- 1Department of Entomology, Texas A&M AgriLife Research, Corpus Christi, TX, United States
- 2Plant Science Research Laboratory, US Department of Agriculture-Agricultural Research Service, Stillwater, OK, United States
- 3Department of Entomology and Plant Pathology, Auburn University, Auburn, AL, United States
- 4Department of Entomology, Texas A&M University, College Station, TX, United States
- 5Department of Agricultural Biology, Colorado State University, Fort Collins, CO, United States
- 6Syngenta Crop Protection, Greensboro, NC, United States
- 7Department of Ecology and Conservation Biology, Texas A&M University, College Station, TX, United States
- 8Department of Entomology & Plant Pathology, Oklahoma State University, Stillwater, OK, United States
The sorghum (Sorghum bicolor [L.]) agroecosystem of North America provided an opportunity to evaluate agroecosystem response to an invading insect herbivore, Melanaphis sorghi (Theobald) (sorghum aphid) (previously published as Melanaphis sacchari Zehntner) (Hemiptera: Aphididae) onto a widely planted crop that experiences a range of agro-landscape and weather conditions. Initial sorghum risk assessments after M. sorghi's invasion in the mid-2010s provided forecasts of range expansion and annual migration, which were based on aphid life history, extent of sorghum cultivation and susceptibility to M. sorghi, and weather (aphid-plant-weather [APW] risk scenario). A more comprehensive risk assessment proposed here brings top-down forces of M. sorghi-natural enemy interactions to the forefront as mediated by agro-landscape and weather conditions (aphid-enemy/landscape-weather mediated [AE/LW] risk scenario). A hypothesis of regional differences in aphids and natural enemies and sensitivity to agro-landscape and weather was tested using empirical data of insect, landscape, and weather data across 5 years and four regions (two in the U.S. Great Plains [South GP and North GP], one farther south (South), and one in the southeast U.S. [South E]). Natural enemies were widespread with two parasitoids and four coccinellid species common across regions, but regional variation in M. sorghi and natural enemy abundance was detected. The AE/LW risk scenario accounted for natural enemy abundance and activity that was highest in the South region, functioned well across agro-landscape and weather conditions, and was accompanied by average low M. sorghi abundance (~23 M. sorghi per leaf). Positive correlations of natural enemy-M. sorghi abundance also occurred in the South GP region where M. sorghi abundance was low (~20 M. sorghi per leaf), and selected natural enemy activity appeared to be mediated by landscape composition. Melanaphis sorghi abundance was highest in the South E region (~136 aphids/leaf) where natural enemy activity was low and influenced by weather. The AE/LW risk scenario appeared suited, and essential in the South region, in assessing risk on a regional scale, and sets the stage for further modeling to generate estimates of the degree of influence of natural enemies under varying agro-landscape and weather conditions considered in the AE/LW risk scenario. Broadly, these findings are relevant in understanding agroecosystem resilience and recommending supportive management inputs in response to insect invasions in context of natural enemy activity and varied environmental conditions.
Introduction
The sorghum, Sorghum bicolor (L.), agroecosystem of North America provided an opportunity to evaluate agroecosystem response to an invading insect herbivore onto a widely planted crop that experiences a range of agro-landscape and weather conditions. Nearly all cultivation of sorghum in the United States of America (U.S.), Mexico, and other countries in the vicinity of the Gulf of Mexico became at risk to Melanaphis sorghi Theobold (sorghum aphid) (Hemiptera: Aphididae) infestations during the mid-2010s onward, as indicated by overlaying M. sorghi infestations with sorghum production (1, 2). A superclone designation of the genotype found on sorghum (3, 4) and the classification of this invasive genotype as M. sorghi with likely origin from Africa or Asia (5) was supported by morphometric and molecular research. Previous publications of M. sorghi on sorghum in North America have used the name Melanaphis sacchari Zehntner [(6), and references therein]. This aphid principally damages sorghum (7) and is suppressed by natural enemies in subtropical and tropical regions (8).
Melanaphis sorghi was not a significant sorghum pest in North America until outbreaks on sorghum began to be detected along the Texas Gulf Coast in 2013 (2). From 2013 to 2015 M. sorghi spread rapidly. The near-continental scale of the invasion extended south into Mexico and the Caribbean islands. Northward, its range extended to the central U.S. (Illinois and Kansas) and along the eastern coast from Florida to North Carolina. It also was detected to lesser extents in Arizona and California (2, 6, 9). The rapid spread and damaging outbreaks supported its characterization as a novel, invasive colonizer causing substantial ecological disruption and economic losses (10). Initial success of M. sorghi as an invader may be attributable to sorghum as a widely available resource and excellent M. sorghi host [i.e., resource availability hypothesis (11) and defense-free space hypothesis (12)], few or ineffective top-down forces imposed by pre-existing aphid natural enemies [i.e., enemy-free space hypothesis (13)], suitability to climate and other abiotic factors (13, 14), and the number of source aphids initially introduced and radiating outward after establishment in the Gulf Coast area [propagule pressure hypothesis (15)], or some combination of these factors (16, 17).
From the perspective of agroecosystem response and resiliency to disturbances caused by insect invasions, sorghums in cultivation were largely lacking in bottom-up contraints to M. sorghi as most were susceptible to M. sorghi with damage resulting in economic loss (2, 18) and supported high M. sorghi reproduction which provided source populations for range expansion (19, 20). Potential top-down forces in M. sorghi population regulation were also a point of discussion during the establishment and early expansion phase of the invasion. The periodic outbreaks of M. sorghi on sorghum overlapped in geographic range with a suite of natural enemies suppressing other aphids occurring in cereal grains grown in the North American Great Plains (6). Melanaphis sorghi on sorghum presented an abundant prey item to add to the aphid herbivore guild on sorghum: Rhopalosiphum maidis (Fitch) (corn leaf aphid), Sipha flava (Forbes) (yellow sugarcane aphid), and Schizaphis graminum (Rondani) (greenbug) (Hemiptera: Aphididae) (21). Within a few years of its introduction, natural enemies preying on M. sorghi consisted of about 19 species including solitary endoparasitoids (Hymenoptera: Aphelinidae, Braconidae), a hyperparasitoid (Hymenoptera: Encyrtidae), lady beetles (Coleoptera: Coccinellidae), hoverflies (Diptera: Syrphidae), lacewings (Neuroptera: Chrysopidae and Hemerobiidae), and pirate bugs (Hemiptera: Anthocoridae) [e.g., (22–26)]. Maxson et al. (26) confirmed development to adults of many of these species fed M. sorghi in the laboratory. Selected lady beetle and lacewing species have been shown to suppress M. sorghi in small experimental arenas (27). Yet these observations were occurring in the background of rapid range expansion of M. sorghi and reports of M. sorghi outbreaks from the North American Great Plains to the southeastern U.S. (2).
Looking back at past cereal aphid invasions in the North American Great Plains, the most recent mass field releases of exotic natural enemies consisted of multiple species of parasitoids and predators released for control of Diuraphis noxia (Mordvilko) (Hemiptera: Aphididae) (Russian wheat aphid) during the later 1980s and early 1990s. Outcomes of these releases suggested that the natural enemy complex preying on D. noxia may be flexible in responding to future aphid invasions onto cereal grains (28). This proposition was based on data indicating that several endemic and long-time resident natural enemies along with a few intentionally introduced species were suppressing D. noxia and S. graminum on wheat in at least part of their range (6, 29, 30). Further, cereal aphid-natural enemy interactions may be influenced by agro-landscape and weather conditions that vary across geographic gradients (30–33). For example, aphid-enemy interactions on cereal grains occur where crop production extends across cool temperate to subtropical climatic zones of North America (6). A latent period of natural enemy response to M. sorghi as a new prey item would help explain the apparent low top-down forces during the establishment and early expansion phases of the invasion.
Ecological modeling approaches have been employed for forecasting agroecosystem disruption by and response to invasive species after establishment (34). Value of such efforts includes predicting range expansion, agricultural impact and need for management interventions, and effects of biotic and abiotic factors (35). For the M. sorghi invasion of sorghum in North America, ecological modeling and other qualitative sorghum risk evaluations initially focused on aphid life history, extent of sorghum cultivation and susceptibility to M. sorghi, and weather (2, 19, 20), which is termed here as the aphid-plant-weather (APW) risk assessment scenario. Host plant considerations in this assessment have included sorghum as an excellent reproductive host of M. sorghi until the plant deteriorates (36) and M. sorghi occurring on sorghum regrowth in harvested grain fields, forage sorghum fields, and the alternative host Sorghum halepense (L.) Pers. (johnsongrass) (37). These plants persist through winter in subtropical areas, providing harborage to M. sorghi (37). These characteristics suggested the persistent high risk of M. sorghi outbreaks in subtropical growing areas of the most southern U.S. (inclusive of two regions of this study) and countries farther south. Models also suggested a northward progression of declining risk characterized by more episodic outbreaks as winter host plant harborage declined in temperate zones and annual M. sorghi migrations into regions with expansive plantings of sorghum appeared driven by capacity of the aphid to move long distances via seasonal wind-aided long-distance flights (19).
A more comprehensive aphid-enemy/landscape-weather mediated (AE/LW) risk assessment scenario brings top-down forces of M. sorghi-natural enemy interactions to the forefront as mediated by agro-landscape conditions (partly as an indicator of bottom-down forces) and weather conditions (as a measure of general abiotic influences). This risk scenario was based on reports of natural enemy activity associated with M. sorghi that appeared to vary across regions. Field populations of Aphelinus sp. (Hymenoptera: Aphelinidae), syrphid larvae, and coccinellids suppressed M. sorghi placed on potted sorghum in Kansas (22). In south and central Texas, Aphelinus nigritus Howard (Hymenoptera: Aphelinidae) became the numerically dominant natural enemy attacking M. sorghi, and suppression was indicated by repeated measurements of M. sorghi and A. nigritus across the growing season (26). Lysiphlebus testaceipes (Cresson) (Hymenoptera: Braconidae) parasitized M. sorghi in northeastern Mexico (25), was infrequently observed parasitizing M. sorghi in Texas and Georgia (26, 38), and was not observed parasitizing M. sorghi in Kansas (22). Coccinellids and selected other predator taxa were commonly reported from south Texas (26) and Mexico (39) but were observed sporadically in Georgia (38). Prior to the M. sorghi invasion, variability in abundance of the natural enemy complex and in corresponding cereal aphid suppression has been reported in wheat and barley across the Great Plains (29, 30).
