- 1School of Agricultural Sciences, Department of Plant Science, University of Zambia, Lusaka, Zambia
- 2Department of Plant health, International Centre of Insect Physiology and Ecology, Nairobi, Kenya
- 3Plant Protection Division, Zambia Agriculture Research Institute, Mount Makulu Research Station, Lusaka, Zambia
The fall armyworm (FAW), Spodoptera frugiperda, an invasive pest originating from the Americas is a serious pest threatening cereal production and food security in Zambia. We studied the prevalence and abundance of natural enemies of FAW in three Agroecological regions (AERs I, II, and III) to identify those that could potentially serve as bio-control agents. Sampling of FAW parasitoids and predators was done along trunk roads at intervals of 10 km. Molecular sequence analysis and morphological characterization were used to identify natural enemies. Over 11 species of FAW natural enemies, including egg, egg-larval, and larval parasitoids, and predators, were identified in Zambia. The mean number of natural enemies and species richness was higher in AER I and IIa. Consequently, egg parasitism was highest in those two regions, at 24.5% and 12.2%, respectively. Larvae parasitism was highest in AER I (4.8%) and AER III (1.9), although no significant differences were observed. The most abundant and widely distributed parasitoid was Drino sp. (Diptera: Tachinidae), while Rhynocoris segmentarius (Germar) (Hemiptera: Reduviidae) and Belanogaster sp. (Hymenoptera: Vespidae) were the most prevalent predators. Our study reveals the presence of two natural enemies belonging to the genus Tiphia and Micromeriella, uncommon to FAW. Significant differences in the number of parasitoids were observed in polycropping, with the highest recovery of 12 ± 10% from maize + cowpeas + pumpkin and watermelon mixed cropping. The higher the rainfall, the lower the number of natural enemies recorded. Variations in rainfall patterns which affect FAW availability, cropping systems and the three AERs may explain natural enemies’ species diversity in Zambia. The information provided in this study can aid the development of a national biological control programme for sustainable management of fall armyworm.
1 Introduction
In Sub-Saharan Africa (SSA), maize is the most important crop (1), but its productivity is constrained by many lepidopteran insect pests such as stemborers (2). The arrival of fall armyworm (FAW) (Spodoptera frugiperda, J. E.Smith (Insecta: Lepidoptera: Noctuidae), exacerbated the food security threat at household and national levels in Zambia. FAW is an invasive species that originated in sub-tropical and tropical regions of the Americas (3, 4). FAW causes significant maize yield losses, ranging from 11.5 to 73% under severe infestation (5, 6). In Brazil alone, costs to control the FAW in maize have exceeded 600 million USD annually (7). The pest is polyphagous and has approximately 353 host plant species (8), including cultivated crops (9, 10), however, it prefers plants from the family Poaceae, maize especially. The female produces up to 2000 eggs and can have 10–12 generations per year (11). In Africa, the FAW was first reported in January 2016 in Nigeria (12, 13). In Zambia, the pest was reported in Chirundu in November of the same year, from where it spread to all ten provinces, infesting and causing damage to maize within three months (14).
The management of Spodoptera species in Zambia is mainly through use of synthetic pesticides. The Government of the Republic of Zambia adopted emergency measures and spent over $3million on the procurement of insecticides, personal protective suits, equipment, early maturing maize varieties, and distribution of requisites to various districts in one year (15, 16). However, synthetic pesticides are generally non-selective, hazardous to the applicator, and highly toxic to natural enemies of the target species (17–19).
Control strategies, such as push–pull (20), maize-legume intercropping (21), microbial control (22), and augmentative biological control strategies (23), are being developed and implemented for the management of FAW. These strategies enhance crop performance and ecosystem services, such as the regulation of pest populations by natural enemies (24). Therefore, the sustainable management of FAW requires an integrated approach that is mindful of natural processes.
Natural enemies, especially parasitoids and predators, are critical components of Integrated Pest Management (IPM). IPM is considered a more sustainable approach for pest control as it relies on the most economical means and with the least hazard to humans and the environment. Although FAW is an invasive pest in Africa, several new associations of the pest with natural enemies already prevalent in Africa have been reported from East Africa (25). Regarding Southern Africa, Durocher-Granger et al. (26) reported the presence in Zambia of Metopius discolour Tosquinet, Chelonus bifoveolatus Szépligeti (Hymenoptera: Braconidae), Coccygidium luteum Brullé (Hymenoptera: Braconidae), Chelonus curvimaculatus Cameron (Hymenoptera: Braconidae), Drino quadrizonula Thomson (Diptera: Tachinidae) Charops sp., Cotesia icipe Fernández-Triana and Fiaboe (Hymenoptera: Braconidae), Euplectrus laphygmae Ferrière (Hymenoptera: Eulophidae), Parapanteles sp., Diadegma sp., Pristomerus sp. and Enicospilus capensis Thunberg (Hymenoptera: Ichneumonoidea). In Mozambique, Caniço et al. (27) reported the occurrence of C. luteum, Charops sp., Metopius cf. discolour Tosquinet (Hymenoptera: Ichneumonidae) and D. quadrizonula. Understanding the abundance and diversity of indigenous FAW natural enemies could facilitate the development of augmentative and conservation biological control programs in Zambia.
Surveys conducted in four locations in Lusaka and Central provinces in Zambia highlighted the new association of existing natural enemies of FAW (26). A more comprehensive assessment of FAW natural enemies in diverse Agroecological regions (AERs) in Zambia is of paramount importance for developing and implementing a robust IPM package to minimize the misuse of chemical pesticides and protect food security. Therefore, the objective of the study was to assess the abundance and diversity of indigenous natural enemies of FAW in AERs I, IIa and III of Zambia and to identify candidates that would serve as biological control agents.
2 Materials and methods
2.1 Study area
Zambia is divided into three mains AERs, and the survey for the natural enemies of FAW was conducted in AERs I, IIa and III (Figure 1 and Table 1). The areas and sites in all the AERs were selected based on reports of FAW occurrence (15). Agroecological region I receives below 800 mm of rainfall per year, with mean daily temperatures ranging from 20 °C to 25 °C during the rainy season and up to 38 °C in the dry, hot season. Agroecological region II experiences rainfall ranging from 800 to 1000 mm and a mean daily temperature range from 20 to 23°C up to 25 °C during the rainy season, and AER III receives between 1000 and 1500 mm annually with a mean daily temperature of 16 °C in the rainy season and 18 °C in the dry season (28).
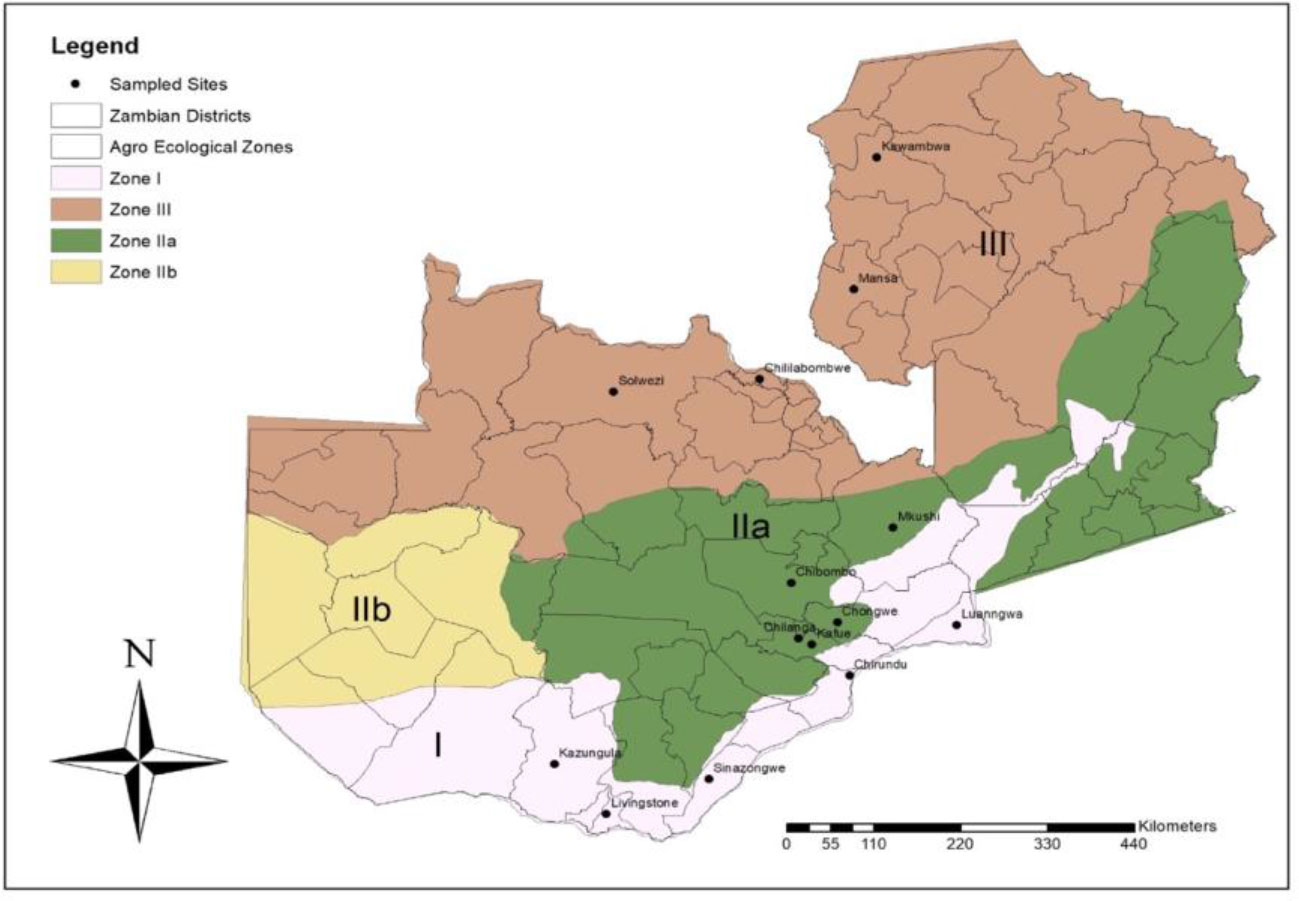
Figure 1 Map showing Agroecological regions and districts surveyed for natural enemies of fall armyworm in Zambia between March and May 2019.
