- 1Joint Program in Transfusion Medicine, Department of Pathology, Brigham and Women’s Hospital, Harvard Medical School, Boston, MA, United States
- 2Harvard Glycomics Center, Harvard Medical School, Boston, MA, United States
- 3Aflac Cancer and Blood Disorders Center, Children's Healthcare of Atlanta, Atlanta, GA, United States
- 4Department of Pediatrics, Emory University School of Medicine, Atlanta, GA, United States
- 5Center for Transfusion and Cellular Therapies, Department of Pathology and Laboratory Medicine, Emory University School of Medicine, Atlanta, GA, United States
Red blood cell (RBC) alloantibodies can result in the rapid removal of incompatible RBCs following transfusion. However, antibody-mediated clearance of RBCs is not the inevitable outcome of an incompatible transfusion. Antibody engagement can also result in the modulation of the target antigen, often rendering RBCs resistant to antibody-mediated removal. Despite this, the factors that regulate antibody-induced RBC removal or antigen modulation remain incompletely understood. Given the ability of CD47 to regulate RBC survival in general, we examined the possible role of CD47 in governing antibody-mediated RBC clearance and antigen modulation. This was achieved by crossing the well-established HEL-OVA-Duffy (HOD) mouse model with CD47 knockout (KO) mice to generate offspring that express the HOD antigen and either WT (HOD CD47 WT), heterozygote (HOD CD47 HET) or KO (HOD CD47 KO) levels of CD47. Using the commonly employed anti-HEL immunization model, our results demonstrate that while antibody engagement of HOD CD47 WT RBCs resulted in rapid antigen modulation in the absence of detectable RBC clearance, antibody binding to HOD CD47 HET RBCs did result in detectable RBC removal despite similar rates and overall levels of antigen modulation. In contrast, despite accelerated clearance of HOD CD47 KO RBCs in the absence of anti-HEL antibodies, the rate of RBC removal and antigen modulation was enhanced in the presence of anti-HEL antibodies. Taken together, these results suggest a role for CD47 in regulating the overall consequence of an incompatible RBC transfusion.
Introduction
Blood transfusion represents one of the most common interventions in medicine. While red blood cell (RBC) transfusion in particular can be lifesaving, it is not without risk (1–3). Variations in RBC surface antigens between individuals can trigger the production of alloantibodies (4). While blood group antigens probably developed as a response to evolutionary pressures from infectious agents and likely play specific roles in the host’s immune system (5–11), the formation of alloantibodies against RBC alloantigens can create challenges in identifying suitable RBC units for subsequent transfusions and increase the risk of hemolytic transfusion reactions. These antibodies can also evanesce to undetectable levels when using standard blood bank testing methods. In patients who require repeated blood transfusions, such as those with certain medical conditions like sickle cell disease (SCD), this poses a significant risk (12, 13). As alloantibodies can go undetected at the time of transfusion, when patients are re-exposed to RBC alloantigens to which they have previously mounted an immune response, it can trigger a recrudescent alloimmune response that results in accelerated clearance of the transfused RBCs that constitutes a delayed hemolytic transfusion reaction (14, 15).
Perhaps in part due to distinct underlying features of immunity (16–18), in patients with SCD, this process can be even more severe (15, 19–22). Anamnestic immune responses that result from alloantigen reexposure not only target the transfused RBCs but can also result in the accelerated clearance of the patient’s own RBCs (15, 19–21). This phenomenon, known as hyperhemolysis, can lead to severe anemia and life-threatening complications, including severe organ damage and death (20, 23–29). Unlike immune-mediated platelet clearance (30, 31), antibody-induced hemolysis can cause significant complications, likely in part due to the impact of free heme on innate immunity, including complement activation (20, 24–29). These transfusion complications can limit the availability of potential long-term treatment options, such as gene therapy or bone marrow transplantation due to challenges in providing safe peri-transplant transfusion support (20, 32–34). Importantly, the impact of RBC alloimmunization on morbidity and mortality is not limited to patients with SCD, but also extends to other transfusion-dependent patient populations (35).
Multiple approaches have been adopted to minimize exposure to alloantigens in patients who require chronic RBC transfusion therapy, including antigen-matching (36), with the goal of decreasing the risk of alloimmunization and its associated complications. Early studies demonstrated that this approach could lower the incidence of immune responses against common alloantigen targets (36). However, the complexity of some alloantigen systems has presented challenges in achieving comprehensive matching for various antigenic structures (37). Recent findings support this, revealing that even with efforts to match for prevalent alloantigens and minimize exposure to alloantigens in general, patients can still develop alloantibodies (38). Consequently, there have been significant efforts aimed at discovering complementary approaches that could proactively inhibit the formation of RBC alloantibodies following alloantigen exposure (39–48). These investigations, encompassing both preclinical and clinical approaches, have shed light on the critical immune mechanisms that trigger the development of alloantibodies following RBC transfusion that may aid in preventing alloimmune responses against other intravascularly delivered antigens (49–55). Further studies have not only pinpointed significant regulators that govern this process but have also enhanced our understanding of patient-specific characteristics that affect the likelihood of antibody production after RBC transfusion (14, 38, 56–66). By identifying pathways that govern RBC alloimmunization, potential therapeutic targets have emerged that might help prevent or at least reduce the risk of alloantibody formation following RBC transfusion.
While the results of preclinical and clinical studies provide important insight into potential strategies that may be employed to actively prevent alloantibody formation, in some patient populations, 30-40% of patients have already developed alloantibodies (1, 67–69). Consequently, while there are efforts to minimize further alloantibody development, strategies focused solely on prevention may be less effective at reducing or eliminating the consequences of RBC alloimmunization in these patients. This is especially apparent when considering patients can form alloantibodies against a highly prevalent antigen or develop a complex mixture of alloantibodies that makes it difficult to find compatible RBC units (70–72). When this occurs, units that become available can remain incompatible for a given alloantigen. This situation becomes especially challenging when patients with complicated alloantibodies present with life-threatening anemia requiring urgent RBC transfusions (70–73). As current transfusion strategies are nearly uniformly aimed at providing compatible RBCs, when situations arise in which compatible units are unavailable, there are few strategies that have been developed to prevent the negative consequences that can result from an incompatible RBC transfusion.
To better define the factors that regulate incompatible transfusion, several preclinical models, utilizing distinct target antigens, have been leveraged (74–89). One of these is the HOD (HEL,OVA,Duffy) system, which incorporates the HEL and OVA model antigens by coupling them to the Duffy blood group antigen as a chimeric fusion protein (88, 89). Studies using this model have demonstrated that antibody-mediated elimination of HOD RBCs appears to occur through interaction of Fcy receptors (FcγR) (82, 90). Consistent with this, previous studies have demonstrated that anti-HOD antibodies targeting different portions of the HOD antigen fail to fix detectable levels of complement (86). However, in addition to this FcγR-dependent pathway, FcγR-independent mechanisms, have also been described (90). Interestingly, the mechanism of clearance can vary depending on the combination of antibodies that the transfused HOD RBCs encounter (77, 78, 87).
Despite many studies examining the mechanisms whereby antibodies induce RBC clearance, several studies indicate that RBCs may not always be removed following an incompatible transfusion (77, 78, 82, 90–94). While there are several possible reasons for this to occur, the ability of antibodies to induce alterations to the target antigen has emerged as a unique outcome of incompatible RBC transfusion that can occur in the absence of any detectable RBC clearance (77, 78, 82, 90–94). This phenomenon, often referred to as antibody-induced antigen modulation, can reduce the levels of detectable antibody present on the RBC surface, often rendering RBCs less susceptible to further antibody-driven clearance (77, 78, 82, 90–94). Interestingly, this antibody-dependent decrease of antigen can also occur in either an FcγR-dependent or -independent manner (77, 78, 87). However, the factors that govern antibody-mediated loss of antigen remain relatively unknown.
Among regulatory proteins on the cell surface, RBCs express CD47, an anti-phagocytic marker expressed on a wide range of cell types (95–97). CD47 is known to prevent cellular removal via its interaction with SIRPα98-100. This interaction has been extensively studied, particularly in the context of cancer cells, which can upregulate CD47 to block immune-mediated phagocytic removal (98). Given the critical role of CD47 in the regulation of cellular removal, levels of CD47 on transfused RBCs may play a critical role in the outcome of incompatible transfusion. Indeed, an interplay between antibody-induced RBC removal and CD47-mediated signals may in part dictate whether antibody-induced antigen modulation or RBC removal occurs following antibody engagement of transfused RBCs.
Given the consequences of incompatible RBC transfusion on patient outcomes, we sought to investigate the role of CD47 as a regulator of antibody-mediated antigen modulation and RBC clearance. To accomplish this, we bred HOD RBC mice with CD47 knockout (KO) mice to create three types of donor RBC populations: one with normal CD47 levels (HOD CD47 WT), one with half the normal levels (CD47 heterozygous; HOD CD47 HET), and one completely lacking CD47 (HOD CD47 KO). Despite possessing similar HOD levels, these three donor RBCs showed varying levels of susceptibility to antibody-mediated RBC clearance. While HOD CD47 WT and HOD CD47 HET RBCs did not exhibit significant clearance following transfusion into non-immunized recipients, HOD CD47 HET RBCs were uniquely sensitive to anti-HEL-mediated RBC removal. In contrast, HOD CD47 KO RBCs exhibited accelerated clearance following transfusion into non-immunized recipients, which was further enhanced and accompanied by accelerated antigen loss following transfusion into anti-HEL immunized recipients. These findings reveal a role for CD47 in controlling both RBC clearance and antigen loss in response to incompatible transfusion, offering important insights into the mechanisms affecting RBC fate following antibody engagement.
Methods
Mice
C57BL/6 (B6) mice were purchased from Charles River Laboratories. B6.129P2-Fcer1gtm1Rav N12 (FcRy constitutive knockout, model 583) mice were obtained from Taconic. HOD transgenic mice were generated as described previously (99). These mice express the triple fusion protein HOD (hen egg lysozyme, a portion of ovalbumin, and Duffy b antigen) on RBCs (via a beta-globin promoter). CD47 deficient mice (B6.129S7-Cd47tm1Fpl/J) were purchased from The Jackson Laboratory. HOD mice were then crossbred with CD47KO mice to create: HOD CD47WT, CD47HET, and CD47KO mice. The Division of Animal Resources and Husbandry at Brigham and Women’s Hospital bred and housed these mice. Experiments were initiated in male and female (approximately 1:1 ratio) mice ages 8-12 weeks old. Each experiment included 3-5 mice per group, with representative data of 3 experiments shown. The experimental protocols and animal procedures described herein were approved by the Institutional Animal Care and Use Committee (IACUC).
Antibodies
APC-conjugated anti-CD47 antibody (clone miap301), anti-CD71 (clone RI7217) and APC-conjugated anti-Ter119 (Ly-76) were obtained from Biolegend. The anti-HEL monoclonal antibodies (2F4 and 4B7), which are mouse IgG1, were obtained from BioXcell. APC-conjugated goat anti-mouse IgG was obtained from Jackson ImmunoResearch Laboratories. Biotinylated anti-mouse C3 (clone RmC11H9) and biotinylated anti-mouse iC3b (clone 10C7) were obtained from Cedarlane. APC-conjugated streptavidin was obtained from Biolegend.
RBC transfusions and antigen level evaluation
HOD and B6 RBCs were labeled with DiI and DiO, respectively, and transfused as previously described (77, 78, 82). Briefly, HOD antigen positive and negative RBCs are labeled with unique fluorescent dyes and mixed at a 1:1 ratio before transfusion into designated recipients as outlined previously (77). Following transfusion, blood samples are assessed at key timepoints and the ratio of antigen positive to negative RBCs is calculated. Multiple time-points are selected to assess the progression of antigen specific RBC clearance, and the 4h time-point is shown to highlight the earliest time-point at which significant differences in clearance are observed. In all clearance experiments, percent survival is calculated by determining the ratio of HOD antigen positive cells to antigen negative control cells transfused together. For normalization of RBC clearance, percent clearance for each group is normalized to its relevant PBS control group. RBC stains were performed as previously described (83, 100). In brief, RBCs were washed three times with phosphate buffered saline (PBS) prior to staining. Then, a saturating concentration of the primary antibody was added and incubated for 15 minutes at room temperature, followed by three washes with PBS. Then, a saturating concentration of the secondary antibody was added if needed, followed by washing three times with PBS. Reticulocytes were distinguished from mature RBCs in whole blood from each donor by CD71 expression, as described previously (51). A Cytek NorthernLights flow cytometer was used to acquire the data followed by data analysis using FlowJo software.
Statistical analyses
GraphPad Prism software was used for statistical analysis. Two groups were analyzed using a student’s t tests, while ≥3 groups were analyzed using a one-way analysis of variance (ANOVA) with Tukey multiple comparison test. P ≤.05 was considered statistically significant.
