- 1Division of Anatomical Pathology, National Health Laboratory Service, University of Pretoria, Hatfield, South Africa
- 2SAMRC Precision Oncology Research Unit (PORU), DSI/NRF SARChI Chair in Precision Oncology and Cancer Prevention (POCP), Pan African Cancer Research Institute (PACRI), University of Pretoria, Hatfield, South Africa
Malignant melanoma, the most aggressive form of skin cancer, is characterized by unpredictable growth patterns, and its mortality rate has remained alarmingly high over recent decades, despite various treatment approaches. One promising strategy for improving outcomes in melanoma patients lies in the early use of biomarkers to predict prognosis. Biomarkers offer a way to gauge patient outlook early in the disease course, facilitating timely, targeted intervention. In recent years, considerable attention has been given to the immune response’s role in melanoma, given the tumor’s high immunogenicity and potential responsiveness to immunologic treatments. Researchers are focusing on identifying predictive biomarkers by examining both cancer cell biology and immune interactions within the tumor microenvironment (TME). This approach has shed light on tumor-infiltrating lymphocytes (TILs), a type of immune cell found within the tumor. TILs have emerged as a promising area of study for their potential to serve as both a prognostic indicator and therapeutic target in melanoma. The presence of TILs in melanoma tissue can often signal a positive immune response to the cancer, with numerous studies suggesting that TILs may improve patient prognosis. This review delves into the prognostic value of TILs in melanoma, assessing how these immune cells influence patient outcomes. It explores the mechanisms through which TILs interact with melanoma cells and the potential clinical applications of leveraging TILs in treatment strategies. While TILs present a hopeful avenue for prognostication and treatment, there are still challenges. These include understanding the full extent of TIL dynamics within the TME and overcoming limitations in TIL-based therapies. Advancements in TIL characterization methods are also critical to refining TIL-based approaches. By addressing these hurdles, TIL-focused research may pave the way for improved diagnostic and therapeutic options, ultimately offering better outcomes for melanoma patients.
1 Introduction
Melanoma, the most deadly type of skin cancer, presents a serious clinical issue due to its rapid spread and resistance to traditional treatments. Melanocytes are responsible for this increasingly common condition (1). The tumor, node, and metastasis (TNM) method is used to histologically classify melanoma. Tumors are categorized based on distinct features such as ulceration, lesion thickness, and mitosis. Additional characteristics used to classify melanoma include lymph node involvement and metastasis distance from the source tumor (2, 3). Multiple studies have demonstrated that melanoma has a complicated, multistage genesis that is impacted by hereditary and environmental factors. Numerous benign lesions have been shown to have alterations in the v-raf murine sarcoma viral oncogene homolog B (BRAF) at codon V600E. However, changes in these genes must also occur in other genes participating in various cellular processes for the disease to proceed (4, 5). Target genes, including phosphatase and tensin homolog (PTEN), neurofibromin 1 (NF1), cyclin-dependent kinase inhibitor 2A (CDKN2A), telomerase reverse transcriptase (TERT), and KIT protooncogene receptor tyrosine kinase (KIT), can be mutated in a way that causes benign nevi to become dormant for a long time before developing carcinogenesis. These mutations result in abnormal activation of the PI3K and MAPK pathways, which are physiologically connected to the proliferation and survival of cells (Figure 1) (6).
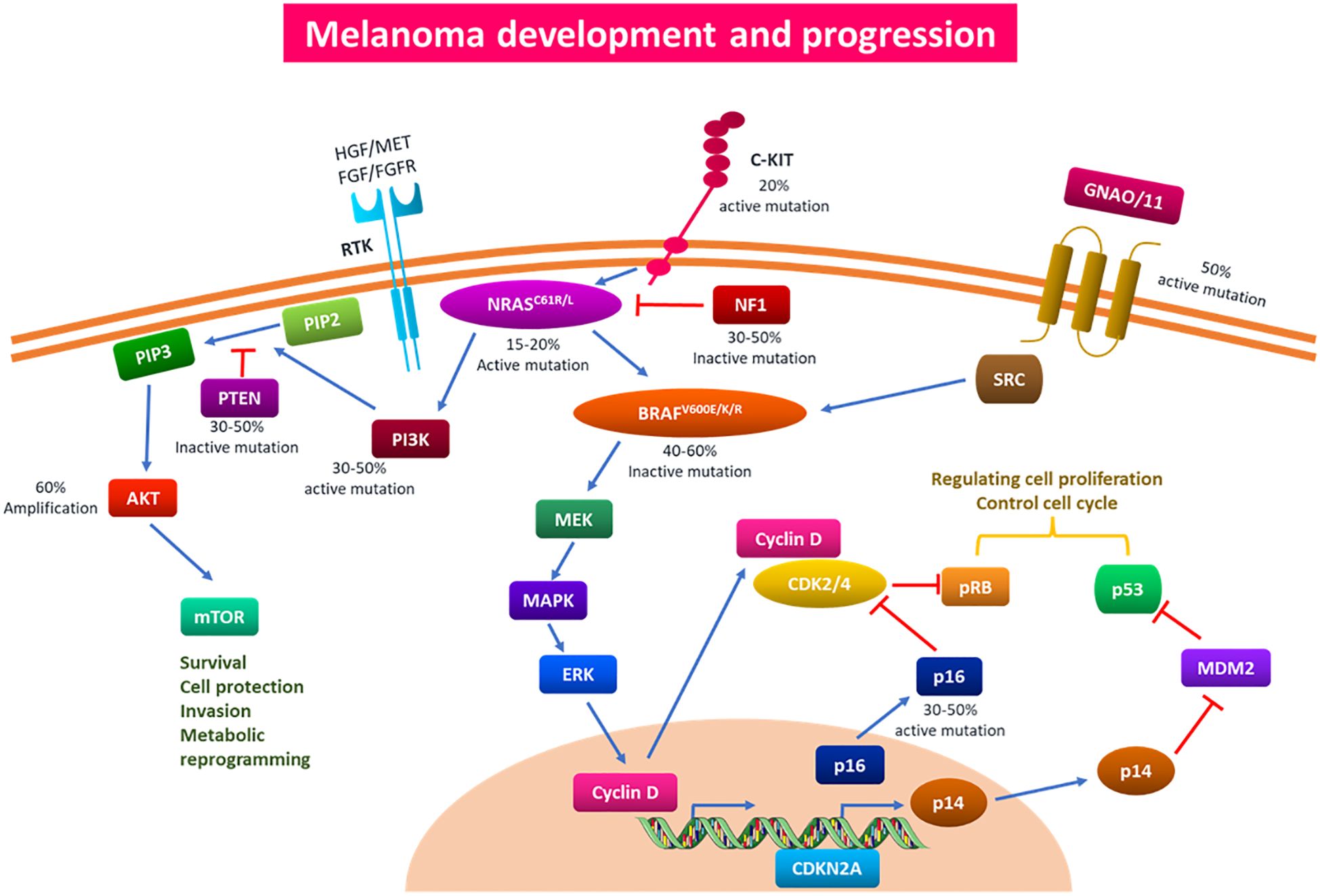
Figure 1. Signaling pathways and genetic mutations implicated in the onset and spread of melanoma. Multiple driver gene alterations are responsible for the genesis and progression of melanoma. Based on particular somatic mutations in various oncogenes, cutaneous melanoma has been classified into four primary subtypes: c-KIT4, GNAQ/11, BRAF, NRAS, NF1, and NF1. Melanoma develops and progresses as a result of these somatic mutations activating the PI3K/AKT and MAPK pathways. Mutations in BRAF can affect the MAPK pathway, which can therefore affect cell cycle regulation and proliferation. The PI3K/AKT and MAPK pathways, which control cell survival, proliferation, invasion, and metabolic programming, can be impacted by mutations in the NRAS gene. In addition to producing medication resistance to traditional therapy, overactivation of the aforementioned pathways can aid in the carcinogenesis, proliferation, invasion, and infiltration of melanoma cells. PI3K/AKT, phosphoinositide 3-kinase/protein kinase B; MAPK, mitogen-activated protein kinase.
The propensity of melanoma to evade treatment and expand to other organs poses ongoing issues in therapeutics, despite constant advancements in the field (7–9). Arnold et al. (10) predicted that by 2040, there will be a 50% increase in newly diagnosed cases of melanoma. This emphasizes how urgently new treatments are needed to buck this trend (10). Notably, the stage of melanoma at diagnosis largely dictates the treatment approach (Table 1). When melanoma is detected in its early stages, the prognosis is very good. Less than 10% of patients with metastases survived for five years, indicating a much higher risk of death. This implies that the primary cause of death linked to melanoma is metastatic illnesses (11).
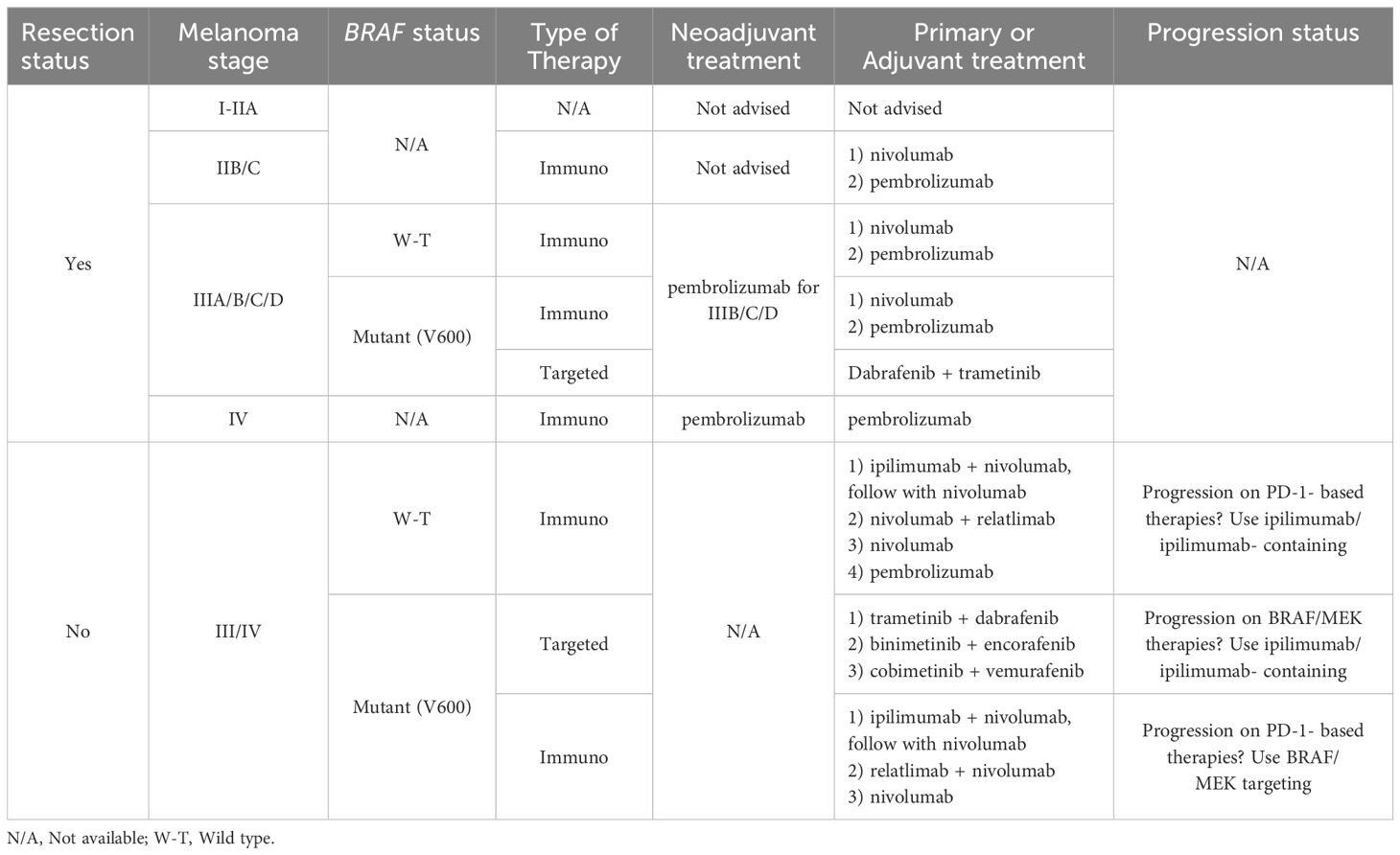
Table 1. A summary table of the 2023 American Society of Clinical Oncology’s guidelines for melanoma therapy standard of care.
Melanoma incidence increased by 320% between 1975 and 2018, with risk variables like nevi count, indoor tanning, UV exposure, and family history all playing a role (12). Although making up a modest portion of cases, melanoma is accountable for roughly 90% of skin cancer deaths. This disease affects both younger and older populations, but elderly people are more likely to experience a rise in incidence. Metastatic spread of melanoma, which initially affects lymph nodes and then most frequently the lungs, is the main source of death from the disease (13). Complete surgical excision is a successful treatment for early-stage melanoma (I–II), with a 99.4% 5-year survival rate (12). However, when melanoma advances, the prognosis gets substantially worse, with stage III and stage IV 5-year survival rates dropping to 68% and 29.8%, respectively (13). Melanoma’s global incidence has been rising, with some countries seeing an annual increase of about 3%. Concomitantly, the disease’s clinical impact is also increasing. According to projections, there would have been 7990 deaths and 97,620 new cases in the United States (US) alone in 2023. The International Agency for Research on Cancer has predicted that in 2020, there would have been 324,635 new instances of melanoma worldwide, along with 57,043 deaths from the illness (14). These figures demonstrate how crucial it is to choose melanoma treatments logically and by the best available data (15).
Understanding melanoma’s aggressiveness and high death rate requires an understanding of its phenotypic subtypes and gene signatures, which have been greatly enhanced by the progression of genomic technologies, particularly single-cell sequencing (16). The prognosis for metastatic melanoma is still bleak. Even though localized melanoma has a high survival rate, this underscores the significance of efficacious therapy (12). Advances in immunotherapy have completely transformed the way that medication therapy is thought of, creating opportunities for more individualized and efficient care (17, 18). Thus far, immunotherapy has resulted in the creation of immune checkpoint inhibitors (ICIs) (Table 2), TME modulators, and oncolytic viral therapy (19, 20). Immune checkpoints are crucial molecules in the cell regulation of the immune response. However, they can be used by tumor cells to subvert immune surveillance (Figure 2) (21). Nevertheless, the use of ICIs in particular has drastically increased the long-term survival of melanoma patients in clinical settings and radically changed how they are managed (22). Disappointingly, individuals receiving ICIs frequently only experience temporary advantages, and it can also have harmful side effects (22, 23).