In AER I, Luangwa, Chirundu, and Sinazongwe have loamy and clay soils, with coarse to fine loam top soils that are slightly acidic to alkaline. The soils have good soil moisture holding characteristics. Kazungula and Livingstone have reddish course soils with medium acidity. The main vegetation in this region is Mopani, with an open deciduous canopy. It is dominated by Colophospermum mopane (28, 29) (Table 1).
Agroecological region II is dominated by sandy, acidic soils that have low nutrient reserves and poor water retention when compared to AER I. These soils dry up easily during dry spells and are prone to leaching in the event of heavy rainfall. Agroecological region II is subdivided into sub-region IIa comprising of the degradation Sandveld plateau, and sub-region IIb comprising the degradation Kalahari sand plateau and Zambezi flood plain in the Western province (30). The vegetation in AER II is dominated by miombo woodland, where the Acacia combretum and Acacia terminalia are the most prevalent tree species in Lusaka, Central, Eastern, and Southern provinces (28) (Table 1).
Soils in AER III are highly weathered, leached, and highly acidic. They have limited plant nutrients to support plant growth but have high biological activity. The vegetation in the region is miombo and a mixture of Chipya and dry evergreen forest (28) (Table 1).
2.2 Assessment of natural enemies of fall armyworm
A survey of natural enemies was conducted in 25 maize fields in AER I, 41 in AER IIa, and 24 in AER III during the late rainy season of maize production from March to May 2019 (Table 2). A minimum of four districts in each AER and five fields per district were sampled. Geolocational data, latitude, longitude, and altitude were taken using GPS (GARMIN: GPS MAPR 78s). Sampling was conducted along main trunk roads at intervals of 10 km on maize and sorghum fields. In each field, 50 plants were examined visually for the presence of FAW and natural enemies (including their egg batches, larva and pupa), following along a ‘W’ configuration transect. Maize and sorghum plants were sampled from the vegetative stage to maturity (31, 32).
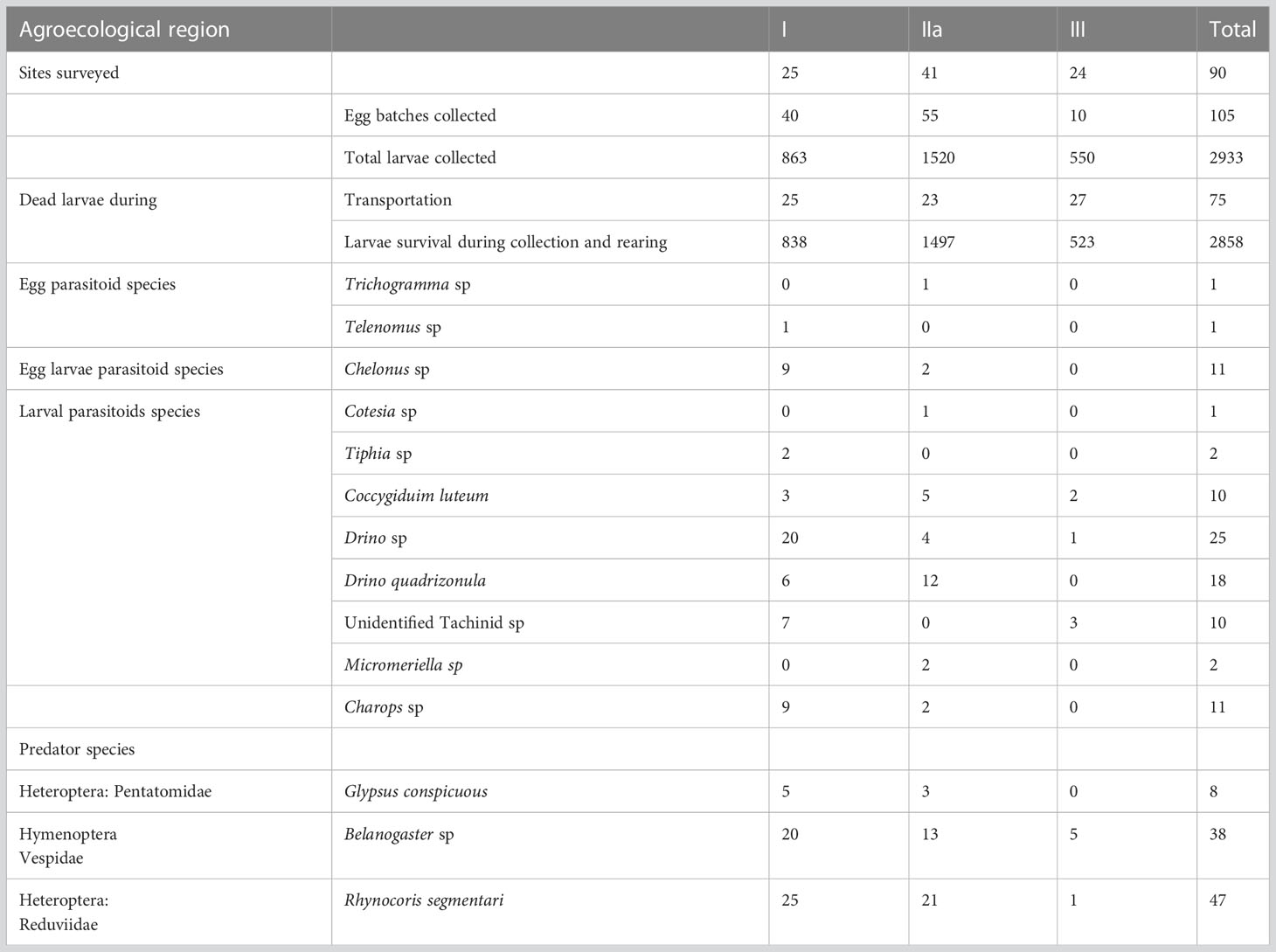
Table 2 Incidence of Fall armyworm eggs, larvae, parasitoids, and predators collected from fields in Agroecological regions I, IIa and III of Zambia in 2019.
Each maize plant was inspected for the presence of natural enemies predating on the FAW egg batches and larvae. The FAW natural enemies were collected directly (predators observed preying on the host) or indirectly (collection of FAW larvae and egg batches from which some parasitoids emerged) from the field. Inspections were done on the middle leaves where egg batches are laid, the upper new leaves, and the whorl where FAW larvae feed and hide.
The eggs were collected by cutting out the leaf tissue with the egg batch from the maize or sorghum plant to avoid dislodging them. The samples were then placed in a petri dish with a piece of paper towel inserted at the base to keep the egg batch and leaf tissue moist. The collection of predators was done simultaneously with FAW eggs and larvae.
Predators seen attacking or feeding on larvae were collected using aspirators and forceps and were put in one-liter jars lined with moistened tissue paper. Some of them were placed in vials with 70% ethanol for preservation. All stages of FAW were collected, and each larvae was placed in a petri dish (9 cm diameter) containing fresh pieces of leaves and stalks as a food source. Before placing the larvae in the petri dish, a piece of tissue paper was laid at the bottom to absorb excess moisture from larvae’s frass and natural diet (replaced every 48 hours). A lid was then placed on top and loosely tied with a rubber band to prevent larvae or parasitoids from escaping.
The Petri dishes were then placed in a thermo-electrical cooler (Campingazi thermoelectric system 12v # 08120OTE 016776) and plugged into the car and delivered to Mt. Makulu Research Station, Entomology laboratory in Chilanga. The larvae were reared on artificial diet (General Diet for Lepidoptera, Product Number F9772, with antibiotic of 14% active chlortetracycline from Frontier TM Agricultural Sciences, Network, DE, USA) in the laboratory. The newly hatched larvae were reared in vials on artificial diet for at least 10 days while observing for egg-larvae parasitoid emergence using a hand lens. Monitoring for parasitoid emergence was done daily in the laboratory.
2.3 Identification of natural enemies
The genomic DNA of adult parasitoids was extracted from the hind legs of the individual insects by using the Isolate II Genomic DNA Kit (Bioline, London, United Kingdom), following the manufacturer’s instructions. The resultant DNA was eluted in a final 50 μl volume, and the quality and quantity checks were done using the Nanodrop 2000 Spectrophotometer (Thermo Fischer Scientific, Wilmington, USA).
