- 1Department of Clinical and Molecular Medicine, “Sapienza” University, S. Andrea University Hospital, Rome, Italy
- 2Translational Research Unit, National Institute for Infectious Diseases “Lazzaro Spallanzani”- Istituto di Ricovero e Cura a Carattere Scientifico (IRCCS), Rome, Italy
- 3Istituti Clinici Scientifici Maugeri, Istituto di Ricovero e Cura a Carattere Scientifico (IRCCS), Tradate, Italy
Tuberculosis (TB) is the leading cause of death in the world from an infectious disease. Its etiologic agent, the Mycobacterium tuberculosis (Mtb), is a slow-growing bacterium that has coexisted in humans for thousands of years. According to the World Health Organization, 10.6 million new cases of TB and over 1 million deaths were reported in 2022. It is widely recognized that patients affected by chronic autoimmune arthritis such as rheumatoid arthritis (RA), psoriatic arthritis (PsA), and ankylosing spondylitis (AS) have an increased incidence rate of TB disease compared to the general population. As conceivable, the risk is associated with age ≥65 years and is higher in endemic regions, but immunosuppressive therapy plays a pivotal role. Several systematic reviews have analysed the impact of anti-TNF-α agents on the risk of TB in patients with chronic autoimmune arthritis, as well as for other biologic disease-modifying immunosuppressive anti-rheumatic drugs (bDMARDs) such as rituximab, abatacept, tocilizumab, ustekinumab, and secukinumab. However, the data are less robust compared to those available with TNF-α inhibitors. Conversely, data on anti-IL23 agents and JAK inhibitors (JAK-i), which have been more recently introduced for the treatment of RA and PsA/AS, are limited. TB screening and preventive therapy are recommended in Mtb-infected patients undergoing bDMARDs and targeted synthetic (ts)DMARDs. In this review, we evaluate the current evidence from randomized clinical trials, long-term extension studies, and real-life studies regarding the risk of TB in patients with RA, PsA, and AS treated with bDMARDs and tsDMARDs. According to the current evidence, TNF-α inhibitors carry the greatest risk of TB progression among bDMARDs and tsDMARDs, such as JAK inhibitors and anti-IL-6R agents. The management of TB screening and the updated preventive therapy are reported.
1 Introduction
Tuberculosis (TB) is the leading cause of death in the world from an infectious disease. Its etiologic agent, the Mycobacterium tuberculosis (Mtb), is a slow-growing bacterium that has coexisted in humans for thousands of years. According to the World Health Organization (WHO), 10.6 million new cases of TB and over 1 million deaths were reported in 2022 (1).
As a respiratory pathogen, the transmission occurs through inhalation of aerosols or droplets containing bacilli expelled by a person with TB disease. An estimated quarter of the world population has been infected with Mtb (1). Most TB cases are reported in low- and middle-income countries. In particular, more than two-thirds of people with TB live in Bangladesh, China, India, Indonesia, Nigeria, Pakistan, Philippines and South Africa (1). This heterogeneous distribution is due to the differences between countries in terms of social and economic development and health-related factors, such as alcohol use disorders, diabetes, HIV infection, smoking and undernourishment, which are known to increase the risk of TB disease (2). In addition to health conditions, immunosuppressive therapies affecting the immune system, including those used for rheumatoid arthritis (RA), psoriatic arthritis (PsA) and ankylosing spondylitis (AS), increase the risk of TB disease in Mtb-infected individuals (3).
Following infection, the majority (90%) control Mtb replication through innate and adaptive immunity establishing a state referred to as TB infection (TBI), and in the past called latent TB infection (4). On the other hand, 5-10% of the infected subjects can develop TB disease; half of them within the first 5 years, and half during their lifetime.
TB is traditionally classified as primary or secondary according to the time between the initial infection and the onset of the clinical disease. Primary TB occurs in previously uninfected subjects after de novo infection, whereas secondary TB develops in a previously sensitized host, and it may occur following reactivation of Mtb infection or reinfection from external source. Indeed, secondary TB usually, but not always, develops in a person with a weakened immune system (5).
In the context of Mtb infection, a dynamic equilibrium between the host and the microbe is present, with bacilli that can switch from a dormant state to intermittent or active replication depending on the capability of the host immune system to contain or not Mtb replication (6, 7). Therefore, TB is referred to as a “continuum process” characterized by different stages between TB infection and TB disease, as described elsewhere (8, 9).
In this review, we revised the current evidence from randomized clinical trials (RCTs), long-term extension studies (LTEs), and real-life studies regarding the risk of TB in patients with chronic autoimmune arthritis including rheumatoid arthritis (RA), psoriatic arthritis (PsA), and ankylosing spondylitis (AS), treated with biologic and targeted disease-modifying immunosuppressive anti-rheumatic drugs (bDMARDs; tsDMARDs).
2 Immunopathogenesis of TB
The immune response to Mtb infection is multifaceted and it involves both innate and adaptive immune response (4, 10). Upon infection, bacilli are phagocytosed by alveolar macrophages, which represent the first defense line against Mtb due to their antimicrobial mechanisms (11, 12). However, Mtb has evolved different mechanisms to avoid its elimination by inhibiting phagosome maturation and acidification, and escaping autophagy in macrophages (13–15), which become a permissive niche for Mtb replication.
As the infection progresses, macrophages migrate into the lung interstitium where they recruit other innate cells such as neutrophils, monocytes, macrophages, and dendritic cells due to the release of cytokines, including TNF-α, IL-1α, IL-6, IL-1β and IFN-γ, thus favouring the dissemination of mycobacteria to uninfected cells. Once activated, T and likely B cells are recruited to the site of infection contributing to the formation of the organized granuloma, a structure known as the hallmark of TB (10). The immune microenvironment within the granuloma influences the prognosis and outcome of TB disease leading to different scenarios: Mtb clearance, bacterial replication causing primary TB, bacterial dormancy, or reactivation of the infection (16–19).
CD4+ Th1 cells producing cytokines such as IFN-γ and TNF-α have been identified as the most important cell subset to control Mtb infection. The differentiation of naïve CD4+ T cells to Th1 cells is promoted by IL-12, a cytokine released by antigen presenting cells (APCs) (20). IFN-γ and TNF-α enhance the antibacterial activity of macrophages by increasing autophagy, promoting phagosome maturation, and inducing the production of antimicrobial peptides. Besides macrophages, IFN-γ and TNF-α activate B cells and the cytotoxic CD8+ T cells. Both cytokines are of utmost importance for the formation and maintenance of a well-organized granuloma (21).
The role of Th17 cells, whose differentiation is induced by IL-23, is controversial. Th17 response seems to be involved in the early steps of protection from Mtb infection, and the recruitment of neutrophils, macrophages, and Th1 cells to the site of infection (22, 23). Th17 cells enhance the expression of cytokines (IL-17A, IL-17F, IL-21 and IL-22) and antimicrobial peptides that lead to phagocytosis of Mtb (23). IL-17 may be released by either innate lymphocytes of the ILC3 class or Th1/Th17, and it seems to be implicated in the maturation process of granulomas (24). However, an overproduction of IL-17 was also associated with exaggerated recruitment of neutrophils and inflammation leading to immunopathology (25, 26). As with IL-17, also the excessive production of other pro-inflammatory cytokines such as TNF-α, IL-1, IFN-γ may result in tissue damage and bacterial growth. A balance is crucial to control progression to TB disease (27).
The pivotal role of IL-12, IFN-γ, and TNF-α in controlling Mtb infection is corroborated by the higher susceptibility to TB disease of the individuals treated with immunosuppressive therapies like TNF-α inhibitors (28–30), or individuals with innate defects of the IL-12/IFN-γ axis (31–33), with HIV infection (34) or with primary immunodeficiencies associated with T-cell deficiency (35).
Mendelian susceptibility to mycobacterial disease (MSMD) is an inborn error of immunity associated with a selective predisposition to mycobacterial infections. MSMD involves specific mutations in 18 genes (IFNG, IFNGR1, IFNGR2, STAT1, IL12B, IL12RB1, IL12RB2, IL23R, RORC, TBX21, IRF8, SPPL2A, ISG15, TYK2, JAK1, ZNFX1, NEMO, CYBB), which are associated with an impaired IFNγ/IL-12 response/production (35). Moreover, patients with defects of CD40 ligand (CD40L) and NF-kB signaling are more susceptible to mycobacterial disease, as this pathway is involved in the IL-12 production (36).
A number of distinct Mendelian disorders are also caused by inborn errors in components of the IL-6 family of cytokines and their signaling pathways (STAT3 and GP130) (37). The majority of patients with TYK2 defects, one of the three Janus kinases (JAKs) associated with GP130 signaling, shows defects in type I antiviral and mycobacterial immunity (38).
In addition to the use of TNF-α inhibitors, the inherited TNF deficiency has been identified as a genetic aetiology of recurrent pulmonary TB in adults observed within 1 year of the end of treatment. TNF deficiency seems to be responsible for the selective impairment of reactive oxygen species (ROS) production by alveolar macrophages. The ROS production is crucial for the phagocytic control of Mtb (39).
Regarding B cells and antibodies (Abs), initially there was some scepticism about their effective contribution to the host defense against Mtb due to the intracellular nature of the pathogen (40, 41). However, although B cells and Abs may not be able on their own to counteract Mtb, the accumulating evidence shows that they can favour and enhance cell-mediated immunity (42). Indeed, Abs binding to Mtb can mediate different processes such as antibody-dependent cellular cytotoxicity, antibody-dependent cellular phagocytosis, and complement activation, thus helping to reduce the mycobacterial burden (42). However, B cells are not limited to antibody production. They can act as antigen-presenting cells by presenting mycobacterial antigens to T cells, thereby inducing their activation. In addition, once activated, B cells can release cytokines, thus affecting the activity of different immune cells (43, 44). The role of B cells in controlling Mtb infection is corroborated by the association of TB disease with reduced B cell count and function (45, 46).
Considering the pivotal role played by the host immune response in controlling Mtb replication, risk factors for the progression to TB disease include immunosuppressive therapies (Figure 1).
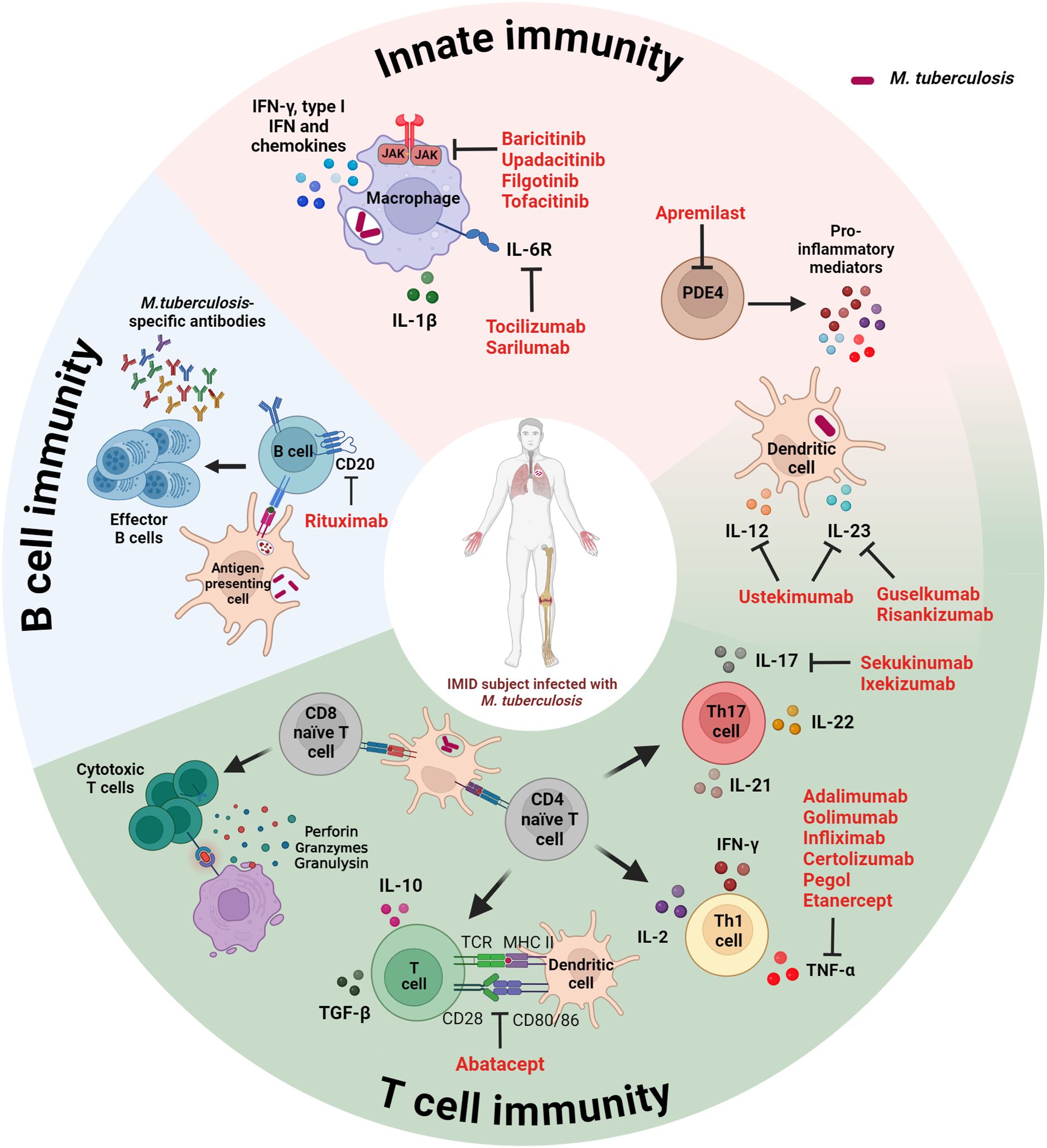
Figure 1. Schematic representation of the immune response targets of disease-modifying immunosuppressive anti-rheumatic drugs used for the management of IMID patients. Both innate and adaptive immunity (B and T cells) play a key role in controlling Mtb infection. Immunosuppressive therapies targeting host immune factors increase the risk for progression to TB. IMID, immune-mediated inflammatory disease; IFN, interferon; IL, interleukin; JAK, Janus kinase; MHC, major histocompatibility complex; PDE4, phosphodiesterase-4; TCR, T cell receptor; TGF, tumor growth factor. Created with BioRender.com.
