- Neuroscience Program, Department of Psychological and Brain Sciences, Colgate University, Hamilton, NY, United States
We examined amino acid appetite in the omnivorous house cricket (Acheta domesticus), a common model organism for both research and teaching. Our first experiment addressed the hypothesis that house crickets can discriminate between sucrose and essential amino acids (EAA), and that preference for the latter would be affected by prior feeding experience. To test this hypothesis, we compared feeding responses of juvenile and adult crickets following pre-feeding with sucrose or an essential amino acid mixture, predicting that sucrose-only pre-feeding would enhance subsequent intake of amino acids in a two-choice preference test. Based on previous studies, we also predicted that amino acid consumption would be enhanced in females compared to males, and in mated compared to virgin females. Hence we compared responses in male and female last instar nymphs, adult males, virgin females, mated females, and mated females allowed to lay eggs. The second experiment examined how extended periods of essential amino acid deprivation (48 h to 6 days) affected appetite for these nutrients in adult male and female insects. Finally, we examined growth and survival of juvenile and adult crickets fed a holidic diet lacking all amino acids and protein. Our results demonstrated that house crickets can distinguish EAA from sucrose and that consumption of the former is enhanced following sucrose-only pre-feeding. We also found sex and developmental differences, with juvenile and virgin females showing a greater preference for EAA than juvenile or adult males. Contrary to expectation, mated females preferred sucrose over EAA both prior to and after egg laying. We also found that the crickets of both sexes increased their intake of EAA when exposed to longer periods of deprivation, indicating that they engage in compensatory feeding on these nutrients. Finally, as expected we found that growth was severely limited in juveniles fed a diet lacking all amino acids, but adults and many juveniles survived for 30 days on this diet.
Introduction
Omnivorous animals forage in complex and heterogenous food landscapes and must choose the correct mix of nutrients for growth and fitness. Many insects have evolved the ability to make such choices and will select an array of foods that offer the correct balance of essential nutrients. Researchers have used artificial diets to examine the regulation of macronutrients, especially protein and carbohydrates, in a number of diverse species (1). These studies have demonstrated that insects feed selectively when offered two foods which differ in protein:carbohydrate ratios, causing intake of each nutrient to match a ratio that promotes species-specific fitness (2–9). Insects also regulate the amount of food consumed and adjust their intake depending on the nutrient density of the food. For example, grasshoppers compensate behaviorally for nitrogen dilute diets by increasing the number and duration of feeding bouts (10); bumble bees decrease consumption of pollen as the concentration of protein increases (11). An additional key finding is that, following protein or carbohydrate deprivation, insects subsequently engage in compensatory feeding on the limited substance to restore nutrient balance. The compensatory behavior can occur following days of deprivation or just a single meal that lacks a macronutrient (12–16).
In addition to macronutrient selection, insects can distinguish other substances, including micronutrients and free amino acids. Amino acids are important since they may signal the presence of protein and are necessary for numerous biochemical functions, including neurotransmitter biosynthesis. The ability of insects to perceive free amino acids and self-select food based on this perception has been studied in Drosophila adults and larvae (17–19) and nectar-feeding insects such as bees, ants, and mosquitoes (20–25). These studies demonstrate that an amino acid and sugar mix may be preferred over sugar alone, and that individual amino acids vary in their ability to stimulate feeding. Studies have also demonstrated that diets lacking essential amino acids (EAAs) can cause rejection of the unbalanced food (26) and enhance attraction to and feeding on amino acid mixtures or protein (17, 27). Lack of even a single EAA can induce this compensatory feeding on amino acid mixtures or protein (24, 28). Adaptive changes in amino acid intake can involve changes in search behavior to locate sources of amino acids or changes in sensory neuron responses to favor amino acid feeding (1, 29).
In addition to nutritional state, responses to protein, carbohydrate, and other nutrients vary with developmental stage, sex, and mating status. For example, Cohen et al. (3) found that carbohydrate consumption varied as cockroach larvae developed through successive instars, with intake highest directly following molting. Carbohydrate intake also shifted in mealworm larvae becoming greater in later compared to earlier instars (9). In Drosophila, larvae chose to linger on yeast patches (30) whereas well-nourished adult Drosophila fed preferentially on sucrose. However, compared to males, female Drosophila show a greater preference for feeding on yeast, a source of protein (18). Likewise adult crickets of both sexes prefer carbohydrate over protein (31, 32), but females consume more protein than males (31). The preference for protein was markedly enhanced in Drosophila females after mating, a response attributed to an elevated need for protein to produce eggs (15, 33). In the cricket Gryllus bimaculatus, protein consumption was also greater in mated compared to virgin females (34). Because the dietary switch occurred after the female crickets laid eggs, Tsukamoto et al. suggest that the enhanced protein appetite reflected the need to produce additional eggs for future episodes of mating and egg laying.
The house cricket (Acheta domesticus) is a common model organism for both research and teaching and is also important commercially as pet food and more recently as a source of protein for human consumption (35). Like many cricket species, A. domesticus is omnivorous, consuming foods that vary greatly in nutritional content across space and time. These feeding habits predict that crickets will be tolerant of temporary nutritional imbalances and able to address imbalances by compensatory feeding when needed nutrients become available (14). Compensatory feeding on macronutrients has been shown in several cricket species, but the ability to detect and self-select amino acids has not yet been studied. The quantity of amino acids consumed affects growth, reproduction, and longevity in insects and, as in mammals, certain amino acids are essential and must be acquired from the diet. We designed experiments to examine the ability of house crickets to detect EAA, and investigated how feeding decisions are affected by nutritional state, age, and sex.
Our first experiment addressed the hypothesis that house crickets can discriminate between sucrose and EAA, and that preference for the latter would be enhanced following a meal containing only sucrose. In this experiment, we used a two-choice preference test to compare food intake and feeding behavior of crickets following pre-feeding with sucrose or EAA. Previous studies have demonstrated that appetite for carbohydrates and protein can vary significantly in insects depending on age and sex. To determine if such variation occurs in house crickets, we tested males and females separately and compared responses in last instar nymphs and adult animals. Previous studies have also reported that female reproductive status affects protein appetite, and hence the first experiment included two additional groups: mated females, and mated females allowed to lay eggs. For the latter comparisons, we predicted that amino acid appetite would be enhanced in females compared to males, and in mated compared to virgin females. In our second experiment, we used extended periods of EAA deprivation to determine if crickets would subsequently engage in compensatory feeding on an amino acid mixture. Finally, to further understand cricket motivation for amino acid consumption, we examined growth and survival of juvenile and adult crickets fed a holidic diet lacking all amino acids and protein.
Materials and methods
Insects and diets
Juvenile and adult A. domesticus were acquired from laboratory-reared colonies originally established using animals from commercial suppliers. They were maintained at 27° C, 12:12 light:dark with ad libitum access to a standard food (Mazuri Cricket Diet) and water. To obtain virgins, females were removed from the colony before molting to adults and housed in female-only tanks (45 x 25 x 25 cm high) prior to testing. Males used in experiments were taken from the mixed sex colonies. For food preference tests, crickets were tested in same sex groups of five in clear plastic containers (19 x 28.5 x 23.5 cm high) equipped with an egg carton shelter and water. For survival tests, crickets were held in the same plastic containers with three adults or ten 4th instar juveniles per container.
Test diets for food preference tests consisted of pure 0.5 M sucrose, a mixture of 10 essential L-amino acids (lysine, tryptophan, histidine, phenylalanine, leucine, isoleucine, threonine, methionine, valine, and arginine), and a mixture of 0.5 M sucrose plus 10 EAA. We opted to incorporate chemicals into agar gel which kept nutrients dissolved and prevented food from being scattered or carried by the crickets during feeding. Pure sucrose was made by combining distilled water with 2% agar and adding sucrose to form a 0.5 M solution. For the amino acid mixture, we followed other protocols by using an equimolar mixture with amino acids each at 10 mM dissolved in distilled water and 2% agar (22, 36, 37, Ignall et al., 2021). For the sucrose/amino acid mixture, amino acids were added to the liquid 0.5 M sucrose agar solution. The latter mixture was used during pre-feeding to ensure that insects seeking sucrose would necessarily ingest amino acids as well.