The mitochondrial COI gene and the ribosomal domain 2 (D2) region of 28S rDNA were amplified through Polymerase Chain Reaction (PCR) using standard primers LepF1 (5’-ATTCAACCAATCATAAAGATATTGG-3’) and LepR1 (5’ –TAAACTTCTGGATGTCCAAAAAATCA- 3’) (33) for the COI gene, and LepD2F (5’- AGTCGTGTTGCTTGATAGTGCAG- 3’) and LepD2R (5’- TTGGTCCGTGTTTCAAGACGGG- 3’) (34, 35) for the D2 region of 28S rDNA. The PCR reactions were performed in total volumes of 20 µL using 5X My Taq Reaction Buffer (Bioline), 0.5 pmol µL-1 of each primer, 0.5 mM MgCl2, 0.0625 U µL-1 My Taq DNA polymerase (Bioline), and 15 ng µL-1 of DNA template. The PCR cycling conditions set in an Eppendorf Mastercycler® nexus gradient thermal cycler (Eppendorf, Germany) included an initial denaturation step at 95°C for 2 min, followed by 40 cycles of 30 sec at 95°C, 30-sec annealing (52°C for LepF1/R1 and 58.8°C for LepD2 F/R), and 1 min at 72°C, with a final elongation step of 10 min at 72°C. The PCR products were separated on a 1.2% agarose gel, and the DNA bands were analysed and documented using a KETA GL imaging system trans-illuminator (Wealtec Corp, Meadowvale Way Sparks, Nevada, USA). The amplified products were purified using Isolate II PCR and Gel Kit (Bioline) and shipped to Macrogen Europe BV (Meibergreef, Amsterdam, the Netherlands), for bi-directional sequencing (using two primers to simultaneously sequence both strands of the PCR product). The successful sequences were assembled and edited using Geneious Version 8 (http://www.geneious.com) (36), generating a consensus sequence for each sample. From the consensus sequence, the forward and reverse primers were identified and removed. For conclusive identification of the species from both markers, similarity searches were conducted by querying the consensus sequences via BLASTn at the GenBank database hosted by the National Centre of Biotechnology Information (NCBI). BLAST (Basic Local Alignment Search Tool) algorithm finds regions of local similarity between sequences, in which consensus sequences were compared to publicly available sequences in GenBank. In addition to this, the query was also done in the Barcode of Life Database (BOLD). The molecular identification of fall armyworm natural enemies was later compared with the morphological identifications undertaken in the Biosystematics Unit, icipe.
2.4 Data analysis
The primer sequences were identified and removed from the consensus sequences generated from both the forward and reverse reads. For conclusive identification of the species from both markers, similarity searches were conducted by querying the consensus sequences via BLAST at the GenBank database hosted by the National Centre of Biotechnology Information (NCBI). The BLAST (Basic Local Alignment Search Tool) algorithm finds regions of local similarity between sequences, in which consensus sequences are compared with reference sequences in the GenBank database. In addition, the query was also done in BOLD (Barcode of Life Database).
The number of egg batches and larvae collected from AERs was recorded and analysed. Percent parasitism (% Parasitism) was calculated as follows: % Egg parasitism = (Number of egg batches parasitized/Total number of egg batches collected) x 100. Larval parasitism was calculated as follows: % Larval parasitism = (Number of live larvae parasitized (Nfp)/Total number of collected larvae (Nfc)) x 100 (27, 37, 38). Parasitoids emergence data from FAW collected in mono, poly and intercrops, as well as predators observed, was recorded.
The number of collected natural enemies was calculated by adding the number of parasitoids and predators and subjected to statistical analysis using R, version 3.6.1 (39). The mean number of egg batches, FAW larvae, % parasitism, number of parasitoids and predators per AER and natural enemies per cropping practice were compared using the Turkey test (P ≤ 0.05).
Regression analysis was used to test the influence of rainfall on the number of natural enemies across study areas. Relative abundance (RA) of the parasitoids and predators was determined by counting the number of individuals of a given parasitoid or predator species (Ni) divided by the total number of all individuals of all parasitoid or predator species (N) and converted to percent values (23). Parasitoid and predator diversity was determined by applying the Shannon–Weiner diversity index (H) (40).
3 Results
3.1 Identification of natural enemies
A total of 90 locations were surveyed across AERs I, IIa and III for the occurrence, abundance and diversity of FAW natural enemies in Zambia (Table 2). A total of 90 larvae parasitoids were recorded from the three AERs of Zambia, with AER I registering the highest (56), followed by AER IIa (26), and lastly AER III (6) (Table 2). The Drino species occurred in all three AERs and recorded the highest number of 25 individual specimens. It was followed by Drino quadrizonula, with 18 specimens, which occurred only in AER I and AER IIa. Other species recorded included 10 specimens of Coccygiduim luteum from all the AERs of Zambia, however, 11 specimens of Chelonus sp were obtained from AERs I and IIa. Three specimens of Charops sp were recorded from Kuzungula district in AER I. Two specimens each of Tiphia sp and Micromeriella sp were obtained from Livingstone and Chongwe districts in AERs I and IIa, respectively. There were also 10 specimens of unidentified Tachinidae species obtained from Luangwa (5) and Livingstone (2) districts in AER I, and in Kawambwa (1) and Mwansabombwe (2) districts in AER III (Table 3).
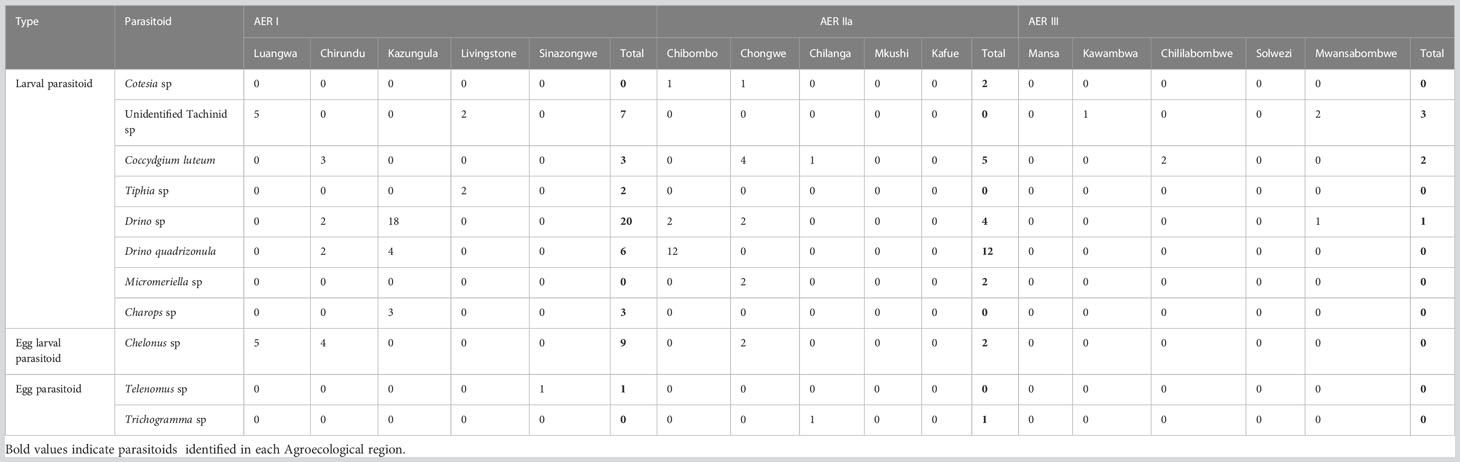
Table 3 Number of parasitoids of fall armyworm egg and larval found in maize fields from Agroecological Regions I, IIa and III of Zambia in 2019.
The total number of predators collected were 93 and the highest number (19) was obtained from Chirundu district in AER I. This was followed by Sinazongwe district (13) in the same AER. Across the AER, AERI recorded the highest (50) predators followed by AER IIa (37) and lastly AERIII (6). The widely distributed predator species were Belanogaster and Rhynocoris segmentarius, which were recorded in all the three AERs (Table 4).

Table 4 Number of predators observed and collected from Agroecological regions I, IIa and III of Zambia in 2019.
From the FAW egg batches and larvae collection, 2 egg parasitoid species emerged from egg batches, while 1 egg–larval parasitoid and 8 larval parasitoid species emerged from larvae. The egg parasitoids were obtained from AER I and AER IIa, while larval parasitoids were obtained from all the three AERs. A total of 3 predatory species predating on FAW larvae were observed.
Molecular characterization of the parasitoid targeting the 28S rDNA D2 gene region identified the parasitoid to the species level. The complete mitochondrial COI gene was obtained from whole genome sequencing, and this resolved the identity of the parasitoid up to species level. A BLAST search of the extracted COI gene generated from this study (GenBank accession number OR058595) had a 100% similarity with Cotesia icipe (MN900735.1). Drino quadrizonula constituted 20% of the total larvae parasitoids reared and was identical to D. quadrizonula (Table 5). This Drino quadrizonula was recovered from AER I and IIa. Rhynocoris segmentarius shared 100% identity with R. segmentarius sp. (GenBank FJ230538.1) (Table 6), and accounted for 50.5% of the larvae predator (Table 4). It was recovered from AER I, IIa, and III.
The highest number of natural enemies was recorded from AER I and AER IIa, while AER III had the lowest (Figure 2). There were significant differences (degrees of freedom (df = 2; P = 0.01) in the occurrence of FAW natural enemies collected from AERs of Zambia.
