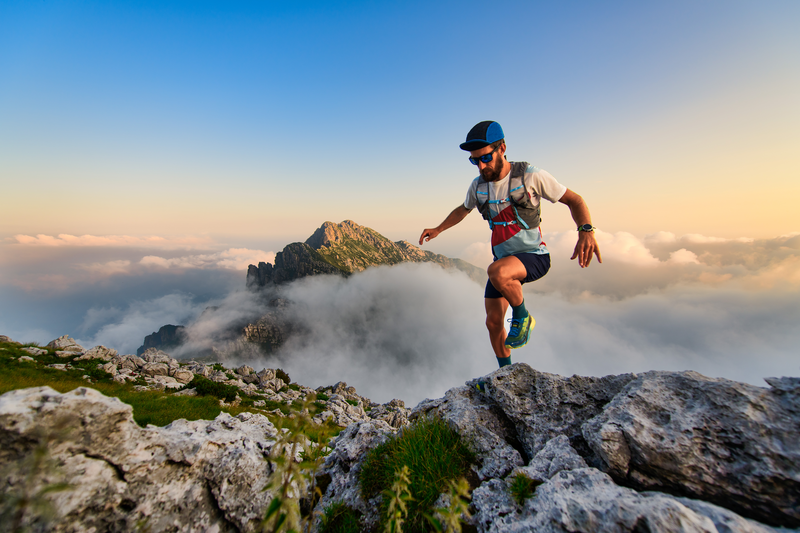
94% of researchers rate our articles as excellent or good
Learn more about the work of our research integrity team to safeguard the quality of each article we publish.
Find out more
ORIGINAL RESEARCH article
Front. Immunol. , 31 January 2025
Sec. Inflammation
Volume 16 - 2025 | https://doi.org/10.3389/fimmu.2025.1486072
This article is part of the Research Topic The Role of Inflammation and Immune Control in Digestive Disease and Therapeutic Approaches View all 20 articles
Background: Abdominal aortic aneurysm (AAA) is a degenerative disease with high mortality. Chronic inflammation plays a vital role in the formation of AAA. Atractylenolide-I (ATL-I) is a major bioactive component of Rhizoma Atractylodis Macrocephalae that exerts anti-inflammatory effects in various diseases. The purpose of this study is to investigate the role of ATL-I in the progression of AAA.
Methods: AAA was constructed in C57BL/6 mice by porcine pancreatic elastase (PPE)-incubation, and the diameter of the aorta was measured by ultrasound. ATL-I was administered by gavage on the second day after modeling to explore its significance in AAA. The pathological and molecular alteration was investigated by immunostaining, ELISA, qRT-PCR and Western blotting.
Results: ATL-I inhibited the dilatation of the abdominal aorta and decreased the incidence of AAA. ATL-I alleviated the infiltration of macrophages in the adventitia and reduced the levels of proinflammatory factor IL-1β and IL-6 in the aorta and circulatory system, while increasing the expression of anti-inflammatory factor IL-10. Moreover, ATL-I restrained loss of smooth muscle cells and elastic fiber degradation by suppressing MMP-2 and MMP-9 expression. Mechanistically, phospho-AMPK expression was elevated in AAA groups, and ATL-I administration suppressed its expression to improve the pathological damage of aorta.
Conclusions: ATL-I meliorated vascular inflammation by targeting AMPK signaling, ultimately inhibiting AAA formation, which provided an alternative agent for AAA treatment.
Abdominal aortic aneurysm (AAA) is a severe vascular disease characterized by focal dilation of the abdominal aorta (1). Once AAA ruptures, the mortality rate is as high as 65-85% (2–4). With the development of open repair and endovascular aneurysm repair (EVAR) therapy, patients with diameters greater than 5.5 cm (women > 4.5 cm) can be effectively treated (5, 6). However, patients with small AAA or those deemed unfit for AAA repair criteria will gradually deteriorate. There is no successful pharmacological treatment to delay expansion or prevent rupture of AAA in humans (7). It highlights an emergent need to discover effective drugs to limit AAA growth.
In AAA development, the arterial wall suffers a destructive remodeling, including upregulation of matrix metalloproteinases (MMPs), loss of vascular smooth muscle cells (VSMCs), and degradation of elastic fibers (8). Chronic inflammation is closely related to vascular remodeling (9, 10). Infiltrated macrophages and VSMCs can secrete various proinflammatory cytokines, such as interleukin-1β (IL-1β) and IL-6, thereby activating more immune cells to aggravate the vascular injury, eventually resulting in probable rupture of AAA (11, 12).
Atractylenolide-I (ATL-I), a major bioactive component of Rhizoma Atractylodis Macrocephalae, exerts anti-oxidant, anti-inflammatory, and anti-tumor effects (13–15). Li et al. demonstrated that ATL-I had an intensely inhibitory effect on ox-LDL-induced inflammatory responses and a protective role in anti-atherosclerosis (16). Wang et al. observed that ATL-I inhibited the osteogenic differentiation of valve interstitial cells through blocking phosphatidylinositol-3-kinase (PI3K)/AKT pathway (17). Furthermore, Wang et al. discovered the promising therapeutic potential of ATL-I for sepsis-induced cardiomyopathy by suppressing macrophage polarization (18). Based on previous reports, we speculated that ATL-I might affect the formation of AAA by exerting anti-inflammatory effects.
In this study, we confirmed that ATL-I could alleviate the pathological lesion and lumen dilatation of AAA by targeting AMPK signaling, which provided a novel therapeutic strategy for patients with AAA.