To test the survival of crickets fed EAA-free food, holidic diets were created following the recipe developed for other orthopterans (6, 38). The complete holidic diet contained 2.4% Wesson salt mix, 0.5% linoleic acid, 0.5% cholesterol, 0.2% Vanderzant vitamin mix, 10 EAA each at 5 mM, 0.5 M sucrose, and 2% agar. The amino acid free diet included all chemicals except the 10 amino acids, and a control diet contained 20% standard cricket food mixed with 2% agar. After chemicals were completely dissolved, solutions were cooled to 55°C, poured into 100 x 15 mm petri dishes, and refrigerated until use. Chemicals were acquired from the following sources: Wesson salt mix (Fisher Scientific, Pittsburg PA), Vanderzant vitamin mix (Frontier Scientific Inc., Logan, UT), L-threonine and L-leucine (Cayman Chemical Co., Ann Arbor, MI), all other chemicals (Sigma- Aldrich Inc., St. Louis, MO).
Experiment 1: Pre-feeding with sucrose or EAA and subsequent food preference
Experiment 1 examined the effects of pre-feeding with sucrose or EAA on subsequent preference for the two foods. The following groups were tested: juveniles (last instar nymphs), adult males, adult virgin females, adult mated females, and adult mated females allowed to lay eggs. For each of the five groups, 16-20 containers were tested, half pre-fed sucrose and half pre-fed the sucrose/EAA mix (80-100 crickets/group; 530 crickets total; each cricket participated in only one test). Crickets were placed in containers and fasted (no food available) for 24 h after which they received food consisting of pure 0.5 M sucrose or 0.5 M sucrose/EAA mix in agar. Food was placed on plastic petri dish (15 mm) lids and set in the tanks for four hours. 24 h later crickets participated in a two-choice preference test in which they received two types of food: pure 0.5 M sucrose and the pure EAA food described above (Figure 1A). Each food type for pre-feeding or choice tests was provided by cutting uniform disks from agar plates, each disk weighing approximately 425 mg and measuring 9 mm in diameter and 5 mm high. In choice tests, the two disks were each placed on a plastic petri dish lid and set adjacent to each other in the tank. As in pre-feeding, crickets were allowed access to the food for four hours. To determine the exact amount of food consumed, each disk was weighed to the nearest 0.1 mg before and after the trial period. If no food of either type was consumed, the tank was eliminated and the experiment replicated with additional crickets to yield the full data set. To correct for evaporation, control disks were treated in an identical manner, but held in containers without crickets for the 4 h period. The amount of food lost to evaporation was converted to percent values which were then subtracted from the test disks after each experiment. All tests were video-taped for the entire four hours and adult cohorts (virgin females, mated females, egg laying females, and adult males pre-fed EAA and sucrose) were subsequently watched by trained observers blind to the treatment group and contents of each dish. The number of visits and the total amount of time crickets spent feeding on each dish were quantified for the first hour of each trial. A visit was recorded when a cricket’s mouthparts contacted food for at least 2 seconds, and time feeding was recorded when a cricket’s mouthparts were in contact with the food. Visits and time feeding by all five crickets were added giving an overall score for each measurement for each tank.
Mated females were acquired by introducing males to tanks housing only adult virgin female crickets (the number of each sex were equal) and allowing the animals to interact freely for 48 h. Under our conditions, males began courtship singing within 15 min of exposure to females and matings were observed within two hours. To acquire females allowed to lay eggs, males were removed after 48 h and a plastic dish (12 cm diameter x 6 cm high) of moistened soil was placed in the container housing the mated females. Females began laying eggs in the soil within 30 minutes and they had access to the soil containers for 24 h. Directly after the egg laying period, these females, mated females, and virgin females were placed in test containers, fasted, pre-fed either pure 0.5 M sucrose or 0.5 M sucrose/all EAA mix, and tested in the two-choice preference assay as described above.
Experiment 2: Extended amino acid deprivation and subsequent food preference
In experiment 2, adult male and virgin female crickets were tested under four different conditions: Group 1, 24 h EAA deprivation; Group 2, 48 h EAA deprivation; Group 3, 72 h EAA deprivation, and Group 4, six days EAA deprivation (Figure 1B). For all experiments, crickets were placed in test containers and fasted for 24 h. After this period, Group 1 received a choice of two food disks: pure 0.5 M sucrose and the mixture of 10 EAA. The food was measured and presented in the manner described above. For Groups 2, 3, and 4 sucrose pre-feeding only was used prior to the two-choice preference test. In Group 2, crickets were supplied with a disk of pure 0.5 M sucrose after the initial 24 h fast and allowed to feed for four hours. The following day, they were given a choice of pure sucrose or pure EAA as described for Group 1. Likewise Groups 3 and 4 received pure sucrose disks each day for 2 and 5 days respectively, and then tested the following day with the sucrose/EAA choice as described for Group 1. Each Group consisted of 16-18 containers (80-90 crickets/group) with equal numbers of males and females. All tests were videotaped for the entire four-hour period.
Experiment 3: Growth and survival in amino acid-deprived crickets
In experiment 3, survival of adult and juvenile crickets over a 30-day period was examined by supplying insects with one of three diets described above: control, holidic with all EAA, and holidic with no EAA. Crickets were housed in plastic tanks (19 x 28.5 x 23.5 cm high) equipped with an egg carton shelter and water. Each tank was supplied with a fresh disk of the assigned food every day. Juvenile crickets (N = 180, 60 per group) were in the 4th instar at the start of the experiment and were housed 10 per tank. Tanks were checked twice a day and dead bodies were immediately removed. This reduced, but did not completely eliminate, cannibalism among juveniles. Consequently, an additional experiment was conducted with similar 4th instar juveniles in isolate containers exposed to the control or no EAA diet (N = 20, 10 per group). To monitor growth, living juveniles were weighed at the start of the experiment and at the end of the 30-day feeding period. Adult crickets (N = 54, 18 per group) were separated by sex and housed three insects per tank.
Statistical analyses
Statistical analyses were conducted using SPSS with statistical significance set at alpha = 0.05. Preliminary analyses of food intake and behavior revealed significant deviations from normality and homogeneity of variance for data in some groups (Shapiro-Wilks test, P < 0.05; Levene’s test. P < 0.05) and several data sets included outliers. Hence we used nonparametric tests to evaluate differences within and among groups. For experiment 1, we initially focused on nutrient choice within each group and used Wilcoxon signed-rank tests to compare food consumption and feeding behavior following pre-feeding with sucrose or EAA. To compare EAA intake among groups, we used a non-parametric analysis of variance (Kruskal-Wallis test) and, where appropriate, conducted multiple comparisons with Dunn-Bonferroni tests. For experiment 2, we also used Kruskal-Wallis and post-hoc Dunn-Bonferroni tests to compare sucrose and EAA intake in male and female crickets subjected to four different periods of EAA deprivation. For experiment 3, Kaplan-Meier log-ranked tests were used to compare survival distributions in groups which received control diet or holidic diets with and without EAA.
Results
The agar test diets were well accepted by the crickets and only four tanks (two juvenile male, one juvenile female, one adult male) were eliminated due to lack of any food consumption. Figure 2 displays the amount of sucrose and EAA food consumed following pre-feeding with each substance (Experiment 1). In adult virgin females, the intake of EAA was significantly greater than sucrose following both sucrose (Z = -2.073, p = 0.038) and EAA pre-feeding (Z = -2.668, p = 0.008). In juvenile females, the intake of EAA was significantly greater than sucrose following pre-feeding with sucrose (Z = -2.366, p = 0.018). In contrast to these results, mated females significantly preferred sucrose following EAA pre-feeding (Z = -2.395, p = 0.017) and females that produced eggs significantly preferred sucrose following pre-feeding with EAA (Z = -2.803. p = 0.005) and sucrose (Z = -2.075, p = 0.035). Other within group comparisons found insignificant differences in sucrose and EAA intake regardless of preparation. Data on feeding behavior was consistent with the preferences noted above (Table 1). A preliminary analysis of 20 randomly selected trials found that most (M = 77%) feeding behavior occurred during the first hour and hence our subsequent analysis focused on this time period. Adult virgin females spent significantly more time feeding on EAA disks compared to sucrose disks after both EAA and sucrose pre-feeding, and visited EAA disks more times after EAA pre-feeding. Mated females spent significantly more time feeding on sucrose disks compared to EAA disks after EAA pre-feeding and visited sucrose disks more; results were similar for mated females allowed to lay eggs (Table 1). Despite significant differences in feeding time, visits, and food intake, each food was sampled multiple times suggesting that crickets chose to consume more sucrose or EAA after sampling both food types.