Results
To test the hypothesis that a balance between RBC removal and antigen loss post-antibody engagement may in part be influenced by CD47, we bred CD47 KO mice, which lack CD47 entirely, with HOD mice across several generations to create donor mice with varying CD47 levels (wild type, heterozygote, or knockout) (Figures 1A, B). We first assessed HOD antigen levels in each donor group (HOD CD47 WT, HOD CD47 HET, and HOD CD47 KO), using a set of monoclonal antibodies that target distinct epitopes on the HEL antigen. Using this approach, no significant differences in HEL levels among the donor groups was observed. This was consistent regardless of whether one or a combination of these monoclonal antibodies was used, suggesting that HEL antigen levels remain comparable across CD47 WT, CD47 het, and CD47 KO HOD RBC donors (Figures 1B-E). We also examined the composition of the peripheral blood collected from each RBC donor. Interestingly, blood collected from CD47 HOD KO donors did contain a slightly higher percentage of reticulocytes than blood collected from CD47 HOD WT donors, and reticulocytes in general exhibited higher levels of HOD antigen expression across all donors. However, no differences in the level of HOD antigen were observed between donors when comparing levels on reticulocytes or mature RBCs (Supplementary Figure 1). This difference in reticulocyte count was insufficient to cause differences in overall HEL levels on RBCs collected from each of the three donors (Supplementary Figure 1; Figures 1B-E), possibly due to the subtle differences in reticulocytes numbers between each group where CD47 HOD KO may have 1% more reticulocytes among the total RBC population than some CD47 HOD WT donors.
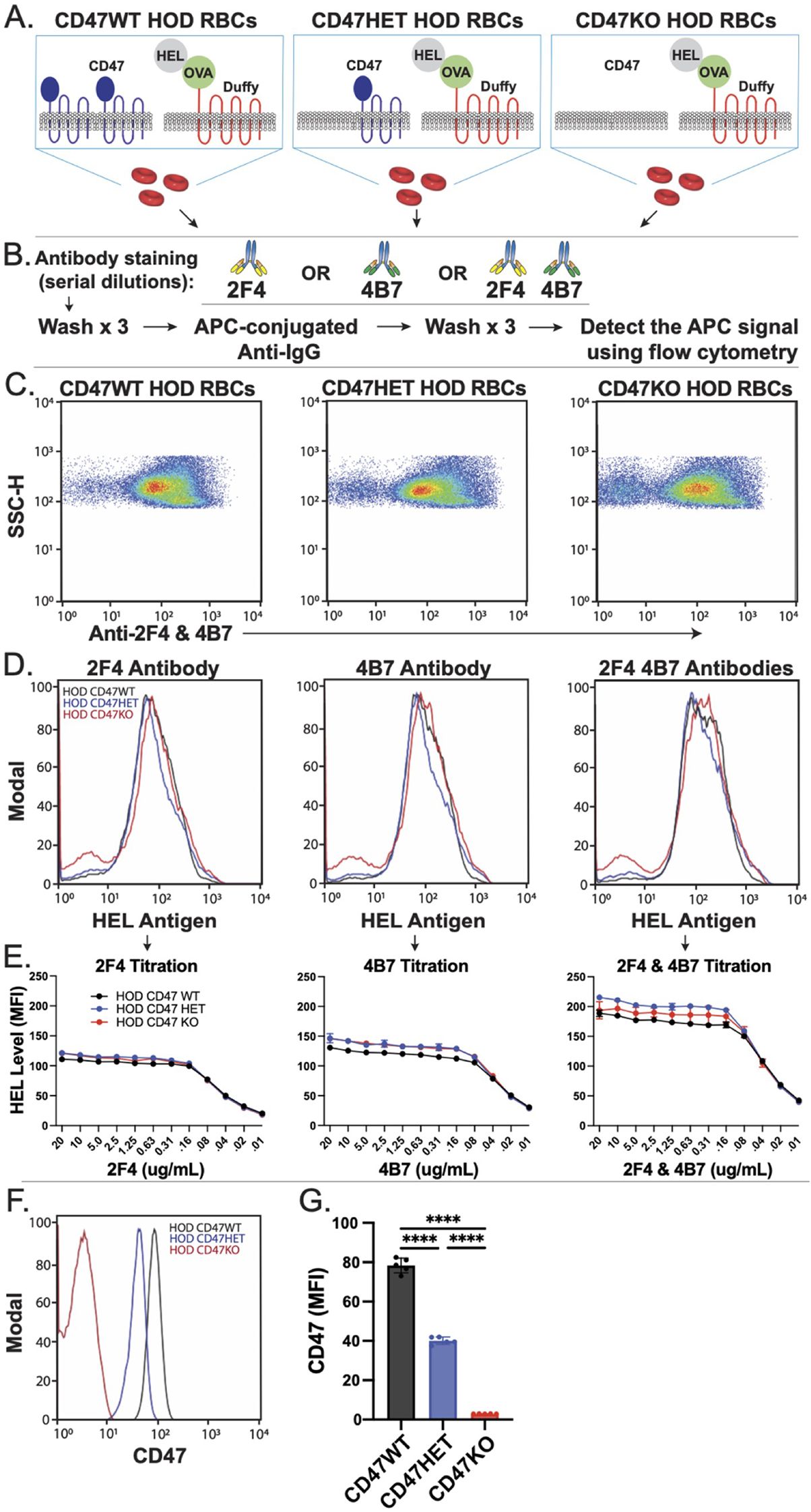
Figure 1. CD47 knockout and heterozygous HOD RBCs possess reduced levels of CD47 with comparable HOD antigen levels. (A) Depictions of distinct HOD CD47 RBC populations used in this model. (B) Protocol for detecting HEL antigen on the surface of HOD CD47 RBCs. (C) Examination of HEL antigen expression on HOD CD47 WT, HOD CD47 heterozygous (HET) or HOD CD47 knockout (KO) shown by dot plots examining anti-HEL antibody (2F4 or 4B7) engagement by side scatter (SSC). (D) Evaluation of anti-HEL antibody binding over a range of concentrations toward HOD CD47 WT, HOD CD47 HET and HOD CD47 KO RBCs by histogram. (E) Quantification of histogram mean fluorescent intensity (MFI) values using a range of concentrations of anti-HEL antibodies individually or in combination toward HOD CD47 RBCs WT, HOD CD47 HET and HOD CD47 KO RBCs. (F) Histogram analysis of CD47 expression on HOD CD47 WT, HOD CD47 HET and HOD CD47 KO RBCs. (G) Quantification of histogram MFI values of CD47 antigen expression on HOD CD47 WT, HOD CD47 HET and HOD CD47 KO RBCs. ****p < 0.0001.
We next sought to ensure that the apparent lack of differences in the HEL antigen on the surface of HOD CD47 WT, CD47 HET and CD47 KO was not due to incomplete saturation of the anti-HEL antibodies used to define HEL levels on the RBC surface. To accomplish this, we titrated each monoclonal antibody over a range of concentrations against each of these three HOD RBC types. Using this approach, comparable levels of HEL on the CD47 WT, CD47 het, and CD47 KO HOD RBCs were observed across a wide range of antibody concentrations (Figure 1E). Additional examination of the HOD antigen using a combination of anti-HEL antibodies yielded similar results. To verify if the offspring of each RBC donor had the expected CD47 levels, we analyzed CD47 on RBCs from each group. As seen in prior studies (95), CD47 expression varied based on gene dosage (Figures 1F, G). RBCs from mice heterozygous for CD47 (HOD CD47 HET) expressed roughly half the CD47 expression levels observed from homozygous donors (HOD CD47 WT) donors. Conversely, RBCs from CD47 KO mice had no detectable CD47 (Figures 1F, G). These findings indicate that crossing the HOD RBC model system with CD47 KO mice can produce distinct donor RBCs with WT, HET or KO levels of CD47 while retaining similar levels of the HOD antigen.
With HOD donor RBCs that express distinct levels of the CD47, we next examined the consequence of transfusing these unique RBC donor populations into recipients immunized with anti-HEL antibodies. To accomplish this, HOD CD47 WT, CD47 HET or CD47 KO RBCs were labeled with the fluorescent dye, DiI, as outlined previously, to facilitate detection post-transfusion (Figures 2A, B). HOD negative RBCs were labeled with a fluorescently distinct dye, DiO, as a control (Figures 2A, B). Consistent with previous findings, transfusion of HOD CD47 WT RBCs into recipients with anti-HEL antibodies failed to impact RBC clearance, regardless of the specific anti-HEL antibody used or even when recipients received a combination of the anti-HEL antibodies (Figures 2C-E). When HOD CD47 HET RBCs were transfused into non-immunized recipients, despite expressing only half the level of CD47, no appreciable difference in RBC survival was detected (Figures 2C-E). Similar results were observed when these same HOD CD47 HET RBCs were transfused into recipients passively immunized with only one anti-HEL monoclonal antibody (Figures 2C-E). In contrast, when HOD CD47 HET RBCs were transfused into recipients immunized with a combination of anti-HEL antibodies, accelerated clearance could be detected (Figures 2C-E). These results suggest that CD47 may regulate RBC sensitivity to antibody-mediated removal.
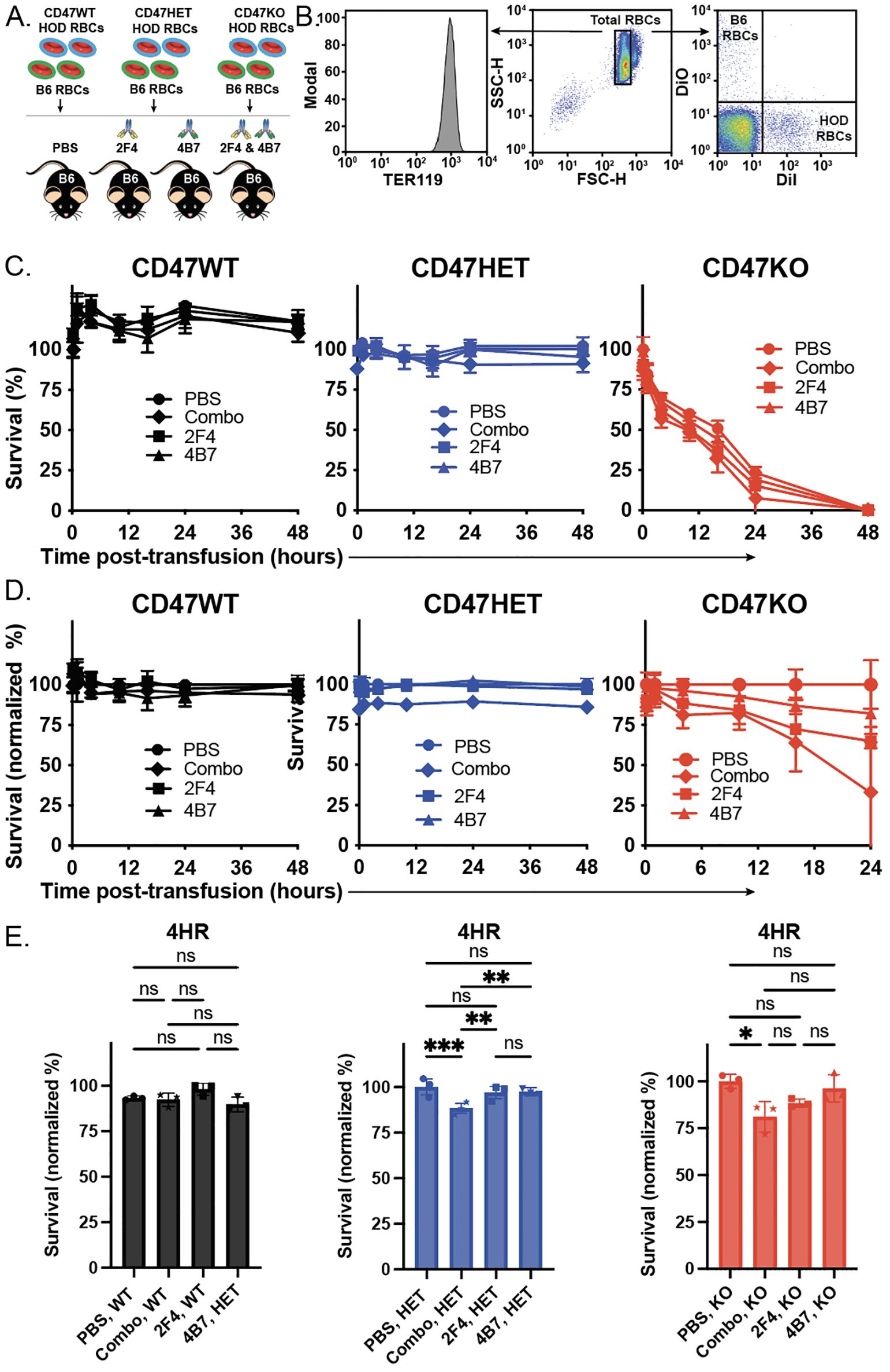
Figure 2. Anti-HEL antibodies enhance clearance of HOD CD47 heterozygous and knockout RBCs, while failing to impact HOD CD47 WT RBC removal. (A) Depiction of the experimental design using HOD CD47 WT, HOD CD47 heterozygous (HET) or HOD CD47 knockout (KO) RBCs transfused into non-immunized (PBS) or recipients immunized with one (2F4 or 4B7) or a combination (2F4 and 4B7) of anti-HEL antibodies. (B) Flow cytometry gating strategy used to detect DiI labeled HOD CD47 WT, HOD CD47 HET and HOD CD47 KO RBCs or DiO labeled B6 RBCs following transfusion into non-immunized or anti-HEL immunized recipients. (C) Survival of transfused HOD CD47 WT, HOD CD47 HET and HOD CD47 KO RBCs over time in comparison to B6 RBCs in the same recipient. (D) Normalized survival of transfused HOD CD47 WT, HOD CD47 HET and HOD CD47 KO RBCs over time in immunized recipients in comparison to the survival observed following transfusion of each population into non-immunized (PBS) recipients evaluated in parallel. Normalized survival for HOD CD47 KO not calculated for time points at which PBS controls reach 0% survival. (E) Bar graph representation of normalized survival of transfused HOD CD47 WT, HOD CD47 HET and HOD CD47 KO RBCs 4 hours following transfusion. ns = not significant. *p<0.05, **p < 0.01, ***p < 0.001.