To compare expectations of the APW and AE/LW risk scenarios with empirical data, we pursued a large-region comparative study to characterize the natural enemy complex, estimate M. sorghi and natural enemy population trends, and detect relationships of natural enemy activity on M. sorghi as mediated by agro-landscape and weather conditions. Four sorghum production regions of North America were selected that experienced M. sorghi outbreaks on sorghum to varying degrees (23, 38, 40) and represented a range of subtropical and temperate climatic zones, intensity of sorghum production, and landscape structure. The working null hypothesis was M. sorghi and natural enemy population trends did not differ among regions and were insensitive to agro-landscape and weather conditions. If differences across the four regions were detected, the results were used to consider which of the two risk scenarios were most representative of the M. sorghi invasion and better represented agroecosystem response to our model system of an invading insect herbivore into a widely planted crop.
Materials and Methods
Sampling Regions and Techniques
Natural enemy and aphid sampling occurred in sorghum fields cultivated for grain at multiple locations within four regions. The regions represented the extensive cereal grain production area of the U.S. portion of the North American Great Plains where M. sorghi quickly spread into widely planted sorghum (two regions labeled South GP and North GP) and its southern extension that included areas of first reported M. sorghi outbreaks where sorghum was a major agro-landscape component (one region labeled South). A southeast U.S. region was included where rapid eastward spread of M. sorghi occurred and sorghum was a lesser component of the agro-landscape (one region labeled South E) (Figure 1). The five-year study (2015–2019) overlapped with, but primarily followed, M. sorghi's rapid geographic expansion from 2013 to 2015 (2). The specific geographies and climates were the Texas Gulf Coast extending from the Rio Grande Valley of southern Texas to near the city of Houston with primarily subtropical temperate climate (South), central Texas to the lower Texas Panhandle and across central Oklahoma with a warm temperate climate (South GP), south and central Alabama with a mix of subtropical and warm temperate climate (South E), and the upper Texas and Oklahoma Panhandles through northern Oklahoma and adjacent southern Kansas with a temperate climate (North GP) (Figure 1).
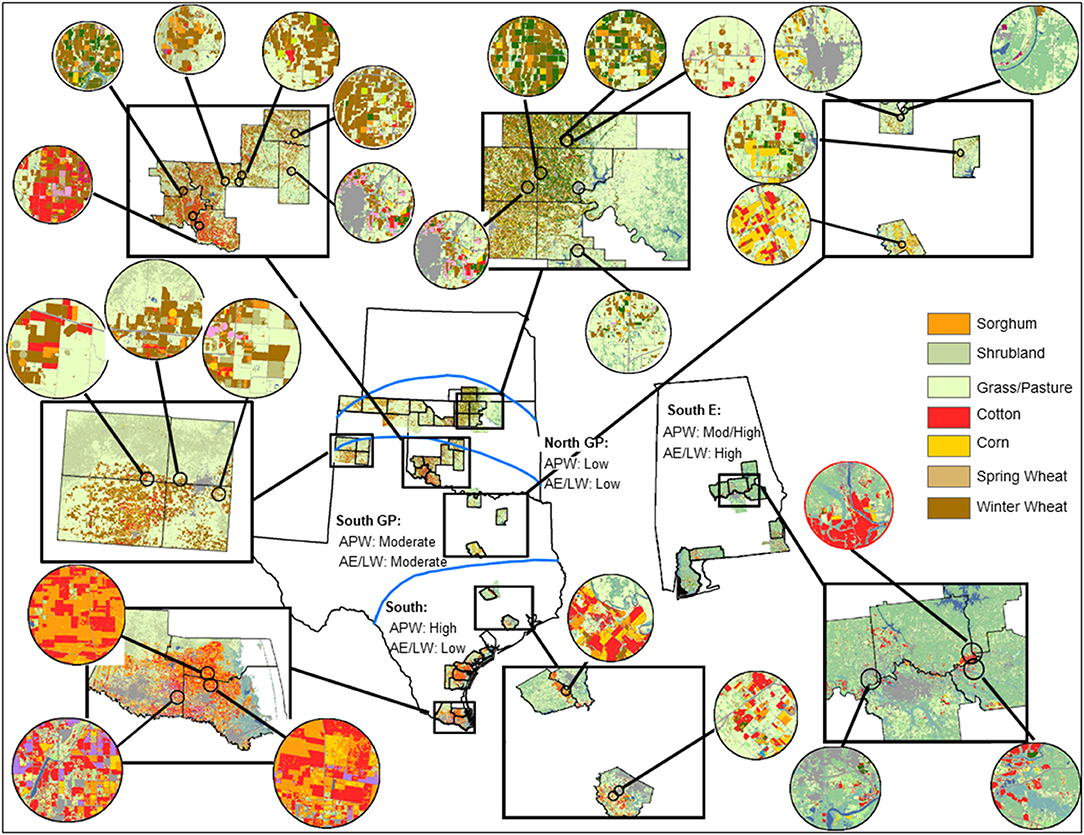
Figure 1. Areas sampled for Melanaphis sorghi and natural enemies across four regions (South, South GP, South E, North GP) generally following a latitudinal transition from subtropical, warm temperate, to temperate climates. Five kilometer radius buffers surrounding sampling locations (circle cut-outs) from counties visited (box cut-outs) are magnified to show the range of agro-landscape features encountered. Land cover symbology (see legend for color-coding) is taken from the website Cropscape for 2018 (41). Risk to M. sorghi (high to low) were assigned by expectations based on the aphid-plant-weather (APW) and aphid-enemy/landscape-weather mediated (AE/LW) risk scenarios (placed below the region labels). See text for rationale of risk assignments.
Sorghum fields sampled were in 40 counties within these four regions and were randomly selected from a list of probable M. sorghi-infested fields provided by local collaborators. Data were taken from multiple fields in each county. On average, per county and year, 3,073 leaves were inspected in 2.5 fields and eight sampling events (numbers of fields and sampling events are given in Table 1). Plant growth stage ranged from mid-vegetative growth through early grain fill stages of reproductive growth and was recorded as a whole field average (Supplementary Material 1). Sampling began when M. sorghi presence was confirmed. Average M. sorghi densities typically fell within the range of 20 and 70 M. sorghi per leaf, which bridged across unlikely to probable sorghum injury and risk to grain production [i.e., 40 M. sorghi per leaf is a commonly used indicator of expected economic injury (18)]. For each sampling event, M. sorghi and natural enemy taxa were counted on two leaves of each plant: the first green leaf toward the base of the plant and the uppermost unfurled leaf below the flag leaf (23). Plants were selected randomly, and no fewer than 60 leaves were inspected per sampling event. The length of inspection time was 20 min or more, with more time spent when aphid and natural enemies were common. For each field, first date of detection of M. sorghi was recorded and initiated two to five sampling events. Raw data were aggregated across sampling events and fields for each county and year, resulting in 87 data records used for analyses of this study (Table 1, Supplementary Material 1). Four data records of the South and South GP regions were derived from previous research (26).
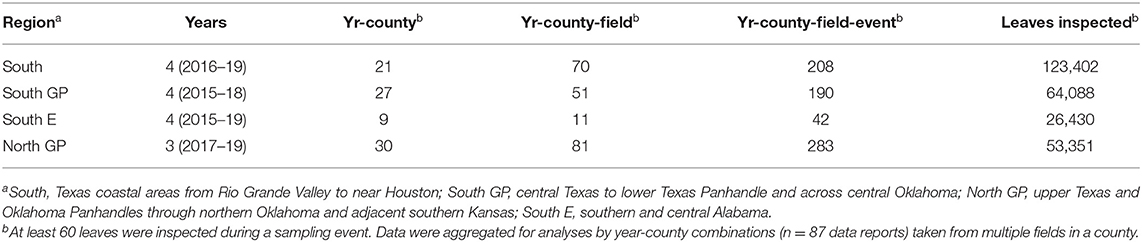
Table 1. Sampling history in four regions where Melanaphis sorghi and its natural enemies were identified and enumerated from 2015 to 2019.
Infrequent detections or differences in the relative proportions of natural enemy counts across taxa did not appear to be affected by sampling method biases. There were no significant differences of the relative proportions of each natural enemy taxa recovered using the two-leaf inspection method adopted by this study to maximize efficiencies compared with a whole plant destructive sampling method. The results (contingency table analyses (42) in Supplementary Material 2) did not reveal differences in the sensitivity of the two methods in detecting parasitoids (mummies) and predators (adults and larvae), or in detecting differences across lower taxa used in this study (see next section). Also, the comparative study focused on regional comparisons of natural enemy abundance and activity and not cross-taxa comparisons, further reducing the concern of sampling method bias across natural enemy taxa. Therefore, the two-leaf sampling method was considered satisfactory for the large region comparative study.
Melanaphis sorghi and Natural Enemy Taxa
Insects selected for analyses of the large-region comparison were M. sorghi, A. nigritus mummies, L. testaceipes mummies, lady beetle larvae, lady beetle adults, syrphid larvae, and lacewing (chrysopids and hemerobiids) larvae. Melanaphis sorghi was the dominant aphid species, with sporadic early season detections of R. maidis, S. graminum, and S. flava. The natural enemy taxa represented those previously identified from the U.S. and Mexico [e.g., (22–26)]. A species check list was generated for each region, with infrequently detected taxa limited to family-level classification. Periodically, starting in 2015, subsets of blue-black non-swollen aphid mummies were reared to adults and identified as A. nigritus based on comparison to voucher specimens [Texas A&M University Insect Collection, voucher #723 (26)]. Similarly, starting in 2017, subsets of light-brown swollen aphid mummies were reared to adults and identified as L. testaceipes based on taxonomic keys specific to parasitoids of cereal aphids (43). Using photographs and references in Maxson et al. (26), adult and larval lady beetles were identified to species during most sampling events, and syrphid and chrysopid larvae were identified to species in the South and South GP regions in 2015 and 2016.
