- Department of Clinical Laboratory, The First People’s Hospital of Kunshan, Kunshan, Jiangsu, China
Outer membrane vesicles (OMVs) and exosomes are essential mediators of host-pathogen interactions. Elucidating their mechanisms of action offers valuable insights into diagnosing and treating infectious diseases and cancers. However, the specific interactions of Helicobacter pylori (H. pylori) with host cells via OMVs and exosomes in modulating host immune responses have not been thoroughly investigated. This review explores how these vesicles elicit inflammatory and immunosuppressive responses in the host environment, facilitate pathogen invasion of host cells, and enable evasion of host defenses, thereby contributing to the progression of gastric diseases and extra-gastric diseases disseminated through the bloodstream. Furthermore, the review discusses the challenges and future directions for investigating OMVs and exosomes, underscoring their potential as therapeutic targets in H. pylori-associated diseases.
1 Introduction
Helicobacter pylori (H. pylori) infection is a prevalent chronic bacterial infection that colonizes the human gastric mucosa, leading to gastrointestinal disorders such as gastritis, peptic ulcers, and gastric cancer (1). As a highly adapted pathogen, H. pylori has evolved intricate mechanisms to modulate host immune responses and establish persistent colonization in the gastric environment (2). Recently, attention has shifted toward outer membrane vesicles (OMVs) released by H. pylori and exosomes derived from infected host cells, which play pivotal roles in host-pathogen interactions.
OMVs are nanoscale structures packed with virulence factors that modulate host immune responses and promote bacterial survival (3, 4). They facilitate the dissemination of bacterial antigens across the gastric epithelial barrier, influencing local and systemic inflammatory responses (3). Similarly, exosomes produced by H. pylori-infected epithelial cells carry molecular signatures reflective of the infection status and can alter immune cell signaling. In particular, exosomes derived from immune cells are crucial mediators of host-pathogen interactions and modulate immune responses significantly. Numerous studies have underscored the importance of these nanosized vesicles, demonstrating their ability to transport bioactive molecules, including proteins, lipids, nucleic acids, and metabolites (5). Research indicates their involvement not only in normal immune responses but also in modifying the tumor microenvironment and influencing the progression of autoimmune diseases (6). By delivering molecular cargo to recipient cells, exosomes are instrumental in the pathogenesis of various diseases, highlighting their critical function in immune system dynamics (7, 8) (Figure 1). Recent studies further emphasize exosomes’ role in mediating communication between H. pylori and host cells, significantly impacting disease progression (9, 10). Despite extensive research on OMVs and exosomes in various bacterial infections (3, 10–12), there is a significant gap in understanding their roles in H. pylori pathogenesis. This review aims to fill this gap by examining the unique interactions between H. pylori-derived OMVs, exosomes, and host immune responses, providing novel insights into their contributions to disease progression. Moreover, investigating the roles of OMVs and exosomes in H. pylori-related diseases, such as gastric cancer, offers opportunities for developing new diagnostic markers and therapeutic strategies.
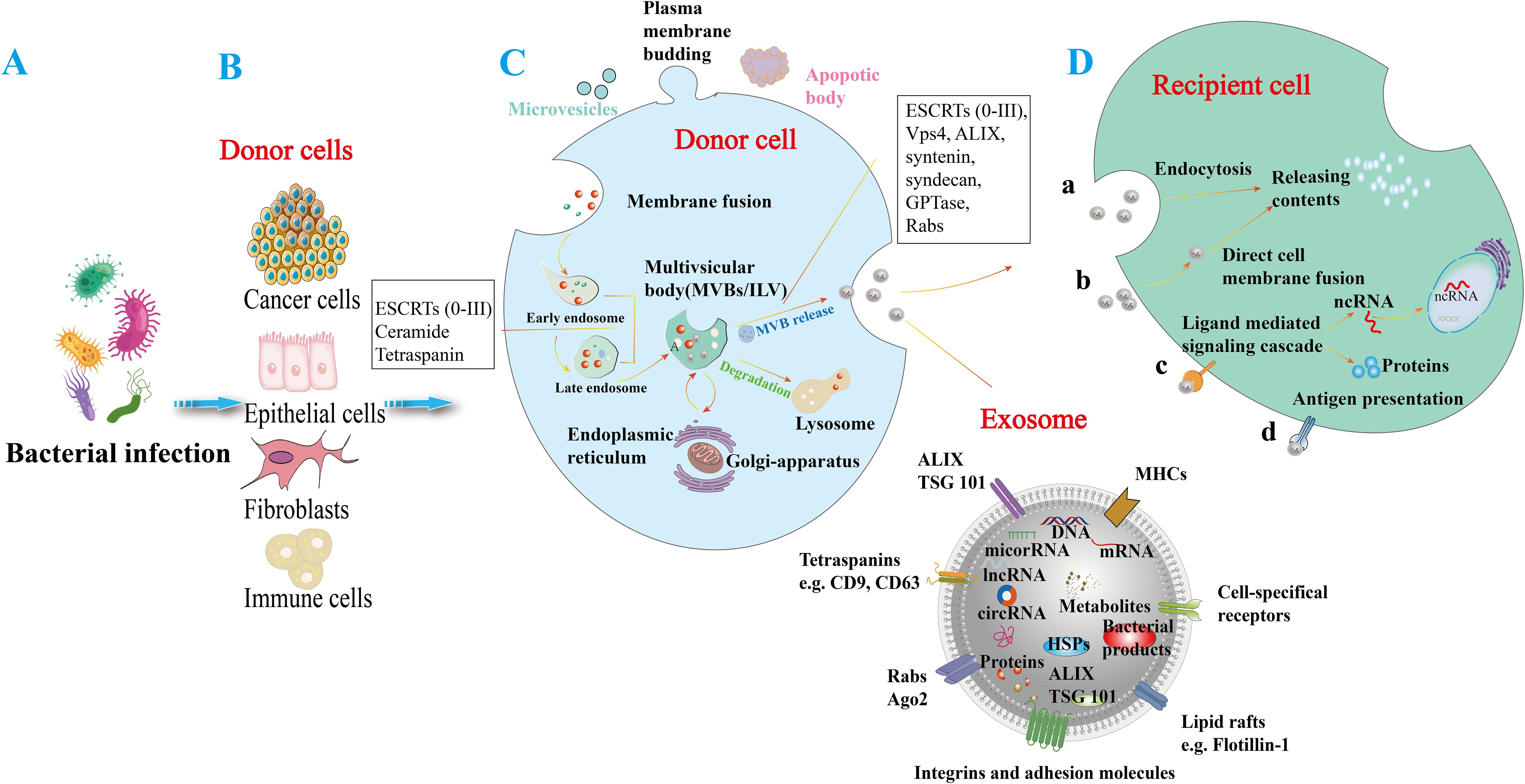
Figure 1. Generation and release of exosomes in response to bacterial infection. (A) Bacterial infections in various cell types can induce the release of exosomes. (B) Various bacterial-infected cell types, including cancer cells, epithelial cells, fibroblasts, and immune cells, can induce the release of exosomes. (C) Microvesicles arise from the outward budding of the plasma membrane, while apoptotic vesicles are lipid bilayer structures generated during programmed cell death. The process of exosome formation begins with the invagination of the plasma membrane to create early endosomes, which then mature into late endosomes. These endosomes produce intraluminal vesicles (ILVs) and utilize biogenesis proteins (e.g., ESCRTs, ALIX, syntaxin, and Rabs) to form multivesicular bodies (MVBs). MVBs can either merge with lysosomes for degradation or fuse with the cell membrane to release their enclosed vesicles into the extracellular space, resulting in exosome release. Exosomal cargo can include various molecules such as DNA, mRNA, small RNAs (miRNA, lncRNA, circRNA), proteins (e.g., heat shock proteins (HSP), ALIX/TSG101), metabolites, and bacterial components. Exosomes are composed of major histocompatibility complexes (MHC), specific cell receptors, lipid rafts, integrin adhesion molecules, Rabs, Ago proteins, tetraspanin proteins, and ESCRT proteins (e.g., ALIX/TSG101). These surface molecules may facilitate the uptake of exosomes by recipient cells. (D) Exosomes can exert their effects through various mechanisms, including endocytosis (a), direct fusion with the recipient cell membrane (b), ligand- and receptor-mediated signaling pathways (c), and antigen presentation (d), which release active molecules such as non-coding RNAs and proteins into target cells.
This review focuses explicitly on the underexplored functions of H. pylori-derived OMVs and exosomes in host immune modulation, intending to stimulate further research and foster innovative management strategies for infections caused by this prevalent bacterium. Additionally, it elucidates how these vesicles contribute to the chronic nature of H. pylori infections and their potential implications for disease development.
2 Roles of H. pylori OMVs
2.1 Mechanisms of OMVs biogenesis
During infection, pathogens directly release vesicles known as membrane vesicles. Gram-negative bacteria employ two main pathways to produce vesicles. The first pathway involves budding off the capsule membrane to form outer membrane vesicles (B-type OMVs). The second pathway requires explosive cell lysis to produce inner vesicles and explosion-derived outer membrane vesicles (E-type OMVs) (13, 14). The membrane composition of OMVs is generally similar to that of the bacterial outer membrane, although not always identical. Some outer membrane components are concentrated in OMVs, while others are absent or diminished. These vesicles, ranging in size from 20 to 500 nm, are crucial for bacterial communication with the environment and significantly contribute to bacterial pathogenesis (15–19) (Figure 2). Like other Gram-negative bacteria, H. pylori release OMVs from its outer membrane. The mechanisms regulating OMV production during H. pylori infections are currently under intense scrutiny. Understanding the precise pathways and regulatory processes involved in OMVs biogenesis during H. pylori infection is essential for grasping the implications of OMVs-mediated communication on disease progression.
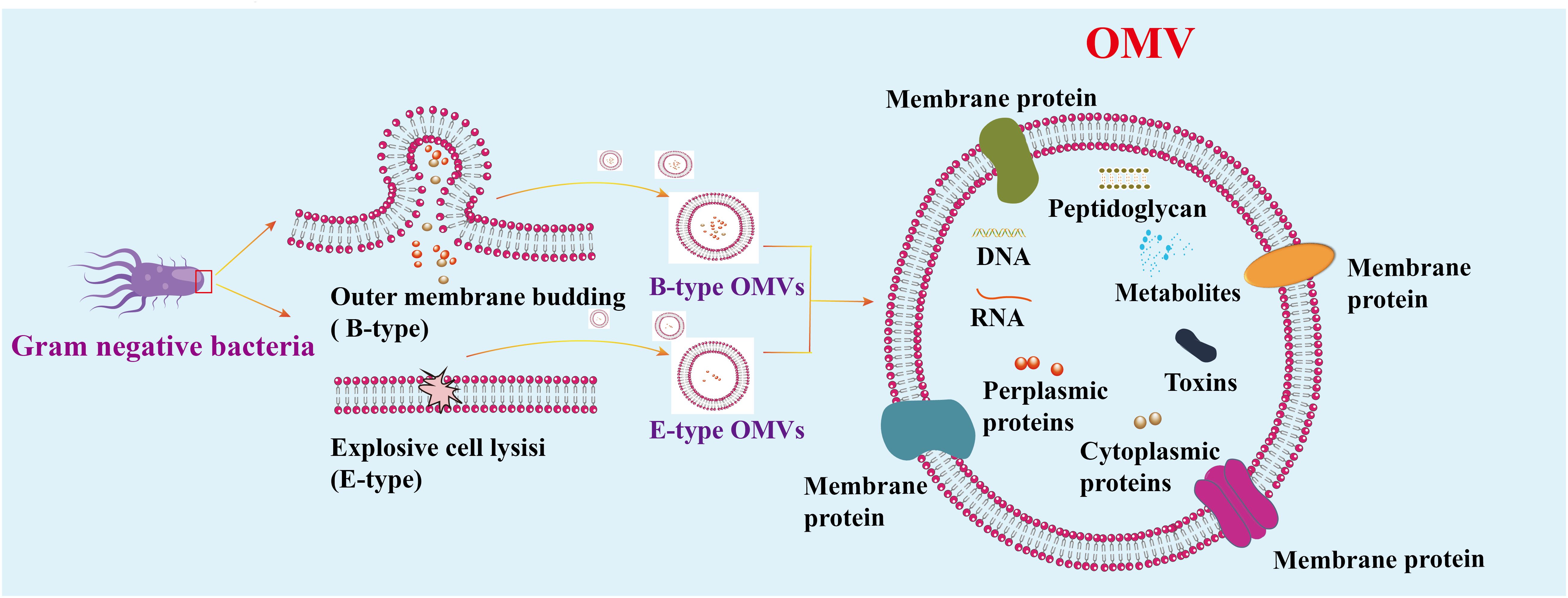
Figure 2. Mechanism of vesicle generation in Gram-negative. Gram-negative bacteria produce two types of OMVs: Type B and Type E. Type E OMVs are generated when explosive cell lysis disrupts the outer membrane. OMVs consist of a continuous lipid bilayer derived from the outer membrane and contain various components, including cytoplasmic and outer membrane proteins, toxins, enzymes, nucleic acids, peptidoglycans, and biomolecules from the parent bacterial cells.