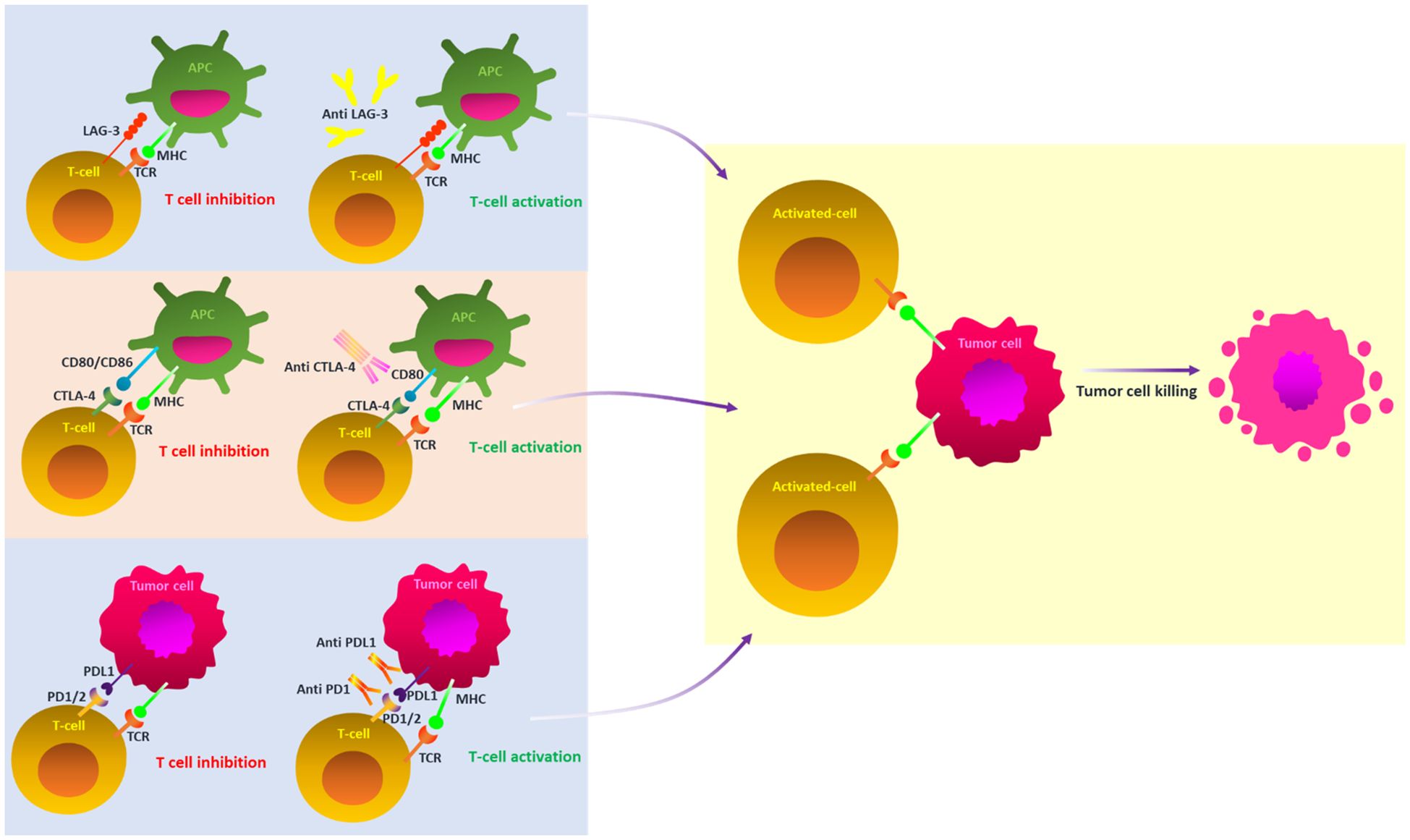
Figure 2. A schematic illustration of key ICI blockade mechanisms in melanoma. Under normal circumstances, the TCR and MHC signaling pathways provide T cell activation; however, these pathways are inhibited by the cooperative action of ICIs inside the tumor microenvironment. ICIs prevent the off signals from being sent by blocking the connections between checkpoints and their companion proteins. This allows the T cells to attack cancer cells. ICI, immune checkpoint inhibitors; PD-1, programmed cell death protein 1; PDL2, PD-2 ligand; PDL-1, PD-1 ligand; CTLA-4, T-lymphocyte antigen 4; TCR, T-cell receptor; MHC, major histocompatibility complex; LAG-3, Lymphocyte-activation gene 3.
The immune system is a complex network of cells, organs, and soluble substances that act as the body’s defense mechanism. One cancer treatment that utilizes this capability is immunotherapy, which develops plans to thwart the spread of cancer (24–26). Numerous molecular drugs that can “teach” the immune system to recognize and eradicate tumor cells have been developed as a result of research on immune control’s perspective on tumor evasion mechanisms (27, 28). In this perspective, because of the significant occurrence of lymphocytic infiltrates, metastatic melanoma is regarded as an ideal instance of immunogenic malignancies (17). Metastatic melanoma patients had an average life expectancy of roughly nine months before the introduction of immunotherapy in 2011. These days, 20% of melanoma patients survive for 10 years after being diagnosed. This is due to the discovery of novel treatment targets and the creation of novel immunotherapy medications (29). ICIs (30–32); adoptive cell therapy (ACT) (33); biological drugs, including interferons, stimulating factors, cytokines (34); and vaccines (34) are among the immunological treatments for melanoma.
ACT encourages the direct isolation of immune cells from patients for therapeutic purposes. These cells can be easily multiplied or genetically altered to improve their ability to combat cancer after being isolated. ACTs are always changing and encompass a variety of techniques: 1) TIL therapy; 2) engineered T cell receptor (TCR) therapy; and 3) chimeric antigen receptor (CAR) T cell therapy. The first cellular treatment for a solid malignancy, lifileucel, was approved by the US Food and Drug Administration (FDA) on February 15, 2024. The patient’s own TILs are used in this ACT immunotherapy. These TILs are isolated from a growing tumor that has been removed, grown to a large number in the lab using the T cell growth factor interleukin-2 (IL-2), and then reinfused into the same patient under specific conditions to target tumor cells. As they circulate within the cancer-bearing patient, these TILs can multiply thousands of times, making them a “living drug.” After a multicenter single-arm study in 73 patients (NCT02360579) demonstrated a cancer regression rate of 31.5% by established oncologic criteria, approval was given for adult patients with advanced melanoma that is unresponsive to other successful treatments (35, 36).
TIL therapy has shown promise in treating advanced melanoma in patients who previously received treatment from other therapeutic methods (BRAF/MEK inhibitors or ICI treatments) (37). As a result, this review explores the prognostic significance of TILs in melanoma. It also examines the processes by which TIL influences melanoma outcomes, clinical consequences, and existing challenges and limitations.
2 Tumor-infiltrating lymphocytes: an overview
It is believed that immune cells in the TME and cancer play a critical role in regulating the growth and spread of malignant tumors. As a result, TILs have been found in metastases of several cancer types as well as primary tumor tissue and lymph nodes that harbor tumors (38). TILs are a polyclonal population comprising CD3+ T-cells (CD8+ and CD4+ T-cells), FOXP3+ regulatory T-cells, and CD20+ B cells (39). Research has been conducted to look into TILs’ prognostic significance in melanoma (40). TIL treatment is a form of immunotherapy in which a biopsy or surgical procedure is used to separate TILs from the tumor location (Figure 3) (41). Interleukin-2 (IL-2) is used to cultivate these cells in vitro. By stimulating CD4+ T-cell proliferation and T-helper cell differentiation, IL-2 enhances the antitumor response (42). Additionally, it has been demonstrated that IL-2 can boost natural killer (NK) cells’ cytotoxic potential and CD8+ T-cells’ antitumor attribute (43). The patient receives another dose of the prepared vaccination (41). A TIL- based drug takes 5-7 weeks to create, and it needs specialized machinery and skilled labor (44).
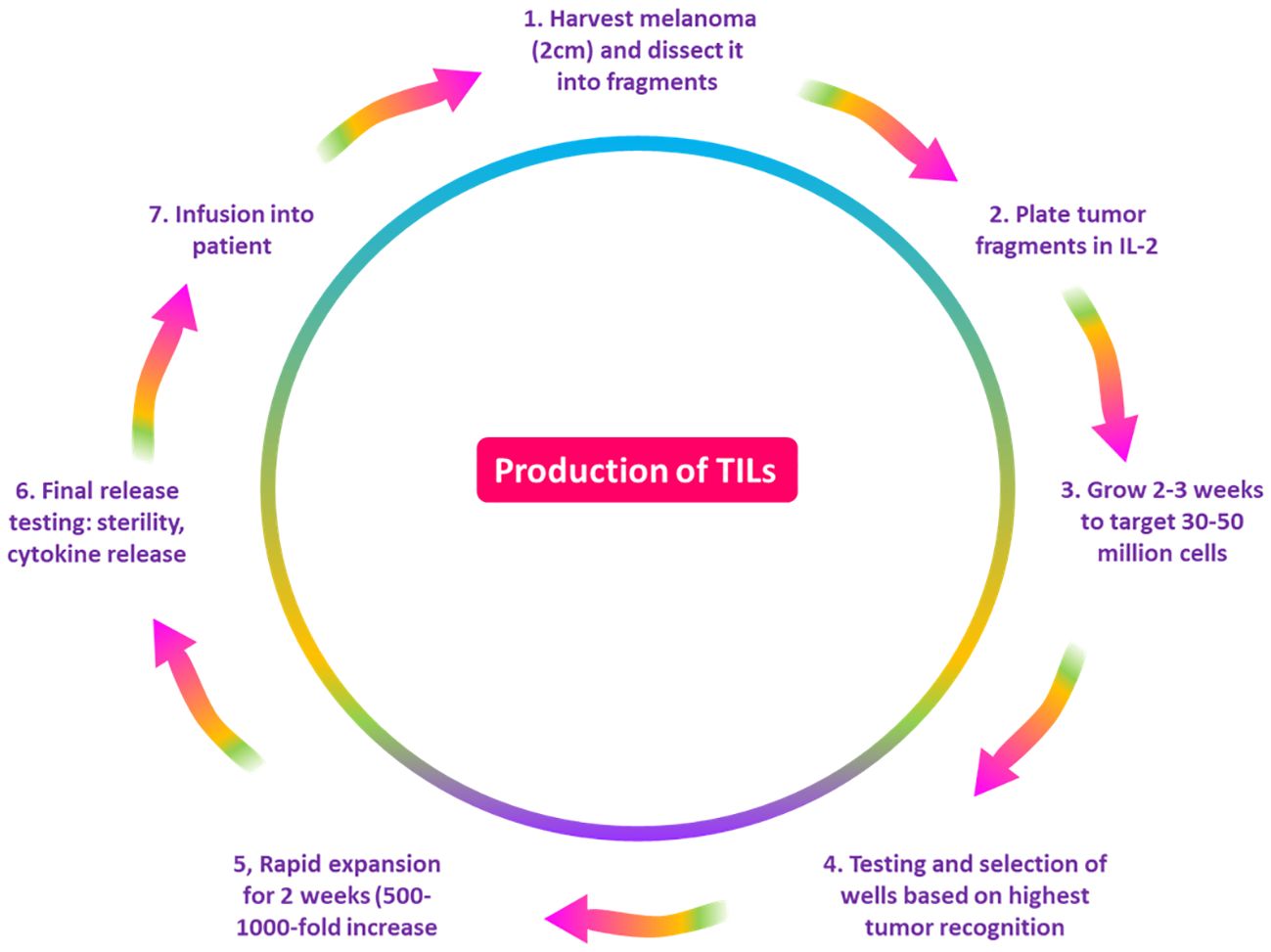
Figure 3. A diagram demonstrating the process of the production of TILs. The NCI/NIH Surgery Branch developed the first techniques for producing lymphocytes that penetrate tumors. First, the patient’s tumor is removed, cut into pieces, and then it is cultured with IL-2 for two to four weeks, or until the number of lymphocytes reaches 30 to 50 million. Following a test against tumor cells, lymphocytes are rapidly expanded for two weeks to reach a target of 50 billion cells, after which they are pumped into the patient. TILs, tumor-infiltrating lymphocytes; NCI, National Cancer Institute; NIH, National Institutes of Health; IL-2, Interleukin 2.
In 1988, Steven Rosenberg was the first to show through a clinical trial that a TIL-based approach might effectively cure melanoma (45). Twenty patients with metastatic melanoma in that research trial were given an infusion of a cultured solution containing TILs and IL-2. Tumor regression was thus seen in 2 out of 5 patients who had previously had therapeutic failure with IL-2. Furthermore, tumor regression was reported in 9 out of 15 people who had not previously received IL-2. Furthermore, regression of tumors was noted in the skin, bones, liver, lungs, subcutaneous adipose tissue, and liver. It was observed that concurrent delivery of IL-2 and previous cyclophosphamide or radiation therapy are major factors in TIL efficacy. The majority of TILs exhibited a CD3+ phenotype, however, patient-specific CD4+ and CD8+ cell counts varied (45). The efficacy of TIL therapy in treating metastatic melanoma patients has been demonstrated by clinical trials, especially in those who are not responding to standard treatments. About 20% of patients saw a full response, indicating the promise of TIL therapy as an individualized immunotherapy. About 50% of patients presented a partial response (37, 46). TIL treatment can extend the lives of people with metastatic melanoma. This is demonstrated by the fact that individuals treated with it had a median overall survival (OS) increase of 6 to 12 months when compared to those receiving standard therapies (15).
3 Prognostic value of TILs in melanoma
3.1 TIL assessment in hematoxylin and eosin
After Clark and colleagues initially described TIL in melanoma (47), several researchers found a correlation between lymphocyte infiltration and increased patient survival (Table 3) (59). Even though studies have revealed that immune cell subsets within human malignancies are extremely diverse, counting TIL using hematoxylin and eosin (HandE) offers crucial information on the TME in some cancer types (60, 61). Based on histology, the immune infiltrate’s location can be classified as intratumoral, stromal, or peritumoral (61, 62). Intratumoral immune cells are found right inside the tumor cells’ malignant “nest” (61). The stromal region is made up of different immune cells, connective tissue, and blood vessels. The invasive front refers to the tumor’s outside edge (62). Thus, the cells in the stroma, neighboring non-involved tissue, and the area surrounding the invasive front can all be referred to as peritumoral (61, 62). There is not yet a single, comprehensive method for determining the total amount of immunological infiltrates in solid tumor tissue stained with HandE. It is possible to evaluate and analyze intratumoral and peritumoral lymphocytes for association with different clinical indicators (61).
