- 1Forest Pest Methods Laboratory, United States Department of Agriculture, Animal and Plant Health Inspection Service, Plant Protection and Quarantine, Science and Technology, Buzzards Bay, MA, United States
- 2Department of Environmental Conservation, University of Massachusetts, Amherst, MA, United States
- 3Beneficial Insects Introduction Research Unit, United States Department of Agriculture, Agricultural Research Service, Newark, DE, United States
- 4Key Laboratory of Forest Protection of National Forestry and Grassland Administration, Ecology and Nature Conservation Institute, Chinese Academy of Forestry, Beijing, China
- 5Department of Plant Sciences and Entomology, University of Rhode Island, Kingston, RI, United States
- 6Department of Entomology and Wildlife Ecology, University of Delaware, Newark, DE, United States
- 7Department of Ecology and Evolutionary Biology, Cornell University, Ithaca, NY, United States
The spotted lanternfly, Lycorma delicatula (Hemiptera: Fulgoridae), an invasive planthopper discovered in Pennsylvania, U.S. in 2014, has spread to many surrounding states despite quarantines and control efforts, and further spread is anticipated. A classical (importation) biological control program would contribute to the long-term management of L. delicatula in the eastern U.S. In its native range of China, Anastatus orientalis (Hymenoptera: Eupelmidae), an egg parasitoid, causes significant mortality. Anastatus orientalis consists of multiple haplotypes that differ in important biological parameters. To delineate the physiological host range of A. orientalis Haplotype C, we completed no-choice and choice testing. No-choice testing of non-target eggs from 36 insect species spanning six orders and 18 families showed that physiologically this haplotype of A. orientalis can develop in a variety of host species eggs from the families Coreidae, Fulgoridae, Pentatomidae, and Saturniidae. Ten of the 16 species that were attacked in the no-choice tests were also attacked in the choice tests. The production of progeny on non-target egg masses was significantly lower than on the controls (L. delicatula egg masses run simultaneously) in the no-choice and choice tests. For the non-target species that were attacked and resulted in female wasp progeny, these females were able to produce their own progeny at the same rate as control females that were reared from the L. delicatula eggs. Larger host eggs corresponded to an increased female-biased sex ratio of the progeny, suggesting that gravid females select them for fertilized eggs. Results from these studies suggest that A. orientalis Haplotype C prefers to parasitize L. delicatula egg masses but is capable of developing in some non-target species.
Introduction
The spotted lanternfly, Lycorma delicatula (White) (Hemiptera: Fulgoridae), is an invasive planthopper first detected in Berks County Pennsylvania in the fall of 2014 (1). It has since spread extensively within Pennsylvania and neighboring states where it threatens the grape, hops, tree fruit, plant nursery, and timber industries (2). Quarantines have been put in place to restrict the movement of plant, wood, and stone products, but because egg masses are cryptic and it is difficult to regulate the movement of all items potentially harboring them (3, 4), the pest has continued to spread and now has established populations in 14 states plus reports in two more (5). Research on host plant utilization indicates that L. delicatula can develop to the adult stage on several host plant species in addition to its favored host, tree-of-heaven (Ailanthus altissima (Mill.) Swin) (6), which further confounds the eradication efforts. Some mortality from resident fungal pathogens (7), egg parasitoids (8), and predation (9) have been noted but the incidences are rare and so far have resulted in inconsequential mortality rates. However, in its native range in China, several parasitoid wasps attack L. delicatula eggs and nymphs and cause significant mortality (10, 11). A classical (or importation) biological control program could contribute to management efforts against L. delicatula.
Anastatus orientalis Yang & Choi (Hymenoptera: Eupelmidae) is a small parasitic wasp that attacks L. delicatula eggs in its native range in China (10), most commonly in northeastern China (11). In Chinese field collections, A. orientalis parasitizes at a relatively high rate, ranging from 20 to 80% of egg masses attacked and up to 40% of eggs parasitized within individual egg masses (11, 12). Additionally, while suitable host plants are abundant for L. delicatula in these same locations in China, populations of L. delicatula are low compared to those of the invasive population in the U.S (11). This suggests that A. orientalis and other natural enemies are having a strong effect on L. delicatula populations in China. Anastatus orientalis was selected as a candidate biological control agent for helping to manage L. delicatula in South Korea where it is also invasive (13, 14). In 2011, the South Korean Rural Development Administration National Institute of Agricultural Sciences initiated a collaboration with the Chinese Academy of Forestry to collect and evaluate A. orientalis as a candidate biological control agent for invasive L. delicatula in Korea (10, 15, 16) and introductions of the parasitoids were made soon afterwards. Anastatus orientalis is being considered as a candidate biological control agent for the invasive populations of L. delicatula in the eastern United States.
Evaluation of the physiological host range is an essential first step to determine whether a natural enemy will be deemed sufficiently host-specific (17, 18) to be suitable for release as a biological control agent. We now know that multiple haplotypes of A. orientalis are present in China (19). Therefore, while initial testing work conducted for the releases in Korea suggested that the haplotype of A. orientalis released in Korea did not attack their species of concern (15), it is essential to test the strain of A. orientalis maintained in U.S. quarantine cultures (20) against the eggs of selected non-target species present in the United States.
Here we outline our results from no-choice and choice tests in which we tested eggs of planthoppers, stink bugs, other hemipterans, silk moths and selected other species that are native to or resident in the United States as potential hosts of A. orientalis. This manuscript describes testing of species of concern present in the eastern United States where L. delicatula currently is invasive. Due to concern about the threat of invasion by L. delicatula to the western United States, simultaneous coordinated testing of species resident to the western coast of the United States was conducted at the University of California Riverside Insectary and Quarantine Facility (21). To evaluate the physiological host range, we first exposed non-target eggs to mated female A. orientalis wasps in no-choice tests where the wasps had access to the non-target species eggs for a full week of exposure. For any species that were parasitized in the no-choice tests, we then conducted choice tests. For choice tests, the parasitoid was offered the target host, L. delicatula, together with the non-target egg masses so that the wasps could choose which (or both) host(s) to parasitize. This provided information on behavior and showed whether a parasitoid would choose to use a non-target when the primary host was also available. We present results of testing more than 30 different non-target species. Testing included Poblicia fuliginosa (Olivier) (Hemiptera: Fulgoridae), which is a closely related native species present in the current invasive range of L. delicatula. Initial priority species also included other species corresponding to taxonomic relatedness, the morphological similarity of egg masses, and occurrence in the same microhabitat as L. delicatula in the landscape.
Materials and methods
Parasitoid colony
The parasitoid colony we tested is maintained in the containment facility at the USDA APHIS Forest Pest Methods Laboratory, Buzzards Bay, MA, and all studies were completed in this facility. Laboratory colonies of A. orientalis were established from parasitized egg masses collected annually from the field in Beijing, China (N39.9925, E116.2109) from 2016 to 2019. To confirm the species and haplotype, we extracted genomic DNA from a single leg pulled from five randomly selected colony specimens using the QIAGEN DNeasy Blood & Tissue Kit (QIAGEN, Germantown, MD). Each specimen was genotyped for two mitochondrial fragments of the COI gene. We aligned the newly generated DNA sequences with reference sequences from the six haplotype groups identified in Wu et al. (19). Two months later, we conducted a second round of genotyping with 40 specimens to ensure colony homogeneity. After another eight months, we conducted the final round of genotyping including eight more specimens from the C colony and another 60 from the other haplotype colonies to ensure no contamination between strains.