Absolute abundance metrics for M. sorghi and natural enemy taxa were reported on a per leaf basis (density). A natural enemy activity metric was calculated as abundance per leaf divided by the number of live un-mummified aphids per leaf (and multiplied by 100 to aid presentation). For parasitoids, this activity metric provided a rough estimate of parasitism and was consistent with the predator activity metric. It can be recalculated to apparent field parasitism by including mummified aphids in the divisor, although this metric also underestimated parasitism because some un-mummified aphids were likely parasitized (44).
Agro-Landscape and Weather Metrics
Agro-landscape composition metrics were selected to represent the crop directly disrupted by the invasive pest (sorghum), and cropland, grassland, and shrubland known to harbor natural enemies of cereal aphids (30–33). To calculate the metrics, georeferenced cropland data layers from 2015 to 2019 corresponding to the 87 year-county data records were managed within a geographic information system (GIS) using ArcMap 10.7 [ESRI, Redlands, CA (45)]. Cropland data layers were downloaded in raster format by state (i.e., Alabama, Kansas, Oklahoma, and Texas) from the website Cropscape (41) and imported into GIS. Circular buffers (5 km radius) were centered on the approximate centroid of each individual field sampled (Figure 1). The 5 km radius was selected to balance preferences for smaller scales appropriate to activity of parasitoids of cereal aphids [e.g., from 2 to 3 km radius (32, 33)] and larger scales more appropriate for activity of predators of cereal aphids (31). Using zonal statistics (45), total numbers of pixels for selected landscape metrics were calculated for each buffer. Overlap of buffers was infrequent and minimal (Figure 1). The agro-landscape composition metrics considered were percent sorghum (percent of pixels classified as sorghum) and percent cropland (percent of pixels associated with corn, cotton, sorghum, other small grains, legumes, and non-alfalfa hays). Two other metrics consisted of lesser managed lands: percent grassland-pasture (percent of pixels associated with various grass species and herbaceous vegetation managed for grazing and hay crops) and percent shrubland (percent of pixels associated with woody shrubs <5 m in height including young or stressed trees and interspersed with grasses) (46).
Weather metrics were calculated using data archived from the nearest weather station to each field and corresponding to each sampling event from 2015 to 2019 (47). Weather stations accessed were commonly at county airports, recording above ground (3 to 10 m) temperature. Data for analyses were calculated as averages from the first to last sampling event: minimum temperature (°C) averaged across daily lowest temperatures, maximum temperature averaged across from daily highest temperatures, and rainfall (cm) summed across daily amount of rainfall. The agro-landscape and weather metrics were merge-matched with the 87 year-county data records for further analysis (Supplementary Material 1).
Data Analysis
Analysis of variance (Proc GLM, 48) was used to detect differences in the insect abundance and activity metrics across the four regions. Year was set as a blocking term to aid in partitioning yearly variation associated with weather and insect abundance that may affect ability to detect regional differences. Replication (i.e., data records) ranged from 21 to 30 for three regions (Table 1). Fewer year-county records were available in the South E region (n = 9), but the region was retained given its contrast with the other regions and the comparable sampling effort that occurred within yr-county records of all regions (Table 1, Figure 1). Tukey's means separation test was used to compare regions when significant in an ANOVA (P < 0.05, 48). The agro-landscape and weather conditions were compared across regions with the same procedure. Data transformations appropriate for count data with zeroes (square root of value + 0.5) and proportion data (arcsine of the square root of value) were done before analyses. Transformations selected were standard to compensate for variation from the ANOVA normality assumption associated with these measurements (48). Untransformed means were presented.
Correlation analyses for data records in each region were conducted [Proc Corr, (49)]. Using transformed data to address possible deviations from normality, Pearson correlations of natural enemy-M. sorghi abundance were used to assess numerical response of selected natural enemy taxa to M. sorghi abundance. The natural enemy activity correlations with agro-landscape and weather metrics were used to explore potential mediation of natural enemy activity by landscape and weather conditions. Five probability levels (P < 0.20, P < 0.10, P < 0.05, P < 0.01, P < 0.001) were used for detecting patterns and contrasts of correlations across regions. The Holm-Bonferroni method (50) was used to sequentially adjust each calculated probability value to compare to the five set critical probability levels for the correlations across regions where each natural enemy taxon occurred (i.e., four correlations of four regions, except the case of L. testaceipes that was not detected in one region). Of interest were the natural enemy abundance-M. sorghi abundance correlations, natural enemy activity-agro-landscape correlations, and natural enemy activity-weather correlations. In lieu of structured experiments to test for potential interactions and collinearity of the agro-landscape and weather variables, the five probability levels allowed for flexibility in considering variables for further multivariate regression model activities (48) for regions of special interest based on the outcomes of regional differences and risk scenario comparison of this study.
Results
Melanaphis sorghi and Natural Enemy Taxa Across Regions
Melanaphis sorghi was detected in all regions and years. Presence of the family-level taxa and species of natural enemies were nearly identical across the four regions (Table 2). Two primary endoparasitoids and four of six coccinellid species were common. Syrphidae, Chrysopidae, and Hemerobiidae were detected in all regions, with eight species common in the South and South GP regions where species were identified (Table 2). These taxa were detected each year. In contrast to the nearly identical natural enemy complex across regions, selected insect abundance and activity varied across years and regions. Yearly variation in abundance of A. nigritus mummies and larvae of syrphids and lacewings was detected (the ANOVAs for the three taxa were significant, for the three F > 5.3; d.f. = 4, 70; P < 0.001), while other abundance metrics and all natural enemy activity metrics adjusting for M. sorghi density did not experience significant year-to-year variation (P > 0.05) (description of year, region, and field identifiers are given in Supplementary Material 1). Although yearly variation was modest, the year term was retained in the ANOVAs to aid partitioning year-to-year variation from the error term used to test for regional differences and to maintain a common analysis structure for all measurements.
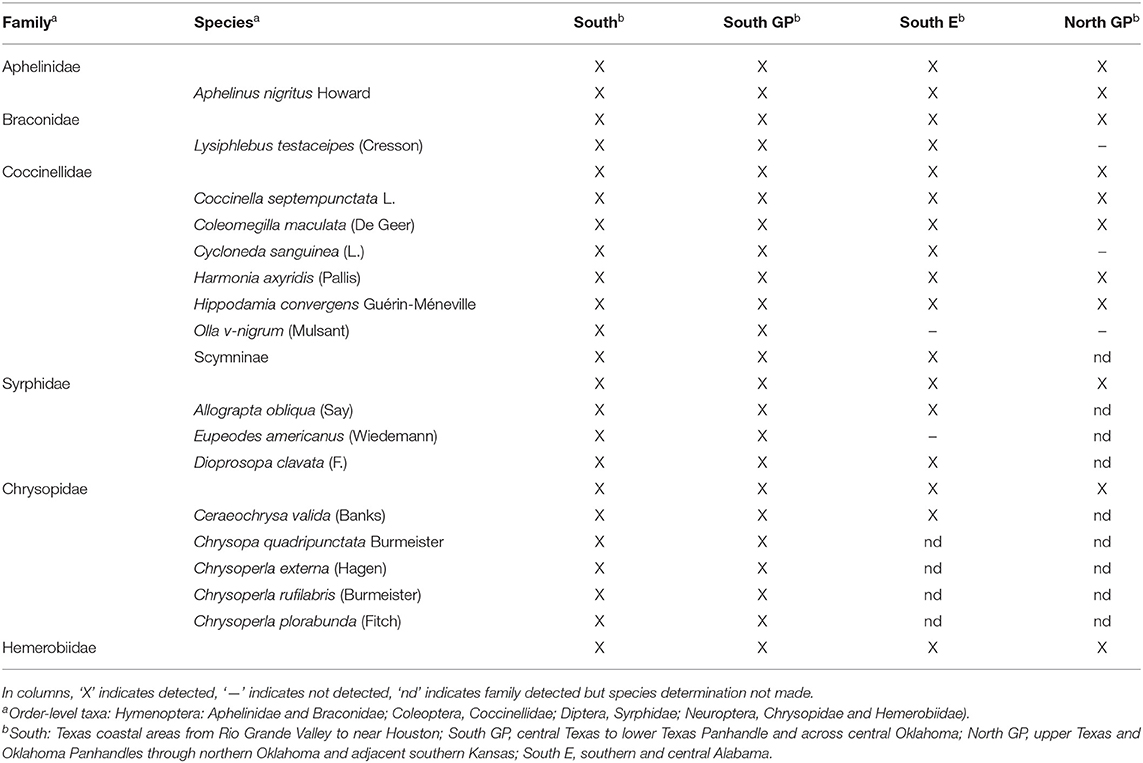
Table 2. Natural enemy taxa present in four regions where Melanaphis sorghi and its natural enemies were identified to family and species when possible, from 2015 to 2019.
Measuring abundance, significant differences across regions were detected for M. sorghi (F = 7.08; d.f. = 3, 79; P = 0.0003) and A. nigritus (F = 6.28; d.f. = 3, 79; P = 0.0007) but differences were not detected for other taxa (P > 0.05) (Figure 2). Abundances of A. nigritus, L. testaceipes, lady beetles, and syrphids were two- to over 10-fold greater in the South region than other regions, although variation was high and only A. nigritus abundance in the South was significantly greater than in other regions (Figure 2). Melanaphis sorghi was most abundant in the South E region where natural enemy abundance, except A. nigritus, was relatively low (Figure 2).