Eight-week-old male C57BL/6J mice were obtained from the laboratory of Jinan University’s animal center and used in this study. All animals were fed in cages and bred in a temperature-controlled room maintained at 22 ± 1°C on a 12-h light-dark cycle with standard food and water. All animal experimental procedures complied with Institutional Animal Care and Use Committee of Jinan University.
According to previously reported surgical methods (19), a mouse model of AAA or sham was induced by perivascular application of PPE (Sigma-Aldrich; 4 U/mg protein) or saline. Briefly, after induction of anesthesia with 3% isoflurane, the lower three-fourths of the infrarenal abdominal aorta was exposed and isolated from the vena cava. 30uL elastase was applied to exposed aortic adventitia and infiltrated for 30 minutes. Then, the abdominal cavity, especially the adventitia, needed to be rinsed with saline. Finally, the abdomen was closed routinely with 6-0 silk sutures. After 14 days, all mice were sacrificed by carbon dioxide inhalation, while the aortas were isolated for pathological examination.
To investigate the effect of ATL-I on AAA, a total of 18 mice were divided by random number table into sham group, AAA group, and ATL-I group with 6 samples in each group. After modeling, mice in ATL-I groups received the ATL-I (20mg/kg/day, 73069-13-3, MCE) by gavage daily for the next 14 days, and mice in AAA group were given the same amount of sterile PBS (20–23). The molecular structure of ATL-I was shown in Figure 1A.
Figure 1. Effect of ATL-I on the abdominal aortic diameter. (A) Molecular structure of ATL-I. (B) Surgical anatomy diagram among the three groups. (C) Representative ultrasonography images of the three groups on the 0 and 14 days after modeling. (D) The diameter of abdominal aorta on the 0 and 14 days after modeling. (E) Incidence of AAA (represented by percentages). (F, G) Adventitial thickness of aorta and representative images of HE staining. *P < 0.05, ***P < 0.001, ****P < 0.0001 vs. the sham group; ##P < 0.01 vs. the AAA group.
To investigate whether ATL-I affects AAA through AMP-activated protein kinase (AMPK) signaling pathway, 24 mice were randomly divided into four groups of six mice in each group. The treatment of the sham group, AAA group and ATL-I group was the same as the groups mentioned above. In the ATL-I+CC group, mice were simultaneously given compound C (CC, 300mg/kg/day, 866405-64-3, MCE) and ATL-I (20mg/kg/day).
The diameter of the subrenal abdominal aorta was measured by B-ultrasound imaging (Vevo 2100, Visual Sonic Inc.) before and 14 days after modeling. After anesthesia with 1.5% isoflurane, the aorta of mouse was detected during systole by a blinded investigator. AAA was defined as the maximum diameter of the subrenal aorta that dilates more than 50% of its baseline (The diameter of the abdominal aorta examined before modeling) (24).
Aortic samples isolated from the mice were fixed with 4% paraformaldehyde for 24h and embedded in paraffin. Serial sections (5μm each) were used for HE staining, elastic fiber staining (G1596, Solarbio) and immunofluorescence staining. The severity of elastic fiber degradation was evaluated on a standard described in a previous study: score 1, <25% degradation; score 2, 25% to 50% degradation; score 3, 50% to 75% degradation; score 4, >75% degradation (25).
Paraffin slides were incubated in xylene and alcohol of different gradients for deparaffin and rehydration. The slides were placed in 10 mM sodium citrate buffer (pH 6, BL619A, Biosharp) at 85°C for 30 minutes and cooled at room temperature for 20 minutes. Then, the slides were incubated in 5% horse serum for 30 minutes at room temperature. Next, the slides were incubated at 4°C overnight with the following primary antibodies: CD68 (1:200, ab125212; Abcam), CD80 (1:200, ab254579, Abcam), CD206 (1:200, AF2535, R&D Systems), α-SMA (1:500, ab5694, Abcam). The following day, the slides were incubated with the corresponding secondary antibodies and DAPI at room temperature for 30 minutes. Immunofluorescence images were obtained using confocal laser scanning microscopy (Leica) and analyzed using Image-J software.
Serum was obtained by centrifugation after blood collection through inferior vena cava. The content of IL-1β, IL-6, IL-10, alanine aminotransferase (ALT), aspertate aminotransferase (AST), creatine, and blood urea nitrogen (BUN) was analyzed using a commercially available ELISA kit (ml098416; ml098430; mIC50274-1; SP30122; CSB-E12649m; LE-M0613; RF8291; MLbio; Spbio; Cusabio; Isite; Ruifan) according to the manufacturer’s protocol. The results were calculated using the standard curve method and expressed as pg/ml.
Total RNA was extracted from the subrenal abdominal aorta using Trizol Reagent (155960-18, Ambion). Then, 1ug of total RNA was subjected to reverse transcription based on the manufacturer’s instructions. The mRNA expression of IL-1β, IL-6 and IL-10 in aorta was analyzed through PCR system (BIO-RAD). The primer sequences are shown in Table 1.
Total protein was extracted with RIPA lysate. Protein concentration was quantified by bicinchoninic acid (BCA) protein assay kit (P0010, Beyotime). The protein was separated by 10% SDS-PAGE, transferred to a polyvinylidene difluoride (PVDF) membrane, and incubated in 5% BSA for 1 hour at room temperature. Then, the membranes were incubated overnight with primary antibodies AMPK (1:1000, 2365, CST), p-AMPK (1:1000, 2535, CST), MMP-9 (1:1000, ab283575, Abcam), MMP-2 (1:1000, ab86607, Abcam), β-actin (1:1000, ab8226, Abcam) at 4°C. The membranes were incubated with secondary antibodies for 1 hour at room temperature the next day. Finally, the protein bands were visualized with the ECL system (BIO-RAD).
