- 1Sericultural & Agri-Food Research Institute, Guangdong Academy of Agricultural Sciences, Guangdong Key Laboratory of Agricultural Products Processing, Guangzhou, China
- 2Guangdong Provincial Engineering Technology Research Center of Special Aquatic Functional Feed, Foshan, China
- 3College of Food Science and Technology, Guangdong Ocean University, Zhanjiang, China
- 4Institute of Animal Health, Guangdong Academy of Agricultural Sciences, Guangzhou, China
This study investigated the effects of dietary supplementation of mulberry leaf oligosaccharides (MLO) on the growth performance, serum biochemistry, glucose and lipid metabolism, antioxidant activity, liver health, and virus resistance in largemouth bass (Micropterus salmoides). The fish were fed with CK (basal diet), MLOL (basal diet supplemented with 0.5%MLO), and MLOH (basal diet supplemented with 1.0% MLO) for 80 days, and then subjected to a 21-day viral challenge experiment. The results showed that MLO supplementation had no adverse effect on the weight gain rate, specific growth rate, feed intake, and condition factor (P > 0.05), but significantly decreased the feed conversion rate and viscerosomatic index (P< 0.05). Moreover, the MLOL and MLOH group had significantly lower contents of triglyceride, blood glucose, and malondialdehyde and activities of serum alanine aminotransferase and aspartate aminotransferase, while significantly higher levels of serum and liver total superoxide dismutase and lower levels of glutathione than the CK group (P< 0.05). MLO supplementation significantly up-regulated the relative expression of glycolytic genes gk and pfk and lipid catabolism genes ppar-α and cpt-1, while obviously down-regulated that of acc, fas, and dgat related to fatty acid synthesis in the liver tissue (P< 0.05). In terms of liver health, MLO supplementation significantly up-regulated the relative expression of anti-inflammatory cytokines il-10 and tgf-β, while decreased that of pro-inflammatory cytokines nf-κb, il-8, and tnf-α in the liver tissue (P< 0.05). The viral challenge test showed that MLO supplementation significantly improved the survival rate of M. salmoides after largemouth bass ranavirus (LMBV) infection. Dietary MLO supplementation promoted liver glucose and lipid metabolism, and improved the immunity and resistance of M. salmoides to LMBV by regulating the PPAR signaling way and inhibiting the NF-kB signaling pathway. The appropriate addition amount of MLO to the diet was determined to be 1.0%.
1 Introduction
Largemouth bass (Micropterus salmoides) is an important economic fish species in the world as well as one of the most important freshwater economic fish in China. According to statistics, the production of M. salmoides in China exceeded 700,000 tons in 2021 (1). Breeding of M. salmoides provides a large amount of high-quality animal protein and brings great economic benefits in China. However, with the expansion of breeding scale and continuous increase in breeding density, the breeding environment has been gradually deteriorated, and the problem of diseases has become increasingly prominent, causing huge economic losses and seriously restricting the sustainable development of the industry (2). In order to treat and control diseases, antibiotics and chemicals are frequently used as therapeutic agents in aquaculture, which can lead to drug residues and have adverse effects on the safety of the environment, humans, and animals (3–5). At present, China has banned the addition of antibiotics in feed, and “reducing resistance to replace resistance” has become a new trend. Therefore, development of environment-friendly and safe alternatives to antibiotics has become a hot research topic. Among them, functional additives with preventive effects such as prebiotics and plant extracts have attracted great research attention (6).
Prebiotics are organic substances that are not directly digested and absorbed by the host, but can selectively promote the growth or activity of a few beneficial bacteria in the colon, thereby improving the health of the host (7, 8). With continuous progress in research on the functions and mechanisms of prebiotics, the application of oligosaccharides in aquaculture animals is becoming increasingly popular (9, 10). Previous research has demonstrated that dietary oligosaccharides can trap pathogenic bacteria and prevent their access to gut mucosa, and improve the activities of digestive enzymes and modulation of gut microbiota, so as to improve the growth and gut health of the reared fish (11, 12). Besides, oligosaccharides can act as immunostimulants in various fish species and significantly change the disease resistance (13, 14).
Mulberry leaf, a component of traditional Chinese medicine rich in polysaccharides, is widely grown in China (15, 16). Mulberry leaf oligosaccharides (MLO) can be obtained through enzymatic hydrolysis of mulberry leaf polysaccharides (MLP) (17). In vitro experiments have shown that MLO can better promote the proliferation of Bifidobacterium and Lactobacillus than glucose or galacto-oligosaccharides. In addition, bacterial cultures inoculated with MLO have higher acetic acid and lactic acid concentrations (18). A recent study has revealed that dietary supplementation of MLO can effectively reduce blood glucose level in type 2 diabetic mice (19). However, there has been limited relevant information on aquatic species. For example, Ramulus mori oligosaccharides could increase the abundance of Fusobacterium and Cetobacterium in the intestine and improve liver morphology, thereby improving the antiviral ability of M. salmoides (20). However, it remains unclear whether MLO can improve the immune and antioxidant capacity and glucose/lipid utilization and metabolism of M. salmoides. Therefore, this study aims to explore the effects of MLO supplementation on the growth performance, antioxidant capacity, glucose and lipid metabolism, liver health, and antiviral ability of M. salmoides, which may help improve the health outcomes in aquaculture.
2 Materials and methods
2.1 Preparation of MLO
MLP was isolated and extracted using the method as described previously (17). Briefly, defatted mulberry leaf powder was extracted with water at 80°C for 4 h at a ratio of 1: 30 (w/v), evaporated and concentrated at 50°C using a rotary evaporator (EYELA N-1100, Tokyo Rikakikai Co. Ltd, Tokyo, Japan), precipitated with 4 volumes of absolute ethanol for 12 h, and then centrifuged at 10,000× g for 10 min. The precipitate was collected and dissolved. The polysaccharide solution (50 mg/mL) was then mixed with Sevage reagent (1-butanol/chloroform, v/v = 1:4) at a ratio of 4: 1 (v/v). The mixture was shaken thoroughly for 30 min and then centrifuged at 10,000× g for 5 min. The aqueous phase separated from the supernatant was added to a quarter of its volume of the Sevage reagent. This process was repeated until the solution presented no absorption peak at 250–280 nm on a UV spectrophotometer (UV-1800, Shimadzu, Kyoto, Japan). The deproteinized liquid was further freeze-dried to obtain MLP and the phenol-sulfuric acid method was used to determine the purity. For production of the enzymatic hydrolysate containing MLO, the MLP was incubated with 1500 U/mL β-dextranase (Shanghai Ryon Biologcial Technology Co., Ltd., Shanghai, China) at 51°C for 4 h. Then, the hydrolysate was placed in a boiling water bath for 10 min to terminate the reaction and then centrifuged at 4000 r/min for 10 min to remove β-dextranase (the enzymatic optimization is not shown here). The supernatant was collected and freeze-dried to obtain MLO.
2.2 Molecular weight determination
The molecular weight (Mw) of MLO was determined by gel permeation chromatography (GPC) in a highperformance liquid chromatography system (LC-2050, Shimadzu, Japan) coupled with RID-20A differential refractive index detector, using a PL aquagel-OH MIXED-M column (7.5 × 300 mm, 8 μm, Agilent, USA). The MLO powder was prepared as a 10 mg/mL aqueous solution and filtered through a 0.22 μm Millipore filter. An aliquot of 20 μL sample solution was injected and eluted at a flow rate of 1.0 mL/min and a column temperature of 35°C with 0.2 mol/L NaNO3 as the mobile phase. PEG of 1892 Da, 5121 Da, 10057 Da, 20552 Da, and 41531 Da were used as reference substances to calculate the Mw of MLO.
2.3 Diet preparation
All ingredients and proximate composition of the experimental diets are shown in Table 1. Fish meal, soybean meal, and peanut bran were the main protein sources; fish oil, soybean oil, and soybean phospholipid were the main fat sources; and wheat flour was the main carbohydrate source. Then, 0% (CK), 0.5% MLO (MLOL), and 1% MLO (MLOH) were supplemented to the basal feed to prepare three isonitrogenous and isolipidic diets. All ingredients were ground through a 250 μm mesh, mixed thoroughly, modulated by 102°C water vapor for 8 min, and then expanded at 95°C by a twin-screw extruder (TDSP120*2-120KW, China), cut into 3 mm (diameter), dried at 75°C and stored at –20°C until use.