We reared the wasps using L. delicatula egg masses collected each year from December to March in Pennsylvania. We collected egg masses whole and intact by cutting them from bark (primarily from A. altissima) and held them in a growth chamber at a constant 5°C with no light. We maintained the A. orientalis laboratory colony by setting up groups of three to five females with one or two males in a medium-sized rearing container (473-ml plastic deli cup, AD16 GenPak, Charlotte, NC) with the following temperature and light conditions: daily high of 25°C and low of 14°C, lights on 5:55 AM to 6:23 PM, 65% RH. These conditions were chosen to emulate environmental conditions in mid-September in Beijing, China, and hereafter will be referred to as ‘Beijing-fall conditions’ and work well for continuous rearing of this haplotype of A. orientalis (20). We provided each set of wasps with a streak of honey as a food source. The wasps were held without access to egg masses for one week (corresponding with their preoviposition period), and then given one L. delicatula egg mass for a period of one week. We held the developing progeny under Beijing-fall conditions for another month then moved to a 25°C constant temperature and a light cycle of 16 hours light and 8 hours dark to promote emergence. Further rearing details are provided in Broadley et al. (20).
Non-target selection, collection, and rearing
We selected non-target species for testing based on relatedness to L. delicatula (prioritizing Poblicia fuliginosa, which is in the same family, Fulgoridae) and other planthoppers, morphological similarity of egg clusters, and based on prior information on species utilized by other Anastatus species (22–25). Potential eastern U.S. non-target species spanning six insect orders and 18 families were field collected or acquired from laboratory colonies for no-choice and choice testing (Table 1). We saved voucher specimens of all species. We acquired species not already established in laboratory colonies as nymphs and adults through field collection in natural habitats, using visual inspection of host plant material, sweep netting, or beating of host plant material. We then moved field collected insects into rearing conditions designed for each species’ needs to produce egg masses. We kept phytophagous species on whole potted host plants or provided plant material such as vegetables. Typical laboratory rearing conditions were set at a temperature of 25°C and a light cycle of 16 hours light and 8 hours dark. We maintained entomophagous species in enclosures mimicking their natural habitat and provisioned them with insects that met their dietary requirements.
No-choice host testing experiments
To prepare for no-choice testing, we gently aspirated recently emerged A. orientalis wasps (24 hours old or less) from their plastic rearing containers. We placed up to five female and five male wasps together in a 1:1 ratio in a small glass rearing container (8 oz wide mouth mason jar 00500, Kerr, Newell, Atlanta, GA). The jars were streaked with honey for food and covered with mesh (no-see-um polyester netting 7250NSW, Bioquip, Rancho Dominguez, CA) to provide ventilation. Before use in experiments, the glass containers were autoclaved, and together with the metal lid rings and mesh, were washed with a one percent Citronox (Alcanox, White Plains, NY) solution, rinsed with DI water, then acetone, and air dried. This cleaning removed chemical traces that might affect the olfactory cues presented to the wasp because previous work found that A. orientalis responded to chemical traces left behind by L. delicatula (25). We placed the rearing containers in secondary plastic containers (6 Quart Storage Box 1642, Sterilite, Townsend, MA) to further isolate the tests from any outside olfactory cues. Before use, the secondary containers were also washed in a 1% Citronox solution and rinsed with DI water, then rinsed with 95% ethanol and left to air dry. The wasps were given a one-week preoviposition period at Beijing-fall conditions (described above).
Following the one-week preoviposition period, we removed all male wasps, and the female wasps were moved individually to a new glass rearing container and given either a non-target egg mass or a L. delicatula egg mass as a control to parasitize. The non-target eggs were put into testing as young as possible with the aim of testing them within less than a week. If they were not run within a five-day window, they were ramped down by moving them into 10°C for half a week then into 5°C until they could be put into testing. When multiple non-target species were tested during the same day, a single set of controls for that day was used for all the simultaneously-tested species, with sufficient numbers of control replicates to match the number of replicates of the non-target species having the most replication on that day. We tested non-target and control host species in separate secondary plastic containers to avoid mixing kairomones and other chemical cues. All wasps were allowed one week of oviposition under Beijing-fall conditions. After the one week, we removed the female wasps from the egg masses and preserved them in 95% ethanol. We placed the egg masses individually in plastic rearing containers (6oz, Clear Hinged Deli Cup, AD06, GenPak, Charlotte, NC, modified to include a mesh lid) and held them in Beijing-fall conditions for one month and then subsequently placed them under 25°C long day conditions for emergence. Our goal was to test 30 replicates for each non-target species, but some of the species were challenging to obtain so less replication was possible. We recorded host nymphal and F1 parasitoid emergence daily (Monday-Friday) until there was no further emergence for one month. We noted the sex of the parasitoids that emerged. Non-target nymphs were saved in 95% ethanol for vouchers.
Egg size measurements
We recorded egg size measurements for each species, including L. delicatula, to determine the mean volume. We measured ten eggs from three of the egg masses being dissected. For circular eggs, we measured the diameter; for ellipsoid and cube-shaped eggs, we measured height, width, and length; for cylindrical and oblate spheroid eggs, we measured width and height; and lastly for pentagonal frustum-shaped eggs, we measured the egg height, the width at the top of the pentagon, and width at the base of the pentagon. The volume of the eggs was calculated from these measurements.
We dissected a subset of available replicates (Table 2) for each non-target species and a comparative subset of L. delicatula egg masses. We waited at least two weeks after the last parasitoid emergence before dissecting the egg masses. For egg masses with no parasitoid emergence, we dissected them after one month. For those dissected, we recorded the fate of each egg.
Fitness of A. orientalis reared from non-target eggs
For each non-target egg mass that had female wasp emergence, we evaluated the fecundity of a randomized subset of these progeny (F1 generation). We placed up to five female wasps in a plastic rearing cup. Five male wasps from a different egg mass of the same species host were added to the rearing cup for a 1:1 ratio. If five males were not available, as many as possible were added, and in the event of no male wasps being present, five male wasps produced from a L. delicatula egg mass were added to ensure that the females could mate. We provisioned them with honey and held them under Beijing-fall conditions for a one-week preoviposition period. Following preoviposition, we removed all male wasps and all except two female wasps. The remaining two female wasps were each placed in a container and given a single L. delicatula egg mass. After one week of oviposition under Beijing-fall conditions, we removed the female wasps and saved them in 95% ethanol. We allowed the egg masses to develop for one month under Beijing-fall conditions before we moved them to 25°C long day conditions for emergence. We recorded nymphal and parasitoid emergence (F2 generation) daily (Monday-Friday) and saved all female wasps in 95% ethanol.
We compared the size of female progeny reared out of non-target hosts (F1 from non-targets) as compared to the simultaneously run controls reared from L. delicatula (F1 from controls) and to progeny produced when these non-target reared females (F1 from non-targets) were given L. delicatula to parasitize (F2 from the non-target females). We measured both hind tibiae from up to 10 F1 and F2 generation female parasitoids from each category from the testing of the non-targets Actias luna (L.) and Halyomorpha halys (Stål). Hind tibia measurements can be a useful proxy for fecundity, mating ability, and longevity (26), which together can suggest greater fitness (27–29). We chose progeny reared from these two non-target species to capture a wide range of egg volumes because A. luna had the largest non-target egg, and H. halys was among the smallest non-target species to produce female progeny. For each replicate, we removed both hind tibiae from female wasps and took measurements using Leica Microsystems model M125 C dissecting microscope with LASX software Version 3.7.2.22383.
Choice host testing experiments
For non-target species that produced wasps in the no-choice tests, we then conducted choice testing. We followed the same procedure as for the no-choice testing except that larger (16 oz wide mouth mason jar 1440061180, Ball, Newell, Atlanta, GA) glass rearing jars were used, and both the non-target and target (L. delicatula) egg mass were provided for oviposition. Following wasp exposure, we placed each egg mass into individual plastic rearing cups so that emergence could be recorded separately. We conducted host specificity testing between September 2018 and June 2021, with no-choice testing starting in September 2018 and choice testing starting in August 2020.
