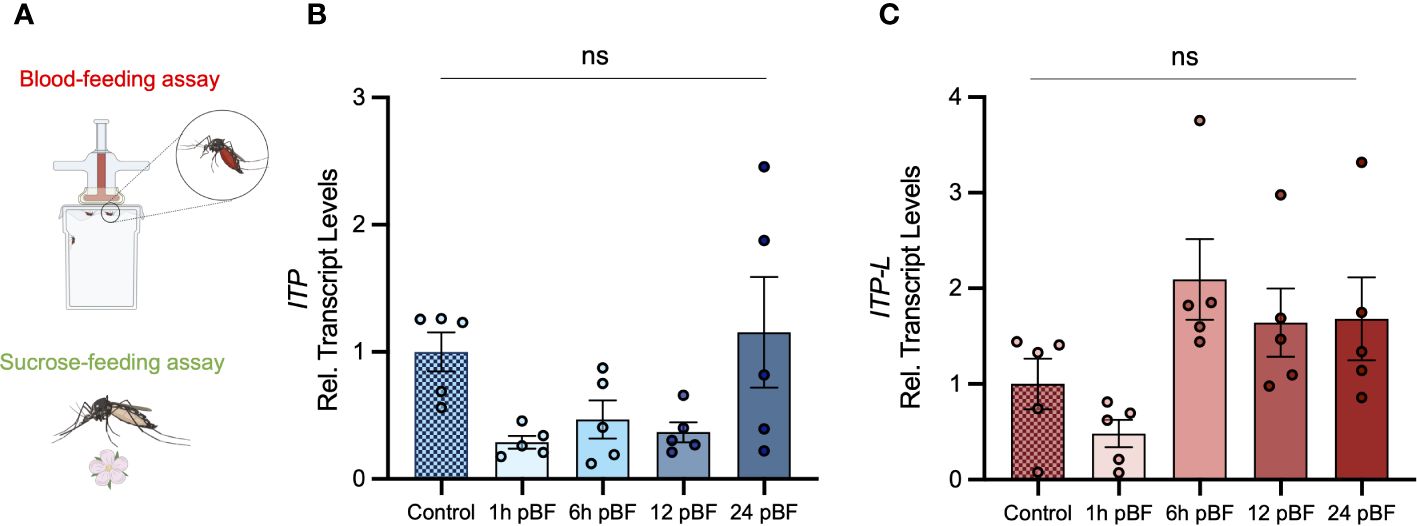
Figure 5 Effect of blood feeding on transcript levels of AedaeItp and AedaeItp-l in adult A aegypti. (A) Four- to six-day old adult females were blood fed and isolated at 1, 6, 12, and 24 post-blood feeding and (B) AedaeItp and (C) AedaeItp-l transcript levels were analyzed, shown relative to control, sucrose-fed females. pBF (post-blood feeding). No significance (ns) reflects comparisons with control, sucrose-fed females (mean ± SEM; one-way ANOVA with Tukey’s multiple comparison, p<0.05, n=5 biological replicates).
dsRNA knockdown of AedaeItp and AedaeItp-l in adult Aedes mosquitoes
RNA interference of AedaeItp and AedaeItp-l expression was accomplished through dsRNA-injections of one-day old adult male and female mosquitoes (Supplementary Figure S3A). Given the conserved exons 1, 2, and 4 between AedaeItp and AedaeItp-l, the dsAedaeItp primers were designed over a common exon 2 resulting in the knockdown of both AedaeItp and AedaeItp-l transcripts. However, dsAedaeItp-l primers were designed over the unique exon 3 allowing knockdown of only the AedaeItp-l transcript. Relative to dsEgfp-injected control mosquitoes, AedaeItp and AedaeItp-l transcripts were significantly reduced by ~75% in four-day old male (Supplementary Figure S3B) and female (Supplementary Figure S3C) mosquitoes injected with dsAedaeItp. Comparatively, dsAedaeItp-l treatment resulted in a significant decrease in AedaeItp-l transcript abundance by ~80% in males (Supplementary Figure S3B) and ~60% in females (Supplementary Figure S3C) in four-day old adults, whereas AedaeItp transcript abundance was unaffected. To confirm injection alone does not influence AedaeItp and AedaeItp-l transcript levels, four-day post-dsEgfp injected animals were compared to four-day old non-injected mosquitoes (Supplementary Figure S3D), with no significant changes in AedaeItp and AedaeItp-l transcript abundance in dsEgfp-injected males and females compared to non-injected mosquitoes. AedaeItp and AedaeItp-l transcript restored to normal levels within six and eight days post-injection in males and eight days post-injection in females (Supplementary Figures S3E–H).
dsRNA knockdown confirms AedaeITP and AedaeITP-L immunolocalization
To further confirm dsAedaeItp- and AedaeItp-l knockdown and differentiate between AedaeITP and AedaeITP-L immunolocalization, staining in the CNS was examined in mosquitoes four-days post-dsRNA injection. Wholemounts of dsAedaeItp-injected male and female mosquitoes showed no AedaeITP- and AedaeITP-L-like immunostaining in the brain (Supplementary Figures S4A, B), abdominal ganglia (Supplementary Figure S4C), and in the terminal ganglion (Supplementary Figure S4D). In contrast, dsAedaeItp-l-injected mosquitoes did not exhibit changes to immunostaining in the brain (Supplementary Figures S4E, F), with similar staining as described above (Figures 3A–E), and in control dsEgfp-injected mosquitoes (Supplementary Figures S5A, B). However, as expected, knockdown of AedaeItp-l resulted in abolished staining of neurosecretory cells in the pre-terminal abdominal ganglia (Supplementary Figure S4G), and interestingly, no change to the immunostaining in the terminal ganglion (Supplementary Figure S4H), with a single pair of immunoreactive cells as described above (Figure 3H) and also observed in control dsEgfp-injected mosquitoes (Supplementary Figures S5C, D).
AedaeItp knockdown influences urine output in adult females
To determine if AedaeITP and/or AedaeITP-L influences ionoregulation and urine excretion, we volume loaded dsAedaeItp and dsAedaeItp-l injected females with saline and measured their urine output over two hours post volume loading. Four-day old HBS-injected females excreted a volume of 429.5 ± 53.49 nL of urine, which was significantly higher (~4-fold) compared to control four-day old non-HBS injected females (106.8 ± 15.39 nL) (Figure 6A). Notably, dsEgfp treated females excreted a similar volume of urine (391.6 ± 61.03 nL) compared to HBS-injected females. In contrast, dsAedaeItp mosquitoes injected with HBS secreted a significantly higher amount of urine, 972.7 ± 70.18 nL, approximately 2.5-fold higher compared to dsEgfp-injected females. Interestingly, no significant change in urine output was observed in dsAedaeItp-l females injected with HBS (604.2 ± 78.87 nL), compared to HBS loaded control (non-dsRNA injected) and dsEgfp-injected females. The overall effect of dsAedaeItp and dsAedaeItp-l injections on urine output was studied by examining abdomen distension immediately after and two hours post-HBS injection. Two hours post-volume loading, a less distended abdomen was observed in dsAedaeItp-injected females (Figures 6H, I) compared to control dsEgfp- and HBS-injected females (Figures 6B–G). Comparatively, a moderately distended abdomen was observed in dsAedaeItp-l-injected females (Figures 6J, K) two hours post saline-injection.