2.2 Functional roles of H. pylori OMVs in host cells
H. pylori OMVs are nanoscale structures critical in bacterial pathogenesis (20). The size, composition, and protein cargo of OMVs vary with the growth stage of H. pylori, influencing their immunogenicity (17). Proteomic analyses have revealed numerous proteins in OMVs, including membrane proteins, porins, adhesins, and toxins, that facilitate interactions with host cells like those of intact bacteria (21). These OMVs elicit robust humoral and mucosal immune responses, predominantly biased toward Th2, making them potential vaccine candidates against H. pylori infection (22).
Moreover, H. pylori OMVs possess biologically active compounds that can be internalized by host cells, affecting signaling pathways and promoting apoptosis (3). For instance, they stimulate interleukin-8 (IL-8) secretion through NF-κB activation, contributing to inflammation (23). Additionally, H. pylori OMVs can enhance and downregulate immune responses, contributing to disease progression (3). Notably, H. pylori OMVs inhibit autophagy in hepatic stellate cells by altering the expression of autophagy-related genes, suggesting a novel mechanism for H. pylori-induced liver disorders (20). These findings underscore the complex involvement of H. pylori OMVs in modulating host cell functions and highlight their potential as therapeutic targets.
3 Roles of exosomes derived from H. pylori-infected cells
3.1 Mechanisms of exosomes formation
Exosomes are vesicles measuring 30-200 nm, encapsulated by a lipid bilayer with a density ranging from 1.13 to 1.19 g/mL (24, 25). Exosomes form through the endosomal pathway via the fusion of multivesicular bodies (MVBs) with the plasma membrane, resulting in the release of intraluminal vesicles (ILVs) (26). Lipids, proteins, and nucleic acids are transferred to the MVBs before entering vacuoles or being released as vesicles after fusion with the plasma membrane (24). Exosomes are integral to the endosomal sorting complexes required for transport (ESCRT) and may also form through an ESCRT-independent pathway to produce exosomes and microvesicles. GTP enzymes, along with proteins like Rabs, ESCRT complexes (ESCRT-0, I, II, and III), Syntenin-1, tumor susceptibility gene 101 (TSG101), programmed cell death 6 interacting (ALIX), syndecan-1, phospholipids, Tetraspanins (CD9 and CD63), ceramides, sphingosine, and soluble N-ethylmaleimide-sensitive factor (NSF) attachment protein receptor proteins (SNARE), play crucial roles in the biogenesis and release of exosomes (27, 28). The mechanisms underlying exosome formation and regulation during bacterial infections remain under investigation; however, it is recognized that exosomes carry specific cargo and can be utilized by bacteria (Figure 1).
3.2 Regulation of exosome secretion in H. pylori-infected cells
Exosomes are also considered novel diagnostic biomarkers in various pathological contexts, including bacterial infections (29). H. pylori is known to regulate exosome secretion from host cells to establish chronic infection (30). Understanding how H. pylori facilitates or impedes exosome release from host cells is essential for deciphering the pathogenesis of H. pylori-associated diseases. H. pylori infection increases exosome release, potentially activating various signaling pathways through virulence factors such as cytotoxin-associated gene A (CagA) and vacuolating cytotoxin A (VacA) (10, 11, 30). CagA induces pro-inflammatory cytokines and chemokines expression, potentially enhancing the secretion of inflammatory exosomes (12). VacA alters the composition and function of host-derived exosomes, which may assist bacterial survival and colonization in the gastric mucosa (30–32). H. pylori infection triggers various cellular stress responses, including endoplasmic reticulum and oxidative stress, associated with increased exosome secretion (30). These stress signals may prompt exosome release as a cellular communication mode and response to the pathogen. Conversely, H. pylori may disrupt normal cellular signaling pathways associated with exosome biogenesis and release, inhibiting exosome secretion (30). Furthermore, H. pylori impacts the lipid composition of host cell membranes, thereby affecting exosome formation and release (30). Alterations in lipid composition may obstruct exosome budding and release, thereby hindering secretion. The precise mechanisms by which H. pylori promotes or inhibits exosome release from host cells are still being elucidated and may vary based on the bacterial strain and host cell type. Future research exploring the regulation of exosome secretion by H. pylori virulence factors may entail examining the specific signaling pathways and molecular interactions involved in this process. Additionally, understanding the content of H. pylori-modulated exosomes and their impact on host immune cells and epithelial cells is crucial for unraveling the intricate mechanisms of bacterial manipulation of host exosomal machinery.
3.3 Functional roles of exosomes in H. pylori infection
H. pylori utilizes exosomes to transport virulence factors, genetic material, and bioactive molecules to host cells, primarily targeting gastric epithelial cells and immune cells, including macrophages and dendritic cells (30, 33, 34). This specificity is vital for the bacterium’s ability to modulate the host immune response, facilitating its survival and persistence (11, 34, 35). Exosomes derived from H. pylori-infected cells deliver key virulence factors, such as CagA and VacA, which directly influence host cell signaling pathways, altering cellular functions and immune responses (36). This delivery can induce significant changes in cell proliferation, apoptosis, and immune activation (30). Transferring bacterial DNA and RNA via exosomes can instigate genetic and epigenetic modifications in recipient cells, ultimately shaping the host’s response to H. pylori infection (37, 38).
An intriguing aspect of exosome biology in the context of H. pylori infection is their potential role in mediating the bacterium’s toxicity. Exosomes derived from H. pylori-infected cells contribute significantly to antibiotic resistance development and the establishment of a pro-inflammatory environment in the gastric mucosa (10, 11, 39), triggering the release of pro-inflammatory cytokines and chemokines that contribute to chronic inflammation, tissue damage, gastritis, and progression to severe H. pylori-related diseases (11, 31). These exosomes could act as shuttles for delivering bacterial toxins or effectors to adjacent cells, enhancing H. pylori’s overall virulence and exacerbating tissue damage. They also have the potential to be used as drug-delivery vehicles to improve treatment outcomes (40). To target H. pylori-infected cells, a proposed approach involves using exosomes derived from macrophages capable of recognizing infected cells, eradicating pathogenic microorganisms, activating innate immune responses, and delivering drugs against H. pylori (40). This method effectively enhances treatment efficiency while sparing healthy stomach cells from side effects. In cancer, exosomes facilitate intercellular communication within the tumor microenvironment (TME) and contribute to drug resistance by transferring specific mRNA, ncRNA, or proteins, presenting therapeutic targets (41, 42). Moreover, transferring genetic material, including antibiotic-resistance genes, among bacterial populations through exosomes could potentially spread resistance, posing challenges to antibiotic therapy for H. pylori and its evolving resistance issue (43). Understanding the interplay between exosomes and H. pylori in the context of drug resistance is crucial to developing novel therapeutic strategies against this persistent pathogen.
3.4 Crosstalk between H. pylori and host cells mediated by exosomes
Transiting H. pylori’s pathogenic signals via exosomal cargo offers insights into how the bacterium manipulates host cell responses to establish persistent infection and evade immune surveillance (11, 35). Additionally, H. pylori infection may alter the composition of exosomes originating from infected cells, influencing the microenvironment and regulating immune responses (44). H. pylori-infected cell exosomes can modulate host cell gene expression profiles by delivering bioactive molecules such as microRNAs (miRNAs) and other regulatory factors, which interact with specific receptors and signaling pathways, triggering downstream cellular responses that promote inflammation, alter gene expression, and induce host cell damage (37). The delivery of these molecules can affect different cellular processes, such as inflammation, cell proliferation, and immune responses, resulting in host cell function dysregulation and the advancement of gastric conditions like chronic gastritis and gastric cancer (37, 45, 46). The involvement of miRNAs in H. pylori-triggered infections and gastric cancers is continuously under investigation, holding promise for the advancement of early preneoplastic stages (47). Aberrant miRNAs in H. pylori-related inflammation and carcinogenesis could be non-invasive biomarkers (48). These findings underscore the ability of H. pylori to reprogram host cellular responses and establish a microenvironment conducive to bacterial survival and disease progression (34).
4 The interaction between H. pylori OMVs and exosomes
Recent literature underscores the critical role of H. pylori OMVs in pathogenesis and elucidates the function of exosomes in intercellular signaling, particularly how bacterial virulence factors can influence exosome content. Infected cell exosomes and OMVs do not always contain bacterial components, but they can carry bacterial materials and host-derived molecules, including post-translationally modified proteins. H. pylori-released OMVs are rich in bioactive molecules, including proteins, lipids, and nucleic acids, directly engaging with host cells. This unique composition enables OMVs to provoke inflammatory responses and facilitate H. pylori invasion of host tissues (3, 10). Notably, virulence factors, such as VacA and CagA, found within OMVs, play critical roles in immune evasion and the induction of apoptosis in host cells (3). Furthermore, the lipid constituents of OMVs can alter the physical properties of host cell membranes, promoting vesicle fusion with target cells and the subsequent delivery of bioactive cargo (49). Conversely, exosomes are pivotal in mediating intercellular communication and orchestrating immune responses. Exosomes secreted by H. pylori-infected cells transport host-derived proteins and microRNAs that can modulate immune activity, suppressing or promoting host defenses against the infection (10). Exosome-derived microRNAs specifically target and regulate the expression of key mRNAs in host cells, resulting in significant alterations to the immune response landscape. Table 1 compares the major components of the most studied molecules and proteins in OMVs and exosomes.
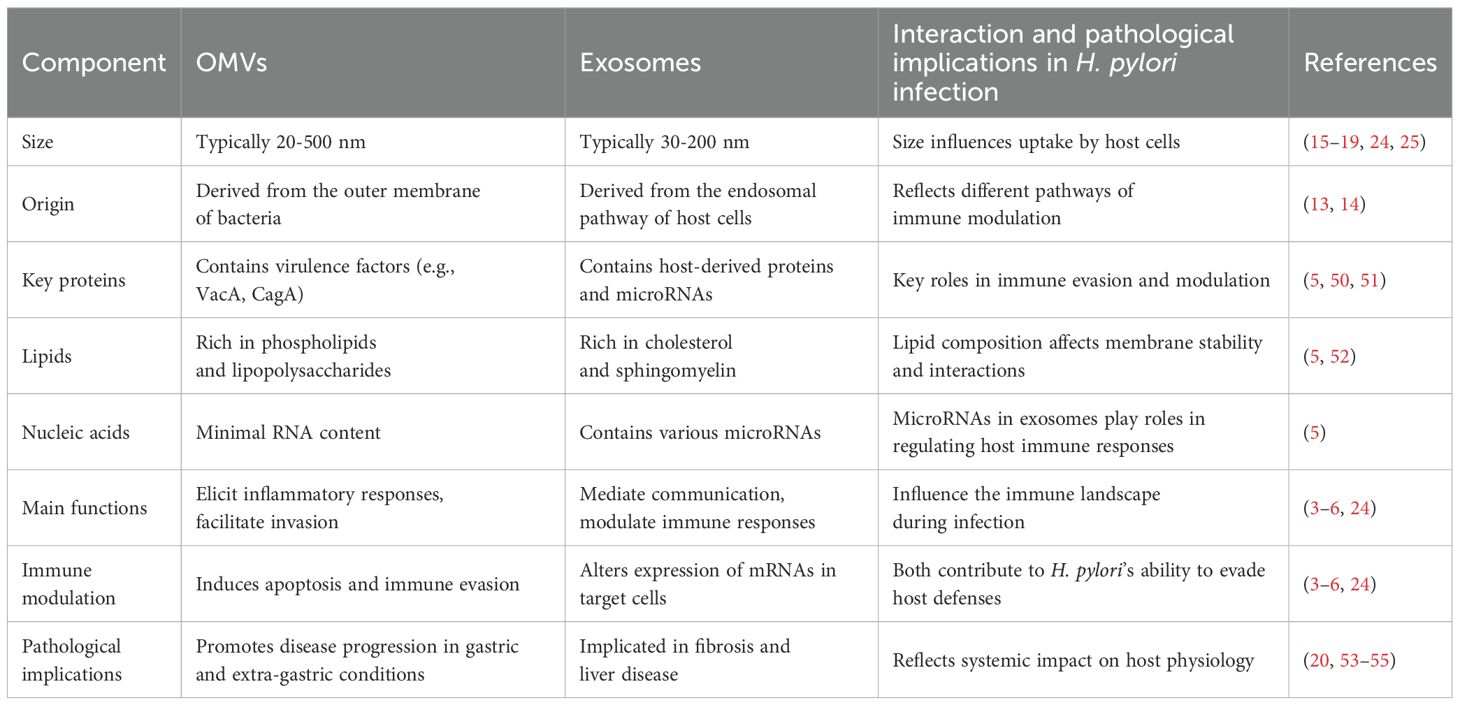
Table 1. Comparison of the major components and pathological implications of OMVs and exosomes in H. pylori infection.