3 Patients with chronic autoimmune arthritis and TB risk
Patients with chronic autoimmune arthritis such as RA, PsA and AS are at higher risk of infections and related complications, which are the main cause of mortality in these conditions (47).
It is widely recognized that patients affected by RA, PsA, and AS have an increased incidence rate of TB disease compared to the general population, primarily related to immunosuppressive therapy rather than the disease itself. As conceivable, the risk is associated with age ≥65 years and is higher in endemic regions, but the use of immunosuppressive therapy plays a key role also in low TB endemic countries (3, 48). There is evidence that corticosteroids (CCS) can increase the risk of TBI reactivation in a dose-dependent manner (49, 50).
Among csDMARDs commonly used for the management of RA, PsA, and AS, leflunomide and azathioprine have emerged as having the highest risk, whereas sulfasalazine and methotrexate appear to confer only low to moderate risk (50, 51). In the last 20 years, biologic DMARDs (bDMARDs) have significantly improved the treatment of rheumatologic diseases, including RA, PsA, and AS, contributing to introduce the ambitious target of remission/minimal disease activity.
Anti-TNFα agents were the first bDMARDs to be adopted and are still the most used worldwide. Currently, 4 anti-TNF monoclonal antibodies and one receptor fusion protein are available: adalimumab (ADA), etanercept (ETN), infliximab (IFX), golimumab (GOL), and certolizumab (CTP). Other bDMARDs are used exclusively for the treatment of RA, including the anti-IL6 receptors tocilizumab and sarilumab, as well as the anti-CD20 rituximab. In contrast, the anti-IL12/23 ustekinumab, anti-IL23 guselkumab and risankizumab, and IL-17 inhibitors secukinumab and ixekizumab are used only for PsA and AS. Finally, the CTLA4-Ig abatacept and JAK inhibitors (JAK-i) are approved for both RA and PsA/AS. The increased risk of TB in patients with chronic autoimmune arthritis undergoing anti-TNF agents is widely recognized (3, 52–55). Although the data are less robust, there are also substantial data available for other bDMARDs such as rituximab, abatacept, tocilizumab, ustekinumab, and secukinumab (56–58).
Conversely, data on anti-IL23 agents and JAK inhibitors (JAK-i), which have been more recently introduced for the treatment of RA and PsA/AS, are scarcer.
4 Specific biological therapy for autoimmune arthritis and TB risk
In the following section, we describe the mechanism of action of bDMARDs and tsDMARDs used for the treatment of RA, PsA, and AS, and analyze how inhibiting specific pathogenic pathways might affect the integrity of the TB granuloma. We then summarize the main data on TB risk associated with these therapeutic agents from RCTs, LTE, and real-world studies in these pathologies (Table 1). Indeed, it is important to underline that RCTs provide very reliable and complete data, but patients with TB disease were not included, and patients with TBI could be enrolled only after having received TB preventive therapy according to local guidelines. On the other hand, real-life studies (registries and observational studies) are affected by a higher risk of bias, but can also report cases of TBI patients who did not receive preventive treatment; thus, they are particularly relevant for inferring the impact of b/tsDMARDs on the natural course of TBI.
4.1 TNF-α inhibitors
TNF-α is a pivotal cytokine in the pathogenesis of RA, PsA, and AS, as it acts on different cells such as synoviocytes, macrophages, chondrocytes, and osteoclasts. It induces local inflammation and pannus formation, contributing also to cartilage degradation and bone erosions (59, 60). High TNF-α levels have been observed in the synovial fluid and synovium of patients with RA and PsA (60).
As previously mentioned, TNF-α is also critical for the formation and maintenance of Mtb granulomas. TNF-α enhances the phagocytic capacity of macrophages, promotes the production of reactive nitrogen and oxygen species to kill intracellular bacteria, and facilitates the recruitment of immune cells at the site of infection (21).
TNF-α inhibitors neutralize TNF-α activity, by disrupting the immune response necessary for granuloma integrity, and leading to mycobacterial growth and dissemination with progression to TB disease.
There are remarkable differences among anti-TNF-α agents in their ability to inhibit TNF-α, that can explain the reported differences in TB risk. Anti-TNF-α mAbs target and neutralize both soluble and membrane-bound TNF-α with high affinity, and also have some cross-reactivity with Lymphotoxin (LT)-α (61). On the other hand, ETN, being a dimeric fusion protein, binds to the trimeric form of soluble TNF-α and, only to a lesser extent, to membrane-bound TNF-α and LT-α (62, 63).
The more comprehensive blockade of TNF-α activity and functions in immune defense mechanisms by anti-TNF-α mAbs may contribute to the observed higher risk of TBI reactivation.
The early clinical trials of IFX and ETN revealed a significant risk of TB, leading to the introduction of mandatory TB screening guidelines for patients starting anti-TNF-α therapy.
In particular, in the ATTRACT and ASPIRE RCTs there were 70 cases of TB disease among patients receiving IFX for an incidence rate (IR) of approximately 0.5-1.0/100 patient-years (PY); the majority of these cases occurred within the first few months of therapy (64).
ETN showed a lower incidence of TB in its pivotal ERA trial; however, the risk was still significant enough to warrant concern with an IR of 0.02-0.1/100PY (65). By the early 2000s, both the Food and Drug Administration (FDA) and the European Medicines Agency (EMA) had issued guidelines recommending TB screening before starting treatment with anti-TNF-α agents.
The subsequent implementation of screening protocols has significantly reduced TB rates, contributing to the lower incidence observed in LTE studies and real-world applications of IFX, ADA, and ETN, as well as with newer anti-TNF-α agents like golimumab and certolizumab pegol (52). Indeed, the IR of TB reactivation in clinical trials and LTEs conducted after the introduction of TB screening, decreased to 0.2-0.3 for IFX and to 0.1-0.2/100PY for ETN and ADA (66–79).
Also, golimumab and certolizumab pegol trials reported relatively low rates of TB. Indeed, the GO-BEFORE and GO-FORWARD golimumab studies found an IR of 0.2/100PY, whereas the RAPID 1-2 certolizumab trials found an IR of 0.1/100PY (80–84).
Different registries evaluated the incidence of TB in patients receiving TNF-α inhibitors after the introduction of TB screening protocols with similar results. Among these, the RABBIT registry reported an IR of 0.14/100PY, the BIOBADASER found an IR of 0.05-0.1/100PY, while the ARTIS registry reported an IR of 0.15/100PY (85–87).
Data from previous systematic reviews and meta-analyses showed similar data, with an IR of 0.18/100PY in rheumatic patients receiving anti-TNF-α, 4 times higher compared to rheumatic patients not receiving these therapies (88). Rigorous screening and prophylactic treatment are required on the summary of product characteristics (SmPC) of anti-TNF-α agents.
4.2 Anti-IL6R
Tocilizumab (TCZ) is a humanized monoclonal antibody targeting the human IL-6 receptor (IL6R). It was approved by EMA in 2009 for the treatment of RA patients. Sarilumab is another fully human monoclonal antibody targeting the IL-6R, more recently approved for RA treatment.
IL-6 is a versatile cytokine with a wide array of functions, including modulation of acute phase reactant pathways, B and T lymphocytes, blood-brain barrier permeability, synovial inflammation, and hematopoiesis. This cytokine plays a crucial role in bridging innate and adaptive immune responses, and in facilitating the recruitment of macrophages. Dysregulation of the IL-6 axis is implicated in the inflammatory pathways of various autoimmune disorders, such as RA.
Previous studies on experimental mice models showed that IL-6 plays a significant role in the protection against Mtb, and the absence of IL-6 leads to an early increase in bacterial load with a concurrent delay in the IFN-γ induction. However, IL6 knockout mice contained and controlled bacterial growth and developed a protective memory response to secondary infection, demonstrating that while IL-6 is involved in stimulating early IFN-γ production, it is not essential for the development of protective immunity against Mtb. The role of IL-6 in human TB remains controversial, and the specific functions of the IL-6 produced by B cells are still poorly understood, despite its abundance in TB-infected lungs. Some studies have reported increased concentrations of IL-6 in the sera of patients with advanced pulmonary TB compared to healthy controls, as well as elevated IL-6 gene expression in peripheral blood cells of TB patients, supporting a potential pathophysiological role (89, 90).
Furthermore, a recent study demonstrated that treatment with in vitro TCZ does not inhibit IFN-γ-specific response on whole blood from patients with TB disease stimulated with two different Mtb antigens, differently from the effects observed with ETN and IFX, both of which led to a reduced IFN-γ response (57, 91, 92).
A comprehensive safety analysis and systematic review published in 2014 assessed the incidence of TB in patients treated with TCZ from RCTs and LTE studies, and found no cases of TB disease among 15485 RA patients (29). A meta-analysis of RCTs trials and LTEs found 9 cases of TBI reactivation on 12509PY for an IR of 0.069/100PY (93). Data from the British Society for Rheumatology Biologics Register for Rheumatoid Arthritis (BSRBR-RA) showed one case of TB disease among 2171 RA patients treated with TCZ, resulting in an IR of 0.026/100PY (94).
Observational studies from European countries and a Japan nationwide study did not detect TB cases in TCZ users (95). Finally, a recently published nationwide observational study on RA patients from Korea, an intermediate TB burden country, reported 10 TB cases on 2185PY for an IR of 0.45/100PY (96).
The IR was similar to ETN and higher in TBI patients than those without TBI, indicating different effects between de novo infection and TB reactivation. Screening for TBI is mandatory in the SmPC of IL6R inhibitors.
4.3 Anti-IL-17
The IL-17 family encompasses six proteins (IL-17A to IL-17F) and five receptors (IL-17RA to IL-17RE). While IL-17A and IL-17F individually possess limited inflammatory potency, their robust inflammatory effects primarily stem from their ability to recruit immune cells and synergize with other pro-inflammatory cytokines like TNF-α, IL-1β, and IL-22. Through the recruitment and activation of neutrophils, IL-17A and IL-17F serve as pivotal components in the innate immune response against extracellular bacteria and fungi. Their protective role is particularly important on mucosal surfaces and skin, where they are rapidly released upon appropriate stimulation, thereby serving as a crucial link between innate and adaptive immune responses.
The involvement of IL-17 in TB pathogenesis has been debated, raising concerns and uncertainties about TB risk. Indeed, early granuloma formation may depend on IL-17A, but IL-17A-induced neutrophil recruitment may also increase pathological lesions and bacterial burden in chronic pulmonary infections. Notably, an in vitro study on a microgranuloma human model demonstrated that anti-TNF-α treatment could induce Mtb reactivation, whereas anti-IL17 treatment was comparable to control, indicating a lack of effect on Mtb dormancy. Moreover, mice lacking both IL-17RA and IL-22 pathways still managed to control TB, suggesting no compensatory relationship between these pathways. In contrast, TNF-α-deficient mice succumbed rapidly (97). In a series of studies by Khader and Cooper, low-dose aerosolized bacteria were delivered to the lower airways of the lungs in IL-17/IL-23 deficient mice (98, 99). Notably, the absence of IL-23 and IL-17 in the lung leads to more severe inflammation, suggesting that these cytokines help maintaining the granuloma integrity in later stages of Mtb-induced inflammation by limiting neutrophil death.
Secukinumab (SEK), a fully human monoclonal IgG1 antibody, specifically targets and inhibits IL-17A. Following the demonstration of its significant efficacy in phase 3 studies in 2015, it was approved for treating PsA and AS in 2016. Pooled data from 5 phase III trials on PsA (FUTURE program), and 4 phase III trials (MEASURE program) on AS, on a total of 2523 and 977 patients respectively, reported 5 cases of new TBI (one PsA and 2 AS patients), and no cases of TB disease (100). In LTE studies (1–5 years) in patients with PsA and AS, the safety profile was consistent with that of previous phase III studies, and no new TB infections or TB disease reactivations were observed (101). Notably, Liu et al. reported zero cases of reactivation among 3 PsA/AS patients with TBI who did not receive TB preventive therapy (102). Ngoc et al. in 2022 described a case of TB disease from Vietnam in a 19-years-old man affected by AS after two years of SEK treatment (103).
Ixekizumab (IXE) also neutralizes IL-17A but, differently from SEK, it is a humanized IgG4 monoclonal antibody. This structural variation contributes to its higher affinity for IL-17. Its approval for PsA and AS was granted in 2018. No TB disease cases from pooled analysis of 3 RCTs (SPIRIT program) on PsA patients were recorded. In the PsA studies, 32 (2.9%) patients resulted in TBI during the study, of whom 20 were discontinued per-protocol. Interestingly, among the remaining 12 patients continuing IXE treatment, no cases of TB reactivation were reported, even though only 7 patients received TBI therapy (104).
Finally, bimekizumab (BMK) represents a humanized IgG1 monoclonal antibody with dual neutralizing effects against both IL-17A and IL-17F. It has been very recently approved for the treatment of PsA and AS. A total of 267 PsA patients from BE COMPLETE trial and its open-label extension 1-yr follow-up (BE VITAL trial), reported no cases of TB disease (105). Data from two phase III BE MOBILES trials on AS, did not report TB disease cases at 1-yr follow-up. Finally, a total of 303 patients with active AS from BE AGILE trial and its open-label extension study reported no cases of TB disease (106, 107). We still need data from real-life studies and longer follow-ups. TB screening is only suggested in the SmPC of anti-IL17 agents.