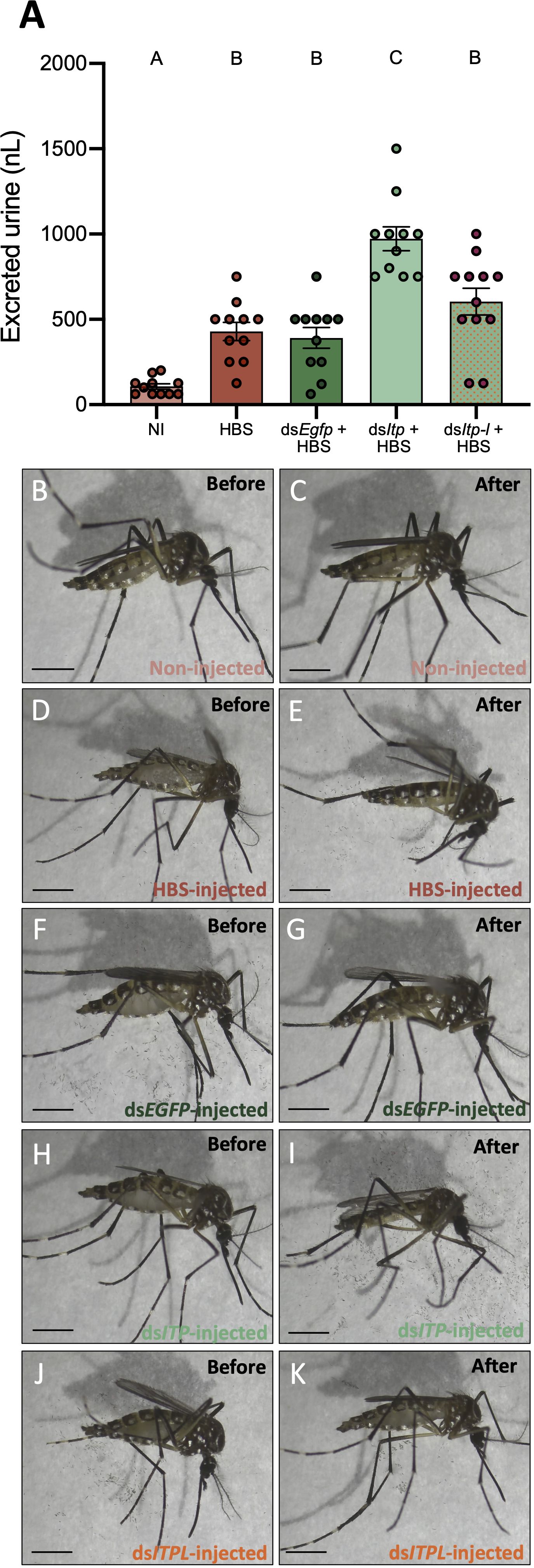
Figure 6 Effect of AedaeItp and AedaeItp-l knockdown on urine excretion in adult female A. aegypti. (A) Four-day old females were injected with 500 nL of a HEPES buffered saline (HBS) and allowed to excrete for two hours. Bars labeled with different letters are significantly different from each other including non-injected (NI) animals (mean ± SEM; one-way ANOVA with Bonferroni multiple comparison, p<0.05, n=11–12). (B–K) Images of female mosquitoes immediately following and two hours post-HBS injection. Scale bars: 1 mm.
AedaeItp and AedaeItp-l knockdown influences male and female reproductive success
To assess the roles of AedaeITP and AedaeITP-L in reproductive behavior and physiology, dsRNA-injected virgin mosquitoes were placed into one of the following mating combinations: 1a) dsAedaeItp or 1b) dsAedaeItp-l females mated with control, non-dsRNA males, 2a) dsAedaeItp or 2b) dsAedaeItp-l males mated with control, non-dsRNA females, and 3a) dsAedaeItp or 3b) dsAedaeItp-l females mated with dsAedaeItp and dsAedaeItp-l males. When dsAedaeItp females were mated with normal males, there was a significant reduction in the incidence of blood feeding (Figure 7A), reduced bloodmeal engorged by the female (Figure 7B), reduction in the number of eggs laid (Figure 7C), and an overall reduction in the percentage of larvae hatching per female (Figure 7D) was observed. More specifically, there was a ~90% reduction in preference for blood feeding by dsAedaeItp females and ~75% reduction in dsAedaeItp-l females (Figure 7A) with both treatments leading to a reduction in the bloodmeal volume imbibed (Figure 7B). Females treated with dsAedaeItp-l oviposited a similar number of eggs and had a comparable percentage of hatched larvae as control dsEgfp-females while dsAedaeItp caused a drastic reduction (~50%) in eggs oviposited by females and a more dramatic impact on larval hatching success (Figures 7C, D). In males injected with either dsAedaeItp or dsAedaeItp-l and mated with normal females, this did not influence female preference for blood feeding or the volume of blood imbibed (Figures 7E, F). However, normal females mated with dsAedaeItp and dsAedaeItp-l injected males had significantly reduced number of eggs oviposited (Figure 7G), as well as a reduced percentage of viable eggs and larvae hatching per female (Figure 7H). Interestingly, dsAedaeItp and dsAedaeItp-l females mated with dsAedaeItp and dsAedaeItp-l males almost completely abolished the preference for blood feeding (Figure 7I) with the few that did blood feed imbibing a significantly lower blood volume (Figure 7J), with almost no eggs laid by the female (Figure 7K), and complete absence of larval hatching (Figure 7L). Notably, the reduced preference for blood feeding limited the number of blood fed females used for subsequent studies. No major changes were observed in the weight of blood fed females post-egg laying (4 days post-feeding) in any of the treatment regimens (Figures 7B, F, J), indicating long term volume balance is not impacted by knockdown of AedaeItp or AedaeItp-l although there was a small (but significant) increase in the weight of AedaeItp-l injected females (Figure 7B). Mated males and females injected with control dsEgfp had similar preference for blood feeding, weight of blood fed females, produced similar number of eggs and comparable larval hatching as non-injected females (Supplementary Figure S6).