Evidence suggests that modifications in exosomal cargo during bacterial infections represent a novel mechanism of systemic pathogenesis (53, 54). For instance, exosomes from H. pylori OMV-infected hepatocytes have been shown to induce the expression of α-smooth muscle actin (α-SMA), tissue inhibitor of metalloproteinases-1 (TIMP-1), β-catenin, and vimentin in hepatic stellate cells (HSCs), while concurrently downregulating E-cadherin gene and protein levels (55). These findings indicate that H. pylori OMVs may affect exosome composition, thereby contributing to HSC activation and the progression of liver fibrosis. Exploring the intricate mechanisms of interaction between H. pylori OMVs and exosomes holds promise for unveiling novel therapeutic strategies for H. pylori-associated diseases. However, the mechanisms underlying these interactions remain unclear due to insufficient research.
5 Modulation of H. pylori OMVs and infected cell exosomes on immune responses
Understanding the specific components of OMVs and exosomes that contribute to H. pylori pathogenesis is crucial for elucidating their roles in inducing immune suppression and the persistence of infection in the host immune environment (Table 2). This review provides an in-depth look at how OMVs released by infected gastric epithelial cells can mobilize and stimulate immune cells, including macrophages and T cells, fostering the persistent inflammatory environment typical of H. pylori-related conditions. Conversely, exosomes originating from H. pylori-infected cells can alter the activity of immune cells, fostering an immunosuppressive setting conducive to bacterial colonization and the circumvention of host immune monitoring (Figure 3).
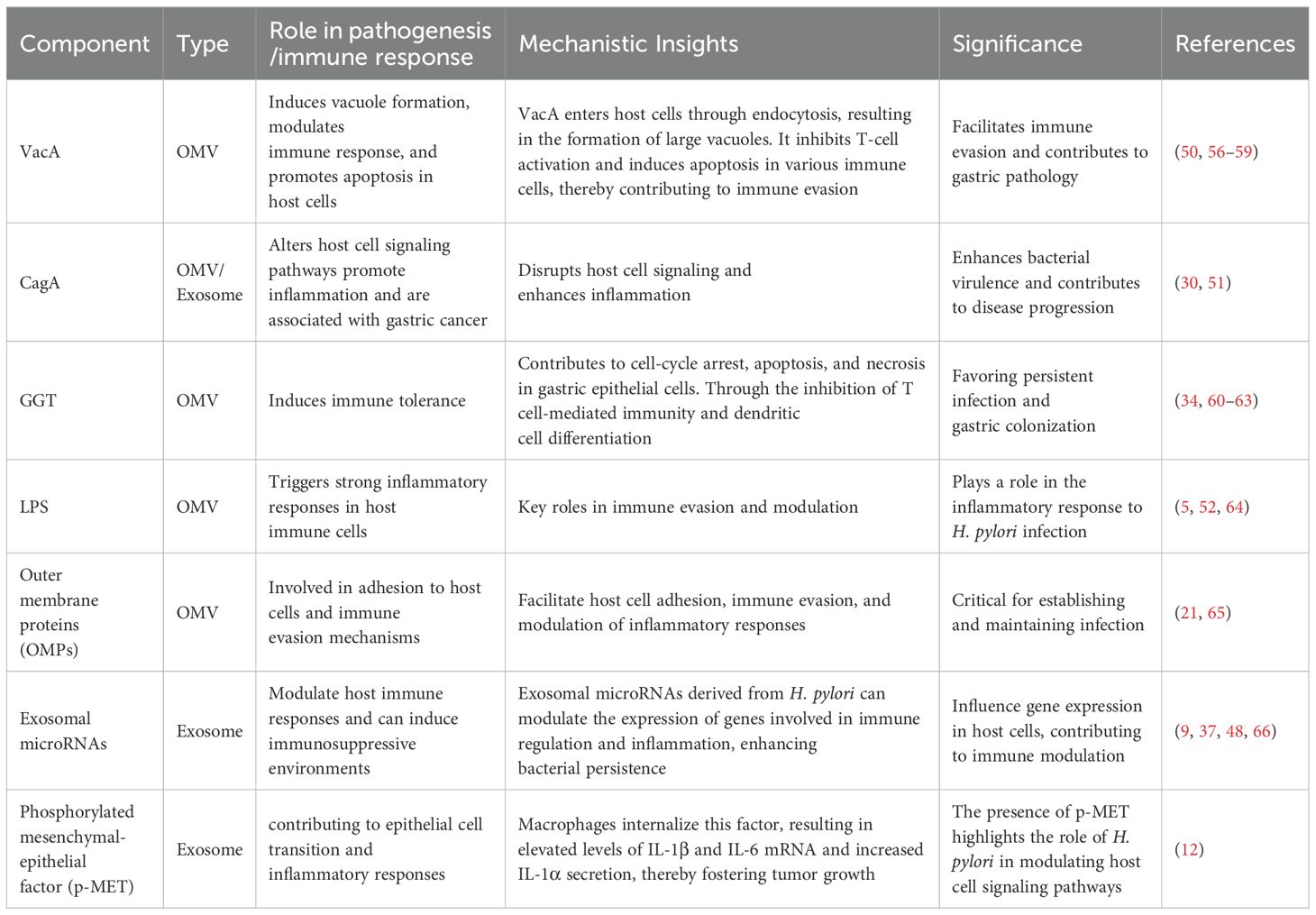
Table 2. Key components of H. pylori OMVs and infected cell exosomes involved in pathogenesis and immune response.
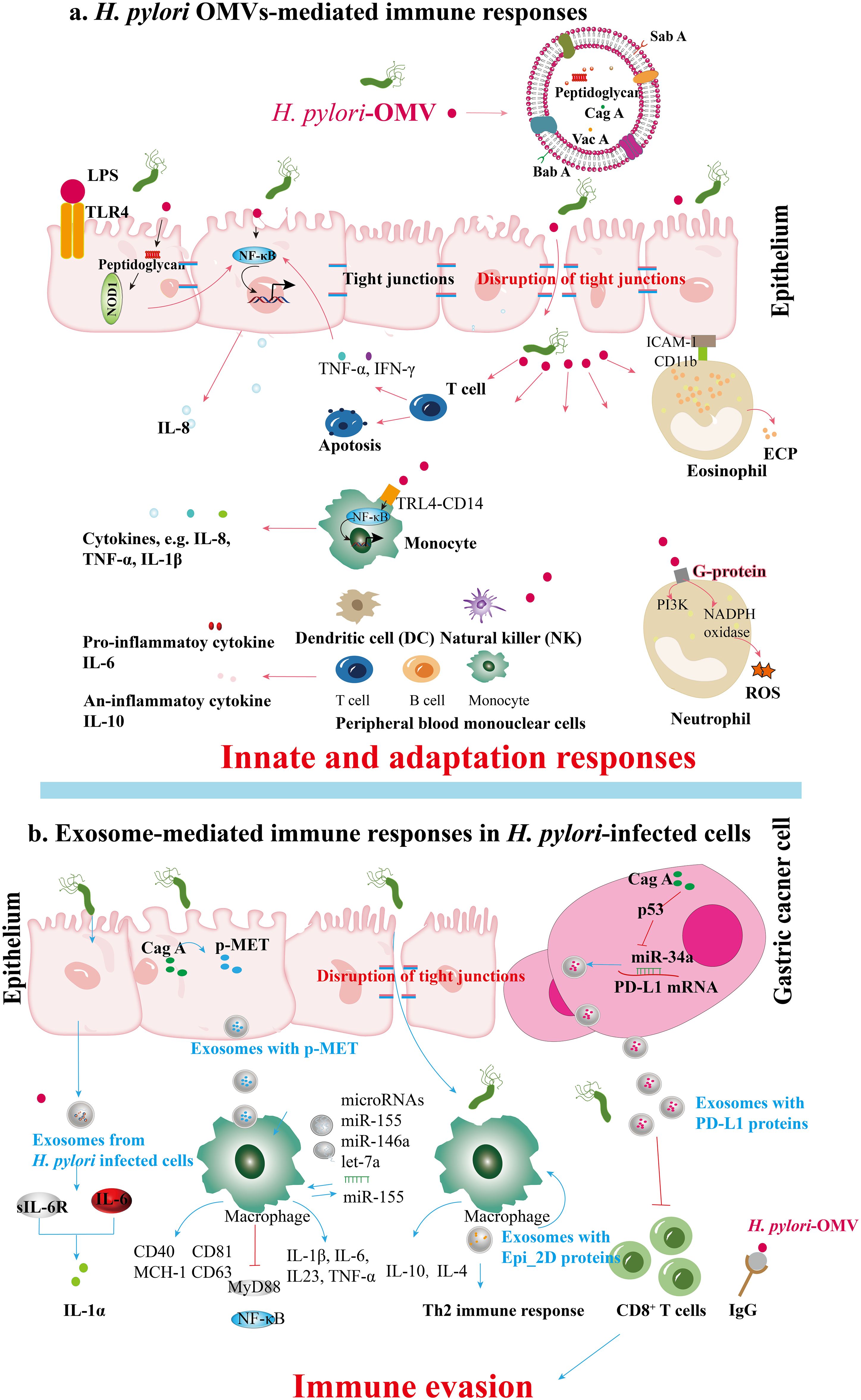
Figure 3. Immune responses mediated by (H) pylori OMVs and infected cell exosomes. (A) BabA and SabA, found in H. pylori OMVs, enhance adhesion to the gastric mucosa. Peptidoglycan within H. pylori OMVs activates the NOD1 response. During H. pylori infection, OMVs induce the secretion of cytokines like TNF-α and IFN-γ from T cells and stimulate gastric epithelial cytokine release (e.g., IL-8) via NF-κB activation. OMV ligands binding TLR4 and CD14 on monocytes activate NF-κB, enhancing cytokine release. H. pylori OMVs upregulate ICAM-1 and CD11b integrin expression, leading to degranulation and ECP release; these OMVs also activate NADPH oxidase in neutrophils through GPCR signaling and kinase pathways, inducing oxidative burst and ROS production. Furthermore, H. pylori OMVs can induce serum IgG responses, potentially aiding immune evasion during H. pylori pathogenesis. (B) Exosomes from H. pylori-infected human gastric epithelial cells secrete GES-1, inducing the expression of soluble IL-6 receptor that mediates the promotion of proinflammatory cytokines such as IL-1α. CagA promotes the disruption of intercellular tight junctions. After H. pylori infection, vesicles containing p-MET are released. Macrophages subsequently take up these vesicles, which release proinflammatory cytokines that enhance tumor growth and stimulate cell proliferation, migration, and invasion. Apart from the cell signaling proteins CD81, CD63, CD40, and MCH-I, exosomes containing miR-155 from H. pylori-infected macrophages also enhance the secretion of inflammatory cytokines IL-23, IL-6, IL-1β, and TNF-α. Simultaneously, miR-155 expression activates MyD88 signaling pathways and NF-κB inflammatory protein in macrophages during H. pylori infection. The exosomes derived from H. pylori-infected cells contain a specific protein, Epi_2D, which triggers a Th2 immune response, producing IL-4 and IL-10. H. pylori’s CagA inhibits p53 and miR-34a, leading to the upregulation of PD-L1 levels in gastric cancer cells, which inhibits CD8+ T cell proliferation and anti-cancer response, promoting immune evasion in gastric cancer.