2.4 Experimental design and feeding trial
Prior to the feeding trial, fish were fed with the basal diet for two weeks to allow acclimation to diet and conditions in an indoor circulating aquaculture system of the Research Institute of Sericulture and Agricultural Product Processing, Guangdong Academy of Agricultural Sciences (Guangzhou, China). After 24 h of fasting, a total of 450 M. salmoides (initial body weight 26.89 ± 1.16 g) were randomly distributed into nine tanks (350 L) with 50 fish in each tank and three tanks in each group. The experiment involved a completely randomized design. Fish were fed twice a day (09: 00 and 16: 30) to achieve an apparent state of satiety, over the 80 days of experiment. The water temperature was kept at 23.0–29.0°C, pH = 7.0–8.2, dissolved oxygen > 6.0 mg/L, ammonia ≤ 0.02 mg/L and nitrite ≤ 0.1 mg/L. Mortality was observed daily and the dead fish were weighed and recorded. The animal husbandry and handling protocols used in this study have been approved by the Animal Care and Use Committee, Guangdong Academy of Agricultural Sciences (GDAAS 258/2019).
2.5 Sampling
At the end of feeding trial, the fish were starved for 24 h before weighing. All fish were anesthetized with 40 mg/L tricaine methanesulfonate (MS-222, Sigma, USA) before sampling. Fish in each tank were counted and weighted to measure the final body weight, weight gain rate, specific growth rate, feed intake, feed conversion ratio, and condition factor. The blood of nine fish per tank was drawn from the caudal vein, kept at 25°C, centrifuged at 3,000 rpm for 15 min, and the supernatant was stored at –80°C for the analysis of serum biochemistry and antioxidant index. Afterwards, the fish were slaughtered and dissected using a sterile scalpel by cutting the belly on ice for determination of the viscerosomatic index and hepatosomatic index.
The livers of three fish per tank were collected and stored at –20°C for the analysis of antioxidant and immunity parameters. The livers of another three fish per tank were collected and immediately stored at –80°C for glucose and lipid metabolism, RNA extraction, and qPCR analysis.
2.6 Laboratory analyses
The nutrient composition and amino acids of the diets were analyzed using AOAC method (21) and determined by high-performance liquid chromatography (22), respectively.
The contents of blood glucose (GLU), low-density lipoprotein cholesterol (LDL-C), high-density lipoprotein cholesterol (HDL-C), total cholesterol (TC), triglycerides (TG), aspartate aminotransferase (AST), alanine aminotransferase (ALT), reduced glutathione (GSH), malondialdehyde (MDA), total antioxidant capacity (T-AOC), and total superoxide dismutase (T-SOD) in the serum or liver were determined with commercial kits (Nanjing Jiancheng Bioengineering Institute Nanjing, China).
The livers (0.5 cm × 0.5 cm × 0.5 cm) of three fish per tank were sampled, rinsed by 0.65% saline (4°C), fixed immediately in the 10% buffered formalin solution for 24 h. The fixed liver tissue was embedded in paraffin, and paraffin sections were made and stained with hematoxylin eosin (HE) using the standard histological techniques. The slices were scanned using a panoramic slicing digital scanner (PANNORAMIC-1000, 3DHISTECH), and the CaseViewer 2.2 (3DHISTECH) software was used to capture tissue photos of the slices (23).
Total RNA was extracted from each liver sample using Trizol reagent (TIANGEN, China) and the RNA quality was tested using Genios plus (Synergy LX, BioTek, USA). An aliquot of 1 mg total RNA was used for reverse transcription. The cDNA was synthesized by RevertAid Reverse Transcriptase (TIANGEN, China) using random primers. The RT-qPCR was performed according to standard protocols in a quantitative thermocycler (MiniOption TM, Bio-Rad, USA). The amplification volume was 10 μL, containing 0.3 μL of the respective primers, 1 μL of cDNA product, 5 μL of SYBR green color qPCR Master Mix (TIANGEN, China), and 3.4 μLof ddH2O. The specific primers used for il-8, il-10, nf-kb, tnf-α, tgf-β, gk, pfk, acc, cpt1, fas, dgat, and ppar-α genes have been reported previously (24–27). The details of the primers and the PCR amplification conditions used for RT-qPCR are shown in Table 2. After the reaction, melting curve analysis was conducted to confirm the presence of a single PCR product in the reaction. The gene expression levels were calculated by the 2-ΔΔCt method (28) with β-actin as the reference gene, and the experiment was performed in triplicate.
2.7 Challenge test
The largemouth bass ranavirus (LMBV) was isolated from diseased largemouth bass and stored in our laboratory (Guangdong Provincial Key Laboratory of Livestock Disease Prevention, Institute of Animal Health, Guangdong Academy of Agricultural Sciences). After 80 days of feeding, 20 fish were randomly selected from each group for challenge experiment. Each fish was injected with 100 μL 4 × 105 TCID50/mL of LMBV infected cell suspernatant through the intraperitoneal cavity. The virus dose was based on the results of our previous experiments (20). The mortality rate was recorded daily for 21 days. The relative percent survival (RPS) was calculated according to the following method.
2.8 Data calculation and statistical analysis
The data were analyzed using one-way ANOVA with Duncan’s method for multiple comparisons between groups. Orthogonal polynomial contrast was used to estimate the linear and quadratic effects of various amounts of MLO added. All data were analyzed using SPSS. The significance level of differences was set at P< 0.05 and the results were expressed as Mean ± SE. GraphPad Prism8.0 software was used to analyze the survival curves, and Log-rank (Mantel-Cox) was used for statistical difference analysis.
3 Results
3.1 Molecular weight of MLO
GPC analysis was conducted to determine the average molecular weight (Mw), number-average molecular weight (Mn), and polydispersity index (Mw/Mn) for the MLO. As shown in Figure 1, the GPC profile of MLO displayed a single symmetrical and sharp peak. The values of Mw, Mw/Mn, and intrinsic viscosity [η] were determined to be 2174 Da, 1.20, and 1.00 mL/g, respectively.