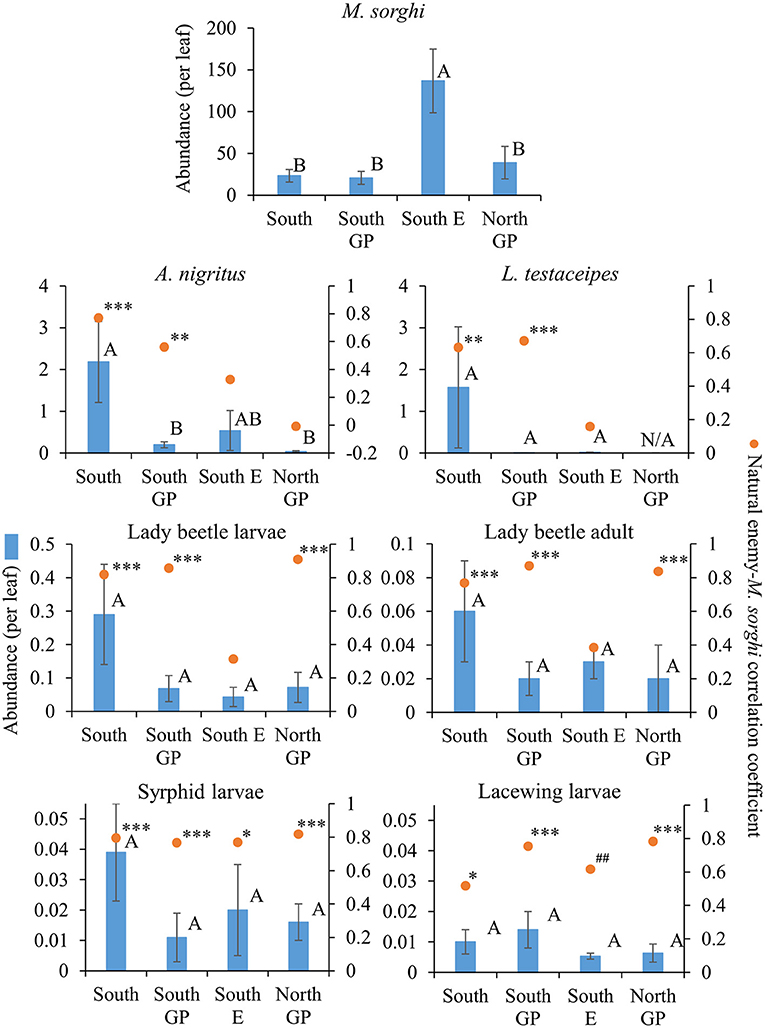
Figure 2. Average (±SEM) abundance (per leaf) (blue bars) of Melanaphis sorghi and natural enemies in each of the four regions from 2015 to 2019. Lack of significant differences across regions indicated by common letters (α = 0.05, Tukey's mean separation test, each taxon analyzed separately). Dots represent natural enemy abundance-M. sorghi abundance correlations, using five probability levels (‘#’ = P < 0.20, ‘##’ = P < 0.10, ‘*’ = P < 0.05, ‘**’ = P < 0.01, ‘***’ = P < 0.001) for pattern detection across regions. The Holm-Bonferroni method (50) was used to adjust the calculated probabilities to compare to these levels across the four regions. See Figure 1 for geographical boundaries of the regions.
Natural enemy activity (adjusted for M. sorghi abundance) supported that the parasitoids, and selected predators to a lesser extent, responded numerically to M. sorghi differently across regions. Specifically, the activity metric of the two parasitoids and larvae of lady beetles and syrphids varied across regions (the ANOVAs for the four taxa were significant, for the four F > 2.8; d.f. = 3, 79; P < 0.05). The activity metrics of the two parasitoids (A. nigritus, L. testaceipes) (Figure 3) and lady beetle larvae (Figure 4) were greatest in the South region, and syrphid activity was relatively high in the South region but did not differ significantly from the North GP region (Figure 5). Related, high field parasitism rates (35 to 90%) attributed to A. nigritus and L. testaceipes were periodically observed in the South region (MJB and AMF, pers. obs.). High variance relative to the mean was observed for L. testaceipes in the South region (Figure 2), where occasional L. testaceipes parasitism exceeding 90% was observed in 2017 onward (MJB and AMF, pers. obs.).
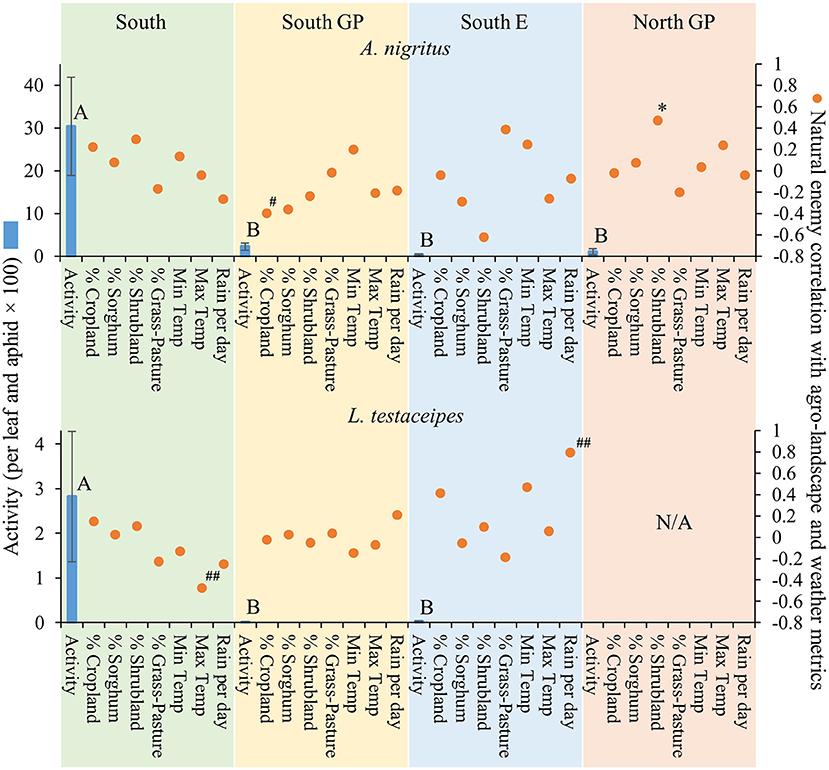
Figure 3. Average (±SEM) activity of Aphelinus nigritus and Lysiphlebus testaceipes (per leaf and aphid x 100) (blue bars) in each of the four regions from 2015 to 2019. Lack of significant differences across regions indicated by common letters (α = 0.05, Tukey's mean separation test, each taxon analyzed separately). Dots represent natural enemy activity-agro-landscape correlations, and natural enemy activity-weather correlations, using five probability levels (‘#’ = P < 0.20, ‘##’ = P < 0.10, ‘*’ = P < 0.05, ‘**’ = P < 0.01, ‘***’ = P < 0.001) for pattern detection across regions. The Holm-Bonferroni method (50) was used to adjust the calculated probabilities to compare to these levels across the regions. See Figure 1 for geographical boundaries of the four regions.
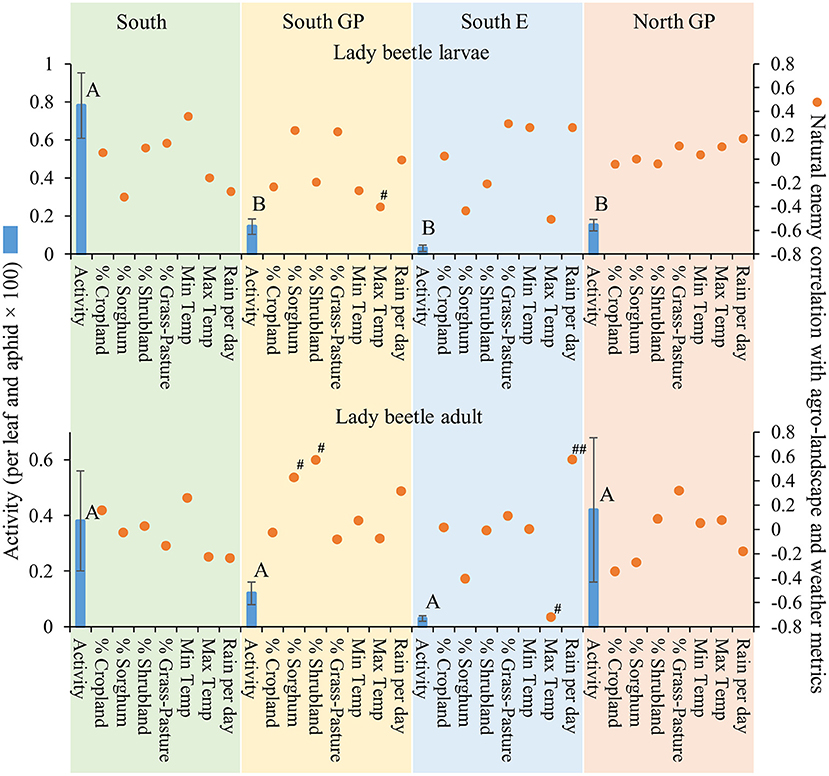
Figure 4. Average (±SEM) activity of lady beetle larvae and adults (per leaf and aphid x 100) (blue bars) in each of the four regions from 2015 to 2019. Lack of significant differences across regions indicated by common letters (α = 0.05, Tukey's mean separation test, each taxon analyzed separately). Dots represent natural enemy activity-agro-landscape correlations, and natural enemy activity-weather correlations, using five probability levels (‘#’ = P < 0.20, ‘##’ = P < 0.10, ‘*’ = P < 0.05, ‘**’ = P < 0.01, ‘***’ = P < 0.001) for pattern detection across regions. The Holm-Bonferroni method (50) was used to adjust the calculated probabilities to compare to these levels across the regions. See Figure 1 for geographical boundaries of the four regions.