All data were presented as the mean ± SD and executed using GraphPad Prism 8.0 software. Statistical differences between groups were performed by one-way ANOVA. P<0.05 was considered as statistically significant.
To explore whether ATL-I could restrain AAA progression, we first detected the diameter of the infrarenal abdominal aorta on the 14th day after modeling. As revealed by Figure 1B, the aorta diameter in the AAA group was significantly increased, which was reversed after ATL-I treatment. At the same time, ultrasound data showed that the infrarenal aorta was dilated with a maximum diameter of 1.23 ± 0.21 mm in the AAA group. However, the baseline of its diameter was 0.57 ± 0.02 mm, and the maximum infrarenal aortic diameter was only slightly increased to 0.91 ± 0.02 mm in the ATL-I group (Figures 1C, D). Ultimately, ATL-I decreased the incidence of AAA (Figure 1E). Additionally, we observed that ATL-I couldn’t change the levels of ALT, AST, creatine and BUN, indicating that it had no obviously toxic side effects on liver and renal function in vivo (Supplementary Figures S1A–D). HE staining results displayed that the adventitia was thicker, accompanied with recruitment of vast inflammatory cells in AAA, and the terrible condition was improved following ATL-I treatment (Figures 1F, G). Taken together, ATL-I administration inhibited the progression of AAA.
Inflammation has been considered as a central player in the progression of AAA. There were a large number of macrophages (CD68+) in the adventitia of AAA (Figures 2A, D). To examine whether the inhibitory effects of ATL-I on AAA progression were associated with changes in M1 or M2 macrophages, we subjected abdominal aortic tissue for immunostaining using CD80 to stain M1 macrophages and CD206 to identify M2 macrophages. As shown in Figures 2B, E the significantly increased CD8 staining was observed in the adventitial region of the aneurysm, which was markedly reduced following ATL-I administration. Nevertheless, ATL-I treatment significantly augment aggregation of M2 macrophages in adventitia (Figures 2C, F). Subsequently, we compared the differences in the levels of inflammatory cytokines among three groups using ELISA and qPCR experiments. As illustrated in Figures 2G–J, the expression of proinflammatory cytokines, such as IL-1β and IL-6, was elevated in the aneurysm and circulating system of mice with AAA, which was restrained after ATL-I treatment. By comparison, decreased anti-inflammatory cytokine IL-10 expression in AAA were reversed by ATL-I (Figures 2K, L). As such, our results demonstrated the anti-inflammatory activity of ATL-I on AAA.
Figure 2. Effect of ATL-I on inflammatory responses in AAA. (A) Representative immunofluorescent images of CD68. (B) Representative immunofluorescent images of CD80. (C) Representative immunofluorescent images of CD206. (D-F) Densitometric analysis of CD68, CD80 and CD206. (G) Changes in circulating levels of IL-1β. (H) Relative mRNA expression of IL-1β in aorta. (I) Changes in circulating levels of IL-6. (J) Relative mRNA expression of IL-6 in aorta. (K) Changes in circulating levels of IL-10. (L) Relative mRNA expression of IL-10 in aorta. *P < 0.05, **P < 0.01, ***P < 0.001, ****P < 0.0001 vs. the sham group; ##P < 0.01, ####P < 0.0001 vs. the AAA group.
Macrophages and VSMCs generate MMP-2 and MMP-9, therefore triggering steady destruction of extracellular matrix (ECM). We next examined α-SMA expression and elastin alteration. As shown in Figures 3A, B, a large amount of VSMCs was lost and remaining VSMCs were disordered in aneurysm, whereas the VSMCs disruption was markedly ameliorated after ATL-I supplement. Similarly, ATL-I administration improved the condition of elastin that showed obvious fracture and degradation in AAA tissue (Figures 3C, D). We further verified the levels of MMP-2 and MMP-9 using western blotting. MMP-2 and MMP-9 were elevated in the AAA group, which was mitigated after ATL-I therapy (Figures 3E, F). Collectively, these results suggested that ATL-I inhibited MMP-2 and MMP-9 expression, thereby attenuating pathological lesions of aorta.
Figure 3. Effect of ATL-I on the histopathological lesion of the aortic wall. (A) Representative images of immunofluorescence. (B) Densitometric analysis of α-SMA. (C) Representative images of elastin staining. (D) Elastin degradation score. (E, F) Representative blots and semiquantitative analysis of MMP-2 and MMP-9. *P < 0.05, **P < 0.01, ***P < 0.001, ****P < 0.0001 vs. the sham group; #P< 0.05, ##P< 0.01 vs. the AAA group.
Though there was no difference in AMPK expression in three groups, the expression of phospho- AMPK (p-AMPK) was downregulated in AAA tissues, which was upregulated following ATL-I treatment (Figure 4A). Therefore, we speculated that ATL-I might exert its inhibitory effect on AAA by activating AMPK phosphorylation. As expected, the administration of compound C, an inhibitor of AMPK, abolished the salutary effects of ATL-I on AAA. It was found that the diameter of aneurysm and adventitial thickness were apparently increased in the ATL-I+CC group (Figures 4B–D). Compound C reduced the phosphorylation level of AMPK, and led to a larger diameter of abdominal aorta and a higher incidence of AAA, compared with ATL-I group (Figures 4E, F). Thus, ATL-I treatment retarded AAA formation partially by targeting AMPK.