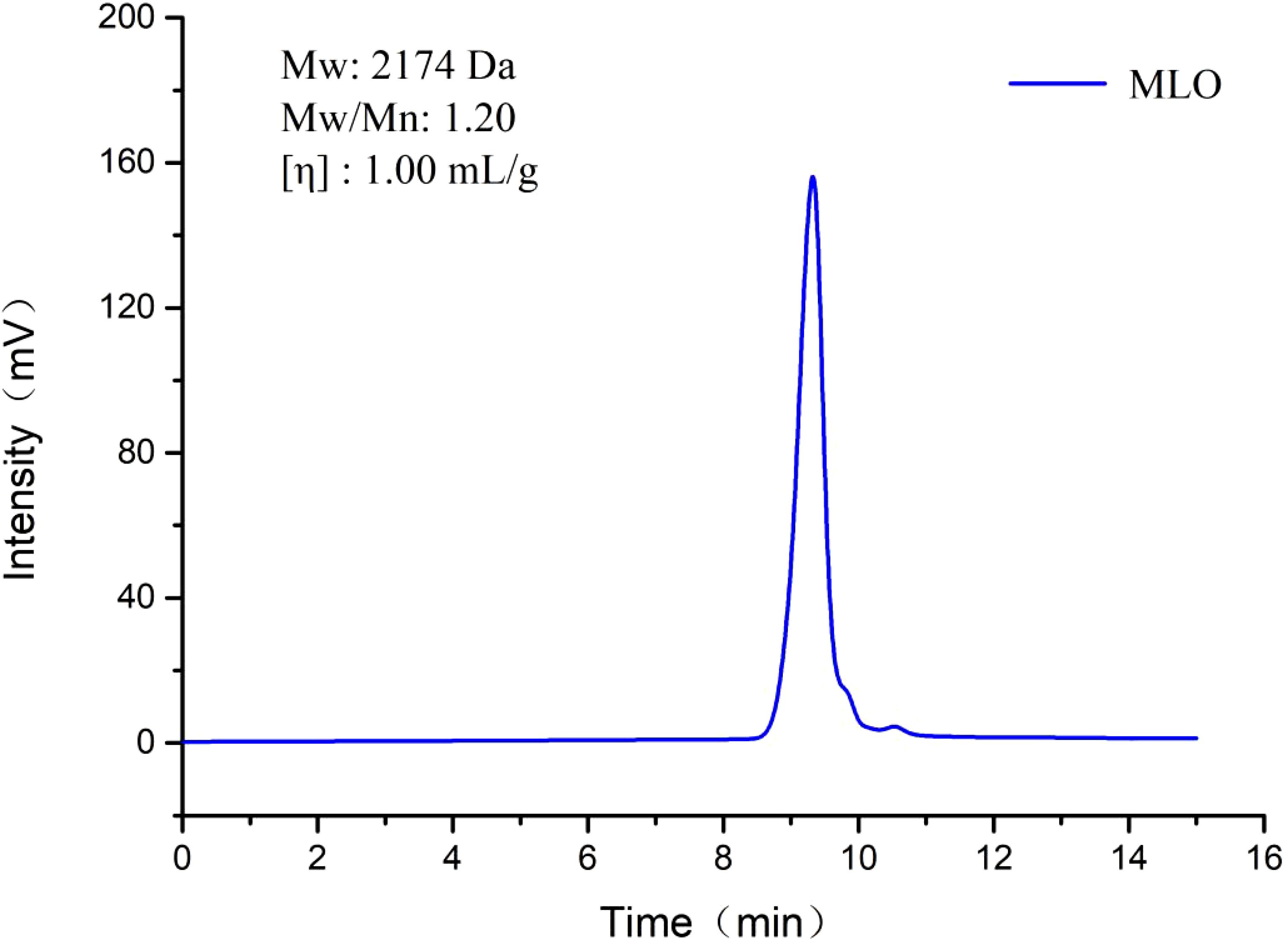
Figure 1. Molecular weight distribution diagram of MLO. Mw, average molecular weight; Mn, number-average molecular weight; Mw/Mn, polydispersity index; [η], intrinsic viscosity.
3.2 MLO supplementation has no adverse effect on the growth performance of largemouth bass
After the feeding experiments, no pathology or abnormal phenomenon was observed in all groups. The effects of dietary MLO level on FBW, WGR, SGR, FI, FCR, CF, HVI, and HSI are shown in Table 3. There was no significant difference in FBW, WGR, SGR, FI, and CF among all groups (P > 0.05). However, compared with the CK group, the MLOL and MLOH group showed significant decreases in FCR and HVI (P< 0.05). Moreover, the MLOH group had significantly lower HSI than the CK group (P< 0.05). With increasing level of MLO added to the diet, there were significant decreases in FCR (linear or quadratic, P< 0.05) and VSI and HSI (linear, P< 0.05).
3.3 MLO improves the serum biochemical parameters of largemouth bass
The biochemical parameters in the serum of M. salmoides after feeding with MLO diets for 80 days are presented in Table 4. The MLOL and MLOH group had significantly lower TG and GLU contents as well AST and ALT activities than the CK group (P< 0.05), but showed no significant change in the contents of TC, LDL-C, and HDL-C in the serum (P > 0.05). With increasing level of MLO added to the diet, there were significant decreases in the serum TG content and ALT activity (linear or quadratic, P< 0.05), GLU content (quadratic, P< 0.05), and ALT activity (linear, P< 0.05).
3.4 MLO modulates glucose and lipid metabolism in the liver
The liver plays an important role in regulating blood glucose homeostasis (29). The MLOL and MLOH groups showed significant increases in the expression of genes related to glycolysis (pfk and gk) and lipid catabolism (ppar-α and cpt1), while significant decreases in that of genes associated with fatty acid synthesis (acc, fas, and dgat) relative to the CK group (P< 0.05) (Figure 2).
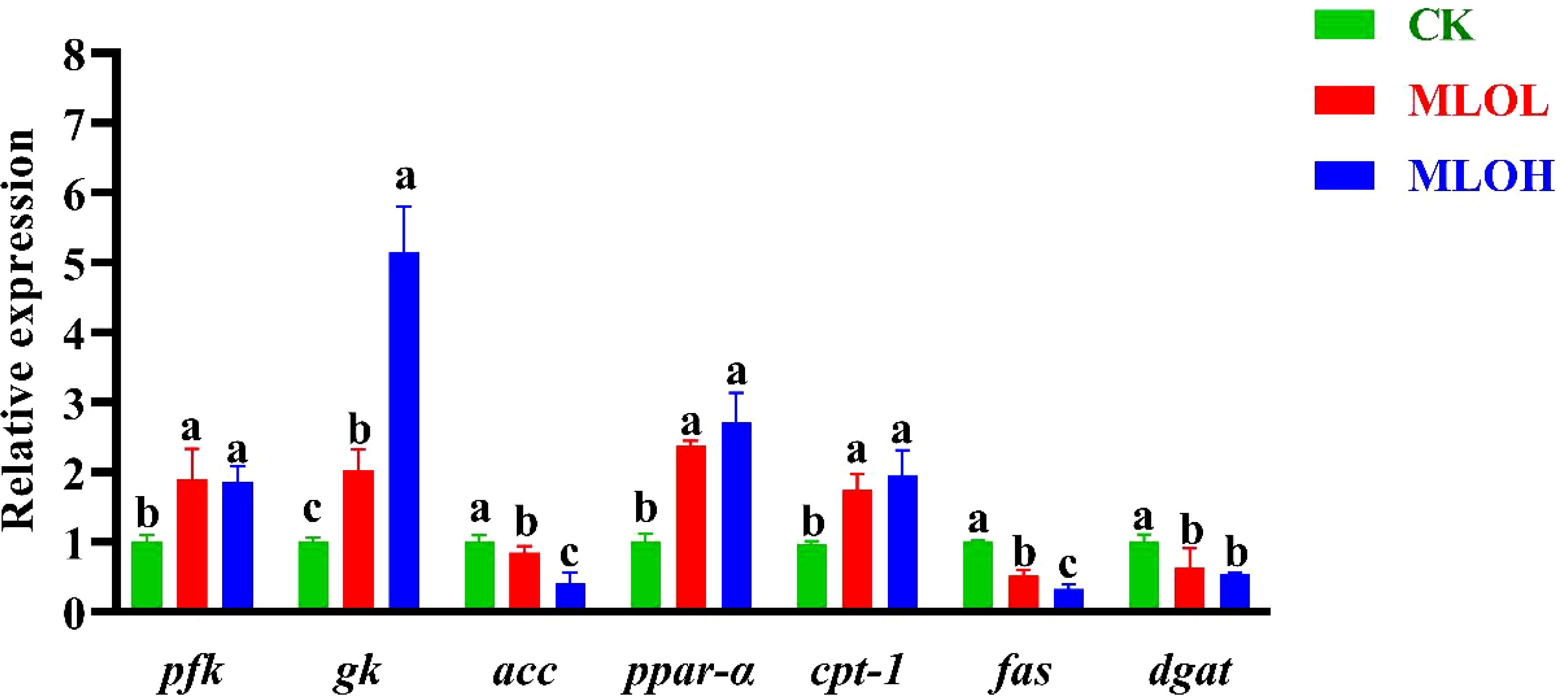
Figure 2. mRNA expression of pfk, gk, acc, ppar-α, cpt-1, fas and dgat in the liver of M. salmoides. CK, basal diet; MLOL, supplemented with 0.5% MLO; MLOH, supplemented with 1.0% MLO. Different letters above the bars denote significant differences among diets (P< 0.05).