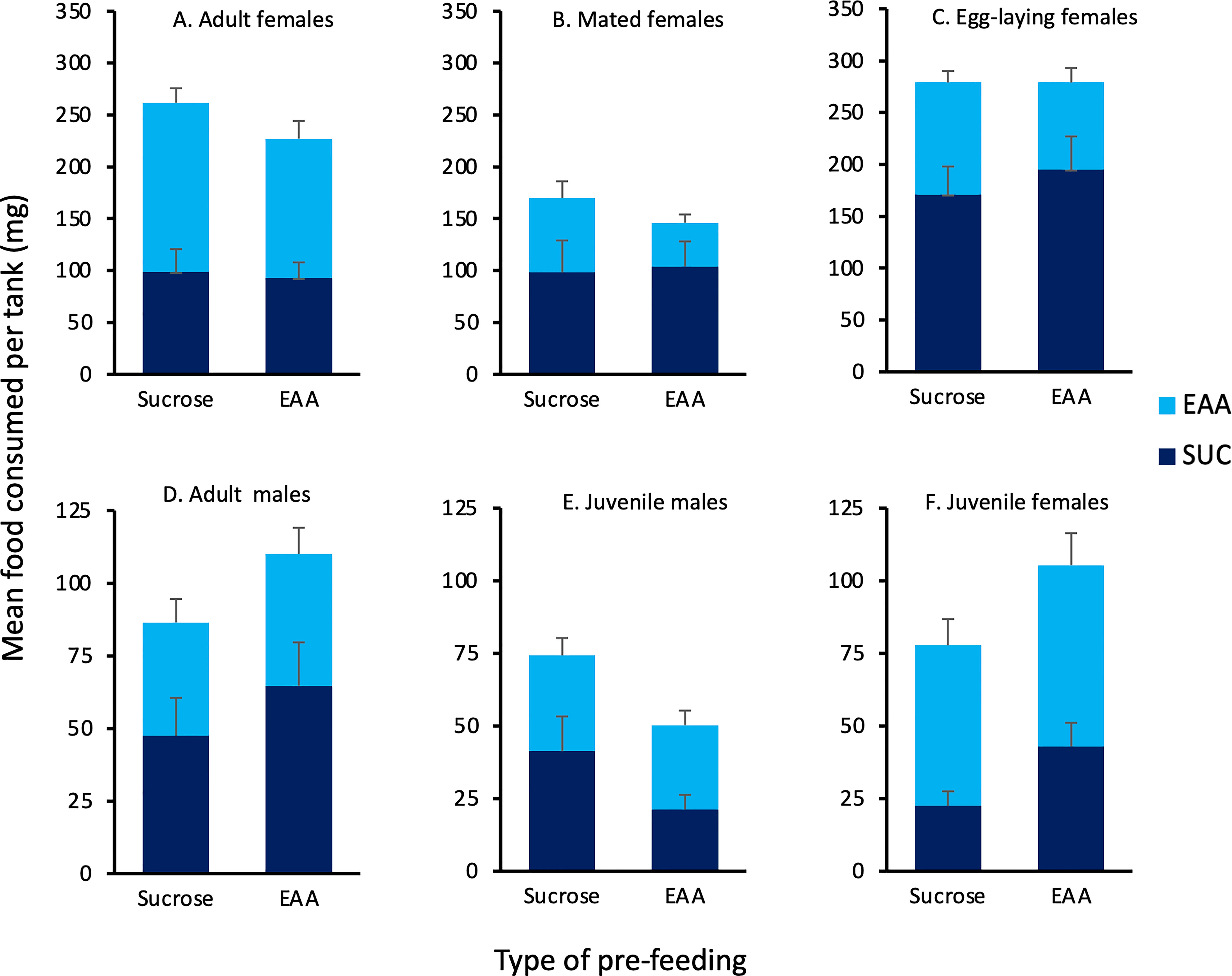
Figure 2 Effects of pre-feeding sucrose or EAA on subsequent consumption of these foods. Bars show the mean amount of sucrose (dark blue) and EAA (light blue) food consumed 24 h after pre-feeding with either sucrose or EAA in the following groups of house crickets: (A) Adult virgin females, (B) Mated females, (C) Egg-laying females, (D) Adult males, (E) Juvenile males, and (F) Juvenile females. See text for statistically significant differences.
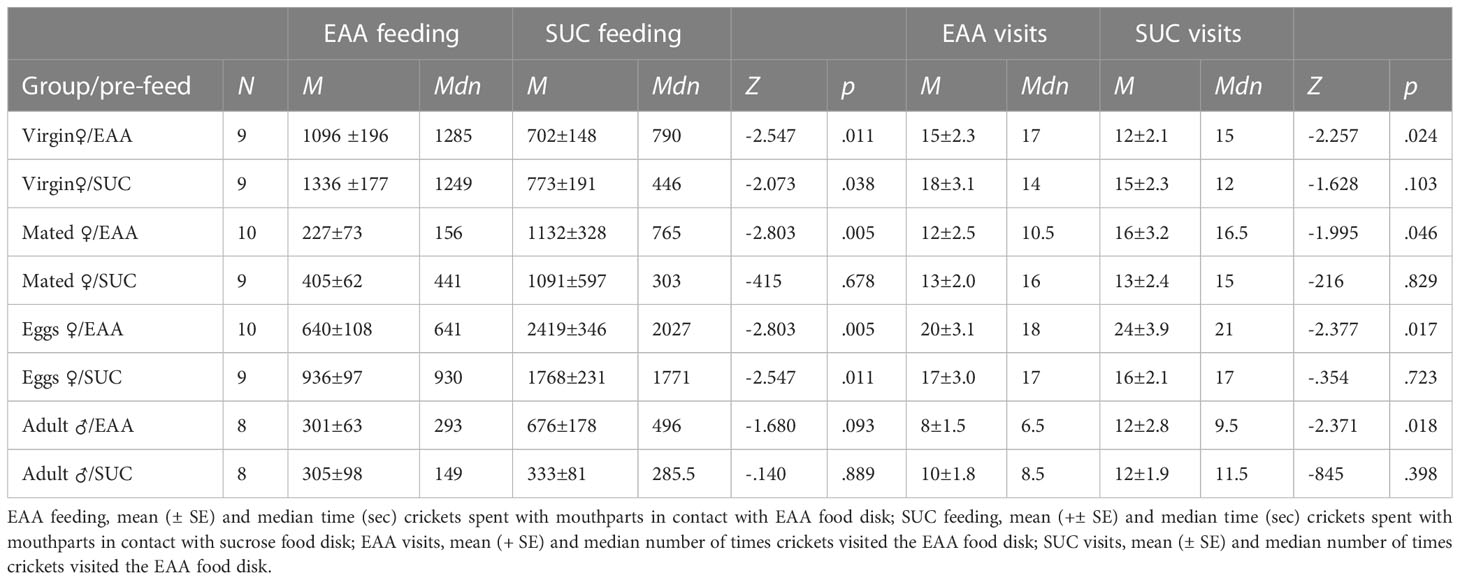
Table 1 Effects of pre-feeding sucrose or EAA on behavioral responses to each food type in adult virgin female, mated female, post-egg laying female (Eggs), and adult male crickets.
To examine EAA intake across groups, we compared the percent of EAA food consumed relative to total food intake in the six test groups (Figure 3). Following sucrose pre-feeding, there was no significant difference among groups in the percent of EAA food consumed (H(5) = 10.61, p = 0.06), though intake tended to be greater in adult and juvenile females. Following EAA pre-feeding, the percent of EAA intake differed significantly among groups (H(5) = 20.281, p = 0.001). Dunn-Bonferroni pairwise comparisons indicated that mated females post-egg laying consumed significantly less EAA food compared to both adult (p = 0.024) and juvenile females (p = 0.005). These data combined with the previous comparisons indicate that females differentiate between sucrose and EAA, and favor EAA consumption over sucrose prior to mating. However, this preference reverses after mating and egg-laying. Under the conditions of Experiment 1, juvenile and adult males did not significantly differ in sucrose and EAA food intake though both groups tended to prefer sucrose.