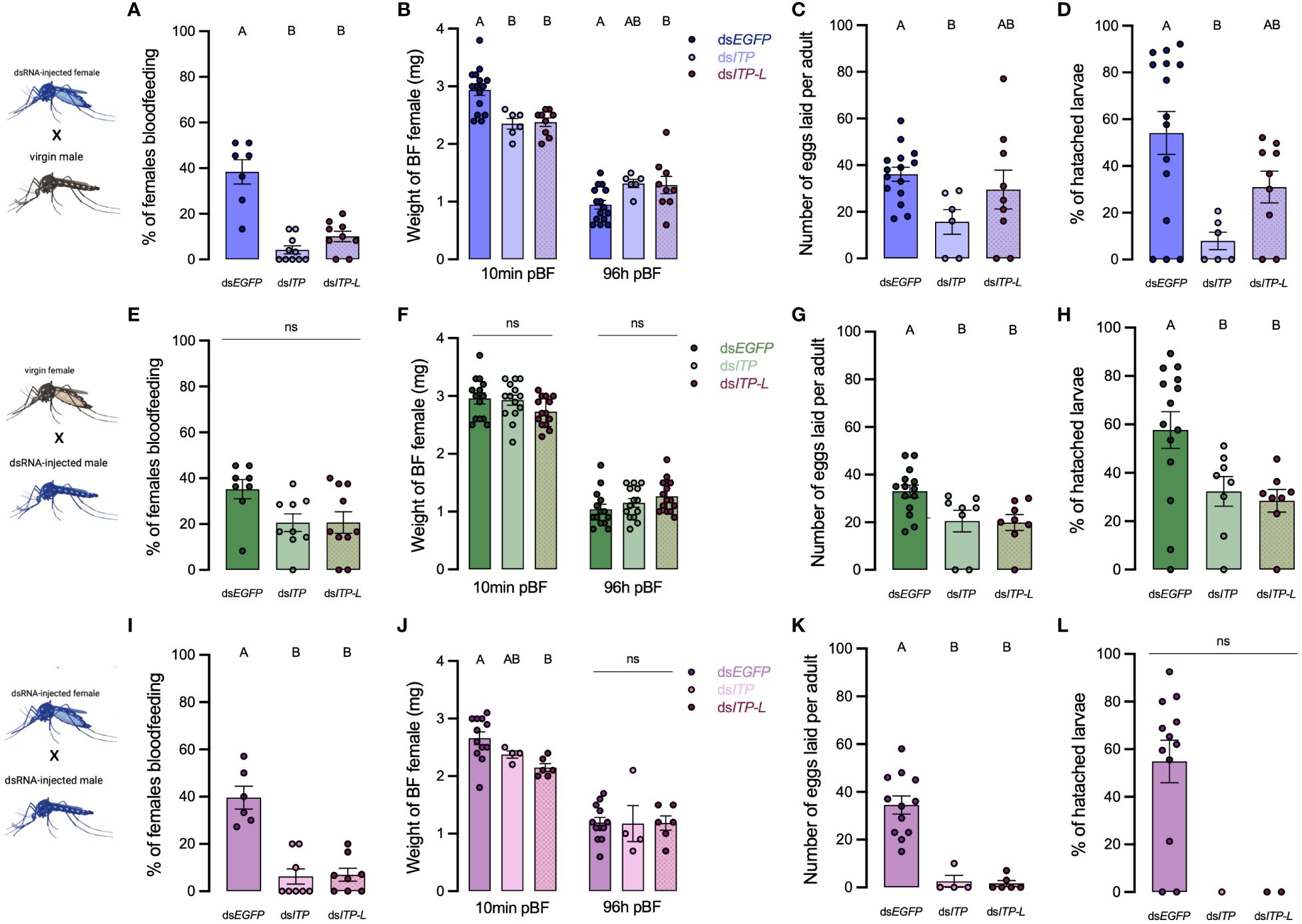
Figure 7 Effect of AedaeItp and AedaeItp-l knockdown on blood-feeding, egg laying, and larval hatching (egg viability) in adult A. aegypti. (A–D) dsAedaeItp or dsAedaeItp-l females mated with normal males, (E–H) dsAedaeItp or dsAedaeItp-l males mated with normal females, and (I–L) dsAedaeItp or dsAedaeItp-l females mated with dsAedaeItp or dsAedaeItp-l males. The effect of dsAedaeItp or dsAedaeItp-l knockdown was tested on (A, E, I) preference for blood feeding, (B, F, J) weight of blood fed female before and after egg collection, (C, G, K) number of eggs oviposited, and (D, H, L) percentage of larval hatching. Bars labeled with different letters are significantly different from control, dsEgfp injected age/time-matched adults (mean ± SEM; one-way ANOVA with Bonferroni multiple comparison, p<0.05, [(A, E, I), n=6–11 mating replicates, each point represents individual replicate values] [(B–D, F–H, J, K), n=1–15, each point represents data from individual females], (ns denotes no statistical significance).
AedaeItp and AedaeItp-l knockdown reduces spermatozoa count
In light of the results above, it supports the notion that knockdown of AedaeItp and AedaeItp-l may have a distinct role in male reproductive biology separate from their effects on females since pairings between normal females and knockdown males revealed females had normal preference for blood feeding as well as bloodmeal weight; however, oviposition rates by females and larval hatching rates were significantly reduced. Considering the overall reduced preference for blood feeding in dsAedaeItp and dsAedaeItp-l females, we speculated that AedaeITP and AedaeITP-L may play an essential role in spermatozoa production and release. Consequently, sperm was collected separately from the testes and seminal vesicles of four-day old dsAedaeItp- and dsAedaeItp-l-injected males (from all three mating conditions described above) and spermathecae of four-day old dsAedaeItp- and AedaeItp-l-injected females (again, from all three mating conditions noted above), and the quantity of mature spermatozoa was compared to dsEgfp-injected animals (Figures 8A, B). For spermatozoa collected from the seminal vesicles, dsAedaeItp- and dsAedaeItp-l-injected females mated with normal males produced similar mature spermatozoa counts compared to control dsEgfp animals (Figure 8C, Supplementary Figures S7A, B, E). Interestingly, knockdown resulted in significant reductions in the number of spermatozoa in the seminal vesicle, from both dsAedaeItp- and dsAedaeItp-l-injected males mated with control females and when mated with dsAedaeItp- and dsAedaeItp-l injected females (Figure 8C, Supplementary Figures S7C, D, F, G). Similar trends were observed for the number of spermatozoa collected directly from the male testes (Figure 8C, Supplementary Figures S7H–N). Comparatively, AedaeItp- and AedaeItp-l knockdown resulted in an ~85% reduction in mature spermatozoa counts in the spermatheca in all three mating conditions (Figure 8C, Supplementary Figures S7O–U). Animals injected with dsEgfp resulted in similar number of spermatozoa in the testes, seminal vesicles, and spermathecae compared to non-injected animals (Supplementary Figure S8).