5.1 Immune cell activation and immune tolerance modulation by H. pylori OMVs and infected cell exosomes
OMVs derived from Gram-negative bacteria, including H. pylori, carry various pathogen-associated molecular patterns (PAMPs), such as lipopolysaccharides (LPS), lipoproteins, peptidoglycans, and bacterial nucleic acids. These PAMPs interact with pattern recognition receptors (PRRs) on host immune cells, particularly epithelial cells in mucosal tissues, influencing host pathology, immune tolerance, and protective immunity (64). H. pylori OMVs activate Toll-like receptor (TLR) signaling pathways, resulting in the release of pro-inflammatory cytokines such as interleukin-1β (IL-1β), tumor necrosis factor-alpha (TNF-α), and interleukin-6 (IL-6), which attract immune cells to the infection site (67, 68). This cytokine release is critical in mediating mucosal damage caused by H. pylori, as it triggers the secretion of chemokines and the activation of nuclear factor-kappa B (NF-κB) (69). Components of H. pylori, including LPS, heat shock protein 60 (HSP60), and nucleic acids, are recognized via various TLRs (70). The subsequent activation of NF-κB and mitogen-activated protein kinase (MAPK) pathways plays a key role in regulating pro- and anti-inflammatory responses, contributing to the immune response and disease progression (70). Cytoplasmic receptors such as nucleotide-binding oligomerization domain 1 (NOD1) and NOD2 recognize OMVs containing peptidoglycan, a major bacterial cell wall component, thus initiating critical innate immune responses (71). Activating NF-κB by H. pylori OMVs that engage NOD1 can increase IL-8 expression, potentially contributing to various gastric disorders (23). During T-cell activation, H. pylori infection promotes the secretion of TNF-α and interferon-gamma (IFN-γ) through NF-κB signaling, which stimulates gastric epithelial cells to produce IL-8 (72, 73). The binding of H. pylori OMVs to TLR4 and CD14 on mononuclear cells triggers NF-κB activation and subsequent cytokine release. Moreover, components within H. pylori OMVs can induce both pro-inflammatory cytokines (e.g., IL-6) and anti-inflammatory cytokines (e.g., IL-10), while also initiating oxidative bursts and apoptosis in Jurkat T cells (74, 75). This oxidative burst is mediated by the activation of nicotinamide adenine dinucleotide phosphate (NADPH) oxidase through components like neutrophil-activating protein A (NAP), leading to upregulation of β2 integrin expression and attraction of leukocytes to epithelial cells (76, 77). Ultimately, this process induces degranulation in eosinophils, resulting in the release of eosinophil cationic protein (ECP) and increased expression of ICAM-1 on epithelial cells and CD11b integrin on eosinophils (78) (Figure 3A).
Exosomes released from infected cells, including those during pathogen infections, have been shown to contain microbial components that modulate host immunity (24, 79). These exosomes can enhance antigen presentation and stimulate macrophages, potentially influencing disease progression (80). In the case of H. pylori, the bacterium can manipulate the release and content of exosomes to promote its survival and colonization in the gastric mucosa, indicating a complex mechanism of exosome-mediated communication that supports chronic infection. Che et al. (2018) investigated the interactions facilitated by exosomes between H. pylori-infected gastric cancer cells and macrophages. Their findings revealed that gastric cancer cells release phosphorylated mesenchymal-epithelial factor (p-MET) following H. pylori infection. Macrophages internalize p-MET, leading to increased levels of IL-1β and IL-6 mRNA and elevated IL-1α secretion, which fosters tumor growth (12). Another study focused on serum exosomes in the IL-6-mediated regulation of inflammatory cytokines. Analysis of exosomes from individuals with chronic gastritis infected with H. pylori showed upregulation of IL-1α and soluble IL-6 receptor (sIL-6R) in human gastric epithelial cells (GES-1). The pro-inflammatory role of IL-6, mediated by sIL-6R, promotes the secretion of additional inflammatory cytokines, while IL-1α contributes to the inflammatory response in various diseases (39, 81–83). Despite the limited number of studies, exosomes play a crucial role in the abnormal inflammatory response induced by H. pylori infection, with specific mechanisms still poorly understood. Exosomes from H. pylori-infected cells can modulate immune cell activation through various pathways, including the regulation by microRNAs such as miR-155, which influences the host immune response (9, 48). Research by Wang et al. (2016) and Atrisco-Morales et al. (2022) highlighted the role of exosomes in promoting inflammatory responses, particularly emphasizing miR-155’s involvement (9, 66). Furthermore, Chen et al. (2018) underscored the immunomodulatory functions of exosomes in educating tumor-associated macrophages (39). Recent studies by Faass et al. (2023) and Ahmed et al. (2021) explored the interactions between H. pylori and immune cells, with Faass et al. examining myeloid cell activation by H. pylori metabolites and Ahmed et al. identifying Epimerase_2 domain-containing protein (Epi_2D) in H. pylori-infected cell exosomes that induce a Th2 immune response (84, 85). These studies highlight the significant impact of exosomes derived from H. pylori-infected cells on immune cell activation (Figure 3B).
H. pylori-infected cell exosomes have been implicated in promoting the differentiation of T helper 17 (Th17) cells, known for producing pro-inflammatory cytokines like IL-17 (86). These exosomes can also shift immune cells toward a regulatory phenotype, inhibiting effector T-cell responses and fostering immune tolerance in the gastric mucosa (87). The immunological functions of exosomes, particularly their involvement in adjusting antigen presentation and immune activation, have been extensively studied (88). Moreover, H. pylori infection triggers a combined Th17/Th1 cell response, where the Th17/IL-17 pathway influences Th1 cell responses, contributing to disease progression (89). The role of regulatory T cells in responding to H. pylori infection has also been investigated, focusing on their role in the anti-bacterial immune response (90). Additionally, H. pylori infection-deriving exosomal proteins, including HSPs and virulence factors, can modulate host cell immune signaling pathways, fostering immune tolerance and persistent infection (91). These findings indicate that H. pylori OMVs and infected cell exosomes can impact the host’s immune response and play a role in chronic infection and immune tolerance.
5.2 Immune evasion modulation by H. pylori OMVs and infected cell exosomes
H. pylori OMVs play a significant role in immune evasion by inducing serum immunoglobulin G (IgG) responses, notably through antibodies that target bacterial Lewis epitopes (92). In hosts with cross-reactive blood group structures, these antibodies may contribute to the autoimmune processes associated with H. pylori pathogenesis. The differential packaging of host mRNA within OMVs as small non-coding RNAs (sncRNAs) further modulates the host immune response (93). For example, Zhang et al. found that sncRNA-2509025 and sncRNA-989262 could reduce IL-8 secretion triggered by lipopolysaccharide or OMVs in AGS cells cultured in vitro, thereby facilitating immune evasion (94). Additionally, Li et al. demonstrated that these sncRNAs play a crucial role in regulating the immune response in H. pylori-infected animal hosts by increasing serum IgG and IgA levels. Their depletion negatively impacted mucosal and humoral immunity, underscoring the direct role of sncRNAs in modulating host immune responses and aiding H. pylori in evading host defenses (95). These results suggest that sncRNAs within H. pylori OMVs directly modulate the host immune response, aiding H. pylori in evading host immunity. Nonetheless, additional investigations on host-pathogen interactions are necessary.
H. pylori employs various strategies to evade host immunity, including modifying surface molecules to avoid detection by innate immune receptors and modulating effector T-cell responses (2, 96, 97). Exosomes released during H. pylori infection serves a dual role, acting as an agent of immunosuppression and immune evasion (24). These exosomes deliver virulence factors and immunomodulatory molecules to host cells, subverting immune defenses and fostering an environment conducive to bacterial survival (98, 99). They promote the expansion of regulatory T cells and inhibit antigen presentation, creating a tolerogenic setting that facilitates bacterial colonization (98). Research has indicated a significant correlation between the presence of CagA protein in tumor tissues, high PD-L1 expression rates, elevated plasma PD-L1 levels, and lymph node metastasis in gastric cancer patients with H. pylori infection. Mechanistically, CagA hinders CD8+ T cell proliferation and anti-cancer responses by enhancing PD-L1 levels in exosomes from gastric cancer cells through p53 and miRNA-34a inhibition. Targeting CagA and extracellularly secreted PD-L1 could improve the efficacy of immunotherapy against H. pylori-related gastric cancer (51) (Figure 3B).
6 Role of H. pylori OMVs and infected cell exosomes in chronic inflammation and pathogenesis
The presence of confirmed and potential virulence factors in H. pylori OMVs suggests that these vesicles play a crucial role in delivering toxins and virulence factors to the gastric epithelium, contributing to the chronic inflammation associated with H. pylori infection. For instance, studies have shown that VacA associated with OMVs can induce cavitation in gastric epithelial cells (58, 59). Additionally, OMVs contain the virulence factor NAPA, which exerts a chemotactic effect on human neutrophils and monocytes, stimulating the production of reactive oxygen intermediates (ROIs) that can damage gastric epithelial cells and enhance nutrient release for the bacteria (76). The uptake of OMVs containing VacA increases cytoplasmic iron levels while concurrently reducing glutathione (GSH), creating an oxidative environment that elevates ROI levels and is associated with DNA damage. Internalized OMVs can induce apoptosis in gastric epithelial cells via a caspase-dependent, mitochondria-independent pathway, leading to decreased cell viability and DNA fragmentation, with activation of caspase-8, -9, and -3 observed despite the absence of cytochrome C release (57). Another virulence factor, gamma-glutamyl transpeptidase (GGT), induces cell cycle arrest, apoptosis, and necrosis by depleting GSH and generating reactive oxygen species, further promoting apoptosis in epithelial cells (61, 100). GGT also enhances H. pylori persistence and colonization by promoting immune tolerance by inhibiting T cell-mediated immunity and dendritic cell differentiation (62, 63).
Exosomes released from H. pylori-infected cells can also induce the release of pro-inflammatory cytokines and chemokines, leading to tissue damage, gastritis, and progression to more severe H. pylori-related diseases (11, 31). For instance, these exosomes modulate the expression of IL-1α in gastric epithelial cells, contributing to chronic gastritis (12). In patients with H. pylori infection, CagA was isolated from serum samples, and its overexpression in gastric epithelial cells resulted in the release of exosomes containing phosphorylated CagA. Treatment of WT-A10 gastric epithelial cells with these exosomes induced an elongated cell morphology characteristic of the hummingbird phenotype (30). A different study revealed that gastric fibroblasts (GF) are direct targets of H. pylori (101). Moreover, gastric fibroblasts (GFs) are direct targets of H. pylori. H. pylori-activated GFs release exosomes with significantly increased levels of miR-124-3p, promoting the expression of cancer-associated fibroblast (CAF) biomarkers. H. pylori infection enhances GF proliferation and migration by facilitating the release of exosomes loaded with miR-124-3p, an inhibitor of SNAI2 (101). This exosomal communication influences the gastric microenvironment, fostering a pro-inflammatory and tumorigenic milieu that facilitates disease progression (11). Furthermore, exosomes facilitate the transfer of molecules such as activated MET protein, instructing tumor-associated macrophages to enhance gastric cancer progression (12). Exosomes from gastric cancer cells also contribute to forming pre-metastatic niches (102), while those from infected gastric epithelial cells can induce carcinogenic changes in recipient cells (103). Overall, these findings underscore the essential role of OMVs and exosome-mediated signaling in the pathogenesis of H. pylori-related gastric diseases (Figure 4).
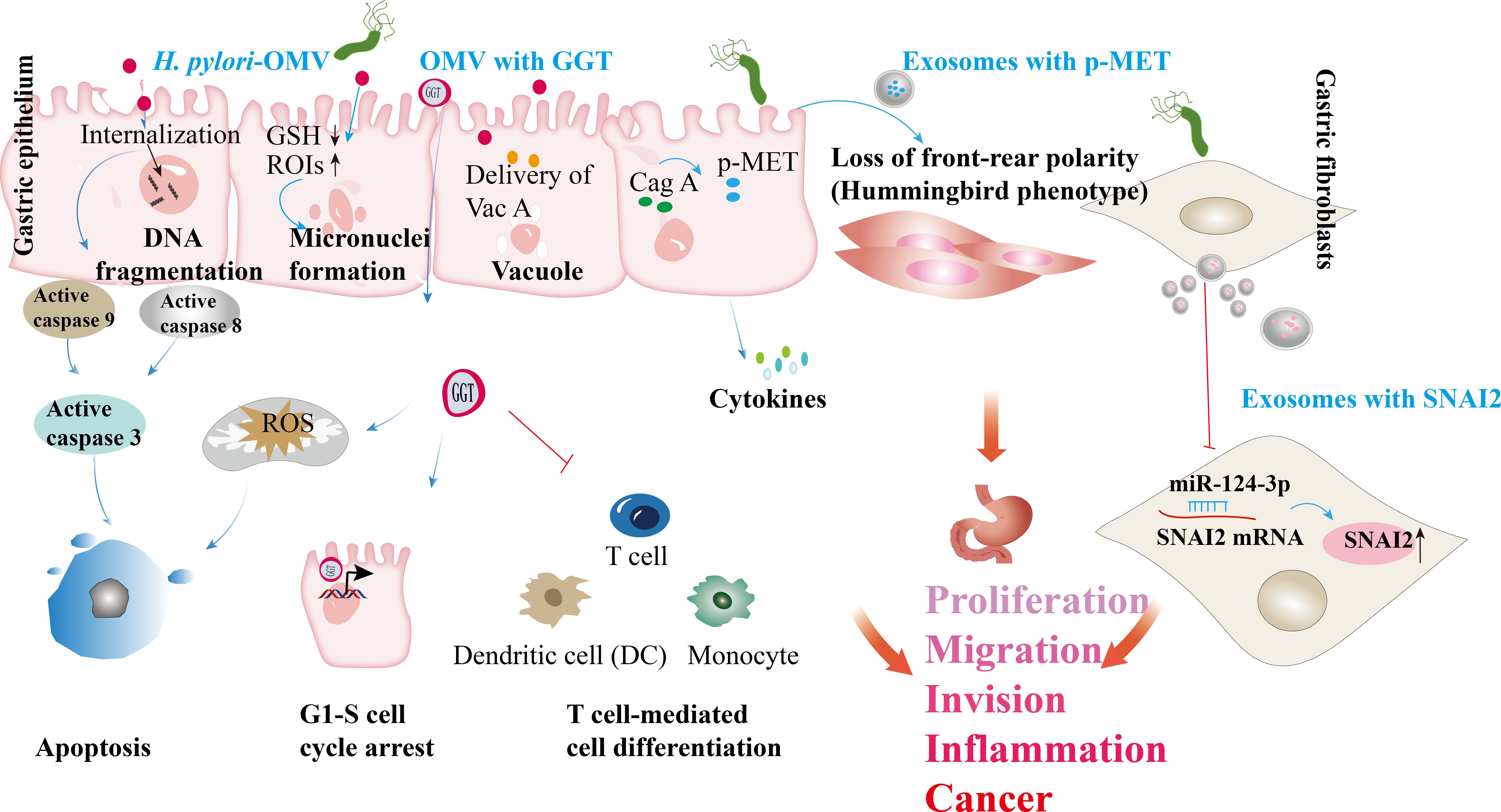
Figure 4. H. pylori OMVs and infected cell exosomes play a role in the development of gastric diseases. Internalizing H. pylori OMVs into epithelial cells triggers DNA fragmentation and activates caspase-8, 9, and 3. The absorption of OMVs carrying the VacA leads to reduced GSH levels (GSH↓). The absorption of OMVs carrying the VacA leads to reduced GSH levels. In this oxidative environment, reactive oxygen intermediates (ROIs) increase (ROIs↑), contributing to DNA damage. GGT from H. pylori triggers the production of ROS, leading to cell apoptosis. GGT can induce cell cycle arrest via nuclear reactions and immune tolerance, suppressing T cell-mediated immunity and dendritic cell differentiation. H. pylori OMVs trigger the formation of micronuclei and vacuoles through VacA. H. pylori infecting host cell-derived exosomes has been linked to gastric cancer development. In H. pylori infection, the virulence factor CagA is delivered to gastric epithelial cells; released exosomes carrying phosphorylated CagA (p-CagA) trigger cellular morphology changes. Post H. pylori infection, the mesenchymal epithelium releases exosomes with p-MET, internalized by macrophages, triggering proinflammatory cytokine release and supporting tumor progression. H. pylori infection enhances the proliferation and migration of gastric fibroblasts (GFs) by promoting the secretion of exosomes containing miR-124-3p, which is associated with the SNAI2 protein levels (↑ indicates increased SNAI2 levels).