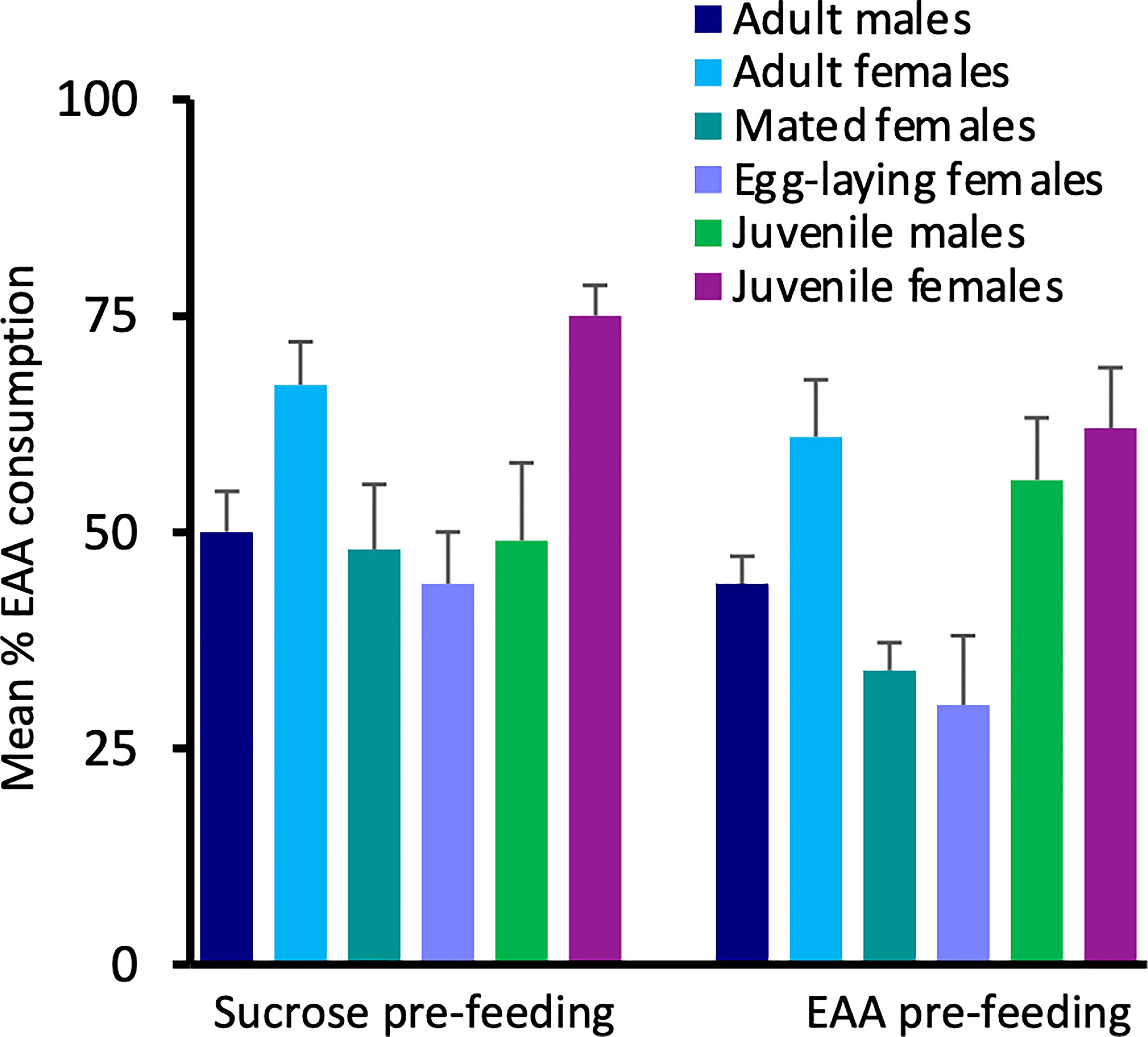
Figure 3 Effects of pre-feeding sucrose or EAA on the percent of EAA food consumed relative to total food consumption. Bars show percent EAA consumption in adult male, adult virgin female, mated female, egg-laying female, juvenile male, and juvenile female house crickets. See text for statistically significant differences.
For Experiment 2, consumption of EAA in adult males differed significantly among the four groups tested after 24 h, 48 h, 72 h, and six days of EAA deprivation (H(3) = 16.9, p = 0.001; Figure 4). We used Dunn-Bonferroni pairwise tests to compare nutrient intake among groups and found that, compared to Group 1 (24 h EAA deprivation), EAA consumption increased significantly in Group 3 (p = 0.039) and Group 4 (p = 0.001), but not Group 2 (p = 0.465). A concomitant decrease in sucrose intake occurred across the four different EAA deprivation periods (H(3) = 19.3, p = 0.001). Dunn-Bonferroni pairwise tests indicated that, compared to Group 1, sucrose consumption decreased significantly in Group 2 (p = 0.04), Group 3 (p = 0.001), and Group 4 (p = 0.001). Because the amount of food consumed varied among groups, we also considered the percent of EAA food consumed relative to total food intake in the four groups (Figure 5). This analysis confirmed the finding that EEA consumption differed among groups (H(3) = 23.8, p = 0.001) and, compared to Group 1, was significantly increased in Groups 3 (p = 0.001) and 4 (p = 0.001), but not Group 2 (p = 0.164). These data indicate that male crickets differentiate between sucrose and EAA, and after 72 h of EAA deprivation their preference switches from sucrose to EAA.
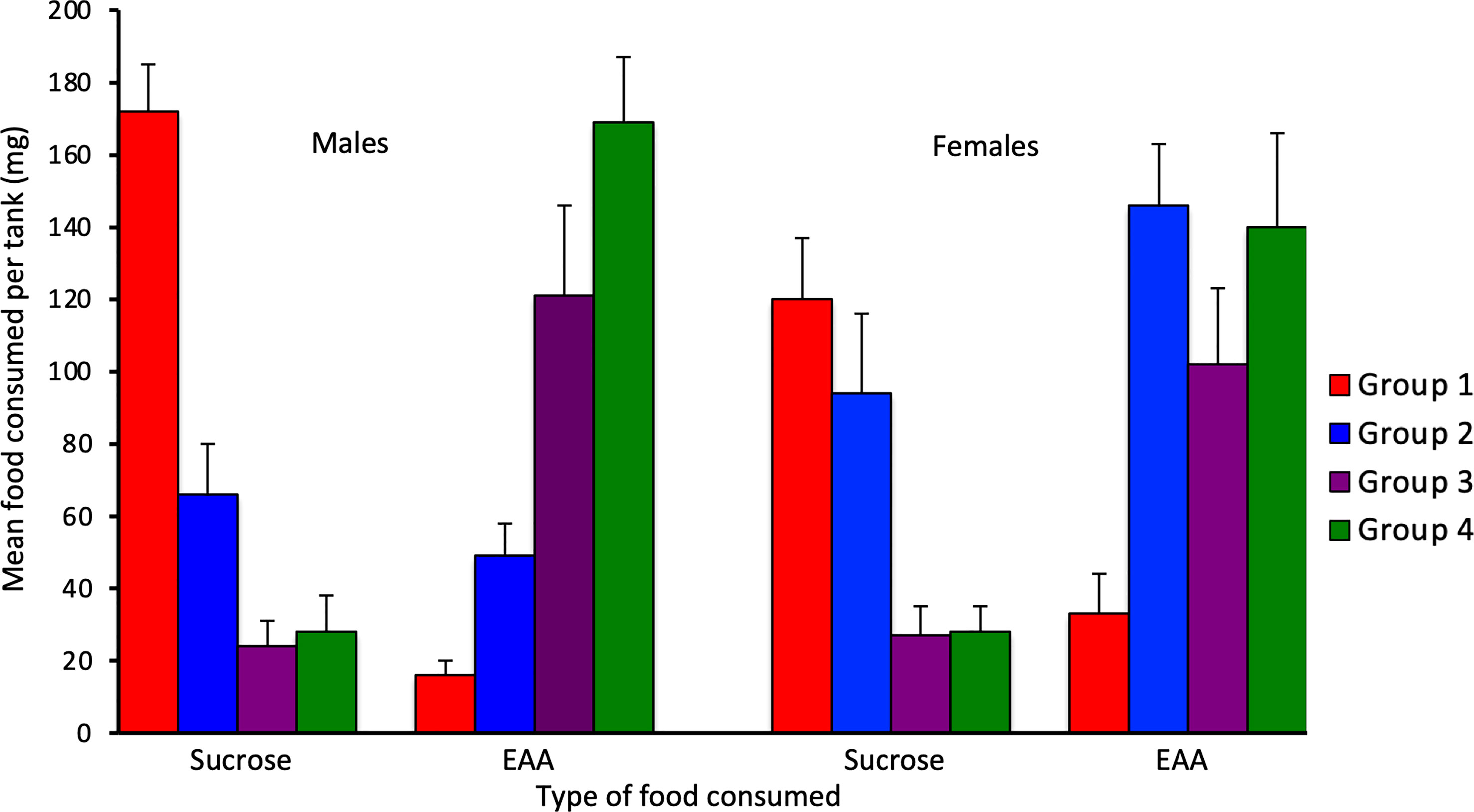
Figure 4 Effects of extended amino acid deprivation in adult male and adult virgin female crickets. Bars show the mean amount of sucrose and EAA consumed in Group 1 (24 h fast only), Group 2 (fed only sucrose for 48 h), Group 3 (fed only sucrose for 72 h) and Group 4 (fed only sucrose for six days). See text for statistically significant differences.
