- 1MOA Key Laboratory of Animal Virology, Zhejiang University Center for Veterinary Sciences, Hangzhou, China
- 2Institute of Comparative Medicine, College of Veterinary Medicine, Yangzhou University, Yangzhou, China
- 3Jiangsu Co-Innovation Center for Prevention and Control of Important Animal Infectious Diseases and Zoonosis, Yangzhou University, Yangzhou, China
Pseudorabies virus (PRV), causing Aujeszky’s disease in swine, has important economic impact on the pig industry in China and even poses a threat to public health. Although this disease has been controlled by vaccination with PRV live attenuated vaccines (LAVs), the potency of PRV LAVs in inducing cellular immunity has not been well characterized. In this study, using PRV Bartha K61 strain (BK61), the most-used PRV LAVs, as a model, we re-examined the cellular immune response elicited by the BK61 in mice and pigs by multicolor flow cytometry. We found that phenotypic activation of T cells, NK cells and B cells was hardly detected after vaccination. However, antigen-specific IFN-γ-producing CD4 T cells rather than CD8 T cells were dominantly detected but at low frequency upon restimulation with live BK61 virus. These BK61-specific CD4 T cells are also able to simultaneously produce TNF-α and IL-2, showing characteristics of multifunctional T cells. However, BK61-specific CD4 T cells showed weak secondary response upon challenge with PRV DX strain. Further vaccination with PRV-infected dendritic cells (DCs) transiently increased the percentage of IFN-γ-positive CD4 and CD8 T cells but eventually restored to low frequency and did not improve the protective efficacy of BK61 against challenge, suggesting that PRV BK61 induced a relatively weak cellular immunity that could not be overcome by the DC vaccination. Similar immune responses were also observed following vaccination with another PRV LAV HD/c in mice and pigs, suggesting that this may be an intrinsic drawback of PRV LAVs in inducing cellular immunity. Our results demonstrated that PRV LAVs elicited a CD4 Th1-biased weak cellular immunity which is implicative for the development of PRV-vectored vaccine.
Introduction
Pseudorabies virus (PRV) is the causative agent of Aujeszky’s disease in swine, causing respiratory disease, abortion, and neurological disorders, and has substantial economic impact on the pig industry, especially in China (1) and even poses a threat to public health due to its transmission to human (2, 3). Since 2011, highly virulent PRV variants have emerged in Bartha K61-vaccinated swine farms in many regions of China (4, 5). The virus belongs to members of the genus Varicellovirus in alphaherpesvirus subfamily, along with several human pathogens such as herpes simplex virus 1 (HSV-1), HSV-2, and varicella-zoster virus (VZV) (6). In addition to the unique natural host of pigs, PRV are able to infect most of mammals, including rodents, dogs, cattle, and horses (7), exhibiting a marked neurotropism by invading the peripheral nervous system (PNS) and occasionally the central nervous system (CNS) of their host species, leading to a lethal and systemic inflammatory response in mice (8).
Aujeszky’s disease in pigs has been well controlled by the use of vaccination with inactivated and live attenuated vaccines (LAVs), especially, by those genetically engineered LAVs lacking virulence-determining gene, for instance, the Bartha K61 strain (7). As virus-vectored vaccines are generally believed to be superior to inactivated vaccines in eliciting both antibody responses and cellular immune responses (9). PRV has been developed into a useful vaccine vector for integrating and expressing foreign proteins for vaccine development (7, 10). By deleting virulence factors, for instance, glycoprotein E (gE), glycoprotein I (gI) and Thymidine kinase (TK), that are non-essential for viral replication and inserting foreign genes from different swine pathogens, recombinant PRV-vectored vaccines have been developed against Porcine circovirus type 2 (11–13), Classical swine fever virus (14), Porcine respiratory and reproductive virus (10), Swine influenza virus and African swine fever virus (15, 16). However, the immune properties of PRV as a viral vector have not been fully addressed, which may restrain its applications.
