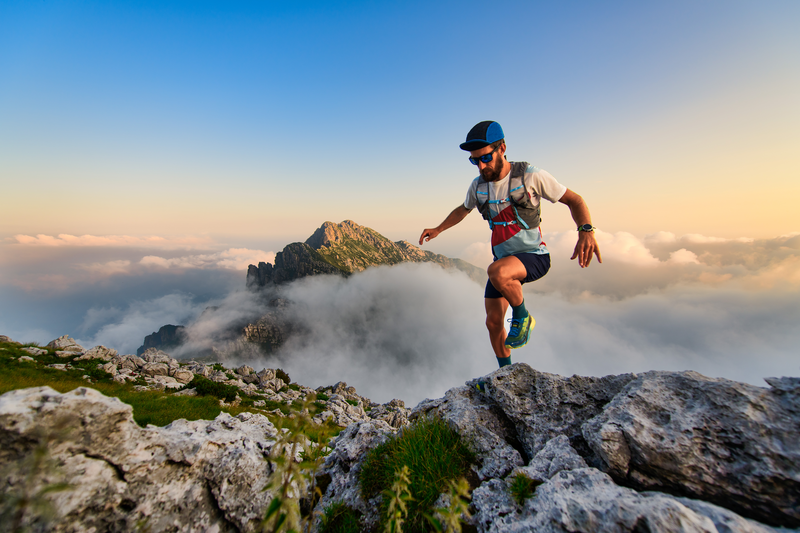
94% of researchers rate our articles as excellent or good
Learn more about the work of our research integrity team to safeguard the quality of each article we publish.
Find out more
BRIEF RESEARCH REPORT article
Front. Immunol. , 28 January 2025
Sec. Autoimmune and Autoinflammatory Disorders : Autoimmune Disorders
Volume 15 - 2024 | https://doi.org/10.3389/fimmu.2024.1516330
This article is part of the Research Topic Novel Biomarkers for Early Diagnosis, involved in Autoimmune and Autoinflammatory Diseases View all 7 articles
Background: Sjögren’s Disease (SjD) is an autoimmune disease characterized by lymphocytic infiltration of salivary and lacrimal glands (LG). The LG produces the protein-rich aqueous component of tears, and SjD-associated autoimmune dacryoadenitis (AD) may thus alter tear autoantibody composition.
Methods: The presence of tertiary lymphoid structures (TLS) in LG from two murine models of SjD-associated AD, male non-obese diabetic (NOD) and male non-obese insulitis resistant (NOR) mice, were evaluated using immunofluorescence. IgG and IgA reactivity in serum and tears from these models were probed in three studies against a panel of 80-120 autoantigens using autoantibody microarrays relative to serum and tears from healthy male BALB/c mice. Sources of Ig in tears were investigated using scRNA-Seq of the LG (GSE132420). Data were analyzed by R package Limma and Seurat.
Results: Analysis of immunofluorescence in LG sections from both SjD models showed TLS. Only one autoantibody was significantly elevated in tears and serum in both SjD models across all studies. Three autoantibodies were significantly elevated in serum but not in tears in both SjD models across all studies. Conversely, six IgG and thirteen IgA autoantibodies (6 sharing the same autoantigen) were significantly elevated in tears but not serum in both SjD models. Igha and Ighg2b expressing cells were identified in the plasma cell cluster of NOD.H2b LG.
Conclusion: NOD and NOR mice with SjD-associated AD have distinct autoantibody profiles in tears and serum. Tear IgA isotype autoantibodies showed a greater diversity than tear IgG autoantibodies. TLS observed in LG are a likely source of the tear autoantibodies.
Sjögren’s Disease (SjD) is characterized by autoimmune exocrinopathy of lacrimal glands (LG) and salivary glands (SG), leading to clinical symptoms of dry eye and dry mouth, respectively (1). Extraglandular manifestations including fatigue, musculoskeletal pain, arthralgia, interstitial lung disease, and tubulointerstitial nephritis frequently occur. SjD patients also have a significantly higher risk of developing B-cell lymphoma (2).
Pathogenesis of SjD is complex and includes genetic predisposition, environmental triggers, and activation of innate and adaptive immunity (3–5). Inflammation of LG and SG via infiltrating immune cells result in the activation of apoptotic pathways in glandular epithelial cells, releasing autoantigens such as ribonucleoprotein complexes Ro/SSA and La/SSB, which further recruit dendritic cells to the exocrine glands and lead to a cycle of inflammation (2). Detection of Ro/SSA autoantibodies in serum is part of the clinical diagnosis of SjD (6). Although the presence of serum SSA autoantibody is weighted equally to a positive SG biopsy in the American-European consensus group (AECG) classification criteria for SjD (7), SSA is not specific to SjD and is elevated in other systemic autoimmune diseases (8–10).
Tear autoantibodies may have potential as diagnostic tools denoting ocular involvement in SjD. It is established that tertiary lymphoid structures (TLS) form in the LG and SG in SjD (11). These localized TLS can generate autoantibodies distinct from those found systemically in disease. As the LG is a target organ in SjD, with autoimmune dacryoadenitis (AD) leading to changes in tear composition and volume, it is possible that the presence of specific tear autoantibodies could aid in distinguishing SjD from other autoimmune and dry eye diseases. Small scale studies of autoantibodies in tears (12, 13) and saliva (14) have shown that SSA and SSB antibodies are detected in these biofluids in some seronegative patients, suggesting that tear and salivary SSA and SSB autoantibodies may be more sensitive than serum measurements. Further, symptoms of dry eye and dry mouth correlate highly with markers of inflammation in the tear fluid and saliva, respectively (15).
To understand the similarities and differences between serum and tear autoantibody expression during disease development in SjD, we analyzed tears and serum from two related murine models relative to healthy control BALB/c mice. The male non-obese diabetic (NOD) mouse spontaneously develops SjD-like AD (16) and has served as a source for identification of tear biomarkers that have been validated in human subjects (17–19). The NOD strain is reported to express serum autoantibodies to SSA and SSB (20). To account for potential confounding factors from diabetes development that occur late in this strain, we also used the haplotype-matched NOD-derived male non-obese diabetes resistant NOR/Ltj mouse (21) to investigate autoantibody composition of tear fluid and serum. The NOR mouse is a recombinant congenic strain with limited regions of the NOD/LtJ genome replaced by the genome of the C57Bl/KsJ strain, resulting in maintenance of SjD-like AD but loss of the late-developing diabetes phenotype (21). We have previously characterized SjD-like AD development in both male NOD and NOR mice, which showed marked similarities (22). Both NOD and NOR strains exhibit sexually dimorphic disease development, where males develop earlier and more severe AD than females, and females develop earlier and more severe autoimmune sialadenitis than males (22–25). Since our primary focus is in SjD-associated AD, we used male mice in this study which recapitulate LG disease. Our analysis identified a few autoantibodies common to tears and serum, but, strikingly, found additional discrete autoantibodies elevated in tears or in serum in these models of SjD.
Three autoantibody microarrays were performed between 2020 and 2022. Both male NOD (#001976, Jackson Laboratory, Sacramento, CA) and male NOR (#002050, Jackson Laboratory, Sacramento, CA) mice were utilized as models of AD in SjD, with healthy male BALB/c (#000651, Jackson Laboratory, Sacramento, CA) as controls. Consistent with previous work (16, 22, 24, 26), NOD/NOR mice aged 8-12 weeks were considered to have early disease, mice aged 12-20 weeks to have intermediate disease, and mice aged >20 weeks to have advanced disease (26). In Study 1, serum was collected from male NOD and BALB/c mice with early (n=3/strain) and advanced (n=3/strain) disease. As these studies showed no major differences in serum autoantibody development across this broad age range in NOD mice, we used mice with intermediate disease for subsequent studies to minimize both the minor variability in time of disease onset and comorbidities occurring later in development (27). For Study 2, tears and serum were collected from male NOR mice with intermediate disease (n=6) and healthy control male BALB/c mice (n=3). While serum autoantibodies were subjected to a complete analysis, tears were pooled from one, two or three mice to assess the limits of detection. For Study 3, tears and serum were collected from male NOD (n=5) and male NOR (n=4) mice with intermediate disease and age-matched male BALB/c mice (n=6). All study procedures conducted on animals were pre-approved by the University of Southern California’s Institutional Animal Care and Use Committee (IACUC) and were conducted in accordance with the GUIDE for Care and Use of Laboratory Animals (8th Edition (28)). ARRIVE checklist is included in Supplementary Material.