To determine whether the complete absence of CD47 impacts the outcome of antibody-engagement on HOD RBC clearance, we next employed the exact same strategy of labeling HOD CD47 KO RBCs, followed by transfusion into non-immunized or anti-HEL immunized recipients. In contrast to the HOD CD47 WT or HOD CD47 HET RBCs, which failed to demonstrate noticeable differences in survival post-transfusion in the absence of anti-HEL antibodies, HOD CD47 KO RBCs exhibited reduced survival following transfusion into non-immunized recipients (Figures 2C-E). In contrast to the outcome of HOD CD47 HET RBC transfusion, this removal was accelerated following transfusion into recipients immunized with only a single monoclonal anti-HEL antibody (Figures 2C-E). The rate of clearance was even more pronounced following HOD CD47 KO transfusion into recipients immunized with both anti-HEL antibodies, where this difference was more apparent when normalizing to clearance rates observed in the absence of anti-HEL antibodies (Figures 2C-E). Taken together, these results suggest that CD47 may not only regulate RBC survival in general but may also influence the consequence of antibody engagement on RBC clearance following an incompatible transfusion.
While analysis of anti-HEL antibody engagement of each HOD RBC donor population demonstrated similar binding activity in vitro, to determine whether differences in HOD RBC CD47 WT, HOD CD47 HET and HOD CD47 KO clearance following transfusion into each immunized recipient may reflect differences in antibody binding in vivo, we next examined antibody engagement at various time points following transfusion. Consistent with the levels of antibody engagement observed following incubation with each monoclonal anti-HEL antibody in vitro, no significant differences in antibody binding could be detected 10 minutes following transfusion into each immunized recipient (Figures 3A, D). In contrast to the enhanced antibody levels observed following incubation of a combination of monoclonal antibodies compared to each monoclonal anti-HEL antibody alone in vitro, decreased antibody binding was observed following transfusion into recipients with a combination as opposed to a single monoclonal anti-HEL antibody (Figures 3A, D).
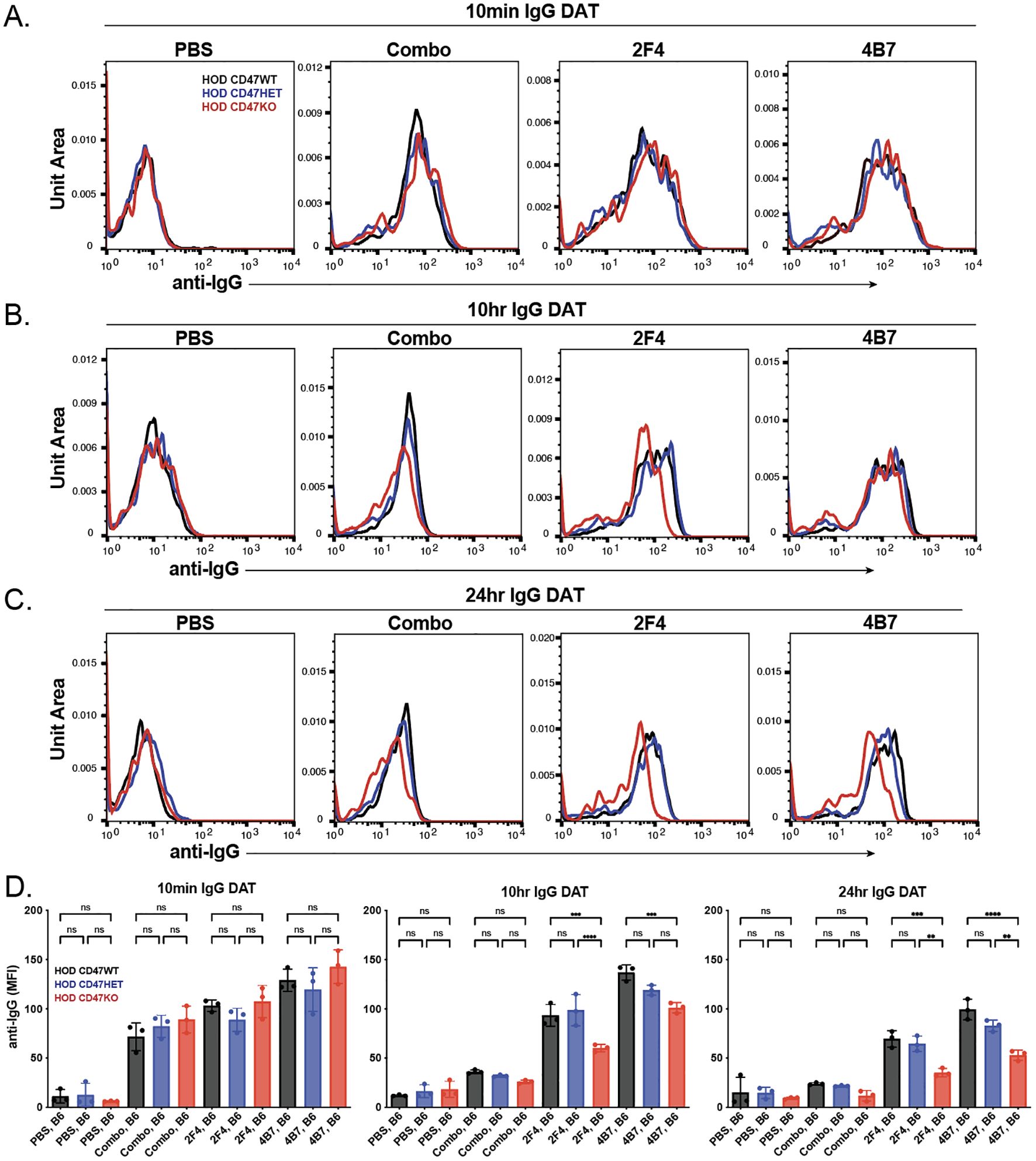
Figure 3. The levels of anti-HEL antibodies decrease over time following transfusion of HOD CD47 WT, heterozygous and knockout RBCs into immunized recipients. Detection of antibody levels on the surface of HOD CD47 WT, HOD CD47 heterozygous (HET) or HOD CD47 knockout (KO) RBCs following transfusion into non-immunized (PBS) or recipients immunized with a single (2F4 or 4B7) or combination (combo - 2F4 and 4B7) of anti-HEL antibodies shown as histograms at 10 minutes (A), 10 hours (B), and 24 hours (C) following transfusion. (D) Quantification of histogram MFI values of antibody bound on HOD CD47 RBCs WT, HOD CD47 HET and HOD CD47 KO RBCs at 10 minutes, 10 hours, and 24 hours post-transfusion. ns = not significant. **p<0.01, ***p < 0.001, ****p < 0.0001.
To assess if there was a sustained variation in detectable antibody levels on each population over time, we analyzed antibody levels on each RBC population at later time points. Similar to the observations 10 minutes after transfusion, each HOD donor RBC population transfused into recipients immunized with a single antibody showed higher detectable antibody levels compared to similar HOD donor RBCs transfused into recipients previously immunized with a combination of anti-HEL antibodies (Figures 3A-D). Interestingly, though the antibody levels on HOD CD47 WT, HOD CD47 HET, and HOD CD47 KO RBCs were initially comparable post-transfusion, a more pronounced decrease in antibody levels was noted on HOD CD47 KO RBCs in recipients previously immunized with a single anti-HEL antibody (Figures 3A-D). This difference in bound antibody levels persisted on each RBC population when examined 24 hours post-transfusion (Figures 3A-D).
Previous data indicated that the reduction in antibody on the surface of antigen-positive RBCs following transfusion can be due to antibody-mediated alterations in the target antigen itself (77–79, 87, 101, 102). To explore this possibility, we analyzed the levels of the HOD antigen in recipients at various time points post-transfusion. We observed no significant change in the HEL antigen levels shortly after transfusion across different types of HOD RBCs (HOD CD47 WT, HOD CD47 HET, and HOD CD47 KO) in recipients who were immunized with a single anti-HEL antibody (Figures 4A, D). However, when each RBC HOD donor population was transfused into recipients who had been immunized with multiple anti-HEL antibodies, significant reductions in the levels of detectable anti-HEL antibodies were noted (Figures 4A, D). Similar to antibody levels, antigen levels continued to decline over time following transfusion into recipients with anti-HEL antibodies, with the rate and magnitude of decreases in antigen detection being more pronounced in the face of a combination of anti-HEL antibodies than individual anti-HEL antibodies alone (Figures 4A-D). These results align with prior results demonstrating that a combination of anti-HEL antibodies is more efficient at inducing reductions in the levels of detectable antigen (77, 78, 87).
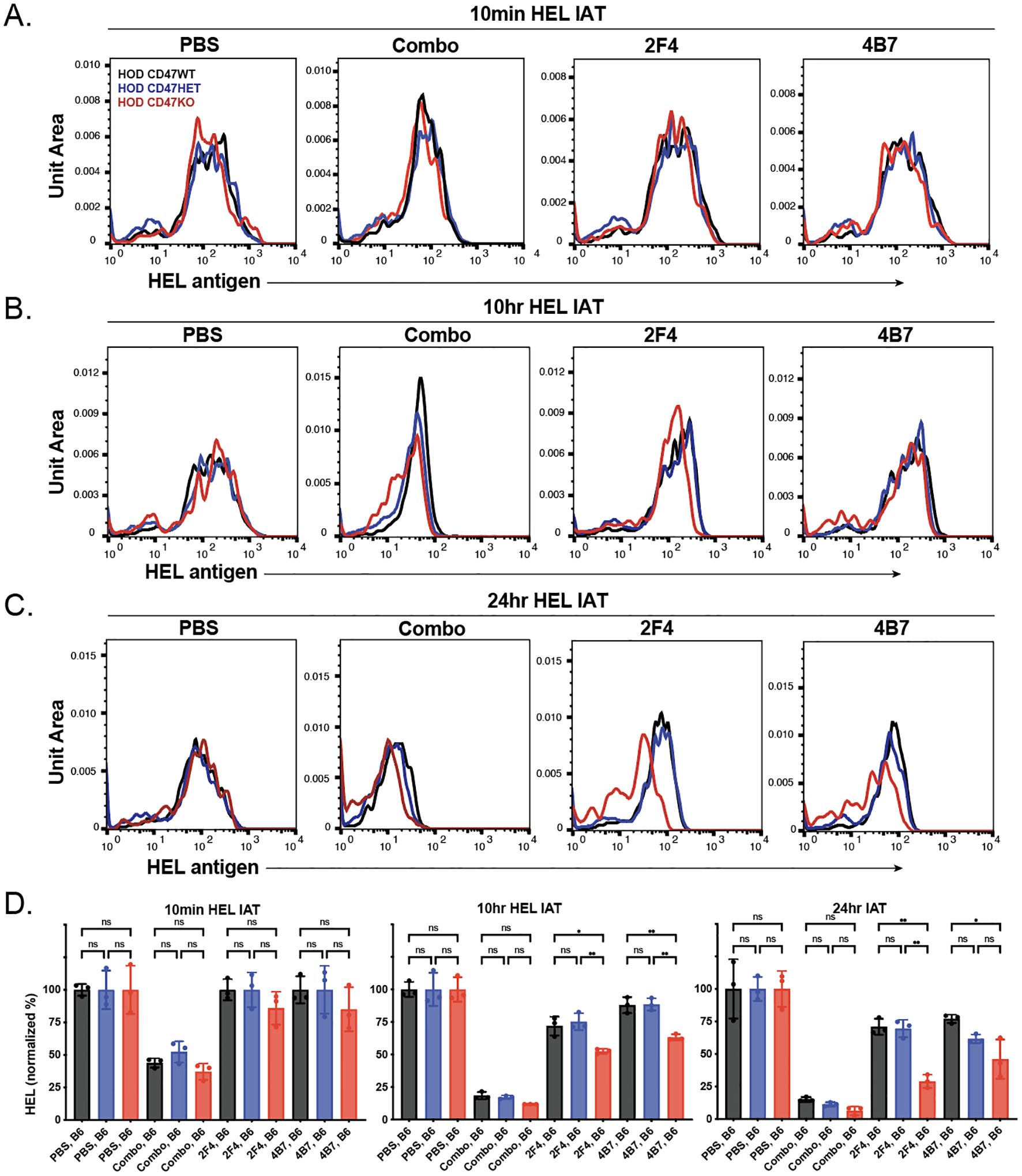
Figure 4. Anti-HEL antibodies reduce HOD antigen levels on HOD CD47 WT, heterozygous and knockout RBCs. Detection of HOD antigen levels on the surface of HOD CD47 WT, HOD CD47 heterozygous (HET) or HOD CD47 knockout (KO) RBCs following transfusion into non-immunized (PBS) or recipients immunized with a single (2F4 or 4B7) or combination (combo - 2F4 and 4B7) of anti-HEL antibodies shown as histograms at 10 minutes (A), 10 hours (B), and 24 hours (C) following transfusion. (D) Quantificaiton of histogram MFI values of HOD antigen on HOD CD47 RBCs WT, HOD CD47 HET and HOD CD47 KO RBCs at 10 minutes, 10 hours, and 24 hours post-transfusion. ns = not significant. *p<0.05, **p<0.01.