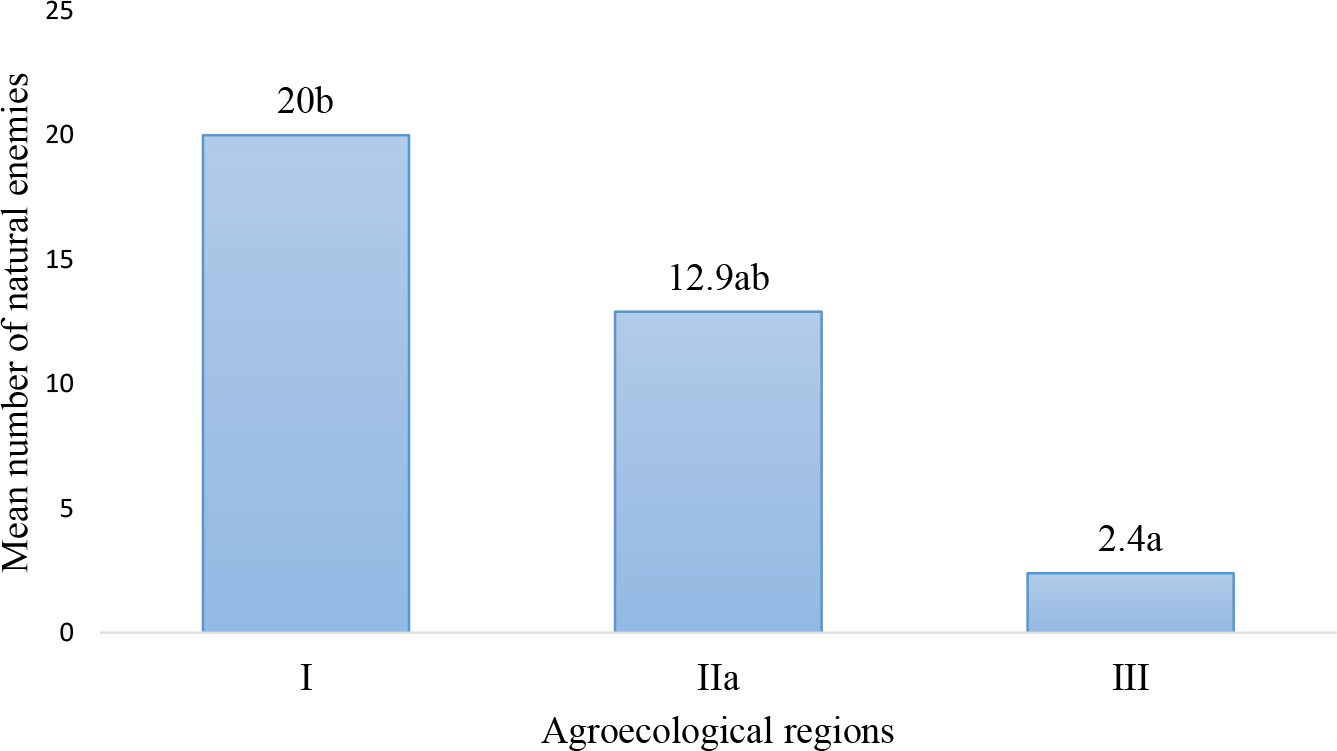
Figure 2 Mean number of natural enemies for fall armyworm recorded from Agroecological regions of Zambia.
3.2 Percent parasitism
3.2.1 Percent egg and larval parasitism
There was a significant difference (P < 0.05) in the number of egg batches obtained from AER IIa, compared with AER I and III. However, egg parasitism was only recorded in AER I and IIa, while larval parasitism was observed in all regions, although there was no significant difference across them (Table 7).
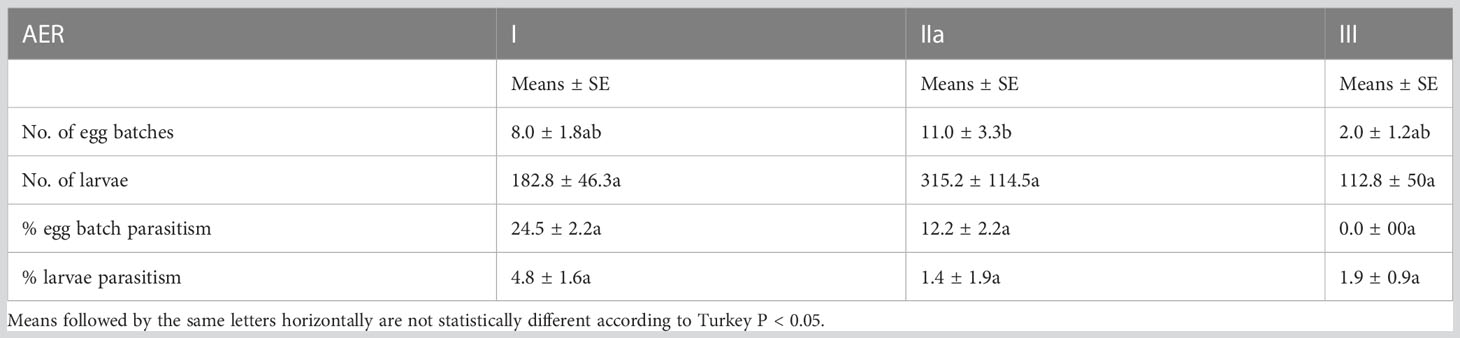
Table 7 Mean percent parasitism of Fall armyworm egg batches and larvae in Agroecological regions I, IIa and III of Zambia in 2019.
3.2.2 Number of fall armyworm natural enemies in maize mono and intercrops
During the survey, FAW larvae were collected from monocrop and intercrop fields. High numbers of natural enemies were collected from intercropped fields (df = 9; P = 0.01). The highest occurrence of natural enemies came from field’s intercroped with maize + cowpeas + pumpkin and watermelon (Table 8). The lowest number of natural enemies was recovered from maize mono crop.
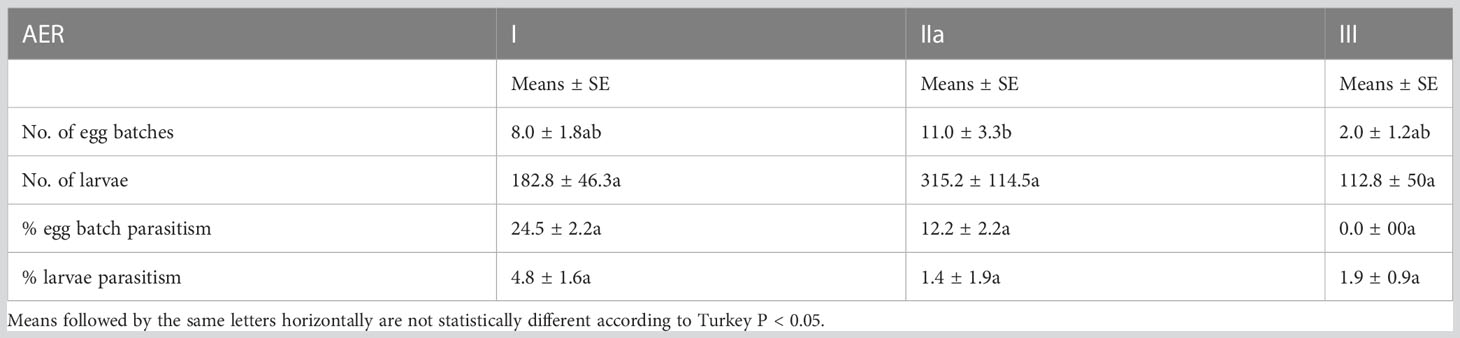
Table 8 Mean number of fall armyworm natural enemies from maize mono and intercrops in Agroecological regions I, IIa and III of Zambia in 2019.
3.2.3 Abundance, diversity, and richness of fall armyworm parasitoids
FAW parasitoid species’ diversity and richness were highest in AER I, 0.2 and 8, respectively. This was followed by AER II with a diversity of 0.1 and richness of 7. AER III had the lowest diversity (0) and richness (4). Unidentified Tachinid sp were the most abundant (50%) in AER III followed by Drino quadrizonula (43%) in AER IIa (Table 9).
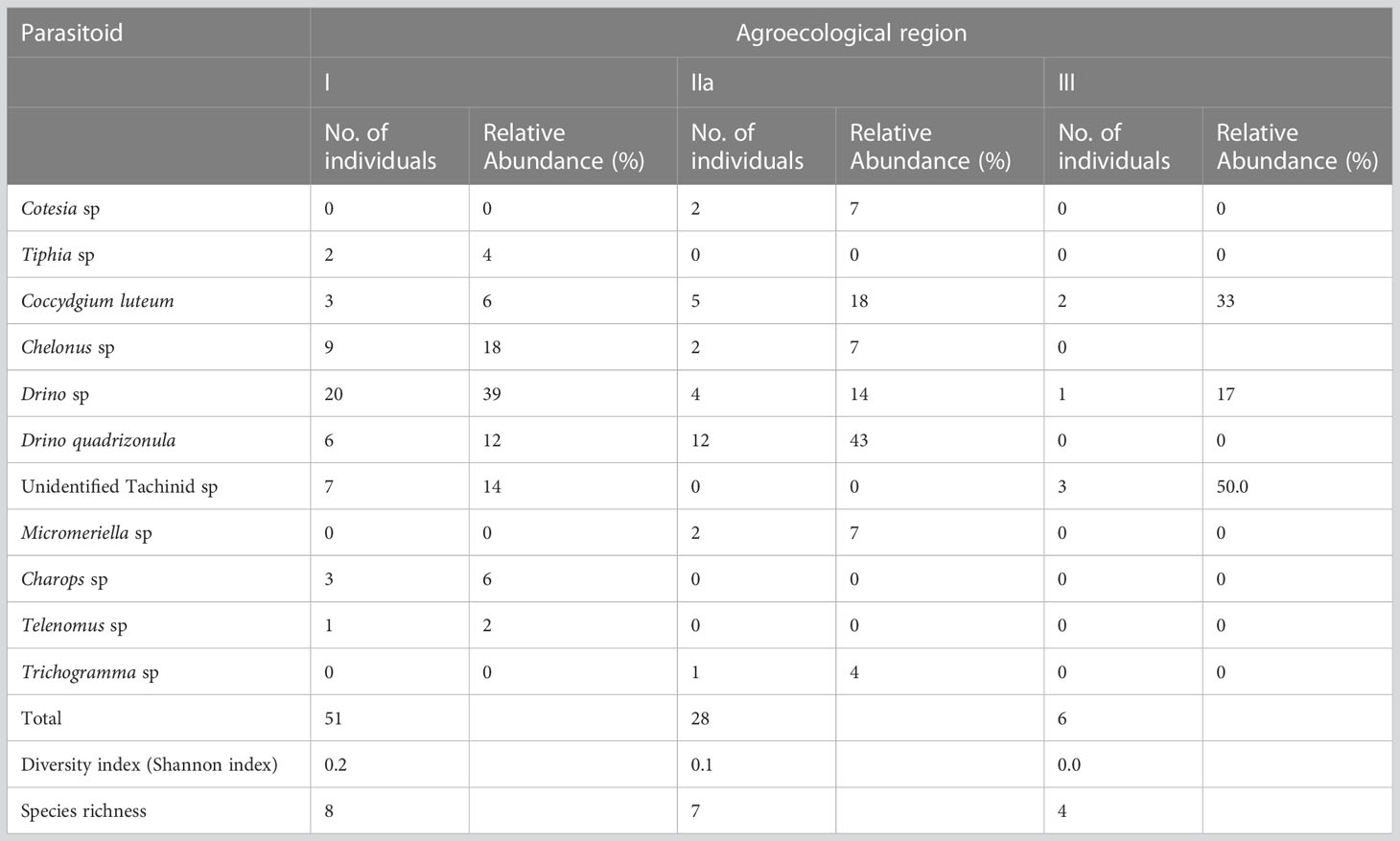
Table 9 Relative abundance, diversity, and richness of fall armyworm parasitoid species in Agroecological regions I, IIa and III in Zambia.
