- 1Institute of Apicultural Research, Chinese Academy of Agricultural Sciences (CAAS), Beijing, China
- 2Key Laboratory of Pollinating Insect Biology, Ministry of Agriculture and Rural Affairs, Beijing, China
- 3Graduate School of Chinese Academy of Agricultural Sciences, Beijing, China
- 4Guangdong Key Laboratory of Animal Conservation and Resource Utilization, Guangdong Public Laboratory of Wild Animal Conservation and Utilization, Institute of Zoology, Guangdong Academy of Sciences, Guangzhou, China
Honey bees play a vital role in providing pollination services for agricultural crops and wild flowering plants. However, the spillover risk of their pathogens to other pollinators or wild insects is becoming a cause for concern. There is some evidence that stingless bees can carry honey bee viruses, but little is known about the presence of honey bee viruses in stingless bees in China. Here, we investigate the occurrence of major honey bee pathogens including bacteria, fungi, and viruses in stingless bees (Apidae: sp.). Our results show that the stingless bees (Apidae: sp.) were mainly infected with DWV-A, but no DWV-B and DWV-C. Phylogenetic analysis on fragments of lp, RdRp, and VP3 of DWV-A indicated that genetic variation in VP3 might an important indicator for host-specific viruses, but it requires further study. Our results indicated that DWV-A is not only the major strain of virus currently circulating in managed bee colonies in China and globally, but in stingless bee species as a whole.
Introduction
Honey bees play an important role in flowering plants in the agri-ecosystem, but viral diseases pose a severe threat to honey bee population growth (1). It was reported that more than 20 bee viruses were identified that affected the health and survival of bees (2). Among them, eight common viruses were Israel acute paralysis virus (IAPV), Deformed wing virus (DWV), Black queen cell virus (BQCV), Sacbrood virus (SBV), Chronic bee paralysis virus (CBPV), Acute bee paralysis virus (ABPV), Kashmir bee virus (KBV), and Kakugo virus (KV) (3–10).
DWV is considered the most prevalent bee virus worldwide due to its close relation to colony decline induced by interactions between Varroa destructor and DWV (11). DWV is a positive single-stranded RNA virus and its genome is about 10 kb in length (12). Currently, three master variants of DWV have been identified: type A (4), type B (13), and type C (14). Initially, only one strain, DWV, was identified in honey bee colonies around the world. Then, DWV-B, previously designated as V. destructor virus-1 (VDV-1), came to be considered as one viral cloud with DWV-A (14). DWV-C is a recently described strain and its impact is still unknown (14). DWV-A is currently the most prevalent genotype and is closely related to colony decline (15–17). The titer of DWV-B is inhibited significantly when DWV-A is a presence (18, 19). In addition, the co-existence of the three genotypes of DWV is a frequent occurrence, leading to their recombination (14, 20–22). Although KV was identified in aggressive workers in 2004 (23), subsequent studies did not find that KV was a primary virus of Apis mellifera colonies (24, 25).
DWV is causing growing concern because it can infect and replicate in multiple invertebrates including bumblebees, solitary bees, wasps, and ants (26, 27). This suggests that DWV is spreading to other insect species, especially wild bee species, and poses a potential threat to their population growth (28–32). It was reported that virulent pathogens and parasites can spill over from cultivated bees to wild bees such as the stingless bee (29, 31, 33), but whether the bee viruses were a presence in stingless bee in China was still unknown.
In this study, we attempted to test whether common honey bee pathogens were present in the stingless bee, Apidae sp. We found that two strains of DWV (DWV-A and DWV-A/KV) were found in stingless bees collected in the Yunnan province of China. Phylogenetic analysis showed that DWV-A or DWV-A/KV from the stingless bees was close to that found in A. mellifera. This suggested that DWV in these stingless bees was most likely obtained from A. mellifera, but it needs to be further confirmed.