Prior studies demonstrated that elimination of RBCs can occur through FcγR-dependent and -independent mechanisms (77, 78, 87). To define whether the augmented HOD RBC clearance observed following transfusion of HOD CD47 HET and HOD CD47 KO RBCs into immunized recipients reflects an FcγR-dependent or -independent process, we examined the clearance of HOD RBCs following transfusion into non-immunized or immunized WT or FcγR KO recipients. As the most pronounced differences in antibody-mediated clearance of HOD CD47 HET and HOD CD47 KO RBCs occurred in recipients immunized with both monoclonal antibodies, a combination of anti-HEL antibodies were used to passively immunize each recipient. In contrast to the enhanced clearance of HOD CD47 HET RBCs observed following transfusion into immunized WT recipients, similar increases in antibody-mediated clearance were not observed following transfusion into immunized FcγR KO when evaluated in parallel (Figures 5A-D). Similarly, antibody-mediated acceleration of HOD RBC CD47 KO removal likewise failed to occur in FcγR KOs when compared to WT recipients (Figures 5A-D).
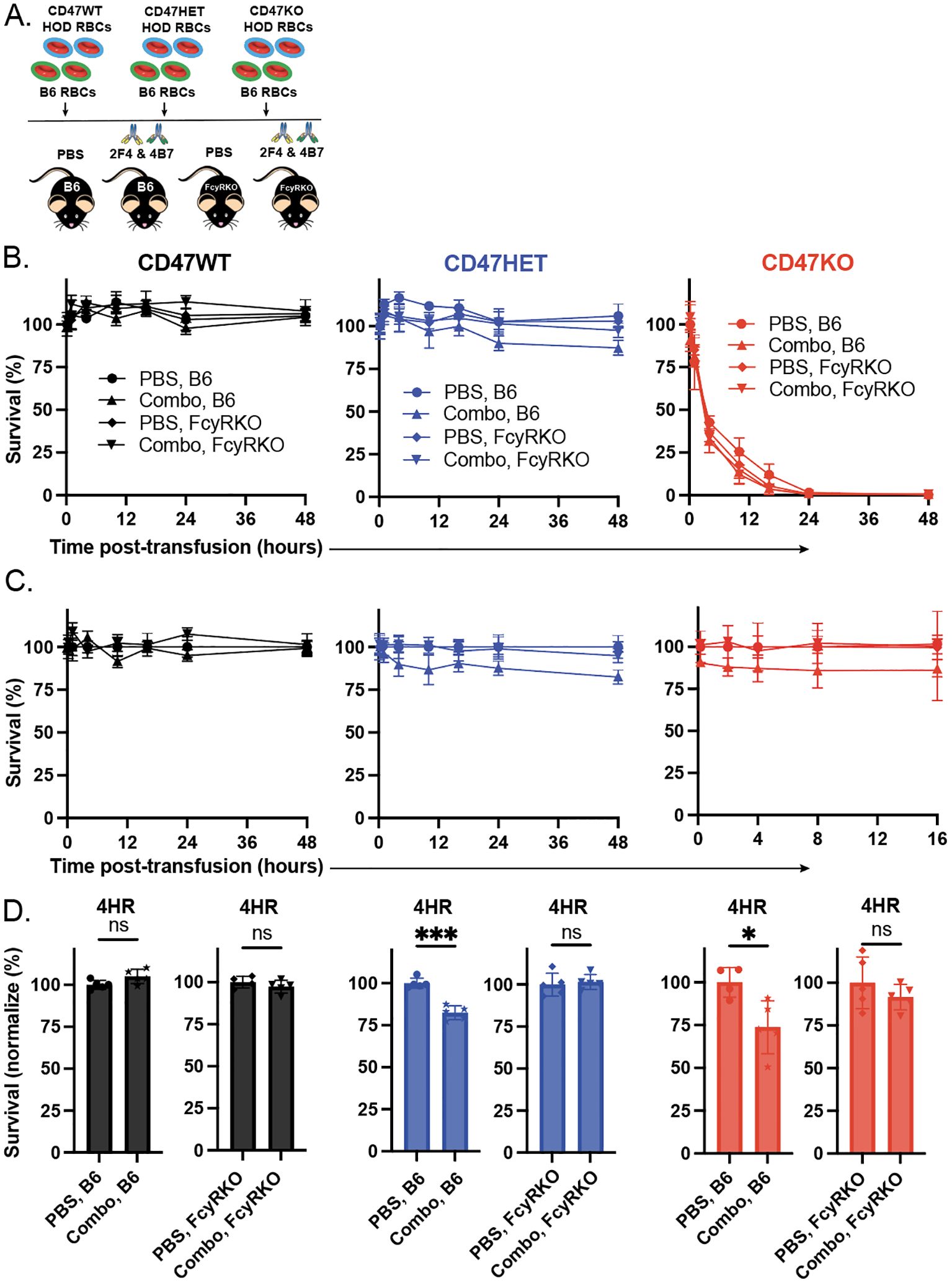
Figure 5. Enhanced antibody-mediated clearance of HOD CD47 heterozygous and knockout RBCs requires Fcγ receptors. (A) Depiction of the experimental design using HOD CD47 WT, HOD CD47 heterozygous (HET) or HOD CD47 knockout (KO) RBCs transfused into WT or Fcγ receptor (FcγR) KO recipients that were either non-immunized (PBS) or immunized with a single (2F4 or 4B7) or combination (combo - 2F4 and 4B7) of anti-HEL antibodies. (B) Survival of transfused HOD CD47 WT, HOD CD47 HET and HOD CD47 KO RBCs over time in comparison to B6 RBCs in the same recipient following transfusion into either WT or FcγR KO recipients that were either non-immunized (PBS) or immunized with a single (2F4 or 4B7) or combination (combo - 2F4 and 4B7) of anti-HEL antibodies. (C) Normalized survival of transfused HOD CD47 WT, HOD CD47 HET and HOD CD47 KO RBCs over time in comparison to B6 RBCs in the same recipient following transfusion into either WT or FcγR KO recipients that were either non-immunized (PBS) or immunized with a single (2F4 or 4B7) or combination (combo - 2F4 and 4B7) of anti-HEL antibodies. Normalized survival for HOD CD47 KO not calculated for time points at which PBS controls reach 0% survival. (D) Bar graph representation of normalized survival of transfused HOD CD47 WT, HOD CD47 HET and HOD CD47 KO RBCs in comparison to B6 RBCs in the same recipient following transfusion into either WT or FcγR KO recipients that were either non-immunized (PBS) or immunized with a single (2F4 or 4B7) or combination (combo - 2F4 and 4B7) of anti-HEL antibodies 4 hours following transfusion. ns = not significant. *p<0.05, ***p < 0.001.
To determine whether any differences in antibody binding could be observed following transfusion of HOD CD47 WT, HOD CD47 HET, and HOD CD47 KO RBCs into anti-HEL immunized WT or FcγR KOs recipients, we next examined antibody levels on the RBC surface. Similar to results obtained following transfusion of each HOD RBC population into anti-HEL immunized WT recipients, significant reductions in the levels of detectable antibody were observed following transfusion into anti-HEL immunized FcγR KOs recipients when evaluated in parallel (Figures 6A-D). Importantly, no difference in antibody levels were observed regardless of whether HOD CD47 WT, HOD CD47 HET, or HOD CD47 KO RBCs were transfused into immunized recipients(Figures 6A-D), suggesting that alterations in clearance did not reflect alterations in antibody levels over the same time period.
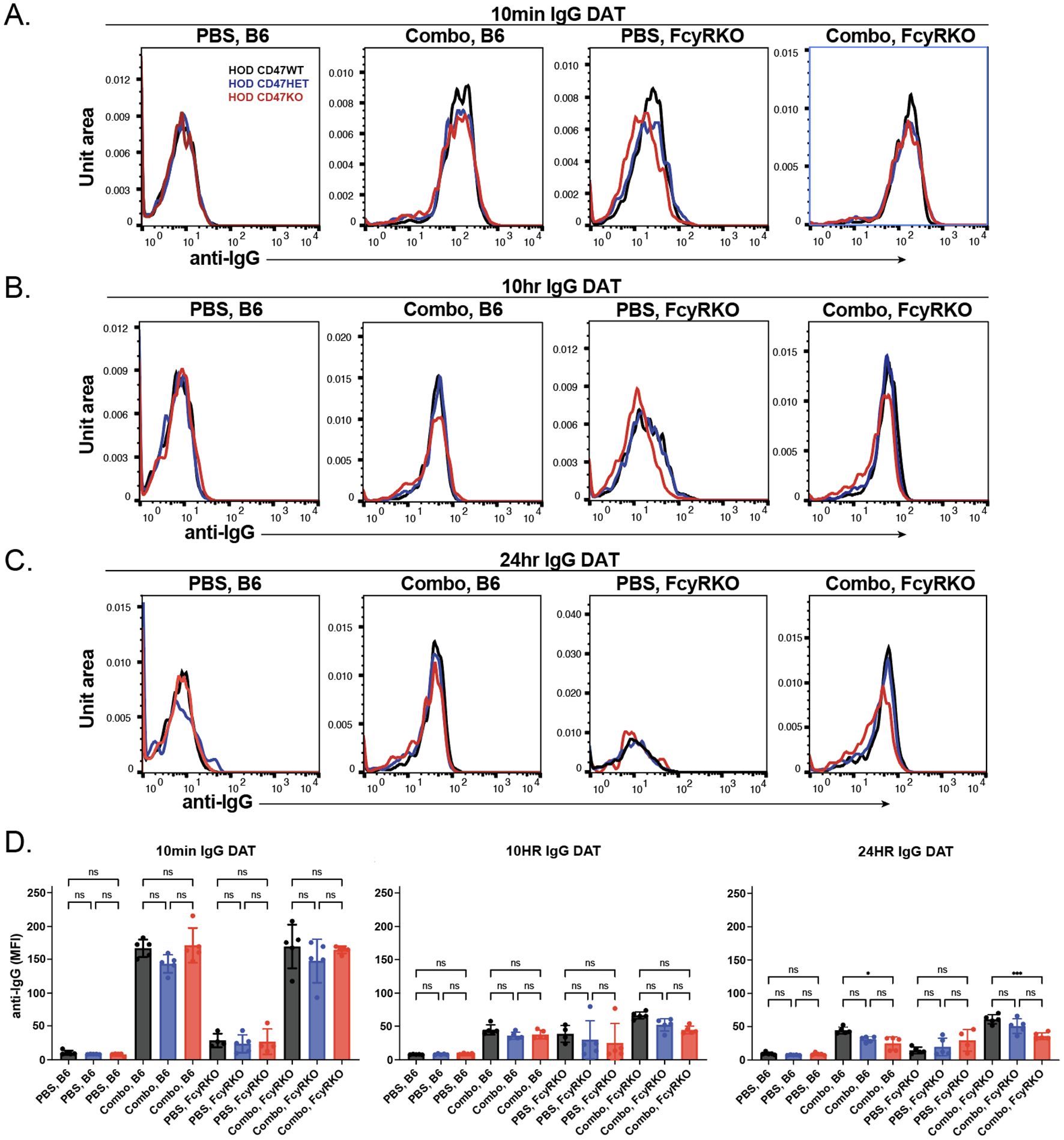
Figure 6. The levels of bound anti-HEL antibodies decrease over time following transfusion of HOD CD47 WT, heterozygous and knockout RBCs into WT and Fcγ receptor immunized recipients. Detection of antibody levels on the surface of HOD CD47 WT, HOD CD47 heterozygous (HET) or HOD CD47 knockout (KO) RBCs following transfusion into WT or Fcγ receptor (FcγR) KO recipients that were either non-immunized (PBS) or immunized with a combination (combo - 2F4 and 4B7) of anti-HEL antibodies shown as histograms at 10 minutes (A), 10 hours (B), and 24 hours (C) following transfusion. (D) Quantification of histogram mean fluorescence intensity (MFI) values of antibody bound on HOD CD47 WT, HOD CD47 HET and HOD CD47 KO RBCs following transfusion into either WT or FcγR KO recipients that were either non-immunized (PBS) or immunized with a combination (combo - 2F4 and 4B7) of anti-HEL antibodies, 10 minutes, 10 hours, and 24 hours post-transfusion. ns = not significant. *p<0.05, ***p < 0.001.