Figure 4. Effect of ATL-I on restraining the progression of AAA through AMPK pathway. (A) Representative blots and semiquantitative analysis of p-AMPK and AMPK. (B) Surgical anatomy diagram. (C) Representative images of HE staining. (D) Adventitial thickness of aorta. (E) Representative blots and semiquantitative analysis of p-AMPK and AMPK. (F) Representative ultrasonography images, the diameter of abdominal aorta, and the incidence of AAA. **P < 0.01, ***P < 0.001, ****P< 0.0001vs. the sham group; ##P < 0.01, ###P < 0.001, ####P < 0.0001 vs. the AAA group.
Subsequently, we examined the levels of macrophages and inflammatory cytokines after the application of compound C. Compared with the ATL-I group, the fluorescence intensity of CD68 was robustly increased in ATL-I+CC group (Figures 5A, D). Similarly, compound C reduced the inhibitory effect of ATL-I on M1 macrophages (Figures 5B, E). In contrast, increased CD206 expression was observed in the adventitia in the ATL-I group, which was restricted by compound C administration (Figures 5C, F). Meanwhile, ATL-I-induced decreased levels of IL-1β and IL-6 in the aorta and circulating system were reraised after the administration of compound C (Figures 5G–J). Our data also demonstrated that ATL-I-triggered the activation of IL-10 was significantly decreased following compound C treatment (Figures 5K, L). Taken together, our results supported the notion that AMPK was required for ATL-I to exert its anti-inflammatory effects on AAA.
Figure 5. Effect of ATL-I on inhibiting inflammatory response of AAA through AMPK pathway. (A) Representative immunofluorescent images of CD68. (B) Representative immunofluorescent images of CD80. (C) Representative immunofluorescent images of CD206. (D-F) Densitometric analysis of CD68, CD80 and CD206. (G) Changes in circulating levels of IL-1β. (H) Relative mRNA expression of IL-1β in aorta. (I) Changes in circulating levels of IL-6. (J) Relative mRNA expression of IL-6 in aorta. (K) Changes in circulating levels of IL-10. (L) Relative mRNA expression of IL-10 in aorta. *P < 0.05, **P < 0.01, ***P < 0.001, ****P < 0.0001 vs. the sham group; #P < 0.05, ##P < 0.01, ###P < 0.001, ####P < 0.0001 vs. the AAA group; $P < 0.05, $$P < 0.01 vs. the ATL-I group.
Using immunostaining and elastic fiber staining, we observed that the α-SMA expression was dampened in the ATL-I+CC group, accompanied by the aggravated deterioration of elastic fibers, which was in line with pathological injury results of AAA as described above (Figures 6A–D). Furthermore, we noticed that the supplement of compound C impaired the inhibitory effects of ATL-I on MMP-2 and MMP-9 expression, as displayed in Figures 6E, F. Collectively, these findings suggested that ATL-I mitigated the pathological lesion of AAA by activating AMPK signaling pathway.
Figure 6. Effect of ATL-I on suppressing histopathological lesion of AAA through AMPK pathway. (A) Representative images of immunofluorescence. (B) Densitometric analysis of α-SMA. (C) Representative images of elastin staining. (D) Elastin degradation score. (E, F) Representative blots and semiquantitative analysis of MMP-2 and MMP-9. *P < 0.05, **P < 0.01, ****P < 0.0001 vs. the sham group; ###P< 0.001, ####P < 0.0001 vs. the AAA group; $P < 0.05, $$$P< 0.001, $$$$P< 0.0001 vs. the ATL-I group.
AAA is a severe disease affecting human health. With the improvement of living standards and the aggravation of the aging population, the incidence of AAA has increased yearly and is up to 4-8% in elderly people over 50 years old (26, 27). The development of AAA is very insidious, and the early patients have no apparent symptoms (28). Once the aneurysm is ruptured, patients present with severe abdominal pain, only 50% of which have the opportunity for emergency treatment. Even so, the 30-day mortality rate is as high as 30%-70% (29, 30). The clinical treatment and management of AAA remains quite a considerable challenge. Therefore, it is of great significance for early diagnosis and intervention of these patients.
Currently, drug therapy using natural substances has received increasing attention (31). Traditional Chinese medicines (TCMs) are considered a promising future for disease therapy by virtue of minimal side effects, low costs, and high efficiency. Prior studies have found that ATL-I, a biologically active herb known as Baizhu, exerts protective effects in a variety of diseases (32–34). ATL-I ameliorated cancer cachexia through decreased the phosphorylation levels of STAT3 and PKM2 (33). ATL-I acted as an inhibitor of TLR4, NF-κB, and MAPK signaling pathways, alleviating acetaminophen-induced acute liver injury (35). Additionally, ATL-I elevated the contents of neurotransmitters (5-HT, dopamine, and norepinephrine) through binding to 5-HT2A, thus improving depression-like phenotypes (36). However, there is no study on the ATL-I on AAA progression.