Mice were anesthetized with Ketamine (60-70 mg/kg)/Xylazine (5-10 mg/kg). Tears were collected using capillaries as described previously (29) following topical carbachol administration to the LG and flash frozen. 0.5-1 mL of blood was collected by cardiac puncture using a 1 mL syringe and added to MiniCollect 0.8 mL gold cap Serum Separator tubes (Greiner Bio-One, Kremsmünster, Austria). After centrifuging the blood at 4°C, 1500 x g for 15 min, serum was obtained and stored at -80°C. For tissue collection, anesthetized mice were euthanized by cervical dislocation and LG were collected and fixed as described below.
For immunofluorescence labeling, LG were collected from male NOR and NOD mice with intermediate disease. LG were fixed, frozen in OCT, sectioned and prepared for immunolabelling as described (19). Sections were incubated overnight at 4˚C with the following primary antibodies: anti-CD3e (T-cell marker, Hamster Anti-Mouse CD3e 500A2, catalog #553238, BD Biosciences, Franklin Lakes, NJ), anti-IgD (Alexa Fluor® 647 anti-mouse IgD antibody, 11-26c.2a, catalog #405708, Biolegend, San Diego, CA), anti-B220 (B cell marker, rabbit anti-mouse RA3-6B2, catalog #MA5-48137, Thermofisher Scientific, Waltham MA), anti-CD21/CD35 (catalog# 13-0211-82, Thermofisher Scientific) and anti-PNAd carbohydrate epitope (Glycam-1 marker, catalog# 553863, BD Biosciences Franklin Lakes, NJ). Sections without primary antibodies served as negative controls. Slides were washed 3x with 0.5% Tween 20 in PBS and incubated with compatible secondary antibodies and additional probes: Alexa Fluor® 568 goat anti-hamster IgG (1:1000, catalog #A-21112), Alexa Fluor® 488 goat anti-rabbit (1:200, catalog# A-11008), Alexa Fluor® Plus 488 donkey anti-rat (1:200, catalog# A48269TR); Alexa Fluor™ Plus 647 Phalloidin (1:200, catalog# A30107), and DAPI (catalog #D1306), all from Thermofisher Scientific, at 37°C for 1 h then washed 3x with 0.5% Tween 20 in PBS (catalog# 18912014, Thermofisher Scientific). Slides were mounted with ProLong anti-fade mounting medium (catalog# P36934, Thermofisher Scientific). Images were acquired using either a ZEISS LSM 800 confocal microscope equipped with an Airyscan detector (Zeiss, Thornwood, NY) or a ZEISS Axioscan 7 Microscope Slide Scanner and analyzed using open source Qupath software version 0.5.1. and imageJ 1.54g.
Autoantigen microarrays were manufactured in the Microarray & Immune Phenotyping core Facility at the University of Texas Southwestern Medical Center (Dallas, TX, USA) and were processed as described previously (30, 31). A selection of autoantigens was made based on published literature of prior known autoantibodies (32, 33). Positive controls were also imprinted on the arrays. Each slide contained 16 identical arrays, enabling processing of 15 samples and PBS as a negative control. Arrays contained 80-120 autoantigens as well as internal controls. Mouse serum or tear samples were treated with DNAse I to remove free-DNA and then applied to autoantigen arrays at a 1:50 dilution. After incubation, autoantibody binding to the antigens on the array was detected at 532 nm (with Cy3 labeled anti-IgG) and, when IgA was analyzed, at 635 nm (with Cy5 labeled anti-IgA) to generate.tiff images. Genepix Pro 7.0 software was used to analyze the images and generate genepix report (GPR) files (Molecular Devices, Sunnyvale, California, USA). The net fluorescent intensity (NFI) of each antigen was generated by subtracting the local background and negative control (PBS) signal. The signal-to-noise ratio (SNR) was also generated for each antigen. Autoantibodies with SNR values of <3 in more than 90% of the samples were considered negative and excluded from further analysis. NFI was normalized with robust linear model using positive controls with different dilutions. NFI and SNR values as well as the R code used in analyzing the data are described in Supplementary Methods S1.1-1.6.
After filtering autoantibodies with SNR <3 for a given mouse group in each array, remaining NFIs were further normalized by fitting a quantile regression by using the Limma (34) voom package for Studies 2 and 3 or the code provided in the Supplementary Methods S1.1 based on Kiripolsky et. al (35). for Study 1, depending upon the distribution of the raw data. Normalized values were log transformed and scaled using the biweight midvariance method to account for variances in scaling. Differential autoantigen expression was determined using the Wilcoxon Rank Sum test and correction for multiple comparisons was done using the Benjamini and Hochberg procedure. The Spearman correlation coefficient was calculated for IgG vs IgA in mouse tears in Study 3. scRNAseq data was generated by Rattner et al. from LG of 12 week old male NOD.H2b (another NOD substrain lacking diabetes that develops SjD-associated symptoms in LG) (36), and age matched male BALB/c controls. Raw bam files were obtained from the European Nucleotide Archive (ENA: PRJNA548013) and raw fastqs were regenerated using ‘bcl2fastq’ and aligned to mouse genome GRCm39 using 10X’s cellRanger 8.0.1. Raw matrix files were analyzed using Seurat. R code used in analyzing the scRNAseq data are described in Supplementary Methods S2.
TLS can form within target organs undergoing chronic inflammatory/autoimmune processes, whose primary function is not lymphogenic in nature. Like germinal centers (GC), they are lymphoid structures where memory lymphocytes and/or precursors can be re-stimulated by antigen which results in clonal expansion (37). We have also shown that miR-155-5p, a marker of GC development (38), is significantly upregulated in the LG of male NOD mice, particularly in the infiltrating lymphocytes (39). In this report, we evaluated the presence of TLS in LG of two murine models of AD in SjD, male NOD and NOR mice. Figure 1 shows representative images from LG from mice of each strain with intermediate disease. Labeling of lymphocytic infiltrates with IgD, a marker of naive follicular B-cells (40, 41) (Figure 1A), and B220, a general marker for B cells (42) (Figure 1B), showed enriched B cell zones enveloping CD3e positive T cells (Figures 1A, B) in the LG. Some foci showed a clear segregation of T and B cells in a GC-like manner. Higher magnification images of TLS in LG from these mouse strains are shown in Figure 1C. These infiltrates were also enriched in other components of TLS including markers of high endothelial venules such as PNAd (43) (Figure 1D) and highly compartmentalized CD21/CD35 positive follicular dendritic cells (Figure 1D). TLS were detected in LG from both strains at early, intermediate and advanced disease, increasing gradually with age (data not shown). For reference, H&E stained LG sections from these same strains at the same age are shown in Supplementary Figure S1.
Figure 1. Representative images of tertiary lymphoid structures (TLS) in LG of male NOD and NOR mice with intermediate disease. Immunofluorescence (IF) images show components of TLS as follows:(A) Antibodies to IgD highlighting follicular B cells (green) and CD3 highlighting T cells (red). DAPI labels nuclei (blue). (B) Antibodies to B220 highlighting B cells, (green) and CD3 highlighting T cells (red). DAPI labels nuclei (blue). Scale bars in (A, B), 500 μm. (C) Higher magnification images of representative TLS in NOD and NOR mouse LG. Left panel shows antibodies to IgD highlighting follicular B cells (green) and CD3 highlighting T cells (red). Right panel shows antibodies to B220 highlighting B cells (green) and CD3 highlighting T cells (red). Scale bar, 100 μm. (D) Left panel shows antibody to PNAd, highlighting high endothelial venules (green) and Alexa Fluor™ Plus 647 Phalloidin highlighting F-actin (red). DAPI labels nuclei (blue). Right panel shows antibodies to CD21/CD35, highlighting follicular dendritic cells (green) and Alexa Fluor™ Plus 647 Phalloidin highlighting F-actin (red). DAPI labels nuclei (blue). Scale bars, 50 μm.
Three separate arrays were conducted to compare IgG autoantibody abundance in both tears and serum from male NOD and NOR mice versus healthy control BALB/c mice. A complete list of autoantigens, their abbreviations, and disease association is provided in the Supplementary Data Sheet S1 file. Study 1 compared serum autoantibody abundance from mice with early and advanced disease in male NOD mice versus age-matched healthy control BALB/c mice. NOD mice at both disease states exhibited significantly increased serum IgG reactivity against four common autoantigens relative to age-matched healthy male BALB/c mice (Supplementary Table S1). These included – Polymyositis/Scleroderma antigen 100 (Pm/Scl-100), Proliferating Cell Nuclear Antigen (PCNA), Anti-liver cytosolic antigen type 1 (LC-1), and Heterodimer Ku protein subunits 70 & 80 (KU P70/P80). Additionally, Small RNA Binding Exonuclease Protection Factor La (La/SSB), threonyl-tRNA synthetase (PL7), Thyroid Peroxidase (TPO), Intrinsic Factor (IF) and Mitochondrial Antigen had significantly elevated serum IgG reactivity at early disease, with greater variability in advanced disease (Supplementary Table S1). For each of the nine antigens above, NOD mice with early and advanced disease showed the same significant differences between healthy (BALB/c) and diseased (NOD) mice (Supplementary Table S1). Therefore, age was not considered a confounder for development of these autoantibodies. The common elevation of these autoantibodies across the spectrum of SjD-associated AD development suggests that they form early in disease and remain elevated. These findings further suggested that use of mice with intermediate disease might capture changes characteristic of AD in SjD, but avoid confounders associated with advanced disease and development of comorbidities occurring >20 weeks of age (27).