3.5 Feeding of MLO enhances the antioxidant capacity of largemouth bass
In the serum, the MLOL and MLOH group had significantly higher activities of T-SOD and GSH (P< 0.05), while no significant difference in the activity of T-AOC (P > 0.05) compared with the CK group. MLO feeding significantly increased the activities of T-SOD, GSH, and T-AOC in the liver, but obviously reduced the MDA content in the serum and liver compared with the CK (P< 0.05) (Table 5). With increasing level of MLO added to the diet, there were significant increases in serum and liver T-SOD and GSH activities (linear or quadratic, P< 0.05), a significant decrease in serum MDA content (linear, P< 0.05), a significant reduction of liver MDA content (linear or quadratic, P< 0.05), and a significant increase in liver T-AOC capacity (linear, P< 0.05).
3.6 MLO supplementation inhibits inflammation in the liver
The expression levels of immune-related genes are shown in Figure 3. Compared with the CK, MLO supplementation significantly up-regulated the relative expression of anti-inflammatory cytokines il-10 and tgf-β, while down-regulated that of pro-inflammatory cytokines nf-κb, il-8, and tnf-α in the liver (P< 0.05) (Figure 3).
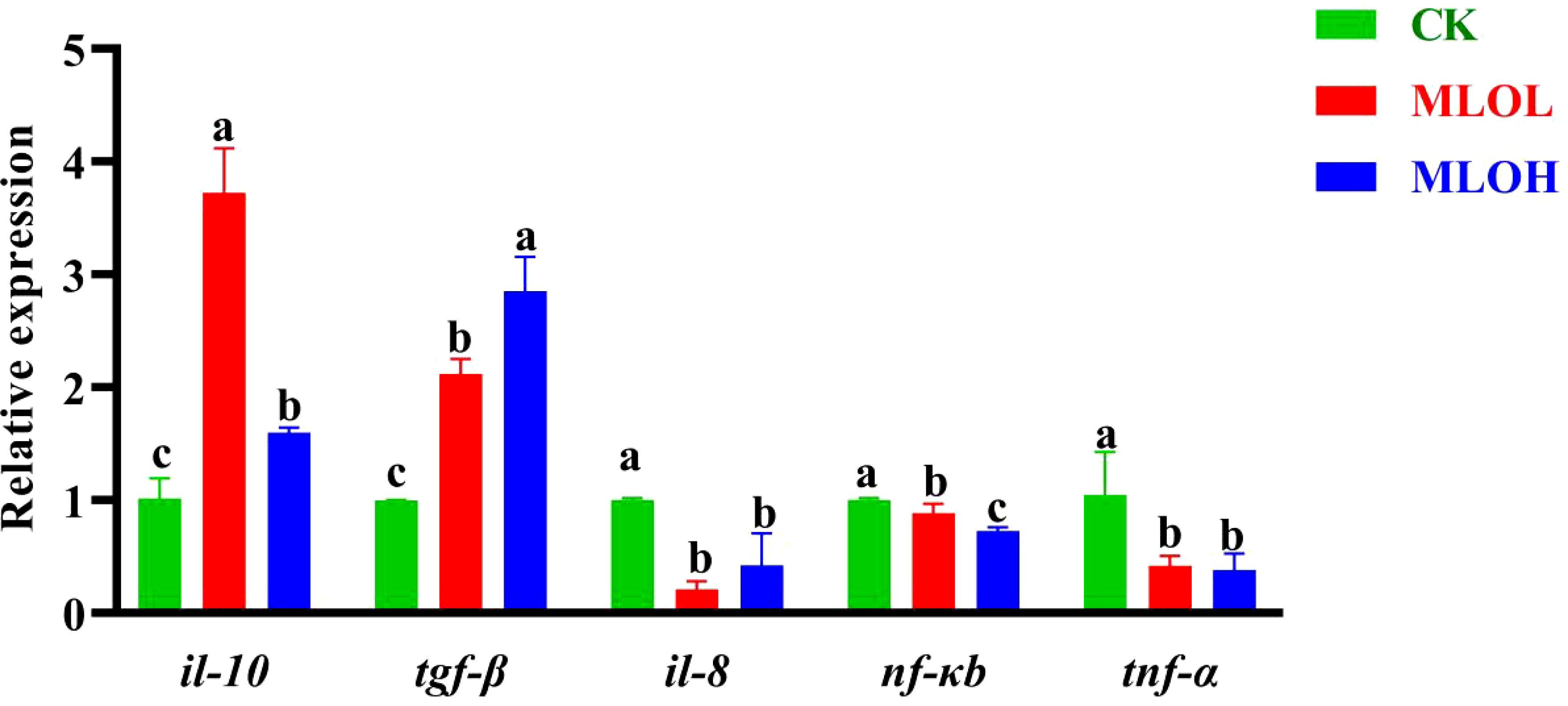
Figure 3. mRNA expression of il-10, tgf-β, il-8, nf-kb, and tnf-α in the liver of M. salmoides. CK, basal diet; MLOL, supplemented with 0.5%MLO; MLOH, supplemented with 1.0% MLO. Different letters above the bars denote significant differences among diets (P< 0.05).
3.7 MLO supplementation maintains the structural integrity of the liver
The liver histomorphology is shown in Figure 4. The liver cells of the CK group showed obvious vacuolation and nuclear displacement, while those of MLOL and MLOH group exhibited much less obvious cell vacuolization and nuclear displacement.
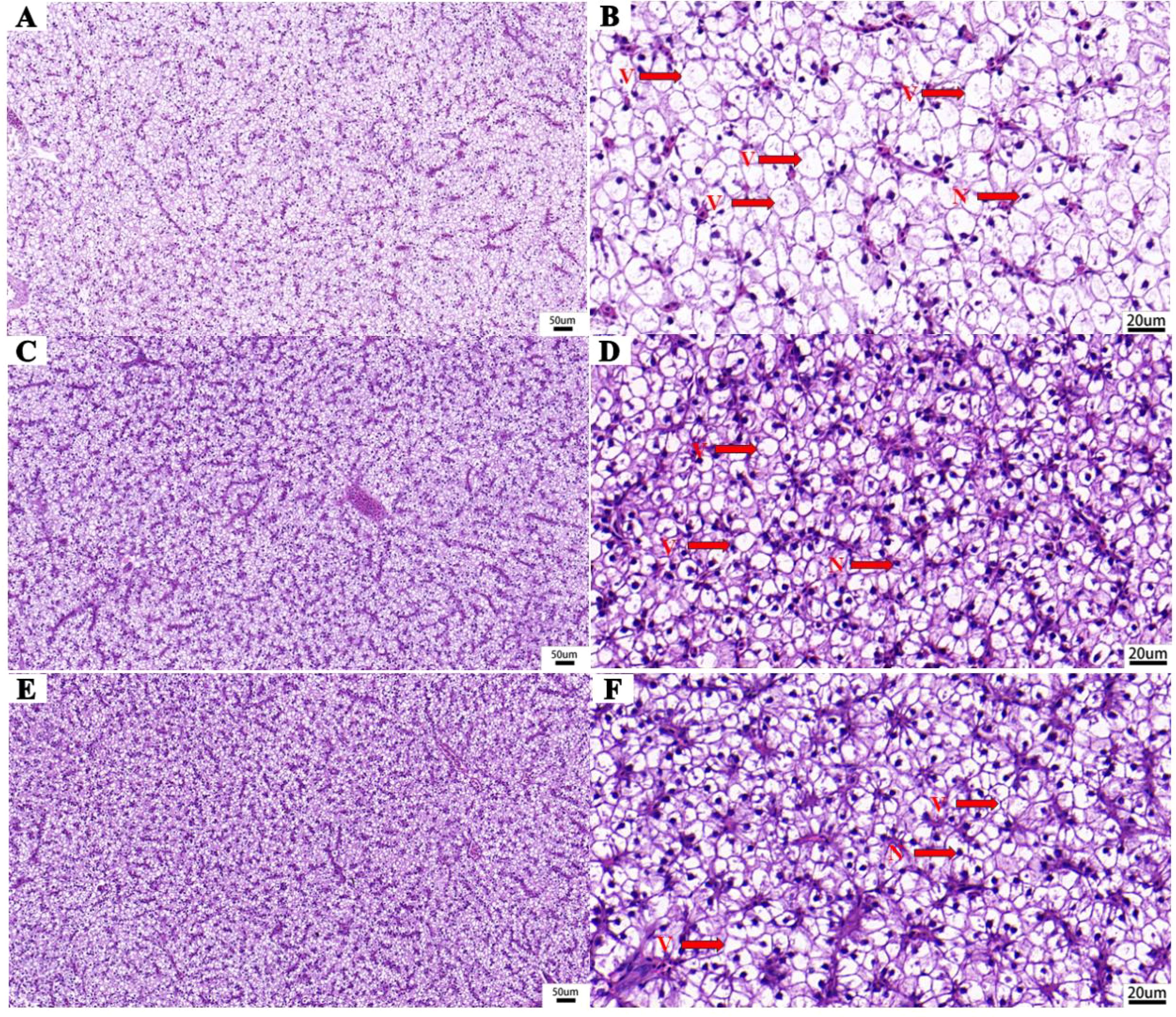
Figure 4. Liver histomorphology (H&E staining) of M. salmoides. (A, B) basal diet; (C, D) supplemented with 0.5% MLO; (E, F) supplemented with 1.0% MLO. V, cytoplasmic vacuolation; N, nucleus.