The pathogenesis of AAA is usually relevant to inflammation and ECM remodeling. A waterfall-like inflammation cascade ascribes to secretion of proinflammatory factors, which recruit more macrophages and lymphocytes to the adventitia (10, 37). Macrophages, VSMCs and fibroblasts secrete large amounts of MMP-9 and MMP-2 to aggravate ECM breakdown (11, 38). Many TCMs have been proven to inhibit the pathological progression of AAA. Paeonol, a peony bark extract, prevented experimental AAA progression by inhibiting the NF-κB pathway (39). Betanin exerted anti-inflammatory activity and ROS scavenging ability in AAA progression (40). At present, many experiments in vivo and in vitro have demonstrated the remarkable anti-inflammation efficacy of ATL-I, and revealed that its mechanism of action was associated with inhibition of inflammatory cells and medium. It was reported that ATL-I dose-dependently inhibited a variety of inflammatory mediator, such as nitric oxide (NO), tumor necrosis factor-α (TNF-α), IL-1β, IL-6, vascular endothelial growth factor (VEGF), and placental growth factor (PlGF) (41–43). Li et al. showed that ATL-I inhibited the proliferation and migration of VSMCs induced by oxidized low density lipoprotein (ox-LDL), and simultaneously reduced the production of mononuclear chemotactic protein 1 (MCP-1) to ameliorate the development of atherosclerotic (16). We thought that ATL-I had anti-AAA potential. As anticipated, ATL-I reduced the degeneration of elastic fibers in the aorta caused by PPE, and inhibit the lumen dilatation, thereby curbing AAA formation. Further researches confirmed that the infiltration of M1 macrophages was reduced, and the M2 differentiation of macrophage was increased following ATL-I administration. At the same time, the levels of pro-inflammatory factors (IL-1β and IL-6) decreased, and the expression of anti-inflammatory factor IL-10 upregulated. More importantly, ATL-I decreased the secretion of MMP-2 and MMP-9, thereby weakening the ECM degeneration. Whether ATL-I has inhibitory effect on lymphocytes deserves further investigation. Collectively, the protective effect of ATL-I on AAA was attributed partially to its anti-inflammatory activity.
AMPK is a serine/threonine kinase in which α subunits (α1 and α2 subtypes) have catalytic activity, while β and γ subunits are involved in maintaining the stability of the heterotrimer complex (44). AMPK, as an energy sensor, plays a role in regulating intracellular energy balance. AMPK is also responsible for influencing the biological process of autophagy, cell proliferation and ECM anabolism (45–47). However, AMPK dysfunction exists in a variety of diseases, including obesity, diabetes, and cardiovascular diseases. Studies have shown that the expression of p-AMPK is decreased in AAA, which contributes to the elevation of MMP-2/9 (48, 49). Mammalian target of rapamycin (mTOR), as a downstream molecule of AMPK, was elevated in AAA, but an inhibitor of mTOR was demonstrated to restrain the progression of AAA. Given the crucial role of AMPK in the biological activity of VSMCs, AMPK might act as a promising intervention target for the prevention and treatment of AAA. In this study, ATL-I could activate AMPK signaling and down-regulated MMP-2/9 levels to inhibit AAA progression, which was rescued after supplementing with AMPK inhibitor. Some studies have shown that JAK2/STAT3 and TLR4 are molecular targets of ATL-I and are also key molecules in AAA progression (35, 50–54). Therefore, when exploring the mechanism of ATL-I regulation of AAA, we try to find other molecular targets to improve the innovation of this study. In subsequent studies, we will continue to explore whether ATL-I also inhibits AAA progression through JAK2/STAT3 or TLR4 pathway in AAA, so as to further clarify the molecular mechanism of ATL-I on AAA. Although ATL-I interrupts the inflammatory cascade, the origin of the initial inflammation in the AAA needs to be further investigated. Moreover, developing ATL-I based on Chinese herbal extracts or active ingredients for clinical application is another strategy worthy of attention.
The datasets presented in this study can be found in online repositories. The names of the repository/repositories and accession number(s) can be found in the article/Supplementary Material.
The animal studies were approved by Institutional Animal Care and Use Committee of Jinan University. The studies were conducted in accordance with the local legislation and institutional requirements. Written informed consent was obtained from the owners for the participation of their animals in this study.
SC: Data curation, Writing – review & editing, Conceptualization, Formal analysis, Investigation, Software, Visualization. XL: Investigation, Project administration, Software, Writing – review & editing, Data curation. XZ: Data curation, Methodology, Project administration, Validation, Writing – review & editing. WL: Project administration, Software, Formal analysis, Methodology, Writing – review & editing. ML: Investigation, Project administration, Software, Validation, Writing – review & editing. HM: Investigation, Software, Writing – review & editing. KZ: Formal analysis, Investigation, Methodology, Project administration, Writing – original draft, Writing – review & editing. QM: Formal analysis, Investigation, Project administration, Software, Writing – original draft, Writing – review & editing. CQ: Data curation, Methodology, Project administration, Resources, Writing – original draft, Writing – review & editing.
The author(s) declare that no financial support was received for the research, authorship, and/or publication of this article.
The authors declare that the research was conducted in the absence of any commercial or financial relationships that could be construed as a potential conflict of interest.
All claims expressed in this article are solely those of the authors and do not necessarily represent those of their affiliated organizations, or those of the publisher, the editors and the reviewers. Any product that may be evaluated in this article, or claim that may be made by its manufacturer, is not guaranteed or endorsed by the publisher.
The Supplementary Material for this article can be found online at: https://www.frontiersin.org/articles/10.3389/fimmu.2025.1486072/full#supplementary-material
Supplementary Figure S1 | (A) Serum ALT(U/L). (B) Serum AST(U/L). (C) Serum creatine (umol/L). (D) Serum BUN (mmol/L).
1. Schanzer A, Oderich GS. Management of abdominal aortic aneurysms. N Engl J Med (2021) 385(18):1690–8. doi: 10.1056/NEJMcp2108504
2. Zalewski D, Chmiel P, Kolodziej P, Borowski G, Feldo M, Kocki J, et al. Dysregulations of key regulators of angiogenesis and inflammation in abdominal aortic aneurysm. Int J Mol Sci (2023) 24(15). doi: 10.3390/ijms241512087
3. Teles J, Teles N, Sousa H. Screening of the abdominal aortic aneurysm: Cost-effectiveness and health benefits. Port J Card Thorac Vasc Surg (2021) 28(2):39–48.