Statistical analyses
To test for the effect of non-target as compared to the controls, we used a Wilcoxon paired-sample test with the no-choice and choice tests analyzed separately. To test the effect of egg size on the resulting sex of wasp progeny produced, we ran a generalized linear model with a logit link function and a binomial distribution. To test for a difference in the number of F2 progeny produced from each F1 female, we used a one-way ANOVA. To compare the mean tibia measurements of female A. orientalis wasps (F1 generation) reared from a large non-target host (A. luna) and a small non-target host (H. halys) as compared to the controls (L. delicatula) and the size of the female progeny of these females, we averaged the measurements of both hind tibia for each individual, then ran a one-way ANOVA and a Tukey-Kramer’s test. All statistics were run using JMP 13.1.0 (SAS Institute Inc.) and figures were constructed using JMP 13.1.0 and R version 4.1.1 (The R Foundation for Statistical Computing).
Results
Parasitoid haplotype
Three rounds of genotyping on 53 A. orientalis specimens from the wasp colony used for this study over a 10-month period showed that the colony was composed of a homogenous population all representing the same species and the same haplotype. All sequences were identical and matched with A. orientalis Haplotype C.
No-choice and choice host-testing experiments
We completed no-choice testing of non-target eggs from 36 insect species spanning six orders and 18 families (Table 3). These tests included planthoppers (including a Fulgoridae) but also tests of non-targets from more distantly related species. Of these 36 species, A. orientalis was able to parasitize the eggs of 16 species and produce F1 progeny. No progeny were produced from any egg masses tested from species in the order Blattodea (cockroaches), Coleoptera (beetles), Mantodea (mantises) or Phasmatodea (stickbugs). However, every species tested in no-choice testing in the families Coreidae (leaf-footed bugs), Fulgoridae (lanternflies), Pentatomidae (stinkbugs), and Saturniidae (giant silk moths) was attacked to some degree.
The production of progeny on non-target egg masses was significantly lower than the production of progeny on the controls (L. delicatula egg masses run simultaneously) in the no-choice tests (Figure 1; χ2 = 1172.97, df = 1, p<0.0001) as well as in the choice tests (Figure 2; χ2 = 481.07, df = 1, p<0.0001). Ten of the 16 species that were attacked in the no-choice tests were also attacked in the choice tests (Table 3), although two of these (Euschistus tristigmus (Say) and Thyanta custator (Fabricius)) showed negligible attack rates. The non-target hosts that experienced the highest attack and the closest attack rates to that of the L. delicatula controls were the giant silk moths (Saturniidae) eggs, followed by stink bugs (Pentatomidae). For Edessa florida Barber, Oebalus pugnax (Fabricius), and Callosamia promethea (Drury), three species that were attacked in the no-choice tests, we were not able to acquire additional eggs for the subsequent choice testing. However, we conclude from the other tests of stink bug and silk moth eggs that these likely would have been utilized. For four species (Acanalonia bivittata (Say), Acanalonia conica (Say), Zelus luridus Stål, and Stagmomantis carolina (Johannson)) that were not attacked in the no-choice testing, we had extra available eggs and so we conducted a small number of choice tests with them as well, and no progeny were produced in the choice tests either. The number of progeny produced in the choice testing was overall lower than in the no-choice testing. This was true for the non-target eggs tested as well as the corresponding L. delicatula controls, and when more attack on non-targets was evident there was also less attack on the L. delicatula controls (as evident in results from testing the saturniids and H. halys).
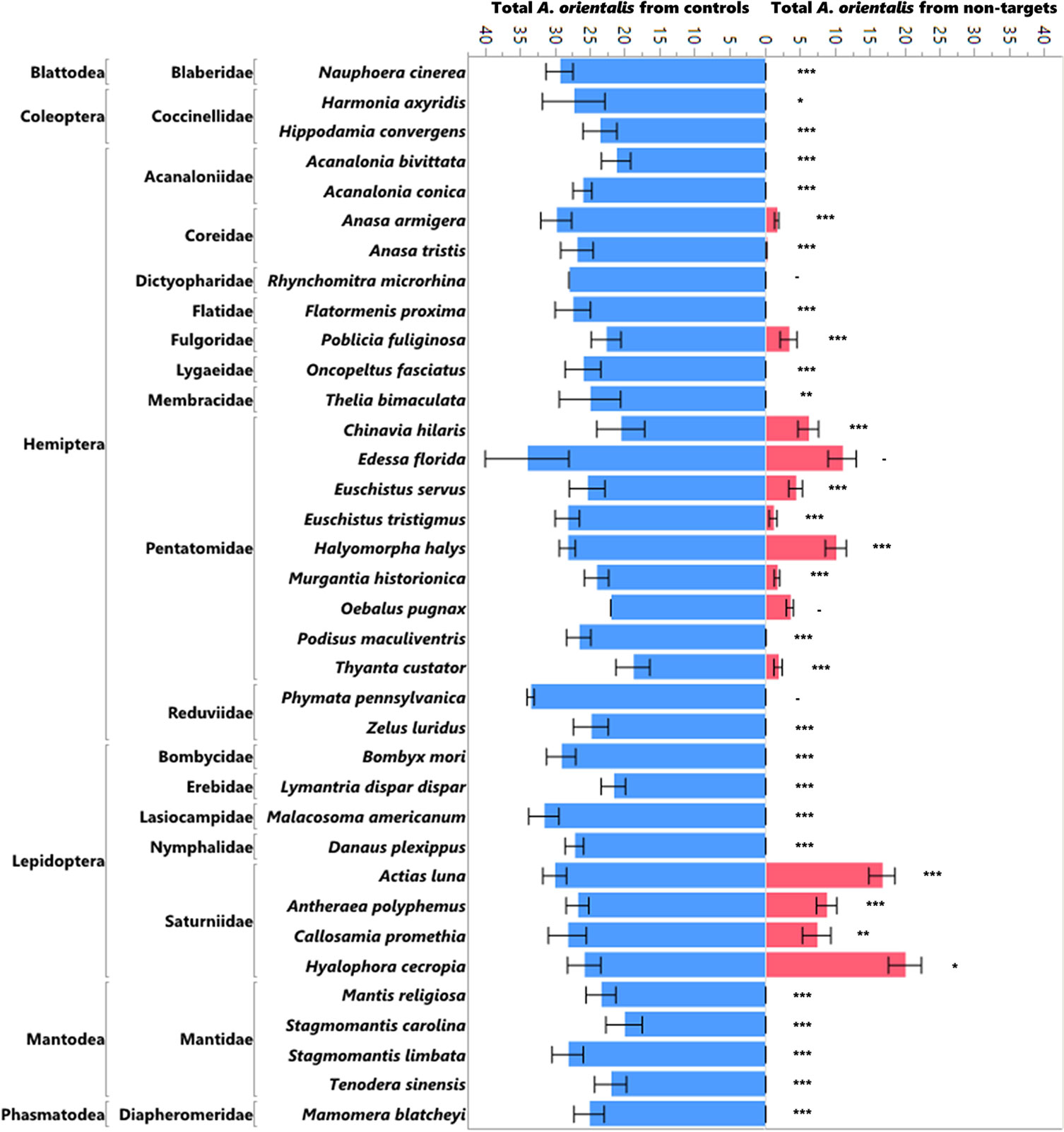
Figure 1 Mean number of A. orientalis produced in non-targets (pink) as compared to simultaneously run controls (blue) in no-choice tests organized by insect order and family. The error bars represent standard errors. Wilcoxon paired-sample test; *P < 0.05; **P < 0.01; ***P < 0.001; and a dash when the sample sizes were too small to run the test.