3.2.4 Abundance, diversity, and richness of fall armyworm predators
The predator species diversity (0.9) and richness (3) were high in AER I and IIa. Rhynocoris segmentarius were the most abundant in AER IIa (57) followed by AER I (50). However, it was the lowest (1) in AER III (Table 10). Belanogaster sp was the most abundant in AER III. Glypsus conspicuus was found only in AER I and IIa (Table 10).
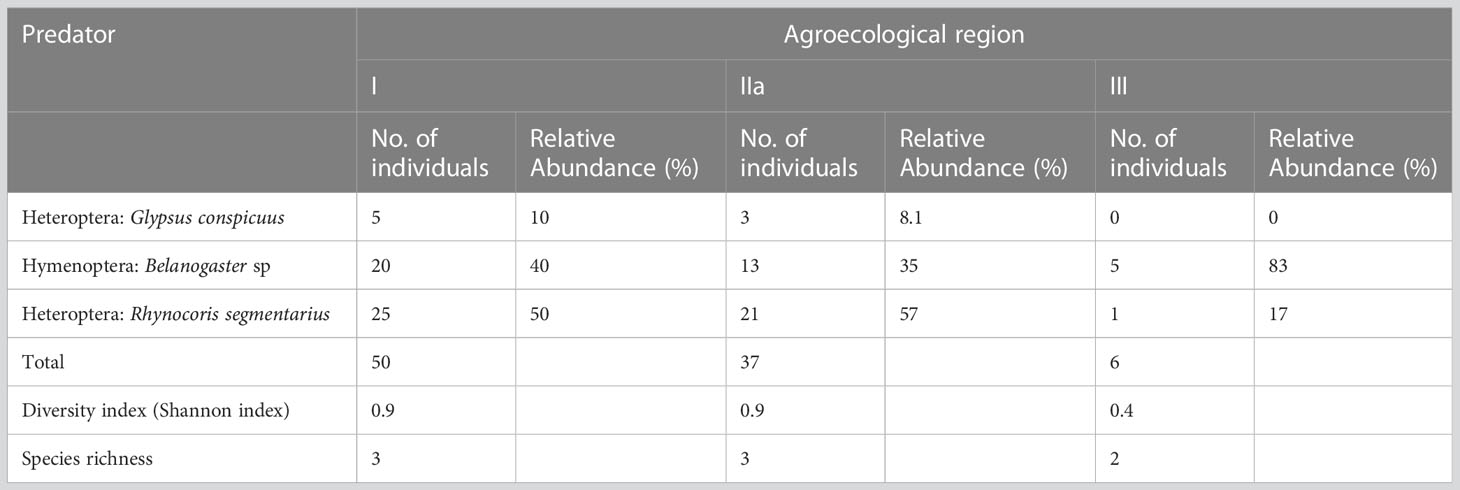
Table 10 Relative abundance, diversity and richness of fall armyworm predator species in Agroecological regions I, IIa and III in Zambia.
3.2.5 Relationships between the number of natural enemies and climatic factors
Significant differences were observed in the number of natural enemies collected in AERs in relation to rainfall (df= 1; P=0.004). A negative correlation was recorded between the number of natural enemies for FAW and rainfall (r2 = 0.48) (Figure 3).
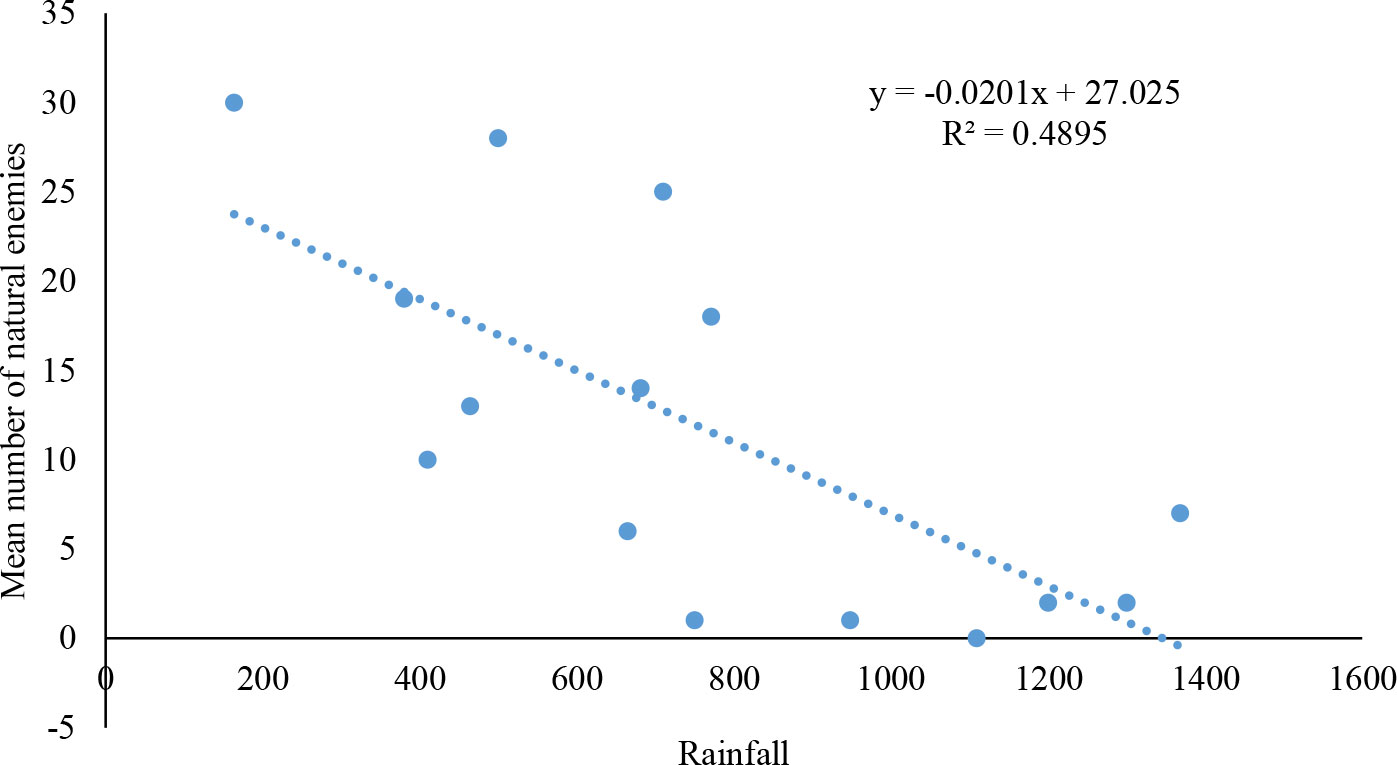
Figure 3 Relationship between fall armyworm natural enemies abundance and rainfall in the studied Agroecological regions of Zambia.
4 Discussions
A total of 11 FAW parasitoid species were collected and identified in this study, with Tachinid species, Drino species, and D. quadrizonula being the most abundant and widely distributed in all three AERs of Zambia. Similar findings have been reported by Shendange and Sathe (41) and Stireman et al. (42), who reported that Tachinid species occur worldwide and in nearly all terrestrial environments. Equally, in Argentina, Dipteran parasitoid species are reported to be FAW’s most widely dispersed biological control agents (43). Chinwada et al. (44) reported that Tachinids were also parasitoids for lepidopteran stemborers in Africa. In Nigeria, Tachinid species have been reported by Murua et al. (45) attacking Spodoptera exampta (Walker). In Ethiopia and Kenya, Palexorista zonota (Diptera: Tachinidae) was among the most-recorded larval parasitoids of FAW (25). In Mozambique, Caniço et al. (27) reported that Tachinid D. quadrizonula Thomson was among FAW’s most abundant larval parasitoids. The wide distribution could be explained by the wide array of lepidopteran pests serving as hosts for Tachinid species. Stemborers and other lepidopteran pests, such as the African armyworm S. exampta, are widely distributed in Zambia (10, 46). The arrival of FAW in Zambia has widened the host range of Tachinid species that lived on stemborers and African armyworm in the past.