3.8 MLO supplementation improves the resistance of largemouth bass to LMBV infection
As shown in Figure 5, the cumulative survival rate was calculated after 21 days of LMBV infection. Compared with the CK group, the MLO groups showed significantly delayed death time of largemouth bass. There was no significant difference in the number of deaths in each group after 14 days of infection. Among different groups, the final survival rates of the CK, MLOL, and MLOH group were 25%, 60%, and 65%, respectively, indicating that MLO supplementation can significantly improve the resistance of largemouth bass to LMBV infection and enhance the survival rate (P< 0.05). Compared with that of the CK, the relative protection rate of MLOL and MLOH was 46.67% and 53.33%, respectively (Figure 5).
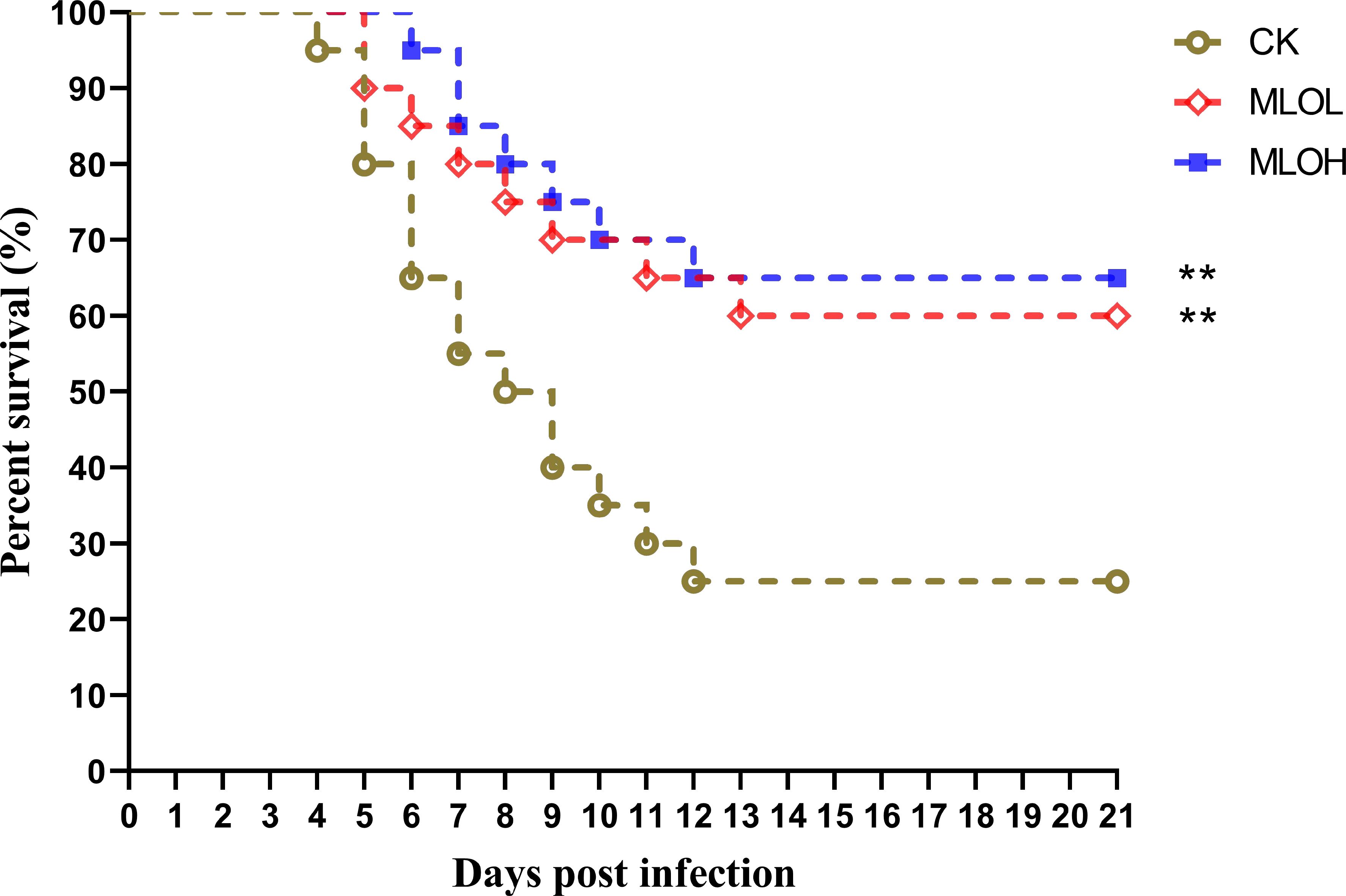
Figure 5. Survival rate curve analysis of M. salmoides after infection with LMBV. CK, basal diet; MLOL, supplemented with 0.5% MLO; MLOH, supplemented with 1.0% MLO (n = 25).
4 Discussion
4.1 Growth performance
To date, there have been numerous studies of the application of oligosaccharides (prebiotics) such as fructooligosaccharides, galactooligosaccharides, and isomaltooligosaccharides in aquaculture, but there has been no report about the application of MLO in aquatic animals. In the present study, diets supplemented with 0.5% and 1% MLO were found to significantly decrease the FCR and HIS, but showed no significant effect on WGR, which may be directly related to the lower feed intake of the treatment groups. Some prebiotics have also been found to have no significant effect on the growth of aquatic animals, such as short-chain fuctooligosaccharides for hybrid tilapia (Oreochromis niloticus×O. aureus) (14) and chito-oligosaccharide (COS) for the same species (30). Previous studies have demonstrated that oligosaccharides can significantly increase the WGR and SGR while decrease the FCR of Oreochromis niloticus (31), hybrid catfish (Pangasianodon gigas × Pangasianodon hypophthalmus) (32) and Caspian trout (Salmo trutta caspius) (33). Besides, another study has revealed that Grobiotic-A has an adverse effect on the growth of M. salmoides (34). These results indicate that prebiotics have different effects on the growth performance of different aquatic animals, which may be related to the type and dosage of the prebiotics used.
4.2 Glucose and lipid metabolism in the liver
Compared with herbivorous and omnivorous species, carnivorous fish have a poor ability to utilize starch as an energy source. Generally, digestible carbohydrate at levels of ≤15–25% is appropriate for marine and carnivorous fish, but the suitable carbohydrate level appears to be lower for M. salmoides (35, 36). Mulberry leaf is a Chinese medicinal component widely used to regulate blood GLU (37). Flavonoids are one of the main classes of active ingredients in mulberry leaves, which contribute to the potential to treat type II diabetes and maintain the glucose metabolism balance of Monopterus albus under high glucose stress (38, 39). It has been demonstrated that M. salmoides can strengthen the glycolysis pathway to cope with the rising of blood GLU level so as to maintain GLU metabolism homeostasis (35, 40). Another study has also revealed that mannan oligosaccharides (MOS) can decrease serum glucose and liver glycogen by increasing the activities of enzymes related to glycolysis in O. niloticus (41). In this study, feeding of MLO diets significantly decreased the serum GLU content in M. salmoides. The liver plays an important role in regulating blood GLU homeostasis (29), and this regulation involves several important pathways, such as gluconeogenesis and glycolysis, where PFK, PK, and GK are limiting enzymes in the glycolysis pathway (40). This study showed that M. salmoides fed with MLO diets had significantly higher mRNA expression of pfk and gk, which is similar to the result reported for M. salmoides (42), indicating that MLO has great potential to lower GLU.