4. He B, Zhan Y, Cai C, Yu D, Wei Q, Quan L, et al. Common molecular mechanism and immune infiltration patterns of thoracic and abdominal aortic aneurysms. Front Immunol (2022) 13:1030976. doi: 10.3389/fimmu.2022.1030976
5. Golledge J, Thanigaimani S, Powell JT, Tsao PS. Pathogenesis and management of abdominal aortic aneurysm. Eur Heart J (2023) 44(29):2682–97. doi: 10.1093/eurheartj/ehad386
6. Puertas-Umbert L, Almendra-Pegueros R, Jimenez-Altayo F, Sirvent M, Galan M, Martinez-Gonzalez J, et al. Novel pharmacological approaches in abdominal aortic aneurysm. Clin Sci (Lond) (2023) 137(15):1167–94. doi: 10.1042/CS20220795
7. Chiew K, Roy IN, Budge J, D'Abate F, Holt P, Loftus IM. The fate of patients opportunistically screened for abdominal aortic aneurysms during echocardiogram or arterial duplex scans. Eur J Vasc Endovasc Surg (2023) 66(2):188–93. doi: 10.1016/j.ejvs.2023.05.042
9. Investigators MRS. Aortic wall inflammation predicts abdominal aortic aneurysm expansion, rupture, and need for surgical repair. Circulation (2017) 136(9):787–97. doi: 10.1161/CIRCULATIONAHA.117.028433
10. Yuan Z, Lu Y, Wei J, Wu J, Yang J, Cai Z. Abdominal aortic aneurysm: Roles of inflammatory cells. Front Immunol (2020) 11:609161. doi: 10.3389/fimmu.2020.609161
11. Maguire EM, Pearce SWA, Xiao R, Oo AY, Xiao Q. Matrix metalloproteinase in abdominal aortic aneurysm and aortic dissection. Pharm (Basel) (2019) 12(3). doi: 10.3390/ph12030118
12. Gurung R, Choong AM, Woo CC, Foo R, Sorokin V. Genetic and epigenetic mechanisms underlying vascular smooth muscle cell phenotypic modulation in abdominal aortic aneurysm. Int J Mol Sci (2020) 21(17). doi: 10.3390/ijms21176334
13. Kim JH, Lee Y, Lee G, Doh EJ, Hong S. Quantitative interrelation between Atractylenolide i, II, and III in atractylodes japonica koidzumi rhizomes, and evaluation of their oxidative transformation using a biomimetic kinetic model. ACS Omega (2018) 3(11):14833–40. doi: 10.1021/acsomega.8b02005
14. Liu P, Zhao G, Zhang L, Gong Y, Gu Y. Atractylenolide I inhibits antibiotic-induced dysbiosis of the intestinal microbiome. Ann Transl Med (2021) 9(20):1539. doi: 10.21037/atm-21-4656
15. Xu H, van der Jeught K, Zhou Z, Zhang L, Yu T, Sun Y, et al. Atractylenolide I enhances responsiveness to immune checkpoint blockade therapy by activating tumor antigen presentation. J Clin Invest (2021) 131(10). doi: 10.1172/JCI146832
16. Li W, Zhi W, Liu F, He Z, Wang X, Niu X. Atractylenolide I restores HO-1 expression and inhibits ox-LDL-induced VSMCs proliferation, migration and inflammatory responses in vitro. Exp Cell Res (2017) 353(1):26–34. doi: 10.1016/j.yexcr.2017.02.040
17. Wang J, Zhang P, Zhang J, Ma Z, Tian X, Liu Y, et al. Atractylenolide-1 targets FLT3 to regulate PI3K/AKT/HIF1-alpha pathway to inhibit osteogenic differentiation of human valve interstitial cells. Front Pharmacol (2022) 13:899775. doi: 10.3389/fphar.2022.899775
18. Wang D, Lin Z, Zhou Y, Su M, Zhang H, Yu L, et al. Atractylenolide I ameliorates sepsis-induced cardiomyocyte injury by inhibiting macrophage polarization through the modulation of the PARP1/NLRP3 signaling pathway. Tissue Cell (2024) 89:102424. doi: 10.1016/j.tice.2024.102424
19. Xue C, Zhao G, Zhao Y, Chen YE, Zhang J. Mouse abdominal aortic aneurysm model induced by perivascular application of elastase. J Vis Exp (2022) (180). doi: 10.3791/63608
20. Zhang WL, Li N, Shen Q, Fan M, Guo XD, Zhang XW, et al. Establishment of a mouse model of cancer cachexia with spleen deficiency syndrome and the effects of Atractylenolide i. Acta Pharmacol Sin (2020) 41(2):237–48. doi: 10.1038/s41401-019-0275-z
21. Zhai L, Sheng Y, Wang J, Zhou X, Li W, Wu S, et al. Atractylenolide I suppresses A1 astrocyte activation to improve depression in mice. Mol Neurobiol (2024) 61(9):7037–45. doi: 10.1007/s12035-024-04025-7
22. Li H, Yu W, Yang Y, Li S, Xu J, Gao C, et al. Combination of Atractylenolide i, Atractylenolide III, and paeoniflorin promotes angiogenesis and improves neurological recovery in a mouse model of ischemic stroke. Chin Med (2024) 19(1):3. doi: 10.1186/s13020-023-00872-z
23. Tang D, Wang RY, Sun KW, Wu Y, Ding L, Mo Y. Network pharmacology-based prediction of active compounds in the wenyang jiedu huayu formula acting on acute-on-chronic liver failure with experimental support in vitro and in vivo. Front Pharmacol (2022) 13:1003479. doi: 10.3389/fphar.2022.1003479