The Tachinids were followed by egg–larval parasitoid Chelonus species, which were recorded from AERs I and IIa only. In the present study, C. luteum was the third most widely dispersed parasitoid and was recorded from all three AERs of Zambia, but in low numbers as compared with Tachinid and Chelonus species. It was more predominant in AERs I and IIa, which might be attributable to the high number of FAW recorded from those regions. In Tanzania and Kenya, C. luteum was the most common species (25) and, similarly, in Mozambique (27).
The absence of some parasitoid species in certain areas is attributed to the differences between locations, rainfall, maize crop stage, pest density and larval stage. Durocher-Granger et al. (26) reported similar factors influencing the occurrence of FAW parasitoids in Zambia. In this study, we demonstrated a negative relationship between rainfall and the number of natural enemies recorded from AERs. Although climatic factors are critical, the absence of Chelonus in AER III could also be due to the low numbers of FAW found in that region. Similar observations were reported in Ghana, where Chelonus sp, the most abundant parasitoid species, was obtained from 7 of 10 AERs (47).
Despite the coverage, egg parasitism was only observed in AERs I and IIa, while larval parasitism was recorded in all three regions. Egg parasitism by Telenomus sp and Trichogramma sp was very low in AERs I and IIa. Koffi et al. (47) reported an identical parasitism rate of 3.6% in a countrywide survey conducted in Ghana. Beserra and Parra (48)Dequech et al. (49), and Sun et al. (50) also reported similar observations. However, relatively higher results were reported in Mozambique (27) and in Uganda (51). The low egg parasitism could partly be explained by the fact that FAW covers its eggs with hairs and the short ovipositor of these small insects could not easily penetrate.
The low parasitism could also be attributed to the new associations of FAW and local parasitoids. FAW is a new pest in Africa (12, 52) and has an apparent association with indigenous lepidopteran parasitoids such as C. icipe, recently described from S. littoralis in Kenya (53), C. luteum, other parasitoids belonging to the subfamily Campopleginae that make them less efficient. However, in the current study, Drino sp contributed parasitism of 0.87%, C. luteum 0.35%, D. quadrizonula 0.34%, and Chelonus sp 0.38%.
Two species Tiphia sp and Micromeriella sp with parasitism rates of 0.07% reported for the first time attacking FAW larvae in Africa. Tiphia belongs to the family Tiphiidae, which are solitary wasps whose parasitoids attack various beetle larvae, especially in the Scarabaeoidea superfamily. Rogers and Potter (54) reported that Tiphid species had 33–58% parasitism. Their low parasitism in FAW could result from the pest being new to the parasitoid, and it is envisaged to increase with time.
Hemipterans (Reduviidae and Pentatomidae) and Hymenopterans (Vespidae) were the most common predators found in AERs I and IIa. This could be attributable to favourable environmental conditions and sufficient food, which enhance their survivability. Rhynocoris segmentarius (Reduviidae) was the most occurring and prevalent predator species in all AERs, with a total number of 47 individuals, followed by Hymenopterans of the Belanogaster genus (Vespidae) with 35 individuals and was equally recorded in all studied regions. Species of the Pentatomidae family were the lowest, with only 8 individuals recorded. The collected species are polyphagous as they prey on several other orders of insect species, including lepidopterans, as reported by Sahayaraj et al. (2020) (55).
Among other predators that were observed in the field and which have been reported in previous studies (56–58) but which were not found predating on FAW larvae or eggs were Hemiptera: stink bugs of unidentified species, Dermaptera: Forficulidae sp – earwig, Neuroptera: Chrysopidae: Chrysoperla sp and Coleoptera: Coccinellidae – ladybird beetles.
However, environmental factors and farming practices may also play an important role. Murua et al. (45) attributed low parasitism rates to temperature and rainfall, which are climatologic factors that have diverse effects on the density of the pest. During vegetative growth, the whorl of maize plants forms a funnel in which FAW larvae feed. The funnels collect water during heavy rains leading to drowning or dislodgement of FAW larvae. Similar observations were reported by Karthik et al. (59) for Plutella xylostella, where intense raindrops in the leaf axils dislodged newly hatched larvae from the plant, and a high proportion were killed by high precipitation. Prolonged periods and high incidence of rains in AER III could have caused low recovery rates natural enemies. The rainfall recorded in the 2018/19 season ranged from 380.4 mm in AER I to 1368.3 mm in AER III. Allen and Smith (60) reported a similar observation for Cotesia medicaginis, which reached its maximum longevity at 55% relative humidity, although longevity decreased markedly at levels above and below this value, of which the number of reproduction circles were reduced per year.
The higher diversity of natural enemies in AERs I and IIa could be attributed to high number of maize fields intercropped with legumes, pumpkins, and squash. Farmers intercropped maize with legumes throughout the year under rainfed and irrigation from Kariba dam, Luangwa and Zambezi rivers during the dry season. The intercropped could have high parasitoid and predator species diversity throughout the year. This is similar to the findings of Altieri et al. (61), who reported that when plant complexity increases in the agroecosystem through intercropping, cover crop and living mulch, the diversity of insects, including parasitoids and predator species, increases. The findings in our study in the intercrop and polycrops in AERs I and IIa are similar to observations by Khan et al. (62). Furthermore, Hind and Hooks (63) stated that increasing the flora complexity of agricultural habitats increased the survival and reproduction of natural enemies that promoted the biological control of FAW.
The absence or low occurrence of the predatory insects in AER III is associated with the absence of the outbreak of lepidopteran species, such as Spodoptera exempta Walker, Eldana saccharina Walker, Chilo partellus Swinhoe and Busseola fusca Fuller that have been reported in AERs I and IIa (64). Furthermore, the absence of a specific combination of crop structure and diversity in AER III could have contributed to the absence of the predatory bug. Mata et al. (65) stated that the effect of plant diversity is distinctly species-specific, with some species showing positive and others negative responses to trees, shrubs and crops.
Some of the fields surveyed were being sprayed with insecticides. This was affirmed through observations of containers and packaging materials for insecticides, such as Cypermethrine and Emamectin benzoate, which were poorly disposed in the fields in Kafue, Mkushi, Solwezi, and Mansa districts. In Zambia, insecticides are the first line of control strategy against FAW. In 2017, the Government of Zambia spent 3 million USD on the chemical control of FAW (15). Blanco et al. (66) and Tambo et al. (67) reported that, generally, control of FAW is usually achieved through the application of synthetic insecticides. Similar findings were reported by Kansiime et al. (68), that 60% of the farmers used insecticides to control FAW in Zambia. Therefore, the application of insecticides to control FAW could have contributed to the low parasitism percentage of the FAW in Zambia. Koffi et al. (47) reported that those fields that were not sprayed with insecticides had the highest parasitism percentages of 60% in Agogo Aburkyi, 55.6% in Legon, 33.3% in Kpong and 23.8% in Sanga in Ghana.
5 Conclusion
The study showed that natural enemies, including egg, egg-larval, and larval parasitoids, and predators are present in Zambia. Tachinid Drino species and C. luteum, R. segmentarius and Belanogaster sp are the most abundant and occurring FAW natural enemies. Egg parasitism is found in AER I and AER IIa while larval parasitism is found in all three surveyed regions. Variations in rainfall patterns affecting FAW availability and cropping systems in the three AERs may explain the differences natural enemies’ species diversity in Zambia. The FAW is a serious pest threatening cereal production in Zambia and information provided in this study can aid the development of a national biological control programme for its sustainable management.
Data availability statement
The original contributions presented in the study are included in the article/supplementary materials. Further inquiries can be directed to the corresponding authors.
Author contributions
GC, SP, PK and TT conceptualized. GC conducted the survey, laboratory experiments and wrote the original draft. GC and RC conducted morphological identification of natural enemies. SS, LO, FK and NS conducted molecular identification. GC, PS, PC, SN and SS revised the manuscript and approved the final draft. All authors contributed to the article and approved the submitted version.
Funding
The authors gratefully acknowledge the Zambia Agriculture Research Institute, and Agriculture Production and Productivity for Southern Africa program for financial support. The authors also appreciate financial support from USAID/OFDA through the project, “Reinforcing and Expanding the Community-Based Fall Armyworm Spodoptera frugiperda (Smith) Monitoring, Forecasting for Early Warning and Timely Management to Protect Food Security and Improve Livelihoods of Vulnerable Communities - CBFAMFEW II” (Grant Number 720FDA20IO00133).
Acknowledgments
The authors are grateful to Demian Mabote Ndalamei, Sakayula Kapalu, Monde Musesha, Wendy Lupiya and Tibonge Mfune for their assistance during the collection of FAW samples. We thank Crop Husbandry Officers and Camp Extension Officers, at locations where FAW samples were collected, for their guidance and support. We thank the Department of Plant Science for providing laboratory space where the FAW samples collected from the field were reared.
Conflict of interest
The authors declare that the research was conducted in the absence of any commercial or financial relationships that could be construed as a potential conflict of interest.
The authors SN, FK, PC, SS declared that they were an editorial board member of Frontiers, at the time of submission. This had no impact on the peer review process and the final decision.
Publisher’s note
All claims expressed in this article are solely those of the authors and do not necessarily represent those of their affiliated organizations, or those of the publisher, the editors and the reviewers. Any product that may be evaluated in this article, or claim that may be made by its manufacturer, is not guaranteed or endorsed by the publisher.