Other autoantibodies were elevated both in the serum of advanced disease NOD mice and age-matched BALB/c mice, namely Signal Recognition Particle 54 kDa protein (SRP54) and Major centromere autoantigen B (CENP-B), (Supplementary Figure S2). This suggests their formation is dependent on age rather than on disease progression. Additionally, a slight decrease was observed in PM/Scl-100 and Glycated Albumin autoantibodies with age (Supplementary Figure S2).
In Study 2, we used NOR mice with intermediate disease. We identified increased immunoreactivity in NOR mouse serum relative to serum of healthy age-matched male BALB/c mice against sixteen autoantigens (Supplementary Table S2). Figure 2A shows the combined results of the analyses from Studies 1 and 2, which highlights elevations in serum autoantibodies common to both NOD and NOR mice. Common IgG autoantibodies distinct to serum included PM/Scl 100, LC1, La/SSB, Mitochondrial Antigen and PCNA, relative to serum from healthy BALB/c controls. In Study 3, we reconfirmed IgG and added IgA immunoreactivity against 80 autoantigens in serum from male NOD and NOR mice with intermediate disease relative to healthy BALB/c controls. This study validated significantly increased serum IgG reactivity against LC1, PCNA and La/SSB in both male NOD and NOR mice (Figure 2B) compared to BALB/c. Thus, these serum autoantibodies were common to NOD and NOR mouse serum across all studies. In addition, arrays used in Study 3 had antigens not present in previous studies including several exclusive to serum (Supplementary Figure S3, Supplementary Table S3).
Figure 2. Differential IgG autoantibody expression in serum in male NOD and NOR mice. (A) Box plots showing autoantibodies significantly increased in serum of male NOD (N=6) mice as compared to male BALB/c (N=6) mice in two different age groups from Study 1 (left graphs). Box plot showing log normalized intensities of autoantibodies significantly upregulated in serum of male NOR mice with intermediate disease (N=6) as compared to age-matched male BALB/c mice (N=3) from Study 2 (right). (B) Box plots showing validated serum IgG autoantibodies increased in serum only from male NOD (n=5) and male NOR (n=4) mice with intermediate disease and age-matched male BALB/c mice (n=6) from Study 3. Moderated t-statistics estimated using Limma R package. (* padj < 0.05, ** padj < 0.01, *** padj < 0.001, **** padj < 0.0001). LC1, Anti-liver cytosolic antigen type 1; PM/Scl-100, polymyositis/Scleroderma antigen 100; PCNA, Proliferating Cell Nuclear Antigen; La/SSB, Small RNA Binding Exonuclease Protection Factor La.
Compared to total serum IgG, total serum IgA was detected at a significantly lower level in male NOD and NOR mice (p < 10-4, One-way ANOVA, Supplementary Figure S4A). The only antigens with significantly elevated IgA reactivity in serum of both NOD and NOR mice with intermediate disease were intrinsic factor (IF) and single stranded DNA (ssDNA) (Supplementary Figure S4B). A modest increase was also observed for Cytochrome C (Cyt C), Fibrinogen and Glycoprotein 2 (GP2) (Supplementary Table S3).
Studies 2 and 3 included tear samples in addition to serum. Several IgG autoantibodies were upregulated in both serum and tears, including autoantibodies to PL7 and KU (P70/P80) in Study 2 (Supplementary Figure S5A), and PL7, Glutamic Acid Decarboxylase 65 (GAD65), and Liver kidney microsome type 1 (LKM1) in Study 3 (Supplementary Figure S5B). The only common autoantibody seen in both Studies 2 and 3 in serum and tears was to PL7. Additional serum autoantibodies, including against intrinsic factor (IF) may be biologically relevant as they were increased across multiple assays and different antibody isotypes but not significant in Study 2 (Supplementary Table S2).
We explored the tear autoantibody profile of IgG and IgA isotypes in tears of both male NOD and NOR mice with intermediate disease versus tears of healthy control BALB/c mice (Table 1). In Study 3, we found 6 IgG autoantibodies that were significantly upregulated in tears of both NOD and NOR mice. These include autoantibodies to Histidyl-tRNA synthetase (Jo-1), Anti-Islet Cell Antigen 512 (IA-2), Tissue Transglutaminase 2 (tTG), Dermatomyositis specific autoantigen Mi-2 (Mi-2), thyroid peroxidase (TPO) and SAE1/SAE2 (Figure 3A). In Study 3, IgA reactivity was tested against the same panel of 80 autoantigens. There was significantly higher IgA reactivity against 14 autoantigens in both male NOD and NOR mouse tears relative to healthy BALB/c tears, of which 13 were unique to tears. These included autoantibodies to tTG, Mi-2, Jo-1, IA-2, GP2, SAE1/SAE2, TPO, PL-7, KS, GAD65, LKM1, La/SSB and Gliadin (Figure 3B). While there was largely an agreement between the upregulated IgG and IgA autoantibody species (Table 1), tears of male NOR mice had significantly higher total IgA than total IgG (padj = 0.0495, two way ANOVA, post-hoc dunn's test), with comparable levels of IgA and IgG in the healthy control male BALB/c (Supplementary Figure S6A). Reactivity to IgA control was significantly higher than reactivity to IgG controls in tears of all mice but was relatively higher in the male NOD and NOR mice compared to BALB/c (Supplementary Figure S6B). Positive correlations between the signal intensities for IgGs and IgAs to the same autoantigens were observed for 70 out of 80 autoantigens tested (Supplementary Methods S1.4) 56 of which were statistically significant. The highest positive correlations were observed for IgGs and IgAs to the six shared epitopes in NOD and NOR, but not for BALB/c controls (Supplementary Figure S7).
Figure 3. Differential autoantibody expression of autoantibodies unique to tears of male NOD and NOR mice. (A) IgG autoantibodies that are unique to tears from male NOD (N=5) and male NOR (N=3) mice with intermediate disease versus male BALB/c (N=3) mice from Study 3. Moderated t-statistics estimated using Limma R package. (B) Differentially expressed IgA autoantibodies in tears of the same male NOD, male NOR and BALB/c mice from Study 3. Moderated t-statistics estimated using Limma R package (ref). p-values adjusted by Benjamini and Hochberg procedure to account for multiple comparisons (* padj < 0.05, ** padj < 0.01, *** padj < 0.001, **** padj < 0.0001). Jo-1, Histidyl-tRNA synthetase; IA-2, protein tyrosine phosphatase-like Anti-Islet Cell Antigen 512; tTG, Tissue Transglutaminase 2; GAD65, Glutamic Acid Decarboxylase 65; LKM1, Liver kidney microsome type 1; Mi-2, Dermatomyositis specific autoantigen Mi-2; PL-7, threonyl-tRNA synthetase; TPO, Thyroid peroxidase; GP2, Glycoprotein 2; IF, Intrinsic Factor; KS, anti-asparaginyl-tRNA synthetase or KS; La/SSB, Small RNA Binding Exonuclease Protection Factor La; SAE1/SAE2, SUMO1 activating enzyme subunit 1/2.
Table 2 summarizes all of the significant autoantibody findings from the study. Serum expression of SSA and SSB autoantibodies is observed in ~25-75% of SjD patients (44, 45), respectively, but are also detected in patients with systemic lupus erythematosus and rheumatoid arthritis (9, 46). This lack of sensitivity and specificity is a challenge for SjD diagnosis. The identification of additional potential diagnostic autoantibodies in SjD has been previously investigated, with researchers reporting serum autoantibodies to SP1, Ca6 or PSP with better sensitivity than antibodies to Ro or La (47). In this study we show for the first time that two related murine disease models of SjD exhibiting AD, the male NOD and NOR mice, express characteristic serum and tear autoantibodies that are largely distinct. The autoantibodies unique to serum were primarily IgG autoantibodies but the autoantibodies unique to tears included IgG and IgA isotypes. Interestingly, the autoantigens recognized by tear IgGs were all represented in tear IgAs.