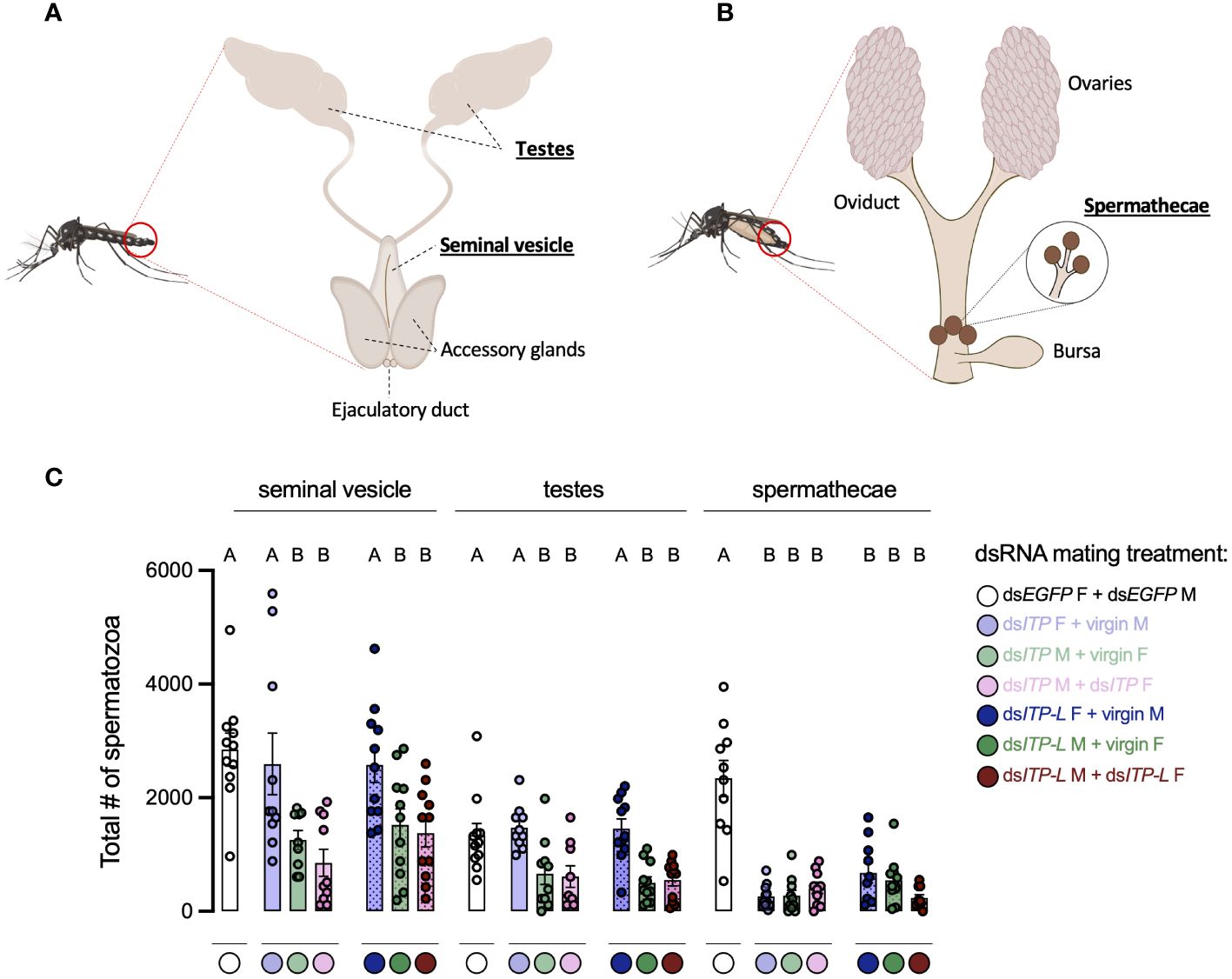
Figure 8 Total number of spermatozoa in the male testes and seminal vesicle along with female spermathecae of adult A. aegypti following RNAi (dsRNA)-mediated knockdown of AedaeItp or AedaeItp-l. Schematic diagram showing the (A) male and (B) female reproductive system of the adult mosquito. (C) Total spermatozoa number within the male testes and seminal vesicle, and female spermathecae of adults four-days after dsAedaeItp or dsAedaeItp-l injection. M, male; F, female. Bars labeled with different letters are significantly different from the organ-specific (seminal vesicle, testes and spermathecae) number of spermatozoa in control, dsEgfp injected adults (mean ± SEM; one-way ANOVA with Bonferroni multiple comparison, p<0.05, n=9–11, each point represents individual replicate values).
Discussion
ITP and ITP-L belong to the CHH family of neuropeptides and have been functionally characterized in many insect species (6, 7, 11, 13, 15, 22, 23, 31). However, while several studies have examined ITP signaling pathways in insects, ITP/ITP-L receptors have generally not been identified and characterized thus far, except for in the domestic silk moth B. mori (6). In a recent study using Drosophila melanogaster as a conserved tumor model, isoform F of ion transport peptide (ITPF) was found to be secreted by gut tumor cells and acts as an antidiuretic hormone targeting the tachykinin receptor (TkR99D) in Malpighian tubules leading to compromised renal function that results in the accumulation of excess fluid (32). This latest report is an intriguing considering tachykinins have been reported as diuretic factors in a number of insects (see 33) including recently in D. melanogaster (34).
In the present study, the A. aegypti ITP and ITP-L peptides have been localized, confirming the distribution of these peptides in neurosecretory cells and processes within the mosquito central nervous system. Additionally, prospective physiological functions have been investigated for AedaeITP and AedaeITP-L including roles in feeding, urine output, and reproductive success of adult male and female mosquitoes. This is the first report that examines the distribution, localization, and physiological function of the ITP/ITP-L signaling system in A. aegypti mosquitoes.
Distribution pattern of AedaeITP and AedaeITP-L in the CNS
Expression profiles of transcripts encoding A. aegypti ITP and ITP-L were measured to reveal potential functional or sex-specific roles for these peptides. Examination of the developmental and tissue-specific expression profile revealed enrichment of AedaeItp and AedaeItp-l in both one- and four-day old male and females, with significant enrichment of AedaeItp in the brain and AedaeItp-l in the abdominal ganglia. The expression and distribution of ITP and ITP-L in the central and peripheral nervous system has been examined in numerous insects (9, 18, 22, 23). While Itp mRNA expression has been detected only in the nervous system, evidence has suggested Itp-l expression in peripheral tissues as well. In T. castaneum, Itp-l transcript expression was found to be highly expressed in the midgut (13), and in the Malpighian tubules and hindgut in S. gregaria (18).
Previous studies in M. sexta revealed that MasITP and MasITPL are differentially expressed in mainly nonoverlapping populations of central and peripheral neurons, which includes neuronal projections from the CNS (9). RT-PCR, immunohistochemistry, and in situ hybridization studies indicated expression of MasITP exclusively in the brain where it was localized to two neuron types; in type Ia2 neurosecretory cells, with axonal projections to the retrocerebral complex (35–38) and in small neurons adjacent to type Ia2 cells, established as interneurons since their projections remain within the protocerebrum (9). Thus, in M. sexta, it was suggested that ITP is released as a neurohormone from type Ia2 cells into the hemolymph, whereas ITP produced in the small interneurons may serve transmitter or modulatory functions in the brain (9). Similarly in S. gregaria, ITP is believed to be synthesized in neurosecretory cells of the pars intercerebralis of the brain where it is then transported for storage and eventual release in the corpora cardiaca (18). The existence of ITP-L transcripts was first reported in S. gregaria (39), while the mature peptide was identified by Dai et al. (9) demonstrating ITP-L transcript and peptide distribution in the central and peripheral nervous system of insects, including B. mori, M. sexta, and the grasshopper, Schistocerca americana. Relatively weak ITP-L immunoreactivity was observed in the brain type Ia2 cells but was found to be completely absent in axons and terminals within the retrocerebral complex (9). ITP-L peptides are abundant in the ventral ganglia, flight muscle, MTs, and ileal tissues, indicating a possible distinctive function from ITP (17, 18).