4.4 Anti-IL-23 and IL-12/23
IL-12 and IL-23 are heterodimeric cytokines containing p35 and p40 subunits, and p19 and p40 subunits, respectively. IL-12 and IL-23 are produced by APCs, such as dendritic cells, macrophages, and monocytes. IL-12 plays a key role in the differentiation of naïve CD4+ T cells into Th1 cells, whereas IL-23 is involved in the expansion and maintenance of Th17 cells. A role for IL-12 and IL-23 dysregulation in the pathophysiology of PsA has been suggested (108).
Ustekinumab (UST) is a human monoclonal antibody that binds the p40 subunit shared by both IL-12 and IL-23, effectively suppressing their functions. It was approved for the treatment of PsA patients in 2013. No TB disease cases were observed neither in pivotal studies (PSUMMIT program) on a total of 1073 PsA patients, nor in their LTE data (109, 110). Few data from available real-life observational studies on PsA patients did not report cases of TB reactivation, and previous reviews have assessed the risk of TB reactivation as very low (111). A case of peritoneal TB in a PsA patient from Philippines, on UST treatment, with multi bio-failure, and after having been treated for latent TB, was observed (112). Screening and treatment of TBI are recommended in the SmPC supplied with UST.
Guselkumab (GUS) is a fully human IgG1λ monoclonal antibody, which specifically binds to the p19 subunit of IL-23. It stands as the first of its class to gain approval for the treatment of PsA patients.
Risankizumab (RSK) is a humanized immunoglobulin G1 monoclonal antibody that specifically inhibits IL-23 by binding to its p19 subunit, and it has been recently approved to treat PsA. Pooled data from DISCOVER 1 and 2 trials on 748 patients with active PsA for GUS reported zero cases of TB reactivation at 1-year follow-up (113, 114). RSK safety data sets from 4 phase II and III trials (KEEPsAKE program) in PsA on a total 1542 patients representing 2741.6PY, reported one case of TB disease in a patient from Taiwan previously treated with a 9-month course of isoniazid prophylaxis (115, 116). There is still very little real-life data on anti-IL-23 in PsA patients with RSK. Takeda et al. reported the case of a 64-year-old man affected by PsA who developed active pulmonary TB after two months of GUS therapy (the patient was negative at baseline TB screening) (117).
Notably, several real-world data are available for patients with psoriasis. A total of 68 and 25 TBI patients, who did not receive any or adequate TBI preventive therapy, were treated with RSK and GUS, respectively (118–122). Remarkably, there were no documented cases of TB reactivation, which corroborates the safety profile of anti-IL-23 agents in patients with TBI who did not receive prophylactic care. In the SmPC of anti-IL-23 agents is indicated that patients should be evaluated for TBI before starting treatment.
4.5 Anti-CD20
Rituximab (RTX) is a chimeric monoclonal antibody targeted against CD20, which is expressed on the surface of normal and malignant B lymphocytes. It was first approved by the FDA in 1997 for the treatment of malignancy, and in February 2006 for the treatment of patients with moderately to severely active RA, who did not adequately respond to one or more anti-TNF-α agents. RTX binds via its F(ab0)2 portion to the CD20 antigen expressed on B lymphocytes, whereas its Fc domain plays immune effector functions to mediate B cell lysis in vitro. RTX cytotoxicity is mediated by three different mechanisms including antibody-dependent cellular cytotoxicity, complement-dependent cytotoxicity, direct disruption of signaling pathways, and triggering of apoptosis (123, 124).
RTX’s targeted action on B cells, which spares the critical TNF-α pathways necessary for TB containment, likely explains its lower associated risk of TB reactivation compared to anti-TNF-α therapies as reported by several data (125–127). No cases of TB disease have been reported in patients receiving RTX in 9 RCTs conducted in 3623 RA patients. In two LTEs in RA patients, two cases of TB disease have been reported during a follow-up time of 9.5 years (IR 0.018/100PY) (29, 128). Data from real-life studies and registries found very few cases of de novo TB infection or TB disease reactivation during RTX treatment (129–132).
Data from observational studies and registries reported very few cases of TB disease. In particular, only one TB case was reported in 2484 RA patients treated with RTX in the German GENIRIS study, and 2 cases from the BSRBR-RA registry during 17154PY (0.012/100PY) (127, 133).
Finally, a meta-analysis including data from several clinical trials and LTEs reported that the IR of TB disease was high (>0.040/100PY) for patients treated with tofacitinib and all biologics but RTX (0.020/100PY) (93). Overall RTX emerged as having one of the lowest pooled IR of TB among bDMARDs. In line with this evidence, the summary of product characteristics of RTX does not specifically mandate routine screening for TB before initiating therapy.
4.6 CTLA-Ig
Abatacept (ABT) is a fully human recombinant fusion protein composed of the extracellular binding domain of human cytotoxic T lymphocyte-associated antigen-4 (CTLA-4), fused to a modified segment of human IgG1. Its mechanism of action involves blocking the CD80/CD86 costimulatory pathways, thus preventing the activation of naïve T cells. ABT binds to CD80 and CD86 on APCs with higher affinity compared to CD28 on T cells, effectively interfering with the interaction between CD28 and CD80/CD86 (134). ABT has been approved by the EMA for the treatment of RA since 2007, 10 years later it received approval for the treatment of PsA patients.
Its peculiar mechanism of immune modulation, which avoids direct cytokine inhibition, seems to be a key factor that likely contributes to its safer profile compared to anti-TNF-α regarding the overall risk of infections and, in particular, TB reactivation.
Pooled data from 8 RCTs and LTE studies on RA patients revealed an overall IR of 0.0066/100PY (135). A recent 10-year international post-marketing study found a very low IR across different registries (ARTIS, FORWARD, RABBIT) with only one event on a total of 9652PY of exposure (136). TB rates were low but slightly higher in two Canadian and USA registries, with 9 cases on 1067PY and 17 cases on 3994PY, respectively (137). Finally, a recent meta-analysis of LTEs showed a low estimated pooled IR of 0.07/100PY (93). Despite these reassuring data, screening for TBI is recommended in the SmPC of abatacept.
4.7 Apremilast
Apremilast, an oral phosphodiesterase-4 (PDE4) inhibitor that effectively modulates various inflammatory mediators, has demonstrated efficacy with a favorable safety profile in several RCTs involving PsA patients with peripheral involvement (138).
These trials excluded patients with TB disease, but they did not require TBI screening for enrolment. This finding is noteworthy: patients with TBI treated with apremilast without preventive therapy showed no instances of reactivation (139).
Analysis of pooled data from the PALACE I–II–III studies involving a total of 1493 PsA patients, reported zero cases of TB disease (140). A comprehensive retrospective analysis using a large US-based claims database, including patients diagnosed with psoriasis and/or PsA who were administered at least one dose of apremilast between 2014 and 2018, identified only two cases of TB disease among 10074 patients (141).
Overall, available data highlight the minimal association of apremilast with TB reactivation. Notably, the prescribing information for apremilast does not mention the necessity for TB screening before initiation of treatment.
4.8 JAK inhibitors
Janus kinase inhibitors (JAK-i) are non-receptor tyrosine kinases associated with the cytoplasmic domain of type I and II cytokine receptors, which are activated after the engagement by their cognate ligands. Once phosphorylated, they phosphorylate signal transducers and activators of transcription (STATs), which then induce gene activation essential for cellular functions like signaling, growth, and survival (142).
The JAK family comprises four cytoplasmic non-receptor tyrosine kinases: JAK1, JAK2, JAK3, and TYK2. JAK-i are categorized into two generations. The first generation includes small molecules like baricitinib (BAR) and tofacitinib (TOF), which act as non-selective inhibitors of JAKs. In contrast, second-generation drugs such as filgotinib (FLG) and upadacitinib (UPA) exhibit more selective inhibitory activity against JAK1 than other JAK (143, 144). UPA and TOF are approved for the treatment of RA, PsA, and AS, whereas FLG and BAR are approved for RA only.
It can be speculated that the broad immunosuppressive effects exerted by the mechanism of action of JAK inhibitors, particularly through the downregulation of IFN-γ, TNF-α, and IL-6, could disrupt critical host defenses, including macrophage activation, granuloma formation, and Mtb containment.
Notably, in a BALB/c mice model, TOF was shown to diminish the control of Mtb, leading to increased bacterial replication in the lungs during chronic paucibacillary TB. This model is designed to replicate latency in a manner analogous to human TBI (145). Screening for TBI is recommended in the SmPC of all JAK-i.
Data on TOF and TB were pooled from 7061 patients across the completed 2 phase I, 10 phase II, 6 phase III, 1 phase IIIb/IV index studies, and 2 open-label LTE studies (total exposure 22875PY). TB disease was reported in 36 (0.5%) patients, with an IR of 0.2/100PY. Pulmonary and non-pulmonary TB occurred in 17 and 19 patients respectively, with most cases occurring in geographical regions endemic to TB (146). Twenty-six cases of TB disease were identified from a post-marketing surveillance analysis of TOF, on a total of 5671 RA patients for an IR of 0.21/100PY, most of which were from regions with high background IR for TB (147). Finally, a retrospective, single-center analysis from Western India reported 4 TB cases on a total of 102 RA patients treated with TOF (148).
Regarding baricitinib, an integrated study from 9 RCTs conducted over 20 countries in patients with RA and one LTE study with a follow-up period of up to 7 years, showed a total IR for TB disease of 0.2/100 PY (15 out of 3770 patients; 14744 PY) (149, 150). The IR did not increase with prolonged exposure and the events occurred mainly in endemic countries.
Data on TB risk in patients receiving UPA are pooled from 12 clinical trials (SELECT program) on 3209 patients with RA (9079.1PY), 907 patients with PsA (1872.3PY) and 182 with AS (320.1PY), showed only one case of TB reactivation (151–153). The long-term extensions analysis on 3209 RA patients for 11661.5PY, recorded one case of disseminated TB, one case of peritoneal TB, two cases of pulmonary TB, one case of female genital tract TB and 174 cases of TBI reactivation (154). No cases of TB disease were recorded in the RCTs and LTE studies conducted in PsA and AS patients, whereas a total of 51 cases of TBI reactivation were reported (155–157). We still have a few real-life data on UPA. In two recent prospective longitudinal multicenter Italian studies in RA patients enrolling 71 and 60 patients respectively, neither TB disease nor new TBI were detected during the 6 months follow-up (158, 159).
The FINCH programme, a 52-week phase 3 RCT evaluated FLG in 833 RA patients, recorded no cases of TB disease (160).
Ninenty-one cases of TB disease reactivation and no new onset TB disease cases were reported in the DARWIN clinical trial and its LTE analysis on 739 RA patients (161). Overall, these findings suggest that JAK-i carry a risk of TB, particularly in endemic regions.
5 Screening strategies and preventive therapy for TB
It is estimated that approximately one-fourth of the global population has an immune response to Mtb, indicating previous exposure or infection with the bacterium (1). Since 2015, the WHO has recommended screening and treating TBI in populations at higher risk of progression to the disease, within preventive actions of the WHO End TB Strategy (3, 162).
Patients with autoimmune diseases candidates for biological treatment are considered at risk of progressing to TB. Therefore, International guidelines (163) recommend screening and TBI preventive treatment of those with a TBI diagnosis who are candidates for biological treatment. Patients are screened for TBI either using skin tests, or interferon-γ release assays (IGRAs) based on the national guidelines in place (6, 164). Skin tests are based on the intradermal inoculation in the forearm of the purified protein derivative (PPD) as in the tuberculin skin tests (TST), or on ESAT-6 and CFP-10, as in the new generation of skin tests (165).
IGRAs are blood tests based on in vitro stimulation with ESAT-6 and CFP-10; the read-out is based on IFN-γ or IP-10 detection that can be performed in automated or semi-automated ways (6, 164).
In countries with BCG vaccination, where TST may show false positives, ESAT-6 and CFP-10 based assays (IGRAs or skin tests) are preferred. If either the skin test or IGRA is positive, the patient is considered with TBI (162, 166) and will undergo a chest X-ray (CXR) to exclude TB disease (162). A baseline CXR can be useful also for those who score negative on skin tests or IGRA to evaluate lung apical scores compatible with spontaneously healed TB, such as non-calcified nodules with distinct margins and fibrotic linear opacity (167). If TBI is diagnosed from a positive skin test or IGRA without lung lesions, or based solely on lung apical scars, preventive treatment is offered to those at high risk to develop TB (162).
TB preventive therapy aims to eliminate the remaining replicating mycobacteria in the body, thus resulting in a lower risk of developing the disease. This has proven to be effective in preventing TB in several populations, including children (162, 168, 169).
6 TB preventive therapy drugs and drug regimens
TB preventive therapy comprises one or two antibiotics and is different from the therapy used for TB disease, in which four antibiotics are used to reduce the likelihood of acquired drug resistance. This assumes that in TBI the acquired drug resistance is unlikely, given the small number of viable bacteria present.
Drugs commonly used for TB preventive therapies may cause adverse reactions such as liver or neuro-toxicities (Table 2). Isoniazid (INH) is an oral antibiotic with intracellular and extracellular activity against Mtb. Isoniazid has been used globally, with an average protective effect for TB of 60% during the observation period (170). The duration is 6 months, although, in 1982, a randomized trial in subjects with fibrotic pulmonary lesions showed that the risk for developing TB disease compared with placebo was reduced by 21%, 65%, and 75%, respectively for 3-, 6-, or 9-months therapy, after 5 years of follow-up (171). However, a 6-month regimen was shown to be more cost-effective than 3 or 12 months for the reduction of side effects, regimen adherence and increased adherence (162, 172–174). Neuropathy can arise as an isoniazid side effect due to the inhibitory effect of isoniazid on the function of pyridoxine metabolites; therefore, pyridoxine (vitamin B6) supplementation is recommended especially in those with alcohol abuse, malnourished, and pregnant women (175).