CPT1 and ACC are key enzymes involved in lipid catabolism and fatty acid synthesis, respectively (43). PPAR-α is a transcription factor that mediates the oxidative breakdown of liver fatty acids, upregulates the expression of fatty acid transport- and oxidation-related gene cpt-1, and promotes the transport of long-chain fatty acids in mitochondrial β-oxidation, thereby improving fatty acid oxidation in mitochondria and peroxisomes (44). It has been reported that MOS can significantly reduce the TG level of O. niloticus and M. salmoides fed with high carbohydrate diets (41, 45). In this study, MLO diet up-regulated the expression of cpt-1 and ppar-α, down-regulated that of fatty acid synthesis-related gene acc, and significantly reduced the content of TG in the serum, indicating that MLO may accelerate lipid metabolism by regulating the expression of liver metabolism-related genes, thereby reducing lipid deposition in the liver.
4.3 Antioxidant and immune responses
Oxidative stress and inflammatory response are interrelated processes with significant contribution to the body’s response to various stresses. There were decreases in serum GLU, AST, and ALT after dietary MLO supplementation in this study, suggesting that dietary MLO has obvious positive effects on the immune function and liver health of M. salmoides, since high concentrations of serum GLU, AST, and ALT are generally related to liver damage or necrosis (46, 47). It is generally believed that hepatocyte is highly sensitive to the nutritional status of fish and the quality of diet. In this study, dietary MLO significantly alleviated liver vacuolization and nuclear displacement, suggesting that it can improve the liver health of M. salmoides. The concentration of MDA can directly reflect the level of lipid peroxidation and the degree of endogenous oxidative damage. GSH and T-SOD are both antioxidant enzymes that assist the maintenance of a healthy cellular antioxidant state. In particular, GSH, as a substrate for glutathione peroxidase and glutathione transferase, reacts with intracellular free radicals and peroxides under the catalysis of both enzymes to maintain the normal physiological functions of cells, making it a critical enzyme for protecting cells from oxidative damage (48). T-SOD is a necessary antioxidant enzyme in all oxygen-breathing organisms, playing important roles in converting superoxide into hydrogen peroxide and removing excess active oxygen (49). Oligosaccharides have been demonstrated to have antioxidant activities in O. niloticus (50). Similarly, in the present study, the increase in MLO supplementation enhanced the GSH and T-SOD activities in the serum and liver while reduced the MDA content in M. salmoides. Therefore, MLO can enhance the antioxidant capacity of M. salmoides.
The inflammatory responses in animals are regulated by the NF-κB/TLRs signaling pathway (49). After activation by pro-inflammatory cytokines (such as il-8, tnf-α, and nf-κb), this signaling pathway can promote cellular immune response and up-regulate the expression of anti-inflammatory cytokines (such as il-10 and tgf-β). This study showed that MLO supplementation remarkably up-regulated the transcription of il-10 and tgf-β in the liver, whereas down-regulated that of il-8, tnf-α, and nf-κb. These results indicate that MLO supplementation can reduce inflammatory response by inhibiting the NF-κB signaling pathway.
4.4 Disease resistance
With the expansion of breeding scale, increase in breeding density, and deterioration of water environment, the problem of diseases is becoming increasingly prominent for M. salmoides. M. salmoides suffers from high mortality rates caused by viral diseases, particularly iridescent virus diseases, which poses serious threats to the aquaculture industry worldwide. M. salmoides infected with iridovirus generally exhibits liver and intestinal necrosis and inflammatory lesions, increased cell apoptosis, and decreased immunity (51, 52).
In the present study, MLO supplementation significantly delayed the death time of M. salmoides infected with LMBV compared with the CK. In addition, the cumulative mortality rate significantly decreased with increasing dietary MLO, indicating that MLO can reduce the LMBV-induced mortality rate. This may be due to the combined effect of MLO induced up-regulation of anti-inflammatory cytokines and down-regulation of pro-inflammatory cytokines, inhibition of NF-κB signaling pathway, and enhancement of the antioxidant capacity (T-SOD and GSH) in the serum and liver. Some studies have also shown that oligosaccharides can stimulate fish tissue, induce the expression of iNOS and produce NO, thereby killing pathogens and enhancing the innate immune response of the body (53). However, further research is still needed to clarify the mechanisms through which these oligosaccharides improve the health and immunity of M. salmoides.
5 Conclusion
In summary, MLO supplementation can significantly reduce the feed conversion rate, enhance the antioxidant capacity and liver glucose and lipid metabolism, improve the immunity by inhibiting the NF-kB signaling pathway, and increase the survival rate of M. salmoides infected with LMBV. This study provides a practical strategy to apply MLO in the diet for improving the immune and antioxidant abilities of M. salmoides.
Data availability statement
The original contributions presented in the study are included in the article, further inquiries can be directed to the corresponding authors.
Ethics statement
The animal study was approved by Collaborative Innovation Center of Aquatic Sciences, Guangdong Academy of Agricultural Sciences. The study was conducted in accordance with the local legislation and institutional requirements.
Author contributions
DZ: Conceptualization, Funding acquisition, Investigation, Methodology, Project administration, Writing – original draft, Writing – review & editing. WZ: Writing – original draft, Data curation, Formal analysis, Investigation, Methodology. BF: Methodology, Writing – original draft, Formal analysis. EL: Methodology, Writing – original draft. LH: Formal analysis, Investigation, Methodology, Writing – original draft. QL: Methodology, Writing – original draft. QY: Writing – review & editing, Methodology, Supervision. YZ: Writing – review & editing, Supervision, Validation. ZL: Methodology, Writing – original draft. FW: Investigation, Writing – review & editing, Methodology. SL: Conceptualization, Writing – review & editing, Supervision, Validation. DX: Conceptualization, Writing – review & editing, Project administration, Supervision, Validation.
Funding
The author(s) declare financial support was received for the research, authorship, and/or publication of this article. This research was funded by the Collaborative Innovation Center of Guangdong Academy of Agricultural Sciences (XT202304); the Basic and Applied Basic Research Foundation of Guangzhou City (202201010474); the Key Science and Technology Research Projects in Foshan City (2220001018588), and the High-level Guangdong Province Agricultural Science and Technology Demonstration City Construction Fund Project in 2023 (2320060002429); the Innovation Foundation of Guangdong Academy of Agricultural Sciences (202113); the Special Funds for 2021 Rural Revitalization Strategy (Jiangke [2021] No. 183).
Conflict of interest
The authors declare that the research was conducted in the absence of any commercial or financial relationships that could be construed as a potential conflict of interest.
Generative AI statement
The author(s) declare that no Generative AI was used in the creation of this manuscript.
Publisher’s note
All claims expressed in this article are solely those of the authors and do not necessarily represent those of their affiliated organizations, or those of the publisher, the editors and the reviewers. Any product that may be evaluated in this article, or claim that may be made by its manufacturer, is not guaranteed or endorsed by the publisher.