References
1. FAOSTAT. (2022). Available at: http://www.fao.org/faostat/en/#data (Accessed September 14, 2022).
2. Midega CA, Toby JA, Bruce TJA, Pickett JA, Khan ZR. Ecological management of cereal stem borers in African smallholder agriculture through behavioral manipulation. Ecol Entomol (2015) 40:70–81. doi: 10.1111/een.12216
3. Nagoshi NR, Georgen G, Tounou AK, Koffi. D. Analysis of strain distribution, migratory potential, and invasion history of fall armyworm populations in northern Sub-Saharan Africa. Sci Rep (2018) 8:3710. doi: 10.1038/s41598-018-21954-1
4. Kenis M, Benelli G, Biondi A, Calatayud PA, Day R, Desneux N, et al. Invasiveness, biology, ecology, and management of the fall armyworm, Spodoptera frugiperda. Entomol Gen (2022), 1–55. doi: 10.1127/entomologia/2022/1659
5. Hruska AJ, Gould F. Fall armyworm (Lepidoptera: Noctuidae) and Diatraea lineolata (Lepidoptera: Pyralidae): impact of larval population level and temporal occurrence on Maize yield in Nicaragua. J Econ Entomol (1997) 90(2):611–22. doi: 10.1016/j.scitotenv.2020.140015
6. Kassie M, Wossen T, De Groote H, Tadele T, Subramanian S, Balew S. Economic impacts of fall armyworm and its management strategies: evidence from southern Ethiopia. Eur Rev Agric Econ (2020) 47(4):1473–501. doi: 10.1093/erae/jbz048
7. Ferreira Filho JBS, Alves L, Gottardo L, Georgino M. Dimensionamento do.custo econômico representado por Spodoptera frugiperda na cultura do milho no Brasil. 48 Congresso Sociedade Brasileira de Economia, Administração e Sociologia Rural 21. Brasília, SOBER (2010).
8. Montezano DG, Specht A, Sosa-Gómez DR, Roque-Specht VF, Sousa-Silva JC, Paula-Moraes SV. Host plants of Spodoptera frugiperda (Lepidoptera: Noctuidae) in the Americas. Afr Entomol (2019) 26(2):286–300. doi: 10.4001/003.026.0286
9. Bueno FOCR, Bueno FA, Moscardi F, Parra PRJ, Hoffmann- campo CB. Lepidoptera Larvae consumption of Soybean foliage: basis for developing multi-species economic thresh holds for pest management decision. Pest Manage Sci (2011) 67:170–4. doi: 10.1002/ps.2047
11. Capinera JL. Fall armyworm, Spodoptera frugiperda (J. E. Smith). Fact sheet. University of Florida (2017). Publication 1999, No. EENY- 98. Revised 2017 and Reviewed 2020, University of Florida.
12. Goergen G, Kumar PL, Sankung SB, Togola A, Tamò M. First report of outbreaks of the fall armyworm Spodoptera frugiperda (J E Smith) (Lepidoptera: Noctuidae), a new alien invasive pest in West and Central Africa. PloS One (2016) 1(10):e0165632. doi: 10.1371/journal.pone.0165632
13. Cock MJW, Beseh PK, Buddie AG, Cafa G, Crozier J. Molecular methods to detect Spodoptera frugiperda in Ghana and implications for monitoring the spread of invasive species in developing countries. Sci Rep (2017) 7:4103. doi: 10.1038/s41598-017-04238-y
14. ZARI. Report on surveillance of the armyworm and stalk borers in Zambia, entomology report. Lusaka, ZARI (2017).
15. DMMU. Experiences of the fall armyworm infestation in Zambia in the 2016/2017 rainfall season. Terminal report. Lusaka, DMMU (2017).
16. FAO. Management of fall armyworm (Spodoptera frugiperda, J. E. Smith) in Zambia. TCP/ZAM/36031 report. Rome, FAO (2020).
17. Lu YH, Wu KM, Jiang YY, Guo YY, Desneux N. Widespread adoption of Bt cotton and insecticide decrease promotes biocontrol services. Nature (2012) 487:362–7. doi: 10.1038/nature11153
18. Wyckhuys KAG, Lu Y, Morales H, Vazquez LL, Legaspi J, Eliopoulos AP, et al. Current status and potential of conservation biological control for agriculture in the developing world. Biol Sci (2013) 65:152–67. doi: 10.1016/j.biocontrol.2012.11.010
19. Chen Y, Guo J, Gao Z, He K, Bai S, Zhang T, et al. Performance of Spodoptera frugiperda (Lepidoptera: Noctuidae) fed on six host plants: potential risks to mid-high latitude crops in China. J Agric Sci (2020) 12:16. doi: 10.5539/jas.v12n10p16
20. Midega CA, Pittchnar J, Picktt JA, Hailu G, Khan ZR. A climate-adapted push pull system effectively controls fall armyworm, Spodoptera frugiperda (J e smith) in maize in East Africa. Crop Prot (2018) 105:10–5. doi: 10.1016/j.cropro.2017.11.003
21. Hailu G, Niassy S, Khan ZR, Ochatum N, Subramanian S. Maize–legume intercropping and push-pull for management of fall armyworm, stemborers, and striga in Uganda. J Agron (2018) 110:2513–22. doi: 10.2134/agronj2018.02.0110
22. Ullah S, Raza ABM, Alkafafy M. Isolation, identification and virulence of indigenous entomopathogenic fungal strains against the peach-potato aphid, Myzus persicae Sulzer (Hemiptera: Aphididae), and the fall armyworm, Spodoptera frugiperda (J.E Smith) (Lepidoptera: Noctuidae). Egypt J Biol Pest Control (2021) l:2. doi: 10.1186/s41938-021-00500-8
23. Agboyi LK, Layodé BFR, Fening KO, Beseh P, Clottey Day VA, Kenis M, et al. Assessing the potential of inoculative field releases of Telenomus remus to control Spodoptera frugiperda in Ghana. Insects (2021) 12:665. doi: 10.3390/insects12080665
24. Harrison RD, Thierfelder C, Baudron F, Chinwada P, Midega C, Schaffner U, et al. Agroecological options for fall armyworm (Spodoptera frugiperda J. E Smith) management: providing low-cost, smallholder friendly solutions to an invasive pest. J Environ Manage (2019) 243:318–30. doi: 10.1016/j.jenvman.2019.05.011
25. Sisay B, Simiyu J, Mendesil E, Likhayo P, Ayalew G, Mohamed S, et al. Fall armyworm, Spodoptera frugiperda infestations in East Africa: assessment of damage and parasitism. Insects (2019) 10(7):195. doi: 10.3390/insects10070195
26. Durocher-Granger L, Mfune T, Musesha M, Lowry A, Reynolds K, Buddie A, et al. Factors influencing the occurrence of fall armyworm parasitoids in Zambia. J Pest Sci (2021) 94:1133–46. doi: 10.1007/s10340-020-01320-9
27. Caniço A, Mexia A, Santos L. First report of native parasitoids of fall armyworm Spodoptera frugiperda Smith (Lepidoptera: Noctuidae) in Mozambique. Insects (2020) 11:615. doi: 10.3390/Insects11090615
28. Bunyolo A, Chirwa B, Muchinda M. Agro–ecological and climatic conditions of Zambia. 1995 Edition. Zambia Seed Technology Handbook, Lusaka (1995).
29. Maki H. Agriculture and forestry in Zambia: present situation and issues for development Japan association for international collaboration of agriculture and forestry. Zambia, Tokyo (2008).
30. Veldkamp WJ. Reconnaissance/semi – detailed semi – quantified land evaluation system for non–irrigated (rainfed) agriculture; first edition. technical guide no. 19. Soil Survey Unit, Research Branch, Department of Agriculture, Republic of Zambia, Lusaka (1987). p. 10.
31. Abendroth LJ, Elmore RW, Boyer MJ, Marlay SK. Corn growth and development. Iowa State University extension, Lowa (2011). PMR 1009.
32. Ransom J. Corn growth and management 1173A quick giude. North Dakota State University, North Dakota (2020).
33. Hajibabaei M, Smith A, Janzen ND, Rodrguez JJ, Whitefield BJ, Hebert DN. A minimalist barcode can identify a specimen whose DNA is degraded. (2006) 6:959–964. doi: 10.1111/j.1471-8286.2006.01470.x
34. Campbell BC, Steffen-Campbell JD, Werren JH. Phylogeny of the Basonia species complex (Hymenoptera: Pteromalidae) inferred from an internal transcribed spacer (ITS2) and 28s rDNA sequences. Insect Mol Biol (1993) 2:225–37. doi: 10.1111/j.1365-2583.1994.tb00142.x
35. Goolsby JA, DeBarro PJ, Makinson JR, Pemberton RW, Hartley DM, Frohlich. D. Matching the origin of an invasive weed for selection of a herbivore haplotype for a biological control programme. Mol Ecol (2006) 15:287–97. doi: 10.1111/j.1365-294X.2005.02788.x
36. Kearse M, Moir R, Wilson A, Stones-Havas S, Cheung M, Sturrock S, et al. An integrated and extendable desktop software platform for the organization and analysis of sequence data. Bioinformatics (2012) 28(12):1647–9. doi: 10.1093/bioinformatics/bts199
37. Pair SD, Raulston JR, Sparks AN, Martin PB. Fall armyworm (Lepidoptera: Noctuidae) parasitoids: different spring distribution and incidence on corn and sorghum in the southern United States and northeastern Mexico. Entomology (1986) 15:342–8. doi: 10.1093/ee/15.2.342
38. Rios-Velasco C, Gallegos-Morales G, Cambero-Campos J, Ma CE, Del Rincón-Castro C, Valenzuela-García R, et al. Natural enemies of the fall armyworm Spodoptera frugiperda (Lepidoptera: Noctuidae) in Coahuila, México. Fla Entomol (2011) 3:723–6. doi: 10.1653/024.094.0349
39. R Core Team. A language and environment for statistical computing. Vienna, Austria: R Foundation for Statistical Computing (2019).