Both humoral and cellular immune responses have been implicated after PRV infection and/or vaccination (17–20). In detail, antibodies-mediated neutralization, antibody-dependent cell-mediated cytotoxicity, and complement-mediated lysis of PRV-infected target cells have been detected in PRV-infected or vaccinated pigs (18, 19). While neutralizing antibody-mediated protection against PRV was well recognized (7, 21), the cellular immune responses to PRV after infection or vaccination were not fully understood. Early studies showed that PRV-immune swine lymphocytes proliferate and secrete lymphokines upon in vitro restimulation with live or inactivated PRV (20, 22) and non-major histocompatibility complex (MHC)-restricted cytotoxicity against PRV-infected and uninfected cells has also been detected in pigs after infection or vaccination (19). PRV-specific lymphocytes or cytotoxic lymphocytes can be detected in the blood and draining lymph node after immunization (23, 24), which included IFN-γ-producing CD4+/CD6+/CD8+ memory T helper cells and CD4−/CD6+/CD8+bright MHC-restricted cytotoxic lymphocytes (22, 25). Further studies showed that gene-depleted PRV mutants were able to induce glycoprotein C-specific cytotoxic T lymphocytes (CTL) in DBA/2 mice and pigs (25, 26) and MHC II-restricted CD4+CD8+ memory T helper cells in miniature pigs that were able to help the secretion of PRV-specific immunoglobulins by PRV-primed B cells (27). However, vaccine-induced T cell-mediated immunity seemed to just play a role in early protection against PRV in pigs (28) and was unable to provide long-term protection by immunization once (29). By in vivo depleting CD4 and CD8 T cells as well as IFN-γ in mice, Bianchi et al. demonstrated that both IFN-γ and CD4 T cells play an important role in conferring protection against lethal PRV infection (30, 31), whereas CD8 T cells response was barely detected and appeared to be non-essential for immune protection (30). Although these studies demonstrated that cellular immunity was induced by different PRV LAVs, the phenotype, functional changes and the magnitude of distinct T cell subsets have not been revealed following PRV vaccination.
In this study, using PRV LAV Bartha-K61 and HD/c as a model, we further characterized the cellular immune response in mice and pigs after immunization. We found that phenotypic activation of T cells, NK cells and B cells was hardly detected after vaccination, but antigen-specific cytokine-producing CD4 T cells were dominantly detected at low frequency. However, PRV-specific CD4 T cells have weak memory response upon challenge and the weak cellular immunity elicited by BK61 could not be enhanced by dendritic cell vaccination, suggesting that there are intrinsic drawbacks and common characteristics for PRV LAVs in inducing Th1-biased immune response.
Materials and methods
Ethics statement
This study was carried out in accordance with the recommendations in the Guide for the Care and Use of Laboratory Animals of Zhejiang University. All animal experiments were approved by the Animal Welfare and Ethics Committee at the College of animal science of Zhejiang University with reference number ZJU20230315.
Animal, cell lines and virus strain
Six to eight-week-old wild-type C57BL/6 mice were purchased from the Center of Comparative Medicine at Yangzhou University. Three to four-week-old healthy piglets were purchased from Kairong biotechnology company (Suzhou, China). All pigs were detected negative for antibodies against PRV-gB and gE antigen, PRRSV, ASFV and CSFV and nucleic acid of PRV, PRRSV, PCV2, CSFV and ASFV. Human embryonic kidney cells (HEK293T) and PCV-free PK-15 cells (ATCC-CCL-33) were cultured in Dulbecco’s modified Eagle’s medium (DMEM; Gibco, America) supplemented with 10% heat-inactivated fetal bovine serum (FBS; Gibco, America). Classical PRV vaccine strain Bartha-K61 (GenBank accession no. JF797217.1) was gifted by Prof. Song Gao at Yangzhou University. Virulent PRV type II strain DX and an attenuated PRV vaccine strain HD/c (gE/TK deletion) was kept in our laboratory (21). All the viruses were propagated in PK-15 cells, titrated, aliquoted in stocks and stored in -80°C in our lab.
Animal experiments
A total of ninety female C57BL/6 mice were divided into two groups, with 45 mice in each group. One group was intramuscularly and subcutaneously immunized with 1×105 TCID50 PRV Bartha K61 in 100μl DMEM (BK61 imm). This dose of Bartha K61 was relatively unlethal to C57BL/6 mice. Another group was mock immunized with 100μl phosphate-buffered saline (PBS). At indicated time-points after immunization, five mice from each group (n=25) were sacrificed, spleens and lymph nodes were harvested for single cell isolation and quantification of viral loads, respectively. Serum samples were collected by tail vein at days 7, 14, and 28 post immunization to determine the antibody titers. At day 30 after immunization, all the remaining mice from both groups (n=20 per group) were challenged with 5×103 TCID50 virulent PRV DX strain. At indicated time-points after challenge, mice from each group (n=4-5) were sacrificed, spleens were harvested for single cell preparation and viral load quantification again. Five mice from each group were spared for recording the survival rate after challenge.