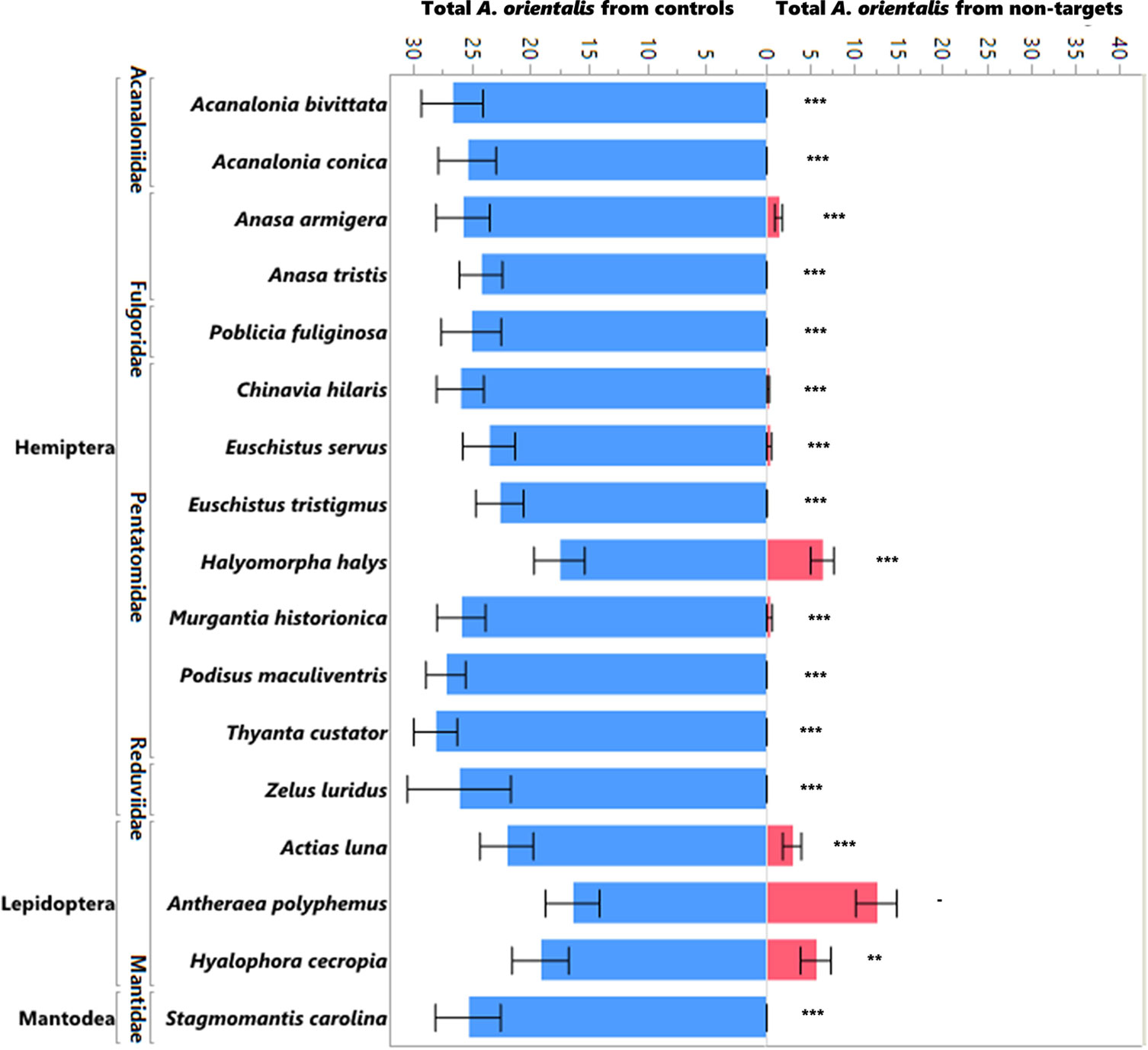
Figure 2 Mean number of A. orientalis produced in non-targets (pink) as compared to simultaneously run controls (blue) in choice tests organized by insect order and family. The figure includes all the species that showed attack in the no-choice tests as well as four additional species (A. bivittata, A. conica, Z. luridus, and S. carolina) that were put into testing. The error bars represent standard errors. Wilcoxon paired-sample test; **P < 0.01; ***P < 0.001; and a dash when the sample sizes were too small to run the test.
For 29 non-target species, a subset of egg masses was dissected (Table 2). We found no unemerged A. orientalis in 18 of the species and very little in the rest. In 11 species (A. luna, Anasa armigera (Say), Anasa tristis (DeGeer), Antheraea polyphemus (Cramer), Chinavia hilaris (Say), Euschistus servus (Say), H. halys, Hyalophora cecropia (L.), Murgantia histrionica (Hahn), P. fuliginosa, T. custator), all of which also successfully reared some A. orientalis, we found low numbers of unemerged A. orientalis present in the host eggs, either in a diapause state or as desiccated adults. Due to the scope of these dissections, we did not quantify dead early immature or encapsulated wasp larvae. Proportions of dissected eggs with unemerged A. orientalis were low, ranging from 0.01 to 0.21 (i.e., 1 to 21%) of egg masses with A. armigera and T. custator showing the lowest rates and A. tristis the highest rates. For all non-target species egg masses with some unemerged wasps, on average 1.44 ± 0.26 wasps per egg masses did not emerge. Parasitized L. delicatula egg masses reared under the same conditions had a similar rate of unsuccessful emergence, a proportion of 0.08 (or 8%) or a mean of 3.05 ± 0.60 wasps per egg mass that did not successfully emerge.
Effect of egg size
The size of the non-target egg presented to the A. orientalis wasps was important not only as a factor in whether the egg was successfully used but also in the resulting sex bias. Egg size had a significant effect on the sex of progeny produced (df = 1; χ2 = 9.26, p = 0.0023; Figure 3A). There was a significant relationship between egg volume and the sex ratio of the progeny produced with larger eggs having a female skewed sex ratio (df = 1; χ2 = 8.25, p = 0.0041; Figure 3B). The giant silk moth eggs overall showed the highest proportion of female progeny and were the only non-target species that resulted in female progeny in both the no-choice tests and the choice tests (Table 3). The giant silk moths also had some of the largest eggs that were put into testing. The species with the next highest ratio of female to male progeny was P. fuliginosa, followed by species of stink bugs.