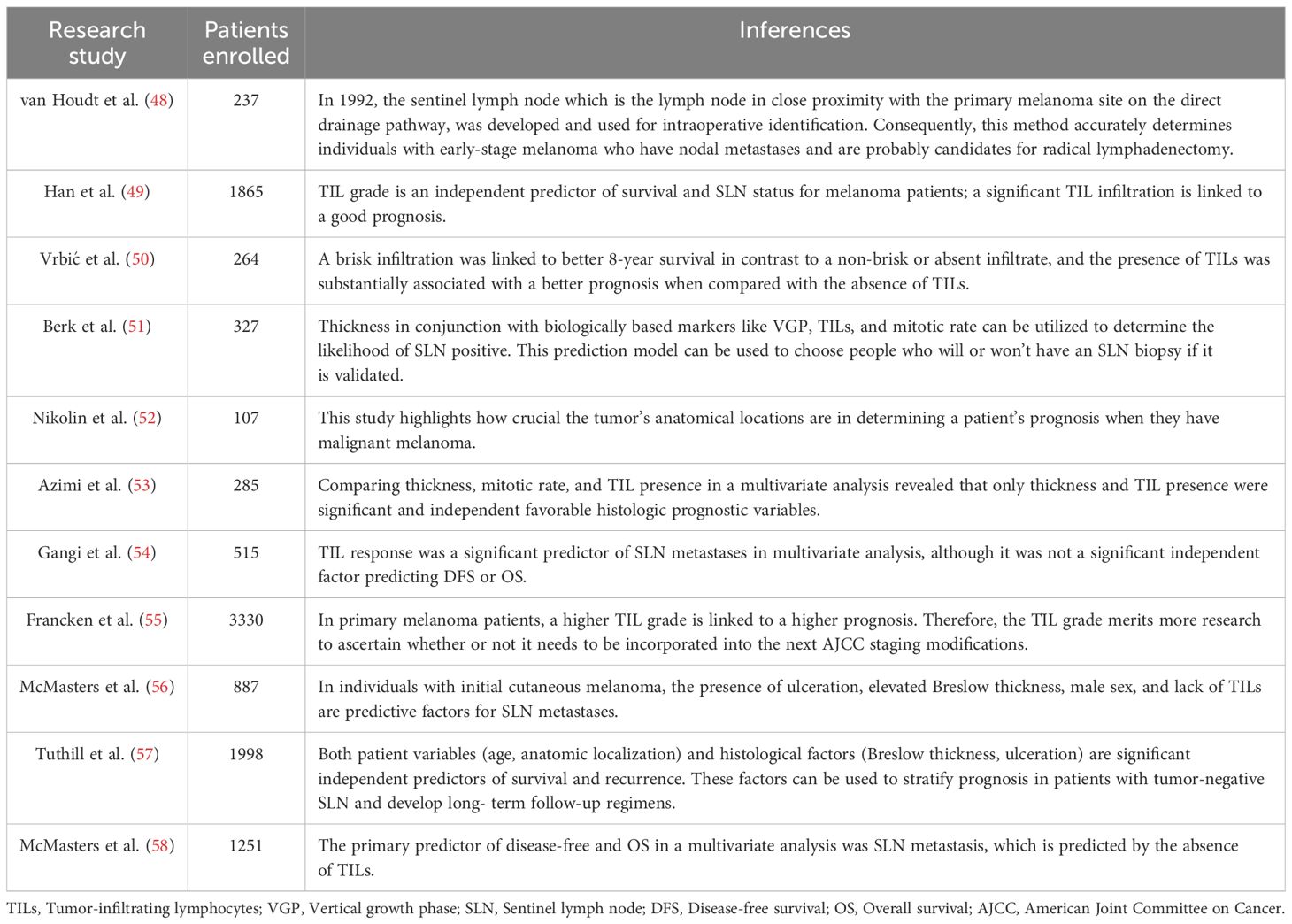
Table 3. Studies in the literature looking at the relationship between overall survival (OS) and TILs in primary melanomas.
It is important to remember, though, that digital full slide images are currently used for the great majority of pathological studies (63, 64). This enables dynamic zooming and panning, high-quality imaging, and the ability to use image analysis software to examine the images (63). Typically, TIL scoring in cutaneous melanoma is limited to spherical inflammatory cells in the intratumoral area, eliminating polymorphonuclear cells (65). Additionally, whereas primary melanoma tumors have been the subject of the majority of TIL investigations, several researchers have also investigated the prognostic significance of TIL examination in metastatic biopsies (66–68).
These days, the Melanoma Institute Australia (MIA) approach and the Clark method are the two main techniques for grading TIL in HandE-stained melanoma tissue (40, 53, 61). The Clark score method was first published in 1989 and defines three unique TIL patterns: absence, non-brisk, and brisk (Figure 4) (47, 63).
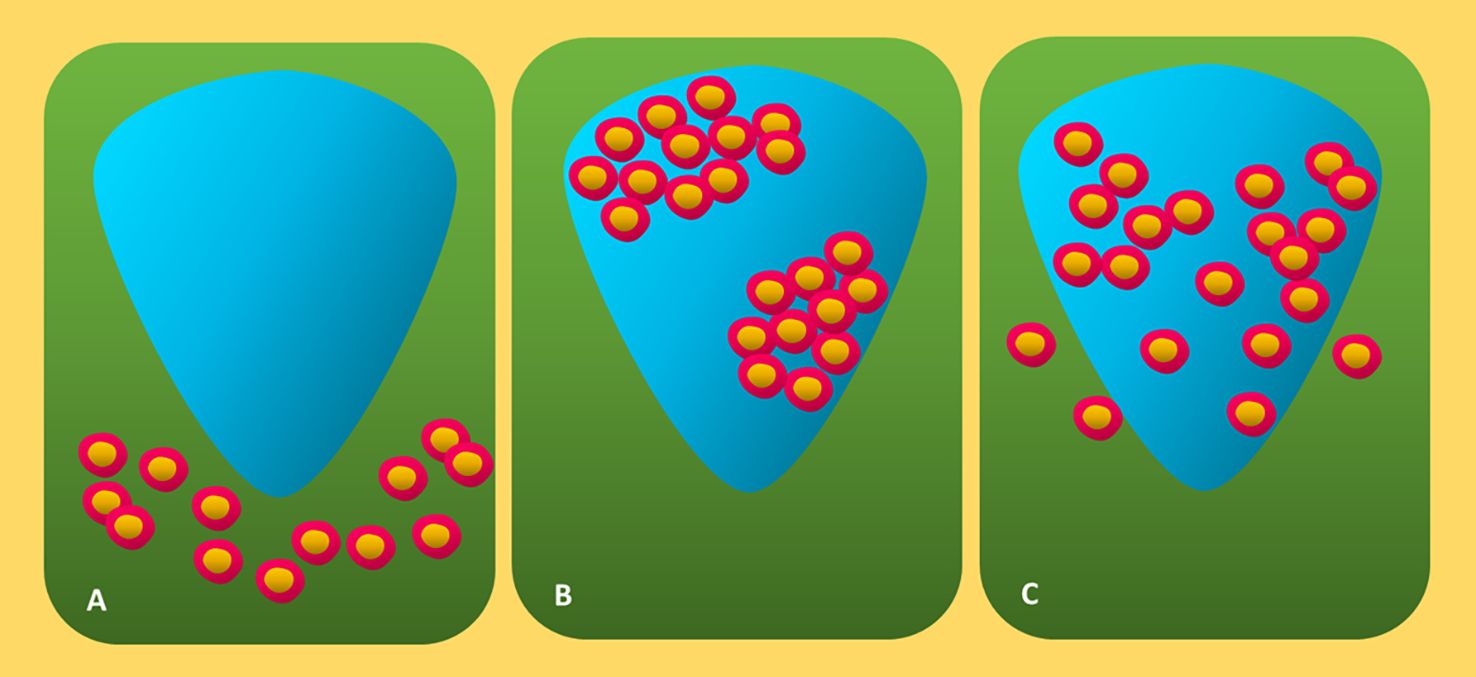
Figure 4. Diagrammatic representation of three forms of canonical TIL invasion in cutaneous melanoma. (A) Absent: Neither lymphocyte infiltration nor lymphocyte presence within the tumor. (B) Non-brisk: One or more dispersed lymphocyte foci. (C) Brisk: Diffuse lymphocyte infiltration near the tumor base or during the tumorigenic vertical development phase. TIL, tumor-infiltrating lymphocytes.
Absent denotes the unavailability of TILs or their inability to invade the tumor (47, 65). Nonbrisk indicates one or more dispersed lymphocyte foci (47, 59). Diffuse lymphocyte infiltration along the tumor’s base or during the tumorigenic vertical growth phase (VGP) is referred to as brisk (47, 60, 69). The brisk TIL patterns scoring was further separated by Clemente and colleagues into two categories: diffuse (infiltrate the invasive region of the tumor entirely) and peripheral (along the tumor base) (60, 70). Lymphocytes in fibrosis-affected areas and perivascular lymphocytes are often excluded from the scoring (60). Due to its great interobserver agreement, reproducibility, and ease of application, the Clark system is still widely used (60, 71). Based on the distribution (diffuse, focal, or multifocal throughout the entire tumor) and density (marked, mild, or moderate) of TIL in the dermis, the MIA grading system is used (53, 60). The definition of the MIA ordinal score (0–3) is as follows: TIL absent in grades 0; mild multifocal or moderate/mild focal infiltrate in grades 1 and 2; marked multifocal or moderate, mild diffuse, or marked focal TIL pattern in grades 3 and 4 (53).
It has been shown by the MIA and Clark scoring systems that higher TIL levels are linked to better prognosis. Clark and colleagues examined the 8-year survival of more than 200 individuals who had primary cutaneous melanoma with various histologic subtypes. Eight-year survival rates for patients with absent TIL were 59, for those with non-brisk TIL patterns it was 75%, and for those with brisk TIL patterns, it was 88% (47). The association between the absence of TIL and a greater number of sentinel lymph node (SLN) metastases is further proof of the biological significance of TIL scoring (72), as defined by Clark and colleagues (47). In melanoma, SLN status continues to be the most significant independent prognostic predictor (73). Using the MIA scoring system, Azimi et al. (53) demonstrated that the TIL grade was independently linked with disease-specific survival (DSS) and inversely associated with SLN-positive in primary cutaneous melanoma (53). There is enough data to show that the presence of TIL is associated with a better prognosis for patients because of the increased host response to the tumor. A meta-analysis of 41 studies on TIL in melanoma revealed that the mere existence of TIL was highly related with enhanced OS. Most of the primary cutaneous melanoma research that was included in this meta-analysis was carried out. The authors provided evidence that brisk TILs were linked to better OS, DSS, prognosis, and recurrence-free survival (RFS) (40). Taken together, these findings show that lymphocytes in the tumor are indicative of the host immune system’s reaction to the malignancy and are, thus, typically linked to favorable clinical outcomes. The International Immuno-Oncology Biomarker Working Group (IOBWG) has suggested a more uniform method for TIL evaluation due to the usefulness of the HandE assessment of TIL (74).
To ascertain this approach’s efficacy for prognosis, melanoma, and other solid tumors must be validated first. Although TIL scoring has shown to be an effective predictive method for melanoma, the immune cells that infiltrate a tumor exhibit a varied range of phenotypes and functions (75). Three main kinds of immune infiltration were determined through histological examination, even though the TME for the majority of malignancies is extremely variable. Tumors with non-T cell-inflamed (cool) and T cell-inflamed (hot) TME can be broadly categorized (76). Elevated IFNγ signaling, PD-L1 expression, a high mutational burden, and a high density of CD8+ TIL are all present in T cell-inflamed malignancies, according to profiling of these subtypes (76, 77). Immunosuppressive myeloid cells or stroma prevent T cells from entering the tumor bulk, resulting in a peripheral buildup of T cells in immune-excluded malignancies (77). On the other hand, cold tumors could be an immune-ignored phenotype with minimal or nonexistent T cell infiltration, significant tumor cell proliferation, no expression of PD-L1, and a low burden of mutations (76, 77). The general categories that are presented here are obtained from tumor tissue histological investigations (77). It is necessary to ascertain the functions of stromal cells, tumor-infiltrating immune cells, and mutational burden in determining the TME phenotype (77, 78). Therefore, one of the most important methods for both pre-clinical and clinical cancer research is the use of IHC for the identification and counting of the primary lymphocyte subsets in the tumor and the assessment of their association to treatment response or patient survival (78, 79).
3.2 Prognostic implications of different TIL subtypes
3.2.1 CD8+ TIL
CD8+ T cells play a crucial role in the adaptive immune response against malignancy (80–82). Since activated CD8+ T cells may directly identify and eliminate cancerous and contaminated cells, they are also called cytotoxic T lymphocytes (CTLs) (80, 81). CTLs are the main immune cells that have the ability to regulate tumor growth and mediate responses to cancer immunotherapies. This is accurate despite the fact that certain immune cells, such as macrophages and NK cells, have the ability to cause tumors (81–83). The primary anticancer function of CTL is the production of cytokines such as IFNγ and tumor necrosis factor (TNF), in addition to the exocytosis of cytotoxic granules that include granzymes and perforin (81, 82). CD8+ T cell priming and recruitment in the TME were reported to be dependent on a subgroup of conventional dendritic cells (cDC), known as cDC1 (84). According to the conceptual framework of cancer immunoediting, the tumor can elude the immune onslaught and foster an immunosuppressive TME following a time of early T cell control (80, 85). Conversely, long-term exposure to antigens, as seen in the setting of cancer and chronic viremia, induces T-cell exhaustion. This condition is characterized by a loss of effector capabilities, unique transcriptional patterns, and a persistent expression of inhibitory surface receptors (like PD-1) (86). Consequently, the translation of histological investigations on CD8+ TIL in tumor tissues is complicated by their varied morphologies and functional profiles in the TME (61, 66).