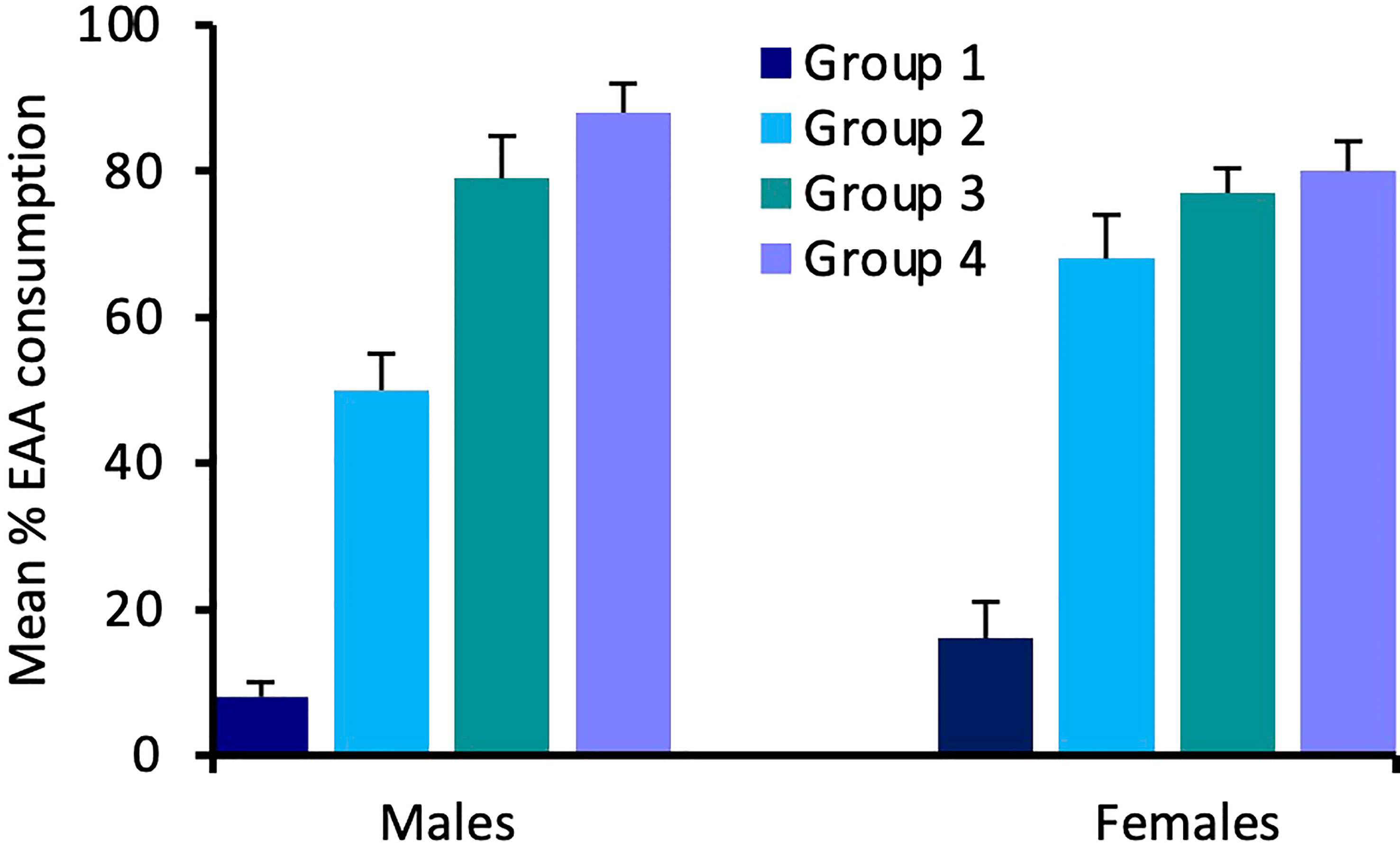
Figure 5 Effects of extended amino acid deprivation on the percent of EAA food consumed relative to total food consumption. Bars show percent EAA consumption in adult male and female crickets in Group 1 (24 h fast only), Group 2 (fed only sucrose for 48 h), Group 3 (fed only sucrose for 72 h) and Group 4 (fed only sucrose for six days). See text for statistically significant differences.
In adult females, consumption of EAA (H(3) = 13.9, p = 0.003) and sucrose (H(3) = 14.6, p = 0.002) also differed significantly among the four groups (Figure 4). For EAA consumption, Dunn-Bonferroni tests indicated that, compared to Group 1, EAA consumption increased significantly in Group 2 (p = 0.003) and Group 4 (p = 0.027), but not Group 3 (p = 0.357). For sucrose, compared to Group 1, consumption decreased in Group 3 (p = 0.011) and Group 4 (p = 0.016), but not Group 2 (p = 0.786). A comparison of the percent of EAA food consumed relative to total food intake revealed significant differences among groups (H(3) = 18.3, p = 0.001) and significant increases in EAA intake in Groups 2 (p = 0.032), 3 (p = 0.003), and 4 (p = 0.001), relative to Group 1 (Figure 5). These data support the previous finding that females differentiate between sucrose and EAA and they switch their preference from sucrose to EAA after 48 h of EAA deprivation.
In Experiment 3, the overall survival of juvenile crickets in community tanks differed significantly among the three diets (log-rank test: X2 = 61.4, p < 0.001, Figure 6A). Pairwise log-rank comparisons indicated that survival in the control diet differed significantly from the EAA diet (X2 = 26.8, p < 0.001) and the No EAA diet (X2 = 63.9, p < 0.001); also, the EAA and No EAA diets differed significantly in juvenile survival (X2 = 10.5, p = 0.001). Crickets fed the control diet had a 98% survival rate, an average increase in weight of 320 mg/cricket, and 78% (47 insects) had molted to adulthood by the end of the 30-day period. Crickets fed the artificial diet with EAA or No EAA had survival rates of 60% and 30%, respectively. However, growth was severely restricted in both groups (average weight increase in EAA group: 21 mg/cricket; No EAA group: 14 mg/cricket) and no insects molted to adulthood. These data indicate that, while both artificial diets limited growth, access to EAA significantly enhanced survival. For juvenile crickets held individually, survival was significantly greater in the control group compared to the crickets in the No EAA group (log rank test: X2 = 9.8, p < 0.002, Figure 6B). All juveniles fed the control diet survived and molted to adulthood during the 30-day period, whereas 60% of the crickets fed the No EAA diet survived and growth was restricted (average weight increase over 30 days was 15 mg/cricket). These data confirm the superior performance of juveniles fed control food and suggest that reduced survivorship in the community tanks fed the No EAA diet may have been due in part to greater levels of cannibalism. All adult crickets of both sexes survived for 30 days when fed the control, EAA, or No EAA diet, indicating that the three diets were able to sustain life for this time period.