Through RNAi-mediated knockdown, we confirmed AedaeITP immunoreactivity in at least four pairs of neurosecretory cells in the anterior region of the protocerebrum and in a single pair of lateral neurosecretory cells in the terminal ganglion. In contrast, AedaeITP-L immunoreactivity was observed in one pair of lateral neurosecretory cells in each abdominal ganglia of the ventral nerve cord. In general, expression patterns of AedaeItp and AedaeItp-l were similar to those described in M. sexta, T. castaneum, B. mori, and D. melanogaster (9, 13, 20, 22). In T. castaneum, Itp expression was in five pairs of brain cells on the dorsal side of the protocerebral hemispheres and in a pair of cells in the abdominal terminal ganglion (13). Similarly, ITP expression was observed in four pairs of brain cells in D. melanogaster. While still inconclusive, previous immunohistochemical studies in other insects allude to protocerebral cells with projections to the corpora cardiaca and allata, and the cells in the terminal abdominal ganglion may have projections to the hindgut (22) and possibly to reproductive organs (13), which provide insight on novel functions for the ITP/ITP-L signaling system. Given the immunoreactivity of AedaeITP in the terminal ganglion, this suggests potential iono-regulatory roles in the hindgut, acting possibly as an anti-diuretic hormone to increase water or ion reabsorption, similar to activity seen in the desert locust (12, 16).
Roles of ITP and ITP-L in feeding and urine excretion
The challenges of osmotic and ionic regulation vary between distinct environmental conditions that the adult mosquito might encounter. In desiccating environments, insects must safeguard water balance and reduce the rate of water loss (40). The current findings reveal an increase in both AedaeItp and AedaeItp-l transcript levels after 48 hours of combined desiccation and starvation stress in both adult male and female mosquitoes, which was not observed in animals undergoing starvation stress alone. These findings corroborate data reported in Drosophila, where ITP was linked as a natural component of desiccation and osmotic stress responses, since both stressors triggered an increase in Itp expression, while ITP knockdown reduced survival under desiccation and osmotic stress (15). DrosoITP plays roles in hunger, thirst, and excretion in Drosophila suggesting that ITP-regulated changes to physiology and behavior represent critical insect responses to cope with reduction in body water (15).
The discovery of the first anti-diuretic hormone mediating its effects on the insect hindgut was described by Audsley et al. (12) when ITP was purified from the corpora cardiaca of the locust, S. gregaria. A conserved Itp gene was later uncovered in the genome of the mosquito, A. aegypti, raising the prospect for a similar role in maintaining iono- and osmo-regulation (9). A. aegypti mosquitoes are reliant on an efficient excretory system comprised of the MTs and hindgut (1), functioning to counter disturbances to their hemolymph. The MTs, which are functional analogs of vertebrate kidneys, are responsible for the formation of primary urine (1), driven by the V-type H+-ATPase (41) that permits transport of Na+ and K+ cations across the membrane (42) via a putative H+/cation exchanger (43). The MTs are regulated by various diuretic and anti-diuretic hormones, which in A. aegypti includes the biogenic amine 5-hydroxytryptamine (5HT) (44, 45), DH31 (46), DH44 (47, 48), kinin-like peptides (49, 50) and CAPA that inhibits the activity of select diuretic hormones (25, 51, 52). The primary urine then enters the hindgut, where it is further modified through secretory and reabsorptive processes. Here, we show that Itp knockdown (but not Itp-l knockdown) leads to increased excretion of urine, supporting a possible anti-diuretic role for AedaeITP. Thus, it suggests that AedaeITP promotes water reabsorption in the hindgut similar to mechanisms described in S. gregaria where SchgrITP was found to stimulate chloride-dependent water reabsorption in the ileum, promoting an increase in Na+, K+ and Cl- transport (12, 16). Interestingly, while SchgrITP promotes a reabsorptive role on ileal tissue, ITP-L did not display any stimulatory effect, instead inhibiting the stimulatory effect of synthetic ITP (21). A key step to understanding the ITP and ITP-L actions in the Aedes mosquito is to identify the as yet unknown A. aegypti ITP receptor. The first presumed receptors for ITP and ITPL were characterized in the silkworm Bombyx mori (6). Specifically, Nagai et al. (6) identified three B. mori orphan GPCRs as receptors for ITP and ITP-L, that all responded to recombinant ITP, with elevating levels of intracellular cGMP upon receptor binding (6), which support the suggested ITP’s mode of action on ileal ion transport involving this second messenger in S. gregaria (53). In the locust, SchgrITP is proposed to bind to two different receptors, a G-protein coupled receptor and a membrane bound guanylate cyclase, on the ileal basolateral membrane, increasing both cyclic GMP (cGMP) and cyclic AMP (cAMP) levels, to regulate ion and fluid transport (53). cGMP stimulates Cl- reabsorption and H+ secretion across the ileum, whereas cAMP stimulates Na+, K+, and Cl- reabsorption (53). To further understand how these second messengers facilitate the physiological actions of SchgrITP on the ileum will require the endogenous ITP receptor(s) to be characterized.
Role of ITP and ITP-L in reproductive behavior and success
Female A. aegypti are day-biting mosquitoes, taking a single or multiple bloodmeals to obtain vitamins, proteins, minerals and other nutrients for egg development (42). Transcript levels of AedaeItp-l and AedaeItp levels remained unchanged over the time points we examined post blood feeding. Nonetheless, to elucidate whether ITP and ITP-L signaling might be involved in mosquito reproductive biology, RNAi was utilized to knockdown expression of Itp and Itp-l in adult A. aegypti. Overall, AedaeItp and AedaeItp-l knockdown female mosquitoes had a lower preference for blood feeding, laid fewer eggs, and had significantly reduced larval hatching. In T. castaneum, ITP and ITP-L are required throughout all life stages and are essential for reproduction and offspring survival (13). Knockdown of both ITP and ITP-L resulted in dramatic decreases in egg numbers and in survival of eggs, with reduced ovaries that lack mature ovarioles in the ITPL knockdown females (13). In contrast, ITP knockdown females had fully developed ovaries, however showed reduced oviposition rates and offspring survival. These developmental defects in T. castaneum were suggested to be due to hormonal imbalance in ovarian development, or indirectly caused by mating deficiencies, preventing exposure to male ejaculatory products essential for completion of ovarian development (13).
Considering the finding that AedaeItp and Itp-l knockdown mosquitoes resulted in fewer eggs laid by females mated with knockdown males, we predicted that transfer of sperm or sperm storage may be targeted. The regulation and entry of sperm into, protection within, and release from the storage organs (seminal vesicle in males, and spermathecae in females) requires both male and female-derived molecules (54–56). In male mosquitoes, spermatogenesis occurs in the paired testes, allowing for mature sperm cells, spermatozoa, to be synthesized (24, 29) and transported to the seminal vesicles via the vas deferens (57–59). During mating, male A. aegypti deposit sperm from the seminal vesicles into the female reproductive tract initially in the bursa and is later transferred into the spermathecae for long-term storage (60, 61). Herein, the results revealed lower spermatozoa counts in testes and seminal vesicles of males with Itp and Itp-l knockdown. Thus, this indicates that AedaeITP/ITP-L knockdown reduces the incidence of blood feeding by females, and additionally, knockdown reduces the number of spermatozoa in male seminal vesicles and in the spermathecae of females, which ultimately results in fewer eggs laid and reduced larval hatching. It remains to be investigated exactly what role AedaeITP and ITP-L play in male and female reproduction or in blood feeding behavior. However, given the already established roles of these neuropeptides in T. castaneum reproduction (13), it is possible that AedaeITP and ITP-L are similarly involved in regulation of male or female reproductive biology or mating behavior, influencing successful mating and transfer of spermatozoa into the female A. aegypti. Indeed ITP and ITP-L are multifunctional neuropeptides involved in metabolism, regulation of water and ion homeostasis, cuticle expansion and melanization, and reproduction (13, 15, 20, 23). The role in reproduction has been supported by ITPL expression in the B. mori male reproductive system with innervations of the accessory glands, the seminal vesicles, and the ejaculatory ducts (62), all organs critical for successful mating and reproduction. ITPL expression has also been found in the seminal fluid of the brown plant hopper, Nilaparvata lugens (23), which is transferred to females during mating. Future research examining AedaeITP and ITP-L signaling can provide greater insight on the actions of these neuropeptides in mosquito reproductive biology.