We next sought to determine whether changes in antibody levels on each HOD RBC population following transfusion into WT or FcγR KOs recipients may be accompanied by alterations in the HOD antigen. To this end, we examined HOD antigen levels on transfused RBCs post-transfusion. Similar to the reductions in antibody levels observed following transfusion into each anti-HEL immunized recipients, the levels of the HOD antigen correspondingly decreased on each HOD RBC population following transfusion into anti-HEL immunized WT or FcγR KO recipients (Figure 7A). To determine whether the loss of antigen in each setting was specific to HOD, we next examined whether similar changes in antigen levels occurred to other RBC surface structures. To test this, we examined Ter119, a common marker used to distinguish RBCs from other cellular populations. While levels of detectable antibody and antigen levels decreased over time in immunized recipients, similar changes in Ter119 were not observed (Figure 7B. Consistent with the role of FcγR in the antibody-mediated clearance what was observed following HOD RBC het and HOD RBC KO transfusion, very little detectable complement deposition could be observed (Figure 7C).
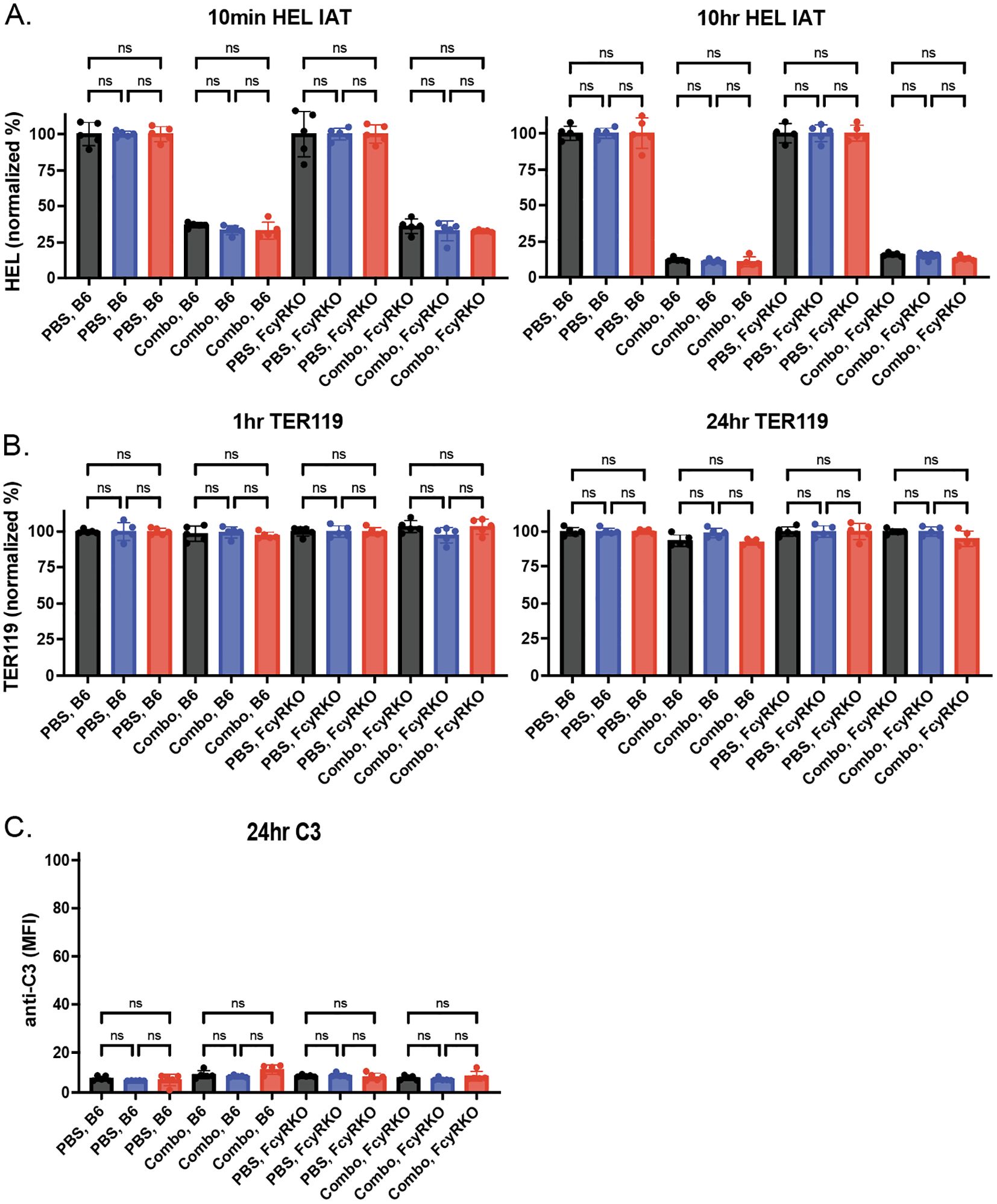
Figure 7. Anti-HEL antibodies reduce levels of HOD without changing Ter119 levels or inducing complement deposition. (A) HOD antigen levels on the surface of HOD CD47 WT, HOD CD47 heterozygous (HET) or HOD CD47 knockout (KO) RBCs following transfusion into WT or Fcγ receptor (FcγR) KO recipients that were either non-immunized (PBS) or immunized with a combination (combo - 2F4 and 4B7) of anti-HEL antibodies shown as histograms at 10 minutes and 10 hours post-transfusion. (B) Levels of Ter119 on the surface of HOD CD47 WT, HOD CD47 HET or HOD CD47 KO RBCs following transfusion into WT or FcγR KO recipients that were either non-immunized (PBS) or immunized with a combination (combo - 2F4 and 4B7) of anti-HEL antibodies shown as histograms at 1 hour and 24 hours post-transfusion. (C) Measurement of C3 deposition on the surface of HOD CD47 WT, HOD CD47 HET or HOD CD47 KO RBCs following transfusion into WT or FcγR KO recipients that were either non-immunized (PBS) or immunized with a combination (combo - 2F4 and 4B7) of anti-HEL antibodies shown as histograms 24 hours post-transfusion. ns = not significant.
Discussion
Our findings reveal that CD47 plays a role in controlling RBC removal and antibody modulation following antibody engagement. These data offer new insights into how key features of RBC might influence their vulnerability to removal following antibody engagement. The ability of CD47 to regulate antibody-mediated removal appears to be dosage dependent, with CD47 levels half that of WT rendering HOD RBCs sensitive to antibody mediated removal while failing to significantly impact RBC survival following transfusion into non-immunized recipients. In contrast, complete loss of CD47 not only impacted HOD RBC survival in the absence of anti-HEL antibodies but appears to further sensitize HOD RBCs to additional antibody-mediated removal through a FcγR-dependent process. Taken together, these data suggest that CD47 may be a key feature of RBCs that regulates the consequences of antibody-mediated removal following an incompatible transfusion.
Due to the unpredictable and often severe outcomes of incompatible blood transfusions, deliberately transfusing patients for the express purpose of defining the outcome of incompatible RBC transfusions is not ethical. However, as some patient populations are particularly prone to developing alloantibodies that can make it very difficult to find fully compatible RBCs (1, 15), defining the key regulators of RBC removal following an incompatible transfusion is key for patients for whom fully compatible blood may not be readily available in a timely manner. To this end, there have been a variety of studies that have leveraged preclinical models to define the impact and outcome of incompatible RBC transfusions (74–76). Early studies utilizing RBCs that express the HEL antigen (membrane bound HEL or mHEL) first identified the ability of antibody engagement to modulate the target antigen in the absence of detectable RBC removal using this approach (77, 78). These studies were followed by similar findings in other model systems, such as the HOD system used in the present study and in model systems that employ distinct target antigens, such as KEL and GPA (74–76, 79–87). In each of these systems, antibody engagement possessed the ability to specifically modulate the target antigen, although the rates of antigen modulation and the overall outcome on RBC survival can differ. These results suggest that the ability of antibodies to modulate a target antigen following engagement may reflect a common outcome that can occur following an incompatible transfusion, though the rate and degree of antigen modulation can vary. It is important to note that similar patterns have been observed in patients treated with antibody-based therapies that target RBC antigens, such as CD38 and RhD (101, 102), suggesting that these observations aren’t limited to preclinical models.
The discovery that antibodies can induce antigen modulation suggests that leveraging this outcome could enhance alterations in the target antigen over RBC elimination in settings where fully compatible RBCs are not available. However, for such possibilities to be realized, the mechanisms responsible for regulating antigen loss over RBC clearance must first be established. Among the natural regulators of RBC removal, CD47 is one of the most well-studied. Initially identified on RBCs as an inhibitor of RBC clearance (95), the interaction between CD47 and SIRPα has been extensively shown to inhibit cellular removal. Cancer cells often exploit CD47 to decrease phagocytic removal, prompting the development of therapies targeting CD47 to improve immune detection and elimination (98). However, the specific influence of CD47 in the context of antibody-driven RBC removal after mismatched transfusions remains relatively unexplored. The differential sensitivity of HOD CD47 HET RBCs to antibody-mediated removal demonstrates that even decreased levels of CD47 can influence the outcome of antibody engagement on RBC survival. Further studies will be needed to determine whether increased levels of CD47 would further protect RBCs otherwise sensitive to antibody-mediated removal from FcγR-mediated clearance. Various iPSC-derived RBC strategies and other forms of RBC modification could possibly be leveraged to enhance the ability of CD47 to inhibit RBC clearance following an incompatible transfusion (103, 104). Manipulation of the CD47-SIRPα axis in the recipient may also represent an alternative avenue to enhance RBC survival following an incompatible transfusion, although much more research needs to be done to determine the feasibility of these possibilities.
The capacity of CD47 to modulate the elimination of RBCs following antibody binding is intriguing and likely reflects to the interplay between the antibody-induced activation of phagocytes via FcγRs and the inhibitory effect of CD47-SIRPα on phagocytosis. Data consistent with this general concept was published decades ago (105, 106), although the molecular mechanisms responsible for regulating phagocytosis remained unknown. Our data suggest that the balance between the inhibitory presence of CD47 and pro- phagocytic effects mediated by FcγR pathways is a key regulator. The ability of antibody engagement to induce antigen modulation likely reflects an evolutionarily conserved pathway whereby antibody bound antigenic material attached to the RBC surface is delivered to sentinel immune cells (107–109). In this setting, the accumulation of immune complexes on the surface of RBCs may provide a mechanism of antigen delivery from distant sites, thereby facilitating a rapid response to blood-borne infection (107–109). The development of alloantibodies following pregnancy or transfusion, followed by re-exposure of RBCs with the target antigen in the setting of clinical practice may mimic this same process. However, in the setting of transfusion, the bolus of RBCs received may quickly overwhelm the ability of antigen modulation to remove antibody-antigen complexes, in contrast to directly causing RBC removal.
While most patients with a positive direct antiglobulin test fail to exhibit significant signs of hemolysis (110), patients with various forms of autoimmune hemolytic anemia clearly possess antibodies capable of causing RBC removal. As a result, a balance between the potency of the antibody to facilitate RBC clearance versus antigen modulation may dictate the ultimate outcome of antibody-antigen interactions on the RBC surface. As CD47 is known to regulate the rate of RBC clearance, it may reflect one factor that regulates this balance between RBC removal and modulation of antigen on the RBC surface following antibody engagement. Interestingly, CD47 can modulate complement, as well as FcγR-dependent cell removal (97). While we have focused on the HOD model in our current study, future studies examining the role of CD47 in other established incompatible RBC transfusion models will provide intriguing insight in the full regulatory potential of CD47 in this setting. In addition to preclinical models of RBC alloimmunization, the recent development of novel models of autoimmune hemolytic anemia will certainly aid in understanding these relationships (111–113).