For pig study, ten piglets were divided into two groups. One group (n=5) was immunized intramuscularly at the neck with 2 ml of 107.5 TCID50/ml PRV HD/c. The other group was immunized with equal volume of DMEM medium. Periphery blood from each pig was collected at 7, 14 and 21 days after immunization. The isolation of PBMCs and intracellular straining of cytokines in T cells were performed and analyzed by flow cytometry as our previous studies (32, 33).
Primary cell preparation
Single-cell suspensions from spleen and lymph nodes were prepared as previously described (33, 34). Briefly, tissues were mechanically disrupted and pushed through a 70 μm nylon cell strainer and the cells were resuspended in 10 ml PBS containing 2% fetal bovine serum (FBS). Red blood cells were removed from single cell suspensions by hypotonic lysis with Gey’s solution. Cells were counted using a hemocytometer and the final cell concentration was adjusted to 2 × 107 cells/mL before cell surface staining.
Dendritic cell differentiation and immunization
Bone marrow-derived dendritic cells (BMDCs) were derived from mouse bone marrow progenitors using GM-CSF and IL-4 (PeproTech, Rocky Hill, NJ) at a final concentration of 10 ng/mL and 2 ng/mL, respectively, as previously described (35). At day 6 of culture, BMDCs were harvested and pulsed with inactivated PRV Bartha K61 (iBK61) or infected with live Bartha K61 (BK61) at a multiplicity of infection (MOI) of 3 for 18 h or indicated length of time. iBK61- or BK61-pulsed BMDCs were washed twice and used as stimulators to activate T cells isolated from PRV-immunized and unimmunized mice.
For DC immunization, BMDCs harvested at day 6 of culture were plated in six-well plate with 6.1×106 cells per well, live PRV Bartha K61 was added up to 1×106 TCID50 per mL (MOI=0.5) and infected for overnight, then BK61-infected BMDCs were harvested and washed twice, and a number of 2.17×106 cells was sampled and lysed to confirm the infection rate by IFA on PK-15 cells. 5×105 BK61-infected BMDCs (around 1×104.6 TCID50 PRV) were used to immunize mice. A total of eighty female C57BL/6 mice were divided into four groups (DMEM, BK61, DC alone and BK61-DCs, respectively), with 20 mice in each group. Group 1 and 2 were intramuscularly immunized with DMEM and 1×105 TCID50 PRV Bartha K61 each mouse (BK61), respectively. Group 3 was intraperitoneally immunized with 5×105 uninfected BMDCs each mouse (DC alone). Group 4 was intraperitoneally immunized with 5×105 BK61-infected BMDCs each mouse containing around 1×104.6 TCID50 PRV Bartha K61 (BK61-DCs). At indicated time-points after immunization, mice were sacrificed, spleens were harvested for single cell isolation. At day 30 after immunization, five mice from each group were challenged and the survival rate of each group was recorded.
Immunophenotypic analysis by flow cytometry
Anti-mouse monoclonal antibodies (mAb) against CD8α (53–6.7), CD44 (1M7), CD62L (MEL14), CD127 (A7R34), KLRG1 (2F1/KLRG1), B220 (RA36B2), CD4 (GK 1.5), TCRβ (H57-597), NK1.1 (PK136), TCRγδ (GL-3) and CD69 (H1.2F3) with different fluorochrome conjugate, were purchased either from BioLegend or BD Bioscience (San Diego, CA). Surface marker staining of cells was carried out as our previous reports (36). For detecting the early activation of distinct immune cells after immunization, 2×106 cells were plated into 96-well V-bottom plates and incubated with 2.4G2 Fcγ RII/RIII blocking mAb for 15 min, then stained for 30 min on ice with 50 μL antibody cocktail containing fluorochrome-conjugated mAbs against CD69, CD8α B220, CD4, TCRβ, NK1.1 and TCRγδ. Afterward, cells were washed and resuspended in 200 μL PBS for flow cytometric analysis.
For defining memory T cells, 2×106 splenocytes were plated and stained for 30 min on ice with 50 μL antibody cocktail containing different fluorochrome-labeled mAbs against markers TCRβ, CD8α, CD44, CD62L, CD127, KLRG1 and CD4. Thereafter, cells were washed and resuspended in 200 μL PBS for flow cytometric analysis. Flow cytometry was performed with a FACS CantoII (BD Biosciences, San Jose, CA) and analyzed using FlowJo software (Tree Star, Ashland, OR).
