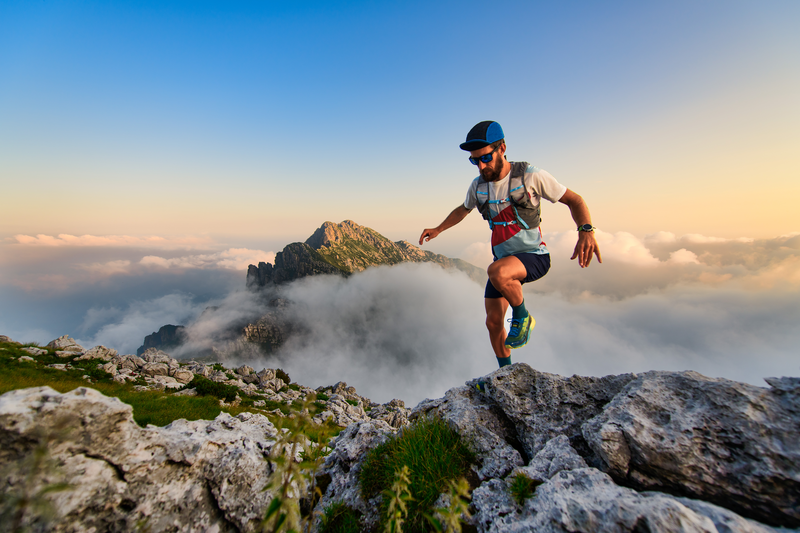
94% of researchers rate our articles as excellent or good
Learn more about the work of our research integrity team to safeguard the quality of each article we publish.
Find out more
EDITORIAL article
Front. Immunol. , 15 January 2025
Sec. B Cell Biology
Volume 16 - 2025 | https://doi.org/10.3389/fimmu.2025.1533646
This article is part of the Research Topic Community Series in BCR Signaling and B Cell Activation: Volume 2 View all 8 articles
Editorial on the Research Topic
Community series in BCR signaling and B cell activation, volume 2
B cell receptor (BCR) signaling plays a fundamental role in B cell development, activation, and antibody responses. The BCR consists of a membrane immunoglobulin (mIg), the antigen recognition subunit, and CD79a and CD79b heterodimer, the signal transducing subunit (1). It belongs to a family of receptors that do not contain intrinsic kinases and transduces signals by interacting with other signaling proteins and organizing supramolecular complexes. While BCR activation mechanisms have been studied extensively, many aspects remain controversial. With the development of new cellular and molecular tools, different models have been proposed. Accumulating evidence points to spatial reorganization of surface BCRs upon antigen binding. In the cross-linking model (CLM), BCR complexes in the resting state reside as monomers. When encountering multivalent antigens but not monovalent antigens, BCR monomers are cross-linked to initiate BCR signaling cascades. It was observed since 1967 that the BCR could be activated only by multivalent antigens, such as the F(ab)2 fragment of anti-BCR antibody rather than its Fab fragment of the antibody, favoring the CLM (2). Direct experimental evidence of the CLM came from fluorescence resonance energy transfer (FRET) microscopy (3), which demonstrates BCR-BCR interactions in living cells.
Although CLM explains the mechanism of BCR triggering by multivalent antigens, CLM cannot explain B cell responses by small-sized soluble antigens. In the conformation-induced oligomerization model, the binding of membrane-bound monovalent antigens leads to surface BCR oligomerization, which is mediated by conformationally unmasked Fc interfaces (4). However, as the conformational unmasking requires some physical forces between epitope and paratope, this model cannot easily explain the BCR triggering by the soluble format of monovalent antigens (monovalent soluble antigen).
B cell response to monovalent soluble antigen may be better explained by the dissociation activation model. This model proposes that surface BCRs exist in an autoinhibitory oligomeric state in resting B cells, and BCR oligomers are dissociated upon antigen binding, which exposes phosphorylation sites of the Immunoreceptor tyrosine-based activation motifs (ITAMs) in the intracellular domain of CD79a/CD79b heterodimer and thus activates B cells (5).