Rifamycins, a group of oral antibiotics such as rifampin and rifapentine, inhibit bacterial RNA synthesis by binding to the DNA-dependent RNA polymerase. These antibiotics are used to treat TBI by themselves or in combination with isoniazid to limit the side effects and the poor adherence to the long treatment duration of isoniazid (162).
Rifampicin regimens, like the 4-month course of rifampicin (4R) (162) or even shorter regimens, like the 3-month course of isoniazid and rifampicin (3HR), showed good safety and completion rates, particularly among children, with dispersible fixed-dose combinations aiding administration (176–178). For adults, however, liver toxicity and completion rate are comparable to those of longer isoniazid preventive therapy (177, 179). Administering a once-weekly dose of isoniazid and rifapentine for 12 weeks (3HP) is associated with lower rates of hepatotoxicity and higher completion rates when compared with isoniazid, although it was linked with the incidence of a hypersensitivity systemic immune response (177). Conversely, a recent meta-analysis indicated an increased incidence of grade 3 and 4 adverse events as well as a greater rate of treatment discontinuation for the 3HP regimen when compared with the 6–9 month isoniazid preventive therapy (IPT) (180).
A regimen of one-month daily INH and rifapentine (1HP) is an alternative for HIV-infected patients, and WHO conditionally recommended it for people aged above 13 years, although additional evaluations of safety and efficacy are needed in people without HIV (162).
For contacts of people with MDR-TB, WHO recommends using levofloxacin daily for six months to protect contacts following exposure to MDR-TB (181, 182).
For isoniazid and rifamycin therapies, as liver damage and neurotoxicity are the main side effects, conditions such as diabetes mellitus or alcoholism predisposing to neuropathy development, or chronic hepatitis B and C predisposing to liver injuries need to be carefully evaluated. Therefore, especially in patients with rheumatological disorders that often experience a metabolic syndrome (183), at baseline before starting therapy, we need to evaluate fast glycemia, glycated hemoglobin, HBsAg/Ab, hepatitis C virus Ab, and transaminase levels. To evaluate the risk of side effects to preventive therapies based on rifapentine, new strategies based on Whole-Blood Gene Signature have been proposed (184).
In patients treated with biological therapies, few studies are available regarding the side effects of preventive TB therapy (3, 185–187). A moderate and transient increase of isoniazid-induced liver damage has been reported (186, 188). Similarly, in a large Italian study, it was shown that 95% (280/295) of rheumatological patients completed TB preventive therapy with isoniazid and 96% with rifampicin (27/28). Importantly, patients who stopped taking isoniazid due to side effects successfully finished their treatment with rifampicin, showing that switching medications can still provide a good option for completing TB preventive therapy (3, 186, 187).
Although the data available are limited, this evidence suggests that patients undergoing biological therapy generally tolerate preventive treatments and complete the full course.
7 Management of preventive therapy in rheumatological patients
Before initiating preventive therapy, the physician needs to conduct a thorough medical history asking for previous exposure to TB cases and for previous liver disease, alcohol use, and concurrent treatments (to identify potential drug interactions). Patients should be informed about the symptoms of potential liver damage and should also be advised on whom to contact if these symptoms appear.
Blood control intervals of blood count, transaminases, γGT, and bilirubin should be fixed at initially 2-weekly then 4-weekly.
Methotrexate, commonly used for RA and PsA, carries a risk of liver toxicity (189). During TB treatment, it is crucial to balance the risk of arthritis flares with potential liver damage in patients taking methotrexate (190). Although specific guidelines are lacking, switching to less hepatotoxic csDMARDs, such as hydroxychloroquine or sulfasalazine, may be advisable, particularly for patients with stable disease activity. Patients with pre-existing liver conditions require even greater caution due to their increased susceptibility to toxic effects.
If preventive therapy is not tolerated, the rheumatologist should consider prescribing anti-rheumatic treatment with a low risk of TB disease reactivation. This procedure must be written and shared with the patient. Afterward, both the rheumatologist and the patient need to carefully monitor the occurrence of possible symptoms of mycobacterial reactivation to promptly isolate the patient to avoid further transmission, diagnosis, and treatment.
Importantly, after a fully completed therapy for TB disease or TBI, no further TB therapy needs to be given. It is assumed that preventive therapy kills all mycobacteria, and therefore no further preventive TB treatment is needed. IGRA results can remain positive, even after preventive therapy (191, 192), because these tests indicate the presence of an immune response against Mtb, not the presence of Mtb itself (193). Few data are present on the importance of repeated annual TB screening in a non-endemic area (185, 194). Evidence suggests that serial IGRA testing among low-risk patients on DMARDs results in a very low incidence of newly diagnosed TBI. Consequently, it is recommended to conduct targeted TBI screening based on risk factors related to TB —such as geographical origin, comorbidities like diabetes, or travel to endemic areas— before IGRA testing, rather than implementing universal annual screening in non-endemic regions (185, 194).
8 Conclusions
In conclusion, based on the available evidence, patients with chronic autoimmune arthritis under immunosuppressive treatment have an increased risk for TB reactivation. Among bDMARDs, TNF-α inhibitors are associated with an increased risk of TB progression compared to other treatments; however, the risk is not negligible, especially for JAK-i and anti-IL-6R agents.
Based on the WHO recommendations, either skin tests or IGRAs are acceptable for TBI screening. Stratification of TB risk is important to drive the bDMARDs choice. The preventive treatment for TB is well tolerated in patients undergoing b and tsDMARDs.
Author contributions
AP-D: Conceptualization, Writing – original draft, Writing – review & editing. AA: Writing – original draft, Writing – review & editing. CL: Writing – review & editing. GM: Writing – review & editing. DG: Conceptualization, Writing – original draft, Writing – review & editing.
Funding
The author(s) declare that financial support was received for the research, authorship, and/or publication of this article. This study was supported by the Italian Ministry of Health “Ricerca Corrente - Linea 4 - Progetto 2 - INMI L. Spallanzani I.R.C.C.S.” funding.
Conflict of interest
The authors declare that the research was conducted in the absence of any commercial or financial relationships that could be construed as a potential conflict of interest.
The author(s) declared that they were an editorial board member of Frontiers, at the time of submission. This had no impact on the peer review process and the final decision.
Publisher’s note
All claims expressed in this article are solely those of the authors and do not necessarily represent those of their affiliated organizations, or those of the publisher, the editors and the reviewers. Any product that may be evaluated in this article, or claim that may be made by its manufacturer, is not guaranteed or endorsed by the publisher.
References
1. WHO. Global tuberculosis report (2023). Available online at: https://www.who.int/teams/global-tuberculosis-programme/tb-reports/global-tuberculosis-report-2023 (Accessed February 4, 2024).
2. Bai W, Ameyaw EK. Global, regional and national trends in tuberculosis incidence and main risk factors: a study using data from 2000 to 2021. BMC Public Health. (2024) 24:12. doi: 10.1186/s12889-023-17495-6
3. Goletti D, Petrone L, Ippolito G, Niccoli L, Nannini C, Cantini F. Preventive therapy for tuberculosis in rheumatological patients undergoing therapy with biological drugs. Expert Rev Anti Infect Ther. (2018) 16:501–12. doi: 10.1080/14787210.2018.1483238
4. Goletti D, Aiello A, Tientcheu LD, Muefong C, Hu TH, Niewold P, et al. Host–pathogen interactions in the context of tuberculosis infection and disease. In: García-Basteiro AL, Öner Eyüboğlu F, Rangaka MX, eds. The Challenge of Tuberculosis in the 21st Century (ERS Monograph). Sheffield, European Respiratory Society (2023), 34–50. doi: 10.1183/2312508X.10024022
5. Lyon SM, Rossman MD. Pulmonary tuberculosis. Microbiol Spectr. (2017) 5:1–13. doi: 10.1128/microbiolspec.tnmi7-0032-2016
6. Goletti D, Delogu G, Matteelli A, Migliori GB. The role of IGRA in the diagnosis of tuberculosis infection, differentiating from active tuberculosis, and decision making for initiating treatment or preventive therapy of tuberculosis infection. Int J Infect Dis. (2022) 124:S12–9. doi: 10.1016/j.ijid.2022.02.047
7. Drain PK, Bajema KL, Dowdy D, Dheda K, Naidoo K, Schumacher SG, et al. Incipient and subclinical tuberculosis: a clinical review of early stages and progression of infection. Clin Microbiol Rev. (2018) 31:e00021–18. doi: 10.1128/CMR.00021-18
8. Coussens AK, Zaidi SMA, Allwood BW, Dewan PK, Gray G, Kohli M, et al. Classification of early tuberculosis states to guide research for improved care and prevention: an international Delphi consensus exercise. Lancet Respir Med. (2024) 12:484–98. doi: 10.1016/S2213-2600(24)00028-6
9. Migliori GB, Ong CWM, Petrone L, D’Ambrosio L, Centis R, Goletti D. The definition of tuberculosis infection based on the spectrum of tuberculosis disease. Breathe (Sheff). (2021) 17:210079. doi: 10.1183/20734735.0079-2021
10. Aiello A, Najafi-Fard S, Goletti D. Initial immune response after exposure to Mycobacterium tuberculosis or to SARS-COV-2: similarities and differences. Front Immunol. (2023) 14:1244556. doi: 10.3389/fimmu.2023.1244556
11. Schön T, Elmberger G, Negesse Y, Pando RH, Sundqvist T, Britton S. Local production of nitric oxide in patients with tuberculosis. Int J Tuberc Lung Dis. (2004) 8:1134–7.