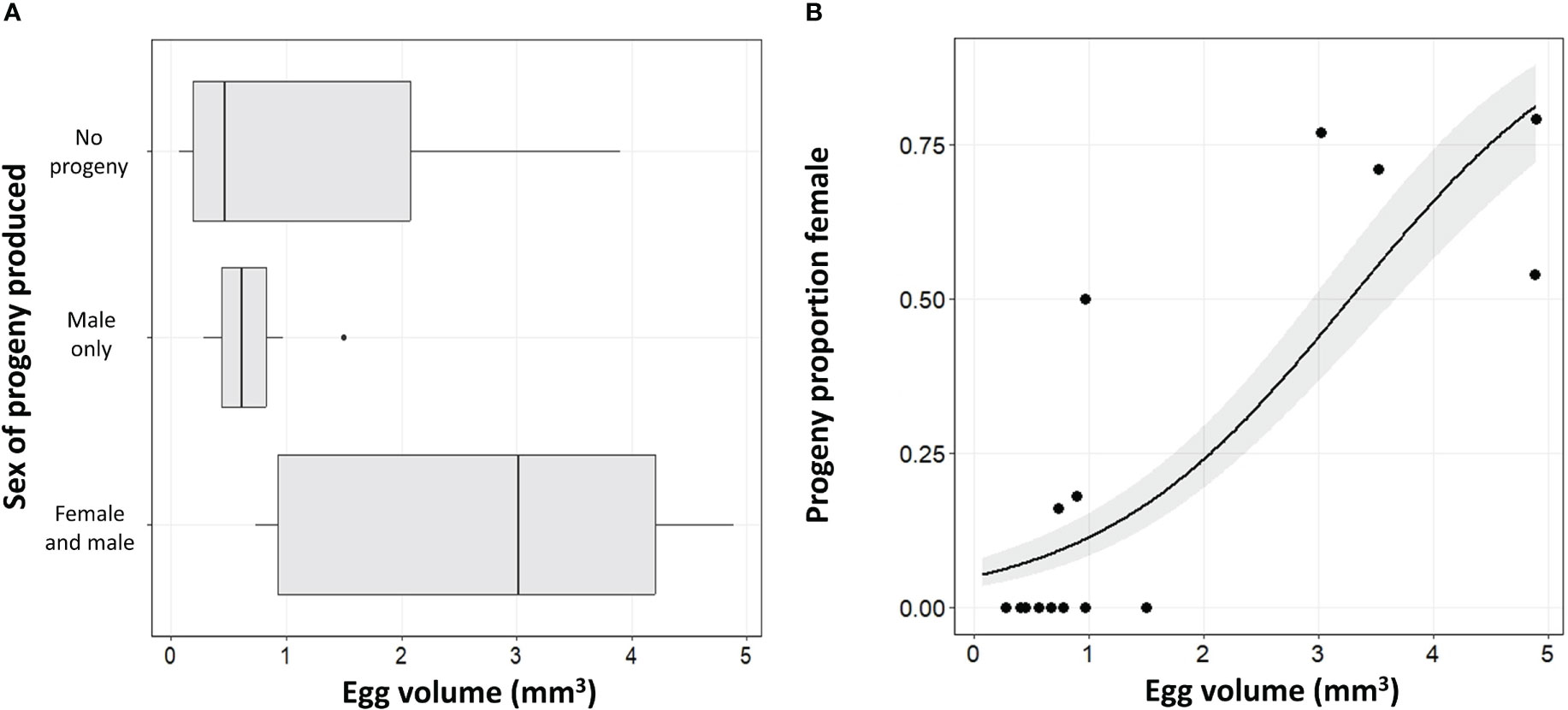
Figure 3 Mean egg volume (mm2) and the resulting wasp emergence patterns of the progeny showing (A) the sex of the progeny that emerged and (B) the proportion female.
Fitness of A. orientalis reared from non-target eggs
For the non-target species that were attacked, and which resulted in female wasp progeny (F1 generation), the F1 females were able to produce their own progeny (F2 generation) at the same rate as the F1 females that were reared from the control L. delicatula eggs. There was no significant difference (df = 5, 387, F = 1.6728, p = 0.1401) between the number of F2 wasp progeny produced by the F1 females reared out from the non-targets as from the F1 females reared out of L. delicatula (Figure 4). By comparing the mean tibia measurements of female A. orientalis wasps (F1 generation) reared on a large non-target host (A. luna) and a small non-target host (H. halys) compared to controls (L. delicatula), we found that the females reared from H. halys eggs were significantly smaller (0.59 mm ± 0.02 SE) than those reared from A. luna (0.88 mm ± 0.01 SE) and L. delicatula (0.94 mm ± 0.01 SE; df = 2, 22, F-ratio = 131.068, p < 0.0001). When these differently sized females, reared from different hosts, were all provided L. delicatula egg masses, their female progeny did not show any significant differences in tibia size (df = 2,27, F-ratio = 0.0655, p = 0.9368). Smaller progeny from non-target hosts were able to attack L. delicatula at the same rate as larger females and their progeny were of normal size.
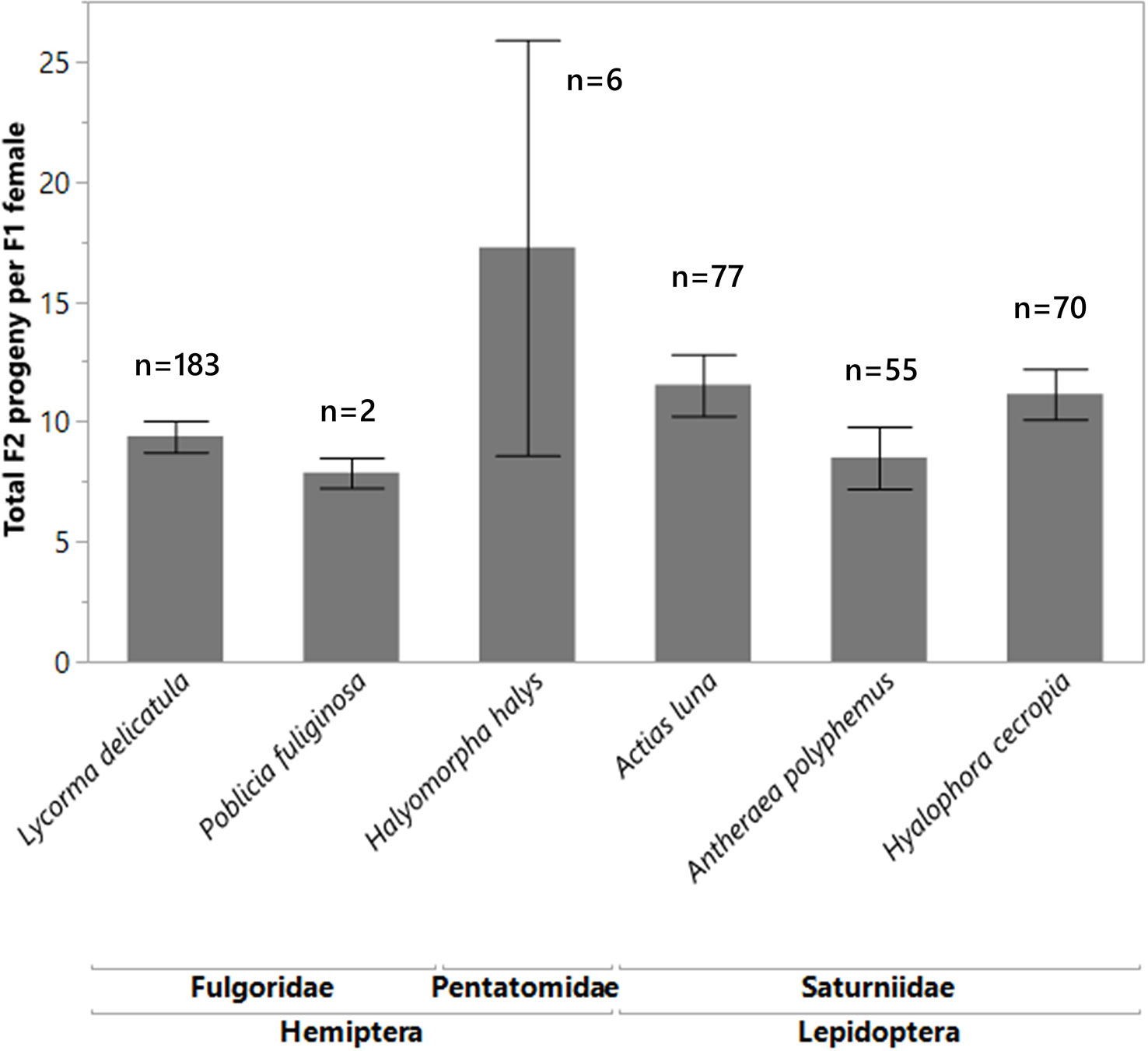
Figure 4 Mean number of F2 progeny wasps produced from spotted lanternfly eggs masses for each F1 female, which was reared from non-target species eggs. There was no significant difference (df = 5, 387, F = 1.6728, p = 0.1401) between the number of progeny produced by females reared out from the non-targets as compared to those reared from L. delicatula. The sample sizes are written above each species.