Materials and Methods
Samples Collection
The samples of stingless bees Apidae sp. were collected in the field in Yunnan province in December 2019. Live bee samples alive were put into a small cage and transported to the laboratory at −80°C until use. We identified the stingless bee species using PCR amplification with the universal primers (LCO-1490 and HCO-2198) previously used (34).
DNA and RNA Extraction
The total DNA was extracted from pooled Apidae sp. workers (~5-10 bees) with the TissuePrep homogenizer (Gening Scientific, Beijing, China). Briefly, bee samples were added 1 ml of lysis buffer and 10 μl of proteinase K and then heated at 65°C in a water bath for 30 min. Subsequently, the supernatant was taken after low-temperature high-speed centrifugation and added to an equal volume of phenol: chloroform: isoamyl alcohol (25:24:1), and then centrifuged at 4°C. Finally, the precipitation was washed with 75% ethanol, and then 30-50 μl ddH2O was added to dissolve the DNA.
The total RNA was isolated from pooled stingless bee samples using TRIzol Kit (Invitrogen, USA) according to the manufacturer's protocol with the TissuePrep homogenizer (Gening Scientific, Beijing, China). The obtained RNA was dissolved in 20 μl of sterile water and stored at −80°C prior to use. The quantity and purity of the RNA were measured using a Nanodrop spectrophotometer (Thermo Scientific, Beijing, China). The cDNA samples were used as templates for PCR amplification with specific primers corresponding to target genes.
PCR Detection
The primer sequences and the target size of amplification for honey bee common pathogens were provided in Supplementary Tables 1, 2. PCR amplification of non-virus pathogens of honey bee was performed in a 20 μl volume containing template DNA, 2×GoTaq Mix (Promega, USA), and corresponding primers as shown in Supplementary Table 1. PCR amplification was performed for 2 min at 94°C, 30 s at 94°C, 30 s at 55°C, 30 s at 72°C for 32 cycles, and 5 min at 72°C.
For the detection of honey bee RNA viruses, the extracted RNA was used to synthesize cDNA using GoScirpt reverse transcriptase (Promega, Madison, WI, USA). The initial cycle was performed for reverse transcription at 80°C for 30 min, and then followed by 1 min at 95°C; 33 cycles of 30 s at 94°C, 30 s at 55°C, and 72°C for 1 min; a final extension of 10 min at 72°C; and cooling to 4°C. The PCR amplification products were separated in a 2% agarose gel stained with GV II (Biomec, China) and photographed with a fluorescent biological-image analysis system (Furi, China). The product size was determined with a 100-bp ladder.
Then, we focused on the amplification on four fragments, part of DWV, RdRp, Lp, and VP3 using the corresponding primers (Supplementary Table 3) with an initial denaturation for 3 min at 98°C, 10 s at 98°C, 20 s at 55°C, 30 s at 72°C for 30 cycles, and 10 min at 72°C.
Phylogenetic Analysis
The target products were purified and sent to be sequenced by Sangon corporation (Sangon, Shanghai, China). The sequencing results were compared with those sequences from GenBank using NCBI (National Centre for Biotechnology Information) BLAST (Basic Local Alignment Search Tool) to determine the similarity. Phylogenetic trees were constructed based on the nucleotide sequences using the maximum likelihood method (Maximum Likelihood) to infer its evolution process as run on MEGA 7 software (35, 36). The 40 nucleotide sequences were selected and their specific information is shown in Supplementary Table 4. The sequences of RdRp, Lp, and VP3 of DWV-A from the Apidae sp. were obtained under the accession numbers of MT899243 (DWV-A), MT899244 (Lp), MT899245 (RdRp), MT899247 (VP3), as well as MT899246 (DWV-A/KV).
Results
Virus Occurrence
We conducted a PCR analysis with stingless bee Apidae sp. collected in wild and screened for the presence of 10 viruses and 6 bacterial or fungal pathogens of honey bees (Supplementary Table S1). Only two strains of DWV, DWV-A and DWV-A/KV, were found and were used to further study the phylogenetic relation among the known strains from bees, Varroa destructor, and wasp species around the world.