12. Castillo EF, Dekonenko A, Arko-Mensah J, Mandell MA, Dupont N, Jiang S, et al. Autophagy protects against active tuberculosis by suppressing bacterial burden and inflammation. Proc Natl Acad Sci U.S.A. (2012) 109:E3168–3176. doi: 10.1073/pnas.1210500109
13. Petruccioli E, Romagnoli A, Corazzari M, Coccia EM, Butera O, Delogu G, et al. Specific T cells restore the autophagic flux inhibited by Mycobacterium tuberculosis in human primary macrophages. J Infect Dis. (2012) 205:1425–35. doi: 10.1093/infdis/jis226
14. Romagnoli A, Etna MP, Giacomini E, Pardini M, Remoli ME, Corazzari M, et al. ESX-1 dependent impairment of autophagic flux by Mycobacterium tuberculosis in human dendritic cells. Autophagy. (2012) 8:1357–70. doi: 10.4161/auto.20881
15. Romagnoli A, Petruccioli E, Palucci I, Camassa S, Carata E, Petrone L, et al. Clinical isolates of the modern Mycobacterium tuberculosis lineage 4 evade host defense in human macrophages through eluding IL-1β-induced autophagy. Cell Death Dis. (2018) 9:624. doi: 10.1038/s41419-018-0640-8
16. McCaffrey EF, Donato M, Keren L, Chen Z, Delmastro A, Fitzpatrick MB, et al. The immunoregulatory landscape of human tuberculosis granulomas. Nat Immunol. (2022) 23:318–29. doi: 10.1038/s41590-021-01121-x
17. Lin PL, Ford CB, Coleman MT, Myers AJ, Gawande R, Ioerger T, et al. Sterilization of granulomas is common in active and latent tuberculosis despite within-host variability in bacterial killing. Nat Med. (2014) 20:75–9. doi: 10.1038/nm.3412
18. Peddireddy V, Doddam SN, Ahmed N. Mycobacterial dormancy systems and host responses in tuberculosis. Front Immunol. (2017) 8:84. doi: 10.3389/fimmu.2017.00084
19. Gideon HP, Phuah J, Myers AJ, Bryson BD, Rodgers MA, Coleman MT, et al. Variability in tuberculosis granuloma T cell responses exists, but a balance of pro- and anti-inflammatory cytokines is associated with sterilization. PloS Pathog. (2015) 11:e1004603. doi: 10.1371/journal.ppat.1004603
20. Kaiko GE, Horvat JC, Beagley KW, Hansbro PM. Immunological decision-making: how does the immune system decide to mount a helper T-cell response? Immunology. (2008) 123:326–38. doi: 10.1111/j.1365-2567.2007.02719.x
21. Lu Y-J, Barreira-Silva P, Boyce S, Powers J, Cavallo K, Behar SM. CD4 T cell help prevents CD8 T cell exhaustion and promotes control of Mycobacterium tuberculosis infection. Cell Rep. (2021) 36:109696. doi: 10.1016/j.celrep.2021.109696
22. Ogongo P, Tezera LB, Ardain A, Nhamoyebonde S, Ramsuran D, Singh A, et al. Tissue-resident-like CD4+ T cells secreting IL-17 control Mycobacterium tuberculosis in the human lung. J Clin Invest. (2021) 131:e142014, 142014. doi: 10.1172/JCI142014
23. Rapolu BL, Pullagurla A, Ganta S, Komaravalli PL, Gaddam SL. Immuno-genetic importance of Th17 in susceptibility to TB. Scandinavian J Immunol. (2021) 94:e13085. doi: 10.1111/sji.13085
24. Ardain A, Domingo-Gonzalez R, Das S, Kazer SW, Howard NC, Singh A, et al. Group 3 innate lymphoid cells mediate early protective immunity against tuberculosis. Nature. (2019) 570:528–32. doi: 10.1038/s41586-019-1276-2
25. Pollara G, Turner CT, Rosenheim J, Chandran A, Bell LCK, Khan A, et al. Exaggerated IL-17A activity in human in vivo recall responses discriminates active tuberculosis from latent infection and cured disease. Sci Transl Med. (2021) 13:eabg7673. doi: 10.1126/scitranslmed.abg7673
26. Cruz A, Khader SA, Torrado E, Fraga A, Pearl JE, Pedrosa J, et al. Cutting edge: IFN-gamma regulates the induction and expansion of IL-17-producing CD4 T cells during mycobacterial infection. J Immunol. (2006) 177:1416–20. doi: 10.4049/jimmunol.177.3.1416
27. Brighenti S, Ordway DJ. Regulation of immunity to tuberculosis. Microbiol Spectr. (2016) 4:1–21. doi: 10.1128/microbiolspec.tbtb2-0006-2016
28. Squire J, Libertin C, Powers H, Nelson J, Brumble L, Laham F, et al. Disseminated mycobacterial infections following TNF inhibitor use revealing inborn errors of immunity. Int J Infect Dis. (2023) 131:162–5. doi: 10.1016/j.ijid.2023.04.007
29. Cantini F, Niccoli L, Goletti D. Tuberculosis risk in patients treated with non-anti-tumor necrosis factor-α (TNF-α) targeted biologics and recently licensed TNF-α inhibitors: data from clinical trials and national registries. J Rheumatol Suppl. (2014) 91:56–64. doi: 10.3899/jrheum.140103
30. da Silva DAA, da Silva MV, Barros CCO, Alexandre PBD, Timóteo RP, Catarino JS, et al. TNF-α blockade impairs in vitro tuberculous granuloma formation and down modulate Th1, Th17 and Treg cytokines. PloS One. (2018) 13:e0194430. doi: 10.1371/journal.pone.0194430
31. Dorman SE, Picard C, Lammas D, Heyne K, van Dissel JT, Baretto R, et al. Clinical features of dominant and recessive interferon gamma receptor 1 deficiencies. Lancet. (2004) 364:2113–21. doi: 10.1016/S0140-6736(04)17552-1
32. Ramirez-Alejo N, Santos-Argumedo L. Innate defects of the IL-12/IFN-γ Axis in susceptibility to infections by mycobacteria and salmonella. J Interferon Cytokine Res. (2014) 34:307–17. doi: 10.1089/jir.2013.0050
33. Boisson-Dupuis S, Bustamante J. Mycobacterial diseases in patients with inborn errors of immunity. Curr Opin Immunol. (2021) 72:262–71. doi: 10.1016/j.coi.2021.07.001
34. Goletti D, Pisapia R, Fusco FM, Aiello A, Van Crevel R. Epidemiology, pathogenesis, clinical presentation and management of TB in patients with HIV and diabetes. Int J Tuberc Lung Dis. (2023) 27:284–90. doi: 10.5588/ijtld.22.0685
35. Boisson-Dupuis S, Bustamante J, El-Baghdadi J, Camcioglu Y, Parvaneh N, Azbaoui SE, et al. Inherited and acquired immunodeficiencies underlying tuberculosis in childhood. Immunol Rev. (2015) 264:103–20. doi: 10.1111/imr.12272
36. Filipe-Santos O, Bustamante J, Haverkamp MH, Vinolo E, Ku C-L, Puel A, et al. X-linked susceptibility to mycobacteria is caused by mutations in NEMO impairing CD40-dependent IL-12 production. J Exp Med. (2006) 203:1745–59. doi: 10.1084/jem.20060085
37. Chen Y-H, Spencer S, Laurence A, Thaventhiran JE, Uhlig HH. Inborn errors of IL-6 family cytokine responses. Curr Opin Immunol. (2021) 72:135–45. doi: 10.1016/j.coi.2021.04.007
38. Minegishi Y, Saito M, Morio T, Watanabe K, Agematsu K, Tsuchiya S, et al. Human tyrosine kinase 2 deficiency reveals its requisite roles in multiple cytokine signals involved in innate and acquired immunity. Immunity. (2006) 25:745–55. doi: 10.1016/j.immuni.2006.09.009
39. Arias AA, Neehus A-L, Ogishi M, Meynier V, Krebs A, Lazarov T, et al. Tuberculosis in otherwise healthy adults with inherited TNF deficiency. Nature. (2024) 633:417–25. doi: 10.1038/s41586-024-07866-3
40. Glatman-Freedman A. The role of antibody-mediated immunity in defense against Mycobacterium tuberculosis: advances toward a novel vaccine strategy. Tuberculosis (Edinb). (2006) 86:191–7. doi: 10.1016/j.tube.2006.01.008
41. Glatman-Freedman A, Casadevall A. Serum therapy for tuberculosis revisited: reappraisal of the role of antibody-mediated immunity against Mycobacterium tuberculosis. Clin Microbiol Rev. (1998) 11:514–32. doi: 10.1128/CMR.11.3.514
42. Rijnink WF, Ottenhoff THM, Joosten SA. B-cells and antibodies as contributors to effector immune responses in tuberculosis. Front Immunol. (2021) 12:640168. doi: 10.3389/fimmu.2021.640168
43. Kozakiewicz L, Phuah J, Flynn J, Chan J. The role of B cells and humoral immunity in Mycobacterium tuberculosis infection. Adv Exp Med Biol. (2013) 783:225–50. doi: 10.1007/978-1-4614-6111-1_12
44. Chan J, Mehta S, Bharrhan S, Chen Y, Achkar JM, Casadevall A, et al. The role of B cells and humoral immunity in Mycobacterium tuberculosis infection. Semin Immunol. (2014) 26:588–600. doi: 10.1016/j.smim.2014.10.005
45. Roy Chowdhury R, Vallania F, Yang Q, Lopez Angel CJ, Darboe F, Penn-Nicholson A, et al. A multi-cohort study of the immune factors associated with M. tuberculosis infection outcomes. Nature. (2018) 560:644–8. doi: 10.1038/s41586-018-0439-x
46. Joosten SA, van Meijgaarden KE, Del Nonno F, Baiocchini A, Petrone L, Vanini V, et al. Patients with tuberculosis have a dysfunctional circulating B-cell compartment, which normalizes following successful treatment. PloS Pathog. (2016) 12:e1005687. doi: 10.1371/journal.ppat.1005687
47. Mehta B, Pedro S, Ozen G, Kalil A, Wolfe F, Mikuls T, et al. Serious infection risk in rheumatoid arthritis compared with non-inflammatory rheumatic and musculoskeletal diseases: a US national cohort study. RMD Open. (2019) 5:e000935. doi: 10.1136/rmdopen-2019-000935
48. Watanabe A, Matsumoto T, Igari H, Sawa J, Yamaguchi Y, Sakatani M. Risk of developing active tuberculosis in rheumatoid arthritis patients on adalimumab in Japan. Int J Tuberc Lung Dis. (2016) 20:101–8. doi: 10.5588/ijtld.15.0283
49. Jick SS, Lieberman ES, Rahman MU, Choi HK. Glucocorticoid use, other associated factors, and the risk of tuberculosis. Arthritis Rheum. (2006) 55:19–26. doi: 10.1002/art.21705
50. Brassard P, Lowe A-M, Bernatsky S, Kezouh A, Suissa S. Rheumatoid arthritis, its treatments, and the risk of tuberculosis in Quebec, Canada. Arthritis Rheum. (2009) 61:300–4. doi: 10.1002/art.24476
51. Sundbaum JK, Arkema EV, Bruchfeld J, Jonsson J, Askling J, Baecklund E. Tuberculosis in biologic-naïve patients with rheumatoid arthritis: risk factors and tuberculosis characteristics. J Rheumatol. (2021) 48:1243–50. doi: 10.3899/jrheum.201251
52. Zhang Z, Fan W, Yang G, Xu Z, Wang J, Cheng Q, et al. Risk of tuberculosis in patients treated with TNF-α antagonists: a systematic review and meta-analysis of randomised controlled trials. BMJ Open. (2017) 7:e012567. doi: 10.1136/bmjopen-2016-012567
53. Cantini F, Niccoli L, Goletti D. Adalimumab, etanercept, infliximab, and the risk of tuberculosis: data from clinical trials, national registries, and postmarketing surveillance. J Rheumatol Suppl. (2014) 91:47–55. doi: 10.3899/jrheum.140102
54. Baronnet L, Barnetche T, Kahn V, Lacoin C, Richez C, Schaeverbeke T. Incidence of tuberculosis in patients with rheumatoid arthritis. A systematic literature review. Joint Bone Spine. (2011) 78:279–84. doi: 10.1016/j.jbspin.2010.12.004
55. Fragoulis GE, Dey M, Zhao S, Schoones J, Courvoisier D, Galloway J, et al. Systematic literature review informing the 2022 EULAR recommendations for screening and prophylaxis of chronic and opportunistic infections in adults with autoimmune inflammatory rheumatic diseases. RMD Open. (2022) 8:e002726. doi: 10.1136/rmdopen-2022-002726
56. Dobler CC. Biologic agents and tuberculosis. Microbiol Spectr. (2016) 4:1–12. doi: 10.1128/microbiolspec.TNMI7-0026-2016
57. Evangelatos G, Koulouri V, Iliopoulos A, Fragoulis GE. Tuberculosis and targeted synthetic or biologic DMARDs, beyond tumor necrosis factor inhibitors. Ther Adv Musculoskelet Dis. (2020) 12:1759720X20930116. doi: 10.1177/1759720X20930116
58. Rath E, Bonelli M, Duftner C, Gruber J, Mandl P, Moazedi-Furst F, et al. National consensus statement by the Austrian Societies for Rheumatology, Pulmonology, Infectiology, Dermatology and Gastroenterology regarding the management of latent tuberculosis and the associated utilization of biologic and targeted synthetic disease modifying antirheumatic drugs (DMARDs). Wien Klin Wochenschr. (2022) 134:751–65. doi: 10.1007/s00508-022-02062-7
59. Bradley J. TNF-mediated inflammatory disease. J Pathol. (2008) 214:149–60. doi: 10.1002/path.2287
60. Blandizzi C, Gionchetti P, Armuzzi A, Caporali R, Chimenti S, Cimaz R, et al. The role of tumour necrosis factor in the pathogenesis of immune-mediated diseases. Int J Immunopathol Pharmacol. (2014) 27:1–10. doi: 10.1177/03946320140270S101
61. Mitoma H, Horiuchi T, Tsukamoto H, Ueda N. Molecular mechanisms of action of anti-TNF-α agents - Comparison among therapeutic TNF-α antagonists. Cytokine. (2018) 101:56–63. doi: 10.1016/j.cyto.2016.08.014
62. Kaymakcalan Z, Sakorafas P, Bose S, Scesney S, Xiong L, Hanzatian DK, et al. Comparisons of affinities, avidities, and complement activation of adalimumab, infliximab, and etanercept in binding to soluble and membrane tumor necrosis factor. Clin Immunol. (2009) 131:308–16. doi: 10.1016/j.clim.2009.01.002
63. Scallon B, Cai A, Solowski N, Rosenberg A, Song X-Y, Shealy D, et al. Binding and functional comparisons of two types of tumor necrosis factor antagonists. J Pharmacol Exp Ther. (2002) 301:418–26. doi: 10.1124/jpet.301.2.418
64. Keane J, Gershon S, Wise RP, Mirabile-Levens E, Kasznica J, Schwieterman WD, et al. Tuberculosis associated with infliximab, a tumor necrosis factor alpha-neutralizing agent. N Engl J Med. (2001) 345:1098–104. doi: 10.1056/NEJMoa011110