H. pylori OMVs and exosomes released from infected cells can enter the bloodstream and reach other tissues, potentially causing extra-gastric ailments. Chronic stomach colonization by H. pylori produces OMVs that disrupt tight junctions in the intestinal epithelium, resulting in increased blood-brain barrier (BBB) permeability. This compromised BBB facilitates the infiltration of OMVs into the brain parenchyma, where they stimulate microglia and astrocytes, exacerbating neuronal damage associated with amyloid β or tau pathology and accelerating neurodegeneration around amyloid plaques, thereby contributing to Alzheimer’s disease (104). Exosomes serve as important carriers of H. pylori components, elucidating the mechanisms of how H. pylori induces localized and systemic changes. For instance, exosomes derived from plasma and gastric epithelial cells can transport H. pylori CagA into circulation, potentially promoting the progression of extra-gastric diseases (30). In the intestinal epithelium, exosomes from H. pylori-positive individuals increase levels of NOD-like receptor family pyrin domain containing 12 (NLRP12), which suppresses the expression of chemokines, such as monocyte chemoattractant protein-1 (MCP-1) and macrophage inflammatory protein-1α (MIP-1α), through inhibition of the Notch signaling pathway, thereby alleviating colitis symptoms (105). CagA-containing exosomes from human gastric epithelial cells transcriptionally modulate the expression of claudin-2 via a caudal-type homeobox 2 (CDX2)-dependent mechanism, impairing intestinal mucosal repair. Additionally, exosomes from H. pylori-infected cells may compromise endothelial function, potentially increasing susceptibility to cardiovascular diseases (106). Macrophages exposed to exosomes rich in microRNAs from H. pylori-infected cells can either suppress H. pylori proliferation or induce pro-inflammatory responses, depending on the specific microRNA content. H. pylori also stimulates gastric epithelial cells to release circulating miR-25-enriched exosomes that modulate the NF-κB pathway via Kruppel-like factor 2 (KLF2). This modulation enhances the expression of intercellular adhesion molecule-1 (ICAM-1), MCP-1, vascular cell adhesion molecule 1 (VCAM-1), and IL-6, potentially accelerating the progression of atherosclerosis (107). Moreover, CagA within exosomes from H. pylori-infected gastric epithelial cells inhibits cholesterol transporter protein transcription by downregulating transcription factors such as peroxisome proliferator-activated receptor gamma (PPARγ) and liver X receptor alpha (LXRα), which promotes the formation of foam cells from macrophages (108). Additionally, CagA-rich exosomes from H. pylori-infected vascular endothelial cells induce reactive oxygen species generation in endothelial cells (109) (Figure 5).
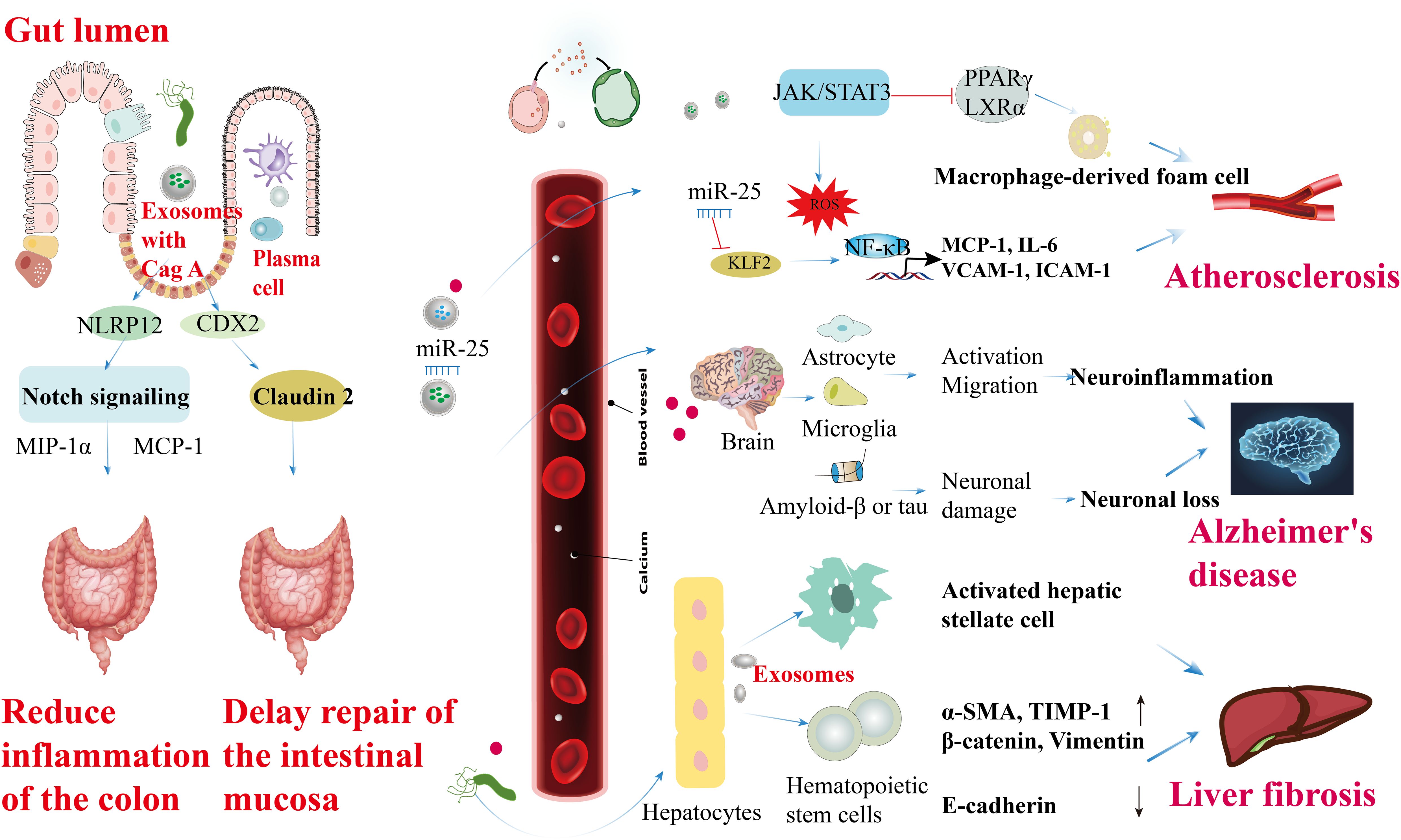
Figure 5. Roles of H. pylori OMVs and infected cell exosomes in the extra-gastric diseases. CagA-containing exosomes enhance NLRP12 expression in intestinal epithelial cells, inhibiting the Notch signaling pathway and reducing the levels of chemokines like MIP-1α and MCP-1, ultimately improving colitis symptoms. Extracellular secretion of CagA by CDX2-dependent mechanism from GES-1 modulates transcriptionally, correlating with claudin-2 protein expression to facilitate intestinal mucosal repair delays. CagA secretion from H. pylori in vascular endothelial cells induces ROS formation, leading to endothelial cell activation, JAK-STAT3 activation factor signaling disruption, promoting macrophage foam cell formation, and atherosclerosis development. Circulating miR-25-containing exosomes can modulate the NF-κB signaling pathway by targeting the transcription factor KLF2, subsequently increasing the expression of ICAM-1, MCP-1, VCAM-1, and IL-6, thus accelerating atherosclerosis in vascular endothelial cells. Furthermore, OMVs produced during chronic H. pylori infection in the intestinal tract activate microglia and astrocytes to promote their migration. This activation, along with the enhancement of amyloid β or tau, can trigger inflammation in the central nervous system, potentially elucidating the higher prevalence of Alzheimer’s disease in individuals with H. pylori infection. Lastly, H. pylori infection induces the expression of α-SMA, TIMP-1, β-catenin, and vimentin (α-SMA, TIMP-1, β-catenin, and vimentin ↑) in hematopoietic stem cells derived from hepatocytes, while downregulating the expression of E-cadherin at both the gene and protein levels (E-cadherin ↓), ultimately contributing to liver fibrosis.
7 Diagnostic and prognostic significance of OMVs and exosomes in H. pylori-associated diseases
Recent research emphasizes the significant role of OMVs and exosomes in managing H. pylori infections, especially concerning rising antibiotic resistance. These vesicles are increasingly recognized for their potential in immune modulation and targeted delivery of antimicrobial agents and as biomarkers in diagnostics. Table 3 outlines key strategies that utilize OMVs and exosomes to enhance treatment efficacy and facilitate non-invasive detection methods for H. pylori-related diseases.
8 Challenges and future directions in investigating OMVs and exosomes associated with H. pylori infection
Understanding the pathogenicity of H. pylori, mainly through its OMVs and related exosomes, is essential for developing effective diagnostic and therapeutic strategies. However, current research in this area faces several limitations that impede a comprehensive understanding of the mechanisms of action and their translational applications. To address these challenges, we summarize the primary limitations of current research in Table 4. These limitations include challenges related to the standardization of isolation methods, the suitability of in vitro models, and gaps in our knowledge about the biogenesis and functions of exosomes in the context of H. pylori infection.
Future research must adopt a focused approach to address these challenges effectively. Table 5 presents key future research directions to improve our understanding of H. pylori OMVs and the effects of exosomes released by infected cells. These research directions emphasize the need to investigate the mechanisms of chronic infection, the interactions between exosomes and gastric microbiota, and the use of multi-omics techniques to clarify the complex interplay between H. pylori and the host immune response.
9 Conclusion
In conclusion, this review focuses on the underexplored role of H. pylori-derived OMVs and exosomes in host immune modulation, aiming to provide new insights into the interactions between bacteria and host cells, with particular emphasis on the dynamic interplay mediated by these vesicles. Although current research has yielded valuable insights, challenges remain in fully elucidating the mechanisms underlying H. pylori OMVs and exosomes derived from infected cells. Future studies should prioritize integrating advanced techniques, such as single-cell sequencing and proteomics, and explore specific signaling pathways and molecular mechanisms to enhance our understanding of OMVs and exosomes. This approach will facilitate the development of innovative strategies for targeted therapeutic interventions in H. pylori-related diseases.
Author contributions
XW: Funding acquisition, Writing – original draft, Writing – review & editing. JW: Writing – original draft, Writing – review & editing. LM: Writing – original draft. YY: Writing – original draft, Writing – review & editing.