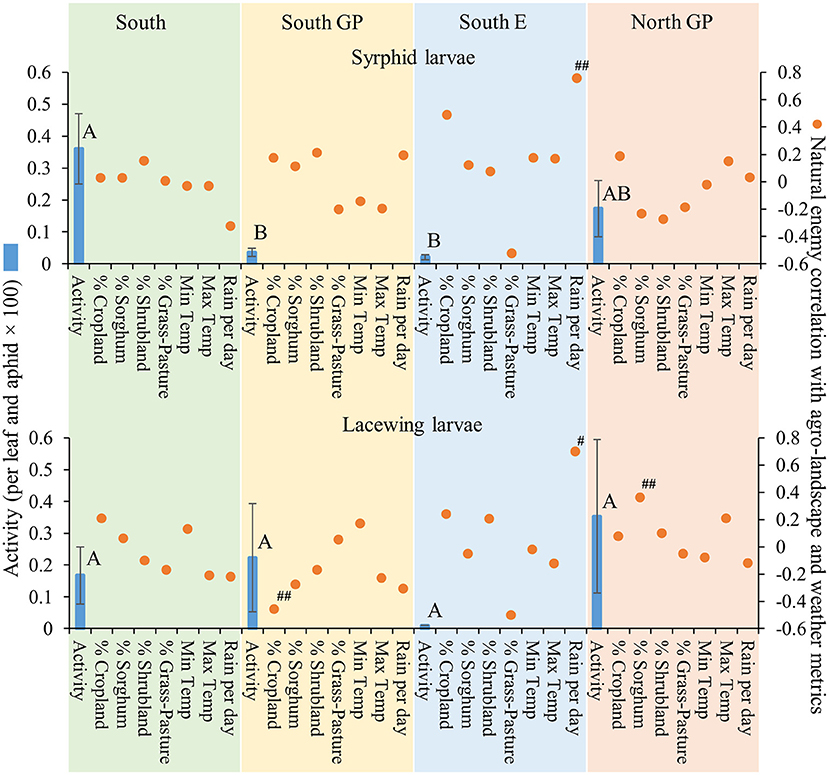
Figure 5. Average (±SEM) activity of syrphid and lacewing larvae (per leaf and aphid x 100) (blue bars) in each of the four regions from 2015 to 2019. Lack of significant differences across regions indicated by common letters (α = 0.05, Tukey's mean separation test, each taxon analyzed separately). Dots represent natural enemy activity-agro-landscape correlations, and natural enemy activity-weather correlations, using five probability levels (‘#’ = P < 0.20, ‘##’ = P < 0.10, ‘*’ = P < 0.05, ‘**’ = P < 0.01, ‘***’ = P < 0.001) for pattern detection across regions. The Holm-Bonferroni method (50) was used to adjust the calculated probabilities to compare to these levels across the regions. See Figure 1 for geographical boundaries of the four regions.
Enemy-M. sorghi Associations Mediated by Agro-Landscape and Weather Conditions
Relevant to interpreting the correlation analyses, significant differences were detected across regions for the agro-landscape and weather metrics (the ANOVAs for the seven metrics were significant, for the seven F > 3.70; d.f. = 3, 79; P < 0.02) (Table 3). Year-to-year variation in minimum temperature was detected (F = 4.82; d.f. = 4, 70; P = 0.0017), while no significant year-to-year variation in other agro-landscape and weather metrics was detected (P > 0.05).
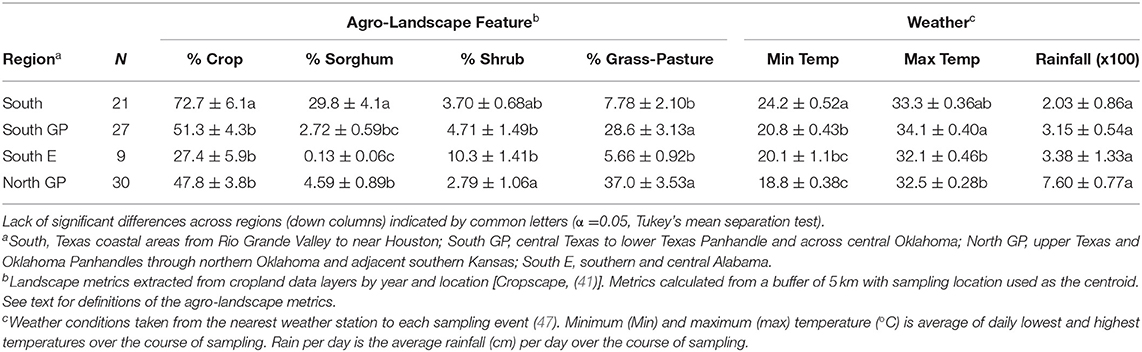
Table 3. Average agro-landscape features and weather conditions experienced by Melanaphis sorghi and natural enemies across four regions, from 2015 to 2019.
As indicated by percent composition of the 5 km buffers of the sampling locations, sorghum and cropland in general were more concentrated in the South region, followed by the two Great Plains regions. Cropland was a substantial landscape component in the South E region, but sorghum was less represented (Table 3 and Figure 1). In contrast, shrubland was common in the South E region, and percent grassland-pasture was about four- to five-fold higher in the South GP and North GP regions than the other two regions. These metrics generally aligned with the historical cropping patterns of the areas sampled (1). Differences were detected for weather metrics across the regions and generally followed a latitudinal gradient of warmer minimum and greater maximum daily temperatures of the subtropical-influenced regions (South and South E regions) compared with the two temperate-influenced Great Plains regions (South GP and North GP regions) (Table 3). The South GP region reached relatively high maximum temperatures as likely influenced by inland weather patterns where sampling occurred. Average rainfall across sampling events did not differ across the regions, although variable rainfall occurred in the South E region as reflected in the high variance relative to the mean (about 40% of the mean) (Table 3).
Different correlation patterns of natural enemy abundance with M. sorghi abundance, and natural enemy activity with agro-landscape and weather conditions, were observed across regions. The South region experienced low average M. sorghi densities (~23 M. sorghi per leaf) (Figure 2) where activities of both parasitoids and lady beetle larvae were high (Figures 3, 4). All natural enemy-M. sorghi abundance correlations were significant and positive using the Holm-Bonferroni adjustment of probability values (Figure 2). In this region, M. sorghi-natural enemy interactions appeared to be functioning independent of or sufficiently robust to mask any agro-landscape and weather influences, as indicated by the lack of significant correlations of natural enemy activity with these metrics (Figures 3–5). In the South GP region, M. sorghi abundance was also low (~21 M. sorghi per leaf), and all natural enemy-M. sorghi abundance correlations were significant and positive in sign (Figure 2). The potential influence of agro-landscape composition on parasitism and predation of selected taxa was more apparent in this region: A. nigritus (Figure 3) and lacewing larvae (Figure 5) were negatively correlated with percent cropland, while lady beetle adults (Figure 4) were negatively correlated with percent shrubland and sorghum.
In contrast, M. sorghi abundance approached or exceeded the 40 M. sorghi per leaf indicator of economic concern [i.e., the economic threshold (18)] in the North GP and South E regions (~38 and ~130 M. sorghi per leaf, respectively) (Figure 2). In the North GP region, significant natural enemy-M. sorghi abundance correlations were limited to predators (Figure 2). In this region, natural enemy activity mediated by agro-landscape conditions was detected: one significant positive correlation of A. nigritus activity with percent shrubland (Figure 3) and another of lacewing larval activity with percent sorghum (Figure 5). Lysiphlebus testaceipes was not observed parasitizing M. sorghi in the North GP region (Figure 2). In the South E region, natural enemy abundance and activity were generally low (Figure 2 through Figure 5). Significant correlations of natural enemy-M. sorghi abundance were limited to syrphid and lacewing larvae (Figure 2). In this region, natural enemy activity was mediated in part by weather. Increasing rainfall was associated with greater L. testaceipes parasitism (Figure 3), lady beetle adults (Figure 4), and syrphid and lacewing activity (Figure 5). Increasing maximum temperature was associated with decreasing lady beetle adult activity (Figure 4). In contrast, only two significant natural enemy-weather correlations of a total of 51 were detected in the other regions (Figure 2 through Figure 5).
Discussion
Comparison of Natural Enemy Data to Previous Observations
The parasitoid and predator species complex in the South region and two Great Plains regions did not change from those previously reported in central and south Texas (23, 26) and overlapped and added to those observed in Kansas (22). A few additional braconid parasitoid species, such as Aphidius spp., and several additional species of coccinellids and syrphids were reported farther south in northeastern to westcentral Mexico (24, 25, 39, 51). In the South E region, family-level taxa of predators reported in this study were consistent with those previously reported (38, 52). Overall, the natural enemy complex was composed of a broad array of taxa observed across 5 years and four regions that were present over much of the geographic range of M. sorghi on sorghum in North America.
Regional differences in natural enemy abundance and activity helped place in perspective earlier reporting of parasitism and predation of M. sorghi. The high A. nigritus activity metric of the South region (Figure 3) equated to a study-long average of ca. 23% apparent percent parasitism and was coupled with low M. sorghi abundance (Figure 2). This activity was comparable to 31% parasitism of M. sorghi attributed to several species in central Mexico (24). Variable parasitoid abundance and activity (Figures 2, 3) were consistent with episodes of high A. nigritus and L. testaceipes parasitism in the South region (MJB and AMF, pers. obs.). Colares et al. (22) in Kansas also detected M. sorghi suppression by Aphelinus spp. using M. sorghi-infested potted sorghum. They did not observe L. testaceipes parasitism of M. sorghi, which corresponded to lack of L. testaceipes parasitism of M. sorghi observed in the North GP region (Figure 2). It is notable that L. testaceipes was observed parasitizing S. graminum and R. maidis that occurred sporadically in the North GP region (NCE, pers. obs.), but M. sorghi tan mummies likely indicative of the braconid L. testaceipes were not detected (Figure 2). The very low parasitoid and predator activities in the South E region across a wide range of aphid densities were consistent with little to no parasitism previously observed in Georgia and Kentucky (38, 52). These findings supported the interpretation that natural enemies were contributing to M. sorghi suppression in the South region, to a lesser extent in the two Great Plains regions, and not in the South E region.