References
1. Zou J, Hu P, Wang M, Chen Z, Wang H, Guo X, et al. Liver injury and metabolic dysregulation in Largemouth bass (Micropterus salmoides) after ammonia exposure. Metabolites. (2023) 13:274. doi: 10.3390/metabo13020274
2. Parsaian M, Shokri M, Pazooki J. The response of benthic foraminifera to aquaculture and industrial pollution: a case study from the Northern Persian Gulf. Mar pollut Bull. (2018) 35:682–93. doi: 10.1016/j.marpolbul.2018.07.073
3. Rico R, Van den Brink P. Probabilistic risk assessment of veterinary medicines applied to four major aquaculture species produced in Asia. Sci Total Environ. (2014) 468:630–41. doi: 10.1016/j.scitotenv.2013.08.063
4. Mehdi Y, Létourneau-Montminy M, Gaucher M, Chorfi Y, Suresh G, Rouissi T, et al. Use of antibiotics in broiler production: global impacts and alternatives. Anim Nutr. (2018) 4:170–78. doi: 10.1016/j.aninu.2018.03.002
5. Chu L, Su D, Wang H, Aili D, Yimingniyazi B, Jiang Q, et al. Association between antibiotic exposure and type 2 diabetes mellitus in middle-aged and older adults. Nutrients. (2023) 15:1290. doi: 10.3390/nu15051290
6. Patel S, Goyal A. The current trends and future perspectives of prebiotics research: a review. 3 Biotech. (2012) 2:115–25. doi: 10.1007/s13205-012-0044-x
7. Gibson G, Probert H, Loo J, Rastall R, Roberfroid M. Dietary modulation of the human colonic microbiota: updating the concept of prebiotics. Nutr Res Rev. (2004) 17:259–75. doi: 10.1079/NRR200479
8. Ringo E, Olsen R, Gifstad T, Dalmo R, Amlund H, Hemre G, et al. Prebiotics in aquaculture: a review. Aquacult Nutr. (2010) 16:117–36. doi: 10.1111/j.1365-2095.2009.00731.x
9. El-Saadony M, Alagawany M, Patra A, Kar I, Tiwari R, Dawood M, et al. The functionality of probiotics in aquaculture: an overview. Fish Shellfish Immun. (2021) 117:36–52. doi: 10.1016/j.fsi.2021.07.007
10. Wee W, Hamid N, Mat K, Khalif R, Rusli N, Rahman M, et al. The effects of mixed prebiotics in aquaculture: a review. Aquacult Fish. (2024) 9:28–34. doi: 10.1016/j.aaf.2022.02.005
11. Akrami R, Nasri-Tajan M, Jahedi A, Jahedi M, Razeghi Mansour M, Jafarpour S. Effects of dietary synbiotic on growth, survival, lactobacillus bacterial count, blood indices and immunity of beluga (Huso huso Linnaeus, 1754) juvenile. Aquacult Nutr. (2015) 21:952–59. doi: 10.1111/anu.12219
12. Akter M, Sutriana A. Dietary supplementation with mannan oligosaccharide influences growth, digestive enzymes, gut morphology, and microbiota in juvenile striped catfish, Pangasianodon hypophthalmus. Aquacult Int. (2016) 24:127–44. doi: 10.1007/s10499-015-9913-8
13. Hoseinifar S, Soleimani N, Ringø E. Effects of dietary fructo-oligosaccharide supplementation on the growth performance, haemato-immunological parameters, gut microbiota and stress resistance of common carp (Cyprinus carpio ) fry. Brit J Nutr. (2014) 112:1296–2. doi: 10.1017/S0007114514002037
14. Liu W, Wang W, Ran C, He S, Yang Y, Zhou Z. Effects of dietary scFOS and lactobacilli on survival, growth, and disease resistance of hybrid tilapia. Aquaculture. (2017) 470:50–5. doi: 10.1016/j.aquaculture.2016.12.013
15. Wang L, Gao H, Sun C, Huang L. Protective application of Morus and its extracts in animal production. Animals. (2022) 12:3541. doi: 10.3390/ani12243541
16. Sánchez MD. World distribution and utilization of mulberry, potential for animal feeding. FAO Anim Prod Health Pap. (2000) 111:1–11.
17. Hu T, Zou Y, Li E, Liao S, Wu H, Wen P. Effects of enzymatic hydrolysis on the structural, rheological, and functional properties of mulberry leaf polysaccharide. Food Chem. (2021) 355:129608. doi: 10.1016/j.foodchem.2021.129608
18. Hu T, Wu H, Yu Y, Xu Y, Li E, Liao S, et al. Preparation, structural characterization and prebiotic potential of mulberry leaf oligosaccharides. Food Funct. (2022) 13:5287–98. doi: 10.1039/D1FO04048K
19. Hu T, Yu Y, Wu J, Xu Y, Xiao G, An K, et al. Structural elucidation of mulberry leaf oligosaccharide and its selective promotion of gut microbiota to alleviate type 2 diabetes mellitus. Food Sci Hum Well. (2024) 13:2161–73. doi: 10.26599/FSHW.2022.9250180
20. Li S, Zhou D, Pang D, Li Q, Li Q, Wang H, et al. Effects of Ramulus mori oligosaccharides on growth performance, serum physiological and biochemical parameters, and immunomodulation in largemouth bass (Micropterus salmoides). Aquaculture. (2024) 589:741008. doi: 10.1016/j.aquaculture.2024.741008
21. AOAC. Official methods of analysis of the association of official agricultural chemists. 16th ed. Gaithersburg: AOAC international (1999). 5th rev.
22. Araújo F, Michelato M, Schemberger M, Salaro A, Vidal L, Da Cruz T, et al. Assessment of isoleucine requirement of fast-growing Nile tilapia fingerlings based on growth performance, amino acid retention, and expression of muscle growth-related and mTOR genes. Aquaculture. (2021) 539:736645. doi: 10.1016/j.aquaculture.2021.736645
23. Su C, Chang C, Chou C, Wu Y, Yang K, Tseng J, et al. L-carnitine ameliorates dyslipidemic and hepatic disorders induced by a high-fat diet via regulating lipid metabolism, self-antioxidant capacity, and inflammatory response. J Funct Foods. (2015) 15:497–8. doi: 10.1016/j.jff.2015.04.007
24. Wei Y, Huang J, Sun H, Feng Z, He Y, Chen Y, et al. Impact of different processing mulberry leaf on growth, metabolism and liver immune function of largemouth bass (Micropterus salmoides). Aquacult Rep. (2023) 29:101508. doi: 10.1016/j.aqrep.2023.101508
25. Xv Z, He G, Wang X, Shun H, Chen Y, Lin S. Mulberry leaf powder ameliorate high starch-induced hepatic oxidative stress and inflammation in fish model. Anim Feed Sci Tech. (2021) 278:115012. doi: 10.1016/j.anifeedsci.2021.115012
26. Che M, Lu Z, Liu L, Li N, Ren L, Chi S. Dietary lysophospholipids improves growth performance and hepatic lipid metabolism of largemouth bass (Micropterus salmoides). Anim Nutr. (2023) 13:426–34. doi: 10.1016/j.aninu.2023.04.007
27. Chen W, Gao S, Chang K, Zhao X, Niu B. Dietary sodium butyrate supplementation improves fish growth, intestinal microbiota composition, and liver health in largemouth bass (Micropterus salmoides) fed high-fat diets. Aquaculture. (2023) 564:739040. doi: 10.1016/j.aquaculture.2022.739040
28. Livak K, Schmittgen T. Analysis of relative gene expression data using real-time quantitative PCR and the 2–ΔΔCt method. Methods. (2001) 25:402–8. doi: 10.1006/meth.2001.1262
29. Rito J, Viegas I, Pardal M, Jones J. Evidence of extensive plasma glucose recycling following a glucose load in seabass. Comp Biochem Phys A. (2017) 211:41–8. doi: 10.1016/j.cbpa.2017.05.009
30. Qin C, Zhang Y, Liu W, Xu L, Yang Y, Zhou Z. Effects of chito-oligosaccharides supplementation on growth performance, intestinal cytokine expression, autochthonous gut bacteria and disease resistance in hybrid tilapia Oreochromis niloticus ♀ × Oreochromis aureus ♂. Fish Shellfish Immun. (2014) 40:267–74. doi: 10.1016/j.fsi.2014.07.010
31. Selim K, Reda R. Beta-glucans and mannan oligosaccharides enhance growth and immunity in Nile tilapia. N Am J Aquacult. (2015) 77:22–30. doi: 10.1080/15222055.2014.951812