Recently, the conformational change model has been proposed to explain how the signal from the extracellular domain interacting with different formats of external antigens can be transduced to the BCR intracellular portion (6). Here, the antigen-induced conformational changes of mIg could be transduced into conformational changes in the spatial relationship between CD79a and CD79b without the pre-requisition of BCR oligomerization. The conformational change of Fc region by antigen binding was also shown in secretory IgE (7). The reorientation of IgE Cϵ2 domains and intricate binding of FcϵRI to IgE Fc region introduced conformational changes and asymmetrical folding back of an IgE Cϵ2 domain on Cϵ4 and Cϵ3, explaining persistent activation of mast cells by IgE binding to allergen.
The functional status of the BCR is controlled and regulated by self-aggregation and its interaction with various partners in the membrane, such as the transmembrane phosphatase CD45 and the stimulatory coreceptor CD19. However, the structural basis for such dynamic interactions remains a challenging question. Recently published cryo-electron microscopic studies confirm the asymmetric organization of the BCR with one CD79a/CD79b heterodimer associating with one mIg and revealed structural interfaces between mIg, CD79a, and CD79b in the membrane and the extracellular space (8–10). Through analyzing the amino acid sequences and three-dimensional structural folding of the transmembrane domains of mIg, CD79a, CD79b, and their interacting partners, as well as other receptors and receptor complexes, in this Research Topic, Reth identified evolutionary conserved leucine zipper motifs in their transmembrane α-helixes. He further proposed potential functions for such motifs in mediating the interactions between mIg and the CD79a/CD79b heterodimer within the BCR and between the BCR and its transmembrane interacting partners, facilitating the formation of different immunoreceptor organizations and signal complexes. Therefore, this motif was named as an immunoreceptor organization and coupling motif (ICOM). While the function of the ICOM motifs remains to be tested, the proposed hypothesis is highly intriguing.
One of the earliest signal events in BCR activation is Ca2+ flux, which is critical for B cell development, proliferation, and differentiation (12, 13). Ca2+ flux is triggered by BCR-induced activation of phospholipase Cγ2, which generates inositol 1,4,5-triphosphate (IP3). IP3 activates the IP3 receptor on the endoplasmic reticulum (ER), leading to Ca2+ release from the ER. Decreased ER Ca2+ levels activate stromal interaction molecule (STIM) proteins on the ER membrane. Active STIM binds to and opens calcium release-activated calcium (CRAC) channels on the plasma membrane, leading to Ca2+ influx (11, 12). How BCR-induced Ca2+ influx is regulated and the roles of Ca2+ influx in different activation, differential, and functional stages of B cells remain elusive. In this Research Topic, Mahtani et al. investigated the role of a member of the calcium-permeable transient receptor potential ion channel family, TRPV5, in B cells using a TRPV5 knockout mouse model. Even though TRPV5 knockout did not significantly impact Ca2+ influx induced by soluble antigen and B cell development, it did cause impaired B cell spreading and contraction in response to membrane-bound antigens and reduced BCR signaling and early T-dependent antigen-specific responses. Also, in this Research Topic, Riobo and Yuseff examined the remodeling of the ER, an intracellular Ca2+ storage, during the immune synapse formation and found that the ER was redistributed towards the immune synapses and around the microtubule organization center in response to membrane-bound antigens. Such ER reorganization was regulated by the stiffness of the antigen-presenting surface. However, the relationship between this antigen-triggered ER reorganization and Ca2+ signal regulation has yet to be examined.