Early analysis indicated that a group of 47 individuals with primary cutaneous melanoma tumors had higher CD8+ TIL levels, which were related to a higher chance of survival. Upon utilizing CD8+ T cell density to stratify the patients, it was observed that the 5-year OS for the low-, moderate-, and high-density cohorts were 25, 44, and 78%, respectively (87). However, in 2011, a larger cohort of more than 180 primary cutaneous melanoma samples revealed no association between patient survival and CD8+ TIL (88). These investigations thus highlight the difficulty in utilizing immunohistochemistry to assess the CD8+ TIL prognostic significance. Numerous studies have looked at the TIL characteristics of metastatic lesions in addition to original tumors. These results show a significant correlation between survival and elevated CD8+ T cell density in Stage III and IV metastatic melanoma tumors (67, 68). As was previously mentioned, CD8+ TIL express a broad range of surface markers and release several functional chemicals (82). Therefore, while investigating the prognostic importance of CD8+ TIL, some research has looked at the utilization of labeling effector molecules or activation markers in addition to identifying CD8. It was demonstrated that TIL positive for the CD8+ T cell effector molecule called Granzyme B (GZMB) was linked to longer O and progression-free survival (PFS) in the Stage II primary melanoma tumors cohort. Additionally, GZMBC TIL were proven to be positive for CD8 by dual IF labeling (48).
Research on the functional condition of T cells in the TME has revealed many biologically significant surface indicators that may help evaluate the prognostic significance of CD8+ TIL in melanoma. Better clinical results in cutaneous melanoma are linked to the presence of the chemokine receptors CCR9 and CXCR3, as well as the c-type lectin NKG2D, on CD8+ TIL. This was corroborated by a flow cytometric analysis of intratumoral CD8+ T cells in metastatic lymph nodes (mLN) (89). The difference between tumor-specific lymphocytes (i.e., those that detect tumor antigens) and bystander TIL is crucial in the TME. In lung and colon cancers, surface-expressed ectonucleotidase CD39 has been shown to be a useful marker for distinguishing tumor-specific CD8+ TIL. This was found by mass cytometry and multiplexed tetramer-based methodology (90). The researchers discovered that T cell immunoreceptors with Ig and ITIM domains (TIGIT), which have been shown to differentiate tumor-specific “exhausted” TIL, and inhibitory receptors like PD-1 are present in bystander TIL (90, 91). The CD8+ TIL diversity in the TME has been shown by sophisticated immunophenotyping technologies. Labeling other markers on CD8+ TIL, such as CD39, TIGIT, or NKG2D, may or may not help predict disease in research and clinical settings, but this is still up for debate. However, when assessing the prognosis of a disease or the potential efficacy of immunotherapy, examination of CD8+ T cells in the TME continues to be one of the most important readouts for intratumoral immune activity (61, 92).
3.2.2 CD4+ TIL
CD4+ T helper (TH) cells are extremely varied and have a phenotype and function similar to CD8+ T cells (93). Moreover, through recently studied pathways, CD4+ T cells are critical for cancer immunity (93, 94). CD4+ T cells have the ability to kill tumor cells directly and cytolytically, as well as create cytokines like IFNγ that strengthen the immune system’s ability to fight cancer (80, 93). Moreover, CD4+ T cells in secondary lymphoid organs can regulate CD8+ CTL and B cell responses. Studies on murine models have demonstrated that CD4+ T cells can boost CD8+ T response efficacy (94). The differentiation of CD4+ T cells into discrete T helper lineages identified by unique transcription factors and cytokine production has also been demonstrated (93, 95). The lineages T follicular helper (TFH), TH1, TH2, TH17, and CD4+FOXP3+ Treg are the most studied of these. It is widely acknowledged that Th1 cells exploit their high IFNγ production to support efficient immune responses against tumors. Consequently, it recruits NK cells and M1 macrophages that have been classically activated in addition to modulating CD8+ TLR activity. Nevertheless, the complex roles that each subgroup plays in various tumor types are still not well understood and need more research (93). Alternatively, Tregs are thought to promote tumorigenesis because of their ability to reduce immunological responses and block effector T cells (96).
As previously indicated, the majority of research on TIL’s prognostic significance in melanoma has used TIL pattern scoring in HandE tissues. Thus, a relatively handful of investigations have looked at TIL subgroups in situ using IHC (61, 66, 79). Using Stage II primary cutaneous melanomas, Van Houdt et al. (48) demonstrated that CD8+ and CD4+ TIL were only associated with improved PFS and not OS, but GZMBC TIL was associated with both prolonged OS and PFS (48). Nevertheless, there are not any solid studies showing the prognostic value of CD4+ TIL evaluation utilizing histopathology in the setting of metastatic melanoma (61, 66). Jacquelot et al. (89) discovered an inverse relationship between the frequencies of CD8+ T cells and the fraction of naïve CD45RA+CD4+ T cells using multi-parameter flow cytometric profiling. This was found in the mLN of patients with stage III cutaneous melanoma. Furthermore, it was observed that patients whose tumors had notably higher percentages of naive CD45RA+CD4+ T cells had significantly shorter PFS. Finally, it was found that CD4+ T cells in metastatic tumors additionally expressed surface markers, PD-1 and CD69. Nevertheless, it is still unclear if evaluating these markers in situ IF or by immunohistochemistry has any predictive value for melanoma (89).
Very little research has used IHC or IF to examine the predictive significance of CD4+ TIL enumeration in melanoma (61, 66). Higher concentrations of CD8+ and CD3+ TIL were successfully linked with OS, but not CD4+ TIL. This was demonstrated by studies that used TMAs created from several anatomic regions of metastatic melanoma samples and IHC to identify the primary TIL subgroups (67). However, in a study that looked only at melanoma metastases inside the SLN and visually counted intratumoral cells, greater CD4+ TIL counts were substantially linked with improved OS and RFS (68). The investigation of distinct TIL subsets within the metastatic SLN can yield valuable insights into the molecular and prognostic aspects of the functions these cells perform in cancer immunity. This is because primary cutaneous melanoma is commonly staged via SLN biopsy (97). The aforementioned data, however, make it more difficult to understand how CD4+ TIL functions in melanoma. Firstly, standardized comparisons across numerous findings are not possible due to the limited number of research looking at melanoma TIL subsets and the variation in methods being utilized to determine and count labeled cells (61, 66). Second, identifying merely the CD4 surface antigen is insufficient to describe CD4+ T helper cell diversity. Although TH1 CD4+ TIL is thought to boost cancer immunity, the functions of TH17 and TH2 are extensively complex. Furthermore, it is unclear how they play a part in the formation and spread of tumors (93, 98). The understanding of how T helper subsets impact tumor formation has primarily come from in vivo mouse models, whereby B16 melanomas have been demonstrated to be eradicated by CD4+ TH1 and TH2 cells (98). However, because T-helper subpopulations are generally defined by the differential expression of important cytokines rather than the expression of distinctive surface markers, investigating them in the context of immunopathology is difficult (93). This has led to several investigations using gene expression profiling to evaluate the presence of TH2 or TH1 hallmark genes in human melanoma samples. According to a report, primary melanoma tumors that spontaneously regress (a clinically confirmed event suggesting host anti-tumor immune response activation) express significantly more TH1-linked genes, such as IL-2 and TNFβ, than tumors that do not. Regressing primary tumors also showed increased gene expression of IFNγ, which is the main TH1 effector cytokine. However, these levels did not become statistically significant (99).
In another investigation, VEGFA production was observed to be greater in CD4+ T cells obtained from the SLN of 13 patients with cutaneous melanoma who had TH2-skewed gene profiles. In this study, both positive and negative SLN were included (100). While the exact mechanism of T cell differentiation into distinct effector subpopulations remains unknown, it is known that specific transcription factors regulate the differentiation of CD4+ T cells into distinct T helper lineages. These include TH1 (T-bet), TH2 (GATA-3), Treg (FOXP3), and TH17 (RORgt), As a result, T-bet+ cell IHC has occasionally been used as a readout for TH1 cells in tumor tissue. Increased levels of T-bet+ cells have been correlated with better patient outcomes in both ovarian and colorectal primary cancers (101, 102). Nevertheless, no comparable research on melanoma has been published in the literature (40, 61, 66). Moreover, studies showing Treg to be T-bet expressing raise doubts regarding the biological validity of using T-bet in dual or single-marker IHC to identify TH1 cells. Levine et al. (103) demonstrated in a seminal study that Treg cells express T-bet consistently in murine models, and that the reduction of T-bet+ Treg in mice led to strong TH1 autoimmune reactions (103). As a result, to evaluate CD4+ TIL in cancer tissues and determine the prognostic and biological significance of each subset, it will be necessary to assess several distinct phenotypic markers in addition to CD4+. This is because earlier studies have cast doubt on previously accepted assertions regarding T helper cell differentiation. This is particularly true given the variety of Treg populations found in cancers and the varying findings from research using FOXP3+ to look at Treg that have penetrated tumors (79, 104).
3.2.3 Natural killer cells
The innate immune system’s effector cells, known as NK cells, are crucial for eliminating tumor and virus-infected cells (105). Additionally, through their interactions with DCs, they take role in the control of adaptive immune responses (106). A balance of signaling pathways initiated by activating (NKG2D, DNAM-1, NKp30, NKp44, NKp46, etc.) and inhibitory receptors (CD94/NKG2A, members of the killer cell immunoglobulin-like receptor family, etc.) receptors determines the activation state of NK cells. Since they are typically scarce in solid tumors, NK cells are thought to primarily contribute to systemic antitumor defense by preventing the growth of hematogenous metastases. Additionally, reduced functional activity and low activating receptor expression are common characteristics of tumor-infiltrating NK cells (107, 108). The impact of immune-suppressive substances generated by tumor cells or nearby stromal cells, which have been reported in a number of tumor forms, including melanoma, may be the source of this (109, 110). Conversely, in certain tumor forms, a higher number of NK cells was linked to a better prognosis (107, 111). However, it should be mentioned that the majority of research used antibodies against the CD56 or CD57 markers, which are expressed by cell types other than NK cells. In contrast, the use of the NKp46 marker, which is thought to be more NK-specific, has only begun to gain traction afterwards (108, 112). A smaller proportion of CD56bright NK cells in melanoma patients is associated with a shorter overall and PFS, even though there is no overall difference in the number of circulating NK cells between healthy donors and melanoma patients (113). In stage IV melanoma, the same relationship was seen for NKp46 on circulating NK cells, where more expression was linked to prolonged survival (114). Nevertheless, according to de Coaña et al. (115), there was no relationship between NKp46 expression and OS; instead, low CD69 expression might be a predictor of improved OS (115). Using a different approach, analyzing RNA-seq data from bulk tumor samples, Cursons and colleagues used a NK cell signature to predict NK cell infiltration into melanoma and showed that this correlated with a better survival (116).
3.2.4 B lymphocytes
B lymphocytes are often seen in relatively small quantities in solid tumors, with a few exceptions. It is unclear how antibody-dependent immunity contributes to the immune response against tumors, and it is still up for debate how the biological activity of tumors is influenced by systemic B-cell response or in situ B-cell accumulation. B lymphocytes have been demonstrated to promote tumor growth or progression in several experimental tumor models (117, 118). However, other research produced the opposite findings (119). According to Nelson (120), the variation in B lymphocytes’ systemic pro- or antitumor effects could be linked to the variety of their functional activities. In addition to secreting various immune suppressive substances and supporting tumor growth through antibodies or immune complexes, B lymphocytes may also serve as efficient antigen-presenting cells that stimulate the anticancer T-cell response (120). T cells make up the majority of melanoma infiltrates, just like in other tumor types, with B-cell frequencies estimated to be between 15% and 20% of all infiltrating lymphocytes (121, 122). The vast majority of B lymphocytes expressing the CD20 marker in studies on 106 primary melanomas were found to be peritumoral, primarily distributed in the stroma surrounding tumor deposits. Additionally, B-cells arranged in dense, follicle-like aggregates were seen in roughly 25% of the samples (123, 124). According to different cancer types and melanoma metastases, these B-cell clusters formed ectopic lymphoid structures with T lymphocytes (125–128). Some of the follicle-like structures showed a network of CD21+ follicular DCs, and in most cases, MECA-79+ HEV-like venules were also seen adjacent to these (124). According to Ladányi et al. (123), there was a correlation between the density of activated (CD25+ or OX40+) T cells and B lymphocytes in the melanoma samples. Additionally, peritumoral densities of B-cells and activated T lymphocytes showed a very bad prognosis in the case of low amounts of both cell types, and intensive B-cell infiltration offered a considerable survival advantage (123). Although there was a trend toward a larger incidence of B-cell aggregates in thicker tumors, their appearance did not correlate with the disease’s outcome (124). The quantity of invading B lymphocytes with a predictive impact was not discovered in a previous investigation with less cases (121).
4 Clinical implications and applications
4.1 TILs as an immunotherapy biomarker
For melanoma, breast cancer, endometrial cancer, colorectal cancer, and non-small cell lung cancer (NSCLC), higher baseline TIL density is linked to better outcomes (ORR in metastatic and pathological complete responses (pCR) in early illness). These solid tumors were treated with ICIs (129–143). Moreover, studies assessing histological samples obtained during treatment demonstrated that changes in TIL density throughout treatment were linked to better results, even in cases where no correlation with baseline TILs was seen (132, 144). The reaction to ICI is also influenced by spatial dispersion in addition to TIL density and change in the TME. Due to its demonstrated ability to offer extra information on early alterations following ICI administration in and around the tumor, the ratio between the invasive margin (IM) and the tumor center (CT) may be of particular relevance. It may also serve as an early predictive biomarker for the effects of treatment (133, 134, 139, 145–147). Findings like these show that the tumor-host environment is a dynamic system that constantly adapts to changes. Furthermore, in patients undergoing ICI treatment, the dynamics in TILs, particularly during and after treatment, may be predictive and prognostic (38).
The impact of acidity and hypoxia on TIL effector proliferation and function further demonstrates the critical role of TME in TIL dynamics. According to certain theories, an acidic environment inhibits IL-2’s stimulatory function, stopping lymphocyte proliferation (148–150). Moreover, CD8+ T cells’ cytolytic activity and cytokine release are hampered by acidosis in the TME (149, 151). Hypoxia, which is frequently brought on by disorganized and inadequate tumor microcirculation (152), impairs the ability of CD8+ and CD4+ T lymphocytes to perform effector tasks and promotes the growth and migration of immune-suppressive cells. Moreover, Zandberg and colleagues reported that the impact of anti-PD-1 therapy is weakened by elevated hypoxia inn HNSCC (153). TIL subsets (e.g., CD8+, Tregs, CD4+, and T-memory), exhaustion markers (e.g., LAG-3, PD-1, and TIM-3), and activation markers (e.g., granzyme B) as well as their link with clinical outcome post ICI treatment were investigated. This is in addition to the overall predictive and productive significance of TILs (140, 141). Although not conclusive as a prognostic biomarker, these studies demonstrate the relationship between TILs and TME after ICI treatment. Additionally, the predictive significance of a TIL varies according to the ICI employed (141). While TILs in tumors may predict ICI treatment, many studies lack an ICI-free arm for reference, making it difficult to draw definitive conclusions. High TIL infiltration and exhaustion/activation indicators are not always associated with a positive or negative response. In ovarian cancer, immune treatment trials have shown minimal response to ICI, even in tumors with significant TIL concentrations (154, 155).