Regional Comparisons and Sorghum Risk Scenarios
Soon after the M. sorghi invasion, ecological modeling and qualitative evaluations of sorghum risk initially focused on aphid life history, extent of sorghum cultivation and susceptibility to M. sorghi, and weather (APW risk assessment scenario). This scenario was useful in evaluating geographic and annual range expansion of M. sorghi (19, 20). The more comprehensive AE/LW risk scenario proposed here drew from the enemy-free space hypothesis (13) and the allied biotic interference hypothesis in the biological control literature (53) to explain M. sorghi outbreaks when natural enemies were lacking, as well as reduced risk of outbreaks when natural enemies numerically responded to M. sorghi (54). The AE/LW risk scenario also incorporated potential influence of weather on M. sorghi-natural enemy interactions based in part on climate suitability (14) among the different regions. Last, bottom-up forces (11, 12) were considered within the context of agro-landscape composition elements (i.e., sorghum, crops collectively, and less managed grassland/shrubland) including serving as harborage for natural enemies of cereal aphids in the North American Great Plains and elsewhere (6, 29, 32, 33, 55, 56).
Applied to the current study, the AE/LW risk scenario accounted for natural enemy abundance and activity that was highest in the South region, functioned well across agro-landscape and weather conditions, and was accompanied by low M. sorghi abundance (~23 M. sorghi per leaf) (Figure 2 through Figure 5). These findings and observations that the South region experienced few M. sorghi outbreaks during the study (MJB, pers. obs.) were inconsistent with the APW risk scenario that predicted high sorghum risk (Figure 1). Positive correlations of natural enemy-M. sorghi abundance were detected for each natural enemy taxon in the South and South GP regions where M. sorghi abundance was low (~20 M. sorghi per leaf) (Figure 2). Also consistent with the AE/LW risk scenario, natural enemy activity of selected taxa appeared to be mediated by landscape composition particularly in the South GP region where M. sorghi abundance was low, and by weather particularly in the South E region where M. sorghi abundance was highest of the regions (~136 aphids/leaf) (Table 3 and Figure 3 through Figure 5). Although risk expectations of the two scenarios were similar in the two Great Plains regions and the South E region, potential mediation of M. sorghi-natural enemy interactions by landscape composition and weather conditions was revealed by considering the AE/LW risk scenario. Broadly, the AE/LW risk scenario appeared suited, and essential in the South region, in assessing risk of pest outbreaks on a regional scale. These findings set the stage for producing risk models with agroecosystem response estimates under current (i.e., extant regions of this study) and changing conditions (i.e., anticipated under climate change) considered in the AE/LW risk scenario.
Prior knowledge of natural enemies of cereal aphids and agro-landscape influences also supported the idea that natural enemies as top-down forces in M. sorghi regulation contribute to shaping sorghum agroecosystem response to the M. sorghi invasion, as seen in past cereal aphid invasions in the North American Great Plains (6). On wheat, parasitism by L. testaceipes was sufficient to prevent populations of S. graminum from reaching damaging population levels (57), and parasitism was positively correlated with plant diversity (cropland and grassland/pasture) (32). Parasitism of D. noxia by Aphelinus albipodus Hayat and Fatima (Hymenoptera: Aphelinidae) was associated with declines in aphid abundance, especially where wheat production was nested in a relatively diverse cropping system (30) and planted with sunflower in a strip cropping system (55). Outside of the Great Plains, Thies et al. (33) studied parasitoids of cereal aphids affected by landscape diversity in Europe and concluded that increasing landscape diversity was associated with both increasing parasitoid abundance and increasing cereal aphid abundance.
From a perspective of managing pests in individual fields, parasitism (L. testaceipes mummy detection) has been incorporated into S. graminum monitoring and management protocols in Oklahoma (57). From a regional or areawide pest management perspective, this study adds to the evidence that parasitism and predation by resident natural enemies are well suited to aphid management in the low-input large-scale cereal agroecosystem of the North American Great Plains (6, 29, 30). For M. sorghi, combining an agroecosystem service of parasitism and predation with use of sorghum resistant to M. sorghi that does not deter parasitism and predation (23, 26) is particularly important where risk is more persistent, variable, or prone to variable natural enemy activity as mediated by agro-landscape and weather conditions (e.g., South E and South GP regions). In such cases, variable top-down forces provided by natural enemies benefit from the addition of bottom-up forces of sorghum resistant to M. sorghi, as well as strategic use of insecticides with low toxicity to natural enemies (26, 58).
Considerations for Agroecosystem Response Modeling
This region-scale pest risk assessment approach of gathering insect sampling data for considering differences in M. sorghi outbreak risk warrants further effort, such as how to best utilize large region trends for addressing risk assessment needs at smaller spatial scales. To this end, correlation patterns identified of potential interest in the large region comparisons may be explored further by considering new or component data used to derive the metrics of this study. For example, agro-landscape metrics not considered in the current study, such as configuration of landscape elements (e.g., edge density and plant diversity metrics) and scale-suitability of the array of natural enemies, may help resolve the correlation patterns of mixed sign that were occasionally detected (i.e., natural enemy activity correlated with landscape composition metrics as seen in the south GP region, Figure 3 through Figure 5) (59). These metrics may be further explored in a GIS using the agro-landscape data layers of the current study (Figure 1). Further multivariate regression modeling, such as stepwise regression or regression modeling with collinearity testing, may generate estimates of the degree of influence of natural enemies under varying agro-landscape and weather conditions considered in the AE/LW risk scenario. We note that collinearity among variables may affect sensitivity of model distinction between regions because of its influence on coefficients and model probability. But for models selected within any region of special interest, multicollinearity is less relevant to mean responses of the dependent variable and prediction (i.e., aphid and natural enemy abundance, and natural enemy activity) (48). The variable selection process of stepwise regression provides additional safeguards (48). Should large sets of landscape and weather metrics be added, such as landscape configuration and diversity metrics, other multivariate techniques such as principal component analysis may be advised to obtain a synthetic smaller set of variables that retains the essential information of the large data set (60).
Additionally, Chaplin-Kramer et al. (56) noted the value and under-use of time sequences of pest and natural enemy monitoring data to make inferences on suppression by comparing population growth rates of the pest and natural enemies. Our use of the current data set averaged this time sequence feature (Table 1) which facilitated the four region comparison and the contrast of the two risk scenarios. For an analytical focus on suppression by natural enemies over time, the component data from all fields and sampling events are available (Supplementary Material 1). Such analyses should consider the possibility of autocorrelation of data across the time sequences and range of the time sequences in the data set. Last, the region-scale risk assessment approach of this study can help guide and prioritize companion structured experimentation. For example, natural enemy exclusion experiments conducted in the South region estimated up to 90% suppression of M. sorghi by A. nigritus and coccinellids (61).
Perspectives in Regional Risk Assessment and Agroecosystem Response to Invaders
Previous ecological modeling and qualitative sorghum risk evaluations focused on the APW risk scenario for M. sorghi and did not include top-down constraints provided by natural enemies. The focus on climate suitability (14), resource availability, and defense-free space afforded by growing sorghums with high yield potential but susceptible to M. sorghi [i.e., widespread cultivation of sorghums susceptible to M. sorghi (11, 12)] and mechanisms of rapid range expansion (19, 20) had value in evaluating geographic and annual range expansion of M. sorghi and the overall initial success of the M. sorghi invasion in North America. Regarding the allied interests of invasive species ecology and biological control (53), the AE/LW risk scenario proposed here added the top-down force of natural enemies as a key element of biotic resistance to M. sorghi (13, 17) to explain the region-specific risk of M. sorghi in the context of potential influence of agro-landscape and weather conditions across a near-continent scale of the M. sorghi invasion.
These findings have implications for climate change affecting plant-aphid-natural enemy interactions that result in change to agroecosystem-based pest management. Triltsch et al. (62) projected that a temperature increase of 3°C would result in reduction of cereal aphid infestations of wheat due to quicker wheat seed maturation, increased predator rates, and lower aphid reproduction rate. Alternatively, parasitism by some species may be adversely affected or remain unchanged while an increase in the host aphid's reproductive rate increases, indicating the species dependency of such predictions (63). The presence of M. sorghi across subtropical and temperate latitudes in North America allows for proposing risk hypotheses as temperate zones of this study warm. Higher M. sorghi abundance may be expected under the APW risk scenario based on warming trend predictions of climate change in the more northern temperate zone range of M. sorghi. But the potential of natural enemy influences of warming temperature in the context of landscape structure provided under the AE/LW sorghum risk scenario should be considered given species dependent effects of warming temperatures (63). Given the similar natural enemy complex across the latitudinal gradient of this study, sorghum-M. sorghi-natural enemy dynamics of the South GP and potentially North GP regions may shift toward lower M. sorghi abundance and higher natural enemy activity as seen in the subtropical influenced South region (Figure 2). One consideration is lag time of climate change effects on landscape structure, particularly managed lands, although such change may have long-term influence.
The AE/LW risk scenario appears more suitable now that the expansion area of M. sorghi appears stable (6). At least in the South region of this study and farther south into Mexico (38), the AE/LW risk scenario appears essential to account for low M. sorghi abundance and high natural enemy activity, where higher M. sorghi abundance and sorghum risk were expected under the APW risk scenario given its focus on climate suitability of sorghum and M. sorghi. Large region trends from the AE/LW risk scenarios may be used for general guidance in recommending supportive management inputs to complement the agroecosystem service of parasitism and predation (Figure 1). Further refinement and quantification of outcomes of the AE/LW sorghum risk scenario may be aided by deep analysis of the decomposed year-county data records, and further modeling of agroecosystem response under current and changing conditions considered in the AE/LW risk scenario. These data may also contribute to meta-analysis efforts that focus on agroecosystem resilience to invasive species [e.g., (56, 64)] and understanding biotic resistance to insect invasions within the context of general influence of abiotic factors (12, 13, 17) that vary across large-scale agroecosystems. Based on this case study, the AE/LW risk scenario appears suited and flexible in assessing risk of pest outbreaks regionally and improves understanding agroecosystem response to invasive insect species where natural enemies vary in their abundance and interaction with M. sorghi along gradients of agro-landscape and weather conditions.