Discussion
Evaluation of the physiological host range is one of the first steps in assessing the risk-benefit potential of a biological control agent to determine if it is sufficiently host-specific (16, 17). The results from this study demonstrate that A. orientalis Haplotype C is physiologically able to develop in non-target egg masses of coreids, other fulgorids, pentatomids, and saturniids. However, the number of progeny produced from the non-targets is consistently lower than from the L. delicatula controls and the attack rates were particularly low when the wasps had a choice of using the non-target egg masses or the L. delicatula egg masses. The fact that we did not see attack on P. fuliginosa in the choice tests, even though the no-choice testing tells us that it is physiologically possible is interesting because, of the species tested, P. fuliginosa is the one most closely related (and biologically similar) to L. delicatula. Additional host range testing of western fulgorid planthoppers, as well as other potential non-targets that are resident to the western United States, was conducted by collaborators at the University of California Riverside (21). Together these studies show that A. orientalis Haplotype C prefers to parasitize L. delicatula egg masses but is willing to attack and is physiologically able to develop in various non-target species suggesting that it is facultatively oligophagous or polyphagous.
We found that A. orientalis Haplotype C is more likely to use large eggs, and more often used these large eggs to produce female progeny, and the progeny from large eggs are larger than those reared from smaller eggs. The three largest eggs included in the study were those of saturniid (giant silk moth) eggs—A. luna, A. polyphemus, and H. cecropia. Along with the two fulgorids— L. delicatula and P. fuliginosa—these showed the highest levels of attack and the highest proportion of female progeny. This is commonly found with other species of egg parasitoids (30, 31). We did not find any notable differences in the number of developing wasps that did not successfully emerge from the egg masses and the rate of this occurrence was very similar in the non-targets as it was for the controls. While H. halys was previously found to not be parasitized by A. orientalis (15), our tests and those of our colleagues (21) found that it could be parasitized. Also, Seo et al. (15) found that the saturniid Antheraea pernyi was only parasitized if the eggs were immature and dissected out of a gravid female. However, we found that older saturniid eggs were readily parasitized by A. orientalis. It may be that this difference in host range is due to differences in the haplotype or strain of A. orientalis tested in these studies. Future studies are planned to test the host range of other detected A. orientalis haplotypes.
Various factors may have affected our results. One factor could be the number of eggs presented to the wasps for each test. Wasps were given enough eggs to satisfy their oviposition needs. On average there were 42.9 ± 3.0 (mean ± SE, n = 84) L. delicatula eggs available in each egg mass tested, from which were produced on average 23.0 ± 0.43 wasp progeny during the one-week exposure period. The non-target replicates had an average of 27.4 ± 0.4 (mean ± SE, n = 725) eggs per replicate. This is a lower number of eggs per replicate than the control L. delicatula because they either did not lay their eggs in large masses or were challenging to rear in numbers. Considering that the mean number of adult wasps produced from the L. delicatula replicates was 23 wasps/egg mass, the wasps were provisioned with enough eggs such they were unlikely to use all available host eggs by the end of the oviposition period. Contamination by resident Anastatus species at the site of L. delicatula egg mass collection is another potential factor. However, parasitism of field-collected eggs by Anastatus species native or resident to the U.S. has been shown to be extremely rare (unpublished data), and no or at least inconsequential numbers would have been present in egg masses used for this study. The rearing temperatures, Beijing-fall conditions, were selected to optimize A. orientalis fitness (20) and are not necessarily the optimal rearing condition for the non-target species. However, for the most conservative tests possible here, we felt it was important to prioritize conditions for the parasitoid rather than for the various hosts. And lastly, when put into testing there was some variability of the age of eggs. Most non-target eggs were tested when they were less than a week old (median age = 6 days old, n=991) however there was variation around this median. The mean age was higher (12.5 ± 0.52 days). When exposed to wasps, the eggs of A. bivittata, A. conica, B. mori, Flatormenis proxima (Walker), P. fuliginosa, and Tenodera sinensis Saussure all had a median age that was greater than a week (13, 20, 49, 21, 30, and 18.5 days, respectively). The eggs of all other species were exposed when less than a week old. The fact that the eggs of these species were overall older may have artificially decreased their attractiveness or viability for A. orientalis as has been seen with other egg parasitoids (32–34). Additionally, the control eggs (of L. delicatula) were collected over the winter, stored in chill as described above, and used throughout the year whenever any of the non-targets were available for testing. However, based on prior research, L. delicatula eggs even up to a year old were viable hosts for A. orientalis with no discernable effect on parasitism rate (20).
While no-choice and choice host range testing are essential steps in assessing the risk-benefit of a candidate classical (or importation) biological control agent, it is important to keep in mind that these tests determine the ability of a parasitoid to physiologically use the hosts. By design, these tests are conducted in a controlled laboratory setting, which purposely limit the complexities of environmental conditions. In addition, any research conducted with A. orientalis in the U.S. as a candidate agent must be done in quarantine containment. Thus, these tests can overestimate ecologically relative host usage due to the limited ecological and environmental aspects of the tests (35, 36). In a field setting many additional factors influence a natural enemy’s ability to locate and utilize a potential host. A parasitoid must first be able to locate the host within a complex habitat, and once found, it may either accept it or decide to continue searching. For example, Trissolcus japonicus (Ashmead), a parasitoid of H. halys, displayed an oligophagous physiological host range in laboratory choice and no-choice assays (37–39) but a more restricted behavior in laboratory behavioral assays and field tests (40, 41).
Studies that focus on a natural enemy’s ability to find hosts include, but are not limited to, testing for attraction response to kairomones left by the non-targets and target pest species or large arena studies where the natural enemy is provided a larger and more complex space to search for the target and non-target hosts. Prior studies that tested the foraging behaviors of A. orientalis when in the presence of residues left by L. delicatula and the oothecal covering of L. delicatula eggs found that wasps detected chemical traces left by the L. delicatula, eliciting a strong arrestment response (42). Subsequent work evaluating ecological host range found that A. orientalis spent significantly more time interacting with chemical traces left behind by L. delicatula than the controls or than with the chemical traces left by P. fuliginosa (unpublished). This provides additional evidence that the preferred host of A. orientalis is L. delicatula. Tests evaluating the host range of A. orientalis in the field in China are planned using sentinel egg masses.
The population of A. orientalis tested in this study was Haplotype C. However, several other haplotypes of A. orientalis have been identified and these have now been separated into isofemale lines (19). Laboratory studies evaluating the rearing of the haplotypes shows that they respond differently to rearing conditions (19). This suggests that these haplotypes are genetically distinct but also that their biologies are distinct. Previous host range testing of A. orientalis conducted before release of it as a biocontrol agent in South Korea showed that it was highly host specific to L. delicatula and that H. halys eggs did not support development of A. orientalis (15). This is different than the testing results we obtained with Haplotype C, suggesting that the haplotype tested for release in South Korea may have been a different haplotype, potentially Haplotype D (19). Unlike our colony of Haplotype C wasps, the line tested for release in South Korea was successfully reared using constant 25°C temperature and long day light conditions, adding further evidence that what was released in Korea and the strain we are studying have different biological characteristics. Additional host range testing will be conducted with the additional genetic lines we have in colony. However, this study, along with the work of Gómez Marco et al. (21), shows that A. orientalis Haplotype C is willing and able to develop in various non-target species but prefers to parasitize L. delicatula.