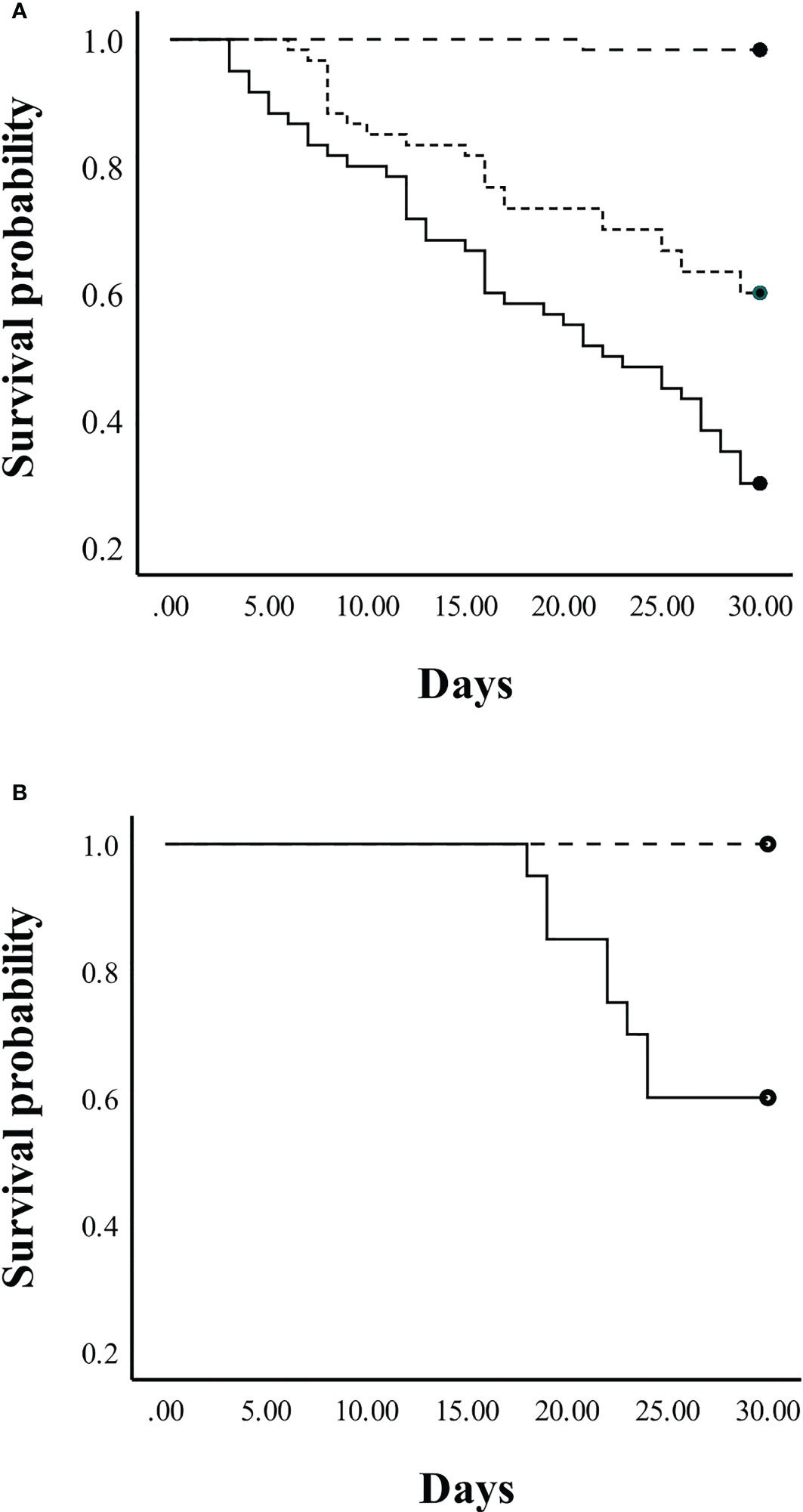
Figure 6 Survival probability of juvenile crickets fed a control diet (dashed line) or a holidic diet with EAA (dotted line) or without EAA (solid line) for 30 days. (A) Survival probability of juvenile crickets housed in same-sex community tanks. (B) Survival probability of juvenile crickets held in isolate tanks. See text for statistically significant differences.
Discussion
The present results contribute several novel findings about nutrient selection in the house cricket. First, we found that house crickets can distinguish EAA from sucrose and that selection of these two nutrients is affected by prior feeding experience. We also found that age and reproductive state affected food choice, with juvenile and virgin females showing a greater preference for EAA than juvenile or adult males. Contrary to previous studies, mated females preferred sucrose over EAA both prior to and after egg laying. We also found that crickets of both sexes increased their intake of EAA when exposed to longer periods of deprivation, suggesting that they engage in compensatory feeding on these nutrients. Finally, we found that growth was severely limited in juveniles fed a diet lacking all amino acids. However, many juveniles and all adults survived on this diet for 30 days indicating that they possess considerable resilience to dietary restriction.
Our results support previous studies which have found that insects respond behaviorally to free amino acids when making feeding decisions. These chemicals may serve as attractants or deterrents in nectar feeders, allowing insects to gain needed nutrients while avoiding excessive amino acid intake (20, 22, 23). In Drosophila, adaptive responses to amino acids may likewise allow larvae (39) and adults to acquire an appropriate balance of these nutrients and respond to deprivation with enhanced amino acid preference. House crickets are highly generalized feeders and are likely to occur in areas where intensive farming creates environments with nutritional imbalances (40). Hence, they may encounter foods that vary in the quantity and quality of amino acids or where abundant food sources lack EAA. Dietary challenges may be exacerbated by the tendency of house crickets to aggregate (41), causing social stress and competition for food (42, 43). Hence the ability to detect EAA and compensate for deprivation is likely to be especially adaptive in these insects. The site of amino acid perception has not yet been established in crickets, but in other insects individual amino acids can be detected by taste sensilla on the legs (18, 44) and labellum (45, 46). Recent studies have also identified molecular receptors for amino acids in Drosophila including the odorant binding protein OBP19b (47), the ionotropic receptor Ir76b (18, 39), and several additional ionotropic and gustatory receptors (46). Comparative research is needed to determine if these mechanisms are conserved across other insect species.
In experiment 1, we found that after 48 h EAA deprivation, juvenile and adult virgin females chose to consume more EAA than sucrose; however, juvenile and adult males did not display a preference for EAA under the same conditions. When protein is limited, free EAA are a source of required nutrients and the attraction of females to EAA is consistent with the tendency of female crickets to consume more protein than males (31). Oogenesis in house crickets begins on day three following adult ecdysis and oviducts are full by day 15 (48), the period of time during which we tested virgin females. Hence the need to produce eggs may drive EAA appetite in females just prior to and after the adult ecdysis. The preference for sucrose after mating and egg laying in female house crickets contrasts with findings in Drosophila (15, 33) and two-spotted crickets (34) in which protein ingestion increased after mating. Our results possibly reflect motivation to replenish energy via carbohydrate feeding immediately following periods of heightened physical activity and decreased food intake which occur during mating and egg laying (48). In future studies, it would be useful to examine food preferences throughout adulthood to better understand the associations between protein or EAA ingestion and oogenesis, mating, and oviposition.
In male crickets, sucrose pre-feeding for 72 h and 6 days induced a switch from sucrose to EAA preference (Experiment 2). Virgin females also consumed more sucrose after a 24 h fast, but consistent with the results of Experiment 1, preferred EAA after 48 h deprivation and one sucrose pre-feeding. These results indicate that both sexes perceive and compensate for EAA deficiency. However, under our experimental conditions, house crickets did so only after a relatively long period of deprivation whereas some other species displayed compensatory selection of carbohydrate and protein after dietary restrictions lasting only a few hours (12, 13). The results of the survival study (Experiment 3) are interesting in this context since they indicate that adult crickets displayed resilience in the face of EAA deprivation given that 100% of adults of both sexes survived for 30 days on sucrose alone. This suggests that, as an omnivorous and opportunistic feeder, adult house crickets have evolved to tolerate temporary nutritional imbalances and may not be under strong selective pressure to very rapidly compensate for dips in EAA availability. The situation is different for juvenile stages since we found that growth and survival were significantly impacted by lack of EAA in 4th instar nymphs. We did not assess dietary choice in early cricket instars, but because optimal growth depends on EAA availability it is possible that a compensatory response to EAA deprivation would occur sooner in juveniles relative to adults.