24. Ullery BW, Hallett RL, Fleischmann D. Epidemiology and contemporary management of abdominal aortic aneurysms. Abdom Radiol (NY) (2018) 43(5):1032–43. doi: 10.1007/s00261-017-1450-7
25. Zhao G, Zhao Y, Lu H, Chang Z, Liu H, Wang H, et al. BAF60c prevents abdominal aortic aneurysm formation through epigenetic control of vascular smooth muscle cell homeostasis. J Clin Invest (2022) 132(21). doi: 10.1172/JCI158309
26. Zil EAA, Aziz F, Medina D, Nejim B, Radtka JF. Fenestrated endovascular abdominal aortic aneurysm repair in octogenarians is associated with higher mortality and an increased incidence of nonhome discharge. J Vasc Surg (2022) 75(6):1846–1854 e7. doi: 10.1016/j.jvs.2022.01.076
27. Wilkinson DA, Daou BJ, Nadel JL, Chaudhary N, Gemmete JJ, Thompson BG, et al. Abdominal aortic aneurysm is associated with subarachnoid hemorrhage. J neurointerv Surg (2021) 13(8):716–21. doi: 10.1136/neurintsurg-2020-016757
28. Serizawa F, Ohara M, Kotegawa T, Watanabe S, Shimizu T, Akamatsu D. The incidence of para-anastomotic aneurysm after open repair surgery for abdominal aortic aneurysm through routine annual computed tomography imaging. Eur J Vasc Endovasc Surg (2021) 62(2):187–92. doi: 10.1016/j.ejvs.2021.01.010
29. Hellawell HN, Mostafa A, Kyriacou H, Sumal AS, Boyle JR. Abdominal aortic aneurysms part one: Epidemiology, presentation and preoperative considerations. J Perioper Pract (2021) 31(7-8):274–80. doi: 10.1177/1750458920954014
30. Hultgren R, Forsberg J, Alfredsson L, Swedenborg J, Leander K. Regional variation in the incidence of abdominal aortic aneurysm in sweden. Br J Surg (2012) 99(5):647–53. doi: 10.1002/bjs.8685
31. Li FS, Weng JK. Demystifying traditional herbal medicine with modern approach. Nat Plants (2017) 3:17109. doi: 10.1038/nplants.2017.109
32. Zhai L, Sheng Y, Wang J, Zhou X, Li W, Wu S, et al. Atractylenolide I suppresses A1 astrocyte activation to improve depression in mice. Mol Neurobiol (2024). doi: 10.1007/s12035-024-04025-7
33. Fan M, Gu X, Zhang W, Shen Q, Zhang R, Fang Q, et al. Atractylenolide I ameliorates cancer cachexia through inhibiting biogenesis of IL-6 and tumour-derived extracellular vesicles. J Cachexia Sarcopenia Muscle (2022) 13(6):2724–39. doi: 10.1002/jcsm.13079
34. Qin Y, Yu Y, Yang C, Wang Z, Yang Y, Wang C, et al. Atractylenolide I inhibits NLRP3 inflammasome activation in colitis-associated colorectal cancer via suppressing Drp1-mediated mitochondrial fission. Front Pharmacol (2021) 12:674340. doi: 10.3389/fphar.2021.674340
35. Du Z, Ma Z, Lai S, Ding Q, Hu Z, Yang W, et al. Atractylenolide I ameliorates acetaminophen-induced acute liver injury via the TLR4/MAPKs/NF-kappaB signaling pathways. Front Pharmacol (2022) 13:797499. doi: 10.3389/fphar.2022.797499
36. Pei H, Du R, He Z, Bi J, Zhai L, Shen H. Atractylenolide I improves behaviors in mice with depression-like phenotype by modulating neurotransmitter balance via 5-HT2A. Phytother Res (2024) 38(1):231–40. doi: 10.1002/ptr.8045
37. Marquez-Sanchez AC, Koltsova EK. Immune and inflammatory mechanisms of abdominal aortic aneurysm. Front Immunol (2022) 13:989933. doi: 10.3389/fimmu.2022.989933
38. Saracini C, Bolli P, Sticchi E, Pratesi G, Pulli R, Sofi F, et al. Polymorphisms of genes involved in extracellular matrix remodeling and abdominal aortic aneurysm. J Vasc Surg (2012) 55(1):171–179 e2. doi: 10.1016/j.jvs.2011.07.051
39. Chen S, Luo K, Bian S, Chen J, Qiu R, Wu X, et al. Paeonol ameliorates abdominal aortic aneurysm progression by the NF-kappaB pathway. Ann Vasc Surg (2021) 77:255–62. doi: 10.1016/j.avsg.2021.06.003
40. Qiu R, Chen S, Hua F, Bian S, Chen J, Li G, et al. Betanin prevents experimental abdominal aortic aneurysm progression by modulating the TLR4/NF-kappaB and Nrf2/HO-1 pathways. Biol Pharm Bull (2021) 44(9):1254–62. doi: 10.1248/bpb.b21-00042
41. Yuan C, Yu C, Sun Q, Xiong M, Ren B, Zhong M, et al. Atractylenolide I alleviates indomethacin-induced gastric ulcers in rats by inhibiting NLRP3 inflammasome activation. J Agric Food Chem (2024) 72(25):14165–76. doi: 10.1021/acs.jafc.3c08188
42. Kong D, Mai Z, Chen Y, Luo L, Liu H, Zhao L, et al. ATL i, acts as a SIRT6 activator to alleviate hepatic steatosis in mice via suppression of NLRP3 inflammasome formation. Pharm (Basel) (2022) 15(12). doi: 10.3390/ph15121526