Funding
The author(s) declare financial support was received for the research, authorship, and/or publication of this article. This work was supported by the Open Fund Project of Jiangsu Key Laboratory of Medical Science and Laboratory Medicine [Grant number JSKLM-Y-2024-015] and Kunshan First People’s Hospital Healthcare Science and Technology Innovation Special Project [Grant number KETDCX202431].
Conflict of interest
The authors declare that the research was conducted in the absence of any commercial or financial relationships that could be construed as a potential conflict of interest.
Generative AI statement
The author(s) declare that no Generative AI was used in the creation of this manuscript.
Publisher’s note
All claims expressed in this article are solely those of the authors and do not necessarily represent those of their affiliated organizations, or those of the publisher, the editors and the reviewers. Any product that may be evaluated in this article, or claim that may be made by its manufacturer, is not guaranteed or endorsed by the publisher.
References
1. Sugano K, Tack J, Kuipers EJ, Graham DY, El-Omar EM, Miura S, et al. Kyoto global consensus report on Helicobacter pylori gastritis. Gut. (2015) 64:1353–67. doi: 10.1136/gutjnl-2015-309252
2. Karkhah A, Ebrahimpour S, Rostamtabar M, Koppolu V, Darvish S, Vasigala VKR, et al. Helicobacter pylori evasion strategies of the host innate and adaptive immune responses to survive and develop gastrointestinal diseases. Microbiol Res. (2019) 218:49–57. doi: 10.1016/j.micres.2018.09.011
3. Chmiela M, Walczak N, Rudnicka K. Helicobacter pylori outer membrane vesicles involvement in the infection development and Helicobacter pylori-related diseases. J BioMed Sci. (2018) 25:78. doi: 10.1186/s12929-018-0480-y
4. Ahmed AAQ, Besio R, Xiao L, Forlino A. Outer Membrane Vesicles (OMVs) as Biomedical Tools and Their Relevance as Immune-Modulating Agents against H. pylori Infections: Current Status and Future Prospects. Int J Mol Sci. (2023) 24:8542. doi: 10.3390/ijms24108542
5. Schweer D, Anand N, Anderson A, McCorkle JR, Neupane K, Nail AN, et al. Human macrophage-engineered vesicles for utilization in ovarian cancer treatment. Front Oncol. (2022) 12:1042730. doi: 10.3389/fonc.2022.1042730
6. Harvey BT, Fu X, Li L, Neupane KR, Anand N, Kolesar JM, et al. Dendritic cell membrane-derived nanovesicles for targeted T cell activation. ACS Omega. (2022) 7:46222–33. doi: 10.1021/acsomega.2c04420
7. Kalluri R, LeBleu VS. The biology, function, and biomedical applications of exosomes. Science. (2020) 367:eaau6977. doi: 10.1126/science.aau6977
8. Pegtel DM, Gould SJ. Exosomes. Annu Rev Biochem. (2019) 88:487–514. doi: 10.1146/annurev-biochem-013118-111902
9. Wang J, Deng Z, Wang Z, Wu J, Gu T, Jiang Y, et al. MicroRNA-155 in exosomes secreted from helicobacter pylori infection macrophages immunomodulates inflammatory response. Am J Transl Res. (2016) 8:3700–9.
10. Wang C, Li W, Shao L, Zhou A, Zhao M, Li P, et al. Both extracellular vesicles from helicobacter pylori-infected cells and helicobacter pylori outer membrane vesicles are involved in gastric/extra gastric diseases. Eur J Med Res. (2023) 28:484. doi: 10.1186/s40001-023-01458-z
11. González MF, Díaz P, Sandoval-Bórquez A, Herrera D, Quest AFG. Helicobacter pylori Outer Membrane Vesicles and Extracellular Vesicles from Helicobacter pylori-Infected Cells in Gastric Disease Development. Int J Mol Sci. (2021) 22:4823. doi: 10.3390/ijms22094823
12. Che Y, Geng B, Xu Y, Miao X, Chen L, Mu X, et al. Helicobacter pylori-induced exosomal MET educates tumour-associated macrophages to promote gastric cancer progression. J Cell Mol Med. (2018) 22:5708–19. doi: 10.1111/jcmm.2018.22.issue-11
13. Toyofuku M, Nomura N, Eberl L. Types and origins of bacterial membrane vesicles. Nat Rev Microbiol. (2019) 17:13–24. doi: 10.1038/s41579-018-0112-2
14. Tulkens J, De Wever O, Hendrix A. Analyzing bacterial extracellular vesicles in human body fluids by orthogonal biophysical separation and biochemical characterization. Nat Protoc. (2020) 15:40–67. doi: 10.1038/s41596-019-0236-5
15. Kaparakis M, Turnbull L, Carneiro L, Firth S, Coleman HA, Parkington HC, et al. Bacterial membrane vesicles deliver peptidoglycan to NOD1 in epithelial cells. Cell Microbiol. (2010) 12:372–85. doi: 10.1111/j.1462-5822.2009.01404.x
16. Turner L, Bitto NJ, Steer DL, Lo C, D’Costa K, Ramm G, et al. Helicobacter pylori outer membrane vesicle size determines their mechanisms of host cell entry and protein content. Front Immunol. (2018) 9:1466. doi: 10.3389/fimmu.2018.01466
17. Zavan L, Bitto NJ, Johnston EL, Greening DW, Kaparakis-Liaskos M. Helicobacter pylori growth stage determines the size, protein composition, and preferential cargo packaging of outer membrane vesicles. Proteomics. (2019) 19:e1800209. doi: 10.1002/pmic.201800209
18. Sartorio MG, Pardue EJ, Feldman MF, Haurat MF. Bacterial outer membrane vesicles: from discovery to applications. Annu Rev Microbiol. (2021) 75:609–30. doi: 10.1146/annurev-micro-052821-031444
19. Olofsson A, Vallström A, Petzold K, Tegtmeyer N, Schleucher J, Carlsson S, et al. Biochemical and functional characterization of Helicobacter pylori vesicles. Mol Microbiol. (2010) 77:1539–55. doi: 10.1111/j.1365-2958.2010.07307.x
20. Shegefti S, Bolori S, Nabavi-Rad A, Dabiri H, Yadegar A, Baghaei K. Helicobacter pylori-derived outer membrane vesicles suppress liver autophagy: A novel mechanism for H. pylori-mediated hepatic disorder. Microb Pathog. (2023) 183:106319. doi: 10.1016/j.micpath.2023.106319
21. Mullaney E, Brown PA, Smith SM, Botting CH, Yamaoka YY, Terres AM, et al. Proteomic and functional characterization of the outer membrane vesicles from the gastric pathogen Helicobacter pylori. Proteomics Clin Appl. (2009) 3:785–96. doi: 10.1002/prca.200800192
22. Liu Q, Li X, Zhang Y, Song Z, Li R, Ruan H, et al. Orally-administered outer-membrane vesicles from Helicobacter pylori reduce H. pylori infection via Th2-biased immune responses in mice. Pathog Dis. (2019) 77:ftz050. doi: 10.1093/femspd/ftz050
23. Choi MS, Ze EY, Park JY, Shin TS, Kim JG. Helicobacter pylori-derived outer membrane vesicles stimulate interleukin 8 secretion through nuclear factor kappa B activation. Korean J Intern Med. (2021) 36:854–67. doi: 10.3904/kjim.2019.432
24. Schorey JS, Cheng Y, Singh PP, Smith VL. Exosomes and other extracellular vesicles in host-pathogen interactions. EMBO Rep. (2015) 16:24–43. doi: 10.15252/embr.201439363
25. Krylova SV, Feng D. The machinery of exosomes: biogenesis, release, and uptake. Int J Mol Sci. (2023) 24:1337. doi: 10.3390/ijms24021337
26. Zhang Y, Liu Y, Liu H, Tang WH. Exosomes: biogenesis, biologic function and clinical potential. Cell Biosci. (2019) 9:19. doi: 10.1186/s13578-019-0282-2
27. Babst M. MVB vesicle formation: ESCRT-dependent, ESCRT-independent and everything in between. Curr Opin Cell Biol. (2011) 23:452–7. doi: 10.1016/j.ceb.2011.04.008
28. Spencer N, Yeruva L. Role of bacterial infections in extracellular vesicles release and impact on immune response. BioMed J. (2021) 44:157–64. doi: 10.1016/j.bj.2020.05.006
29. Wang J, Li Y, Wang N, Wu J, Ye X, Jiang Y, et al. Functions of exosomal non-coding RNAs to the infection with Mycobacterium tuberculosis. Front Immunol. (2023) 14:1127214. doi: 10.3389/fimmu.2023.1127214
30. Shimoda A, Ueda K, Nishiumi S, Murata-Kamiya N, Mukai SA, Sawada S, et al. Exosomes as nanocarriers for systemic delivery of the Helicobacter pylori virulence factor CagA. Sci Rep. (2016) 6:18346. doi: 10.1038/srep18346
31. Choi HI, Choi JP, Seo J, Kim BJ, Rho M, Han JK, et al. Helicobacter pylori-derived extracellular vesicles increased in the gastric juices of gastric adenocarcinoma patients and induced inflammation mainly via specific targeting of gastric epithelial cells. Exp Mol Med. (2017) 49:e330. doi: 10.1038/emm.2017.47
32. Kusters JG, van Vliet AH, Kuipers EJ. Pathogenesis of Helicobacter pylori infection. Clin Microbiol Rev. (2006) 19:449–90. doi: 10.1128/CMR.00054-05
33. Sgouras D, Tegtmeyer N, Wessler S. Activity and functional importance of helicobacter pylori virulence factors. Adv Exp Med Biol. (2019) 1149:35–56. doi: 10.1007/5584_2019_358
34. Polakovicova I, Jerez S, Wichmann IA, Sandoval-Bórquez A, Carrasco-Véliz N, Corvalán AH. Role of microRNAs and Exosomes in Helicobacter pylori and Epstein-Barr Virus Associated Gastric Cancers. Front Microbiol. (2018) 9:636. doi: 10.3389/fmicb.2018.00636
35. Naumann M, Sokolova O, Tegtmeyer N, Backert S. Helicobacter pylori: A paradigm pathogen for subverting host cell signal transmission. Trends Microbiol. (2017) 25:316–28. doi: 10.1016/j.tim.2016.12.004
36. Blaser N, Backert S, Pachathundikandi SK. Immune cell signaling by helicobacter pylori: impact on gastric pathology. Adv Exp Med Biol. (2019) 1149:77–106. doi: 10.1007/5584_2019_360
37. Cadamuro AC, Rossi AF, Maniezzo NM, Silva AE. Helicobacter pylori infection: host immune response, implications on gene expression and microRNAs. World J Gastroenterol. (2014) 20:1424–37. doi: 10.3748/wjg.v20.i6.1424
38. Fernandez-Gonzalez E, Backert S. DNA transfer in the gastric pathogen Helicobacter pylori. J Gastroenterol. (2014) 49:594–604. doi: 10.1007/s00535-014-0938-y
39. Chen Y, Wang X, Yu Y, Xiao Y, Huang J, Yao Z, et al. Serum exosomes of chronic gastritis patients infected with Helicobacter pylori mediate IL-1α expression via IL-6 trans-signalling in gastric epithelial cells. Clin Exp Immunol. (2018) 194:339–49. doi: 10.1111/cei.13200
40. Khoei SG, Sadeghi H, Saidijam M. The use of exosome carrier to augmentation of Helicobacter pylori infection treatment. Stem Cell Investig. (2020) 7:23. doi: 10.21037/sci-2020-028
41. Zhong Y, Li H, Li P, Chen Y, Zhang M, Yuan Z, et al. Exosomes: A new pathway for cancer drug resistance. Front Oncol. (2021) 11:743556. doi: 10.3389/fonc.2021.743556
42. Li S, Yi M, Dong B, Jiao Y, Luo S, Wu K. The roles of exosomes in cancer drug resistance and its therapeutic application. Clin Transl Med. (2020) 10:e257. doi: 10.1186/s12967-019-02199-6
43. Hu Y, Zhang M, Lu B, Dai J. Helicobacter pylori and antibiotic resistance, A continuing and intractable problem. Helicobacter. (2016) 21:349–63. doi: 10.1111/hel.2016.21.issue-5
44. Rodrigues M, Fan J, Lyon C, Wan M, Hu Y. Role of extracellular vesicles in viral and bacterial infections: pathogenesis, diagnostics, and therapeutics. Theranostics. (2018) 8:2709–21. doi: 10.7150/thno.20576
45. Belair C, Darfeuille F, Staedel C. Helicobacter pylori and gastric cancer: possible role of microRNAs in this intimate relationship. Clin Microbiol Infect. (2009) 15:806–12. doi: 10.1111/j.1469-0691.2009.02960.x