65. Mohan AK, Coté TR, Block JA, Manadan AM, Siegel JN, Braun MM. Tuberculosis following the use of etanercept, a tumor necrosis factor inhibitor. Clin Infect Dis. (2004) 39:295–9. doi: 10.1086/421494
66. Antoni CE, Kavanaugh A, Kirkham B, Tutuncu Z, Burmester GR, Schneider U, et al. Sustained benefits of infliximab therapy for dermatologic and articular manifestations of psoriatic arthritis: results from the infliximab multinational psoriatic arthritis controlled trial (IMPACT). Arthritis Rheum. (2005) 52:1227–36. doi: 10.1002/art.20967
67. Baranauskaite A, Raffayová H, Kungurov NV, Kubanova A, Venalis A, Helmle L, et al. Infliximab plus methotrexate is superior to methotrexate alone in the treatment of psoriatic arthritis in methotrexate-naive patients: the RESPOND study. Ann Rheum Dis. (2012) 71:541–8. doi: 10.1136/ard.2011.152223
68. St Clair EW, van der Heijde DMFM, Smolen JS, Maini RN, Bathon JM, Emery P, et al. Combination of infliximab and methotrexate therapy for early rheumatoid arthritis: a randomized, controlled trial. Arthritis Rheum. (2004) 50:3432–43. doi: 10.1002/art.20568
69. Maini RN, Breedveld FC, Kalden JR, Smolen JS, Furst D, Weisman MH, et al. Sustained improvement over two years in physical function, structural damage, and signs and symptoms among patients with rheumatoid arthritis treated with infliximab and methotrexate. Arthritis Rheum. (2004) 50:1051–65. doi: 10.1002/art.20159
70. Westhovens R, Yocum D, Han J, Berman A, Strusberg I, Geusens P, et al. The safety of infliximab, combined with background treatments, among patients with rheumatoid arthritis and various comorbidities: a large, randomized, placebo-controlled trial. Arthritis Rheum. (2006) 54:1075–86. doi: 10.1002/art.21734
71. Keystone EC, Kavanaugh AF, Sharp JT, Tannenbaum H, Hua Y, Teoh LS, et al. Radiographic, clinical, and functional outcomes of treatment with adalimumab (a human anti-tumor necrosis factor monoclonal antibody) in patients with active rheumatoid arthritis receiving concomitant methotrexate therapy: a randomized, placebo-controlled, 52-week trial. Arthritis Rheum. (2004) 50:1400–11. doi: 10.1002/art.20217
72. Breedveld FC, Weisman MH, Kavanaugh AF, Cohen SB, Pavelka K, van Vollenhoven R, et al. The PREMIER study: A multicenter, randomized, double-blind clinical trial of combination therapy with adalimumab plus methotrexate versus methotrexate alone or adalimumab alone in patients with early, aggressive rheumatoid arthritis who had not had previous methotrexate treatment. Arthritis Rheum. (2006) 54:26–37. doi: 10.1002/art.21519
73. van der Heijde D, Breedveld FC, Kavanaugh A, Keystone EC, Landewé R, Patra K, et al. Disease activity, physical function, and radiographic progression after longterm therapy with adalimumab plus methotrexate: 5-year results of PREMIER. J Rheumatol. (2010) 37:2237–46. doi: 10.3899/jrheum.100208
74. Keystone EC, Kavanaugh A, Weinblatt ME, Patra K, Pangan AL. Clinical consequences of delayed addition of adalimumab to methotrexate therapy over 5 years in patients with rheumatoid arthritis. J Rheumatol. (2011) 38:855–62. doi: 10.3899/jrheum.100752
75. Mease PJ, Ory P, Sharp JT, Ritchlin CT, Van den Bosch F, Wellborne F, et al. Adalimumab for long-term treatment of psoriatic arthritis: 2-year data from the Adalimumab Effectiveness in Psoriatic Arthritis Trial (ADEPT). Ann Rheum Dis. (2009) 68:702–9. doi: 10.1136/ard.2008.092767
76. Huang F, Gu J, Zhu P, Bao C, Xu J, Xu H, et al. Efficacy and safety of adalimumab in Chinese adults with active ankylosing spondylitis: results of a randomised, controlled trial. Ann Rheum Dis. (2014) 73:587–94. doi: 10.1136/annrheumdis-2012-202533
77. Smolen JS, Nash P, Durez P, Hall S, Ilivanova E, Irazoque-Palazuelos F, et al. Maintenance, reduction, or withdrawal of etanercept after treatment with etanercept and methotrexate in patients with moderate rheumatoid arthritis (PRESERVE): a randomised controlled trial. Lancet. (2013) 381:918–29. doi: 10.1016/S0140-6736(12)61811-X
78. Klareskog L, Gaubitz M, Rodriguez-Valverde V, Malaise M, Dougados M, Wajdula J, et al. A long-term, open-label trial of the safety and efficacy of etanercept (Enbrel) in patients with rheumatoid arthritis not treated with other disease-modifying antirheumatic drugs. Ann Rheum Dis. (2006) 65:1578–84. doi: 10.1136/ard.2005.038349
79. Dougados M, Braun J, Szanto S, Combe B, Geher P, Leblanc V, et al. Continuous efficacy of etanercept in severe and advanced ankylosing spondylitis: results from a 12-week open-label extension of the SPINE study. Rheumatol (Oxford). (2012) 51:1687–96. doi: 10.1093/rheumatology/kes125
80. Cantini F, Goletti D. Biologics and tuberculosis risk: the rise and fall of an old disease and its new resurgence. J Rheumatol Suppl. (2014) 91:1–3. doi: 10.3899/jrheum.140095
81. Hsia EC, Cush JJ, Matteson EL, Beutler A, Doyle MK, Hsu B, et al. Comprehensive tuberculosis screening program in patients with inflammatory arthritides treated with golimumab, a human anti-tumor necrosis factor antibody, in Phase III clinical trials. Arthritis Care Res (Hoboken). (2013) 65:309–13. doi: 10.1002/acr.21788
82. Bykerk VP, Cush J, Winthrop K, Calabrese L, Lortholary O, de Longueville M, et al. Update on the safety profile of certolizumab pegol in rheumatoid arthritis: an integrated analysis from clinical trials. Ann Rheum Dis. (2015) 74:96–103. doi: 10.1136/annrheumdis-2013-203660
83. van der Heijde D, Dougados M, Landewé R, Sieper J, Maksymowych WP, Rudwaleit M, et al. Sustained efficacy, safety and patient-reported outcomes of certolizumab pegol in axial spondyloarthritis: 4-year outcomes from RAPID-axSpA. Rheumatol (Oxford). (2017) 56:1498–509. doi: 10.1093/rheumatology/kex174
84. Mease PJ, Fleischmann R, Deodhar AA, Wollenhaupt J, Khraishi M, Kielar D, et al. Effect of certolizumab pegol on signs and symptoms in patients with psoriatic arthritis: 24-week results of a Phase 3 double-blind randomised placebo-controlled study (RAPID-PsA). Ann Rheum Dis. (2014) 73:48–55. doi: 10.1136/annrheumdis-2013-203696
85. Gómez-Reino JJ, Carmona L, Valverde VR, Mola EM, Montero MD, BIOBADASER Group. Treatment of rheumatoid arthritis with tumor necrosis factor inhibitors may predispose to significant increase in tuberculosis risk: a multicenter active-surveillance report. Arthritis Rheum. (2003) 48:2122–7. doi: 10.1002/art.11137
86. Askling J, Fored CM, Brandt L, Baecklund E, Bertilsson L, Cöster L, et al. Risk and case characteristics of tuberculosis in rheumatoid arthritis associated with tumor necrosis factor antagonists in Sweden. Arthritis Rheum. (2005) 52:1986–92. doi: 10.1002/art.21137
87. Listing J, Strangfeld A, Kary S, Rau R, von Hinueber U, Stoyanova-Scholz M, et al. Infections in patients with rheumatoid arthritis treated with biologic agents. Arthritis Rheum. (2005) 52:3403–12. doi: 10.1002/art.21386
88. Ai J-W, Zhang S, Ruan Q-L, Yu Y-Q, Zhang B-Y, Liu Q-H, et al. The risk of tuberculosis in patients with rheumatoid arthritis treated with tumor necrosis factor-α Antagonist: A metaanalysis of both randomized controlled trials and registry/cohort studies. J Rheumatol. (2015) 42:2229–37. doi: 10.3899/jrheum.150057
89. Linge I, Tsareva A, Kondratieva E, Dyatlov A, Hidalgo J, Zvartsev R, et al. Pleiotropic effect of IL-6 produced by B-lymphocytes during early phases of adaptive immune responses against TB infection. Front Immunol. (2022) 13:750068. doi: 10.3389/fimmu.2022.750068
90. Buha I, Škodrić-Trifunović V, Adžić-Vukičević T, Ilić A, Blanka-Protić A, Stjepanovic M, et al. Relevance of TNF-α, IL-6 and IRAK1 gene expression for assessing disease severity and therapy effects in tuberculosis patients. J Infect Dev Ctries. (2019) 13:419–25. doi: 10.3855/jidc.10949
91. Appelberg R, Castro AG, Pedrosa J, Minóprio P. Role of interleukin-6 in the induction of protective T cells during mycobacterial infections in mice. Immunology. (1994) 82:361–4.
92. Saunders BM, Frank AA, Orme IM, Cooper AM. Interleukin-6 induces early gamma interferon production in the infected lung but is not required for generation of specific immunity to Mycobacterium tuberculosis infection. Infect Immun. (2000) 68:3322–6. doi: 10.1128/IAI.68.6.3322-3326.2000
93. Souto A, Maneiro JR, Salgado E, Carmona L, Gomez-Reino JJ. Risk of tuberculosis in patients with chronic immune-mediated inflammatory diseases treated with biologics and tofacitinib: a systematic review and meta-analysis of randomized controlled trials and long-term extension studies. Rheumatol (Oxford). (2014) 53:1872–85. doi: 10.1093/rheumatology/keu172
94. Rutherford AI, Subesinghe S, Hyrich KL, Galloway JB. Serious infection across biologic-treated patients with rheumatoid arthritis: results from the British Society for Rheumatology Biologics Register for Rheumatoid Arthritis. Ann Rheum Dis. (2018) 77:905–10. doi: 10.1136/annrheumdis-2017-212825
95. Takeuchi T, Kameda H. The Japanese experience with biologic therapies for rheumatoid arthritis. Nat Rev Rheumatol. (2010) 6:644–52. doi: 10.1038/nrrheum.2010.154
96. Jung SM, Han M, Kim EH, Jung I, Park Y-B. Comparison of developing tuberculosis following tumor necrosis factor inhibition and interleukin-6 inhibition in patients with rheumatoid arthritis: a nationwide observational study in South Korea, 2013-2018. Arthritis Res Ther. (2022) 24:157. doi: 10.1186/s13075-022-02842-6
97. Segueni N, Tritto E, Bourigault M-L, Rose S, Erard F, Le Bert M, et al. Controlled Mycobacterium tuberculosis infection in mice under treatment with anti-IL-17A or IL-17F antibodies, in contrast to TNFα neutralization. Sci Rep. (2016) 6:36923. doi: 10.1038/srep36923
98. Cooper AM, Khader SA. The role of cytokines in the initiation, expansion, and control of cellular immunity to tuberculosis. Immunol Rev. (2008) 226:191–204. doi: 10.1111/j.1600-065X.2008.00702.x
99. Khader SA, Cooper AM. IL-23 and IL-17 in tuberculosis. Cytokine. (2008) 41:79–83. doi: 10.1016/j.cyto.2007.11.022
100. Elewski BE, Baddley JW, Deodhar AA, Magrey M, Rich PA, Soriano ER, et al. Association of secukinumab treatment with tuberculosis reactivation in patients with psoriasis, psoriatic arthritis, or ankylosing spondylitis. JAMA Dermatol. (2021) 157:43–51. doi: 10.1001/jamadermatol.2020.3257
101. Gottlieb AB, Deodhar A, Mcinnes IB, Baraliakos X, Reich K, Schreiber S, et al. Long-term safety of secukinumab over five years in patients with moderate-to-severe plaque psoriasis, psoriatic arthritis and ankylosing spondylitis: update on integrated pooled clinical trial and post-marketing surveillance data. Acta Derm Venereol. (2022) 102:adv00698. doi: 10.2340/actadv.v102.563
102. Liu S, He Z, Wu W, Jin H, Cui Y. Safety of secukinumab in the treatment of patients with axial spondyloarthritis and concurrent hepatitis B virus infection or latent tuberculosis infection. Clin Rheumatol. (2023) 42:2369–76. doi: 10.1007/s10067-023-06630-8
103. Ngoc CT, Khoa BD, Nguyen HK, Le LB, Hiep DV, Duc NM. Active pulmonary tuberculosis in a patient with secukinumab treatment. Radiol Case Rep. (2023) 18:239–42. doi: 10.1016/j.radcr.2022.10.032
104. Mease P, Roussou E, Burmester G-R, Goupille P, Gottlieb A, Moriarty SR, et al. Safety of ixekizumab in patients with psoriatic arthritis: results from a pooled analysis of three clinical trials. Arthritis Care Res (Hoboken). (2019) 71:367–78. doi: 10.1002/acr.23738
105. Coates LC, Landewé R, McInnes IB, Mease PJ, Ritchlin CT, Tanaka Y, et al. Bimekizumab treatment in patients with active psoriatic arthritis and prior inadequate response to tumour necrosis factor inhibitors: 52-week safety and efficacy from the phase III BE COMPLETE study and its open-label extension BE VITAL. RMD Open. (2024) 10:e003855. doi: 10.1136/rmdopen-2023-003855
106. van der Heijde D, Deodhar A, Baraliakos X, Brown MA, Dobashi H, Dougados M, et al. Efficacy and safety of bimekizumab in axial spondyloarthritis: results of two parallel phase 3 randomised controlled trials. Ann Rheum Dis. (2023) 82:515–26. doi: 10.1136/ard-2022-223595
107. Baraliakos X, Deodhar A, Dougados M, Gensler LS, Molto A, Ramiro S, et al. Safety and efficacy of bimekizumab in patients with active ankylosing spondylitis: three-year results from a phase IIb randomized controlled trial and its open-label extension study. Arthritis Rheumatol. (2022) 74:1943–58. doi: 10.1002/art.42282
108. Johnsson HJ, McInnes IB. Interleukin-12 and interleukin-23 inhibition in psoriatic arthritis. Clin Exp Rheumatol. (2015) 33:S115–118.