ITP and ITP-L peptides are highly homologous to the CHH peptides, which has been linked to molting, energy metabolism, immune defense, reproduction, and homeostatic regulation of osmotic and other stress responses (7, 63). In locusts, ITP stimulates fluid reabsorption and Cl-, Na+, and K+ transport, while inhibiting secretion of H+ in the ileum (16, 18). In Drosophila, ITP plays an essential role in development and locomotion (14, 15), and water homeostasis by protecting the fly from water loss by increasing thirst, reducing excretion rate, and promoting ingestion of water (15). Studies have also established functions of ITP during ecdysis in M. sexta (11) and wing expansion in N. lugens (23), while ITP-L has been linked to ovarian maturation in T. castaneum (13) and produced as a seminal fluid protein in N. lugens (23). Differences in the primary structure and cellular distribution patterns of ITP and ITP-L peptides suggest they may serve different biological functions. In conclusion, the current results expand our understanding of ITP and ITP-L in insects, providing evidence of differential expression, insight into their cell-specific distribution, and revealing novel independent physiological roles for these neuropeptides in the A. aegypti mosquito. Importantly, these findings also contribute towards our understanding of A. aegypti reproductive biology, which is of medical importance given their propensity of feeding on human hosts and role as a vector of several viruses. As such, given these neuropeptides appear to hold pleiotropic actions related to successful mating and reproduction, further insights into ITP and ITP-L signaling could contribute towards development of novel strategies for decreasing the fitness of these vectors, that may improve control of these anthropophilic mosquitoes.
Data availability statement
The datasets presented in this study can be found in online repositories. The names of the repository/repositories and accession number(s) can be found below: https://borealisdata.ca/dataverse/PaluzziLab, N/A.
Ethics statement
The manuscript presents research on animals that do not require ethical approval for their study.
Author contributions
FS: Conceptualization, Data curation, Formal analysis, Investigation, Methodology, Validation, Visualization, Writing – original draft, Writing – review & editing. J-PP: Conceptualization, Data curation, Formal analysis, Funding acquisition, Methodology, Project administration, Resources, Software, Supervision, Validation, Visualization, Writing – review & editing.
Funding
The author(s) declare financial support was received for the research, authorship, and/or publication of this article. This research was funded by: Natural Sciences and Engineering Research Council of Canada (NSERC) Discovery Grant (JPP), Ontario Ministry of Research Innovation Early Researcher Award (JPP), and NSERC CGS-D (FS) and the Carswell Scholarships in the Faculty of Science, York University (FS).
Conflict of interest
The authors declare that the research was conducted in the absence of any commercial or financial relationships that could be construed as a potential conflict of interest.
Publisher’s note
All claims expressed in this article are solely those of the authors and do not necessarily represent those of their affiliated organizations, or those of the publisher, the editors and the reviewers. Any product that may be evaluated in this article, or claim that may be made by its manufacturer, is not guaranteed or endorsed by the publisher.
Supplementary material
The Supplementary Material for this article can be found online at: https://www.frontiersin.org/articles/10.3389/finsc.2024.1374325/full#supplementary-material
References
1. Coast G. The endocrine control of salt balance in insects. Gen Comp Endocrinol. (2007) 152:332–8. doi: 10.1016/j.ygcen.2007.02.018
2. Strand MR, Brown MR, Vogel KJ. Mosquito peptide hormones: diversity, production, and function. Adv Insect Physiol. (2016) 51:145–88. doi: 10.1016/bs.aiip.2016.05.003
3. Schoofs L, De Loof A, Van Hiel MB. Neuropeptides as regulators of behavior in insects. Annu Rev Entomol. (2017) 62:35–52. doi: 10.1146/annurev-ento-031616-035500
4. Nässel DR, Zandawala M, Kawada T, Satake H. Tachykinins: neuropeptides that are ancient, diverse, widespread and functionally pleiotropic. Front Neurosci. (2019) 13:1262. doi: 10.3389/fnins.2019.01262
5. Chen H-Y, Toullec J-Y, Lee. The crustacean hyperglycemic hormone superfamily: progress made in the past decade. Front Endocrinol. (2020) 11:578958. doi: 10.3389/fendo.2020.578958
6. Nagai C, Mabashi-Asazuma H, Nagasawa H, Nagata S. Identification and characterization of receptors for ion transport peptide (ITP) and ITP-like (ITPL) in the silkworm Bombyx mori. J Biol Chem. (2014) 289:32166–77. doi: 10.1074/jbc.M114.590646
7. Webster SG, Keller R, Dircksen H. The CHH-superfamily of multifunctional peptide hormones controlling crustacean metabolism, osmoregulation, moulting, and reproduction. Gen Comp Endocrinol. (2012) 175:217–33. doi: 10.1016/j.ygcen.2011.11.035
8. Keller R. Crustacean neuropeptides: structures, functions and comparative aspects. Experientia. (1992) 48:439–48. doi: 10.1007/BF01928162
9. Dai L, Zitnan D, Adams ME. Strategic expression of ion transport peptide gene products in central and peripheral neurons of insects. J Comp Neurol. (2007) 500:353–67. doi: 10.1002/cne.21192
10. Endo H, Nagasawa H, Watanabe T. Isolation of a cDNA encoding a CHH-family peptide from the silkworm Bombyx mori. Insect Biochem Mol Biol. (2000) 30:355–61. doi: 10.1016/S0965-1748(99)00129-0
11. Drexler AL, Harris CC, dela Pena MG, Asuncion-Uchi M, Chung S, Webster S, et al. Molecular characterization and cell-specific expression of an ion transport peptide in the tobacco hornworm, Manduca sexta. Cell Tissue Res. (2007) 329:391–408. doi: 10.1007/s00441-007-0391-9