Many additional discoveries remain to be made about cricket diet selection. Further investigations of feeding behavior at different life stages would contribute additional information on the adjustments insects make to optimize nutritional balance as they mature and reproduce (3). In addition, other major behavioral events such as aggressive interactions and courtship singing may trigger changes in nutrient selection, a possibility that remains to be examined in crickets and other species. Another topic of interest concerns the perception and selection of individual amino acids. Previous work has shown that some insects distinguish among single amino acids; for example, Drosophila larvae are most attracted aspartic acid and avoid leucine (19, 39), and honey bees are more attracted to essential amino acids, especially phenylalanine, compared to nonessential amino acids (23). Such information is not yet available in crickets and would be useful for comparative purposes and as a foundation for future physiological studies. The latter are important since the neural mechanisms used by crickets to sense amino acids, both externally and internally, are not yet elucidated. Finally, amino acid appetite may differ between insects from inbred laboratory colonies and their wild counterparts. For example, in the laboratory locusts regulate nutrient intake in a consistent manner, whereas wild locusts display considerable plasticity in their feeding behavior across generations and seasons (49). Also, gut microbial communities differ between wild crickets and those confined and fed a laboratory diet, a factor that may affect how amino acids are synthesized and degraded (50, 51). Our study examined amino acid appetite in laboratory animals fed a commercial cricket diet and more general conclusions could be drawn by extending the experiments to wild populations.
Data availability statement
The raw data supporting the conclusions of this article will be made available by the authors, without undue reservation.
Author contributions
AT designed the study, ran experiments, analyzed data, and wrote the original draft of the manuscript. EV, LJ, SH, MP, and MS contributed to data collection and analysis. All authors contributed to the article and approved the submitted version.
Funding
We thank the Colgate Research Council and Colgate University Division of Natural Sciences for contributing funding for this project.
Conflict of interest
The authors declare that the research was conducted in the absence of any commercial or financial relationships that could be construed as a potential conflict of interest.
Publisher’s note
All claims expressed in this article are solely those of the authors and do not necessarily represent those of their affiliated organizations, or those of the publisher, the editors and the reviewers. Any product that may be evaluated in this article, or claim that may be made by its manufacturer, is not guaranteed or endorsed by the publisher.
References
1. Behmer ST. Insect herbivore nutrient regulation. Annu Rev Entomol. (2009) 54:165–87. doi: 10.1146/annurev.ento.54.110807.090537
2. Waldbauer GP, Cohen RW, Friedman S. Self-selection of an optimal nutrient mix from defined diets by larvae of the corn earworm, Heliothis zea (Boddie). Physiol Zool. (1984) 57(6):590–7. doi: 10.1086/physzool.57.6.30155985
3. Cohen RW, Heydon SL, Waldbauer GP, Friedman S. Nutrient self-selection by the omnivorous cockroach Supella longipalpa. J Insect Physiol (1987) 33(2):77–82. doi: 10.1016/0022-1910(87)90077-1
4. Chambers PG, Simpson SJ, Raubenheimer D. Behavioural mechanisms of nutrient balancing in Locusta migratoria nymphs. Anim Behav (1995) 50:1513–23. doi: 10.1016/0003-3472(95)80007-7
5. Jones SA, Raubenheimer D. Nutritional regulation in nymphs of the German cockroach, Blattella germanica. J Insect Physiol (2001) 47(10):1169–80. doi: 10.1016/S0022-1910(01)00098-1
6. Raubenheimer D, Simpson SJ. Nutrient balancing in grasshoppers: Behavioural and physiological correlates of dietary breadth. J Exp Biol (2003) 206(10):1669–81. doi: 10.1242/jeb.00336
7. Rho MS, Lee KP. Geometric analysis of nutrient balancing in the mealworm beetle, Tenebrio molitor l. (Coleoptera: Tenebrionidae). J Insect Physiol (2014) 71:37–45. doi: 10.1016/j.jinsphys.2014.10.001
8. Wang P, Furlong MJ, Walsh TK, Zalucki MP. Moving to keep fit: feeding behavior and movement of Helicoverpa armigera (Lepidoptera: Noctuidae) on artificial diet with different protein: Carbohydrate ratios. J Insect Sci (2019) 19(5):20. doi: 10.1093/jisesa/iez098
9. Rho MS, Lee KP. Behavioural and physiological regulation of protein and carbohydrates in mealworm larvae: A geometric analysis. J Insect Physiol (2022) 136:104329. doi: 10.1016/j.jinsphys.2021.104329
10. Yang Y, Joern A. Compensatory feeding in response to variable food quality by Melanoplus differentialis. Physiol Entomol. (1994) 19(1):75–82. doi: 10.1111/j.1365-3032.1994.tb01077.x
11. Vaudo AD, Patch HM, Mortensen DA, Tooker JF, Grozinger CM. Macronutrient ratios in pollen shape bumble bee (Bombus impatiens) foraging strategies and floral preferences. Proc Natl Acad Sci (2016) 113(28):E4035–42. doi: 10.1073/pnas.1606101113
12. Simpson S, Simmonds M, Blaney W, Jones J. Compensatory dietary selection occurs in larval Locusta migratoria but not Spodoptera littoralis after a single deficient meal during ad libitum feeding. Physiol Entomol. (1990) 15(2):235–42. doi: 10.1111/j.1365-3032.1990.tb00511.x
13. Simmonds M, Simpson S, Blaney W. Dietary selection behavior in Spodoptera littoralis–the effects of conditioning diet and conditioning period on neural responsiveness and selection behavior. J Exp Biol (1992) 162:73–90. doi: 10.1242/jeb.162.1.73
14. Raubenheimer D, Jones SA. Nutritional imbalance in an extreme generalist omnivore: Tolerance and recovery through complementary food selection. Anim Behav (2006) 71:1253–62. doi: 10.1016/j.anbehav.2005.07.024
15. Ribeiro C, Dickson BJ. Sex peptide receptor and neuronal TOR/S6K signaling modulate nutrient balancing in Drosophila. Curr Biol.: CB (2010) 20(11):1000–5. doi: 10.1016/j.cub.2010.03.061
16. Ganguly A, Dey M, Scott C, Duong V-K, Arun Dahanukar A. Dietary macronutrient imbalances lead to compensatory changes in peripheral taste via independent signaling pathways. J Neurosci (2021) 41(50):10222–46. doi: 10.1523/JNEUROSCI.2154-20.2021
17. Toshima N, Tanimura T. Taste preference for amino acids is dependent on internal nutritional state in Drosophila melanogaster. J Exp Biol (2012) 215(Pt 16):2827–32. doi: 10.1242/jeb.069146
18. Ganguly A, Pang L, Duong V-K, Lee A, Schoniger H, Varady E, et al. A molecular and cellular context-dependent role for Ir76b in detection of amino acid taste. Cell Rep (2017) 18(3):737–50. doi: 10.1016/j.celrep.2016.12.071
19. Kudow N, Miura D, Schleyer M, Toshima N, Gerber B, Tanimura T. Preference for and learning of amino acids in larval Drosophila. Biol Open (2017) 6(3):365–9. doi: 10.1242/bio.020412
20. Inouye D, Waller G. Responses of honey bees (Apis mellifera) to amino acid solutions mimicking floral nectars. Ecology (1984) 65(2):618–25. doi: 10.2307/1941424
21. Alm J, Ohnmeiss TE, Lanza J, Vriesenga L. Preference of cabbage white butterflies and honey bees for nectar that contains amino acids. Oecologia (1990) 84(1):53–7. doi: 10.1007/BF00665594