Table 2. Summary of upregulated IgG and IgA autoantibodies in serum only, tears only or in both serum and tears.
While murine models do not completely recapitulate all features of human disease, the NOD mouse in particular has been used to generate many findings validated in SjD patients, and/or to recapitulate findings made in SjD patients, particularly with respect to LG and SG disease. NOD mice exhibit significant AD and autoimmune sialoadenitis, as well as reduced tear and salivary flow (22, 48–52), which are defining characteristics of SjD patients. A series of novel autoantibodies to three salivary gland proteins, salivary gland protein 1 (SP-1), carbonic anhydrase 6 (CA6) and parotid secretory protein (PSP) identified in 2 SjD murine models including the NOD mouse were subsequently validated in 45% of SjD patients meeting clinical diagnostic criteria who lacked autoantibodies to Ro or La (47). In particular, these anti-CA6 autoantibodies appear to delineate a particular SjD population with DED having younger disease onset and more severe disease (53). NOD mice also provided initial evidence for elevated cathepsin S levels (29) and decreased cystatin C levels (17) in tears, both of which which were subsequently validated in SjS patients (17, 18). NOD mice also provided evidence for mislocalization of aquaporin 5 in exocrine glands (54), which again is seen in SjD patients (54). While exploration of the expression of these new autoantibodies identified in NOD and NOR mice in the tears and serum of SjD patients is a future goal, past similarities in disease manifestation and biomarker discovery and validation suggest our findings have potential for clinical impact.
Tear biomarkers are of increasing interest in vision science and other diseases, since changes in the tear film are correlated with multiple conditions ranging from SjD (17, 18), dry eye disease (55), Parkinson’s disease (56, 57) and Alzheimer’s disease (58). Tear biomarker tests offered in a clinical laboratory-based format could use collection methods already in clinical use in optometry and ophthalmology clinics. For instance, the Schirmer’s test uses a filter-paper strip marked in mm to measure tear flow, and many biomarker discovery studies are based on adaptation of this strip for sample collection, and subsequent elution and measurement of the marker of interest. The currently approved InflammaDry test is a clinically-approved point of care test (55) that measures increased matrix metalloproteinase 9 levels indicative of dry eye disease. The InflammaDry test uses microfiltration technology on a Schirmer’s-like collection strip combined with a simple colorimetric indicator that can be visually assessed relative to a standard, similar to a pregnancy or COVID-19 test. Such tests would not require special equipment and could be readily incorporated into specialties such as rheumatology, with minimal additional training. Ongoing challenges in the tear biomarker field include those of standardization, which may be specific to the nature of the biomarker (59). Given the limited volume available in patients with dry eye disorders, like SjD, sensitivity of detection of the biomarker is also important. We have focused on analysis of autoantibodies in mice of intermediate disease, after confirming similar patterns of serum autoantibody expression in early and late stage disease in Study 1. The common SjD serum autoantibodies (SSA, SSB, ANA, RF) are found in patients as early as 20 years prior to development of disease signs and symptoms. A study by Theander et al. showed that at least one autoantibody was detected pre-disease in 80% of patients (60). Although autoantibody titers may vary, the autoantibody profile is often stable over time (61). As we did not detect significant differences in the serum autoantibody profile between early and advanced disease groups in Study 1, we chose to focus on intermediate disease. We felt that mice at this stage would 1) have a more robust formation of LG TLS than at early stages, and 2) might exhibit fewer confounders associated with development of symptoms in other organs. Since tear autoantibodies are less well understood, it is possible that their autoantibody profile is more dynamic than in serum. Future studies will explore the time course of development of these autoantibodies across a more comprehensive disease range.
IgA is the second most common antibody isotype in serum, after IgG (62). Nearly 90% of the IgA found in serum is monomeric IgA1, produced in the bone marrow, which plays no role at the mucosal surface. However, the IgA found in mucosal tissues, such as the LG is secreted as dimeric IgA (dIgA), produced by resident plasma cells in the glandular interstitium. dIgA binds to polymeric Immunoglobulin Receptor (pIgR) expressed on the basolateral membrane of polarized LG acinar cells and is transcytosed to the apical surface where pIgR is cleaved and dIgA is released into tears as secretory IgA (sIgA) (63). In SjD, TLS are found in LG (64). IgA autoantibodies generated by solitary plasma cells at early disease stages as well as from local TLS in established disease would therefore be recovered in tears due to this robust active transport process.
IgG present in tears is either produced locally in the LG or originates from the circulation. Since distinct IgGs are present in tears relative to serum, it is unlikely that tear IgGs originate from the circulation. Previous studies have shown that the number of IgG producing plasma cells in salivary gland biopsies from SjD patients increases with disease progression (62), reducing the IgA to IgG ratio. We have analyzed published data from a single cell RNA-Seq study on LG from healthy male BALB/c and male NOD.H2b disease model mice (another NOD substrain lacking diabetes that develops SjD-associated LG disease) (36). As shown in Supplementary Figures S8A, B, the population of naïve B cells and plasma cells in the LG is markedly increased in NOD mouse, and NOD mouse plasma cells show a significant increase in IgG production (based on Ighg2b gene expression). Analysis of genes encoding IgA, IgM and IgG genes in different B cell populations from the LGs support the increased local expression of IgG (Supplementary Figure S8C). IgG is not known to be actively transported, and its presence in tears is likely due to passive paracellular transport, a pathway recently reported to occur in LG (63). Paracellular leakage may increase with SjD progression. Disruption of tight junctions is demonstrated in salivary glands of SjD patients and NOD mice, facilitated by proinflammatory cytokines such as Il-17A. degradation of extracellular matrix, epithelial cell apoptosis, and diminishing tissue organization (61).
The plausibility of detecting SjD autoantibodies in tears was previously shown in a small study by Toker et. al (12) that demonstrated that roughly 16 of the 28 patients positive for serum SSA and 14 of the patients positive for serum SSB were positive for SSA and SSB respectively, in tears. However, this study only investigated IgG autoantibodies. The Toker study and similar studies conducted in saliva have also shown the presence of SSA and SSB in tears or saliva of seronegative patients (12–14). To our knowledge, no investigations have studied the complete spectrum of autoantibodies present in tears, nor compared them to the spectrum of serum autoantibodies in SjD patients or SjD disease models. We found that the autoantibody repertoire of male NOD and NOR mice tears was quite distinct from that found in their serum. Increased IgG and IgA reactivity to Jo1, IA2, tTG, Mi-2, TPO and SAE1/SAE2 was seen only in tears, but not in serum.
In the healthy eye, conjunctiva associated lymphoid tissue (CALT) comprises a secondary lymphoid organ (SLO) that is crucial to ocular defense against pathogens. Changes in number and size of CALT in healthy mice are seen upon exposure to pollen (65), induction of dry eye disease (65) or with age (66). The autoantibodies observed in the tears of healthy male BALB/c mice in our study may be produced by CALT, in addition to resident LG plasma cells in order to maintain ocular health. On the other hand, TLS, while similar to SLO, develop in organ-specific autoimmune diseases such as SjD (37, 67) where they are not normally present. Their formation involves B-cell activation and differentiation into autoantibody producing plasma cells via a series of somatic hypermutation and class switch recombination of the Ig genes (1). These structures actively support molecular machinery responsible for pathogenic autoantibody production. Our data show the presence of characteristic TLS within LG of male NOR and NOD mice. We also observe expression of other components of TLS including PNAd, a marker of high endothelial venules in the dark zone (43), and CD21/CD35, a marker of follicular dendritic cells of the light zone (68). The tear-specific IgA and IgG autoantibodies seen in NOD and NOR mice may be largely produced from LG TLS, although a recent study has also suggested that CALT of patients with autoimmune eye-related diseases including SjD and graft-versus-host disease can produce tear autoantibodies to citrullinated proteins (69). In either case, tear IgA autoantibodies appear to be generated locally since they differ both in isotype and antigen from those expressed in serum in the same animals.
An important question is to understand how tear autoantibodies are related to disease development and progression in SjD. Many studies have suggested that the autoantigens triggering production of SSA and SSB autoantibodies, Ro and La, are released locally in exocrine tissue due to early disease triggers (viral infection, ER stress, apoptosis) where they can be processed in TLS to generate autoantibodies (70). Other anatomical features of TLS suggest that local TLS are most likely to express local autoantigens (37). For instance, early events in antigen presentation in exocrine glands are facilitated by the epithelial cells themselves, through the process of autoimmune epithelitis (71). TLS may have poorly developed lymphatics relative to the more organized SLO in lymphoid tissue. In the absence of these lymphatics, mature dendritic cells carrying antigen from other peripheral sites may not be able to reach TLS.