Phylogenetic Analysis
Based on the alignment of amplification fragments of DWV, RdRp, Lp, and VP3 with nucleotide sequences of representative DWV strains from honey bees (A. mellifera, A. ceranae), bumblebee, wasp, and a parasite (Varroa destructor), phylogenetic analysis was conducted by the neighbor-joining method with 1000 bootstrap replications using MEGA 7.0 (Figure 1). The resulting phylogenetic trees indicated that DWV-A, lp, and RdRp fragments displayed relatively similar phylogenies in different hosts, and DWV strain from stingless bee was close to those of A. mellifera, but the VP3 fragment was different from those of lp and RdRp. In the phylogenetic tree of DWV-A/KV, DWV-A/KV of stingless bee was clustered with those of A. mellifera and revealed two major groups from China and other countries (Figure 2). These suggested that DWV in stingless bee is close to that of A. mellifera no matter whether DWV-A or DWV-A/KV.
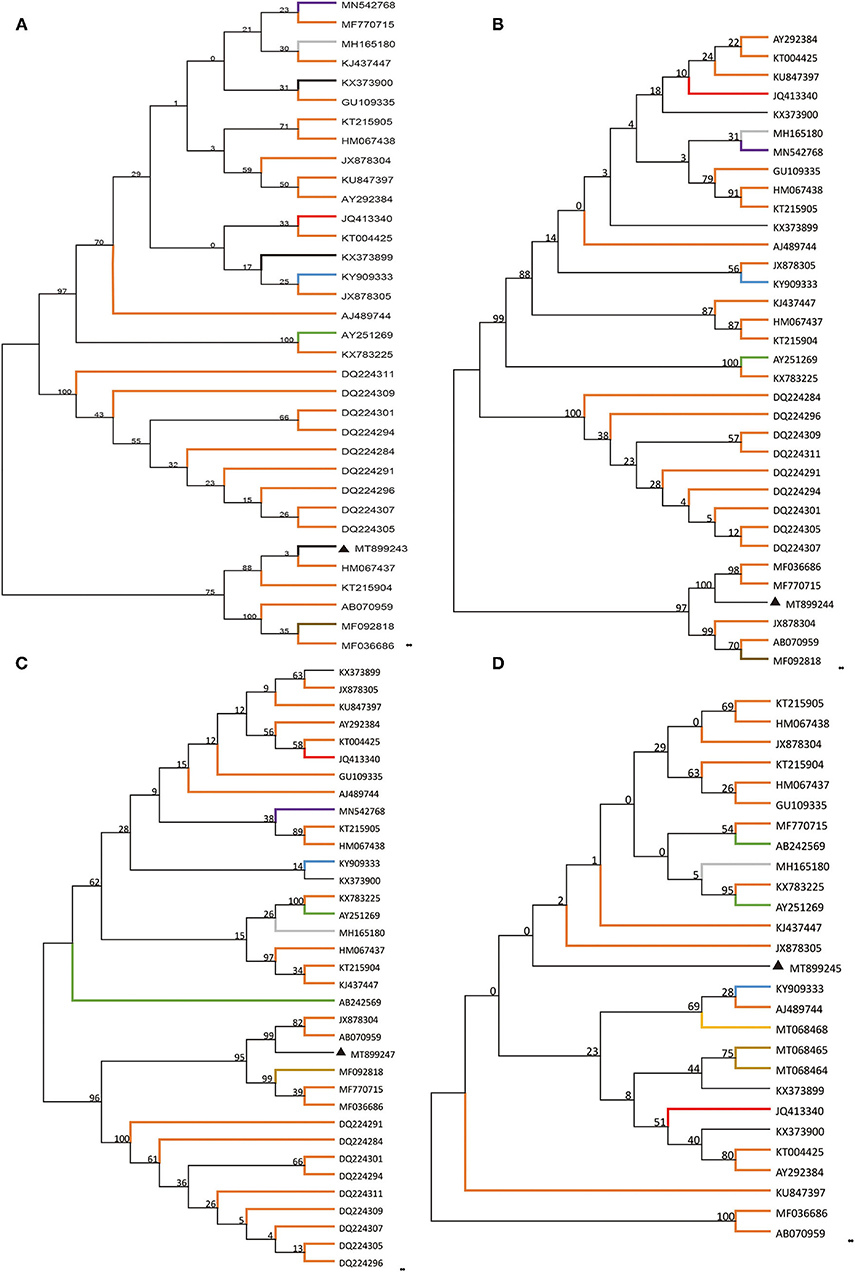
Figure 1. Phylogenetic reconstruction of four fragments of DWV showing host and geographic structure. The host included different bee, wasp, and ant species as well as Varroa destructor. Phylogenetic analysis on four genomic fragments, part of DWV-A (A), Lp (B), VP3 (C), and RdRp (D). The maximum likelihood method (ML) and bootstrap resampling (1,000 repetitions) were used to construct a phylogenetic