109. McInnes IB, Kavanaugh A, Gottlieb AB, Puig L, Rahman P, Ritchlin C, et al. Efficacy and safety of ustekinumab in patients with active psoriatic arthritis: 1 year results of the phase 3, multicentre, double-blind, placebo-controlled PSUMMIT 1 trial. Lancet. (2013) 382:780–9. doi: 10.1016/S0140-6736(13)60594-2
110. Ritchlin C, Rahman P, Kavanaugh A, McInnes IB, Puig L, Li S, et al. Efficacy and safety of the anti-IL-12/23 p40 monoclonal antibody, ustekinumab, in patients with active psoriatic arthritis despite conventional non-biological and biological anti-tumour necrosis factor therapy: 6-month and 1-year results of the phase 3, multicentre, double-blind, placebo-controlled, randomised PSUMMIT 2 trial. Ann Rheum Dis. (2014) 73:990–9. doi: 10.1136/annrheumdis-2013-204655
111. Gossec L, Siebert S, Bergmans P, de Vlam K, Gremese E, Joven-Ibáñez B, et al. Long-term effectiveness and persistence of ustekinumab and TNF inhibitors in patients with psoriatic arthritis: final 3-year results from the PsABio real-world study. Ann Rheum Dis. (2023) 82:496–506. doi: 10.1136/ard-2022-222879
112. Lynch M, Roche L, Horgan M, Ahmad K, Hackett C, Ramsay B. Peritoneal tuberculosis in the setting of ustekinumab treatment for psoriasis. JAAD Case Rep. (2017) 3:230–2. doi: 10.1016/j.jdcr.2017.02.001
113. Deodhar A, Helliwell PS, Boehncke W-H, Kollmeier AP, Hsia EC, Subramanian RA, et al. Guselkumab in patients with active psoriatic arthritis who were biologic-naive or had previously received TNFα inhibitor treatment (DISCOVER-1): a double-blind, randomised, placebo-controlled phase 3 trial. Lancet. (2020) 395:1115–25. doi: 10.1016/S0140-6736(20)30265-8
114. Mease PJ, Rahman P, Gottlieb AB, Kollmeier AP, Hsia EC, Xu XL, et al. Guselkumab in biologic-naive patients with active psoriatic arthritis (DISCOVER-2): a double-blind, randomised, placebo-controlled phase 3 trial. Lancet. (2020) 395:1126–36. doi: 10.1016/S0140-6736(20)30263-4
115. Kristensen LE, Keiserman M, Papp K, McCasland L, White D, Lu W, et al. Efficacy and safety of risankizumab for active psoriatic arthritis: 52-week results from the KEEPsAKE 1 study. Rheumatol (Oxford). (2023) 62:2113–21. doi: 10.1093/rheumatology/keac607
116. Östör A, Van den Bosch F, Papp K, Asnal C, Blanco R, Aelion J, et al. Efficacy and safety of risankizumab for active psoriatic arthritis: 100-week results from the KEEPsAKE 2 randomized clinical trial. Rheumatol Ther. (2024) 11:633–48. doi: 10.1007/s40744-024-00657-2
117. Takeda K, Yanagitani N. Guselkumab for treating immune checkpoint inhibitor-induced psoriatic arthritis. Ann Rheum Dis. (2022) 81(10):annrheumdis–2022-222628. doi: 10.1136/annrheumdis-2022-222628
118. Manzanares N, Vilarrasa E, López A, Alonso ML, Velasco M, Riera J, et al. No tuberculosis reactivations in psoriasis patients initiating new generation biologics despite untreated latent tuberculosis infection: Multicenter case series of 35 patients. J Eur Acad Dermatol Venereol. (2024) 38:e26–8. doi: 10.1111/jdv.19406
119. Mastorino L, Dapavo P, Trunfio M, Avallone G, Rubatto M, Calcagno A, et al. Risk of reactivation of latent tuberculosis in psoriasis patients on biologic therapies: A retrospective cohort from a tertiary care centre in northern Italy. Acta Derm Venereol. (2022) 102:adv00821. doi: 10.2340/actadv.v102.1982
120. Torres T, Chiricozzi A, Puig L, Lé AM, Marzano AV, Dapavo P, et al. Treatment of psoriasis patients with latent tuberculosis using IL-17 and IL-23 inhibitors: A retrospective, multinational, multicentre study. Am J Clin Dermatol. (2024) 25:333–42. doi: 10.1007/s40257-024-00845-4
121. Kearney N, Byrne N, Kirby B, Hughes R. Successful use of guselkumab in the treatment of severe hidradenitis suppurativa. Clin Exp Dermatol. (2020) 45:618–9. doi: 10.1111/ced.14199
122. He C-X, Wu C, Zhang L, Jin H-Z. Interleukin-17A inhibitors in patients with psoriasis and tuberculosis infection: A 2-year prospective study on safety without preventive treatment. Dermatol Ther (Heidelb). (2024) 14:893–906. doi: 10.1007/s13555-024-01130-2
123. Edwards JCW, Szczepanski L, Szechinski J, Filipowicz-Sosnowska A, Emery P, Close DR, et al. Efficacy of B-cell-targeted therapy with rituximab in patients with rheumatoid arthritis. N Engl J Med. (2004) 350:2572–81. doi: 10.1056/NEJMoa032534
124. Silverman GJ, Weisman S. Rituximab therapy and autoimmune disorders: prospects for anti-B cell therapy. Arthritis Rheum. (2003) 48:1484–92. doi: 10.1002/art.10947
125. van Vollenhoven RF, Emery P, Bingham CO, Keystone EC, Fleischmann R, Furst DE, et al. Longterm safety of patients receiving rituximab in rheumatoid arthritis clinical trials. J Rheumatol. (2010) 37:558–67. doi: 10.3899/jrheum.090856
126. Keystone EC, Cohen SB, Emery P, Kremer JM, Dougados M, Loveless JE, et al. Multiple courses of rituximab produce sustained clinical and radiographic efficacy and safety in patients with rheumatoid arthritis and an inadequate response to 1 or more tumor necrosis factor inhibitors: 5-year data from the REFLEX study. J Rheumatol. (2012) 39:2238–46. doi: 10.3899/jrheum.120573
127. Rutherford AI, Patarata E, Subesinghe S, Hyrich KL, Galloway JB. Opportunistic infections in rheumatoid arthritis patients exposed to biologic therapy: results from the British Society for Rheumatology Biologics Register for Rheumatoid Arthritis. Rheumatol (Oxford). (2018) 57:997–1001. doi: 10.1093/rheumatology/key023
128. van Vollenhoven RF, Emery P, Bingham CO, Keystone EC, Fleischmann RM, Furst DE, et al. Long-term safety of rituximab in rheumatoid arthritis: 9.5-year follow-up of the global clinical trial programme with a focus on adverse events of interest in RA patients. Ann Rheum Dis. (2013) 72:1496–502. doi: 10.1136/annrheumdis-2012-201956
129. Nisar MK, Rafiq A, Östör AJK. Biologic therapy for inflammatory arthritis and latent tuberculosis: real world experience from a high prevalence area in the United Kingdom. Clin Rheumatol. (2015) 34:2141–5. doi: 10.1007/s10067-015-3099-3
130. Tarkiainen M, Tynjälä P, Vähäsalo P, Lahdenne P. Occurrence of adverse events in patients with JIA receiving biologic agents: long-term follow-up in a real-life setting. Rheumatol (Oxford). (2015) 54:1170–6. doi: 10.1093/rheumatology/keu457
131. Gottenberg J-E, Ravaud P, Bardin T, Cacoub P, Cantagrel A, Combe B, et al. Risk factors for severe infections in patients with rheumatoid arthritis treated with rituximab in the autoimmunity and rituximab registry. Arthritis Rheum. (2010) 62:2625–32. doi: 10.1002/art.27555
132. Tony H-P, Burmester G, Schulze-Koops H, Grunke M, Henes J, Kötter I, et al. Safety and clinical outcomes of rituximab therapy in patients with different autoimmune diseases: experience from a national registry (GRAID). Arthritis Res Ther. (2011) 13:R75. doi: 10.1186/ar3337
133. Wendler J, Burmester GR, Sörensen H, Krause A, Richter C, Tony H-P, et al. Rituximab in patients with rheumatoid arthritis in routine practice (GERINIS): six-year results from a prospective, multicentre, non-interventional study in 2,484 patients. Arthritis Res Ther. (2014) 16:R80. doi: 10.1186/ar4521
134. Picchianti Diamanti A, Rosado MM, Scarsella M, Germano V, Giorda E, Cascioli S, et al. Abatacept (cytotoxic T lymphocyte antigen 4-immunoglobulin) improves B cell function and regulatory T cell inhibitory capacity in rheumatoid arthritis patients non-responding to anti-tumour necrosis factor-α agents. Clin Exp Immunol. (2014) 177:630–40. doi: 10.1111/cei.12367
135. Weinblatt ME, Moreland LW, Westhovens R, Cohen RB, Kelly SM, Khan N, et al. Safety of abatacept administered intravenously in treatment of rheumatoid arthritis: integrated analyses of up to 8 years of treatment from the abatacept clinical trial program. J Rheumatol. (2013) 40:787–97. doi: 10.3899/jrheum.120906
136. Simon TA, Suissa S, Skovron ML, Frisell T, Askling J, Michaud K, et al. Infection outcomes in patients with rheumatoid arthritis treated with abatacept and other disease-modifying antirheumatic drugs: Results from a 10-year international post-marketing study. Semin Arthritis Rheum. (2024) 64:152313. doi: 10.1016/j.semarthrit.2023.152313
137. Cantini F, Niccoli L, Capone A, Petrone L, Goletti D. Risk of tuberculosis reactivation associated with traditional disease modifying anti-rheumatic drugs and non-anti-tumor necrosis factor biologics in patients with rheumatic disorders and suggestion for clinical practice. Expert Opin Drug Saf. (2019) 18:415–25. doi: 10.1080/14740338.2019.1612872
138. Picchianti-Diamanti A, Spinelli FR, Rosado MM, Conti F, Laganà B. Inhibition of phosphodiesterase-4 in psoriatic arthritis and inflammatory bowel diseases. Int J Mol Sci. (2021) 22:2638. doi: 10.3390/ijms22052638
139. Torres T, Brembilla NC, Langley RG, Warren RB, Thaçi D, Kolios AGA, et al. Treatment of psoriasis with biologic and non-biologic targeted therapies in patients with latent tuberculosis infection or at risk for tuberculosis disease progression: Recommendations from a SPIN-FRT expert consensus. J Eur Acad Dermatol Venereol. (2024) 39(1):52–69. doi: 10.1111/jdv.20287
140. Gladman DD, Kavanaugh A, Gómez-Reino JJ, Wollenhaupt J, Cutolo M, Schett G, et al. Therapeutic benefit of apremilast on enthesitis and dactylitis in patients with psoriatic arthritis: a pooled analysis of the PALACE 1-3 studies. RMD Open. (2018) 4:e000669. doi: 10.1136/rmdopen-2018-000669
141. Hagberg KW, Persson R, Vasilakis-Scaramozza C, Niemcryk S, Peng M, Paris M, et al. Herpes zoster and tuberculosis risk with apremilast compared to biologics, DMARDs and corticosteroids to treat psoriasis and psoriatic arthritis. Clin Epidemiol. (2020) 12:153–61. doi: 10.2147/CLEP.S239511
142. Fragoulis GE, McInnes IB, Siebert S, JAK-inhibitors. New players in the field of immune-mediated diseases, beyond rheumatoid arthritis. Rheumatol (Oxford). (2019) 58:i43–54. doi: 10.1093/rheumatology/key276
143. Mohamed M-EF, Beck D, Camp HS, Othman AA. Preferential inhibition of JAK1 relative to JAK3 by upadacitinib: exposure-response analyses of ex vivo data from 2 phase 1 clinical trials and comparison to tofacitinib. J Clin Pharmacol. (2020) 60:188–97. doi: 10.1002/jcph.1513
144. McInnes IB, Byers NL, Higgs RE, Lee J, Macias WL, Na S, et al. Comparison of baricitinib, upadacitinib, and tofacitinib mediated regulation of cytokine signaling in human leukocyte subpopulations. Arthritis Res Ther. (2019) 21:183. doi: 10.1186/s13075-019-1964-1
145. Maiga M, Lun S, Guo H, Winglee K, Ammerman NC, Bishai WR. Risk of tuberculosis reactivation with tofacitinib (CP-690550). J Infect Dis. (2012) 205:1705–8. doi: 10.1093/infdis/jis269
146. Cohen SB, Tanaka Y, Mariette X, Curtis JR, Lee EB, Nash P, et al. Long-term safety of tofacitinib for the treatment of rheumatoid arthritis up to 8.5 years: integrated analysis of data from the global clinical trials. Ann Rheum Dis. (2017) 76:1253–62. doi: 10.1136/annrheumdis-2016-210457
147. Winthrop KL, Park S-H, Gul A, Cardiel MH, Gomez-Reino JJ, Tanaka Y, et al. Tuberculosis and other opportunistic infections in tofacitinib-treated patients with rheumatoid arthritis. Ann Rheum Dis. (2016) 75:1133–8. doi: 10.1136/annrheumdis-2015-207319
148. Phatak S, Khenat A, Malandkar M, Amin S. Real-world evidence of the effectiveness and safety of generic tofacitinib in rheumatoid arthritis patients: a retrospective, single-centre analysis from Western India. Clin Rheumatol. (2022) 41:2961–6. doi: 10.1007/s10067-022-06205-z
149. Smolen JS, Genovese MC, Takeuchi T, Hyslop DL, Macias WL, Rooney T, et al. Safety profile of baricitinib in patients with active rheumatoid arthritis with over 2 years median time in treatment. J Rheumatol. (2019) 46:7–18. doi: 10.3899/jrheum.171361
150. Chen Y-C, Yoo DH, Lee CK, Li K-J, Won J-E, Wu W-S, et al. Safety of baricitinib in East Asian patients with moderate-to-severe active rheumatoid arthritis: An integrated analysis from clinical trials. Int J Rheum Dis. (2020) 23:65–73. doi: 10.1111/1756-185X.13748
151. Burmester GR, Cohen SB, Winthrop KL, Nash P, Irvine AD, Deodhar A, et al. Safety profile of upadacitinib over 15 000 patient-years across rheumatoid arthritis, psoriatic arthritis, ankylosing spondylitis and atopic dermatitis. RMD Open. (2023) 9:e002735. doi: 10.1136/rmdopen-2022-002735
152. Genovese MC, Fleischmann R, Combe B, Hall S, Rubbert-Roth A, Zhang Y, et al. Safety and efficacy of upadacitinib in patients with active rheumatoid arthritis refractory to biologic disease-modifying anti-rheumatic drugs (SELECT-BEYOND): a double-blind, randomised controlled phase 3 trial. Lancet. (2018) 391:2513–24. doi: 10.1016/S0140-6736(18)31116-4
153. Smolen JS, Pangan AL, Emery P, Rigby W, Tanaka Y, Vargas JI, et al. Upadacitinib as monotherapy in patients with active rheumatoid arthritis and inadequate response to methotrexate (SELECT-MONOTHERAPY): a randomised, placebo-controlled, double-blind phase 3 study. Lancet. (2019) 393:2303–11. doi: 10.1016/S0140-6736(19)30419-2
154. Cohen SB, van Vollenhoven RF, Winthrop KL, Zerbini CAF, Tanaka Y, Bessette L, et al. Safety profile of upadacitinib in rheumatoid arthritis: integrated analysis from the SELECT phase III clinical programme. Ann Rheum Dis. (2021) 80:304–11. doi: 10.1136/annrheumdis-2020-218510
155. McInnes IB, Anderson JK, Magrey M, Merola JF, Liu Y, Kishimoto M, et al. Trial of upadacitinib and adalimumab for psoriatic arthritis. N Engl J Med. (2021) 384:1227–39. doi: 10.1056/NEJMoa2022516
156. Mease PJ, Lertratanakul A, Anderson JK, Papp K, Van den Bosch F, Tsuji S, et al. Upadacitinib for psoriatic arthritis refractory to biologics: SELECT-PsA 2. Ann Rheum Dis. (2021) 80:312–20. doi: 10.1136/annrheumdis-2020-218870
157. van der Heijde D, Baraliakos X, Sieper J, Deodhar A, Inman RD, Kameda H, et al. Efficacy and safety of upadacitinib for active ankylosing spondylitis refractory to biological therapy: a double-blind, randomised, placebo-controlled phase 3 trial. Ann Rheum Dis. (2022) 81:1515–23. doi: 10.1136/ard-2022-222608
158. Baldi C, Parisi S, Falsetti P, Sota J, Ditto MC, Capassoni M, et al. Efficacy and safety of upadacitinib in rheumatoid arthritis: real-life experience from a prospective longitudinal multicentric study. J Clin Med. (2024) 13:401. doi: 10.3390/jcm13020401
159. Picchianti Diamanti A, Cattaruzza MS, Salemi S, Di Rosa R, Sesti G, De Lorenzo C, et al. Clinical and ultrasonographic remission in bio-naïve and bio-failure patients with rheumatoid arthritis at 24 weeks of upadacitinib treatment: the UPARAREMUS real-life study. Rheumatol Ther. (2024) 11(5):1347–61. doi: 10.1007/s40744-024-00712-y
160. Combe B, Kivitz A, Tanaka Y, van der Heijde D, Simon JA, Baraf HSB, et al. Filgotinib versus placebo or adalimumab in patients with rheumatoid arthritis and inadequate response to methotrexate: a phase III randomised clinical trial. Ann Rheum Dis. (2021) 80:848–58. doi: 10.1136/annrheumdis-2020-219214
161. Winthrop KL, Tanaka Y, Takeuchi T, Kivitz A, Matzkies F, Genovese MC, et al. Integrated safety analysis of filgotinib in patients with moderately to severely active rheumatoid arthritis receiving treatment over a median of 1.6 years. Ann Rheum Dis. (2022) 81:184–92. doi: 10.1136/annrheumdis-2021-221051
162. WHO. WHO consolidated guidelines on tuberculosis Module 1: prevention - tuberculosis preventive treatment . Available online at: https://www.who.int/publications/i/item/9789240096196 (Accessed September 9, 2024).