With this process in mind, we considered the common IgA and IgG tear autoantigens– Jo-1, IA2, tTG, Mi2, SAE1/SAE2 and TPO. Synergism of IgG and IgA autoantibodies to common autoantigens is known to potentiate interferon responses in systemic lupus erythematosus (72). Given the high degree of correlation observed between IgG and IgA for these six shared antigens in tears of SjD model mice, their role in synergistically propagating autoimmunity in LG requires further investigation. The same apoptotic processes that may lead to exposure of Ro and La may expose additional autoantigens leading to autoantibody development to Jo-1, tTG, Mi2 and SAE1/SAE2. Anti-Jo-1 IgG and IgA autoantibodies target histidyl-tRNA synthetase (73), a major biosynthetic enzyme. Serum IgG elevation of this antigen is associated with anti-synthetase syndrome (74–76). Mi2 autoantigen is a key marker of antimitochondrial autoantibodies. These antibodies have been strongly associated with primary biliary cholangitis (44). Notably, anti-Jo-1 and Mi2 IgG were found to be absent in sera of 26 SjD patients and were only detected in patients with myositis (77), Similarly, anti- SAE1/SAE2 IgG is reported to be exclusively found in serum of patients with Myositis. Tissue transglutaminase (tTG) is an abundant protein found in multiple tissues in both intracellular and extracellular spaces that catalyzes protein cross linking (78). IgA autoantibodies to tTG are commonly used to diagnose celiac disease in children (79). However, it is notable that autoantibodies to this protein are detected in saliva from SjD (80).
It is unclear how local autoantigens could contribute to tear autoantibodies to thyroid peroxidase (TPO), a tissue-restricted thyroid secretory protein important in generation of thyroglobulin or IA-2, an islet-specific autoantigen for type 1 diabetes (81, 82). Autoantibodies to TPO are commonly seen in autoimmune thyroid diseases such as Hashimoto’s disease (83). Interestingly, diabetes, autoimmune thyroiditis, myositis (84, 85) and primary biliary cholangitis (associated with autoantibody to Mi2, above) have been explored as potential co-morbidities in SjD (27, 86), Autoimmune thyroiditis is a co-morbidity in the NOD mouse in 5-15% of animals (27), while diabetes is a defining phenotype in the NOD mouse. While the presence of autoantibodies to these proteins is unsurprising given co-morbidities of the disease model, their concentration in tears instead of in serum is surprising.
Some non-traditional serum autoantibodies can be associated with SjD in patients with overlapping co-morbidities (87). What is unusual about our findings is the discovery of these autoantibodies in tears. All of our results suggest that tear autoantibodies are produced locally, implying that they originate in TLS present in the LG. LG TLS are unique to the SjD disease phenotype. Thus, the detection of these autoantibodies in tears should be highly specific for SjD while also suggesting the possible involvement of comorbidities in other organs. Although serum autoantibodies are a common finding in rheumatic autoimmune diseases, their exact role in pathogenesis remains unclear. Autoantibodies against extracellular regions of proteins can cause pathogenicity if they disturb the function of the target protein. In fact, this has been proposed as one way to distinguish the pathogenic versus non-pathogenic potential of antibodies, by evaluating whether autoantigen is exposed or present within cells (88). Other work even categorizes the types of pathogenicity associated with different types of autoantibody interactions with external epitopes (89). Most of the antigens identified in this study are directed against intracellular proteins, avoiding immediate categorization as potentially pathogenic. However, despite evidence that the autoantibodies present in serum, tears, and saliva are produced in the affected exocrine glands (71), the direct contribution of autoantibodies to exocrine gland dysfunction in SjD has not yet been shown. It is becoming increasingly clear that the exocrine epithelial cells play a crucial role in in the pathogenesis of the disease, as they accrue antigen-presenting properties. They may also facilitate exposure of intracellular antigens by secretion of exosomes containing autoantigenic proteins, such as Ro/SSA and La/SSB or through the release of autophagic blebs containing antigenic proteins due the enhanced cell death. The presence of certain autoantibodies have also been linked to specific clinical manifestations (87). Some autoantibodies present in tears may also have direct pathological effects on the ocular surface; for instance, Kwon et al. showed that anti-citrullinated protein antibodies in tears contribute to ocular surface inflammation in autoimmune-mediated dry eye (69).
Previously, studies have found salivary anti-muscarinic receptor autoantibodies had higher sensitivity in diagnosing younger patients with SjD at an earlier stage of disease progression (90). Another study validated salivary anti-histone and anti-tTG autoantibodies in 34 patients with SjD with an area under the receiver operator curve (AROC) of 0.87 or higher (80), which is significantly better than the AROC for serum SSA and SSB. Adding tTG and histone autoantibodies to salivary Ro and La gave an AROC of 0.99 (80). This study also found significant elevation of anti-cardiolipin autoantibodies in SjD patient saliva when compared to healthy controls and SLE patients. This lends further credence to the utility of testing local biofluids in SjD. Thus, we propose tear autoantibodies may outperform serum biomarkers in sensitivity and specificity in reflecting ocular manifestations of SjD. Future studies will explore additional aspects of tear autoantibody development in mice including variability in their time course of expression, and their sensitivity to treatments that reduce TLS in the LG. Importantly future studies will also explore their abundance in tears of SjD patients.
The datasets analyzed for this study can be found in the GEO: GSE286502, GSE286503, GSE286504.
The animal study was approved by Institutional Animal Care and Use Committee (IACUC), University of Southern California. The study was conducted in accordance with the local legislation and institutional requirements.
SK: Conceptualization, Data curation, Formal analysis, Investigation, Methodology, Software, Validation, Visualization, Writing – original draft, Writing – review & editing. SA: Data curation, Visualization, Writing – original draft, Writing – review & editing. YJ: Data curation, Writing – review & editing, Methodology. JM: Funding acquisition, Writing – review & editing. ME: Conceptualization, Methodology, Supervision, Writing – original draft, Writing – review & editing. IR: Data curation, Methodology, Writing – review & editing. CZ: Data curation, Methodology, Software, Writing – review & editing. PR: Methodology, Resources, Writing – review & editing. SH: Conceptualization, Funding acquisition, Investigation, Project administration, Resources, Supervision, Writing – original draft, Writing – review & editing.
The author(s) declare financial support was received for the research, authorship, and/or publication of this article. This work was supported by RO1 EY011386 to SHA and R01 EY026635 to SH and JM. Further support for the project came from P30EY029220, and an unrestricted departmental grant from Research to Prevent Blindness (RPB), New York, NY 10022.
The authors declare that the research was conducted in the absence of any commercial or financial relationships that could be construed as a potential conflict of interest.
The author(s) declared that they were an editorial board member of Frontiers, at the time of submission. This had no impact on the peer review process and the final decision.
The author(s) declare that no Generative AI was used in the creation of this manuscript.
All claims expressed in this article are solely those of the authors and do not necessarily represent those of their affiliated organizations, or those of the publisher, the editors and the reviewers. Any product that may be evaluated in this article, or claim that may be made by its manufacturer, is not guaranteed or endorsed by the publisher.
The Supplementary Material for this article can be found online at: https://www.frontiersin.org/articles/10.3389/fimmu.2024.1516330/full#supplementary-material
1. Brito-Zeron P, Baldini C, Bootsma H, Bowman SJ, Jonsson R, Mariette X, et al. Sjogren syndrome. Nat Rev Dis Primers. (2016) 2:16047. doi: 10.1038/nrdp.2016.47
2. Wu KY, Kulbay M, Tanasescu C, Jiao B, Nguyen BH, Tran SD. An overview of the dry eye disease in sjögren’s syndrome using our current molecular understanding. Int J Mol Sci. (2023) 24:1580. doi: 10.3390/ijms24021580
3. Maehara T, Moriyama M, Hayashida JN, Tanaka A, Shinozaki S, Kubo Y, et al. Selective localization of T helper subsets in labial salivary glands from primary sjogren’s syndrome patients. Clin Exp Immunol. (2012) 169:89–99. doi: 10.1111/j.1365-2249.2012.04606.x
4. Rios-Rios WJ, Sosa-Luis SA, Torres-Aguilar H. T cells subsets in the immunopathology and treatment of sjogren’s syndrome. Biomolecules. (2020) 10(11):1539–58. doi: 10.3390/biom10111539
5. Chen W, Yang F, Xu G, Ma J, Lin J. Follicular helper T cells and follicular regulatory T cells in the immunopathology of primary sjogren’s syndrome. J Leukocyte Biol. (2021) 109:437–47. doi: 10.1002/JLB.5MR1020-057RR
6. Siso-Almirall A, Meijer JM, Brito-Zeron P, Conangla L, Flores-Chavez A, Gonzalez de Paz L, et al. Practical guidelines for the early diagnosis of sjogren’s syndrome in primary healthcare. Clin Exp Rheumatol. (2021) 39 Suppl 133:197–205. doi: 10.55563/clinexprheumatol/pal3z7
7. Vitali C, Del Papa N. Classification criteria for sjögren’s syndrome. Sjögren’s Syndrome. (2016), 47–60. doi: 10.1016/B978-0-12-803604-4.00004-6
8. Franceschini F, Cavazzana I. Anti-ro/ssa and la/ssb antibodies. Autoimmunity. (2005) 38:55–63. doi: 10.1080/08916930400022954
9. Cavazzana I, Franceschini F, Quinzanini M, Manera C, Del Papa N, Maglione W, et al. Anti-ro/ssa antibodies in rheumatoid arthritis: clinical and immunologic associations. Clin Exp Rheumatol. (2006) 24:59–64.