Although differences in reticulocyte number could theoretically contribute to the rate and magnitude of HOD RBC clearance observed in this study, differences in reticulocytes percentages between HOD CD47 WT versus HOD CD47 KO among the overall donor units were subtle. Consistent with this, there was a lack of a detectable difference in total HEL levels in the peripheral blood among units tested from HOD CD47 WT, HOD CD47 HET and HOD CD47 KO donors. Indeed, only a 1% difference in the total reticulocytes was observed in the total RBC population between HOD CD47 WT versus HOD CD47 KO units. As nearly 25% of the transfused RBCs cleared following antibody engagement of HOD CD47 HET or HOD CD47 KO RBCs, if all the reticulocytes cleared following antibody engagement, it is unlikely that a possible preferential reticulocyte clearance could account for all the antibody-mediated clearance observed following transfusion of HOD CD47 HET or CD47 KO RBCs into immunized recipients. However, it is possible that reticulocytes impact this effect through other, unknown mechanisms and that differences in reticulocyte content may contribute to subtle differences in overall RBC clearance.
While our current work is focused on the effects of CD47 levels on RBC clearance and antigen-modulation following an incompatible transfusion, levels of CD47 may also play an important role in regulating alloimmune responses following transfusion, including antibody-mediated immunosuppression. Changes in the duration of RBC circulation and antigen levels, both of which are modulated by CD47 in the current model, have been suggested to be key factors in the induction of an alloimmune response (114). Additionally, differences in RBC unit composition (%reticulocyte) in HOD CD47 KO when compared to HOD CD47 WT, but not in HOD CD47 HET donors, could also play a role in alloimmunization. Reticulocytes in general displayed a higher level of HOD antigen expression. Whether this difference impacts alloimmunization of each unit in the presence or absence of anti-HEL antibodies remains to be tested. As recent studies have suggested a higher immunogenic potential for reticulocyte-rich RBC units (51), this remains a distinct possibility and should be investigated in future studies.
As with any study, the current study is not without limitations. First and foremost, this is a preclinical model and may or may not reflect similar outcomes following incompatible RBC transfusion in patients. However, as noted previously, given limitations in the ability to deliberately expose patients to incompatible RBCs due to the inability to predict outcomes, similarly defining the impact of antibody engagement following incompatible transfusion clinically remains difficult to routinely study. In cases where patients have received antibody-based treatments that target RBCs, antigen loss has occurred (101, 102). However, whether CD47 regulates this process remains unknown and will require further study. The potential role of CD47 on RBC removal and antigen loss following antibody engagement of target antigens employed in other models of incompatible RBC transfusion biology will also need to be examined. As RBCs have been noted to experience differences in CD47 upon storage (115), this was the primary focus of the present study. Similarly, while our current study design strove to isolate variable levels of CD47 on transfused RBCs, whether global differences in CD47 levels of recipients may also play a role in RBC clearance or alloimmunization following transfusion remains to be explored. While such studies will require the development of similar genetic deletion models of CD47 on distinct RBC model antigen backgrounds, such studies will be important to define the extent to which CD47 regulates distinct forms of antibody-mediated RBC removal. Despite these limitations, the present results suggest that, at least in the HOD model, CD47 may govern the consequences of antibody engagement with implications in key factors that regulate the outcome of incompatible RBC transfusion.
Data availability statement
The raw data supporting the conclusions of this article will be made available by the authors, without undue reservation.
Ethics statement
The animal study was approved by Mass General Brigham IACUC committee. The study was conducted in accordance with the local legislation and institutional requirements.
Author contributions
RJ: Conceptualization, Formal analysis, Investigation, Writing – original draft, Writing – review & editing, Data curation, Methodology, Project administration. MC: Conceptualization, Writing – original draft, Writing – review & editing. JL: Conceptualization, Writing – original draft, Writing – review & editing. LC: Conceptualization, Writing – original draft, Writing – review & editing. PZ: Conceptualization, Writing – original draft, Writing – review & editing. SC: Conceptualization, Writing – original draft, Writing – review & editing. SS: Conceptualization, Writing – original draft, Writing – review & editing, Formal analysis, Funding acquisition, Investigation, Resources. CA: Conceptualization, Data curation, Formal analysis, Funding acquisition, Investigation, Project administration, Resources, Writing – original draft, Writing – review & editing.
Funding
The author(s) declare that financial support was received for the research and/or publication of this article. This work, including salary support, materials and mice, was supported by NHLBI award 1R01HL165975-01, P01HL171803 to SS and NHLBI award 5R01HL154034-04 to CA, NHLBI awards 5K12HL141953-05 and 1K08HL171877-01 to RJ.
Conflict of interest
The authors declare that the research was conducted in the absence of any commercial or financial relationships that could be construed as a potential conflict of interest.
Generative AI statement
The author(s) declare that no Generative AI was used in the creation of this manuscript.
Publisher’s note
All claims expressed in this article are solely those of the authors and do not necessarily represent those of their affiliated organizations, or those of the publisher, the editors and the reviewers. Any product that may be evaluated in this article, or claim that may be made by its manufacturer, is not guaranteed or endorsed by the publisher.
Supplementary material
The Supplementary Material for this article can be found online at: https://www.frontiersin.org/articles/10.3389/fimmu.2025.1548548/full#supplementary-material.
References
1. Yazdanbakhsh K, Ware RE, Noizat-Pirenne F. Red blood cell alloimmunization in sickle cell disease: pathophysiology, risk factors, and transfusion management. Blood. (2012) 120:528–37. doi: 10.1182/blood-2011-11-327361
2. Pirenne F, Floch A, Diop S. Alloimmunisation against red blood cells in sickle cell disease: transfusion challenges in high-income and low-income countries. Lancet Haematol. (2023) 10:e468–76. doi: 10.1016/S2352-3026(23)00066-2
3. Arthur CM, Stowell SR. The development and consequences of red blood cell alloimmunization. Annu Rev Pathol. (2023) 18:537–64. doi: 10.1146/annurev-pathol-042320-110411
4. Yazdanbakhsh K. Mechanisms of sickle cell alloimmunization. Transfus Clin Biol. (2015) 22:178–81. doi: 10.1016/j.tracli.2015.05.005
5. Jajosky RP, Wu SC, Zheng L, Jajosky AN, Jajosky PG, Josephson CD, et al. ABO blood group antigens and differential glycan expression: Perspective on the evolution of common human enzyme deficiencies. iScience. (2023) 26:105798. doi: 10.1016/j.isci.2022.105798
6. Wu SC, Arthur CM, Jan HM, Garcia-Beltran WF, Patel KR, Rathgeber MF, et al. Blood group A enhances SARS-CoV-2 infection. Blood. (2023) 142:742–7. doi: 10.1182/blood.2022018903
7. Wu SC, Jan HM, Vallecillo-Zúniga ML, Rathgeber MF, Stowell CS, Murdock KL, et al. Whole microbe arrays accurately predict interactions and overall antimicrobial activity of galectin-8 toward distinct strains of Streptococcus pneumoniae. Sci Rep. (2023) 13:5324. doi: 10.1038/s41598-023-27964-y
8. Jan HM, Wu SC, Stowell CJ, Vallecillo-Zúniga ML, Paul A, Patel KR, et al. Galectin-4 antimicrobial activity primarily occurs through its C-terminal domain. Mol Cell Proteomics. (2024) 23:100747. doi: 10.1016/j.mcpro.2024.100747
9. Wu SC, Ho AD, Kamili NA, Wang J, Murdock KL, Cummings RD, et al. Full-length galectin-3 is required for high affinity microbial interactions and antimicrobial activity. Front Microbiol. (2021) 12:731026. doi: 10.3389/fmicb.2021.731026
10. Blenda AV, Kamili NA, Wu SC, Abel WF, Ayona D, Gerner-Smidt C, et al. Galectin-9 recognizes and exhibits antimicrobial activity toward microbes expressing blood group-like antigens. J Biol Chem. (2022) 298:101704. doi: 10.1016/j.jbc.2022.101704
11. Wu SC, Kamili NA, Dias-Baruffi M, Josephson CD, Rathgeber MF, Yeung MY, et al. Innate immune Galectin-7 specifically targets microbes that decorate themselves in blood group-like antigens. iScience. (2022) 25:104482. doi: 10.1016/j.isci.2022.104482
12. Vingert B, Tamagne M, Habibi A, Pakdaman S, Ripa J, Elayeb R, et al. Phenotypic differences of CD4(+) T cells in response to red blood cell immunization in transfused sickle cell disease patients. Eur J Immunol. (2015) 45:1868–79. doi: 10.1002/eji.201445187
13. Dean CL, Maier CL, Roback JD, Stowell SR. Multiple hemolytic transfusion reactions misinterpreted as severe vaso-occlusive crisis in a patient with sickle cell disease. Transfusion. (2019) 59:448–53. doi: 10.1111/trf.2019.59.issue-2
14. Meinderts SM, Gerritsma JJ, Sins JWR, de Boer M, van Leeuwen K, Biemond BJ, et al. Identification of genetic biomarkers for alloimmunization in sickle cell disease. Br J Haematol. (2019) 186:887–99. doi: 10.1111/bjh.v186.6
15. Nickel RS, Hendrickson JE, Fasano RM, Meyer EK, Winkler AM, Yee MM, et al. Impact of red blood cell alloimmunization on sickle cell disease mortality: a case series. Transfusion. (2016) 56:107–14. doi: 10.1111/trf.2016.56.issue-1
16. Zhong H, Bao W, Friedman D, Yazdanbakhsh K. Hemin controls T cell polarization in sickle cell alloimmunization. J Immunol. (2014) 193:102–10. doi: 10.4049/jimmunol.1400105
17. Liu Y, Pal M, Bao W, Shi PA, Lobo CA, An X, et al. Type I interferon is induced by hemolysis and drives antibody-mediated erythrophagocytosis in sickle cell disease. Blood. (2021) 138:1162–71. doi: 10.1182/blood.2021011629
18. Madany E, Lee J, Halprin C, Seo J, Baca N, Majlessipour F, et al. Altered type 1 interferon responses in alloimmunized and nonalloimmunized patients with sickle cell disease. EJHaem. (2021) 2:700–10. doi: 10.1002/jha2.v2.4
19. Narbey D, Habibi A, Chadebech P, Mekontso-Dessap A, Khellaf M, Lelièvre JD, et al. Incidence and predictive score for delayed hemolytic transfusion reaction in adult patients with sickle cell disease. Am J Hematol. (2017) 92:1340–8. doi: 10.1002/ajh.v92.12
20. Thein SL, Pirenne F, Fasano RM, Habibi A, Bartolucci P, Chonat S, et al. Hemolytic transfusion reactions in sickle cell disease: underappreciated and potentially fatal. Haematologica. (2020) 105:539–44. doi: 10.3324/haematol.2019.224709
21. Habibi A, Mekontso-Dessap A, Guillaud C, Michel M, Razazi K, Khellaf M, et al. Delayed hemolytic transfusion reaction in adult sickle-cell disease: presentations, outcomes, and treatments of 99 referral center episodes. Am J Hematol. (2016) 91:989–94. doi: 10.1002/ajh.v91.10