22. Blüthgen N, Fiedler K. Preferences for sugars and amino acids and their conditionality in a diverse nectar-feeding ant community. J Anim Ecol (2004) 73(1):155–66. doi: 10.1111/j.1365-2656.2004.00789.x
23. Hendriksma HP, Oxman KL, Shafir S. Amino acid and carbohydrate tradeoffs by honey bee nectar foragers and their implications for plant-pollinator interactions. J Insect Physiol (2014) 69:56–64. doi: 10.1016/j.jinsphys.2014.05.025
24. Csata E, Gautrais J, Bach A, Blanchet J, Ferrante J, Fournier F, et al. Ant foragers compensate for the nutritional deficiencies in the colony. Curr Biol.: CB (2020) 30(1):135–142.e4. doi: 10.1016/j.cub.2019.11.019
25. Ignell R, Okawa S, Englund J-E, Hill SR. Assessment of diet choice by the yellow fever mosquito Aedes aegypti. Physiol Entomol. (2010) 35(3):274–86. doi: 10.1111/j.1365-3032.2010.00740.x
26. Bjordal M, Arquier N, Kniazeff J, Pin JP, Léopold P. Sensing of amino acids in a dopaminergic circuitry promotes rejection of an incomplete diet in Drosophila. Cell (2014) 156(3):510–21. doi: 10.1016/j.cell.2013.12.024
27. Corrales-Carvajal VM, Faisal AA, Ribeiro C. Internal states drive nutrient homeostasis by modulating exploration-exploitation trade-off. ELife (2016) 5:e19920. doi: 10.7554/eLife.19920
28. Leitão-Gonçalves R, Carvalho-Santos Z, Francisco AP, Fioreze GT, Anjos M, Baltazar C, et al. Commensal bacteria and essential amino acids control food choice behavior and reproduction. PloS Biol (2017) 15(4):e2000862. doi: 10.1371/journal.pbio.2000862
29. Toshima N, Schleyer M. Neuronal processing of amino acids in Drosophila: From taste sensing to behavioural regulation. Curr Opin Insect Sci (2019) 36:39–44. doi: 10.1016/j.cois.2019.07.007
30. Schwarz S, Durisko Z, Dukas R. Food selection in larval fruit flies: Dynamics and effects on larval development. Die Naturwissenschaften (2014) 101(1):61–8. doi: 10.1007/s00114-013-1129-z
31. Harrison SJ, Raubenheimer D, Simpson SJ, Godin J-GJ, Bertram SM. Towards a synthesis of frameworks in nutritional ecology: Interacting effects of protein, carbohydrate and phosphorus on field cricket fitness. Proc Biol Sci (2014) 281(1792):20140539. doi: 10.1098/rspb.2014.0539
32. Fukumura K, Nagata S. Behavioral tracing demonstrates dietary nutrient discrimination in two-spotted crickets Gryllus bimaculatus. Biosci. Biotechnol. Biochem (2017) 81(10):1990–3. doi: 10.1080/09168451.2017.1343119
33. Vargas MA, Luo N, Yamaguchi A, Kapahi P. A role for S6 kinase and serotonin in postmating dietary switch and balance of nutrients in D. melanogaster. Curr Biol.: CB (2010) 20(11):1006–11. doi: 10.1016/j.cub.2010.04.009
34. Tsukamoto Y, Kataoka H, Nagasawa H, Nagata S. Mating changes the female dietary preference in the two-spotted cricket, Gryllus bimaculatus. Front Physiol (2014) 5:95. doi: 10.3389/fphys.2014.00095
35. Morales-Ramos JA, Rojas MG, Dossey AT, Berhow M. Self-selection of food ingredients and agricultural by-products by the house cricket, Acheta domesticus (Orthoptera: Gryllidae): A holistic approach to develop optimized diets. PloS One (2020) 15(1):e0227400. doi: 10.1371/journal.pone.0227400
36. Paoli PP, Donley D, Stabler D, Saseendranath A, Nicolson SW, Simpson SJ, et al. Nutritional balance of essential amino acids and carbohydrates of the adult worker honeybee depends on age. Amino Acids (2014) 46(6):1449–58. doi: 10.1007/s00726-014-1706-2
37. Stabler D, Paoli PP, Nicolson SW, Wright GA. Nutrient balancing of the adult worker bumblebee (Bombus terrestris) depends on the dietary source of essential amino acids. J Exp Biol (2015) 218(Pt 5):793–802. doi: 10.1242/jeb.114249
38. Simpson S, Abisgold J. Compensation by locusts for changes in dietary nutrients–behavioral mechanisms. Physiol Entomol. (1985) 10(4):443–52. doi: 10.1111/j.1365-3032.1985.tb00066.x
39. Croset V, Schleyer M, Arguello JR, Gerber B, Benton R. A molecular and neuronal basis for amino acid sensing in the Drosophila larva. Sci Rep (2016) 6:34871. doi: 10.1038/srep34871
40. Ismail I. Biological and ecological studies on house cricket, Acheta domesticus l (Orthopt, gryllidae) in giza region, Egypt. Z Fur Angewandte Entomologie-Journal Appl Entomol. (1978) 85(3):230–5. doi: 10.1111/j.1439-0418.1978.tb04035.x
41. Mcfarlane J, Steeves E, Alli I. Aggregation of larvae of the house cricket, acheta domesticus (l), by propionic acid present in the excreta. J Chem Ecol (1983) 9(9):1307–15. doi: 10.1007/BF00994799
42. Nosil P. Food fights in house crickets, Acheta domesticus, and the effects of body size and hunger level. Can J Zool. (2002) 80(3):409–17. doi: 10.1139/Z02-018
43. Gutiérrez Y, Fresch M, Ott D, Brockmeyer J, Scherber C. Diet composition and social environment determine food consumption, phenotype and fecundity in an omnivorous insect. R Soc Open Sci (2020) 7(4):200100. doi: 10.1098/rsos.200100
44. Van Der WM, Van Naters G, Den Otter CJ. Amino acids as taste stimuli for tsetse flies. Physiol Entomol. (1998) 23:278–84. doi: 10.1046/j.1365-3032.1998.233083.x
45. Park J, Carlson JR. Physiological responses of the drosophila labellum to amino acids. J Neurogenet. (2018) 32(1):27–36. doi: 10.1080/01677063.2017.1406934
46. Aryal B, Dhakal S, Shrestha B, Lee Y. Molecular and neuronal mechanisms for amino acid taste perception in the Drosophila labellum. Curr Biol (2022) 32(6):1376–1386.e4. doi: 10.1016/j.cub.2022.01.060
47. Rihani K, Fraichard S, Chauvel I, Poirier N, Delompré T, Neiers F, et al. A conserved odorant binding protein is required for essential amino acid detection in Drosophila. Commun Biol (2019) 2:425. doi: 10.1038/s42003-019-0673-2
48. Clifford CW. The biology of egg production in the house cricket, Acheta domesticus L." (1985). LSU Historical Dissertations and Theses. 4046. Available at: https://digitalcommons.lsu.edu/gradschool_disstheses/4046
49. Le Gall M, Beye A, Diallo M, Cease AJ. Generational variation in nutrient regulation for an outbreaking herbivore. Oikos (2022) 2022(7):1–15. doi: 10.1111/oik.09096
50. Smith CC, Srygley RB, Healy F, Swaminath K, Mueller UG. Spatial structure of the Mormon cricket gut microbiome and its predicted contribution to nutrition and immune function. Front Microbiol (2017) 8:801. doi: 10.3389/fmicb.2017.00801
Keywords: insect, cricket, amino acid, diet, feeding behavior
Citation: Tierney AJ, Velazquez E, Johnson L, Hiranandani S, Pauly M and Souvignier M (2023) Nutritional and reproductive status affect amino acid appetite in house crickets (Acheta domesticus). Front. Insect Sci. 3:1120413. doi: 10.3389/finsc.2023.1120413
Received: 09 December 2022; Accepted: 17 March 2023;
Published: 04 April 2023.
Edited by:
Marion Le Gall, Arizona State University, United StatesReviewed by:
Sampat Ghosh, Andong National University, Republic of KoreaShinji Nagata, The University of Tokyo, Japan
Copyright © 2023 Tierney, Velazquez, Johnson, Hiranandani, Pauly and Souvignier. This is an open-access article distributed under the terms of the Creative Commons Attribution License (CC BY). The use, distribution or reproduction in other forums is permitted, provided the original author(s) and the copyright owner(s) are credited and that the original publication in this journal is cited, in accordance with accepted academic practice. No use, distribution or reproduction is permitted which does not comply with these terms.
*Correspondence: Ann Jane Tierney, YXRpZXJuZXlAY29sZ2F0ZS5lZHU=