10. Martin-Nares E, Hernandez-Molina G. Novel autoantibodies in sjogren’s syndrome: A comprehensive review. Autoimmun Rev. (2019) 18:192–8. doi: 10.1016/j.autrev.2018.09.003
11. Risselada AP, Looije MF, Kruize AA, Bijlsma JW, van Roon JA. The role of ectopic germinal centers in the immunopathology of primary sjogren’s syndrome: A systematic review. Semin Arthritis Rheumatism. (2013) 42:368–76. doi: 10.1016/j.semarthrit.2012.07.003
12. Toker E, Yavuz S, Direskeneli H. Anti-ro/ssa and anti-la/ssb autoantibodies in the tear fluid of patients with sjogren’s syndrome. Br J Ophthalmol. (2004) 88:384–7. doi: 10.1136/bjo.2003.028340
13. Zandbelt M, te Boome L, Klasen I, van de Putte L, van den Hoogen F. Tear fluid measurement of anti-ss-a and anti-ss-B antibody in anti-ss-a and anti-ss-B seronegative sjogren’s syndrome patients. Clin Exp Rheumatol. (2009) 27:536.
14. Kamounah S, Tayob N, Chiang S, Wei F, Park JK, Kwon HM, et al. Immunoassay detects salivary anti-ssa/ro-52 autoantibodies in seronegative patients with primary sjogren’s syndrome. Immunohorizons. (2023) 7:554–61. doi: 10.4049/immunohorizons.2300043
15. Chen X, Aqrawi LA, Utheim TP, Tashbayev B, Utheim OA, Reppe S, et al. Elevated cytokine levels in tears and saliva of patients with primary sjogren’s syndrome correlate with clinical ocular and oral manifestations. Sci Rep. (2019) 9:7319. doi: 10.1038/s41598-019-43714-5
16. Humphreys-Beher MG. Animal models for autoimmune disease-associated xerostomia and xerophthalmia. Adv Dental Res. (1996) 10:73–5. doi: 10.1177/08959374960100011501
17. Edman MC, Janga SR, Meng Z, Bechtold M, Chen AF, Kim C, et al. Increased cathepsin S activity associated with decreased protease inhibitory capacity contributes to altered tear proteins in sjogren’s syndrome patients. Sci Rep. (2018) 8:11044. doi: 10.1038/s41598-018-29411-9
18. Hamm-Alvarez SF, Janga SR, Edman MC, Madrigal S, Shah M, Frousiakis SE, et al. Tear cathepsin S as a candidate biomarker for sjogren’s syndrome. Arthritis Rheumatol. (2014) 66:1872–81. doi: 10.1002/art.38633
19. Kakan SS, Edman MC, Yao A, Okamoto CT, Nguyen A, Hjelm BE, et al. Tear mirnas identified in a murine model of sjögren’s syndrome as potential diagnostic biomarkers and indicators of disease mechanism. Front Immunol. (2022) 13:833254. doi: 10.3389/fimmu.2022.833254
20. Skarstein K, Wahren M, Zaura E, Hattori M, Jonsson R. Characterization of T cell receptor repertoire and anti-ro/ssa autoantibodies in relation to sialadenitis of nod mice. Autoimmunity. (1995) 22:9–16. doi: 10.3109/08916939508995294
21. Prochazka M, Serreze DV, Frankel WN, Leiter EH. Nor/lt mice: mhc-matched diabetes-resistant control strain for nod mice. Diabetes. (1992) 41:98–106. doi: 10.2337/diab.41.1.98
22. Ju Y, Janga SR, Klinngam W, MacKay JA, Hawley D, Zoukhri D, et al. Nod and nor Mice Exhibit Comparable Development of Lacrimal Gland Secretory Dysfunction but Nod Mice Have More Severe Autoimmune Dacryoadenitis. Exp Eye Res. (2018) 176:243–51. doi: 10.1016/j.exer.2018.09.002
23. Roescher N, Lodde BM, Vosters JL, Tak PP, Catalan MA, Illei GG, et al. Temporal changes in salivary glands of non-obese diabetic mice as a model for sjogren’s syndrome. Oral Dis. (2012) 18:96–106. doi: 10.1111/j.1601-0825.2011.01852.x
24. Janga SR, Shah M, Ju Y, Meng Z, Edman MC, Hamm-Alvarez SF. Longitudinal analysis of tear cathepsin S activity levels in male non-obese diabetic mice suggests its potential as an early stage biomarker of sjogren’s syndrome. Biomarkers. (2019) 24:91–102. doi: 10.1080/1354750X.2018.1514656
25. Takahashi M, Ishimaru N, Yanagi K, Haneji N, Saito I, Hayashi Y. High incidence of autoimmune dacryoadenitis in male non-obese diabetic (Nod) mice depending on sex steroid. Clin Exp Immunol. (1997) 109:555–61. doi: 10.1046/j.1365-2249.1997.4691368.x
26. Hunger RE, Carnaud C, Vogt I, Mueller C. Male gonadal environment paradoxically promotes dacryoadenitis in nonobese diabetic mice. J Clin Invest. (1998) 101:1300–9. doi: 10.1172/JCI1230
27. Aubin AM, Lombard-Vadnais F, Collin R, Aliesky HA, McLachlan SM, Lesage S. The nod mouse beyond autoimmune diabetes. Front Immunol. (2022) 13:874769. doi: 10.3389/fimmu.2022.874769
28. Council NR. Guide for the Care and Use of Laboratory Animals. Eight. Washington, DC: The National Academies Press (2011). p. 246.