22. Vidler JB, Gardner K, Amenyah K, Mijovic A, Thein SL. Delayed haemolytic transfusion reaction in adults with sickle cell disease: a 5-year experience. Br J Haematol. (2015) 169:746–53. doi: 10.1111/bjh.2015.169.issue-5
23. Pirenne F, Pondarre C. Alloimmunization and hyperhemolysis in sickle cell disease. Hematol Am Soc Hematol Educ Program. (2023) 2023:653–9. doi: 10.1182/hematology.2023000499
24. Roumenina LT, Bartolucci P, Pirenne F. The role of complement in post-transfusion hemolysis and hyperhemolysis reaction. Transfus Med Rev. (2019) 33:225–30. doi: 10.1016/j.tmrv.2019.09.007
25. Dumas G, Habibi A, Onimus T, Merle JC, Razazi K, Mekontso Dessap A, et al. Eculizumab salvage therapy for delayed hemolysis transfusion reaction in sickle cell disease patients. Blood. (2016) 127:1062–4. doi: 10.1182/blood-2015-09-669770
26. Chonat S, Mener A, Verkerke H, Stowell SR. Role of complement in alloimmunization and hyperhemolysis. Curr Opin Hematol. (2020) 27:406–14. doi: 10.1097/MOH.0000000000000610
27. Merle NS, Grunenwald A, Rajaratnam H, Gnemmi V, Frimat M, Figueres ML, et al. Intravascular hemolysis activates complement via cell-free heme and heme-loaded microvesicles. JCI Insight. (2018) 3:1–17. doi: 10.1172/jci.insight.96910
28. Merle NS, Paule R, Leon J, Daugan M, Robe-Rybkine T, Poillerat V, et al. P-selectin drives complement attack on endothelium during intravascular hemolysis in TLR-4/heme-dependent manner. Proc Natl Acad Sci U.S.A. (2019) 116:6280–5. doi: 10.1073/pnas.1814797116
29. Gerogianni A, Dimitrov JD, Zarantonello A, Poillerat V, Chonat S, Sandholm K, et al. Heme interferes with complement factor I-dependent regulation by enhancing alternative pathway activation. Front Immunol. (2022) 13:901876. doi: 10.3389/fimmu.2022.901876
30. Arthur CM, Patel SR, Sullivan HC, Winkler AM, Tormey CA, Hendrickson JE, et al. CD8+ T cells mediate antibody-independent platelet clearance in mice. Blood. (2016) 127:1823–7. doi: 10.1182/blood-2015-10-673426
31. Waterman HR, Kapp LM, Munday A, Odem-Davis K, Zimring JC. Transfusion-induced alloimmunization and platelet refractoriness in a mouse model: mechanisms and interventions. Transfusion. (2016) 56:91–100. doi: 10.1111/trf.2016.56.issue-1
32. Chou ST, Alsawas M, Fasano RM, Field JJ, Hendrickson JE, Howard J, et al. American Society of Hematology 2020 guidelines for sickle cell disease: transfusion support. Blood Adv. (2020) 4:327–55. doi: 10.1182/bloodadvances.2019001143
33. Allen ES, Srivastava K, Hsieh MM, Fitzhugh CD, Klein HG, Tisdale JF, et al. Immunohaematological complications in patients with sickle cell disease after haemopoietic progenitor cell transplantation: a prospective, single-centre, observational study. Lancet Haematol. (2017) 4:e553–61. doi: 10.1016/S2352-3026(17)30196-5
34. Saib I, Alahmari B, Alsadi H, Alaskar A, Hejazi A, Salama H, et al. Effect of ABO mismatch and red blood cell alloimmunization on the outcome of hematopoietic cell transplantation for sickle cell disease. Transplant Cell Ther. (2025) 31(1):30.e1–e8. doi: 10.1016/j.jtct.2024.11.003
35. Viayna E, Gehrie EA, Blanchette C, Meny GM, Noumsi G, Huber M, et al. Red cell alloimmunization is associated with increased health care costs, longer hospitalizations, and higher mortality. Blood Adv. (2022) 6:5655–8. doi: 10.1182/bloodadvances.2022006982
36. Vichinsky EP, Earles A, Johnson RA, Hoag MS, Williams A, Lubin B. Alloimmunization in sickle cell anemia and transfusion of racially unmatched blood. N Engl J Med. (1990) 322:1617–21. doi: 10.1056/NEJM199006073222301
37. Chou ST, Jackson T, Vege S, Smith-Whitley K, Friedman DF, Westhoff CM High prevalence of red blood cell alloimmunization in sickle cell disease despite transfusion from Rh-matched minority donors. Blood. (2013) 122:1062–71. doi: 10.1182/blood-2013-03-490623
38. Zheng Y, Gossett JM, Chen PL, Barton M, Ryan M, Yu J, et al. Proinflammatory state promotes red blood cell alloimmunization in pediatric patients with sickle cell disease. Blood Adv. (2023) 7:4799–808. doi: 10.1182/bloodadvances.2022008647
39. Zerra PE, Patel SR, Jajosky RP, Arthur CM, McCoy JW, Allen JWL, et al. Marginal zone B cells mediate a CD4 T-cell-dependent extrafollicular antibody response following RBC transfusion in mice. Blood. (2021) 138:706–21. doi: 10.1182/blood.2020009376
40. Jash A, Pridmore T, Collins JB, Hay AM, Hudson KE, Luckey CJ, et al. Complement C3 and marginal zone B cells promote IgG-mediated enhancement of RBC alloimmunization in mice. J Clin Invest. (2024) 134:1–14. doi: 10.1172/JCI167665
41. Mener A, Patel SR, Arthur CM, Chonat S, Wieland A, Santhanakrishnan M, et al. Complement serves as a switch between CD4+ T cell-independent and -dependent RBC antibody responses. JCI Insight. (2018) 3:1–19. doi: 10.1172/jci.insight.121631
42. Maier CL, Jajosky RP, Patel SR, Verkerke HP, Fuller MD, Allen JW, et al. Storage differentially impacts alloimmunization to distinct red cell antigens following transfusion in mice. Transfusion. (2023) 63:457–62. doi: 10.1111/trf.17251
43. Arneja A, Salazar JE, Jiang W, Hendrickson JE, Zimring JC, Luckey CJ. Interleukin-6 receptor-alpha signaling drives anti-RBC alloantibody production and T-follicular helper cell differentiation in a murine model of red blood cell alloimmunization. Haematologica. (2016) 101:e440–4. doi: 10.3324/haematol.2016.149278
44. Jajosky RP, Patel KR, Allen JWL, Zerra PE, Chonat S, Ayona D, et al. Antibody-mediated antigen loss switches augmented immunity to antibody-mediated immunosuppression. Blood. (2023) 142:1082–98. doi: 10.1182/blood.2022018591
45. Patel SR, Bennett A, Girard-Pierce K, Maier CL, Chonat S, Arthur CM, et al. Recipient priming to one RBC alloantigen directly enhances subsequent alloimmunization in mice. Blood Adv. (2018) 2:105–15. doi: 10.1182/bloodadvances.2017010124
46. Patel SR, Gibb DR, Girard-Pierce K, Zhou X, Rodrigues LC, Arthur CM, et al. Marginal zone B cells induce alloantibody formation following RBC transfusion. Front Immunol. (2018) 9:2516. doi: 10.3389/fimmu.2018.02516
47. Gibb DR, Liu J, Natarajan P, Santhanakrishnan M, Madrid DJ, Eisenbarth SC, et al. Type I IFN is necessary and sufficient for inflammation-induced red blood cell alloimmunization in mice. J Immunol. (2017) 199:1041–50. doi: 10.4049/jimmunol.1700401
48. Gibb DR, Liu J, Santhanakrishnan M, Natarajan P, Madrid DJ, Patel S, et al. B cells require Type 1 interferon to produce alloantibodies to transfused KEL-expressing red blood cells in mice. Transfusion. (2017) 57:2595–608. doi: 10.1111/trf.2017.57.issue-11
49. Zerra PE, Cox C, Baldwin WH, Patel SR, Arthur CM, Lollar P, et al. Marginal zone B cells are critical to factor VIII inhibitor formation in mice with hemophilia A. Blood. (2017) 130:2559–68. doi: 10.1182/blood-2017-05-782912
50. Arthur CM, Zerra PE, Shin S, Wang J, Song X, Doering CB, et al. Nonhuman glycans can regulate anti-factor VIII antibody formation in mice. Blood. (2022) 139:1312–7. doi: 10.1182/blood.2020009210
51. Thomas TA, Qiu A, Kim CY, Gordy DE, Miller A, Tredicine M, et al. Reticulocytes in donor blood units enhance red blood cell alloimmunization. Haematologica. (2023) 108:2639–51. doi: 10.3324/haematol.2023.282815
52. Soldatenko A, Hoyt LR, Xu L, Calabro S, Lewis SM, Gallman AE, et al. Innate and adaptive immunity to transfused allogeneic RBCs in mice requires myD88. J Immunol. (2022) 208:991–7. doi: 10.4049/jimmunol.2100784
53. Zerra PE, Arthur CM, Chonat S, Maier CL, Mener A, Shin S, et al. Fc gamma receptors and complement component 3 facilitate anti-fVIII antibody formation. Front Immunol. (2020) 11:905. doi: 10.3389/fimmu.2020.00905
54. Stowell SR, Smith NH, Zimring JC, Fu X, Palmer AF, Fontes J, et al. Addition of ascorbic acid solution to stored murine red blood cells increases posttransfusion recovery and decreases microparticles and alloimmunization. Transfusion. (2013) 53:2248–57. doi: 10.1111/trf.2013.53.issue-10
55. Jajosky R, Patel SR, Wu SC, Patel K, Covington M, Vallecillo-Zúniga M, et al. Prior immunization against an intracellular antigen enhances subsequent red blood cell alloimmunization in mice. Blood. (2023) 141:2642–53. doi: 10.1182/blood.2022016588
56. Jardine L, Publicover A, Bigley V, Hale G, Pearce K, Dickinson A, et al. A comparative study of reduced dose alemtuzumab in matched unrelated donor and related donor reduced intensity transplants. Br J Haematol. (2015) 168:874–81. doi: 10.1111/bjh.13239
57. Campbell-Lee SA, Gvozdjan K, Choi KM, Chen YF, Saraf SL, Hsu LL, et al. Red blood cell alloimmunization in sickle cell disease: assessment of transfusion protocols during two time periods. Transfusion. (2018) 58:1588–96. doi: 10.1111/trf.2018.58.issue-7
58. Zheng Y, Pollak J, Henderson K, Hendrickson JE, Tormey CA. A novel association between high red blood cell alloimmunization rates and hereditary hemorrhagic telangiectasia. Transfusion. (2018) 58:775–80. doi: 10.1111/trf.2018.58.issue-3
59. Tamagne M, Pakdaman S, Bartolucci P, Habibi A, Galactéros F, Pirenne F, et al. Whole-blood phenotyping to assess alloimmunization status in transfused sickle cell disease patients. Blood Adv. (2021) 5:1278–82. doi: 10.1182/bloodadvances.2020003537
60. Gerritsma J, Oomen I, Meinderts S, van der Schoot CE, Biemond BJ, van der Bom JG, et al. Back to base pairs: What is the genetic risk for red bloodcell alloimmunization? Blood Rev. (2021) 48:100794. doi: 10.1016/j.blre.2020.100794
61. Noizat-Pirenne F, Tournamille C, Bierling P, Roudot-Thoraval F, Le Pennec PY, Rouger P, et al. Relative immunogenicity of Fya and K antigens in a Caucasian population, based on HLA class II restriction analysis. Transfusion. (2006) 46:1328–33. doi: 10.1111/j.1537-2995.2006.00900.x
62. Sippert E.Â., Visentainer JE, Alves HV, Rodrigues C, Gilli SC, Addas-Carvalho M, et al. Red blood cell alloimmunization in patients with sickle cell disease: correlation with HLA and cytokine gene polymorphisms. Transfusion. (2017) 57:379–89. doi: 10.1111/trf.13920
63. Evers D, van der Bom JG, Tijmensen J, de Haas M, Middelburg RA, de Vooght K, et al. Splenectomy protects humans from red cell alloimmunization. Blood. (2016) 128(22):24. doi: 10.1182/blood.V128.22.24.24
64. Karafin MS, Tan S, Tormey CA, Spencer BR, Hauser RG, Norris PJ, et al. Prevalence and risk factors for RBC alloantibodies in blood donors in the Recipient Epidemiology and Donor Evaluation Study-III (REDS-III). Transfusion. (2019) 59:217–25. doi: 10.1111/trf.2019.59.issue-1
65. Evers D, Zwaginga JJ, Tijmensen J, Middelburg RA, de Haas M, de Vooght KM, et al. Treatments for hematologic Malignancies in contrast to those for solid cancers are associated with reduced red cell alloimmunization. Haematologica. (2017) 102:52. doi: 10.3324/haematol.2016.152074
66. Nakahara H, Cheedarla N, Verkerke HP, Cheedarla S, Wu SC, Hendrickson JE, et al. Enhanced IgG immune response to COVID-19 vaccination in patients with sickle cell disease. Br J Haematol. (2023) 202:937–41. doi: 10.1111/bjh.18899
67. Pirenne F, Yazdanbakhsh K. How I safely transfuse patients with sickle-cell disease and manage delayed hemolytic transfusion reactions. Blood. (2018) 131:2773–81. doi: 10.1182/blood-2018-02-785964
68. Tormey CA, Hendrickson JE. Transfusion-related red blood cell alloantibodies: induction and consequences. Blood. (2019) 133:1821–30. doi: 10.1182/blood-2018-08-833962
69. Campbell-Lee SA, Kittles RA. Red blood cell alloimmunization in sickle cell disease: listen to your ancestors. Transfus Med Hemother. (2014) 41:431–5. doi: 10.1159/000369513