5 Current challenges and limitations of TIL-based therapies
TIL-based therapy is not yet the preferred treatment for metastatic melanoma patients, since it has been recently approved, and only a few patients have been treated. ICI continues to be the main therapeutic option for metastatic melanoma patients as detailed in Table 2. Treatment-associated adverse events, treatment resistance, and TIL synthesis requirements are some of these issues. First, only well-funded medical facilities with the capacity to accommodate the required technologies to manage the TIL workflow and any treatment-emergent adverse events (TEAs) that can arise during patient hospitalization are largely able to produce TILs due to their labor-intensive and complex nature. Expanding the production of TIL products centrally is essential because it may enable the use of TIL-based ACT widely and affordably. One example of this is Iovance Biotherapeutics, which developed a central manufacturing facility to produce Lifileucel, an autologous TIL product that is cryopreserved (37, 156). The FDA’s recent approval of lifileucel (Amtagvi) for advanced melanoma seems encouraging (157).
Second, to get reinfusion, the patient must also follow a pre-conditioning program, the majority of treatment-related toxicities being recorded as side effects. These toxicities fall into the category of uncommon autoimmune-related toxicities, which are usually caused by tumor-linked antigens on healthy cells expressing non-specifically. The reintroduction of lymphocytes or cytokine-related toxicities, which are caused by the high doses of IL-2 that are frequently administered with TIL therapy to enhance the lymphocytes’ anticancer activity, can target these antigens (44, 158). It has been shown that this preparative lymphodepleting treatment, which consists of fludarabine and cyclophosphamide, increases the efficiency of TIL-based ACT. Nevertheless, cellular processes behind this effect are still unclear, and there is a significant trade-off in terms of severe toxicities (159).
Lastly, resistance to TIL use is possible, as it is in many situations. There is a high prevalence of both innate and acquired resistance. Innate resistance is defined as the patient’s observed lack of reaction after the first therapeutic administration, while acquired resistance is the developed resistance that appears following a patient’s prior positive response (156). There are three primary distinctions in the mechanisms resulting in different resistance forms: (1) TME-driven T-cell depletion and/or malfunction; (2) immune-suppressive cells interfering with TME-driven T-cell recognition; and (3) limitations on T-cell migration to the tumor (156, 159). Even while our understanding of the mechanics driving these resistance occurrences is growing, more has to be done to improve TIL curation. Current research has shown promise in combining TIL therapy with other treatments. One such study is the randomized prospective phase II trial (NCT02621021), which is being carried out to find out if patients with metastatic melanoma may experience higher response rates when pembrolizumab is added to TIL/IL-2 therapy (160).
6 Future directions and research priorities
6.1 Advanced techniques for TIL characterization
An analysis of the TIL profiling literature reveals that determining TIL characteristics and density provides a valuable mechanistic understanding of tumor immunology (60, 61). Furthermore, it was reported that TIL profiling provides predictive information about a successful response to ICI in addition to prognostic value for patient outcomes (61, 161, 162). Yet even when it comes to well-researched tumor forms like melanoma, the literature also shows that there is disagreement on how to identify, count, and score TIL subtypes (61, 66). Furthermore, highly multiplexed techniques are needed to find novel spatial correlations and phenotypic features. Therefore, two important facets of advanced characterization techniques in cancer and their application to cutaneous melanoma are discussed below. “Immunoscore” is another scoring system for immune cells that infiltrate tumors is initially explored. This algorithm has demonstrated exceptional predictive potential and reliability in cases of colorectal cancer (163, 164). Secondly, in situ, immunophenotyping techniques that show a lot of promise for revealing the TME’s complexity, especially its immunological background are explored.
6.1.1 Immunoscore
Currently, the IHC-based identification of many TIL-specific markers is one of the main methods for identifying the lymphocyte infiltrates in the TME (including FOXP3, CD3, and CD8) on successive tumor tissue slices (61, 79). Therefore, measuring lymphocyte densities as ratios like CD4/CD8 or CD8/FOXP3 was studied and has demonstrated prognostic value in other types of tumors. However, it still needs to be validated and thoroughly examined (165, 166). Nevertheless, a brand-new intratumoral lymphocyte scoring system called the “Immunoscore” has emerged as an effective predictive tool in colorectal cancer. According to digitally quantified densities of IHC-labeled CD8+ and CD3+ T cells in the CT and at the IM, immunoscore is a scale that ranges from I0-I4 (164, 167). Using specialist image analysis software (Immunoscore® Analyzer, HalioDx, France), an operator first recognizes specific locations (tumor, necrosis, healthy tissue, etc.), and then confirms the CD8 and CD3 stains. The software automatically determines an IM that extends 360 mm into the healthy tissue and 360 mm into the malignancy. Immunoscore outperformed the TNM staging system used by the AJCC/IUCC (American Joint Committee on Cancer, International Union for Cancer Control) in stage I, II, and III colorectal cancer. This relates to DFS, OS, and DSS prognostic variables. The primary tumor (T), involvement of the LN (N), and metastases (M) are examined by the TNM staging system (167). Tissue biopsies from more than 2,500 patients with colorectal cancer were examined by Immunoscore in a worldwide multi-center study spanning more than 13 nations. The results showed that the test had excellent repeatability and consistency among various sites. In contrast to patients with a lower Immunoscore, those with an elevated Immunoscore had a considerably lower chance of experiencing a disease recurrence after five years (163).
Attempts were made to apply Immunoscore to other tumor types. These include melanoma, non-small cell lung cancer (NSCLC), and breast cancer, due to its effectiveness in colon cancer. As a result, efforts to clinically validate Immunoscore as a prognostic and predictive marker (for ICI) in various other tumor types are still ongoing. TIL markers including FOXP3 and CD20 have also been added to the Immunoscore evaluation in melanoma (168). Overall, the potential for prognosticating clinical outcomes or ICI predictive efficacy of incorporating new immune markers into a more thorough Immunoscore may be greatly increased, given that Immunoscore has not yet been well investigated for melanoma and several other tumor types (39).
6.1.2 Advanced TIL characterization
When novel imaging and molecular tools become available, the capacity to examine many signals simultaneously offers enormous promise for the identification of actionable and clinically significant immune markers in cancer. Single-molecule fluorescent in situ hybridization (FISH) has made it possible to evaluate numerous RNA molecules at a single-cell resolution, according to studies. A recent report also showed how to multiplex the profiling of up to 40 proteins at once using an iterative immunofluorescence (4i) based method (169, 170). These technologies are still in the early stages of research; therefore, it is unknown how well they will work for in situ tumor tissue immunophenotyping. Many new image analysis software and detection techniques, such as nucleotide tagging, cyclic immunofluorescence, or metal ion tagging, have been recently developed. This is for the simultaneous detection of numerous protein markers (171). The GeoMx™ digital spatial profiling (DSP) method, which uses antibody probes or RNA attached to photocleavable oligonucleotides, was also recently disclosed by NanoString Technologies. To see tumor (like PanCK) and immunological (like CD45) areas in fluorescent markers-labeled tissue, UV light is channeled toward specific areas of interest using digital micromirror devices. Nevertheless, although this method permits the simultaneous and spatially resolved detection of several protein markers or RNA in FFPE tissue, it is not capable of multiplex viewing of multiple markers on individual cells (172).
7 Conclusion
The various functions of TILs in the dynamics of malignant melanoma progression and their importance for the prognosis of melanoma have been discussed in this review. The genetic alterations and signaling pathways linked to the development and metastasis of melanoma were also discussed. Additionally, the effect of melanoma immunotherapy on TIL biology has been examined. Prognostic implications of different TIL subtypes including CD8+, CD4+, NK cells, and B lymphocytes were also explored. Finally, we highlighted the present obstacles and limitations of TIL-based therapeutics, as well as improved approaches for TIL characterization. Overall, a growing body of research demonstrates the significance of specific TIL indicators for assessing response to melanoma immunotherapy and supports their prognostic significance in melanoma. TILs will become more and more useful biomarkers in upcoming studies by incorporating improved techniques and immunobiological understanding, which will enhance melanoma prognosis and clinical management. The FDA’s recent approval of Lifileucel is a step forward in the treatment of melanoma and improved patient outcomes. Future research is therefore trying to expand TIL treatment to include common epithelial malignancies, which account for more than 80% of all cancer fatalities and for which checkpoint inhibition has had little effect. Because neoantigen recognition is less prevalent in TIL than in melanoma TIL, this has necessitated screening and selection of TIL. Consequently, a number of early achievements have demonstrated the potential and promise of TIL for these illnesses (36).
Author contributions
MB: Writing – original draft, Writing – review & editing, Conceptualization. TM: Writing – review & editing. RH: Writing – review & editing. ZD: Writing – review & editing, Conceptualization, Funding acquisition.
Funding
The author(s) declare financial support was received for the research, authorship, and/or publication of this article. This work was funded by the South African Medical Research Council (SAMRC), grant number 23108, and the National Research Foundation (NRF), grant number 138139.
Conflict of interest
The authors declare that the research was conducted in the absence of any commercial or financial relationships that could be construed as a potential conflict of interest.
Publisher’s note
All claims expressed in this article are solely those of the authors and do not necessarily represent those of their affiliated organizations, or those of the publisher, the editors and the reviewers. Any product that may be evaluated in this article, or claim that may be made by its manufacturer, is not guaranteed or endorsed by the publisher.
References
1. Long GV, Swetter SM, Menzies AM, Gershenwald JE, Scolyer RA. Cutaneous melanoma. Lancet. (2023) 402:485–502. doi: 10.1016/S0140-6736(23)00821-8
2. Amin MB, Greene FL, Edge SB, Compton CC, Gershenwald JE, Brookland RK, et al. The eighth edition AJCC cancer staging manual: continuing to build a bridge from a population-based to a more “personalized” approach to cancer staging. CA: Cancer J Clin. (2017) 67:93–9. doi: 10.3322/caac.21388
3. Balch CM, Gershenwald JE, Soong S-J, Thompson JF, Atkins MB, Byrd DR, et al. Final version of 2009 AJCC melanoma staging and classification. J Clin Oncol. (2009) 27:6199–206. doi: 10.1200/JCO.2009.23.4799
4. Pollock PM, Cohen-Solal K, Sood R, Namkoong J, Martino JJ, Koganti A, et al. Melanoma mouse model implicates metabotropic glutamate signaling in melanocytic neoplasia. Nat Genet. (2003) 34:108–12. doi: 10.1038/ng1148
5. Shain AH, Yeh I, Kovalyshyn I, Sriharan A, Talevich E, Gagnon A, et al. The genetic evolution of melanoma from precursor lesions. New Engl J Med. (2015) 373:1926–36. doi: 10.1056/NEJMoa1502583
6. Leonardi GC, Falzone L, Salemi R, Zanghì A, Spandidos DA, Mccubrey JA, et al. Cutaneous melanoma: From pathogenesis to therapy. Int J Oncol. (2018) 52:1071–80. doi: 10.3892/ijo.2018.4287
7. Siegel RL, Miller KD, Goding Sauer A, Fedewa SA, Butterly LF, Anderson JC, et al. Colorectal cancer statistic. CA: Cancer J Clin. (2020) 70:145–64. doi: 10.3322/caac.21601
8. Ferlay J, Shin HR, Bray F, Forman D, Mathers C, Parkin DM. Estimates of worldwide burden of cancer in 2008: GLOBOCAN 2008. Int J Cancer. (2010) 127:2893–917. doi: 10.1002/ijc.v127:12
9. Siegel RL, Miller KD, Wagle NS, Jemal A. Cancer statistic. CA: Cancer J Clin. (2023) 73:7–48. doi: 10.3322/caac.21763
10. Arnold M, Singh D, Laversanne M, Vignat J, Vaccarella S, Meheus F, et al. Global burden of cutaneous melanoma in 2020 and projections to 2040. JAMA Dermatol. (2022) 158:495–503. doi: 10.1001/jamadermatol.2022.0160
11. Bomar L, Senithilnathan A, Ahn C. Systemic therapies for advanced melanoma. Dermatol Clinics. (2019) 37:409–23. doi: 10.1016/j.det.2019.05.001
12. Lazaroff J, Bolotin D. Targeted therapy and immunotherapy in melanoma. Dermatol Clinics. (2023) 41:65–77. doi: 10.1016/j.det.2022.07.007
13. Di Raimondo C, Lozzi F, Di Domenico PP, Campione E, Bianchi L. The diagnosis and management of cutaneous metastases from melanoma. Int J Mol Sci. (2023) 24:14535. doi: 10.3390/ijms241914535
14. Seth R, Agarwala SS, Messersmith H, Alluri KC, Ascierto PA, Atkins MB, et al. Systemic therapy for melanoma: ASCO guideline update. J Clin Oncol. (2023) 41:4794–820. doi: 10.1200/JCO.23.01136
15. Kim HJ, Kim YH. Molecular frontiers in melanoma: pathogenesis, diagnosis, and therapeutic advances. Int J Mol Sci. (2024) 25:2984. doi: 10.3390/ijms25052984
16. Hossain SM, Eccles MR. Phenotype switching and the melanoma microenvironment; impact on immunotherapy and drug resistance. Int J Mol Sci. (2023) 24:1601. doi: 10.3390/ijms24021601
17. Sanlorenzo M, Vujic I, Posch C, Dajee A, Yen A, Kim S, et al. Melanoma immunotherapy. Cancer Biol Ther. (2014) 15:665–74. doi: 10.4161/cbt.28555
18. Di Giacomo AM, Calabro L, Danielli R, Fonsatti E, Bertocci E, Pesce I, et al. Long-term survival and immunological parameters in metastatic melanoma patients who responded to ipilimumab 10 mg/kg within an expanded access programme. Cancer Immunol Immunother. (2013) 62:1021–8. doi: 10.1007/s00262-013-1418-6
19. Knight A, Karapetyan L, Kirkwood JM. Immunotherapy in melanoma: recent advances and future directions. Cancers. (2023) 15:1106. doi: 10.3390/cancers15041106
20. Gurzu S, Beleaua MA, Jung I. The role of tumor microenvironment in development and progression of Malignant melanomas-a systematic review. Rom J Morphol Embryol. (2018) 59:23–8.