12. Audsley N, Mcintosh C, Phillips JE. Isolation of a neuropeptide from locust corpus cardiacum which influences ileal transport. J Exp Biol. (1992) 173:261–74. doi: 10.1242/jeb.173.1.261
13. Begum K, Li B, Beeman RW, Park Y. Functions of ion transport peptide and ion transport peptide-like in the red flour beetle Tribolium castaneum. Insect Biochem Mol Biol. (2009) 39:717–25. doi: 10.1016/j.ibmb.2009.08.005
14. Johard HAD, Yoishii T, Dircksen H, Cusumano P, Rouyer F, Helfrich-Förster C, et al. Peptidergic clock neurons in Drosophila: ion transport peptide and short neuropeptide F in subsets of dorsal and ventral lateral neurons. J Comp Neurol. (2009) 516:59–73. doi: 10.1002/cne.22099
15. Gáliková M, Dircksen H, Nässel DR. The thirsty fly: ion transport peptide (ITP) is a novel endocrine regulator of water homeostasis in Drosophila. PloS Genet. (2018) 14:e1007618. doi: 10.1371/journal.pgen.1007618
16. Audsley N, McIntosh C, Phillips JE. Actions of ion-transport peptide from locust corpus cardiacum on several hindgut transport processes. J Exp Biol. (1992) 173:275–88. doi: 10.1242/jeb.173.1.275
17. Phillips JE, Audsley N. Neuropeptide control of ion and fluid transport across locust hindgut. Integr Comp Biol. (1995) 35:503–14. doi: 10.1093/icb/35.6.503
18. Meredith J, Ring M, Macins A, Marschall J, Cheng NN, Theilmann D, et al. Locust ion transport peptide (ITP): primary structure, cDNA and expression in a baculovirus system. J Exp Biol. (1996) 199:1053–61. doi: 10.1242/jeb.199.5.1053
19. Phillips JE, Meredith J, Audsley N, Richardson N, Macins A, Ring M. Locust ion transport peptide (ITP): a putative hormone controlling water and ionic balance in terrestrial insects. Integr Comp Biol. (1998) 38:461–70. doi: 10.1093/icb/38.3.461
20. Dircksen H. Insect ion transport peptides are derived from alternatively spliced genes and differentially expressed in the central and peripheral nervous system. J Exp Biol. (2009) 212:401–12. doi: 10.1242/jeb.026112
21. Ring M, Meredith J, Wiens C, MacIns A, Brock HW, Phillips JE, et al. Expression of Schistocerca gregaria ion transport peptide (ITP) and its homologue (ITP-L) in a baculovirus/insect cell system. Insect Biochem Mol Biol. (1998) 28:51–8. doi: 10.1016/S0965-1748(97)00096-9
22. Dircksen H, Tesfai LK, Albus C, Nässel DR. Ion transport peptide splice forms in central and peripheral neurons throughout postembryogenesis of Drosophila melanogaster. J Comp Neurol. (2008) 509:23–41. doi: 10.1002/cne.21715
23. Yu B, Li DT, Wang SL, Xu HJ, Bao YY, Zhang CX. Ion transport peptide (ITP) regulates wing expansion and cuticle melanism in the brown planthopper, Nilaparvata lugens. Insect Mol Biol. (2016) 25:778–87. doi: 10.1111/imb.12262
24. Rocco DA, Kim DH, Paluzzi J-P. Immunohistochemical mapping and transcript expression of the GPA2/GPB5 receptor in tissues of the adult mosquito, Aedes aegypti. Cell Tissue Res. (2017) 369:313–30. doi: 10.1007/s00441-017-2610-3
25. Sajadi F, Curcuruto C, Al Dhaheri A, Paluzzi J-P. Anti-diuretic action of a CAPA neuropeptide against a subset of diuretic hormones in the disease vector Aedes aegypti. J Exp Biol. (2018) 221(7):jeb177089. doi: 10.1242/jeb.177089
26. Livak KJ, Schmittgen TD. Analysis of relative gene expression data using real-time quantitative PCR and the 2(-delta delta C(T)) method. Methods. (2001) 25:402–8. doi: 10.1006/meth.2001.1262
27. Paluzzi JP, Vanderveken M, O’Donnell MJ. The heterodimeric glycoprotein hormone, GPA2/GPB5, regulates ion transport across the hindgut of the adult mosquito, Aedes aegypti. PLoS One. (2014) 9:e86386.
28. Calkins TL, Piermarini PM. Pharmacological and genetic evidence for gap junctions as potential new insecticide targets in the yellow fever mosquito, Aedes aegypti. PloS One. (2015) 10:e0137084. doi: 10.1371/journal.pone.0137084
29. Rocco DA, Garcia ASG, Scudeler EL, dos Santos DC, Nóbrega RH, Paluzzi JPV. Glycoprotein hormone receptor knockdown leads to reduced reproductive success in male Aedes aegypti. Front Physiol. (2019) 10:266. doi: 10.3389/fphys.2019.00266
30. Durant AC, Donini A. Ammonium transporter expression in sperm of the disease vector Aedes aegypti mosquito influences male fertility. Proc Natl Acad Sci USA. (2020) 117:29712–9. doi: 10.1073/pnas.2011648117
31. Sun L, Zhang Z, Zhang R, Yu Y, Yang F, Tan A. Molecular disruption of ion transport peptide receptor results in impaired water homeostasis and developmental defects in Bombyx mori. Front Physiol. (2020) 11:424. doi: 10.3389/fphys.2020.00424
32. Xu W, Li G, Chen Y, Ye X, Song W. A novel antidiuretic hormone governs tumor-induced renal dysfunction. Nature. (2023) 624:425–32. doi: 10.1038/s41586-023-06833-8
33. Nässel DR, Pauls D, Huetteroth W. Neuropeptides in modulation of Drosophila behavior: how to get a grip on their pleiotropic actions. Curr Opin Insect Sci. (2019) 36:1–8. doi: 10.1016/j.cois.2019.03.002
34. Agard M, Zandawala M, Paluzzi JP. Another fly diuretic hormone: tachykinins increase fluid and ion transport by adult Drosophila melanogaster Malpighian ‘renal’ tubules. bioRxiv. (2024). doi: 10.1101/2024.02.22.581479
35. Copenhaver PF, Truman JW. Metamorphosis of the cerebral neuroendocrine system in the moth Manduca sexta. J Comp Neurol. (1986) 249:186–204. doi: 10.1002/cne.902490206
36. Homberg U, Davis NT, Hildebrand JG. Peptide-immunocytochemistry of neurosecretory cells in the brain and retrocerebral complex of the sphinx moth Manduca sexta. J Comp Neurol. (1991) 303:35–52. doi: 10.1002/cne.903030105
37. Žitňan D, Kingan TG, Kramer SJ, Beckage NE. Accumulation of neuropeptides in the cerebral neurosecretory system of Manduca sexta larvae parasitized by the braconid wasp Cotesia congregata. J Comp Neurol. (1995) 356:83–100. doi: 10.1002/cne.903560106
38. Žitňan D, Adams ME. Neuroendocrine regulation of insect ecdysis. In: Gilbert LI, Iatrou K, Gill SS, editors. Comprehensive Molecular Insect Science. (2005) 3:1–60.