Intracellular cytokine staining
2×106 cells were plated into 96 round-bottom-well plates and stimulated with or without live BK61 at MOI of 1 or inactivated BK61-pulsed DCs for 18h. Brefeldin A (Sigma, St. Louis, MO) was added for the last 6 h of co-culture at a final concentration of 10 μg/ml. After incubation, cells were harvested and stained for cell surface makers TCRβ, CD8α, and CD4 as above-mentioned protocol, and then fixed with 4% paraformaldehyde for 10 min and permeabilized twice by Fixation/Permeabilization solution (BD Biosciences) following the manufacturer’s protocol. Subsequently, cells were stained with APC-conjugated anti-IFN-γ (XMG1.2, Biolegend), FITC-conjugated anti-TNF-α (MP6-XT22, Biolegend) and PE-conjugated anti-IL-2 mAbs (JES6-5H4, Biolegend). Flow cytometry was performed as described above.
Statistical analysis
Statistical analyses were performed using Prism software 5.0 (GraphPad, La Jolla, CA). When comparing experimental values from two groups of mice, two-tailed student’s t-tests were used. When comparing experimental values from multiple groups, one-way ANOVA Bonferroni post-tests were used. Statistically significant differences are noted (****P < 0.0001; ***P < 0.001; **P < 0.01; *P < 0.05).
Results
Vaccination with PRV Bartha K61 induced no detectable phenotypic activation of NK cells, B cells and T cells
Attenuated live vaccines was shown to elicit potent cellular immune responses in mice or human after immunization that significant increase of CD44hiCD62L-, CD44hiCD62L+ or IFN-γ-producing T cells was observed (9, 37–39). Therefore, we anticipated that vigorous activation of distinct lymphocytes should occur after PRV LAV vaccination. Thus, we detected the expression of CD69 and CD44 on lymphocyte subsets to evaluate the very early activation and antigen-experienced effector and memory T cells in mice after immunization (40, 41). Surprisingly, vaccination with PRV Bartha K61 (BK61) did not induce the upregulation of CD69 on NK cells, B cells, γδ T cells, and CD4 and CD8 T cells at day 4, 7 and 14 after immunization (dpi) (Figures 1A, B). Instead, PRV BK61 immunization even led to the reduction of CD69-positive CD4 and CD8 T cells at 4 dpi (Figure 1B). To further confirm if there is late activation of T cells or memory T cell development, we detected the CD44 expression on T cells. As shown in Figures 1C, D, PRV BK61 immunization did not increase the percentage of CD44hi-postive effector and memory CD4 and CD8 T cells at any time-point after vaccination, compared to the un-immunized mice. These results suggested that PRV BK61 induced no detectable phenotypic activation of NK cells, B cells and T cells in mice.