tree. The number on each branch of the phylogenetic tree represents the bootstrap value (1,000 repetitions), and the branches with different colors indicate different species infected by different DWV strains. The different colors indicate DWV strains from a different host. Apis mellifera, Apis cerana, Vespa crabro, Varroa destructor, vespids wasp, ant, polistes chinesis, Vespula vulgaris, stingless bees, bombus, and unknown host are indicated in orange, gray, blue, green, brown, purple, yellow, khaki, red, gray-blue, and black, respectively.
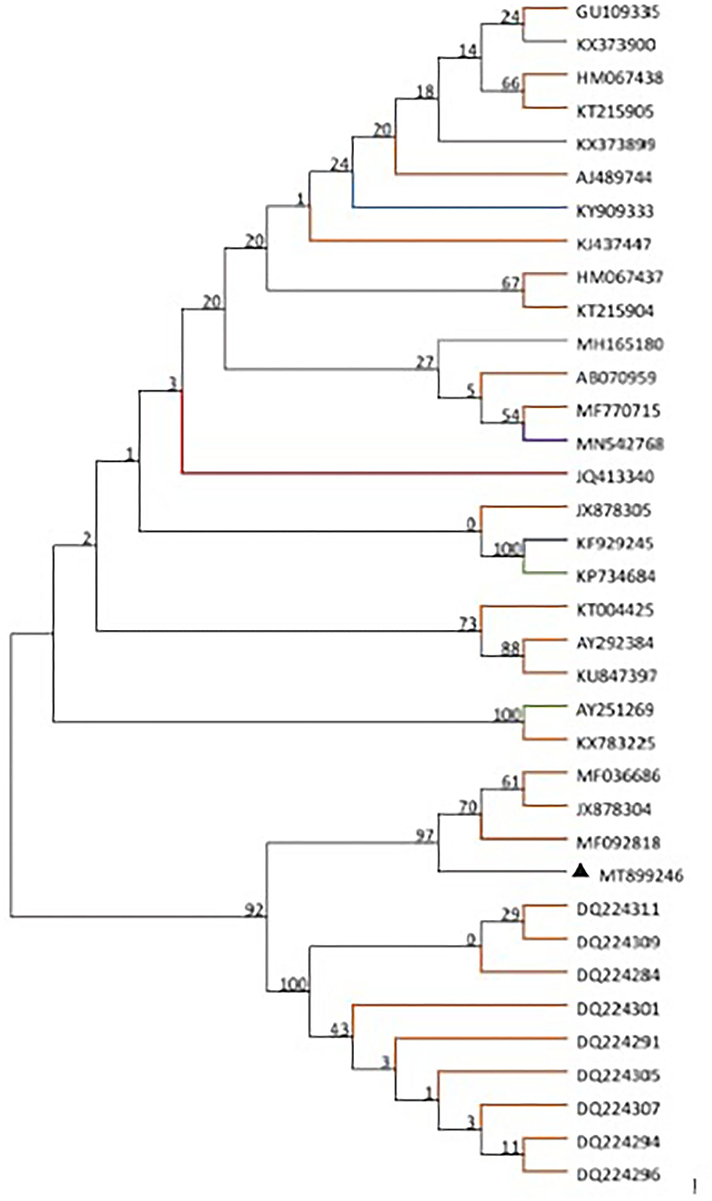
Figure 2. Phylogenetic tree of DWV-A/KV from different insect species including bee, wasp, and ant as well as Varroa destructor. The maximum likelihood method (ML) and bootstrap resampling (1,000 repetitions) were used to construct a phylogenetic tree. The number on each branch of the phylogenetic tree represents the bootstrap value (1,000 repetitions), and the branches with different colors indicate different species infected by different DWV strains. The different colors indicate DWV strains from different hosts. Apis mellifera, Apis cerana, Vespa crabro, Varroa destructor, vespids wasp, ant, polistes chinesis, Vespula vulgaris, stingless bees, bombus, and unknown host are indicated in orange, gray, blue, green, brown, purple, yellow, khaki, red, gray-blue, and black, respectively.