21. Splendiani E, Besharat ZM, Covre A, Maio M, Di Giacomo AM, Ferretti E. Immunotherapy in melanoma: Can we predict response to treatment with circulating biomarkers? Pharmacol Ther. (2024), 108613. doi: 10.1016/j.pharmthera.2024.108613
22. Weiss SA, Kluger H. CheckMate-067: raising the bar for the next decade in oncology. J Clin Oncol. (2022) 40:111. doi: 10.1200/JCO.21.02549
23. Wolchok JD, Chiarion-Sileni V, Gonzalez R, Grob J-J, Rutkowski P, Lao CD, et al. Long-term outcomes with nivolumab plus ipilimumab or nivolumab alone versus ipilimumab in patients with advanced melanoma. J Clin Oncol. (2022) 40:127–37. doi: 10.1200/JCO.21.02229
24. Stark MS, Woods SL, Gartside MG, Bonazzi VF, Dutton-Regester K, Aoude LG, et al. Frequent somatic mutations in MAP3K5 and MAP3K9 in metastatic melanoma identified by exome sequencing. Nat Genet. (2012) 44:165–9. doi: 10.1038/ng.1041
25. Beadling C, Jacobson-Dunlop E, Hodi FS, Le C, Warrick A, Patterson J, et al. KIT gene mutations and copy number in melanoma subtypes. Clin Cancer Res. (2008) 14:6821–8. doi: 10.1158/1078-0432.CCR-08-0575
26. Handolias D, Hamilton A, Salemi R, Tan A, Moodie K, Kerr L, et al. Clinical responses observed with imatinib or sorafenib in melanoma patients expressing mutations in KIT. Br J Cancer. (2010) 102:1219–23. doi: 10.1038/sj.bjc.6605635
27. Herrscher H, Robert C. Immune checkpoint inhibitors in melanoma in the metastatic, neoadjuvant, and adjuvant setting. Curr Opin Oncol. (2020) 32:106–13. doi: 10.1097/CCO.0000000000000610
28. Falcone I, Conciatori F, Bazzichetto C, Ferretti G, Cognetti F, Ciuffreda L, et al. Tumor microenvironment: Implications in melanoma resistance to targeted therapy and immunotherapy. Cancers. (2020) 12:2870. doi: 10.3390/cancers12102870
29. Ascierto PA, Mandalà M, Ferrucci PF, Guidoboni M, Rutkowski P, Ferraresi V, et al. Sequencing of ipilimumab plus nivolumab and encorafenib plus binimetinib for untreated BRAF-mutated metastatic melanoma (SECOMBIT): a randomized, three-arm, open-label phase II trial. J Clin Oncol. (2023) 41:212–21. doi: 10.1200/JCO.21.02961
30. Baltussen JC, Welters MJ, Verdegaal EM, Kapiteijn E, Schrader AM, Slingerland M, et al. Predictive biomarkers for outcomes of immune checkpoint inhibitors (ICIs) in melanoma: A systematic review. Cancers. (2021) 13:6366. doi: 10.3390/cancers13246366
31. Simsek M, Tekin SB, Bilici M. Immunological agents used in cancer treatment. Eurasian J Med. (2019) 51:90. doi: 10.5152/eurasianjmed.2018.18194
32. Lugowska I, Teterycz P, Rutkowski P. Immunotherapy of melanoma. Contemp Oncol/Współczesna Onkol. (2018) 2018:61–7. doi: 10.5114/wo.2018.73889
33. Chen C, Gao FH. Th17 cells paradoxical roles in melanoma and potential application in immunotherapy. Front Immunol. (2019) 10:187. doi: 10.3389/fimmu.2019.00187
34. Choubey D. Type I interferon (IFN)-inducible Absent in Melanoma 2 proteins in neuroinflammation: implications for Alzheimer’s disease. J Neuroinflamm. (2019) 16:236. doi: 10.1186/s12974-019-1639-5
35. Rosenberg SA. Lymphocytes as a living drug for cancer. Science. (2024) 385:25–6. doi: 10.1126/science.adp1130
36. Kwong MM, Yang JC. Lifileucel: FDA-approved T-cell therapy for melanoma. Oncol. (2024) 29:648–50. doi: 10.1093/oncolo/oyae136
37. Sarnaik AA, Hamid O, Khushalani NI, Lewis KD, Medina T, Kluger HM, et al. Lifileucel, a tumor-infiltrating lymphocyte therapy, in metastatic melanoma. J Clin Oncol. (2021) 39:2656–66. doi: 10.1200/JCO.21.00612
38. Brummel K, Eerkens AL, De Bruyn M, Nijman HW. Tumour-infiltrating lymphocytes: from prognosis to treatment selection. Br J Cancer. (2023) 128:451–8. doi: 10.1038/s41416-022-02119-4
39. Maibach F, Sadozai H, Seyed Jafari SM, Hunger RE, Schenk M. Tumor-infiltrating lymphocytes and their prognostic value in cutaneous melanoma. Front Immunol. (2020) 11:2105. doi: 10.3389/fimmu.2020.02105
40. Fu Q, Chen N, Ge C, Li R, Li Z, Zeng B, et al. Prognostic value of tumor-infiltrating lymphocytes in melanoma: a systematic review and meta-analysis. Oncoimmunology. (2019) 8:e1593806. doi: 10.1080/2162402X.2019.1593806
41. Wang S, Sun J, Chen K, Ma P, Lei Q, Xing S, et al. Perspectives of tumor-infiltrating lymphocyte treatment in solid tumors. BMC Med. (2021) 19:140. doi: 10.1186/s12916-021-02006-4
42. Wrangle JM, Patterson A, Johnson CB, Neitzke DJ, Mehrotra S, Denlinger CE, et al. IL-2 and beyond in cancer immunotherapy. J Interferon Cytokine Res. (2018) 38:45–68. doi: 10.1089/jir.2017.0101
43. Conlon KC, Miljkovic MD, Waldmann TA. Cytokines in the treatment of cancer. J Interferon Cytokine Res. (2019) 39:6–21. doi: 10.1089/jir.2018.0019
44. Rohaan MW, Van Den Berg JH, Kvistborg P, Haanen JB. Adoptive transfer of tumor-infiltrating lymphocytes in melanoma: a viable treatment option. J Immunother Cancer. (2018) 6:102. doi: 10.1186/s40425-018-0391-1
45. Rosenberg SA, Packard BS, Aebersold PM, Solomon D, Topalian SL, Toy ST, et al. Use of tumor-infiltrating lymphocytes and interleukin-2 in the immunotherapy of patients with metastatic melanoma. A preliminary report. New Engl J Med. (1988) 319:1676–80. doi: 10.1056/NEJM198812223192527
46. Chesney JA. Tumor-infiltrating lymphocyte therapy in metastatic melanoma. Clin Adv Hematol Oncol: HandO. (2023) 21:49–51.
47. Clark WH Jr., Elder DE, Guerry Iv D, Braitman LE, Trock BJ, Schultz D, et al. Model predicting survival in stage I melanoma based on tumor progression. JNCI: J Natl Cancer Institute. (1989) 81:1893–904. doi: 10.1093/jnci/81.24.1893
48. Van Houdt IS, Sluijter BJ, Moesbergen LM, Vos WM, De Gruijl TD, Molenkamp BG, et al. Favorable outcome in clinically stage II melanoma patients is associated with the presence of activated tumor infiltrating T-lymphocytes and preserved MHC class I antigen expression. Int J Cancer. (2008) 123:609–15. doi: 10.1002/ijc.v123:3
49. Han D, Zager JS, Shyr Y, Chen H, Berry LD, Iyengar S, et al. Clinicopathologic predictors of sentinel lymph node metastasis in thin melanoma. J Clin Oncol. (2013) 31:4387–93. doi: 10.1200/JCO.2013.50.1114
50. Vrbić S, Filipović S, Pejić I, Vrbić M, Filipović A. Sensitivity, specificity, positive and negative predictive value of serum S-100 beta protein in patients with Malignant melanoma. J BU ON: Off J Balkan Union Oncol. (2003) 8:139–41.
51. Berk DR, Johnson DL, Uzieblo A, Kiernan M, Swetter SM. Sentinel lymph node biopsy for cutaneous melanoma: the Stanford experience 1997-2004. Arch Dermatol. (2005) 141:1016–22. doi: 10.1001/archderm.141.8.1016
52. Nikolin B, Djan I, Trifunovic J, Dugandzija T, Novkovic D, Djan V, et al. MIA, S100 and LDH as important predictors of overall survival of patients with stage IIb and IIc melanoma. J BUON. (2016) 21:691–7.
53. Azimi F, Scolyer RA, Rumcheva P, Moncrieff M, Murali R, Mccarthy SW, et al. Tumor-infiltrating lymphocyte grade is an independent predictor of sentinel lymph node status and survival in patients with cutaneous melanoma. J Clin Oncol. (2012) 30:2678–83. doi: 10.1200/JCO.2011.37.8539
54. Gangi A, Essner R, Giuliano A. Long-term clinical impact of sentinel lymph node biopsy in breast cancer and cutaneous melanoma. Q J Nucl Med Mol Imaging. (2014) 58:95–104.
55. Francken AB, Shaw HM, Thompson JF, Soong S-J, Accortt NA, Azzola MF, et al. The prognostic importance of tumor mitotic rate confirmed in 1317 patients with primary cutaneous melanoma and long follow-up. Ann Surg Oncol. (2004) 11:426–33. doi: 10.1245/ASO.2004.07.014
56. Mcmasters KM, Wong SL, Edwards MJ, Ross MI, Chao C, Noyes RD, et al. Factors that predict the presence of sentinel lymph node metastasis in patients with melanoma. Surgery. (2001) 130:151–6. doi: 10.1067/msy.2001.115830
57. Tuthill RJ, Unger JM, Liu P, Flaherty LE, Sondak VK. Risk assessment in localized primary cutaneous melanoma: a Southwest Oncology Group study evaluating nine factors and a test of the Clark logistic regression prediction model. Am J Clin Pathol. (2002) 118:504–11. doi: 10.1309/WBF7-N8KH-71KT-RVQ9
58. Mcmasters KM, Reintgen DS, Ross MI, Gershenwald JE, Edwards MJ, Sober A, et al. Sentinel lymph node biopsy for melanoma: controversy despite widespread agreement. J Clin Oncol. (2001) 19:2851–5. doi: 10.1200/JCO.2001.19.11.2851
59. Mihm MC Jr., Mulé JJ. Reflections on the histopathology of tumor-infiltrating lymphocytes in melanoma and the host immune response. Cancer Immunol Res. (2015) 3:827–35. doi: 10.1158/2326-6066.CIR-15-0143
60. Lee N, Zakka LR, Mihm MC Jr., Schatton T. Tumour-infiltrating lymphocytes in melanoma prognosis and cancer immunotherapy. Pathology. (2016) 48:177–87. doi: 10.1016/j.pathol.2015.12.006
61. Barnes TA, Amir E. HYPE or HOPE: the prognostic value of infiltrating immune cells in cancer. Br J Cancer. (2017) 117:451–60. doi: 10.1038/bjc.2017.220
62. Ruiter D, Bogenrieder T, Elder D, Herlyn M. Melanoma–stroma interactions: structural and functional aspects. Lancet Oncol. (2002) 3:35–43. doi: 10.1016/S1470-2045(01)00620-9
63. Pantanowitz L, Sharma A, Carter AB, Kurc T, Sussman A, Saltz J. Twenty years of digital pathology: an overview of the road travelled, what is on the horizon, and the emergence of vendor-neutral archives. J Pathol Inf. (2018) 9:40. doi: 10.4103/jpi.jpi_69_18
64. Kather JN, Suarez-Carmona M, Charoentong P, Weis C-A, Hirsch D, Bankhead P, et al. Topography of cancer-associated immune cells in human solid tumors. Elife. (2018) 7:e36967. doi: 10.7554/eLife.36967
65. Němejcová K, Tichá I, Bártů M, Kodet O, Důra M, Jakša R, et al. Comparison of five different scoring methods in the evaluation of inflammatory infiltration (tumor-infiltrating lymphocytes) in superficial spreading and nodular melanoma. Pigment Cell Melanoma Res. (2019) 32:412–23. doi: 10.1111/pcmr.12757
66. Ladányi A. Prognostic and predictive significance of immune cells infiltrating cutaneous melanoma. Pigment Cell Melanoma Res. (2015) 28:490–500. doi: 10.1111/pcmr.2015.28.issue-5
67. Erdag G, Schaefer JT, Smolkin ME, Deacon DH, Shea SM, Dengel LT, et al. Immunotype and immunohistologic characteristics of tumor-infiltrating immune cells are associated with clinical outcome in metastatic melanoma. Cancer Res. (2012) 72:1070–80. doi: 10.1158/0008-5472.CAN-11-3218
68. Kakavand H, Vilain RE, Wilmott JS, Burke H, Yearley JH, Thompson JF, et al. Tumor PD-L1 expression, immune cell correlates and PD-1+ lymphocytes in sentinel lymph node melanoma metastases. Modern Pathol. (2015) 28:1535–44. doi: 10.1038/modpathol.2015.110
69. Elder DE. Pathology of melanoma. Surg Oncol Clinics. (2015) 24:229–37. doi: 10.1016/j.soc.2014.12.002
70. Clemente CG, Mihm MC Jr., Bufalino R, Zurrida S, Collini P, Cascinelli N. Prognostic value of tumor infiltrating lymphocytes in the vertical growth phase of primary cutaneous melanoma. Cancer: Interdiscip Int J Am Cancer Soc. (1996) 77:1303–10. doi: 10.1002/(SICI)1097-0142(19960401)77:7<1303::AID-CNCR12>3.0.CO;2-5
71. Busam KJ, Antonescu CR, Marghoob AA, Nehal KS, Sachs DL, Shia J, et al. Histologic classification of tumor-infiltrating lymphocytes in primary cutaneous Malignant melanoma: a study of interobserver agreement. Am J Clin Pathol. (2001) 115:856–60. doi: 10.1309/G6EK-Y6EH-0LGY-6D6P
72. Taylor RC, Patel A, Panageas KS, Busam KJ, Brady MS. Tumor-infiltrating lymphocytes predict sentinel lymph node positivity in patients with cutaneous melanoma. J Clin Oncol. (2007) 25:869–75. doi: 10.1200/JCO.2006.08.9755
73. Balch CM, Soong S-J, Gershenwald JE, Thompson JF, Reintgen DS, Cascinelli N, et al. Prognostic factors analysis of 17,600 melanoma patients: validation of the American Joint Committee on Cancer melanoma staging system. J Clin Oncol. (2001) 19:3622–34. doi: 10.1200/JCO.2001.19.16.3622