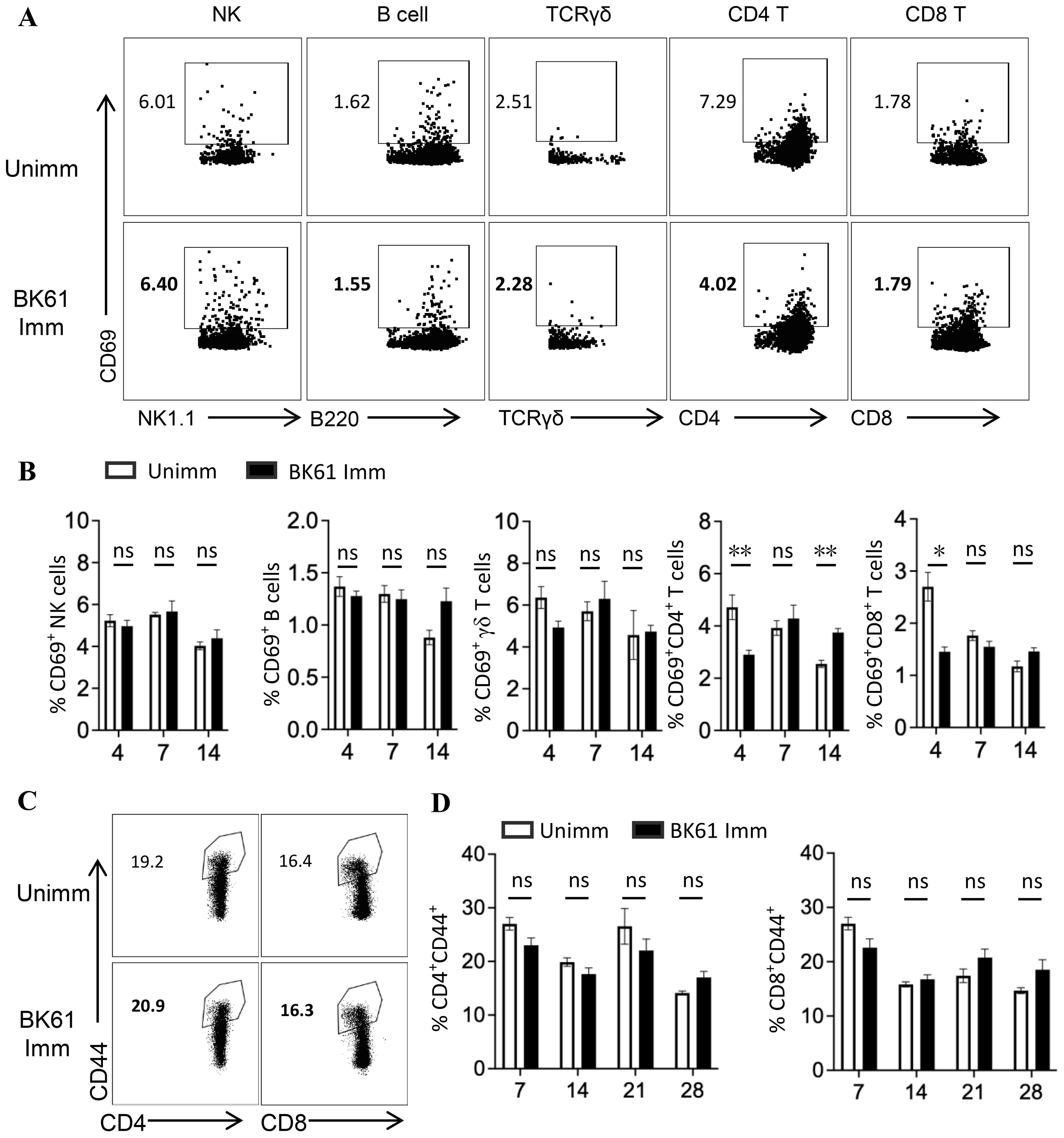
Figure 1. Vaccination with PRV Bartha K61 induce no detectable phenotypic activation of NK cells, B cells and T cells. C57BL/6 mice were immunized with or without PRV Bartha K61 (BK61) at a titer of 105 TCID50 and euthanized at indicated time-points, splenocytes were prepared for phenotypic analysis by flow cytometry. (A) Representative dot-plots depict CD69 expression on NK cell, B cell, γδ T cell, CD4 and CD8 T cells from unimmunized (upper row) and immunized mice (lower row) at 7 dpi. Numbers indicate the percentages of CD69-positive cells. (B) Kinetic changes of the percentages of CD69-positive NK cells, B cells, γδ T cells, CD4 and CD8 T cells in the spleen of unimmunized and BK61-immunized mice. (C) Representative dot-plots of CD44hi CD4 and CD8 T cells from unimmunized and immunized mice at 14 dpi. Numbers indicate the percentages of CD44hi-positive T cells. (D) Kinetic changes of the percentages of CD44hi CD4 and CD8 T cells in the spleen of unimmunized and BK61-immunized mice. Data shown are mean ± SD from four mice per group. ns, no statistical significance; *P < 0.05, **P < 0.01.
PRV BK61 immunization induced antigen-specific CD4 and CD8 T cells at low frequency
To further determine whether PRV BK61 vaccination induce PRV-specific T cells response, we examined the frequency of IFN-γ-producing T cells upon ex vivo restimulation of the BK61-primed T cells with live BK61 through intracellular cytokine staining (ICS). As shown in Figures 2A, B, we detected more IFN-γ-producing CD4 and CD8 T cells in the BK61-immunized mice, compared to the unimmunized control, but the frequencies of both IFN-γ+CD4+ and IFN-γ+CD8+ T cells were very low (less than 0.5%). Further analysis of the kinetics of PRV-specific T cells showed that both IFN-γ+CD4+ and IFN-γ+CD8+ T cells peaked at 14 dpi in terms of percentage but the magnitude of IFN-γ+CD4+ T cells was higher than that of IFN-γ+CD8+ T cells and PRV-specific CD4 T cells were detected earlier (4 dpi) than PRV-specific CD8 T cells (Figure 2B). These results indicated that PRV BK61 induced a dominant CD4 Th1 response in mice with low magnitude.