70. Chou ST, Evans P, Vege S, Coleman SL, Friedman DF, Keller M, et al. RH genotype matching for transfusion support in sickle cell disease. Blood. (2018) 132:1198–207. doi: 10.1182/blood-2018-05-851360
71. Chou ST, Liem RI, Thompson AA. Challenges of alloimmunization in patients with haemoglobinopathies. Br J Haematol. (2012) 159:394–404. doi: 10.1111/bjh.2012.159.issue-4
72. Fasano RM, Sullivan HC, Bray RA, Gebel HM, Meyer EK, Winkler AM, et al. Genotyping applications for transplantation and transfusion management: the emory experience. Arch Pathol Lab Med. (2017) 141:329–40. doi: 10.5858/arpa.2016-0277-SA
73. Chang DY, Wankier Z, Arthur CM, Stowell SR. The ongoing challenge of RBC alloimmunization in the management of patients with sickle cell disease. Presse Med. (2023) 52:104211. doi: 10.1016/j.lpm.2023.104211
74. Hod EA, Cadwell CM, Liepkalns JS, Zimring JC, Sokol SA, Schirmer DA, et al. Cytokine storm in a mouse model of IgG-mediated hemolytic transfusion reactions. Blood. (2008) 112:891–4. doi: 10.1182/blood-2008-01-132092
75. Hod EA, Zimring JC, Spitalnik SL. Lessons learned from mouse models of hemolytic transfusion reactions. Curr Opin Hematol. (2008) 15:601–5. doi: 10.1097/MOH.0b013e328311f40a
76. Zimring JC, Spitalnik SL. Pathobiology of transfusion reactions. Annu Rev Pathol. (2015) 10:83–110. doi: 10.1146/annurev-pathol-012414-040318
77. Zimring JC, Hair GA, Chadwick TE, Deshpande SS, Anderson KM, Hillyer CD, et al. Nonhemolytic antibody-induced loss of erythrocyte surface antigen. Blood. (2005) 106:1105–12. doi: 10.1182/blood-2005-03-1040
78. Zimring JC, Cadwell CM, Chadwick TE, Spitalnik SL, Schirmer DA, Wu T, et al. Nonhemolytic antigen loss from red blood cells requires cooperative binding of multiple antibodies recognizing different epitopes. Blood. (2007) 110:2201–8. doi: 10.1182/blood-2007-04-083097
79. Girard-Pierce KR, Stowell SR, Smith NH, Arthur CM, Sullivan HC, Hendrickson JE, et al. A novel role for C3 in antibody-induced red blood cell clearance and antigen modulation. Blood. (2013) 122:1793–801. doi: 10.1182/blood-2013-06-508952
80. Liepkalns JS, Cadwell CM, Stowell SR, Hod EA, Spitalnik SL, Zimring JC. Resistance of a subset of red blood cells to clearance by antibodies in a mouse model of incompatible transfusion. Transfusion. (2013) 53:1319–27. doi: 10.1111/j.1537-2995.2012.03910.x
81. Stowell SR, Henry KL, Smith NH, Hudson KE, Halverson GR, Park JC, et al. Alloantibodies to a paternally derived RBC KEL antigen lead to hemolytic disease of the fetus/newborn in a murine model. Blood. (2013) 122:1494–504. doi: 10.1182/blood-2013-03-488874
82. Stowell SR, Liepkalns JS, Hendrickson JE, Girard-Pierce KR, Smith NH, Arthur CM, et al. Antigen modulation confers protection to red blood cells from antibody through Fcgamma receptor ligation. J Immunol. (2013) 191:5013–25. doi: 10.4049/jimmunol.1300885
83. Arthur CM, Allen JWL, Verkerke H, Yoo J, Jajosky RP, Girard-Pierce K, et al. Antigen density dictates RBC clearance, but not antigen modulation, following incompatible RBC transfusion in mice. Blood Adv. (2021) 5:527–38. doi: 10.1182/bloodadvances.2020002695
84. Bernardo L, Yu H, Amash A, Zimring JC, Lazarus AH. IgG-mediated immune suppression to erythrocytes by polyclonal antibodies can occur in the absence of activating or inhibitory fcgamma receptors in a full mouse model. J Immunol. (2015) 195:2224–30. doi: 10.4049/jimmunol.1500790
85. Yu H, Stowell SR, Bernardo L, Hendrickson JE, Zimring JC, Amash A, et al. Antibody-mediated immune suppression of erythrocyte alloimmunization can occur independently from red cell clearance or epitope masking in a murine model. J Immunol. (2014) 193:2902–10. doi: 10.4049/jimmunol.1302287
86. Maier CL, Mener A, Patel SR, Jajosky RP, Bennett AL, Arthur CM, et al. Antibody-mediated immune suppression by antigen modulation is antigen-specific. Blood Adv. (2018) 2:2986–3000. doi: 10.1182/bloodadvances.2018018408
87. Mener A, Patel SR, Arthur CM, Stowell SR. Antibody-mediated immunosuppression can result from RBC antigen loss independent of Fcgamma receptors in mice. Transfusion. (2019) 59:371–84. doi: 10.1111/trf.2019.59.issue-1
88. Hendrickson JE, Hod EA, Cadwell CM, Eisenbarth SC, Spiegel DA, Tormey CA, et al. Rapid clearance of transfused murine red blood cells is associated with recipient cytokine storm and enhanced alloimmunogenicity. Transfusion. (2011) 51:2445–54. doi: 10.1111/j.1537-2995.2011.03162.x
89. Jajosky RP, Ayona D, Mener A, Stowell SR, Arthur CM. Dynamics of antibody engagement of red blood cells. Vivo vitro. Front Immunol. (2024) 15:1475470. doi: 10.3389/fimmu.2024.1475470
90. Liepkalns JS, Hod EA, Stowell SR, Cadwell CM, Spitalnik SL, Zimring JC. Biphasic clearance of incompatible red blood cells through a novel mechanism requiring neither complement nor Fcgamma receptors in a murine model. Transfusion. (2012) 52:2631–45. doi: 10.1111/j.1537-2995.2012.03647.x
91. Stowell SR, Arthur CM, Girard-Pierce KR, Sullivan HC, Santhanakrishnan M, Natarajan P, et al. Anti-KEL sera prevents alloimmunization to transfused KEL RBCs in a murine model. Haematologica. (2015) 100:e394–397. doi: 10.3324/haematol.2015.128603
92. Gruber DR, Richards AL, Howie HL, Hay AM, Lebedev JN, Wang X, et al. Passively transferred IgG enhances humoral immunity to a red blood cell alloantigen in mice. Blood Adv. (2020) 4:1526–37. doi: 10.1182/bloodadvances.2019001299
93. Shinde P, Howie HL, Stegmann TC, Hay AM, Waterman HR, Szittner Z, et al. IgG subclass determines suppression versus enhancement of humoral alloimmunity to kell RBC antigens in mice. Front Immunol. (2020) 11:1516. doi: 10.3389/fimmu.2020.01516
94. Jash A, Usaneerungrueng C, Howie HL, Qiu A, Luckey CJ, Zimring JC, et al. Antibodies to low-copy number RBC alloantigen convert a tolerogenic stimulus to an immunogenic stimulus in mice. Front Immunol. (2021) 12:629608. doi: 10.3389/fimmu.2021.629608
95. Oldenborg PA, Zheleznyak A, Fang YF, Lagenaur CF, Gresham HD, Lindberg FP. Role of CD47 as a marker of self on red blood cells. Science. (2000) 288:2051–4. doi: 10.1126/science.288.5473.2051
96. Brown E, Hooper L, Ho T, Gresham H. Integrin-associated protein: a 50-kD plasma membrane antigen physically and functionally associated with integrins. J Cell Biol. (1990) 111:2785–94. doi: 10.1083/jcb.111.6.2785
97. Oldenborg PA, Gresham HD, Lindberg FP. CD47-signal regulatory protein alpha (SIRPalpha) regulates Fcgamma and complement receptor-mediated phagocytosis. J Exp Med. (2001) 193:855–62. doi: 10.1084/jem.193.7.855
98. Chao MP, Weissman IL, Majeti R. The CD47-SIRPalpha pathway in cancer immune evasion and potential therapeutic implications. Curr Opin Immunol. (2012) 24:225–32. doi: 10.1016/j.coi.2012.01.010
99. Desmarets M, Cadwell CM, Peterson KR, Neades R, Zimring JC. Minor histocompatibility antigens on transfused leukoreduced units of red blood cells induce bone marrow transplant rejection in a mouse model. Blood. (2009) 114:2315–22. doi: 10.1182/blood-2009-04-214387
100. Mener A, Arthur CM, Patel SR, Liu J, Hendrickson JE, Stowell SR. Complement component 3 negatively regulates antibody response by modulation of red blood cell antigen. Front Immunol. (2018) 9:676. doi: 10.3389/fimmu.2018.00676
101. Sullivan HC, Arthur CM, Thompson L, Patel SR, Stowell SR, Hendrickson JE, et al. Anti-RhD reduces levels of detectable RhD antigen following anti-RhD infusion. Transfusion. (2018) 58:542–4. doi: 10.1111/trf.2018.58.issue-2
102. Sullivan HC, Gerner-Smidt C, Nooka AK, Arthur CM, Thompson L, Mener A, et al. Daratumumab (anti-CD38) induces loss of CD38 on red blood cells. Blood. (2017) 129:3033–7. doi: 10.1182/blood-2016-11-749432
103. Deng J, Lancelot M, Jajosky R, Deng Q, Deeb K, Saakadze N, et al. Erythropoietic properties of human induced pluripotent stem cells-derived red blood cells in immunodeficient mice. Am J Hematol. (2022) 97:194–202. doi: 10.1002/ajh.26410
104. An HH, Gagne AL, Maguire JA, Pavani G, Abdulmalik O, Gadue P, et al. The use of pluripotent stem cells to generate diagnostic tools for transfusion medicine. Blood. (2022) 140:1723–34. doi: 10.1182/blood.2022015883
105. LoBuglio AF, Cotran RS, Jandl JH. Red cells coated with immunoglobulin G: binding and sphering by mononuclear cells in man. Science. (1967) 158:1582–5. doi: 10.1126/science.158.3808.1582
106. Abramson N, Lo Buglio AF, Jandl JH, Cotran RS. The interaction between human monocytes and red cells. Binding characteristics. J Exp Med. (1970) 132:1191–206. doi: 10.1084/jem.132.6.1191
107. Sherwood TA, Virella G. The binding of immune complexes to human red cells: complement requirements and fate of the RBC-bound IC after interaction with human phagocytic cells. Clin Exp Immunol. (1986) 64:195–204.
108. Nardin A, Lindorfer MA, Taylor RP. How are immune complexes bound to the primate erythrocyte complement receptor transferred to acceptor phagocytic cells? Mol Immunol. (1999) 36:827–35.
109. Craig ML, Waitumbi JN, Taylor RP. Processing of C3b-opsonized immune complexes bound to non-complement receptor 1 (CR1) sites on red cells: phagocytosis, transfer, and associations with CR1. J Immunol. (2005) 174:3059–66. doi: 10.4049/jimmunol.174.5.3059
110. Zarandona JM, Yazer MH. The role of the Coombs test in evaluating hemolysis in adults. CMAJ. (2006) 174:305–7. doi: 10.1503/cmaj.051489
111. Dei Zotti F, Qiu A, D'Agati VD, Jagnarine S, Kyritsis E, Miller A, et al. Mitigation of checkpoint inhibitor-induced autoimmune hemolytic anemia through modulation of purinergic signaling. Blood. (2024) 144(15):1581–94. doi: 10.1182/blood.2024024230
112. Dei Zotti F, Qiu A, La Carpia F, Moriconi C. amp]]amp; Hudson, K.E. A new murine model of primary autoimmune hemolytic anemia (AIHA). Front Immunol. (2021) 12:752330. doi: 10.3389/fimmu.2021.752330
113. Howie HL, Hudson KE. Murine models of autoimmune hemolytic anemia. Curr Opin Hematol. (2018) 25:473–81. doi: 10.1097/MOH.0000000000000459
114. Ryder AB, Zimring JC, Hendrickson JE. Factors influencing RBC alloimmunization: lessons learned from murine models. Transfus Med Hemother. (2014) 41:406–19. doi: 10.1159/000368995
Keywords: red blood cell, alloimmunization, incompatible transfusion, antibodies, antigen modulation
Citation: Jajosky RP, Covington ML, Liu J, Chai L, Zerra PE, Chonat S, Stowell SR and Arthur CM (2025) CD47 regulates antigen modulation and red blood cell clearance following an incompatible transfusion. Front. Immunol. 16:1548548. doi: 10.3389/fimmu.2025.1548548
Received: 19 December 2024; Accepted: 21 February 2025;
Published: 04 April 2025.
Edited by:
Magali J. Fontaine, University of Maryland, United StatesReviewed by:
Arijita Jash, University of Virginia, United StatesRosario Hernández Armengol, Cedars Sinai Medical Center, United States
Copyright © 2025 Jajosky, Covington, Liu, Chai, Zerra, Chonat, Stowell and Arthur. This is an open-access article distributed under the terms of the Creative Commons Attribution License (CC BY). The use, distribution or reproduction in other forums is permitted, provided the original author(s) and the copyright owner(s) are credited and that the original publication in this journal is cited, in accordance with accepted academic practice. No use, distribution or reproduction is permitted which does not comply with these terms.
*Correspondence: Sean R. Stowell, c3JzdG93ZWxsQGJ3aC5oYXJ2YXJkLmVkdQ==; Connie M. Arthur, Y21hcnRodXJAYndoLmhhcnZhcmQuZWR1
†These authors have contributed equally to this work