74. Hendry S, Salgado R, Gevaert T, Russell PA, John T, Thapa B, et al. Assessing tumor-infiltrating lymphocytes in solid tumors: a practical review for pathologists and proposal for a standardized method from the International Immuno-Oncology Biomarkers Working Group: Part 2: TILs in melanoma, gastrointestinal tract carcinomas, non–small cell lung carcinoma and mesothelioma, endometrial and ovarian carcinomas, squamous cell carcinoma of the head and neck, genitourinary carcinomas, and primary brain tumors. Adv Anatomic Pathol. (2017) 24:311–35. doi: 10.1097/PAP.0000000000000161
75. Joyce JA, Fearon DT. T cell exclusion, immune privilege, and the tumor microenvironment. Science. (2015) 348:74–80. doi: 10.1126/science.aaa6204
76. Van Der Woude LL, Gorris MA, Halilovic A, Figdor CG, De Vries IJM. Migrating into the tumor: a roadmap for T cells. Trends Cancer. (2017) 3:797–808. doi: 10.1016/j.trecan.2017.09.006
77. Hegde PS, Karanikas V, Evers S. The where, the when, and the how of immune monitoring for cancer immunotherapies in the era of checkpoint inhibition. Clin Cancer Res. (2016) 22:1865–74. doi: 10.1158/1078-0432.CCR-15-1507
78. Becht E, Giraldo NA, Dieu-Nosjean M-C, Sautès-Fridman C, Fridman WH. Cancer immune contexture and immunotherapy. Curr Opin Immunol. (2016) 39:7–13. doi: 10.1016/j.coi.2015.11.009
79. Fridman WH, Zitvogel L, Sautès–Fridman C, Kroemer G. The immune contexture in cancer prognosis and treatment. Nat Rev Clin Oncol. (2017) 14:717–34. doi: 10.1038/nrclinonc.2017.101
80. Gonzalez H, Hagerling C, Werb Z. Roles of the immune system in cancer: from tumor initiation to metastatic progression. Genes Dev. (2018) 32:1267–84. doi: 10.1101/gad.314617.118
81. Martínez-Lostao L, Anel A, Pardo J. How do cytotoxic lymphocytes kill cancer cells? Clin Cancer Res. (2015) 21:5047–56. doi: 10.1158/1078-0432.CCR-15-0685
82. Durgeau A, Virk Y, Corgnac S, Mami-Chouaib F. Recent advances in targeting CD8 T-cell immunity for more effective cancer immunotherapy. Front Immunol. (2018) 9:14. doi: 10.3389/fimmu.2018.00014
83. Morvan MG, Lanier LL. NK cells and cancer: you can teach innate cells new tricks. Nat Rev Cancer. (2016) 16:7–19. doi: 10.1038/nrc.2015.5
84. Böttcher JP, Sousa CRE. The role of type 1 conventional dendritic cells in cancer immunity. Trends Cancer. (2018) 4:784–92. doi: 10.1016/j.trecan.2018.09.001
85. Teng MW, Galon J, Fridman WH, Smyth MJ. From mice to humans: developments in cancer immunoediting. J Clin Invest. (2015) 125:3338–46. doi: 10.1172/JCI80004
86. Mclane LM, Abdel-Hakeem MS, Wherry EJ. CD8 T cell exhaustion during chronic viral infection and cancer. Annu Rev Immunol. (2019) 37:457–95. doi: 10.1146/annurev-immunol-041015-055318
87. Piras F, Colombari R, Minerba L, Murtas D, Floris C, Maxia C, et al. The predictive value of CD8, CD4, CD68, and human leukocyte antigen-D-related cells in the prognosis of cutaneous Malignant melanoma with vertical growth phase. Cancer: Interdiscip Int J Am Cancer Soc. (2005) 104:1246–54. doi: 10.1002/cncr.v104:6
88. Jensen TO, Schmidt H, Møller HJ, Donskov F, Høyer M, Sjoegren P, et al. Intratumoral neutrophils and plasmacytoid dendritic cells indicate poor prognosis and are associated with pSTAT3 expression in AJCC stage I/II melanoma. Cancer. (2012) 118:2476–85. doi: 10.1002/cncr.v118.9
89. Jacquelot N, Roberti MP, Enot DP, Rusakiewicz S, Semeraro M, Jégou S, et al. Immunophenotyping of stage III melanoma reveals parameters associated with patient prognosis. J Invest Dermatol. (2016) 136:994–1001. doi: 10.1016/j.jid.2015.12.042
90. Simoni Y, Becht E, Fehlings M, Loh CY, Koo S-L, Teng KWW, et al. Bystander CD8+ T cells are abundant and phenotypically distinct in human tumour infiltrates. Nature. (2018) 557:575–9. doi: 10.1038/s41586-018-0130-2
91. Chauvin J-M, Pagliano O, Fourcade J, Sun Z, Wang H, Sander C, et al. TIGIT and PD-1 impair tumor antigen–specific CD8+ T cells in melanoma patients. J Clin Invest. (2015) 125:2046–58. doi: 10.1172/JCI80445
92. Jacquelot N, Pitt JM, Enot DP, Roberti MP, Duong CP, Rusakiewicz S, et al. Immune biomarkers for prognosis and prediction of responses to immune checkpoint blockade in cutaneous melanoma. Oncoimmunology. (2017) 6:e1299303. doi: 10.1080/2162402X.2017.1299303
93. Kim HJ, Cantor H. CD4 T-cell subsets and tumor immunity: the helpful and the not-so-helpful. Cancer Immunol Res. (2014) 2:91–8. doi: 10.1158/2326-6066.CIR-13-0216
94. Borst J, Ahrends T, Bąbała N, Melief CJ, Kastenmüller W. CD4+ T cell help in cancer immunology and immunotherapy. Nat Rev Immunol. (2018) 18:635–47. doi: 10.1038/s41577-018-0044-0
95. Raphael I, Nalawade S, Eagar TN, Forsthuber TG. T cell subsets and their signature cytokines in autoimmune and inflammatory diseases. Cytokine. (2015) 74:5–17. doi: 10.1016/j.cyto.2014.09.011
96. Ward-Hartstonge KA, Kemp RA. Regulatory T-cell heterogeneity and the cancer immune response. Clin Trans Immunol. (2017) 6:e154. doi: 10.1038/cti.2017.43
97. Bichakjian CK, Halpern AC, Johnson TM, Hood AF, Grichnik JM, Swetter SM, et al. Guidelines of care for the management of primary cutaneous melanoma. J Am Acad Dermatol. (2011) 65:1032–47. doi: 10.1016/j.jaad.2011.04.031
98. Eftimie R, Hamam H. Modelling and investigation of the CD4+ T cells–macrophages paradox in melanoma immunotherapies. J Theor Biol. (2017) 420:82–104. doi: 10.1016/j.jtbi.2017.02.022
99. Lowes MA, Bishop GA, Crotty K, Barnetson RSC, Halliday GM. T helper 1 cytokine mRNA is increased in spontaneously regressing primary melanomas. J Invest Dermatol. (1997) 108:914–9. doi: 10.1111/1523-1747.ep12292705
100. Grotz TE, Jakub JW, Mansfield AS, Goldenstein R, Enninga EAL, Nevala WK, et al. Evidence of Th2 polarization of the sentinel lymph node (SLN) in melanoma. Oncoimmunology. (2015) 4:e1026504. doi: 10.1080/2162402X.2015.1026504
101. Ling A, Lundberg IV, Eklöf V, Wikberg ML, Öberg Å, Edin S, et al. The infiltration, and prognostic importance, of Th1 lymphocytes vary in molecular subgroups of colorectal cancer. J Pathol: Clin Res. (2016) 2:21–31. doi: 10.1002/cjp2.v2.1
102. Xu Y, Chen L, Xu B, Xiong Y, Yang M, Rui X, et al. Higher numbers of T-Bet+ tumor-infiltrating lymphocytes associate with better survival in human epithelial ovarian cancer. Cell Physiol Biochem. (2017) 41:475–83. doi: 10.1159/000456600
103. Levine AG, Mendoza A, Hemmers S, Moltedo B, Niec RE, Schizas M, et al. Stability and function of regulatory T cells expressing the transcription factor T-bet. Nature. (2017) 546:421–5. doi: 10.1038/nature22360
104. Tallerico R, Todaro M, Di Franco S, Maccalli C, Garofalo C, Sottile R, et al. Human NK cells selective targeting of colon cancer–initiating cells: a role for natural cytotoxicity receptors and MHC class I molecules. J Immunol. (2013) 190:2381–90. doi: 10.4049/jimmunol.1201542
105. Smyth MJ, Hayakawa Y, Takeda K, Yagita H. New aspects of natural-killer-cell surveillance and therapy of cancer. Nat Rev Cancer. (2002) 2:850–61. doi: 10.1038/nrc928
106. Degli-Esposti MA, Smyth MJ. Close encounters of different kinds: dendritic cells and NK cells take centre stage. Nat Rev Immunol. (2005) 5:112–24. doi: 10.1038/nri1549
107. Fregni G, Perier A, Avril MF, Caignard A. NK cells sense tumors, course of disease and treatments. Consequences for NK-based therapies. OncoImmunology. (2012) 1:38–47. doi: 10.4161/onci.1.1.18312
108. Platonova S, Cherfils-Vicini J, Damotte D, Crozet L, Vieillard V, Validire P, et al. Profound alterations of intratumoral NK cell phenotype and function in lung carcinoma. Cancer Res. (2011) 71:5412–22. doi: 10.1158/0008-5472.CAN-10-4179
109. Balsamo M, Scordamaglia F, Pietra G, Manzini C, Cantoni C, Boitano M, et al. Melanoma-associated fibroblasts modulate NK cell phenotype and antitumor cytotoxicity. Proc Natl Acad Sci. (2009) 106(49):20847–52.
110. Pietra G, Manzini C, Rivara S, Vitale M, Cantoni C, Petretto A, et al. Melanoma cells inhibit natural killer cell function by modulating the expression of activating receptors and cytolytic activity. Cancer Res. (2012) 72(6):1407–15.
111. Senovilla L, Vacchelli E, Galon J, Adjemian S, Eggermont A, Fridman WH, et al. Prognostic and predictive value of the immune infiltrate in cancer. OncoImmunology. (2012) 1:1323–43. doi: 10.4161/onci.22009
112. Remark R, Alifano M, Cremer I, Lupo A, Dieu-Nosjean MC, Riquet M, et al. Characteristics and clinical impacts of the immune environments in colorectal and renal cell carcinoma lung metastases: influence of tumor origin. Clin Cancer Res. (2013) 19:4079–91. doi: 10.1158/1078-0432.CCR-12-3847
113. De Jonge K, Ebering A, Nassiri S, Hajjami HM-E, Ouertatani-Sakouhi H, Baumgaertner P, et al. Circulating CD56bright NK cells inversely correlate with survival of melanoma patients. Sci Rep. (2019) 9:4487. doi: 10.1038/s41598-019-40933-8
114. Fregni G, Messaoudene M, Fourmentraux-Neves E, Mazouz-Dorval S, Chanal J, Maubec E, et al. Phenotypic and functional characteristics of blood natural killer cells from melanoma patients at different clinical stages. PloS One. (2013) 8:e76928. doi: 10.1371/journal.pone.0076928
115. de Coaña YP, Wolodarski M, Àvila IVDH, Nakajima T, Rentouli S, Lundqvist A, et al. PD-1 checkpoint blockade in advanced melanoma patients: NK cells, monocytic subsets and host PD-L1 expression as predictive biomarker candidates. OncoImmunology. (2020) 9:178688. doi: 10.1080/2162402X.2020.1786888
116. Cursons J, Souza-Fonseca-Guimaraes F, Foroutan M, Anderson A, Hollande F, Hediyeh-Zadeh S, et al. Gene signature predicting natural killer cell infiltration and improved survival in melanoma patients. Cancer Immunol Res. (2019) 7:1162–74. doi: 10.1158/2326-6066.CIR-18-0500
117. Qin Z, Richter G, Sch€uler T, Ibe S, Cao X, Blankenstein T. B cells inhibit induction of T cell-dependent tumor immunity. Nat Med. (1998) 4:627–30. doi: 10.1038/nm0598-627
118. de Visser KE, Korets LV, Coussens LM. De novo carcinogenesis promoted by chronic inflammation is B lymphocyte dependent. Cancer Cell. (2005) 7:411–23. doi: 10.1016/j.ccr.2005.04.014
119. DiLillo DJ, Yanaba K, Tedder TF. B cells are required for optimal CD4+ and CD8+ T cell tumor immunity: therapeutic B cell depletion enhances B16 melanoma growth in mice. J Immunol. (2010) 184:4006–16. doi: 10.4049/jimmunol.0903009
120. Nelson BH. CD20+ B cells: the other tumor-infiltrating lymphocytes. J Immunol. (2010) 185:4977–82. doi: 10.4049/jimmunol.1001323
121. Hillen F, Baeten CIM, van de Winkel A, Creytens D, van der Schaft DWJ, Winnepenninckx V, et al. Leukocyte infiltration and tumor cell plasticity are parameters of aggressiveness in primary cutaneous melanoma. Cancer Immunol Immunother. (2008) 57:97–106. doi: 10.1007/s00262-007-0353-9
122. Hussein MR, Elsers DAH, Fadel SA, Omar AEM. Immunohistological characterization of tumour infiltrating lymphocytes in melanocytic skin lesions. J Clin Pathol. (2006) 59:316–24. doi: 10.1136/jcp.2005.028860
123. Ladányi A, Kiss J, Mohos A, Somlai B, Liszkay G, Gilde K, et al. Prognostic impact of B-cell density in cutaneous melanoma. Cancer Immunol Immunother. (2011) 60:1729–38. doi: 10.1007/s00262-011-1071-x
124. Ladányi A, Sebestyen T, Mohos A, Liszkay G, Somlai B, Toth E, et al. Ectopic lymphoid structures in primary cutaneous melanoma. Pathol Oncol Res. (2014) 20:981–5. doi: 10.1007/s12253-014-9784-8
125. Cipponi A, Mercier M, Seremet T, Baurain JF, Th eate I, van den Oord J, et al. Neogenesis of lymphoid structures and antibody responses occur in human melanoma metastases. Cancer Res. (2012) 72:3997–4007. doi: 10.1158/0008-5472.CAN-12-1377
126. Coronella-Wood JA, Hersh EM. Naturally occurring Bcell responses to breast cancer. Cancer Immunol Immunother. (2003) 52:715–38. doi: 10.1007/s00262-003-0409-4
127. Dieu-Nosjean MC, Antoine M, Danel C, Heudes D, Wislez M, Poulot V, et al. Long-term survival for patients with non–small-cell lung cancer with intratumoral lymphoid structures. J Clin Oncol. (2008) 26:4410–7. doi: 10.1200/JCO.2007.15.0284