Data availability statement
The raw data supporting the conclusions of this article will be made available by the authors, without undue reservation.
Author contributions
HB, SS, DP, KH, SD, JK, and JG designed the study. X-YW and L-MC provided the parasitoids for the study. HB, SS, DP, YW, and SD ran the studies. HB, SS, DP, KH, LT, TH, CB, AR and JK provided non-target insects to test. HB, SS, DP, and YW summarized the results. HB, SS, DP, TH, AR, JK, YW, and JG wrote the manuscript. All authors contributed to the article and approved the submitted version.
Funding
This work was made possible, in part, by funding from USDA Animal and Plant Health Inspection Service (APHIS) Plant Protection Act Section 7721 including funding from projects 6.0388 (2019), 6.0182 (2020), and 6.0978 (2021).
Acknowledgments
We thank Dr. Miriam Cooperband and her team as well as Matthew Travis, Greg Parra, Jeremy Wells, and Kerry Handelong for their help in providing spotted lanternfly egg mass collecting sites and collecting egg masses. For their assistance in conducting these experiments, we thank Liam Sullivan, Theresa Murphy, Kevin Grieb, Corrine Losch, and John McCormack. Thank you to Mary Cornelius, Kit Straley, and Alex Baranowski for their assistance rearing and providing non-targets. We thank Francesc Gómez Marco and Mark Hoddle for their feedback throughout these studies. This document may not necessarily express USDA views. The USDA is an equal opportunity employer. Mention of a concept, idea, trade name, or commercial product in this publication is solely for the purpose of providing specific information and does not imply recommendation or endorsement by the USDA.
Conflict of interest
The authors declare that the research was conducted in the absence of any commercial or financial relationships that could be construed as a potential conflict of interest.
Publisher’s note
All claims expressed in this article are solely those of the authors and do not necessarily represent those of their affiliated organizations, or those of the publisher, the editors and the reviewers. Any product that may be evaluated in this article, or claim that may be made by its manufacturer, is not guaranteed or endorsed by the publisher.
References
1. Barringer LE, Donovall LR, Spichiger S-E, Lynch D, Henry D. The first new world record of Lycorma delicatula (Insecta: Hemiptera: Fulgoridae). Entomol News (2015) 125(1):20–3. doi: 10.3157/021.125.0105
2. Urban JM. Perspective: Shedding light on spotted lanternfly impacts in the USA. Pest Manag Sci (2020) 76(1):10–7. doi: 10.1002/ps.5619
3. Urban JM, Calvin D, Hills-Stevenson J. Early response (2018–2020) to the threat of spotted lanternfly, Lycorma delicatula (Hemiptera: Fulgoridae) in Pennsylvania. Ann Entomological Soc America (2021) 114(6):709–18. doi: 10.1093/aesa/saab030
4. Urban JM, Leach H. Biology and management of the spotted lanternfly, Lycorma delicatula (Hemiptera: Fulgoridae), in the United States. Annu Rev Entomol (2022) 68:151–167. doi: 10.1146/annurev-ento-120220-111140
5. New York State Inegrated Pest Management. Cornell University college of agriculture and life sciences, in: Spotted lanternfly reported distribution updated February 27 (2012). Available at: https://cals.cornell.edu/new-york-state-integrated-pest-management/outreach-education/whats-bugging-you/spotted-lanternfly/spotted-lanternfly-reported-distribution-map (Accessed 5, 2022).
6. Dechaine AC, Sutphin M, Leskey TC, Salom SM, Kuhar TP, Pfeiffer DG. Phenology of Lycorma delicatula (Hemiptera: Fulgoridae) in Virginia, USA. Environ Entomology (2021) 50(6):1267–75. doi: 10.1093/ee/nvab107
7. Clifton EH, Castrillo LA, Gryganskyi A, Hajek AE. A pair of native fungal pathogens drives decline of a new invasive herbivore. Proc Natl Acad Sci (2019) 116(19):9178–80. doi: 10.1073/pnas.1903579116
8. Liu H. Occurrence, seasonal abundance, and superparasitism of Ooencyrtus kuvanae (Hymenoptera: Encyrtidae) as an egg parasitoid of the spotted lanternfly (Lycorma delicatula) in north America. Forests (2019) 10(2). doi: 10.3390/f10020079
9. Barringer LE, Smyers E. Predation of the spotted lanternfly, Lycorma delicatula (White) (Hemiptera: Fulgoridae) by two native hemiptera. Entomol News (2016) 126(1):71–3, 3. doi: 10.3157/021.126.0109
10. Yang ZQ, Choi WY, Cao LM, Wang XY, Hou ZR. A new species of Anastatus (Hymenoptera Eulpelmidae) from China, parasitizing eggs of Lycorma delicatula (Homoptera Fulgoridae). Zool Syst (2015) 40(3):290–302. doi: 10.11865/zs.20150305
11. Xin B, Zhang YL, Wang XY, Cao LM, Hoelmer KA, Broadley HJ, et al. Exploratory survey of spotted lanternfly (Hemiptera: Fulgoridae) and its natural enemies in China. Environ Entomol (2021) 50(1):36–45. doi: 10.1093/ee/nvaa137
12. Choi MY, Yang ZQ, Wang XY, Tang YL, Hou ZR, Kim JH, et al. Parasitism rate of egg parasitoid Anastatus orientalis (Hymenoptera: Eupelmidae) on Lycorma delicatula (Hemiptera: Fulgoridae) in China. Korean J Appl Entomology (2014) 53(2):135–9. doi: 10.5656/KSAE.2014.01.1.075
13. Park J-D, Kim M-Y, Lee S-G, Shin S-C, Kim J-H, Park I-K. Biological characteristics of Lycorma delicatula and the control effects of some insecticides. Korean J Appl entomology (2009) 48(1):53–7. doi: 10.5656/KSAE.2009.48.1.053
14. Kim H, Kim S, Lee Y, Lee H-S, Lee S-J, Lee J-H. Tracing the origin of Korean invasive populations of the spotted lanternfly, Lycorma delicatula (Hemiptera: Fulgoridae). Insects (2021) 12(6). doi: 10.3390/insects12060539
15. Seo M, Kim JH, Seo BY, Park C, Choi BR, Kim HH, et al. Mass rearing techniques of Anastatus orientalis (Hymenoptera: Eupelmidae), as the egg-parastioid of Lycorma delicatula (Hemiptera: Fulgoridae): An using method of Antheraea pernyi (Lepidoptera: Saturniidae) and L. delicatula eggs in laboratory. Korean J Appl Entomology (2018) 57(4):243–51. doi: 10.5656/KSAE.2018.08.0.035
16. Seo M, Kim JH, Seo BY, Park C, Choi BR, Kim KH, et al. Effect of temperature on reproduction and parasitism of the egg parasitoid, Anastatus orientalis (Hymenoptera: Eupelmidae). J Asia-Pacific Entomology (2019) 22(4):1013–8. doi: 10.1016/j.aspen.2019.08.009
17. Van Driesch R, Hoddle M, Center T. Control of pest and weeds by natural enemies: An introduction to biological control. Malden MA: Blackwell Publishing (2008). doi: 10.1653/024.092.0237
18. Hajek AE, Eilenberg J. Natural enemies: An introduction to biological control. New York, New York: Cambridge University Press (2018).
19. Wu Y, Broadley HJ, Vieira KA, McCormack JJ, Losch C, McGraw AR, et al. Cryptic genetic diversity and associated ecological differences of Anastatus orientalis, an egg parasitoid from China of the spotted lanternfly. Front Insect Sci – Invasive Insect Species.