43. Chen LG, Jan YS, Tsai PW, Norimoto H, Michihara S, Murayama C, et al. Anti-inflammatory and antinociceptive constituents of atractylodes japonica koidzumi. J Agric Food Chem (2016) 64(11):2254–62. doi: 10.1021/acs.jafc.5b05841
44. O'Neill LA, Hardie DG. Metabolism of inflammation limited by AMPK and pseudo-starvation. Nature (2013) 493(7432):346–55. doi: 10.1038/nature11862
45. Huang R, Guo F, Li Y, Liang Y, Li G, Fu P, et al. Activation of AMPK by triptolide alleviates nonalcoholic fatty liver disease by improving hepatic lipid metabolism, inflammation and fibrosis. Phytomedicine (2021) 92:153739. doi: 10.1016/j.phymed.2021.153739
46. Barcena ML, Tonini G, Haritonow N, Breiter P, Milting H, Baczko I, et al. Sex and age differences in AMPK phosphorylation, mitochondrial homeostasis, and inflammation in hearts from inflammatory cardiomyopathy patients. Aging Cell (2023) 22(8):e13894. doi: 10.1111/acel.13894
47. Feng Y, Zhang Y, Xiao H. AMPK and cardiac remodelling. Sci China Life Sci (2018) 61(1):14–23. doi: 10.1007/s11427-017-9197-5
48. Yu Q, Zeng S, Hu R, Li M, Liu Q, Wang Y, et al. Dexmedetomidine alleviates abdominal aortic aneurysm by activating autophagy Via AMPK/mTOR pathway. Cardiovasc Drugs Ther (2023). doi: 10.1007/s10557-023-07483-8
49. Wang S, Zhang C, Zhang M, Liang B, Zhu H, Lee J, et al. Activation of AMP-activated protein kinase alpha2 by nicotine instigates formation of abdominal aortic aneurysms in mice in vivo. Nat Med (2012) 18(6):902–10. doi: 10.1038/nm.2711
50. Huang JM, Zhang GN, Shi Y, Zha X, Zhu Y, Wang MM, et al. Atractylenolide-i sensitizes human ovarian cancer cells to paclitaxel by blocking activation of TLR4/MyD88-dependent pathway. Sci Rep (2014) 4:3840. doi: 10.1038/srep03840
51. Wang M, Zhang TH, Li Y, Chen X, Zhang Q, Zheng Y, et al. Atractylenolide-i alleviates hyperglycemia-induced heart developmental malformations through direct and indirect modulation of the STAT3 pathway. Phytomedicine (2024) 129:155698. doi: 10.1016/j.phymed.2024.155698
52. Liu H, Zhang G, Huang J, Ma S, Mi K, Cheng J, et al. Atractylenolide I modulates ovarian cancer cell-mediated immunosuppression by blocking MD-2/TLR4 complex-mediated MyD88/NF-kappaB signaling in vitro. J Transl Med (2016) 14(1):104. doi: 10.1186/s12967-016-0845-5
53. Li Y, Wang Y, Liu Z, Guo X, Miao Z, Ma S. Atractylenolide I induces apoptosis and suppresses glycolysis by blocking the JAK2/STAT3 signaling pathway in colorectal cancer cells. Front Pharmacol (2020) 11:273. doi: 10.3389/fphar.2020.00273
Keywords: abdominal aortic aneurysm, atractylenolide-I, inflammation, AMPK, treatment
Citation: Chen S, Liu X, Zhou X, Lin W, Liu M, Ma H, Zhong K, Ma Q and Qin C (2025) Atractylenolide-I prevents abdominal aortic aneurysm formation through inhibiting inflammation. Front. Immunol. 16:1486072. doi: 10.3389/fimmu.2025.1486072
Received: 25 August 2024; Accepted: 08 January 2025;
Published: 31 January 2025.
Edited by:
Shuai Wang, Guangzhou University of Chinese Medicine, ChinaReviewed by:
Xiaokang Wang, Guangdong Medical University, ChinaCopyright © 2025 Chen, Liu, Zhou, Lin, Liu, Ma, Zhong, Ma and Qin. This is an open-access article distributed under the terms of the Creative Commons Attribution License (CC BY). The use, distribution or reproduction in other forums is permitted, provided the original author(s) and the copyright owner(s) are credited and that the original publication in this journal is cited, in accordance with accepted academic practice. No use, distribution or reproduction is permitted which does not comply with these terms.
*Correspondence: Keli Zhong, emhvbmdrZWxpQDEyNi5jb20=; Qiming Ma, MTE0NjkzMDI4MEBxcS5jb20=; Chengjian Qin, cWluY2hlbmdqaWFuNDdAMTYzLmNvbQ==
Disclaimer: All claims expressed in this article are solely those of the authors and do not necessarily represent those of their affiliated organizations, or those of the publisher, the editors and the reviewers. Any product that may be evaluated in this article or claim that may be made by its manufacturer is not guaranteed or endorsed by the publisher.
Research integrity at Frontiers
Learn more about the work of our research integrity team to safeguard the quality of each article we publish.