128. Messina JL, Fenstermacher DA, Eschrich S, Qu X, Berglund AE, Lloyd MC, et al. 12-chemokine gene signature identifies lymph node-like structures in melanoma: potential for patient selection for immunotherapy? Sci Rep. (2012) 2:765. doi: 10.1038/srep00765
129. Loi S, Michiels S, Adams S, Loibl S, Budczies J, Denkert C, et al. The journey of tumor-infiltrating lymphocytes as a biomarker in breast cancer: clinical utility in an era of checkpoint inhibition. Ann Oncol. (2021) 32:1236–44. doi: 10.1016/j.annonc.2021.07.007
130. Huang Y, Liao H, Zhang Y, Yuan R, Wang F, Gao Y, et al. Prognostic value of tumor-infiltrating FoxP3+ T cells in gastrointestinal cancers: a meta analysis. PloS One. (2014) 9:e94376. doi: 10.1371/journal.pone.0094376
131. Bianchini G, Huang C, Egle D, Bermejo B, Zamagni C, Thill M, et al. LBA13 Tumour infiltrating lymphocytes (TILs), PD-L1 expression and their dynamics in the NeoTRIPaPDL1 trial. Ann Oncol. (2020) 31:S1145–6. doi: 10.1016/j.annonc.2020.08.2241
132. Tumeh PC, Harview CL, Yearley JH, Shintaku IP, Taylor EJ, Robert L, et al. PD-1 blockade induces responses by inhibiting adaptive immune resistance. Nature. (2014) 515:568–71. doi: 10.1038/nature13954
133. Gide TN, Silva IP, Quek C, Ahmed T, Menzies AM, Carlino MS, et al. Close proximity of immune and tumor cells underlies response to anti-PD-1 based therapies in metastatic melanoma patients. Oncoimmunology. (2020) 9:1659093. doi: 10.1080/2162402X.2019.1659093
134. Harder N, Schönmeyer R, Nekolla K, Meier A, Brieu N, Vanegas C, et al. Automatic discovery of image-based signatures for ipilimumab response prediction in Malignant melanoma. Sci Rep. (2019) 9:7449. doi: 10.1038/s41598-019-43525-8
135. Madonna G, Ballesteros-Merino C, Feng Z, Bifulco C, Capone M, Giannarelli D, et al. PD-L1 expression with immune-infiltrate evaluation and outcome prediction in melanoma patients treated with ipilimumab. Oncoimmunology. (2018) 7:e1405206. doi: 10.1080/2162402X.2017.1405206
136. Wong PF, Wei W, Smithy JW, Acs B, Toki MI, Blenman KR, et al. Multiplex quantitative analysis of tumor-infiltrating lymphocytes and immunotherapy outcome in metastatic melanoma. Clin Cancer Res. (2019) 25:2442–9. doi: 10.1158/1078-0432.CCR-18-2652
137. Loupakis F, Depetris I, Biason P, Intini R, Prete AA, Leone F, et al. Prediction of benefit from checkpoint inhibitors in mismatch repair deficient metastatic colorectal cancer: role of tumor infiltrating lymphocytes. Oncologist. (2020) 25:481–7. doi: 10.1634/theoncologist.2019-0611
138. Kato K, Doki Y, Ura T, Hamamoto Y, Kojima T, Tsushima T, et al. Long-term efficacy and predictive correlates of response to nivolumab in Japanese patients with esophageal cancer. Cancer Sci. (2020) 111:1676–84. doi: 10.1111/cas.v111.5
139. Konstantinopoulos PA, Luo W, Liu JF, Gulhan DC, Krasner C, Ishizuka JJ, et al. Phase II study of avelumab in patients with mismatch repair deficient and mismatch repair proficient recurrent/persistent endometrial cancer. J Clin Oncol. (2019) 37:2786–94. doi: 10.1200/JCO.19.01021
140. Pignon J-C, Jegede O, Shukla SA, Braun DA, Horak CE, Wind-Rotolo M, et al. irRECIST for the evaluation of candidate biomarkers of response to nivolumab in metastatic clear cell renal cell carcinoma: analysis of a phase II prospective clinical trial. Clin Cancer Res. (2019) 25:2174–84. doi: 10.1158/1078-0432.CCR-18-3206
141. Ficial M, Jegede OA, Sant’angelo M, Hou Y, Flaifel A, Pignon J-C, et al. Expression of T-cell exhaustion molecules and human endogenous retroviruses as predictive biomarkers for response to nivolumab in metastatic clear cell renal cell carcinoma. Clin Cancer Res. (2021) 27:1371–80. doi: 10.1158/1078-0432.CCR-20-3084
142. Zheng L, Qin S, Si W, Wang A, Xing B, Gao R, et al. Pan-cancer single-cell landscape of tumor-infiltrating T cells. Science. (2021) 374:abe6474. doi: 10.1126/science.abe6474
143. Gataa I, Mezquita L, Rossoni C, Auclin E, Kossai M, Aboubakar F, et al. Tumour-infiltrating lymphocyte density is associated with favourable outcome in patients with advanced non–small cell lung cancer treated with immunotherapy. Eur J Cancer. (2021) 145:221–9. doi: 10.1016/j.ejca.2020.10.017
144. Amaria RN, Reddy SM, Tawbi HA, Davies MA, Ross MI, Glitza IC, et al. Neoadjuvant immune checkpoint blockade in high-risk resectable melanoma. Nat Med. (2018) 24:1649–54. doi: 10.1038/s41591-018-0197-1
145. Uryvaev A, Passhak M, Hershkovits D, Sabo E, Bar-Sela G. The role of tumor-infiltrating lymphocytes (TILs) as a predictive biomarker of response to anti-PD1 therapy in patients with metastatic non-small cell lung cancer or metastatic melanoma. Med Oncol. (2018) 35:1–9. doi: 10.1007/s12032-018-1080-0
146. Spassova I, Ugurel S, Terheyden P, Sucker A, Hassel JC, Ritter C, et al. Predominance of central memory T cells with high T-cell receptor repertoire diversity is associated with response to PD-1/PD-L1 inhibition in Merkel cell carcinoma. Clin Cancer Res. (2020) 26:2257–67. doi: 10.1158/1078-0432.CCR-19-2244
147. Schoenfeld JD, Hanna GJ, Jo VY, Rawal B, Chen Y-H, Catalano PS, et al. Neoadjuvant nivolumab or nivolumab plus ipilimumab in untreated oral cavity squamous cell carcinoma: a phase 2 open-label randomized clinical trial. JAMA Oncol. (2020) 6:1563–70. doi: 10.1001/jamaoncol.2020.2955
148. Gaggero S, Martinez-Fabregas J, Cozzani A, Fyfe PK, Leprohon M, Yang J, et al. IL-2 is inactivated by the acidic pH environment of tumors enabling engineering of a pH-selective mutein. Sci Immunol. (2022) 7:eade5686. doi: 10.1126/sciimmunol.ade5686
149. Giatromanolaki A, Koukourakis IM, Balaska K, Mitrakas AG, Harris AL, Koukourakis MI. Programmed death-1 receptor (PD-1) and PD-ligand-1 (PD-L1) expression in non-small cell lung cancer and the immune-suppressive effect of anaerobic glycolysis. Med Oncol. (2019) 36:1–12. doi: 10.1007/s12032-019-1299-4
150. Loeffler D, Juneau P, Masserant S. Influence of tumour physico-chemical conditions on interleukin-2-stimulated lymphocyte proliferation. Br J Cancer. (1992) 66:619–22. doi: 10.1038/bjc.1992.326
151. Calcinotto A, Filipazzi P, Grioni M, Iero M, De Milito A, Ricupito A, et al. Modulation of microenvironment acidity reverses anergy in human and murine tumor-infiltrating T lymphocytes. Cancer Res. (2012) 72:2746–56. doi: 10.1158/0008-5472.CAN-11-1272
152. Multhoff G, Vaupel P. Hypoxia compromises anti-cancer immune responses. Oxygen transport to tissue XLI. (2020), 131–43.
153. Zandberg DP, Menk AV, Velez M, Normolle D, Depeaux K, Liu A, et al. Tumor hypoxia is associated with resistance to PD-1 blockade in squamous cell carcinoma of the head and neck. J Immunother Cancer. (2021) 9. doi: 10.1136/jitc-2020-002088
154. Peng H, He X, Wang Q. Immune checkpoint blockades in gynecological cancers: A review of clinical trials. Acta Obstet Gynecol Scand. (2022) 101:941–51. doi: 10.1111/aogs.v101.9
155. Khatoon E, Parama D, Kumar A, Alqahtani MS, Abbas M, Girisa S, et al. Targeting PD-1/PD-L1 axis as new horizon for ovarian cancer therapy. Life Sci. (2022) 306:120827. doi: 10.1016/j.lfs.2022.120827
156. Qin SS, Melucci AD, Chacon AC, Prieto PA. Adoptive T cell therapy for solid tumors: pathway to personalized standard of care. Cells. (2021) 10:808. doi: 10.3390/cells10040808
157. Isaak AJ, Clements GR, Buenaventura RGM, Merlino G, Yu Y. Development of personalized strategies for precisely battling Malignant melanoma. Int J Mol Sci. (2024) 25:5023. doi: 10.3390/ijms25095023
158. Betof Warner A, Corrie PG, Hamid O. Tumor-infiltrating lymphocyte therapy in melanoma: facts to the future. Clin Cancer Res. (2023) 29(10):1835–54.
159. Feins S, Kong W, Williams EF, Milone MC, Fraietta JA. An introduction to chimeric antigen receptor (CAR) T-cell immunotherapy for human cancer. Am J Hematol. (2019) 94:S3–9. doi: 10.1002/ajh.v94.S1
160. Goff. A Prospective Randomized and Phase 2 Trial for Metastatic Melanoma Using Adoptive Cell Therapy with tumor Infiltrating lymphocytes plus IL-2 either alone or following the administration of pembrolizumab. (2023). Available online at: https://clinicaltrials.gov/study/NCT02621021.
161. Havel JJ, Chowell D, Chan TA. The evolving landscape of biomarkers for checkpoint inhibitor immunotherapy. Nat Rev Cancer. (2019) 19:133–50. doi: 10.1038/s41568-019-0116-x
162. Buder-Bakhaya K, Hassel JC. Biomarkers for clinical benefit of immune checkpoint inhibitor treatment—a review from the melanoma perspective and beyond. Front Immunol. (2018) 9:1474. doi: 10.3389/fimmu.2018.01474
163. Pagès F, Mlecnik B, Marliot F, Bindea G, Ou F-S, Bifulco C, et al. International validation of the consensus Immunoscore for the classification of colon cancer: a prognostic and accuracy study. Lancet. (2018) 391:2128–39. doi: 10.1016/S0140-6736(18)30789-X
164. Galon J, Mlecnik B, Bindea G, Angell HK, Berger A, Lagorce C, et al. Towards the introduction of the ‘Immunoscore’in the classification of Malignant tumours. J Pathol. (2014) 232:199–209. doi: 10.1002/path.2014.232.issue-2
165. Takada K, Kashiwagi S, Goto W, Asano Y, Takahashi K, Takashima T, et al. Use of the tumor-infiltrating CD8 to FOXP3 lymphocyte ratio in predicting treatment responses to combination therapy with pertuzumab, trastuzumab, and docetaxel for advanced HER2-positive breast cancer. J Trans Med. (2018) 16:1–11. doi: 10.1186/s12967-018-1460-4
166. Wang K, Shen T, Siegal GP, Wei S. The CD4/CD8 ratio of tumor-infiltrating lymphocytes at the tumor-host interface has prognostic value in triple-negative breast cancer. Hum Pathol. (2017) 69:110–7. doi: 10.1016/j.humpath.2017.09.012
167. Angell HK, Bruni D, Barrett JC, Herbst R, Galon J. The immunoscore: colon cancer and beyond. Clin Cancer Res. (2020) 26:332–9. doi: 10.1158/1078-0432.CCR-18-1851
168. Galon J, Lugli A, Bifulco C, Pages F, Masucci G, Marincola FM, et al. World-wide immunoscore task force: meeting report from the “Melanoma Bridge”, Napoli, November 30th–December 3r. United States: Springer (2017).
169. Moffitt JR, Hao J, Bambah-Mukku D, Lu T, Dulac C, Zhuang X. High-performance multiplexed fluorescence in situ hybridization in culture and tissue with matrix imprinting and clearing. Proc Natl Acad Sci. (2016) 113:14456–61. doi: 10.1073/pnas.1617699113
170. Gut G, Herrmann MD, Pelkmans L. Multiplexed protein maps link subcellular organization to cellular states. Science. (2018) 361:eaar7042. doi: 10.1126/science.aar7042
171. Parra ER, Francisco-Cruz A, Wistuba II. State-of-the-art of profiling immune contexture in the era of multiplexed staining and digital analysis to study paraffin tumor tissues. Cancers. (2019) 11:247. doi: 10.3390/cancers11020247
Keywords: melanoma, tumor-infiltrating lymphocytes, prognosis, adoptive cell therapy (ACT), immunotherapy, immune checkpoint inhibitors (ICIs), CD8+, CD4+
Citation: Bida M, Miya TV, Hull R and Dlamini Z (2024) Tumor-infiltrating lymphocytes in melanoma: from prognostic assessment to therapeutic applications. Front. Immunol. 15:1497522. doi: 10.3389/fimmu.2024.1497522
Received: 17 September 2024; Accepted: 04 November 2024;
Published: 06 December 2024.
Edited by:
Wenxue Ma, University of California, San Diego, United StatesReviewed by:
María Marcela Barrio, Fundación Cáncer, ArgentinaIrfan Naseem Bandey, University of Texas MD Anderson Cancer Center, United States
Copyright © 2024 Bida, Miya, Hull and Dlamini. This is an open-access article distributed under the terms of the Creative Commons Attribution License (CC BY). The use, distribution or reproduction in other forums is permitted, provided the original author(s) and the copyright owner(s) are credited and that the original publication in this journal is cited, in accordance with accepted academic practice. No use, distribution or reproduction is permitted which does not comply with these terms.
*Correspondence: Meshack Bida, TWVzaGFjay5iaWRhQG5obHMuYWMuemE=; Zodwa Dlamini, em9kd2EuZGxhbWluaUB1cC5hYy56YQ==