Discussion
The detection results showed that DWV-A or DWV-A/KV occurred in the stingless bee (Apidae sp.), but not DWV-B and C. Previous studies showed that DWV-A and -C were detected in the Brazilian stingless bee Melipona subnitida (37), as well as in Scaptotrigona mexicana (38), and Tetragonisca fiebrigi (39). Our results meanwhile showed that only one genotype, DWV-A or DWV-A/KV, was found in stingless bee Apiade sp, suggesting that the DWV-A genotype was possibly limited in stingless bee Apiade sp from the Yunnan province of China. It can be seen from the evolutionary tree that the bee virus DWV has indeed spilled over to other species.
The current study showed that DWV strains in stingless bee were clustered in the same branch with those of other Asian strains in geographical regions based on the DWV-A, lp, and VP3 nucleotides sequences but not RdRp fragment. Although phylogenetic analysis on DWV-A sequences indicated that DWV-A in Apidae sp. was close to that found in the UK, the other three fragments were close to those of Asia. Similar results were obtained in the phylogeny of DWV-A/KV of Apidae sp. (Figure 2). This suggested that DWV has evolved its specific characterization in genomic sequence during the process of co-evolution with hosts in local regions.
On the other side, although DWV-A was present in stingless bees in China, this DWV-A was not close to that of stingless bees from other regions such as Argentina (39). Our results showed that DWV-A in Apidae sp. was close to that in A. mellifera but not to other stingless bee species from other regions. In addition, hornets such as Vespa crabro were found to be infected by DWV (40) but our phylogenetic analysis showed that DWV in Apidae sp. was not close to that of V. crabro. This indicated that DWV-A of Apidae sp. was possibly obtained from A. mellifera in China, but further experiments need to be performed.
Data Availability Statement
The original contributions presented in the study are included in the article/Supplementary Material, further inquiries can be directed to the corresponding author/s.
Author Contributions
CH conceived this manuscript. LZ, YD, HZ, and MZ performed the experiments. LZ and CH analyzed the data and wrote the manuscript. All authors contributed to the article and approved the submitted version.
Funding
This research was funded by the Agricultural Science and Technology Innovation Program (CAAS-ASTIP-2021-IAR), the Fundamental Research Funds for CAAS (Y2020PT17), and the Young Talent Program of CAAS, GDAS Special Project of Science and Technology Development (2018GDASCX-0107).
Conflict of Interest
The authors declare that the research was conducted in the absence of any commercial or financial relationships that could be construed as a potential conflict of interest.
Publisher's Note
All claims expressed in this article are solely those of the authors and do not necessarily represent those of their affiliated organizations, or those of the publisher, the editors and the reviewers. Any product that may be evaluated in this article, or claim that may be made by its manufacturer, is not guaranteed or endorsed by the publisher.
Acknowledgments
We would like to express our thanks to the beekeepers for their help with sample collection.