Data Availability Statement
The original contributions presented in the study are included in the article Supplementary Material, further inquiries can be directed to the corresponding authors.
Author Contributions
MB and NE contributed to conception and design of the study. MB, NE, IE, AJ, AF, and BE organized the database. MB performed the statistical analysis and wrote the first draft of the manuscript. All authors contributed to manuscript revision, read, and approved the submitted version.
Funding
We thank the United Sorghum Checkoff Program for financial support. The USDA Agricultural Research Service also provided funding through the Areawide Pest Management Program, project 3072-22000 (017-03-S, 017-05-S, and 017-04-S). Funders evaluated and provided comments on application for funding. This research was conducted partly under the umbrella of USDA NIFA Hatch project TEX0-2-9394 assigned to MB at Texas A&M AgriLife Research.
Conflict of Interest
JG was employed by Syngenta Crop Protection.
The remaining authors declare that the research was conducted in the absence of any commercial or financial relationships that could be construed as a potential conflict of interest.
Publisher's Note
All claims expressed in this article are solely those of the authors and do not necessarily represent those of their affiliated organizations, or those of the publisher, the editors and the reviewers. Any product that may be evaluated in this article, or claim that may be made by its manufacturer, is not guaranteed or endorsed by the publisher.
Acknowledgments
Special thank to those helping in insect sampling and identification in the field: D. Anderson, K. Fuhrmann, T. Ahrens, L. Pruter, L. DeLeon, A. Reyes, and J. Glover (Corpus Christi, TX), M. Wleczyk, Z. Shihabuddin, M. Killian, and S. Fennessy (Rosenberg, TX), E. Huff and S.B. Neupane (Amarillo, TX), and C. Ray (Auburn, AL). Mention of trade names or commercial products in this publication is solely for the purpose of providing specific information and does not imply recommendation or endorsement by the USDA. USDA is an equal opportunity provider and employer.
Supplementary Material
The Supplementary Material for this article can be found online at: https://www.frontiersin.org/articles/10.3389/finsc.2022.830997/full#supplementary-material
References
1. (USDA NASS) U.S. Department of Agriculture, National Agricultural Statistics Service. 2016. Crop production, 2015 summary, Cr Pr 2-1. Available online at: https://usda.library.cornell.edu/concern/publications/k3569432s (accessed September 28, 2021).
2. Bowling RD, Brewer MJ, Kerns DL, Gordy J, Seiter N, Elliott NE, et al. Sugarcane aphid (Hemiptera: Aphididae): a new pest on sorghum in North America. J Integr Pest Manag. (2016) 7:12. doi: 10.1093/jipm/pmw011
3. Harris-Shultz K, Brewer MJ, Wadl PA Ni X, Wang H A. sugarcane aphid ‘super-clone’ predominates on sorghum and johnsongrass from four US states. Crop Sci. (2018) 58:2533–41. doi: 10.2135/cropsci2018.03.0151
4. Nibouche S, Costet L, Holt JR, Jacobson A, Pekarcik A, Sadeyen J, et al. Invasion of sorghum in the Americas by a new sugarcane aphid (Melanaphis sacchari) superclone. PLoS ONE. (2018) 13:e0196124. doi: 10.1371/journal.pone.0196124
5. Nibouche S, Costet L, Medina RF, Holt JR, Sadeyen J, Zoogones A-S, et al. Morphometric and molecular discrimination of the sugarcane aphid, Melanaphis sacchari, (Zehntner, 1897) and the sorghum aphid Melanaphis sorghi (Theobald, 1904). PLoS ONE. (2021) 16:e0241881. doi: 10.1371/journal.pone.0241881
6. Brewer MJ, Peairs FB, Elliott NC. Invasive cereal aphids of North America: ecology and pest management. Annu Rev Entomol. (2019) 64:73–93. doi: 10.1146/annurev-ento-011118-111838
7. Blackman RL, Eastop VF. Aphids on the World's Crops: An Identification and Information Guide. New York: John Wiley & Sons. (1984)
8. Singh BU, Padmaja PG, Seetharama N. Biology and management of the sugarcane aphid, Melanaphis sacchari (Zehntner) (Homoptera: Aphididae), in sorghum: a review. Crop Prot. (2004) 23:739–55. doi: 10.1016/j.cropro.2004.01.004
9. Harris-Shultz K, Ni X, Wadl PA, Wang X, Wang H, Wang H. Microsatellite markers reveal a predominant sugarcane aphid (Homoptera: Aphididae) clone is found on sorghum in seven states and one territory of the USA. Crop Sci. (2017) 57:2064–72. doi: 10.2135/cropsci2016.12.1010
10. Davis MA, Thompson K. Eight ways to be a colonizer; two ways to be an invader: a proposed nomenclature scheme for invasion. Bull Ecol Soc Am. (2000) 81: 226–230. Available online at: http://www.jstor.org/stable/20168448
11. Sher AA, Hyatt L. The disturbed resource-flux invasion matrix: a new framework for patterns of plant invasion. Biol Invasions. (1999) 1:107–14. doi: 10.1023/A:1010050420466
12. Gandhi KJK, Herms DA. Direct and indirect effects of alien insect herbivores on ecological processes and interactions in forests of eastern North America. Biol Invasions. (2010) 12:389–405. doi: 10.1007/s10530-009-9627-9
13. Jeffries MJ, Lawton JH. Enemy free space and the structure of ecological communities. Biol J Linn Soc. (1984) 23:269–86. doi: 10.1111/j.1095-8312.1984.tb00145.x
14. Barbet-Massin M, Rome Q, Villemant C, Courchamp R. Can species distribution models really predict the expansion of invasive species? PLoS ONE. (2018) 13:e0193085. doi: 10.1371/journal.pone.0193085
15. Lockwood JL, Cassey P, Blackburn T. The role of propagule pressure in explaining species invasions. Trends Ecol Evol. (2005) 20:223–8. doi: 10.1016/j.tree.2005.02.004
16. Jeschke JM. General hypotheses in invasion ecology. Divers Distribut. (2014) 20:1229–34. doi: 10.1111/ddi.12258
17. Levine JM, Adler PB, Yelenik SG. A meta-analysis of biotic resistance to exotic plant invasions. Ecol Lett. (2004) 7: 975–989. doi: 10.1111/j.1461-0248.2004.00657.x
18. Gordy JW, Brewer MJ, Bowling RD, Buntin GD, Seiter NJ, Kerns DL, et al. Development of economic thresholds for sugarcane aphid (Hemiptera: Aphididae) in susceptible grain sorghum hybrids. J Econ Entomol. (2019) 112:1251–9. doi: 10.1093/jee/toz028
19. Wang HH, Grant WE, Elliott NC, Brewer MJ, Koralewshi TE, Westbrook JK, et al. Integrated modelling of the life cycle and aeroecology of wind-borne pests in temporally variable spatially heterogeneous environment. Ecol Modelling. (2019) 399:23–38. doi: 10.1016/j.ecolmodel.2019.02.014
20. Koralewski TE, Wang HH, Grant WE, Brewer MJ, Elliott NC, Westbrook JK, et al. Integrating models of atmospheric dispersion and crop-pest dynamics: linking detection of local aphid infestations to forecasts of region-wide invasion of cereal crops. Ann Entomol Soc Amer. (2020) 113:79–87. doi: 10.1093/aesa/saz047
21. Michels GJ, Burd JD IPM. Case studies: sorghum. In: H van Emden and R Harrington, editors. Aphids as Crop Pests CAB. Oxfordshire, UK. (2007) pp. 627–38. doi: 10.1079/9780851998190.0627
22. Colares F, Michaud JP, Bain CL. Torres JB. Recruitment of aphidophagous arthropods to sorghum plants infested with Melanaphis sacchari and Schizaphis graminum (Hemiptera: Aphididae). Biol Control. (2015) 90:16–24. doi: 10.1016/j.biocontrol.2015.05.009
23. Brewer MJ, Gordy JW, Kerns DL, Woolley JB, Rooney WL, Bowling RD. Sugarcane aphid population growth, plant injury, and natural enemies on selected grain sorghum hybrids in Texas and Louisiana. J Econ Entomol. (2017) 110:2109–18. doi: 10.1093/jee/tox204
24. Salas-Araiza MD, López-Gutiérrez DR, Martínez-Jaime OA, Guzman-Mendoza R. Parasitoids of sugarcane aphid, Melanaphis sacchari, at Irapuato, Guanajuato, Mexico. Southwest Entomol. (2017) 42:1091–4. doi: 10.3958/059.042.0403
25. Rodríguez-del-Bosque LA, Rodríguez-Vélez B, Sarmiento-Cordero MA, Arredondo-Bernal HC. Natural enemies of Melanaphis sacchari on grain sorghum in Northeastern Mexico. Southwest Entomol. (2018) 43: 277–79. doi: 10.3958/059.043.0103
26. Maxson EL, Brewer MJ, Rooney WL, Woolley JB. Species composition and abundance of the natural enemies of sugarcane aphid, Melanaphis sacchari (Zehntner) (Hemiptera: Aphididae), on sorghum in Texas. Proc Entomol Soc Wash Soc. (2019) 121:657–80. doi: 10.4289/0013-8797.121.4.657
27. Hewlett JA, Szczepaniec A, Eubanks MD. The effects of sugarcane aphid density in sorghum on predation by lady beetles and lacewings. Biol Control. (2019) 129:171–7. doi: 10.1016/j.biocontrol.2018.10.015
28. Brewer MJ, Noma T, Elliott NC. Hymenopteran parasitoids and dipteran predators of the invasive aphid Diuraphis noxia after enemy introductions: temporal variation and implication for future aphid invasions. Biol Control. (2005) 33:315–23. doi: 10.1016/j.biocontrol.2005.03.013
29. Giles K, Hein G, Peairs F. Areawide pest management of cereal aphids in dryland wheat systems of the Great Plains, USA. In: Koul O, Cuperus GW, Elliott NC, editors. Areawide Pest Management: Theory and Implementation. Wallingford, Oxfordshire, UK: CAB International. (2008) p. 441–466 doi: 10.1079/9781845933722.0441
30. Brewer MJ, Noma T, Elliott NC, Kravchenko AN, Hild AL. A landscape view of cereal aphid parasitoid dynamics reveals sensitivity to farm- and region-scale vegetation structure. Eur J Entomol. (2008)105: 503–511. doi: 10.14411/eje.2008.066
31. Elliott NC, Kieckhefer RW, Lee JH, French BW. Influence of within-field and landscape factors on aphid predator populations in wheat. Landscape Ecol. (1999) 14:239–52. doi: 10.1023/A:1008002528345
32. Elliott NC, Brewer MJ, Giles KL. Landscape context affects aphid parasitism by Lysiphlebus testaceipes (Hymenoptera: Aphidiinae) in wheat fields. Environ Entomol. (2018) 47:803–11. doi: 10.1093/ee/nvy035
33. Thies C, Roschewitz I, Tscharntke T. The landscape context of cereal aphid-parasitoid interactions. Proc Royal Soc B. (2005) 272:203–10. doi: 10.1098/rspb.2004.2902
34. Bianchi FJJA, Van Der Werf W. Model evaluation of the function of prey in non-crop habitats for biological control by ladybeetles in agricultural landscapes. Ecol Modell. (2004) 171:177–93. doi.org/10.1016/j.ecolmodel.2003.08.003 doi: 10.1016/j.ecolmodel.2003.08.003
35. Tonnang HEZ, Hervé BDB, Biber-Freudenberger L, Salifu D, Subramanian S, Ngowi VB, et al. Advances in crop insect modelling methods—towards a whole system approach. Ecolog Modelling. (2017) 354:88–103. doi: 10.1016/j.ecolmodel.2017.03.015
36. Hinson P. Effect of Temperature on the Development of Sugarcane Aphid, Melanaphis sacchari, on Sorghum. Canyon, TX: West Texas A&M University. (2017).