BCR signaling regulation is essential to maintain the balance of appropriate B cell activation toward non-self-antigens but not self-antigens. Although the antigen-binding affinity of the BCR is generally translated into the strength of BCR signaling, the fine-tuning of BCR signaling is required to generate effective antibody responses and prevent autoreactive responses. The BCR signaling is finely tuned by the interaction of the BCR with signaling molecules or costimulatory molecules. In this Research Topic, Hong and Kwak discussed specific traits of antigens with pathogenic potential and their impacts on BCR signaling and B cells’ ability to distinguish between self and non-self-antigens. The physical properties of antigens, such as solubility or stiffness and antigen spacing or valency, can regulate the organization of the BCR signalosomes or dynamic interactions among the signaling molecules. Proximal BCR signaling is initially triggered via tyrosine phosphorylation of ITAMs in the cytoplasmic tails of CD79a and CD79b by the Src family or Syk tyrosine kinases. While the role of tyrosine kinases in BCR signaling is well known, the role of serine/threonine kinase (STK) in BCR signaling is not completely understood. Han et al. discussed the role of various STKs in B cell development and antibody function.
The tonic BCR signaling that is independent of antigenic engagement is essential for B cell development and survival, but its establishment and maintenance remain elusive. The constitutive activation of PI-3 kinase has been shown to rescue the B cell survival signaling in BCR-deficient B cells (13). Research Topic, Pérez-Pérez et al. reported that Lrba-/- B cells showed heightened basal signaling, such as elevated NF-κB activation and Nur77 expression, but were defective in responding to BCR engagement, suggesting the involvement of LRBA in basal or tonic BCR signaling. In this Research Topic, Rossmanith et al. investigated the B cell defects in patients with ERCC2 mutation that is associated with trichothiodystrophy-1 (TTD1) and nucleotide excision repair. Patients with ERCC2 mutations exhibited hypogammaglobulinemia, decreased antibody responses upon vaccination, increased radiosensitivity, and decreased naive and transitional B cells, but the mechanism of defective BCR signaling in ERCC2-mutated B cells is still poorly understood.
This Research Topic brings together current understanding and recent advances in BCR signaling mechanisms, regulation, and their implications in B cell function and disease. The collected studies provide new insights into the complex molecular mechanisms underlying B cell activation and regulation, contributing to our understanding of immune responses and potential therapeutic targets.
WS: Investigation, Supervision, Writing – original draft, Writing – review & editing. TK: Investigation, Supervision, Writing – original draft, Writing – review & editing. WL: Conceptualization, Investigation, Writing – original draft, Writing – review & editing.
The author(s) declare that financial support was received for the research, authorship, and/or publication of this article. This work was supported by grants from Ministry of Science and Technology of China Grants (2021YFC2300500 and 2021YFC2302403), National Natural Science Foundation of China (32430035 and 32141004), Shenzhen Medical Research Fund (B2402012 and C2404002) and Beijing Natural Science Foundation (Z230014) to WL. This study was also supported by research funds from Center for Life Sciences, Institute for Immunology at Tsinghua University, and Vanke School of Public Health at Tsinghua University to WL. TK was supported by National Research Foundation of Korea grant funded by the Korea government (MSIT) (2023R1A2C2004510 and RS-2024-00405650).
The authors declare that the research was conducted in the absence of any commercial or financial relationships that could be construed as a potential conflict of interest.
The author(s) declared that they were an editorial board member of Frontiers, at the time of submission. This had no impact on the peer review process and the final decision.
All claims expressed in this article are solely those of the authors and do not necessarily represent those of their affiliated organizations, or those of the publisher, the editors and the reviewers. Any product that may be evaluated in this article, or claim that may be made by its manufacturer, is not guaranteed or endorsed by the publisher.