39. Macins A, Meredith J, Zhao Y, Brock HW, Phillips JE. Occurrence of ion transport peptide (ITP) and ion transport-like peptide (ITP-L) in orthopteroids. Arch Insect Biochem Physiol. (1999) 40:107–18. doi: 10.1002/(ISSN)1520-6327
40. Terhzaz S, Cabrero P, Robben JH, Radford JC, Hudson BD, Milligan G, et al. Mechanism and function of drosophila capa GPCR: a desiccation stress-responsive receptor with functional homology to human neuromedinu receptor. PloS One. (2012) 7:e29897. doi: 10.1371/journal.pone.0029897
41. Wieczorek H. The insect V-ATPase, a plasma membrane proton pump energizing secondary active transport: molecular analysis of electrogenic potassium transport in the tobacco hornworm midgut. J Exp Biol. (1992) 172:335–43. doi: 10.1242/jeb.172.1.335
42. Beyenbach KW. Transport mechanisms of diuresis in Malpighian tubules of insects. J Exp Biol. (2003) 206:3845–56. doi: 10.1242/jeb.00639
43. Wieczorek H, Beyenbach KW, Huss M, Vitavska O. Vacuolar-type proton pumps in insect epithelia. J Exp Biol. (2009) 212:1611–9. doi: 10.1242/jeb.030007
44. Veenstra JA. Effects of 5-hydroxytryptamine on the Malpighian tubules of Aedes aegypti. J Insect Physiol. (1988) 34:299–304. doi: 10.1016/0022-1910(88)90139-4
45. Clark TM, Bradley TJ. Additive effects of 5-HT and diuretic peptide on Aedes Malpighian tubule fluid secretion. Comp Biochem Physiol Mol Integr Physiol. (1998) 119:599–605. doi: 10.1016/S1095-6433(97)00472-8
46. Coast GM, Garside CS, Webster SG, Schegg KM, Schooley DA. Mosquito natriuretic peptide identified as a calcitonin-like diuretic hormone in Anopheles Gambiae (Giles). J Exp Biol. (2005) 208:3281–91. doi: 10.1242/jeb.01760
47. Clark TM, Hayes TK, Beyenbach KW. Dose-dependent effects of CRF-like diuretic peptide on transcellular and paracellular transport pathways. Am J Physiol. (1998) 274:F834–40. doi: 10.1152/ajprenal.1998.274.5.F834
48. Clark TM, Hayes TK, Holman GM, Beyenbach KW. The concentration-dependence of CRF-like diuretic peptide: mechanisms of action. J Exp Biol. (1998) 201:1753–62. doi: 10.1242/jeb.201.11.1753
49. Pietrantonio PV, Jagge C, Taneja-Bageshwar S, Nachman RJ, Barhoumi R. The mosquito Aedes aegypti (L.) leucokinin receptor is a multiligand receptor for the three Aedes kinins. Insect Mol Biol. (2005) 14:55–67. doi: 10.1111/j.1365-2583.2004.00531.x
50. Lu HL, Kersch C, Pietrantonio PV. The kinin receptor is expressed in the Malpighian tubule stellate cells in the mosquito Aedes aegypti (L.): a new model needed to explain ion transport? Insect Biochem Mol Biol. (2011) 41:135–40. doi: 10.1016/j.ibmb.2010.10.003
51. Sajadi F, Uyuklu A, Paputsis C, Lajevardi A, Wahedi A, Ber LT, et al. CAPA neuropeptides and their receptor form an anti-diuretic hormone signaling system in the human disease vector, Aedes aegypti. Sci Rep. (2020) 10:1755. doi: 10.1038/s41598-020-58731-y
52. Sajadi F, Vergara-Martínez MF, Paluzzi J-PV. The V-type H+-ATPase is targeted in antidiuretic hormone control of the Malpighian “renal” tubules. Proc Natl Acad Sci USA. (2023) 120:e2308602120. doi: 10.1073/pnas.2308602120
53. Audsley N, Jensen D, Schooley DA. Signal transduction for Schistocerca gregaria ion transport peptide is mediated via both cyclic AMP and cyclic GMP. Peptides (N.Y.). (2013) 41:74–80. doi: 10.1016/j.peptides.2012.11.001
54. Avila FW, Ram KR, Bloch Qazi MC, Wolfner MF. Sex peptide is required for the efficient release of stored sperm in mated Drosophila females. Genetics. (2010) 186:595–600. doi: 10.1534/genetics.110.119735
55. Schnakenberg SL, Matias WR, Siegal ML. Sperm-storage defects and live birth in Drosophila females lacking spermathecal secretory cells. PloS Biol. (2011) 9:e1001192. doi: 10.1371/journal.pbio.1001192
56. Avila FW, Mattei AL, Wolfner MF. Sex peptide receptor is required for the release of stored sperm by mated Drosophila melanogaster females. J Insect Physiol. (2015) 76:1–6. doi: 10.1016/j.jinsphys.2015.03.006
57. Clements A. The Biology of Mosquitoes: Development, Nutrition and Reproduction. Chapman & Hall, London (2000) 327-335.
58. Oliva CF, Damiens D, Benedict MQ. Male reproductive biology of Aedes mosquitoes. Acta Trop. (2014) 132:S12–9. doi: 10.1016/j.actatropica.2013.11.021
59. Rocco DA, Paluzzi JPV. Functional role of the heterodimeric glycoprotein hormone, GPA2/GPB5, and its receptor, LGR1: an invertebrate perspective. Gen Comp Endocrinol. (2016) 234:20–7. doi: 10.1016/j.ygcen.2015.12.011
60. Degner EC, Harrington LC. A mosquito sperm’s journey from male ejaculate to egg: mechanisms, molecules, and methods for exploration. Mol Reprod Dev. (2016) 83:897–911. doi: 10.1002/mrd.22653
61. Camargo C, Ahmed-Braimah YH, Amaro IA, Harrington LC, Wolfner MF, Avila FW. Mating and blood-feeding induce transcriptome changes in the spermathecae of the yellow fever mosquito Aedes aegypti. Sci Rep. (2020) 10:14899. doi: 10.1038/s41598-020-71904-z
62. Klöcklerova V, Gáliková Z, Roller L, Zitnan D. Differential expression of ITP and ITPL indicate multiple functions in the silkworm Bombyx mori. Cell Tissue Res. (2023) 392:715–731. doi: 10.1007/s00441-023-03752-y
Keywords: ion transport peptide, ionoregulation, feeding, reproductive biology, anti-diuretic
Citation: Sajadi F and Paluzzi J-PV (2024) Molecular characterization, localization, and physiological roles of ITP and ITP-L in the mosquito, Aedes aegypti. Front. Insect Sci. 4:1374325. doi: 10.3389/finsc.2024.1374325
Received: 21 January 2024; Accepted: 21 March 2024;
Published: 09 April 2024.
Edited by:
Peter M. Piermarini, The Ohio State University, United StatesReviewed by:
Kenneth Veland Halberg, University of Copenhagen, DenmarkAndrew Nuss, University of Nevada, Reno, United States
Copyright © 2024 Sajadi and Paluzzi. This is an open-access article distributed under the terms of the Creative Commons Attribution License (CC BY). The use, distribution or reproduction in other forums is permitted, provided the original author(s) and the copyright owner(s) are credited and that the original publication in this journal is cited, in accordance with accepted academic practice. No use, distribution or reproduction is permitted which does not comply with these terms.
*Correspondence: Jean-Paul V. Paluzzi, cGFsdXp6aUB5b3JrdS5jYQ==