20. Broadley HJ, Gould JR, Sullivan LT, Wang XY, Hoelmer KA, Hicken ML, et al. Life history and rearing of Anastatus orientalis (Hymenoptera: Eupelmidae), an egg parasitoid of the spotted lanternfly (Hemiptera: Fulgoridae). Environ Entomol (2020) 50(1):28–35. doi: 10.1093/ee/nvaa124
21. Gómez Marco F, Yanega D, Ruiz M, Hoddle MS. Proactive classical biological control of Lycorma delicatula (Hemiptera: Fulgoridae) in California (U.S.): Host range testing of Anastatus orientalis (Hymenoptera: Eupelmidae). Front Insect Science (2023) 3. doi: 10.3389/finsc.2023.1134889
22. Narasimham AU, Sankaran T. Biology of Anastatus umae (Hymenoptera: Eupelmidae), an oothecal parasite of Neostylopyga rhombifolia. Colemania (1982) 1:135–40.
23. Mendel MJ, Shaw PB, Owens JC. Life-history characteristics of Anastatus semiflavidus (Hymenoptera: Eupelmidae), an egg parasitoid of the range caterpillar, Hemileuca oliviae (Lepidoptera: Saturniidae) over a range of temperatures. Environ Entomol (1987) 16(5):1035–41. doi: 10.1093/ee/16.5.1035
24. Narasimham AU. Comparative biological parameters of Comperia merceti (Compere) (Hym., encyrtidae) and Anastatus tenuipes Bolivar (Hym., eupelmidae), oothecal parasitoids of the cockroach Supella longipalpa (Fab.). Biol Control (1992) 2(1):73–7. doi: 10.1016/1049-9644(92)90078-R
25. Stahl JM, Babendreier D, Marazzi C, Caruso S, Costi E, Maistrello L, et al. Can Anastatus bifasciatus be used for augmentative biological control of the brown marmorated stink bug in fruit orchards? Insects (2019) 10(4):108. doi: 10.3390/insects10040108
26. Roitberg BD, Boivin G, Vet LEM. Fitness, parastioids, and biological control: An opinion. Can Entomologist (2001) 133(3):429–38. doi: 10.4039/Ent133429-3
27. Flanagan KE, West SA, Godfray HCJ. Local mate competition, variable fecundity and information use in a parasitoid. Anim Behav (1998) 56(1):191–8. doi: 10.1006/anbe.1998.0768
28. Sagarra LA, Vincent C, Stewart RK. Body size as an indicator of parasitoid quality in Male and female Anagyrus kamali (Hymenoptera: Encyrtidae). Bull Entomological Res (2001) 91(5):363–7. doi: 10.1079/BER2001121
29. Kasamatsu E, Abe J. Influence of body size on fecundity and sperm management in the parasitoid wasp Anisopteromalus calandrae. Physiol Entomol (2015) 40(3):223–31. doi: 10.1111/phen.12106
30. Stahl JM, Babendreier D, Haye T. Using the egg parasitoid Anastatus bifasciatus against the invasive brown marmorated stink bug in Europe: Can non-target effects be ruled out? J Pest Sci (2018) 91(3):1005–17. doi: 10.1007/s10340-018-0969-x
31. Charnov EL, Los-den Hartogh R, Jones W, Van den Assem J. Sex ratio evolution in a variable environment. Nature (1981) 289(5793):27–33. doi: 10.1038/289027a0
32. Hintz JL, Andow DA. Host age and host selection Bytrichogramma nubilale. Entomophaga (1990) 35(1):141–50. doi: 10.1007/BF02374310
33. Rocha Ld, Kolberg R, Mendonça JrM, Redaelli LR. Effects of egg age of Spartocera dentiventris (Berg) (Hemiptera: Coreidae) on parasitism by Gryon gallardoi (Brethes) (Hymenoptera: Scelionidae). Neotropical Entomology (2006) 35:654–9. doi: 10.1590/S1519-566X2006000500013
34. Hanks LM, Gould JR, Paine TD, Millar JG, Wang Q. Biology and host relations of Avetianella longoi (Hymenoptera: Encyrtidae), an egg parasitoid of the eucalyptus longhorned borer (Coleoptera: Cerambycidae). Ann Entomological Soc America (1995) 88(5):666–71. doi: 10.1093/aesa/88.5.666
35. Van Driesche R, Murray T, Reardon R. Assessing host ranges for parasitoids and predators used for classical biological control: A guide to best practice. (West Virginia: United States Department of Agriculture Forest Health Technology Enterprise Team, Morgantown) (2004).
36. Haye T, Goulet H, Mason PG, Kuhlmann U. Does fundamental host range match ecological host range? A retrospective case study of a lygus plant bug parasitoid. Biol Control (2005) 35(1):55–67. doi: 10.1016/j.biocontrol.2005.06.008
37. Lara JR, Pickett CH, Kamiyama MT, Figueroa S, Romo M, Cabanas C, et al. Physiological host range of Trissolcus japonicus in relation to Halyomorpha halys and other pentatomids from California. BioControl (2019) 64(5):513–28. doi: 10.1007/s10526-019-09950-4
38. Zhang J, Zhang F, Gariepy T, Mason P, Gillespie D, Talamas E, et al. Seasonal parasitism and host specificity of Trissolcus japonicus in northern China. J Pest Sci (2017) 90(4):1127–41. doi: 10.1007/s10340-017-0863-y
39. Sabbatini-Peverieri G, Boncompagni L, Mazza G, Paoli F, Dapporto L, Giovannini L, et al. Combining physiological host range, behavior and host characteristics for predictive risk analysis of. Trissolcus japonicus J Pest Sci (2021) 94(3):1003–16. doi: 10.1007/s10340-020-01311-w
40. Bertoldi V, Rondoni G, Brodeur J, Conti E. An egg parasitoid efficiently exploits cues from a coevolved host but not those from a novel host. Front Physiol (2019) 10:746. doi: 10.3389/fphys.2019.00746
41. Milnes JM, Beers EH. Trissolcus japonicus (Hymenoptera: Scelionidae) causes low levels of parasitism in three north American pentatomids under field conditions. J Insect Sci (2019) 19(4):15. doi: 10.1093/jisesa/iez074
Keywords: biological control, Eupelmidae, Fulgoridae, invasive species, natural enemy
Citation: Broadley HJ, Sipolski SJ, Pitt DB, Hoelmer KA, Wang X-y, Cao L-m, Tewksbury LA, Hagerty TJ, Bartlett CR, Russell AD, Wu Y, Davis SC, Kaser JM, Elkinton JS and Gould JR (2023) Assessing the host range of Anastatus orientalis, an egg parasitoid of spotted lanternfly (Lycorma delicatula) using Eastern U.S. non-target species. Front. Insect Sci. 3:1154697. doi: 10.3389/finsc.2023.1154697
Received: 31 January 2023; Accepted: 14 March 2023;
Published: 18 April 2023.
Edited by:
Frank H. Koch, Forest Service (USDA), United StatesReviewed by:
Osariyekemwen Uyi, University of Benin, NigeriaKier Klepzig, University of Georgia, United States
Copyright © 2023 Broadley, Sipolski, Pitt, Hoelmer, Wang, Cao, Tewksbury, Hagerty, Bartlett, Russell, Wu, Davis, Kaser, Elkinton and Gould. This is an open-access article distributed under the terms of the Creative Commons Attribution License (CC BY). The use, distribution or reproduction in other forums is permitted, provided the original author(s) and the copyright owner(s) are credited and that the original publication in this journal is cited, in accordance with accepted academic practice. No use, distribution or reproduction is permitted which does not comply with these terms.
*Correspondence: Hannah J. Broadley, hannah.j.broadley@usda.gov