29. Li X, Wu K, Edman M, Schenke-Layland K, MacVeigh-Aloni M, Janga SR, et al. Increased expression of cathepsins and obesity-induced proinflammatory cytokines in lacrimal glands of male nod mouse. Invest Ophthalmol Vis Sci. (2010) 51:5019–29. doi: 10.1167/iovs.09-4523
30. Zhu H, Luo H, Yan M, Zuo X, Li QZ. Autoantigen microarray for high-throughput autoantibody profiling in systemic lupus erythematosus. Genomics Proteomics Bioinf. (2015) 13:210–8. doi: 10.1016/j.gpb.2015.09.001
31. Rajasinghe LD, Li QZ, Zhu C, Yan M, Chauhan PS, Wierenga KA, et al. Omega-3 fatty acid intake suppresses induction of diverse autoantibody repertoire by crystalline silica in lupus-prone mice. Autoimmunity. (2020) 53:415–33. doi: 10.1080/08916934.2020.1801651
32. Kramer JM, Holodick NE, Vizconde TC, Raman I, Yan M, Li QZ, et al. Analysis of igm antibody production and repertoire in a mouse model of sjogren’s syndrome. J Leukocyte Biol. (2016) 99:321–31. doi: 10.1189/jlb.2A0715-297R
33. Li QZ, Xie C, Wu T, Mackay M, Aranow C, Putterman C, et al. Identification of autoantibody clusters that best predict lupus disease activity using glomerular proteome arrays. J Clin Invest. (2005) 115:3428–39. doi: 10.1172/JCI23587
34. Ritchie ME, Phipson B, Wu D, Hu Y, Law CW, Shi W, et al. Limma powers differential expression analyses for rna-sequencing and microarray studies. Nucleic Acids Res. (2015) 43:e47. doi: 10.1093/nar/gkv007
35. Kiripolsky J, Shen L, Liang Y, Li A, Suresh L, Lian Y, et al. Systemic manifestations of primary sjogren’s syndrome in the nod.B10sn-H2(B)/J mouse model. Clin Immunol. (2017) 183:225–32. doi: 10.1016/j.clim.2017.04.009
36. Rattner A, Heng JS, Winer BL, Goff LA, Nathans J. Normal and sjogren’s syndrome models of the murine lacrimal gland studied at single-cell resolution. Proc Natl Acad Sci United States America. (2023) 120:e2311983120. doi: 10.1073/pnas.2311983120
37. Pipi E, Nayar S, Gardner DH, ColaFrancesco S, Smith C, Barone F. Tertiary lymphoid structures: autoimmunity goes local. Front Immunol. (2018) 9:1952. doi: 10.3389/fimmu.2018.01952
38. Hu R, Kagele DA, Huffaker TB, Runtsch MC, Alexander M, Liu J, et al. Mir-155 promotes T follicular helper cell accumulation during chronic, low-grade inflammation. Immunity. (2014) 41:605–19. doi: 10.1016/j.immuni.2014.09.015
39. Singh Kakan S, Li X, Edman MC, Okamoto CT, Hjelm BE, Hamm-Alvarez SF. The mirna landscape of lacrimal glands in a murine model of autoimmune dacryoadenitis. Invest Ophthalmol Vis Sci. (2023) 64:1. doi: 10.1167/iovs.64.4.1
40. Hauser AE, Kerfoot SM, Haberman AM. Cellular choreography in the germinal center: new visions from in vivo imaging. Semin Immunopathol. (2010) 32:239–55. doi: 10.1007/s00281-010-0214-z
41. Gatto D, Brink R. The germinal center reaction. J Allergy Clin Immunol. (2010) 126:898–907. doi: 10.1016/j.jaci.2010.09.007
42. Rodig SJ, Shahsafaei A, Li B, Dorfman DM. The cd45 isoform B220 identifies select subsets of human B cells and B-cell lymphoproliferative disorders. Hum Pathol. (2005) 36:51–7. doi: 10.1016/j.humpath.2004.10.016
43. Barone F, Bombardieri M, Manzo A, Blades MC, Morgan PR, Challacombe SJ, et al. Association of cxcl13 and ccl21 expression with the progressive organization of lymphoid-like structures in sjogren’s syndrome. Arthritis Rheum. (2005) 52:1773–84. doi: 10.1002/art.21062
44. Alarcon-Segovia D. Symptomatic sjogren’s syndrome in mixed connective tissue disease. J Rheumatol. (1976) 3:191–5.
45. Bournia VK, Vlachoyiannopoulos PG. Subgroups of sjogren syndrome patients according to serological profiles. J Autoimmun. (2012) 39:15–26. doi: 10.1016/j.jaut.2012.03.001
46. Decker P, Moulinet T, Pontille F, Cravat M, De Carvalho Bittencourt M, Jaussaud R. An updated review of anti-ro52 (Trim21) antibodies impact in connective tissue diseases clinical management. Autoimmun Rev. (2022) 21:103013. doi: 10.1016/j.autrev.2021.103013
47. Shen L, Suresh L, Lindemann M, Xuan J, Kowal P, Malyavantham K, et al. Novel autoantibodies in sjogren’s syndrome. Clin Immunol. (2012) 145:251–5. doi: 10.1016/j.clim.2012.09.013
48. Anderson MS, Bluestone JA. The nod mouse: A model of immune dysregulation. Annu Rev Immunol. (2005) 23:447–85. doi: 10.1146/annurev.immunol.23.021704.115643
49. da Costa SR, Wu K, Veigh MM, Pidgeon M, Ding C, Schechter JE, et al. Male nod mouse external lacrimal glands exhibit profound changes in the exocytotic pathway early in postnatal development. Exp Eye Res. (2006) 82:33–45. doi: 10.1016/j.exer.2005.04.019
50. Doyle ME, Boggs L, Attia R, Cooper LR, Saban DR, Nguyen CQ, et al. Autoimmune dacryoadenitis of nod/ltj mice and its subsequent effects on tear protein composition. Am J Pathol. (2007) 171:1224–36. doi: 10.2353/ajpath.2007.070388
51. Hu Y, Nakagawa Y, Purushotham KR, Humphreys-Beher MG. Functional changes in salivary glands of autoimmune disease-prone nod mice. Am J Physiol. (1992) 263:E607–14. doi: 10.1152/ajpendo.1992.263.4.E607
52. Jonsson MV, Delaleu N, Brokstad KA, Berggreen E, Skarstein K. Impaired salivary gland function in nod mice: association with changes in cytokine profile but not with histopathologic changes in the salivary gland. Arthritis Rheum. (2006) 54:2300–5. doi: 10.1002/art.21945
53. Karakus S, Baer AN, Agrawal D, Gurakar M, Massof RW, Akpek EK. Utility of novel autoantibodies in the diagnosis of sjogren’s syndrome among patients with dry eye. Cornea. (2018) 37:405–11. doi: 10.1097/ICO.0000000000001471
54. Soyfoo MS, Chivasso C, Perret J, Delporte C. Involvement of aquaporins in the pathogenesis, diagnosis and treatment of sjogren’s syndrome. Int J Mol Sci. (2018) 19(11):3392–07. doi: 10.3390/ijms19113392
55. Chotikavanich S, de Paiva CS, Li de Q, Chen JJ, Bian F, Farley WJ, et al. Production and activity of matrix metalloproteinase-9 on the ocular surface increase in dysfunctional tear syndrome. Invest Ophthalmol Vis Sci. (2009) 50:3203–9. doi: 10.1167/iovs.08-2476
56. Hamm-Alvarez SF, Janga SR, Edman MC, Feigenbaum D, Freire D, Mack WJ, et al. Levels of oligomeric alpha-synuclein in reflex tears distinguish parkinson’s disease patients from healthy controls. biomark Med. (2019) 13:1447–57. doi: 10.2217/bmm-2019-0315
57. Hamm-Alvarez SF, Okamoto CT, Janga SR, Feigenbaum D, Edman MC, Freire D, et al. Oligomeric alpha-synuclein is increased in basal tears of parkinson’s patients. biomark Med. (2019) 13:941–52. doi: 10.2217/bmm-2019-0167
58. van de Sande N, Ramakers I, Visser PJ, Verhey FRJ, Verbraak FD, Bouwman FH, et al. Tear biomarkers for alzheimer’s disease screening and diagnosis (the tearad study): design and rationale of an observational longitudinal multicenter study. BMC Neurol. (2023) 23:293. doi: 10.1186/s12883-023-03335-y
59. Gijs M, Arumugam S, van de Sande N, Webers CAB, Sethu S, Ghosh A, et al. Pre-analytical sample handling effects on tear fluid protein levels. Sci Rep. (2023) 13:1317. doi: 10.1038/s41598-023-28363-z
60. Theander E, Jonsson R, Sjostrom B, Brokstad K, Olsson P, Henriksson G. Prediction of sjogren’s syndrome years before diagnosis and identification of patients with early onset and severe disease course by autoantibody profiling. Arthritis Rheumatol. (2015) 67:2427–36. doi: 10.1002/art.39214
61. Lee AYS, Wang JJ, Gordon TP, Reed JH. Phases and natural history of sjogren’s disease: A new model for an old disease? Arthritis Care Res. (2023) 75:1580–7. doi: 10.1002/acr.25011
62. Woof JM, Kerr MA. The function of immunoglobulin a in immunity. J Pathol. (2006) 208:270–82. doi: 10.1002/path.1877
63. Knop E, Knop N, Claus P. Local production of secretory iga in the eye-associated lymphoid tissue (Ealt) of the normal human ocular surface. Invest Ophthalmol Vis Sci. (2008) 49:2322–9. doi: 10.1167/iovs.07-0691
64. Bjordal O, Norheim KB, Rodahl E, Jonsson R, Omdal R. Primary sjogren’s syndrome and the eye. Surv Ophthalmol. (2020) 65:119–32. doi: 10.1016/j.survophthal.2019.10.004