37. Esquivel IL, Faris AM, Brewer MJ. Sugarcane aphid, Melanaphis sacchari (Hemiptera: Aphididae), abundance on sorghum and johnsongrass in a laboratory and field setting. Crop Prot. (2021) 148:105715. doi: 10.1016/j.cropro.2021.105715
38. Lahiri S, Ni X, Buntin GD, Toews MD. Parasitism of Melanaphis sacchari (Hemiptera: Aphididae) by Lysiphlebus testaceipes (Hymenoptera: Braconidae) in the greenhouse and field. J Entomol Sci. (2020) 55:14–24. doi: 10.18474/0749-8004-55.1.14
39. Rodriguez-Velez JM, Uribe-Mu CA, Sarmiento-Cordero MA, Rodriguez-Velez B, Cruz-Rodriguez JA, Contreras-Ramos A, et al. Relationships among Melanaphis sacchari, its predator insect communities, and weather conditions in sorghum crops at Colima, Mexico. Southwest Entomol. (2021) 46:47–58. doi: 10.3958/059.046.0104
40. Szczepaniec A. Assessment of a density-based action threshold for suppression of sugarcane aphids, (Hemiptera: Aphididae), in the southern High Plains. J Econ Entomol. (2018) 111: 2201–2207.
41. (USDA NASS) U.S. Department of Agriculture, National Agricultural Statistics Service. CropScape—cropland data layer. National Agricultural Statistics Service. (2021). Available online at: https://nassgeodata.gmu.edu/CropScape (accessed Jan. 11, 2021).
42. Freund JE, Walpole RE. Mathematical statistics, 3rd ed. Englewood Cliffs, NJ: Prentice-Hall. (1980).
43. Pike KS, Starý P, Miller T, Allison D, Boydston L, Graf G, Gillespie R. Small-grain aphid parasitoids (Hymenoptera: Aphelinidae and Aphidiidae) of Washington: distribution, relative abundance, seasonal occurrence, and key to known North American species. Environ Entomol. (1997) 26:1299–311. doi: 10.1093/ee/26.6.1299
44. McAuslane HM, Johnson FA, Knauft DA, Colvin DL. Seasonal abundance and within-plant distribution of parasitoids of Bemisia tabaci (Homoptera: Aleyrodidae) in peanuts. Environ Entomol. (1993) 22:1043–50. doi: 10.1093/ee/22.5.1043
45. ESRI. Esri products. (2021). Available online at: https://www.esri.com/en-us/arcgis/products/index on (accessed April 12, 2021).
46. (USGS) U.S. Geological Survey. Multi-resolution Land Characteristics Consortium. (2021). Available online at: https://www.mrlc.gov/ (accessed September 28, 2021).
47. Weather Underground. 2021. Weather Underground. Available online at: https://www.wunderground.com (accessed on Mar. 30, 2021).
48. Neter J, Kutner M, Wasserman W, Nachsheim C. Applied linear statistical models, 4th ed. New York: McGraw-Hill. (2004).
49. Littell RC, Freund RJ, Spector PC. System for linear models, 3rd ed. Cary, NC: SAS Institute Inc. (1991).
50. Holm S. A simple sequentially rejective multiple test procedure. Scandinavian J Stat. (1979) 6:65–70.
51. Payan-Arzapalo MA, Catzim CES, Luque RG, Liera JEG, Juarez MGY, Ahuja MLR. Parasitism and hyperparasitism in Melanaphis sacchari Zehntner in sorghum in Mexico. Southwest Entomol. (2018) 43:433–7. doi: 10.3958/059.043.0214
52. Mercer NH. Management of sugarcane aphid (Melanaphis sacchari) using biological and cultural tactics in Kentucky. Theses and Dissertations– Entomology. (2020) Available online at: https://uknowledge.uky.edu/entomology_etds/51 (accessed on September 28, 2021).
53. Schultz AN, Lucardi RD, Marsico TD. Strengthening the ties that bind: an evaluation of cross-disciplinary communication between invasion ecologists and biological control researchers in entomology. Annals Entomol Soc Amer. (2021) 114:163–74. doi: 10.1093/aesa/saaa052
54. Readshaw JL. The numerical response of predators to prey density. J Appl Ecol. (1973) 10:342–51.
55. Ahern RG, Brewer MJ. Effect of different wheat production systems on the presence of two parasitoids (Hymenoptera: Aphelinidae; Braconidae) of the Russian wheat aphid in the North American Great Plains. Agric Ecosys Environ. (2002) 92:201–10. doi: 10.1016/S0167-8809(01)00296-1
56. Chaplin-Kramer R, O'Rourke ME, Blitzer EJ, Kremen C A. meta-analysis of crop pest and natural enemy response to landscape complexity. Ecol Lett. (2011) 14:922–32. doi: 10.1111/j.1461-0248.2011.01642.x
57. Giles KL, Jones DB, Royer TA, Elliott NC, Kindler SD. Development of a sampling plan in winter wheat that estimates cereal aphid parasitism levels and predicts population suppression. J Econ Entomol. (2003) 96:975–82. doi: 10.1093/jee/96.3.975
58. Sharma H. Host-plant resistance to insects in sorghum and its role in integrated pest management. Crop Prot. (1993) 12:11–34. doi: 10.1016/0261-2194(93)90015-B
59. Martin EA, Dainese M, Clough Y, Baldi A, et al. The interplay of landscape composition and configuration: new pathways to manage functional biodiversity and agroecosystem services across Europe. Ecol Lett. (2019) 22: 1083–94. doi: 10.1111/ele.13265
61. Faris AM, Elliott NC, Brewer MJ. Suppression of the sugarcane aphid, Melanaphis sacchari Zehntner (Hemiptera: Aphididae), by resident natural enemies on susceptible and resistant sorghum hybrids. Environ Entomol. (2022) 50. doi: 10.1093/ee/nvab147
62. Triltsch H, Freier B, Rossberg D. Consequences of new findings on climate change with relatively warmer nights on the wheat-cereal aphid–predator interaction in computer simulations. Arch Phytopathol Plant Prot. (1998) 31:363–7. doi: 10.1080/03235409809383246
63. van Baaren J, Le Lann C, van Alphen JM. Consequences of climate change for aphid-based multi-trophic systems. In: Kindlmann P, Dixon AFG, Michaud JP, editor. Aphid biodiversity under environmental change. Springer. (2010) p. 55–68. doi: 10.1007/978-90-481-8601-3_4
Keywords: Melanaphis sacchari, invasive species management, ecological modeling, ecosystem services, biological control, agroecosystem resilience
Citation: Brewer MJ, Elliott NC, Esquivel IL, Jacobson AL, Faris AM, Szczepaniec A, Elkins BH, Gordy JW, Pekarcik AJ, Wang H-H, Koralewski TE, Giles KL, Jessie CN and Grant WE (2022) Natural Enemies, Mediated by Landscape and Weather Conditions, Shape Response of the Sorghum Agroecosystem of North America to the Invasive Aphid Melanaphis sorghi. Front. Insect Sci. 2:830997. doi: 10.3389/finsc.2022.830997
Received: 07 December 2021; Accepted: 17 February 2022;
Published: 12 April 2022.
Edited by:
Hugo A. Benítez, Universidad Católica del Maule, ChileReviewed by:
Cristian Villagra, Universidad Metropolitana de Ciencias de la Educación, ChileRodrigo M. Barahona-Segovia, University of Los Lagos, Chile
Copyright © 2022 Brewer, Elliott, Esquivel, Jacobson, Faris, Szczepaniec, Elkins, Gordy, Pekarcik, Wang, Koralewski, Giles, Jessie and Grant. This is an open-access article distributed under the terms of the Creative Commons Attribution License (CC BY). The use, distribution or reproduction in other forums is permitted, provided the original author(s) and the copyright owner(s) are credited and that the original publication in this journal is cited, in accordance with accepted academic practice. No use, distribution or reproduction is permitted which does not comply with these terms.
*Correspondence: Michael J. Brewer, bWpicmV3ZXJAYWcudGFtdS5lZHU=
†These authors share senior authorship