1. Reth M. Antigen receptors on B lymphocytes. Annu Rev Immunol. (1992) 10:97–121. doi: 10.1146/annurev.iy.10.040192.000525
2. Woodruff MF, Reid B, James K. Effect of antilymphocytic antibody and antibody fragments on human lymphocytes. Vitro Nature. (1967) 215:591–4. doi: 10.1038/215591a0
3. Tolar P, Sohn HW, Pierce SK. The initiation of antigen-induced B cell antigen receptor signaling viewed in living cells by fluorescence resonance energy transfer. Nat Immunol. (2005) 6:1168–76. doi: 10.1038/ni1262
4. Tolar P, Pierce SK. A conformation-induced oligomerization model for B cell receptor microclustering and signaling. Curr Top Microbiol Immunol. (2010) 340:155–69. doi: 10.1007/978-3-642-03858-7_8
5. Yang J, Reth M. Oligomeric organization of the B-cell antigen receptor on resting cells. Nature. (2010) 467:465–9. doi: 10.1038/nature09357
6. Shen Z, Liu S, Li X, Wan Z, Mao Y, Chen C, Liu W. Conformational change within the extracellular domain of B cell receptor in B cell activation upon antigen binding. eLife. (2019) 8:e42271. doi: 10.7554/eLife.42271
7. Wan T, Beavil RL, Fabiane SM, Beavil AJ, Sohi MK, Keown M, et al. The crystal structure of IgE Fc reveals an asymmetrically bent conformation. Nat Immunol. (2002) 3:681–6. doi: 10.1038/ni811
8. Su Q, Chen M, Shi Y, Zhang X, Huang G, Huang B, et al. Cryo-EM structure of the human IgM B cell receptor. Science. (2022) 377:875–80. doi: 10.1126/science.abo3923
9. Dong Y, Pi X, Bartels-Burgahn F, Saltukoglu D, Liang Z, Yang J, et al. Structural principles of B cell antigen receptor assembly. Nature. (2022) 612:156–61. doi: 10.1038/s41586-022-05412-7
10. Ma X, Zhu Y, Dong D, Chen Y, Wang S, Yang D, et al. Cryo-EM structures of two human B cell receptor isotypes. Science. (2022) 377:880–5. doi: 10.1126/science.abo3828
11. Baba Y, Kurosaki T. Impact of Ca2+ signaling on B cell function. Trends Immunol. (2011) 32:589–94. doi: 10.1016/j.it.2011.09.004
12. Baba Y, Matsumoto M, Kurosaki T. Calcium signaling in B cells: Regulation of cytosolic Ca2+ increase and its sensor molecules, STIM1 and STIM2. Mol Immunol. (2014) 62:339–43. doi: 10.1016/j.molimm.2013.10.006
Keywords: B cell, B cell receptor (BCR), B cell activation, antibody, antigen, co-receptor, cytoskeleton
Citation: Song W, Kim TJ and Liu W (2025) Editorial: Community series in BCR signaling and B cell activation, volume 2. Front. Immunol. 16:1533646. doi: 10.3389/fimmu.2025.1533646
Received: 24 November 2024; Accepted: 02 January 2025;
Published: 15 January 2025.
Edited and Reviewed by:
Harry W Schroeder, University of Alabama at Birmingham, United StatesCopyright © 2025 Song, Kim and Liu. This is an open-access article distributed under the terms of the Creative Commons Attribution License (CC BY). The use, distribution or reproduction in other forums is permitted, provided the original author(s) and the copyright owner(s) are credited and that the original publication in this journal is cited, in accordance with accepted academic practice. No use, distribution or reproduction is permitted which does not comply with these terms.
*Correspondence: Wenxia Song, d2VueHNvbmdAdW1kLmVkdQ==; Tae Jin Kim, dGpraW1Ac2trdS5lZHU=; Wanli Liu, bGl1bGFiQHRzaW5naHVhLmVkdS5jbg==
Disclaimer: All claims expressed in this article are solely those of the authors and do not necessarily represent those of their affiliated organizations, or those of the publisher, the editors and the reviewers. Any product that may be evaluated in this article or claim that may be made by its manufacturer is not guaranteed or endorsed by the publisher.
Research integrity at Frontiers
Learn more about the work of our research integrity team to safeguard the quality of each article we publish.