40. Krebs CJ. Ecological methodology. 2nd edition. Benjamin Cummings, upper saddle River, New Jersey (1999).
41. Shendange N, Sathe TV. Tachinid as good biocontrol agent of agricultural pests. Biol Sci (2016) 4(1):79–83. doi: 10.17812/blj.2016.4110
42. Stireman JO III, Cerretti P, O’Hara JE, Blaschke JD, Moulton JK. Molecular phylogeny and evolution of world Tachinidae (Diptera). Mol Phylogenet Evol (2019) 139:106358. doi: 10.1016/j.ympev.2018.12.002
43. Faithpraise F, Idung J, Chatwin C, Young R, Birch P. Modelling the control of African armyworm (Spodoptera exempta) infestations in cereal crops by deploying naturally beneficial insects. Biosyst Eng (2015) 129:268–76. doi: 10.1016/j.biosystemseng.2014.11.001
44. Chinwada P, Nyamutukwa S, Marandure A, Zitsanza ES. Biology and development of Sturmiopsis parasitica (Diptera: Tachinidae) on different species and populations of maize stem borers in Zimbabwe and an assessment of its suitability for redistribution in Africa. Afr Entomol (2014) 22(4):828–37. doi: 10.4001/003.022.0408
45. Murua G, Molina – Ochoa J, Coviella C. Population dynamics of the fall armyworm, spodoptera frugiperda (Lepidoptera: Noctuidae) and its parasitoids in northwestern Argentina. Fla Entomol (2006) 89(2):175–82. doi: 10.1653/0015-4040(2006)89[175:PDOTFA]2.0.CO;2
46. Okech SHO, Neukermans LMNR, Chinsembu KC. Agro ecological distribution of major stalk borers of maize in Zambia. Int J Trop Insect Sci (1994) 15:167–72. doi: 10.1017/S174275840001540X
47. Koffi D, Kyerematen R, Eziah VR, Agboka K, Adom M, Goergen G, et al. Natural enemies of the fall armyworm, Spodoptera frugiperda (J.E. smith) (Lepidoptera: noctuidae) in Ghana. Fla Entomol (2020) 103(1):85–90. doi: 10.1653/024.103.0414
48. Beserra EB, Parra JRP. Biologia e parasitismo de Trichogramma atopovirilia Oatman and Platner e Trichogramma pretiosum Riley (Hymenoptera: Trichogrammatidae) em ovos de Spodoptera frugiperda (J.E. Smith) (Lepidoptera, Noctuidae). Rev Bras Entomol (2004) 48(1):119–26. doi: 10.1590/S0085-56262004000100020
49. Dequech BST, Camara C, Soares Sturza V, do Prado Ribeiro L, Barbosa Querino R, Poncio R. Population fluctuation of Spodoptera frugiperda eggs and natural parasitism by Trichogramma in maize. Acta Sci Agron (2013) 35:295–300. doi: 10.4025/actasciagron.v35i3.16769
50. Sun JW, Hu HY, Nkunika POY, Dai P, Xu W. Performance of two trichogrammatid species from Zambia on fall armyworm, Spodoptera frugiperda (J. E. Smith) (Lepidoptera: Noctuidae). Insects (2021) 12:859. doi: 10.3390/insect12100859
51. Otim MH, Aropet SA, Opio M, Kanyesigye D, Opolot NH, Tay TW. Parasitoid distribution and parasitism of the fall armyworm Spodoptera frugiperda (Lepidoptera: Noctuidae) in different maize producing regions of Uganda. Insects (2021) 12(2):121. doi: 10.3390/insects12020121
52. Tendeng E, Labou B, Diatte M, Djiba S, Diarra K. The fall armyworm Spodoptera frugiperda (J. E. Smith), a new pest of maize in Africa: biology and first native natural enemies detected. Int J Biol Chem Sci (2019) 13:1011–26. doi: 10.4314/ijbcs.v13i2.35
53. Agbodzavu MK, Lagat ZO, Gikungu M, Rwomushana I, Ekesi S, Fiaboe KM. Performance of the newly identified endoparasitoid Cotesia icipe Fernandez-Triana and Fiaboe on Spodoptera littoralis (Boisduval). J Appl Entomol (2018) 142:646–53. doi: 10.1111/jen.12514
54. Rogers ME, Potter DA. Biology of Tiphia pygidialis (Hymenoptera: Tiphiidae), a parasitoid of masked chafer (Coleoptera: Scarabaeidae) grubs, with notes on the seasonal occurrence of Tiphia vernalis in Kentucky. Environ Entomol (2004) 33(3):520–7. doi: 10.1603/0046-225X-33.3.520
55. Sahayaraj K, Kalidas S, Estelle LYL. Bioefficacy of Rhynocoris longifrons (Stall) (Heteroptera: Reduviidae) against multiple cotton pests under screen house and field conditions. Sci Rep (2020) 10:6637. doi: 10.1038/s41598-02-63768-0
56. Sueldo MR, Octavio A, Bruzzone E, Virla G. Characterization of the earwig, Doru lineare, as a predator of larvae of the fall armyworm, Spodoptera frugiperda. A functional response study. Insect Sci (2010) 10:38. doi: 10.1673/031.010.3801
57. Torres TB, Barros EM, Coelho RR, Pimental RM. Zoophytophagous pentatomids feeding on plants and implication for biological control. Arthropod Plant Inte (2010) 4:219–27. doi: 10.1007/s11829-010-9095-2
58. Gontijo PC, Neto DOA, Oliveira RL, Michaud JP, Carvalho GA. Non-target impacts of soybean insecticidal seed treatments on the life history and behavior of Podisus nigrispinus, a predator of fall armyworm. (2018) 191:342–349. doi: 10.1016/j.chemosphere.2017.10.062.
59. Karthik S, Reddy MS, Yashaswini G. Climate change and its potential impacts on insect-plant interactions. In: Harris SA, editor. The nature, causes, effects and mitigation of climate change on the environment. London: IntechOpen (2021). doi: 10.5772/intechopen.98203
60. Allen WW, Smith RF. Some factors influencing the efficiency of Apanteles medicaginis Muesebeck (Hymenoptera: Braconidae) as a parasite of the alfalfa caterpillar Colias philodice eurytheme Boisduval. Hilgardia (1958) 28:1–42. doi: 10.3733/hilg.v28n01p001
61. Altieri MA, Wilson RC, Schmidt LL. The effects of living mulches and weed cover on the dynamics of foliage and soil-arthropod communities in three crop system. Crop Prot (1985) 4:201–13. doi: 10.1016/0261-2194(85)90018-3
62. Khan ZR, James DG, Midega C, Pickett JA. Chemical ecology and conservation. Biol Control (2008) 45:210–24. doi: 10.1016/j.biocontrol.2007.11.009
63. Hind J, Hooks CRR. Population dynamics of arthropods in a sunhemp zucchini interplanting system. Crop Prot (2013) 53:6–1. doi: 10.1016/j.cropro.2013.06.003
64. Sohati P, Mukanga M, Musonda EM. Distribution of cereal stemborers in Zambia and release of Cotesia flavipes Cameron, an exotic natural enemy of Chilo partellus (Swinhoe). Insect Sci (2001) 21:4. doi: 10.1017/S1742758400008407
65. Mata L, Threlfall CG, Williams NS, Hahs AK, Malipatil M, Stork NE, et al. Conserving herbivorous and predatory insects in urban green spaces. Sci Rep (2017) 7:40970. doi: 10.1038/srep40970
66. Blanco CA, Pellegaud JG, Nava-Camberos U, Lugo-Barrera D, Vega-Aquino P, Coello J, et al. Maize pests in Mexico and challenges for the adoption of integrated pest management programs. J Integr Pest Manage (2014) 5(4):E1–9. doi: 10.1603/IPM14006
67. Tambo A, Kansiime MK, Magambi I, Rwomushana I, Kenis M. Understanding smallholders’ responses to fall armyworm (Spodoptera frugiperda) invasion: evidence from five African countries. Sci Total Environ (2020) 740. doi: 10.1016/j.scitotenv.2020.140015
Keywords: fall armyworm, agroecological region, parasitoids, predators, biological control, Zambia
Citation: Chipabika G, Sohati PH, Khamis FM, Chikoti PC, Copeland R, Ombura L, Kachapulula PW, Tonga TK, Niassy S and Sevgan S (2023) Abundance, diversity and richness of natural enemies of the fall armyworm, Spodoptera frugiperda (J.E. Smith) (Lepidoptera: Noctuidae), in Zambia. Front. Insect Sci. 3:1091084. doi: 10.3389/finsc.2023.1091084
Received: 06 November 2022; Accepted: 26 June 2023;
Published: 20 October 2023.
Edited by:
Fangneng Huang, Louisiana State University, United StatesReviewed by:
Renata Bažok, University of Zagreb, CroatiaAleksandra Konjevic, University of Novi Sad, Serbia
Copyright © 2023 Chipabika, Sohati, Khamis, Chikoti, Copeland, Ombura, Kachapulula, Tonga, Niassy and Sevgan. This is an open-access article distributed under the terms of the Creative Commons Attribution License (CC BY). The use, distribution or reproduction in other forums is permitted, provided the original author(s) and the copyright owner(s) are credited and that the original publication in this journal is cited, in accordance with accepted academic practice. No use, distribution or reproduction is permitted which does not comply with these terms.
*Correspondence: Gilson Chipabika, gilsonchipabika@gmail.com