46. Zabaleta J. MicroRNA: A bridge from H. pylori infection to gastritis and gastric cancer development. Front Genet. (2012) 3:294. doi: 10.3389/fgene.2012.00294
47. Libânio D, Dinis-Ribeiro M, Pimentel-Nunes P. Helicobacter pylori and microRNAs: Relation with innate immunity and progression of preneoplastic conditions. World J Clin Oncol. (2015) 6:111–32. doi: 10.5306/wjco.v6.i5.111
48. Săsăran MO, Meliţ LE, Dobru ED. MicroRNA modulation of host immune response and inflammation triggered by helicobacter pylori. Int J Mol Sci. (2021) 22:1406. doi: 10.3390/ijms22031406
49. Wei S, Li X, Wang J, Wang Y, Zhang C, Dai S, et al. Outer membrane vesicles secreted by helicobacter pylori transmitting gastric pathogenic virulence factors. ACS Omega. (2022) 7:240–58. doi: 10.1021/acsomega.1c04549
50. Xu XH, Shao SL, Guo D, Ge LN, Wang Z, Liu P, et al. Roles of microRNAs and exosomes in Helicobacter pylori associated gastric cancer. Mol Biol Rep. (2023) 50:889–97. doi: 10.1007/s11033-022-08073-x
51. Wang J, Deng R, Chen S, Deng S, Hu Q, Xu B, et al. Helicobacter pylori CagA promotes immune evasion of gastric cancer by upregulating PD-L1 level in exosomes. iScience. (2023) 26:108414. doi: 10.1016/j.isci.2023.108414
52. Keenan JI, Davis KA, Beaugie CR, McGovern JJ, Moran AP. Alterations in Helicobacter pylori outer membrane and outer membrane vesicle-associated lipopolysaccharides under iron-limiting growth conditions. Innate Immun. (2008) 14:279–90. doi: 10.1177/1753425908096857
53. Fleming A, Sampey G, Chung MC, Bailey C, van Hoek ML, Kashanchi F, et al. The carrying pigeons of the cell: exosomes and their role in infectious diseases caused by human pathogens. Pathog Dis. (2014) 71:109–20. doi: 10.1111/2049-632X.12135
54. Wang J, Yao Y, Chen X, Wu J, Gu T, Tang X. Host derived exosomes-pathogens interactions: Potential functions of exosomes in pathogen infection. BioMed Pharmacother. (2018) 108:1451–9. doi: 10.1016/j.biopha.2018.09.174
55. Zahmatkesh ME, Jahanbakhsh M, Hoseini N, Shegefti S, Peymani A, Dabin H, et al. Effects of exosomes derived from helicobacter pylori outer membrane vesicle-infected hepatocytes on hepatic stellate cell activation and liver fibrosis induction. Front Cell Infect Microbiol. (2022) 12:857570. doi: 10.3389/fcimb.2022.857570
56. Ricci V, Chiozzi V, Necchi V, Oldani A, Romano M, Solcia E, et al. Free-soluble and outer membrane vesicle-associated VacA from Helicobacter pylori: Two forms of release, a different activity. Biochem Biophys Res Commun. (2005) 337:173–8. doi: 10.1016/j.bbrc.2005.09.035
57. Ayala G, Torres L, Espinosa M, Fierros-Zarate G, Maldonado V, Meléndez-Zajgla J. External membrane vesicles from Helicobacter pylori induce apoptosis in gastric epithelial cells. FEMS Microbiol Lett. (2006) 260:178–85. doi: 10.1111/j.1574-6968.2006.00305.x
58. Fiocca R, Necchi V, Sommi P, Ricci V, Telford J, Cover TL, et al. Release of Helicobacter pylori vacuolating cytotoxin by both a specific secretion pathway and budding of outer membrane vesicles. Uptake of released toxin and vesicles by gastric epithelium. J Pathol. (1999) 188:220–6. doi: 10.1002/(SICI)1096-9896(199906)188:2<220::AID-PATH307>3.0.CO;2-C
59. Keenan J, Day T, Neal S, Cook B, Perez-Perez G, Allardyce R, et al. A role for the bacterial outer membrane in the pathogenesis of Helicobacter pylori infection. FEMS Microbiol Lett. (2000) 182:259–64. doi: 10.1111/j.1574-6968.2000.tb08905.x
60. Ricci V, Giannouli M, Romano M, Zarrilli R. Helicobacter pylori gamma-glutamyl transpeptidase and its pathogenic role. World J Gastroenterol. (2014) 20:630–8. doi: 10.3748/wjg.v20.i3.630
61. Zhang G, Ducatelle R, Pasmans F, D’Herde K, Huang L, Smet A, et al. Effects of Helicobacter suis γ-glutamyl transpeptidase on lymphocytes: modulation by glutamine and glutathione supplementation and outer membrane vesicles as a putative delivery route of the enzyme. PloS One. (2013) 8:e77966. doi: 10.1371/journal.pone.0077966
62. Kim KM, Lee SG, Kim JM, Kim DS, Song JY, Kang HL, et al. Helicobacter pylori gamma-glutamyltranspeptidase induces cell cycle arrest at the G1-S phase transition. J Microbiol. (2010) 48:372–7. doi: 10.1007/s12275-010-9293-8
63. Saini M, Kashyap A, Bindal S, Saini K, Gupta R. Bacterial gamma-glutamyl transpeptidase, an emerging biocatalyst: insights into structure-function relationship and its biotechnological applications. Front Microbiol. (2021) 12:641251. doi: 10.3389/fmicb.2021.641251
64. Riley DR, Sieber KB, Robinson KM, White JR, Ganesan A, Nourbakhsh S, et al. Bacteria-human somatic cell lateral gene transfer is enriched in cancer samples. PloS Comput Biol. (2013) 9:e1003107. doi: 10.1371/journal.pcbi.1003107
65. Penfold CN, Li C, Zhang Y, Vankemmelbeke M, James R. Colicin A binds to a novel binding site of TolA in the Escherichia coli periplasm. Biochem Soc Trans. (2012) 40:1469–74. doi: 10.1042/BST20120239
66. Atrisco-Morales J, Ramírez M, Castañón-Sánchez CA, Román-Román A, Román-Fernández IV, Martínez-Carrillo DN, et al. In peripheral blood mononuclear cells helicobacter pylori induces the secretion of soluble and exosomal cytokines related to carcinogenesis. Int J Mol Sci. (2022) 23:8801. doi: 10.3390/ijms23158801
67. Hu Y, Liu JP, Zhu Y, Lu NH. The importance of toll-like receptors in NF-κB signaling pathway activation by helicobacter pylori infection and the regulators of this response. Helicobacter. (2016) 21:428–40. doi: 10.1111/hel.2016.21.issue-5
68. Smith SM. Role of Toll-like receptors in Helicobacter pylori infection and immunity. World J Gastrointest Pathophysiol. (2014) 5:133–46. doi: 10.4291/wjgp.v5.i3.133
69. Crabtree JE. Role of cytokines in pathogenesis of Helicobacter pylori-induced mucosal damage. Dig Dis Sci. (1998) 43:46s–55s. doi: 10.1159/000016881
70. Pachathundikandi SK, Lind J, Tegtmeyer N, El-Omar EM, Backert S. Interplay of the gastric pathogen helicobacter pylori with toll-like receptors. BioMed Res Int. (2015) 2015:192420. doi: 10.1155/2015/192420
71. Thay B, Damm A, Kufer TA, Wai SN, Oscarsson J. Aggregatibacter actinomycetemcomitans outer membrane vesicles are internalized in human host cells and trigger NOD1- and NOD2-dependent NF-κB activation. Infect Immun. (2014) 82:4034–46. doi: 10.1128/IAI.01980-14
72. Maeda S, Akanuma M, Mitsuno Y, Hirata Y, Ogura K, Yoshida H, et al. Distinct mechanism of Helicobacter pylori-mediated NF-kappa B activation between gastric cancer cells and monocytic cells. J Biol Chem. (2001) 276:44856–64. doi: 10.1074/jbc.M105381200
73. Ismail S, Hampton MB, Keenan JI. Helicobacter pylori outer membrane vesicles modulate proliferation and interleukin-8 production by gastric epithelial cells. Infect Immun. (2003) 71:5670–5. doi: 10.1128/IAI.71.10.5670-5675.2003
74. Winter J, Letley D, Rhead J, Atherton J, Robinson K. Helicobacter pylori membrane vesicles stimulate innate pro- and anti-inflammatory responses and induce apoptosis in Jurkat T cells. Infect Immun. (2014) 82:1372–81. doi: 10.1128/IAI.01443-13
75. Parker H, Keenan JI. Composition and function of Helicobacter pylori outer membrane vesicles. Microbes Infect. (2012) 14:9–16. doi: 10.1016/j.micinf.2011.08.007
76. Satin B, Del Giudice G, Della Bianca V, Dusi S, Laudanna C, Tonello F, et al. The neutrophil-activating protein (HP-NAP) of Helicobacter pylori is a protective antigen and a major virulence factor. J Exp Med. (2000) 191:1467–76. doi: 10.1084/jem.191.9.1467
77. Unemo M, Aspholm-Hurtig M, Ilver D, Bergström J, Borén T, Danielsson D, et al. The sialic acid binding SabA adhesin of Helicobacter pylori is essential for nonopsonic activation of human neutrophils. J Biol Chem. (2005) 280:15390–7. doi: 10.1074/jbc.M412725200
78. Ko SH, Jeon JI, Kim YJ, Yoon HJ, Kim H, Kim N, et al. Helicobacter pylori outer membrane vesicle proteins induce human eosinophil degranulation via a β2 Integrin CD11/CD18- and ICAM-1-dependent mechanism. Mediators Inflammation. (2015) 2015:301716. doi: 10.1155/2015/301716
79. Schorey JS, Bhatnagar S. Exosome function: from tumor immunology to pathogen biology. Traffic. (2008) 9:871–81. doi: 10.1111/j.1600-0854.2008.00734.x
80. Alenquer M, Amorim MJ. Exosome biogenesis, regulation, and function in viral infection. Viruses. (2015) 7:5066–83. doi: 10.3390/v7092862
81. Rose-John S. IL-6 trans-signaling via the soluble IL-6 receptor: importance for the pro-inflammatory activities of IL-6. Int J Biol Sci. (2012) 8:1237–47. doi: 10.7150/ijbs.4989
82. Rose-John S. The soluble interleukin 6 receptor: advanced therapeutic options in inflammation. Clin Pharmacol Ther. (2017) 102:591–8. doi: 10.1002/cpt.v102.4
83. Malik A, Kanneganti TD. Function and regulation of IL-1α in inflammatory diseases and cancer. Immunol Rev. (2018) 281:124–37. doi: 10.1111/imr.2018.281.issue-1
84. Faass L, Hauke M, Stein SC, Josenhans C. Innate immune activation and modulatory factors of Helicobacter pylori towards phagocytic and nonphagocytic cells. Curr Opin Immunol. (2023) 82:102301. doi: 10.1016/j.coi.2023.102301
85. Ahmed AAQ, Qi F, Zheng R, Xiao L, Abdalla AME, Mao L, et al. The impact of ExHp-CD (outer membrane vesicles) released from Helicobacter pylori SS1 on macrophage RAW 264.7 cells and their immunogenic potential. Life Sci. (2021) 279:119644. doi: 10.1016/j.lfs.2021.119644
86. Liu C, Zhang Z, Zhu M. Immune responses mediated by th17 cells in helicobacter pylori infection. Integr Med Int. (2016) 3:57–63. doi: 10.1159/000446317
87. Larussa T, Leone I, Suraci E, Imeneo M, Luzza F. Helicobacter pylori and T helper cells: mechanisms of immune escape and tolerance. J Immunol Res. (2015) 2015:981328. doi: 10.1155/2015/981328
88. Greening DW, Gopal SK, Xu R, Simpson RJ, Chen W. Exosomes and their roles in immune regulation and cancer. Semin Cell Dev Biol. (2015) 40:72–81. doi: 10.1016/j.semcdb.2015.02.009
89. Shi Y, Liu XF, Zhuang Y, Zhang JY, Liu T, Yin Z, et al. Helicobacter pylori-induced Th17 responses modulate Th1 cell responses, benefit bacterial growth, and contribute to pathology in mice. J Immunol. (2010) 184:5121–9. doi: 10.4049/jimmunol.0901115
90. Dixon B, Hossain R, Patel RV, Algood HMS. Th17 cells in helicobacter pylori infection: a dichotomy of help and harm. Infect Immun. (2019) 87:e00363–19. doi: 10.1128/IAI.00363-19
91. Zhang W, Jiang X, Bao J, Wang Y, Liu H, Tang L. Exosomes in pathogen infections: A bridge to deliver molecules and link functions. Front Immunol. (2018) 9:90. doi: 10.3389/fimmu.2018.00090
92. Hynes SO, Keenan JI, Ferris JA, Annuk H, Moran AP. Lewis epitopes on outer membrane vesicles of relevance to Helicobacter pylori pathogenesis. Helicobacter. (2005) 10:146–56. doi: 10.1111/j.1523-5378.2005.00302.x