163. Migliori GB, Wu SJ, Matteelli A, Zenner D, Goletti D, Ahmedov S, et al. Clinical standards for the diagnosis, treatment and prevention of TB infection. Int J Tuberc Lung Dis. (2022) 26:190–205. doi: 10.5588/ijtld.21.0753
164. Kontsevaya I, Cabibbe AM, Cirillo DM, DiNardo AR, Frahm N, Gillespie SH, et al. Update on the diagnosis of tuberculosis. Clin Microbiol Infect. (2024) 30:1115–22. doi: 10.1016/j.cmi.2023.07.014
165. Alonzi T, Repele F, Goletti D. Research tests for the diagnosis of tuberculosis infection. Expert Rev Mol Diagn. (2023) 23:783–95. doi: 10.1080/14737159.2023.2240230
166. Bartalesi F, Vicidomini S, Goletti D, Fiorelli C, Fiori G, Melchiorre D, et al. QuantiFERON-TB Gold and the TST are both useful for latent tuberculosis infection screening in autoimmune diseases. Eur Respir J. (2009) 33:586–93. doi: 10.1183/09031936.00107608
167. Kang J, Jeong DH, Yoo B, Lee C-K, Kim Y-G, Hong S, et al. The usefulness of routine chest radiograph examinations in patients treated with TNF inhibitors for inflammatory arthritis in South Korea. Respir Med. (2018) 143:109–15. doi: 10.1016/j.rmed.2018.09.005
168. Tahan TT, Rossoni AM de O, Bedim Dos Santos M, da Silveira JTP, de Oliveira SP, Rodrigues C de O. Tuberculosis preventive treatment in children and adolescents: an observational study of secondary data. J Pediatr (Rio J). (2023) 99:399–405. doi: 10.1016/j.jped.2023.02.002
169. Otero L, Zetola N, Campos M, Zunt J, Bayer A, Curisinche M, et al. Isoniazid preventive therapy completion in children under 5 years old who are contacts of tuberculosis cases in Lima, Peru: study protocol for an open-label, cluster-randomized superiority trial. Trials. (2023) 24:54. doi: 10.1186/s13063-022-07062-6
170. Jagi JL, Thomas C, Gudi SK, Undela K. Efficacy, safety, and tolerability of isoniazid preventive therapy for tuberculosis in people living with HIV. AIDS. (2023) 37:455–65. doi: 10.1097/QAD.0000000000003436
171. International Union Against Tuberculosis Committee on Prophylaxis. Efficacy of various durations of isoniazid preventive therapy for tuberculosis: five years of follow-up in the IUAT trial. International Union Against Tuberculosis Committee on Prophylaxis. Bull World Health Organ. (1982) 60:555–64.
172. Snider DE, Caras GJ, Koplan JP. Preventive therapy with isoniazid. Cost-effectiveness of different durations of therapy. JAMA. (1986) 255:1579–83. doi: 10.1001/jama.1986.03370120057023
173. Nolan CM, Goldberg SV, Buskin SE. Hepatotoxicity associated with isoniazid preventive therapy: a 7-year survey from a public health tuberculosis clinic. JAMA. (1999) 281:1014–8. doi: 10.1001/jama.281.11.1014
174. LoBue PA, Moser KS. Use of isoniazid for latent tuberculosis infection in a public health clinic. Am J Respir Crit Care Med. (2003) 168:443–7. doi: 10.1164/rccm.200303-390OC
175. Fox GJ, Dobler CC, Marais BJ, Denholm JT. Preventive therapy for latent tuberculosis infection-the promise and the challenges. Int J Infect Dis. (2017) 56:68–76. doi: 10.1016/j.ijid.2016.11.006
176. Grace SG. Barriers to the implementation of isoniazid preventive therapy for tuberculosis in children in endemic settings: A review. J Paediatr Child Health. (2019) 55:278–84. doi: 10.1111/jpc.14359
177. Sterling TR, Villarino ME, Borisov AS, Shang N, Gordin F, Bliven-Sizemore E, et al. Three months of rifapentine and isoniazid for latent tuberculosis infection. N Engl J Med. (2011) 365:2155–66. doi: 10.1056/NEJMoa1104875
178. Sun H-Y, Huang Y-W, Huang W-C, Chang L-Y, Chan P-C, Chuang Y-C, et al. Twelve-dose weekly rifapentine plus isoniazid for latent tuberculosis infection: A multicentre randomised controlled trial in Taiwan. Tuberculosis (Edinb). (2018) 111:121–6. doi: 10.1016/j.tube.2018.05.013
179. Lee M-R, Huang H-L, Lin S-W, Cheng M-H, Lin Y-T, Chang S-Y, et al. Isoniazid concentration and NAT2 genotype predict risk of systemic drug reactions during 3HP for LTBI. J Clin Med. (2019) 8:812. doi: 10.3390/jcm8060812
180. Melnychuk L, Perlman-Arrow S, Lisboa Bastos M, Menzies D. A systematic review and meta-analysis of tuberculous preventative therapy adverse events. Clin Infect Dis. (2023) 77:287–94. doi: 10.1093/cid/ciad246
181. WHO. Tuberculosis preventive treatment: rapid communicationSeptember 2, 2024. Available online at: https://www.who.int/publications/i/item/9789240089723 (Accessed February 13, 2024).
182. Silva JT, San-Juan R, Fernández-Ruiz M, Aguado JM. Fluoroquinolones for the treatment of latent Mycobacterium tuberculosis infection in liver transplantation. World J Gastroenterol. (2019) 25:3291–8. doi: 10.3748/wjg.v25.i26.3291
183. Cai W, Tang X, Pang M. Prevalence of metabolic syndrome in patients with rheumatoid arthritis: an updated systematic review and meta-analysis. Front Med (Lausanne). (2022) 9:855141. doi: 10.3389/fmed.2022.855141
184. Huang HL, Lee JY, Lo YS, Liu IH, Huang SH, Huang YW, et al. Whole-blood 3-gene signature as a decision aid for rifapentine-based tuberculosis preventive therapy. Clin Infect Dis. (2022) 75:743–52. doi: 10.1093/cid/ciac003
185. Fornaro M, Stano S, Goletti D, Semeraro A, Cantatore FP, Maruotti N, et al. Prevalence and management of tuberculosis infection in Apulian rheumatologic patients treated with biologics: An observational cohort 10-year study from the BIOPURE registry. Eur J Clin Invest. (2023) 53:e13913. doi: 10.1111/eci.13913
186. Cansu DÜ, Güncan S, Bilge NŞY, Kaşifoğlu T, Korkmaz C. Does isoniazid chemoprophylaxis increase the frequency of hepatotoxicity in patients receiving anti-TNF-α agent with a disease-modifying antirheumatic drug? Eur J Rheumatol. (2014) 1:62–6. doi: 10.5152/eurjrheumatol.2014.019
187. Bray M-G, Poulain C, Dougados M, Gossec L. Frequency and tolerance of antituberculosis treatment according to national guidelines for prevention of risk of tuberculosis due to tumor necrosis factor blocker treatment. Joint Bone Spine. (2010) 77:135–41. doi: 10.1016/j.jbspin.2009.10.012
188. Hanta I, Ozbek S, Kuleci S, Sert M, Kocabas A. Isoniazid intervention for latent tuberculosis among 86 patients with rheumatologic disease administered with anti-TNFalpha. Clin Rheumatol. (2007) 26:1867–70. doi: 10.1007/s10067-007-0591-4
189. Conway R, Carey JJ. Risk of liver disease in methotrexate treated patients. World J Hepatol. (2017) 9:1092–100. doi: 10.4254/wjh.v9.i26.1092
190. Vanhoof J, Landewe S, Van Wijngaerden E, Geusens P. High incidence of hepatotoxicity of isoniazid treatment for tuberculosis chemoprophylaxis in patients with rheumatoid arthritis treated with methotrexate or sulfasalazine and anti-tumour necrosis factor inhibitors. Ann Rheum Dis. (2003) 62:1241–2. doi: 10.1136/ard.2002.004598
191. Goletti D, Parracino MP, Butera O, Bizzoni F, Casetti R, Dainotto D, et al. Isoniazid prophylaxis differently modulates T-cell responses to RD1-epitopes in contacts recently exposed to Mycobacterium tuberculosis: a pilot study. Respir Res. (2007) 8:5. doi: 10.1186/1465-9921-8-5
192. Belay M, Tulu B, Younis S, Jolliffe DA, Tayachew D, Manwandu H, et al. Detection of Mycobacterium tuberculosis complex DNA in CD34-positive peripheral blood mononuclear cells of asymptomatic tuberculosis contacts: an observational study. Lancet Microbe. (2021) 2:e267–75. doi: 10.1016/S2666-5247(21)00043-4
193. Repele F, Alonzi T, Navarra A, Farroni C, Salmi A, Cuzzi G, et al. Detection of Mycobacterium tuberculosis DNA in CD34+ peripheral blood mononuclear cells of adults with tuberculosis infection and disease. Int J Infect Dis. (2024) 141S:106999. doi: 10.1016/j.ijid.2024.106999
194. Palacios CF, Chowdhary V, Hao R, Danve A, Malinis M. Is yearly interferon gamma release assay latent tuberculosis infection screening warranted among patients with rheumatological diseases on disease-modifying drugs in non-endemic settings? PloS One. (2024) 19:e0306337. doi: 10.1371/journal.pone.0306337
Keywords: tuberculosis disease, TB infection, rheumatoid arthritis, psoriatic arthritis, ankylosing spondylitis, biologic DMARDs, JAK inhibitors, preventive therapy
Citation: Picchianti-Diamanti A, Aiello A, De Lorenzo C, Migliori GB and Goletti D (2025) Management of tuberculosis risk, screening and preventive therapy in patients with chronic autoimmune arthritis undergoing biotechnological and targeted immunosuppressive agents. Front. Immunol. 16:1494283. doi: 10.3389/fimmu.2025.1494283
Received: 10 September 2024; Accepted: 06 January 2025;
Published: 03 February 2025.
Edited by:
Subash Babu, International Centers for Excellence in Research (ICER), IndiaReviewed by:
Dimitrios Vassilopoulos, National and Kapodistrian University of Athens, GreeceMarco Fornaro, University of Bari Aldo Moro, Italy
Javeed Ali Shah, University of Washington, United States
Copyright © 2025 Picchianti-Diamanti, Aiello, De Lorenzo, Migliori and Goletti. This is an open-access article distributed under the terms of the Creative Commons Attribution License (CC BY). The use, distribution or reproduction in other forums is permitted, provided the original author(s) and the copyright owner(s) are credited and that the original publication in this journal is cited, in accordance with accepted academic practice. No use, distribution or reproduction is permitted which does not comply with these terms.
*Correspondence: Delia Goletti, ZGVsaWEuZ29sZXR0aUBpbm1pLml0
†These authors have contributed equally to this work and share first authorship