Supplementary Material
The Supplementary Material for this article can be found online at: https://www.frontiersin.org/articles/10.3389/finsc.2021.748074/full#supplementary-material
References
1. Klein AM, Vaissière BE, Cane JH, Steffan-Dewenter I, Cunningham SA, Kremen C, et al. Importance of pollinators in changing landscapes for world crops. Proc Biol Sci. (2007) 1608:303–13. doi: 10.1098/rspb.2006.3721
2. Brutscher LM, McMenamin AJ, Flenniken ML. The buzz about honey bee viruses. PLoS Pathog. (2016) 8:e1005757. doi: 10.1371/journal.ppat.1005757
3. Maori E, Lavi S, Mozes-Koch R, Gantman Y, Peretz Y, Edelbaum O, et al. Isolation and characterization of Israeli acute paralysis virus, a dicistrovirus affecting honeybees in Israel: evidence for diversity due to intra- and inter-species recombination. J Gen Virol. (2007) 12:3428–38. doi: 10.1099/vir.0.83284-0
4. Lanzi G, de Miranda JR, Boniotti MB, Cameron CE, Lavazza A, Capucci L, et al. Molecular and biological characterization of deformed wing virus of honeybees (Apis mellifera L.). J Virol. (2006) 10:4998–5009. doi: 10.1128/JVI.80.10.4998-5009.2006
5. Leat N, Ball B, Govan V, Davison S. Analysis of the complete genome sequence of black queen-cell virus, a picorna-like virus of honey bees. J Gen Virol. (2000) 8:2111–9. doi: 10.1099/0022-1317-81-8-2111
6. Ghosh RC, Ball BV, Willcocks MM, Carter MJ. The nucleotide sequence of sacbrood virus of the honey bee: an insect picorna-like virus. J Gen Virol. (1999) 6:1541–9. doi: 10.1099/0022-1317-80-6-1541
7. Olivier V, Blanchard P, Chaouch S, Lallemand P, Schurr F, Celle O, et al. Molecular characterisation and phylogenetic analysis of chronic bee paralysis virus, a honey bee virus. Virus Res. (2008) 132:59–68. doi: 10.1016/j.virusres.2007.10.014
8. Govan VA, Leat N, Allsopp M, Davison S. Analysis of the complete genome sequence of acute bee paralysis virus shows that it belongs to the novel group of insect-infecting RNA viruses. Virology. (2000) 2:457–63. doi: 10.1006/viro.2000.0616
9. de Miranda JR, Drebot M, Tyler S, Shen M, Cameron CE, Stoltz DB, et al. Complete nucleotide sequence of Kashmir bee virus and comparison with acute bee paralysis virus. J Gen Virol. (2004) 8:2263–70. doi: 10.1099/vir.0.79990-0
10. Fujiyuki T, Ohka S, Takeuchi H, Ono M, Nomoto A, Kubo T. Prevalence and phylogeny of Kakugo virus, a novel insect picorna-like virus that infects the honeybee (Apis mellifera L.), under various colony conditions. J Virol. (2006) 23:11528–38. doi: 10.1128/JVI.00754-06
11. De Miranda JR, Genersch E. Deformed wing virus. J Invertebr pathol. (2010) 103:S48–61. doi: 10.1016/j.jip.2009.06.012
12. Berényi O, Bakonyi T, Derakhshifar I, Köglberger H, Topolska G, Ritter W, et al. Phylogenetic analysis of deformed wing virus genotypes from diverse geographic origins indicates recent global distribution of the virus. Appl Environ Microbiol. (2007) 11:3605–11. doi: 10.1128/AEM.00696-07
13. Ongus JR, Peters D, Bonmatin JM, Bengsch E, Vlak JM, van Oers MM. Complete sequence of a picorna-like virus of the genus Iflavirus replicating in the mite Varroa destructor. J Gen Virol. (2004) 12:3747–55. doi: 10.1099/vir.0.80470-0