65. Steven P, Schwab S, Kiesewetter A, Saban DR, Stern ME, Gehlsen U. Disease-specific expression of conjunctiva associated lymphoid tissue (Calt) in mouse models of dry eye disease and ocular allergy. Int J Mol Sci. (2020) 21(20):7514–28. doi: 10.3390/ijms21207514
66. Siebelmann S, Gehlsen U, Huttmann G, Koop N, Bolke T, Gebert A, et al. Development, alteration and real time dynamics of conjunctiva-associated lymphoid tissue. PloS One. (2013) 8:e82355. doi: 10.1371/journal.pone.0082355
67. Dong Y, Wang T, Wu H. Tertiary lymphoid structures in autoimmune diseases. Front Immunol. (2023) 14:1322035. doi: 10.3389/fimmu.2023.1322035
68. Jonsson MV, Skarstein K. Follicular dendritic cells confirm lymphoid organization in the minor salivary glands of primary sjogren’s syndrome. J Oral Pathol Med. (2008) 37:515–21. doi: 10.1111/j.1600-0714.2008.00674.x
69. Kwon J, Surenkhuu B, Raju I, Atassi N, Mun J, Chen YF, et al. Pathological consequences of anti-citrullinated protein antibodies in tear fluid and therapeutic potential of pooled human immune globulin-eye drops in dry eye disease. Ocular Surface. (2020) 18:80–97. doi: 10.1016/j.jtos.2019.10.004
70. Katsiougiannis S, Tenta R, Skopouli FN. Endoplasmic reticulum stress causes autophagy and apoptosis leading to cellular redistribution of the autoantigens ro/sjogren’s syndrome-related antigen a (Ssa) and la/ssb in salivary gland epithelial cells. Clin Exp Immunol. (2015) 181:244–52. doi: 10.1111/cei.12638
71. Verstappen GM, Pringle S, Bootsma H, Kroese FGM. Epithelial-immune cell interplay in primary sjogren syndrome salivary gland pathogenesis. Nat Rev Rheumatol. (2021) 17:333–48. doi: 10.1038/s41584-021-00605-2
72. Waterman HR, Dufort MJ, Posso SE, Ni M, Li LZ, Zhu C, et al. Lupus iga1 autoantibodies synergize with igg to enhance plasmacytoid dendritic cell responses to rna-containing immune complexes. Sci Transl Med. (2024) 16:eadl3848. doi: 10.1126/scitranslmed.adl3848
73. Notarnicola A, Preger C, Lundstrom SL, Renard N, Wigren E, Van Gompel E, et al. Longitudinal assessment of reactivity and affinity profile of anti-jo1 autoantibodies to distinct hisrs domains and a splice variant in a cohort of patients with myositis and anti-synthetase syndrome. Arthritis Res Ther. (2022) 24:62. doi: 10.1186/s13075-022-02745-6
74. Yoshida S, Akizuki M, Mimori T, Yamagata H, Inada S, Homma M. The precipitating antibody to an acidic nuclear protein antigen, the jo-1, in connective tissue diseases. A marker for a subset of polymyositis with interstitial pulmonary fibrosis. Arthritis Rheum. (1983) 26:604–11. doi: 10.1002/art.1780260505
75. Hochberg MC, Feldman D, Stevens MB, Arnett FC, Reichlin M. Antibody to jo-1 in polymyositis/dermatomyositis: association with interstitial pulmonary disease. J Rheumatol. (1984) 11:663–5.
76. Bernstein RM, Morgan SH, Chapman J, Bunn CC, Mathews MB, Turner-Warwick M, et al. Anti-jo-1 antibody: A marker for myositis with interstitial lung disease. Br Med J (Clin Res Ed). (1984) 289:151–2. doi: 10.1136/bmj.289.6438.151
77. Ronnelid J, Barbasso Helmers S, Storfors H, Grip K, Ronnblom L, Franck-Larsson K, et al. Use of a commercial line blot assay as a screening test for autoantibodies in inflammatory myopathies. Autoimmun Rev. (2009) 9:58–61. doi: 10.1016/j.autrev.2009.03.005
78. Iismaa SE, Mearns BM, Lorand L, Graham RM. Transglutaminases and disease: lessons from genetically engineered mouse models and inherited disorders. Physiol Rev. (2009) 89:991–1023. doi: 10.1152/physrev.00044.2008
79. Troncone R, Maurano F, Rossi M, Micillo M, Greco L, Auricchio R, et al. Iga antibodies to tissue transglutaminase: an effective diagnostic test for celiac disease. J Pediatr. (1999) 134:166–71. doi: 10.1016/s0022-3476(99)70410-5
80. Hu S, Vissink A, Arellano M, Roozendaal C, Zhou H, Kallenberg CG, et al. Identification of autoantibody biomarkers for primary sjogren’s syndrome using protein microarrays. Proteomics. (2011) 11:1499–507. doi: 10.1002/pmic.201000206
81. Bonifacio E, Lampasona V, Genovese S, Ferrari M, Bosi E. Identification of protein tyrosine phosphatase-like ia2 (Islet cell antigen 512) as the insulin-dependent diabetes-related 37/40k autoantigen and a target of islet-cell antibodies. J Immunol. (1995) 155:5419–26. doi: 10.4049/jimmunol.155.11.5419
82. Winnock F, Christie MR, Batstra MR, Aanstoot HJ, Weets I, Decochez K, et al. Autoantibodies to a 38-kda glycosylated islet cell membrane-associated antigen in (Pre)Type 1 diabetes: association with ia-2 and islet cell autoantibodies. Diabetes Care. (2001) 24:1181–6. doi: 10.2337/diacare.24.7.1181
83. Vargas-Uricoechea H, Nogueira JP, Pinzon-Fernandez MV, Schwarzstein D. The usefulness of thyroid antibodies in the diagnostic approach to autoimmune thyroid disease. Antibodies (Basel). (2023) 12(3):48–70. doi: 10.3390/antib12030048
84. Espitia-Thibault A, Masseau A, Neel A, Espitia O, Toquet C, Mussini JM, et al. Sjogren’s syndrome-associated myositis with germinal centre-like structures. Autoimmun Rev. (2017) 16:154–8. doi: 10.1016/j.autrev.2016.12.006
85. Kanellopoulos P, Baltoyiannis C, Tzioufas AG. Primary sjogren’s syndrome associated with inclusion body myositis. Rheumatol (Oxford England). (2002) 41:440–4. doi: 10.1093/rheumatology/41.4.440
86. Vilchez-Oya F, Balastegui Martin H, Garcia-Martinez E, Corominas H. Not all autoantibodies are clinically relevant. Classic and novel autoantibodies in sjogren’s syndrome: A critical review. Front Immunol. (2022) 13:1003054. doi: 10.3389/fimmu.2022.1003054
87. Kyriakidis NC, Kapsogeorgou EK, Tzioufas AG. A comprehensive review of autoantibodies in primary sjogren’s syndrome: clinical phenotypes and regulatory mechanisms. J Autoimmun. (2014) 51:67–74. doi: 10.1016/j.jaut.2013.11.001
88. Burbelo PD, Iadarola MJ, Keller JM, Warner BM. Autoantibodies targeting intracellular and extracellular proteins in autoimmunity. Front Immunol. (2021) 12:548469. doi: 10.3389/fimmu.2021.548469
89. Ludwig RJ, Vanhoorelbeke K, Leypoldt F, Kaya Z, Bieber K, McLachlan SM, et al. Mechanisms of autoantibody-induced pathology. Front Immunol. (2017) 8:603. doi: 10.3389/fimmu.2017.00603
Keywords: Sjögren’s disease, autoimmune disease, IgA autoantibodies, IgG autoantibodies, tear film, serum
Citation: Singh Kakan S, Abdelhamid S, Ju Y, MacKay JA, Edman MC, Raman I, Zhu C, Raj P and Hamm-Alvarez SF (2025) Serum and tear autoantibodies from NOD and NOR mice as potential diagnostic indicators of local and systemic inflammation in Sjögren’s disease. Front. Immunol. 15:1516330. doi: 10.3389/fimmu.2024.1516330
Received: 24 October 2024; Accepted: 30 December 2024;
Published: 28 January 2025.
Edited by:
Jan Stępniak, Medical University of Lodz, PolandReviewed by:
Stelvio Tonello, University of Eastern Piedmont, ItalyCopyright © 2025 Singh Kakan, Abdelhamid, Ju, MacKay, Edman, Raman, Zhu, Raj and Hamm-Alvarez. This is an open-access article distributed under the terms of the Creative Commons Attribution License (CC BY). The use, distribution or reproduction in other forums is permitted, provided the original author(s) and the copyright owner(s) are credited and that the original publication in this journal is cited, in accordance with accepted academic practice. No use, distribution or reproduction is permitted which does not comply with these terms.
*Correspondence: Sarah F. Hamm-Alvarez, c2hhbHZhckB1c2MuZWR1
Disclaimer: All claims expressed in this article are solely those of the authors and do not necessarily represent those of their affiliated organizations, or those of the publisher, the editors and the reviewers. Any product that may be evaluated in this article or claim that may be made by its manufacturer is not guaranteed or endorsed by the publisher.
Research integrity at Frontiers
Learn more about the work of our research integrity team to safeguard the quality of each article we publish.