32. Hahor W, Thongprajukaew K, Suanyuk N. Effects of dietary supplementation of oligosaccharides on growth performance, gut health and immune response of hybrid catfish (Pangasianodon gigas × Pangasianodon hypophthalmus). Aquaculture. (2019) 507:97–7. doi: 10.1016/j.aquaculture.2019.04.010
33. Jami M, Kenari A, Paknejad H, Mohseni M. Effects of dietary b-glucan, mannan oligosaccharide, Lactobacillus plantarum and their combinations on growth performance, immunity and immune related gene expression of Caspian trout, Salmo trutta caspius (Kessler, 1877). Fish Shellfish Immun. (2019) 91:202–8. doi: 10.1016/j.fsi.2019.05.024
34. Rossi W, Allen K, Habte-Tsion H, Meesala K. Supplementation of glycine, prebiotic, and nucleotides in soybean meal-based diets for largemouth bass (Micropterus salmoides): effects on production performance, whole-body nutrient composition and retention, and intestinal histopathology. Aquaculture. (2021) 532:736031. doi: 10.1016/j.aquaculture.2020.736031
35. Ma H, Mou M, Pu D, Lin S, Chen Y, Luo L. Effect of dietary starch level on growth, metabolism enzyme and oxidative status of juvenile largemouth bass, Micropterus salmoides. Aquaculture. (2019) 498:482–87. doi: 10.1016/j.aquaculture.2018.07.039
36. Li S, Wang A, Wei Z, Liu R, Wang D, Chen N. Insulin receptors in largemouth bass (Micropterus salmoides): Cloning, characterization, tissue expression profile and transcriptional response to glucose tolerance test. Aquac Res. (2021) 52:625–34. doi: 10.1111/are.14919
37. Hassan F, Arshad M, Li M, Rehman M, Loor J, Huang J. Potential of mulberry leaf biomass and its flavonoids to improve production and health inruminants:mechanistic insights and prospects. Animals. (2020) 10:2076. doi: 10.3390/ani10112076
38. Duan Y, Dai H, An Y, Cheng L, Shi L, Lv Y, et al. Mulberry leaf flavonoids inhibit liver inflammation in type 2 diabetes rats by regulating TLR4/MyD88/NF-κB signaling pathway. Evidence-Based Complementary Altern Med. (2022) 2022:3354062. doi: 10.1155/2022/3354062
39. Shi Y, Zhong L, Fan Y, Zhang J, Zhong H, Liu X, et al. The protective effect of mulberry leaf flavonoids on high-carbohydrate-induced liver oxidative stress, inflammatory response and intestinal microbiota disturbance in Monopterus albus. Antioxidants. (2022) 11:976. doi: 10.3390/antiox11050976
40. Chen S, Jiang X, Liu N, Ren M, Wang Z, Li M, et al. Effects of dietary berberine hydrochloride inclusion on growth, antioxidant capacity, glucose metabolism and intestinal microbiome of largemouth bass (Micropterus salmoides). Aquaculture. (2022) 552:738023. doi: 10.1016/j.aquaculture.2022.738023
41. Wang T, Wu H, Li W, Xu R, Qiao F, Du Z, et al. Effects of dietary mannan oligosaccharides (MOS) supplementation on metabolism, inflammatory response and gut microbiota of juvenile Nile tilapia (Oreochromis niloticus) fed with high carbohydrate diet. Fish Shellfish Immun. (2022) 130:550–59. doi: 10.1016/j.fsi.2022.09.052
42. Li S, Sang C, Turchini G, Wang A, Zhang J, Chen N. Starch in aquafeeds: the benefits of a high amylose to amylopectin ratio and resistant starch content in diets for the carnivorous fish, largemouth bass (Micropterus salmoides). Brit J Nutr. (2020) 124:1145–55. doi: 10.1017/S0007114520002214
43. Wu X, Huang T. Recent development in acetyl-CoA carboxylase inhibitors and their potential as novel drugs. Future Med Chem. (2020) 12:533–61. doi: 10.4155/fmc-2019-0312
44. Schlaepfer I, Joshi M. CPT1A-mediated fat oxidation, mechanisms, and therapeutic potential. Endocrinology. (2020) 161:bqz046. doi: 10.1210/endocr/bqz046
45. Wang T, Xu R, Qiao F, Du Z, Zhang M. Effects of mannan oligosaccharides (MOS) on glucose and lipid metabolism of largemouth bass (Micropterus salmoides) fed with high carbohydrate diet. Anim Feed Sci Tech. (2022) 292:115449. doi: 10.1016/j.anifeedsci.2022.115449
46. Wang L, Liu W, Lu K, Xu W, Cai D, Zhang C, et al. Effects of dietary carbohydrate/lipid ratios on non-specific immune responses, oxidative status and liver histology of juvenile yellow catfish pelteobagrus fulvidraco. Aquaculture. (2014) 426:41–8. doi: 10.1016/j.aquaculture.2014.01.022
47. Peng K, Zhou Y, Wang Y, Wang G, Huang Y, Cao J. Inclusion of condensed tannins in Lateolabrax japonicus diets: effects on growth, nutrient digestibility, antioxidant and immune capacity and copper sulphate stress resistance. Aquacult Rep. (2020) 18:100525. doi: 10.1016/j.aqrep.2020.100525
48. Liu Y, Li Y, Xiao Y, Peng Y, He J, Chen C, et al. Mulberry leaf powder regulates antioxidative capacity and lipid metabolism in finishing pigs. Anim Nutr. (2021) 7:421–29. doi: 10.1016/j.aninu.2020.08.005
49. Meng Q, Chen J, Xu C, Huang Y, Wang Y, Wang T, et al. The characterization, expression and activity analysis of superoxide dismutases (SODs) from Procambarus clarkii. Aquaculture. (2013) 406:131–40. doi: 10.1016/j.aquaculture.2013.05.008
50. El-Nobi G, Hassanin M, Khalil A, Mohammed A, Amer S, Montaser M, et al. Synbiotic effects of saccharomycescerevisiae, mannan oligosaccharides, and β-glucan on innate immunity, antioxidant status, and disease resistance of Nile tilapia, Oreochromis niloticus. Antibiotics. (2021) 10:567. doi: 10.3390/antibiotics10050567
51. Zhao L, Zhong Y, Luo M, Zheng G, Huang J, Wang G, et al. Largemouth bass ranavirus: current status and research progression. Aquacult Rep. (2023) 32:101706. doi: 10.1016/j.aqrep.2023.101706
52. Xu W, Zhang Z, Lai F, Yang J, Qin Q, Huang Y, et al. Transcriptome analysis reveals the host immune response upon LMBV infection in largemouth bass (Micropterus salmoides). Fish Shellfish Immun. (2023) 137:108753. doi: 10.1016/j.fsi.2023.108753
53. Lin S, Jiang Y, Chen Y, Luo L, Doolgindachbaporn S, Yuangsoi B. Effects of astragalus polysaccharides (APS) and chitooligosaccharides (COS) on growth, immune response and disease resistance of juvenile largemouth bass, Micropterus salmoides. Fish Shellfish Immun. (2017) 70:40–7. doi: 10.1016/j.fsi.2017.08.035
Keywords: largemouth bass, growth, serum biochemistry, liver metabolism, inflammation
Citation: Zhou D, Zhong W, Fu B, Li E, Hao L, Li Q, Yang Q, Zou Y, Liu Z, Wang F, Liao S and Xing D (2025) Dietary supplementation of mulberry leaf oligosaccharides improves the growth, glucose and lipid metabolism, immunity, and virus resistance in largemouth bass (Micropterus salmoides). Front. Immunol. 16:1525992. doi: 10.3389/fimmu.2025.1525992
Received: 11 November 2024; Accepted: 09 January 2025;
Published: 28 January 2025.
Edited by:
Samad Rahimnejad, University of Murcia, SpainReviewed by:
Omid Safari, Ferdowsi University of Mashhad, IranHamed Ghafarifarsani, Urmia University, Iran
Copyright © 2025 Zhou, Zhong, Fu, Li, Hao, Li, Yang, Zou, Liu, Wang, Liao and Xing. This is an open-access article distributed under the terms of the Creative Commons Attribution License (CC BY). The use, distribution or reproduction in other forums is permitted, provided the original author(s) and the copyright owner(s) are credited and that the original publication in this journal is cited, in accordance with accepted academic practice. No use, distribution or reproduction is permitted which does not comply with these terms.
*Correspondence: Sentai Liao, bGlhb3N0QDE2My5jb20=; Dongxu Xing, ZG9uZ3h1eGluZ0AxMjYuY29t
†These authors have contributed equally to this work