14. Mordecai GJ, Brettell LE, Martin SJ, Dixon D, Jones IM, Schroeder DC. Superinfection exclusion and the long-term survival of honey bees in Varroa-infested colonies. ISME J. (2016) 5:1182–91. doi: 10.1038/ismej.2015.186
15. Martin SJ, Highfield AC, Brettell L, Villalobos EM, Budge GE, Powell M, et al. Global honey bee viral landscape altered by a parasitic mite. Science. (2012) 6086:1304–6. doi: 10.1126/science.1220941
16. Schroeder DC, Martin SJ. Deformed wing virus: the main suspect in unexplained honeybee deaths worldwide. Virulence. (2012) 7:589–91. doi: 10.4161/viru.22219
17. Francis RM, Nielsen SL, Kryger P. Varroa-virus interaction in collapsing honey bee colonies. PLoS ONE. (2013) 3:e57540. doi: 10.1371/journal.pone.0057540
18. Mordecai GJ, Wilfert L, Martin SJ, Jones IM, Schroeder DC. Diversity in a honey bee pathogen: first report of a third master variant of the deformed wing virus quasispecies. ISME J. (2016) 5:1264–73. doi: 10.1038/ismej.2015.178
19. McMahon DP, Natsopoulou ME, Doublet V, Fürst M, Weging S, Brown MJ, et al. Elevated virulence of an emerging viral genotype as a driver of honeybee loss. Proc Biol Sci. (2016) 1833:20160811. doi: 10.1098/rspb.2016.0811
20. Moore J, Jironkin A, Chandler D, Burroughs N, Evans DJ, Ryabov EV. Recombinants between deformed wing virus and Varroa destructor virus-1 may prevail in Varroa destructor-infested honeybee colonies. J Gen Virol. (2011) 1:156–61. doi: 10.1099/vir.0.025965-0
21. Ryabov EV, Wood GR, Fannon JM, Moore JD, Bull JC, Chandler D, et al. A virulent strain of deformed wing virus (DWV) of honeybees (Apis mellifera) prevails after Varroa destructor-mediated, or in vitro, transmission. PLoS Pathog. (2014) 6:e1004230. doi: 10.1371/journal.ppat.1004230
22. Dalmon A, Desbiez C, Coulon M, Thomasson M, Le Conte Y, Alaux C, et al. Evidence for positive selection and recombination hotspots in Deformed wing virus (DWV). Sci Rep. (2017) 7:41045. doi: 10.1038/srep41045
23. Fujiyuki T, Takeuchi H, Ono M, Ohka S, Sasaki T, Nomoto A, et al. Novel insect picorna-like virus identified in the brains of aggressive worker honeybees. J Virol. (2004) 78:1093–100. doi: 10.1128/JVI.78.3.1093-1100.2004
24. Forgách P, Bakonyi T, Tapaszti Z, Nowotny N, Rusvai M. Prevalence of pathogenic bee viruses in Hungarian apiaries: situation before joining the European Union. J Invertebr Pathol. (2008) 98:235–8. doi: 10.1016/j.jip.2007.11.002
25. Runckel C, Flenniken ML, Engel JC, Ruby JG, Ganem D, Andino R, et al. Temporal analysis of the honey bee microbiome reveals four novel viruses and seasonal prevalence of known viruses, Nosema, and Crithidia. PLoS ONE. (2011) 6:e20656. doi: 10.1371/journal.pone.0020656
26. Evison SE, Roberts KE, Laurenson L, Pietravalle S, Hui J, Biesmeijer JC, et al. Pervasiveness of parasites in pollinators. PLoS ONE. (2012) 1:e30641. doi: 10.1371/journal.pone.0030641
27. Manley R, Boots M, Wilfert L. Emerging viral disease risk to pollinating insects: ecological, evolutionary and anthropogenic factors. J Appl Ecol. (2015) 2:331–40. doi: 10.1111/1365-2664.12385
28. Wilfert L, Long G, Leggett HC, Schmid-Hempel P, Butlin R, Martin SJ, et al. Deformed wing virus is a recent global epidemic in honeybees driven by Varroa mites. Science. (2016) 6273:594–7. doi: 10.1126/science.aac9976