93. Luo X, Esberard M, Bouloc P, Jacq A. A small regulatory RNA generated from the malK 5’ Untranslated region targets gluconeogenesis in vibrio species. mSphere. (2021) 6:e0013421. doi: 10.1128/mSphere.00134-21
94. Zhang H, Zhang Y, Song Z, Li R, Ruan H, Liu Q, et al. sncRNAs packaged by Helicobacter pylori outer membrane vesicles attenuate IL-8 secretion in human cells. Int J Med Microbiol. (2020) 310:151356. doi: 10.1016/j.ijmm.2019.151356
95. Li B, Xu Y, Xu T, Guo Z, Xu Q, Li Y, et al. Disruption of sncRNA Improves the Protective Efficacy of Outer Membrane Vesicles against Helicobacter pylori Infection in a Mouse Model. Infect Immun. (2022) 90:e0026722. doi: 10.1128/iai.00267-22
96. Fan J, Zhu J, Xu H. Strategies of Helicobacter pylori in evading host innate and adaptive immunity: insights and prospects for therapeutic targeting. Front Cell Infect Microbiol. (2024) 14:1342913. doi: 10.3389/fcimb.2024.1342913
97. Wang X, Zhao G, Shao S, Yao Y. Helicobacter pylori triggers inflammation and oncogenic transformation by perturbing the immune microenvironment. Biochim Biophys Acta Rev Cancer. (2024) 1897:189139. doi: 10.1016/j.bbcan.2024.189139
98. Mejías-Luque R, Gerhard M. Immune evasion strategies and persistence of helicobacter pylori. Curr Top Microbiol Immunol. (2017) 400:53–71. doi: 10.1007/978-3-319-50520-6_3
99. Lina TT, Alzahrani S, Gonzalez J, Pinchuk IV, Beswick EJ, Reyes VE. Immune evasion strategies used by Helicobacter pylori. World J Gastroenterol. (2014) 20:12753–66. doi: 10.3748/wjg.v20.i36.12753
100. Shirin H, Pinto JT, Liu LU, Merzianu M, Sordillo EM, Moss SF. Helicobacter pylori decreases gastric mucosal glutathione. Cancer Lett. (2001) 164:127–33. doi: 10.1016/S0304-3835(01)00383-4
101. Li J, Li X, Zhang Z, Wang S, Huang X, Min L, et al. Helicobacter pylori promotes gastric fibroblast proliferation and migration by expulsing exosomal miR-124-3p. Microbes Infect. (2024) 26:105236. doi: 10.1016/j.micinf.2023.105236
102. Gao J, Li S, Xu Q, Zhang X, Huang M, Dai X, et al. Exosomes promote pre-metastatic niche formation in gastric cancer. Front Oncol. (2021) 11:652378. doi: 10.3389/fonc.2021.652378
103. González MF, Burgos-Ravanal R, Shao B, Heinecke J, Valenzuela-Valderrama M, Corvalán AH, et al. Extracellular vesicles from gastric epithelial GES-1 cells infected with Helicobacter pylori promote changes in recipient cells associated with Malignancy. Front Oncol. (2022) 12:962920. doi: 10.3389/fonc.2022.962920
104. Park AM, Tsunoda I. Helicobacter pylori infection in the stomach induces neuroinflammation: the potential roles of bacterial outer membrane vesicles in an animal model of Alzheimer’s disease. Inflammation Regener. (2022) 42:39. doi: 10.1186/s41232-022-00224-8
105. Chen Y, Huang J, Li H, Li P, Xu C. Serum exosomes derived from Hp-positive gastritis patients inhibit MCP-1 and MIP-1α expression via NLRP12-Notch signaling pathway in intestinal epithelial cells and improve DSS-induced colitis in mice. Int Immunopharmacol. (2020) 88:107012. doi: 10.1016/j.intimp.2020.107012
106. Xia X, Zhang L, Chi J, Li H, Liu X, Hu T, et al. Helicobacter pylori infection impairs endothelial function through an exosome-mediated mechanism. J Am Heart Assoc. (2020) 9:e014120. doi: 10.1161/JAHA.119.014120
107. Li N, Liu SF, Dong K, Zhang GC, Huang J, Wang ZH, et al. Exosome-Transmitted miR-25 Induced by H. pylori Promotes Vascular Endothelial Cell Injury by Targeting KLF2. Front Cell Infect Microbiol. (2019) 9:366. doi: 10.3389/fcimb.2019.00366
108. Yang S, Xia YP, Luo XY, Chen SL, Li BW, Ye ZM, et al. Exosomal CagA derived from Helicobacter pylori-infected gastric epithelial cells induces macrophage foam cell formation and promotes atherosclerosis. J Mol Cell Cardiol. (2019) 135:40–51. doi: 10.1016/j.yjmcc.2019.07.011
109. Tahmina K, Hikawa N, Takahashi-Kanemitsu A, Knight CT, Sato K, Itoh F, et al. Transgenically expressed Helicobacter pylori CagA in vascular endothelial cells accelerates arteriosclerosis in mice. Biochem Biophys Res Commun. (2022) 618:79–85. doi: 10.1016/j.bbrc.2022.06.010
110. Batrakova EV, Kim MS. Using exosomes, naturally-equipped nanocarriers, for drug delivery. J Control Release. (2015) 219:396–405. doi: 10.1016/j.jconrel.2015.07.030
111. Conway BR. Drug delivery strategies for the treatment of Helicobacter pylori infections. Curr Pharm Des. (2005) 11:775–90. doi: 10.2174/1381612053381819
112. Fonseca DR, Chitas R, Parreira P, Martins MCL. How to manage Helicobacter pylori infection beyond antibiotics: The bioengineering quest. Appl Materials Today. (2024) 37. doi: 10.1016/j.apmt.2024.102123
113. Liang Y, Duan L, Lu J, Xia J. Engineering exosomes for targeted drug delivery. Theranostics. (2021) 11:3183–95. doi: 10.7150/thno.52570
114. Fu S, Wang Y, Xia X, Zheng JC. Exosome engineering: Current progress in cargo loading and targeted delivery. NanoImpact. (2020) 20:100261. doi: 10.1016/j.impact.2020.100261
115. Qin Y, Lao YH, Wang H, Zhang J, Yi K, Chen Z, et al. Combatting Helicobacter pylori with oral nanomedicines. J Mater Chem B. (2021) 9:9826–38. doi: 10.1039/D1TB02038B
116. Kamankesh M, Yadegar A, Llopis-Lorente A, Liu C, Haririan I, Aghdaei HA, et al. Future nanotechnology-based strategies for improved management of helicobacter pylori infection. Small. (2024) 20:e2302532. doi: 10.1002/smll.202302532
117. Every AL. Key host-pathogen interactions for designing novel interventions against Helicobacter pylori. Trends Microbiol. (2013) 21:253–9. doi: 10.1016/j.tim.2013.02.007
118. Luan X, Sansanaphongpricha K, Myers I, Chen H, Yuan H, Sun D. Engineering exosomes as refined biological nanoplatforms for drug delivery. Acta Pharmacol Sin. (2017) 38:754–63. doi: 10.1038/aps.2017.12
119. Song Z, Li B, Zhang Y, Li R, Ruan H, Wu J, et al. Outer Membrane Vesicles of Helicobacter pylori 7.13 as Adjuvants Promote Protective Efficacy Against Helicobacter pylori Infection. Front Microbiol. (2020) 11:1340. doi: 10.3389/fmicb.2020.01340
120. Ayala G, Escobedo-Hinojosa WI, de la Cruz-Herrera CF, Romero I. Exploring alternative treatments for Helicobacter pylori infection. World J Gastroenterol. (2014) 20:1450–69. doi: 10.3748/wjg.v20.i6.1450
121. Wu X, Jin S, Ding C, Wang Y, He D, Liu Y. Mesenchymal stem cell-derived exosome therapy of microbial diseases: from bench to bed. Front Microbiol. (2021) 12:804813. doi: 10.3389/fmicb.2021.804813
122. Vítor JM, Vale FF. Alternative therapies for Helicobacter pylori: probiotics and phytomedicine. FEMS Immunol Med Microbiol. (2011) 63:153–64. doi: 10.1111/j.1574-695X.2011.00865.x
123. Lekmeechai S, Su YC, Brant M, Alvarado-Kristensson M, Vallström A, Obi I, et al. Helicobacter pylori outer membrane vesicles protect the pathogen from reactive oxygen species of the respiratory burst. Front Microbiol. (2018) 9:1837. doi: 10.3389/fmicb.2018.01837
124. Okuda Y, Shimura T, Iwasaki H, Katano T, Kitagawa M, Nishigaki R, et al. Serum exosomal dicer is a useful biomarker for early detection of differentiated gastric adenocarcinoma. Digestion. (2021) 102:640–9. doi: 10.1159/000510993
125. Jiang Z, Huang A-l, Tao X-h, Wang P-l. Diagnosis of Helicobacter pylori infection and diseases associated with Helicobacter pylori by Helicobacter pylori outer membrane proteins. World J Gastroenterol. (2004) 10:3464–9. doi: 10.3748/wjg.v10.i23.3464
126. Wang YK, Kuo FC, Liu CJ, Wu MC, Shih HY, Wang SS, et al. Diagnosis of Helicobacter pylori infection: Current options and developments. World J Gastroenterol. (2015) 21:11221–35. doi: 10.3748/wjg.v21.i40.11221
127. Khalilpour A, Kazemzadeh-Narbat M, Tamayol A, Oklu R, Khademhosseini A. Biomarkers and diagnostic tools for detection of Helicobacter pylori. Appl Microbiol Biotechnol. (2016) 100:4723–34. doi: 10.1007/s00253-016-7495-7
128. Sousa C, Ferreira R, Santos SB, Azevedo NF, Melo LDR. Advances on diagnosis of Helicobacter pylori infections. Crit Rev Microbiol. (2023) 49:671–92. doi: 10.1080/1040841X.2022.2125287
129. Lin J, Li J, Huang B, Liu J, Chen X, Chen XM, et al. Exosomes: novel biomarkers for clinical diagnosis. ScientificWorldJournal. (2015) 2015:657086. doi: 10.1155/tswj.v2015.1
130. Vakil N, Vaira D. Non-invasive tests for the diagnosis of H. pylori infection. Rev Gastroenterol Disord. (2004) 4:1–6.
131. Sabbagh P, Mohammadnia-Afrouzi M, Javanian M, Babazadeh A, Koppolu V, Vasigala VKR, et al. Diagnostic methods for Helicobacter pylori infection: ideals, options, and limitations. Eur J Clin Microbiol Infect Dis. (2019) 38:55–66. doi: 10.1007/s10096-018-3414-4
132. Windle HJ. Isolation of outer membrane vesicles from helicobacter pylori. Methods Mol Biol. (2021) 2283:123–30. doi: 10.1007/978-1-0716-1302-3_13
133. Melo J, Pinto V, Fernandes T, Malheiro AR, Osório H, Figueiredo C, et al. Isolation method and characterization of outer membranes vesicles of helicobacter pylori grown in a chemically defined medium. Front Microbiol. (2021) 12:654193. doi: 10.3389/fmicb.2021.654193
134. Xie J, Li Q, Haesebrouck F, Van Hoecke L, Vandenbroucke RE. The tremendous biomedical potential of bacterial extracellular vesicles. Trends Biotechnol. (2022) 40:1173–94. doi: 10.1016/j.tibtech.2022.03.005
135. Carriere J, Barnich N, Nguyen HTT. Exosomes: from functions in host-pathogen interactions and immunity to diagnostic and therapeutic opportunities. Rev physiol Biochem Pharmacol. (2016) 172:39–75. doi: 10.1007/112_2016_7
Keywords: Helicobacter pylori, exosome, outer membrane vesicles, immunomodulation, inflammation
Citation: Wang X, Wang J, Mao L and Yao Y (2024) Helicobacter pylori outer membrane vesicles and infected cell exosomes: new players in host immune modulation and pathogenesis. Front. Immunol. 15:1512935. doi: 10.3389/fimmu.2024.1512935
Received: 17 October 2024; Accepted: 27 November 2024;
Published: 13 December 2024.
Edited by:
Won Fen Wong, University of Malaya, MalaysiaReviewed by:
Naresh Kumar, The Ohio State University, United StatesNamrata Anand, University of Chicago Medical Center, United States
Copyright © 2024 Wang, Wang, Mao and Yao. This is an open-access article distributed under the terms of the Creative Commons Attribution License (CC BY). The use, distribution or reproduction in other forums is permitted, provided the original author(s) and the copyright owner(s) are credited and that the original publication in this journal is cited, in accordance with accepted academic practice. No use, distribution or reproduction is permitted which does not comply with these terms.
*Correspondence: Xiuping Wang, MTg4NTI4NjIyMjhAMTYzLmNvbQ==