29. Graystock P, Goulson D, Hughes WO. Parasites in bloom: flowers aid dispersal and transmission of pollinator parasites within and between bee species. Proc Biol Sci. (2015) 1813:20151371. doi: 10.1098/rspb.2015.1371
30. Gisder S, Genersch E. Special issue: honey bee viruses. Viruses. (2015) 10:5603–8. doi: 10.3390/v7102885
31. McMahon DP, Fürst MA, Caspar J, Theodorou P, Brown MJF, Paxton RJ. A sting in the spit: widespread cross-infection of multiple RNA viruses across wild and managed bees. J Anim Ecol. (2015) 3:615–24. doi: 10.1111/1365-2656.12345
32. Tehel A, Brown MJ, Paxton RJ. Impact of managed honey bee viruses on wild bees. Curr Opin Virol. (2016) 19:16–22. doi: 10.1016/j.coviro.2016.06.006
33. Graystock P, Goulson D, Hughes WO. The relationship between managed bees and the prevalence of parasites in bumblebees. PeerJ. (2014) 12:e522. doi: 10.7717/peerj.522
34. Yang S, Gayral P, Zhao H, Wu Y, Jiang X, Wu Y, et al. Occurrence and molecular phylogeny of honey bee viruses in Vespids. Viruses. (2020) 1:6. doi: 10.3390/v12010006
35. Tamura K, Nei M. Estimation of the number of nucleotide substitutions in the control region of mitochondrial DNA in humans and chimpanzees. Mol Biol Evol. (1993) 3:512–26.
36. Kumar S, Stecher G, Tamura K. MEGA7: molecular evolutionary genetics analysis Version 7.0 for bigger datasets. Mol Biol Evol. (2016) 7:1870–4. doi: 10.1093/molbev/msw054
37. de Souza FS, Kevill JL, Correia-Oliveira ME, de Carvalho CAL, Martin SJ. Occurrence of deformed wing virus variants in the stingless bee Melipona subnitida and honey bee Apis mellifera populations in Brazil. J Gen Virol. (2019) 2:289–94. doi: 10.1099/jgv.0.001206
38. Guzman-Novoa E, Hamiduzzaman MM, Anguiano-Baez R, Correa-Benítez A, Castañeda-Cervantes E. First detection of honey bee viruses in stingless bees in North America. J Apic Res. (2015) 54:93–5. doi: 10.1080/00218839.2015.1100154
39. Alvarez LJ, Reynaldi FJ, Ramello PJ, Garcia MLG, Sguazza GH, Abrahamovich AH, et al. Detection of honey bee viruses in Argentinian stingless bees (Hymenoptera: Apidae). Insect Soc. (2017) 65:191–7. doi: 10.1007/s00040-017-0587-2
Keywords: honey bee viruses, deformed wing virus, stingless bee, Apidae sp, pathogen spillover
Citation: Zhang L, Deng Y, Zhao H, Zhang M and Hou C (2021) Occurrence and Phylogenetic Analysis of DWV in Stingless Bee (Apidae sp.) in China: A Case Report. Front. Insect Sci. 1:748074. doi: 10.3389/finsc.2021.748074
Received: 27 July 2021; Accepted: 18 October 2021;
Published: 12 November 2021.
Edited by:
Wei-Fone Huang, Fujian Agriculture and Forestry University, ChinaReviewed by:
Rameshwor Pudasaini, Tribhuvan University, NepalTerd Disayathanoowat, Chiang Mai University, Thailand
Copyright © 2021 Zhang, Deng, Zhao, Zhang and Hou. This is an open-access article distributed under the terms of the Creative Commons Attribution License (CC BY). The use, distribution or reproduction in other forums is permitted, provided the original author(s) and the copyright owner(s) are credited and that the original publication in this journal is cited, in accordance with accepted academic practice. No use, distribution or reproduction is permitted which does not comply with these terms.
*Correspondence: Chunsheng Hou, aG91Y2h1bnNoZW5nJiN4MDAwNDA7Y2Fhcy5jbg==