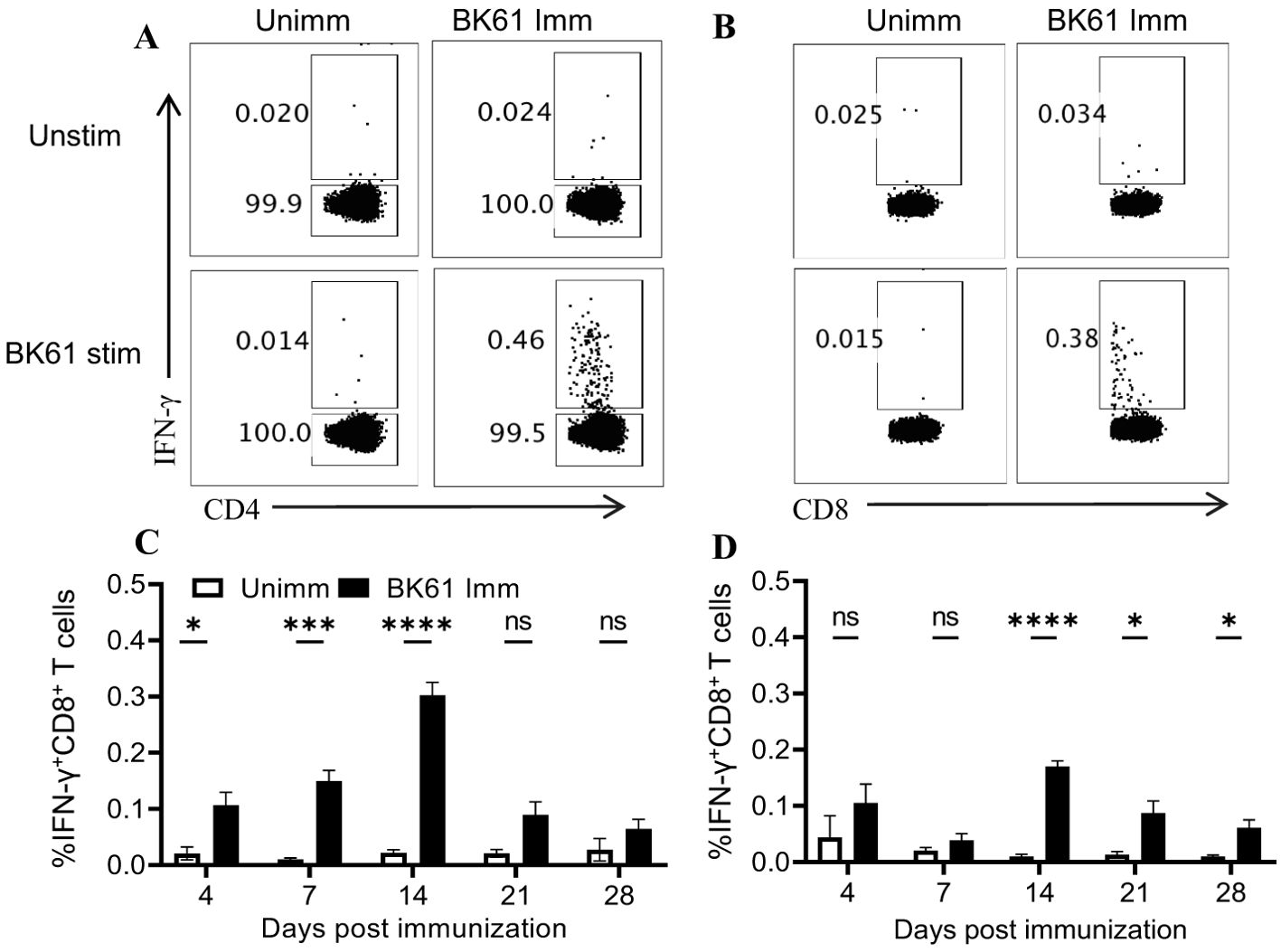
Figure 2. PRV BK61 immunization induce antigen-specific CD4 and CD8 T cells at low frequency. Splenocytes were prepared from PRV BK61-immunized and unimmunized C57BL/6 mice at indicated time-points after immunization and restimulated with or without live BK61 for 18 hours and followed by intracellular staining of IFN-γ and analyzed by flow cytometry. (A, B) Representative dot-plots of IFN-γ-producing CD4 and CD8 T cells from unimmunized (left) and immunized mice (right) at 14 dpi, respectively. Numbers indicate the percentages of IFN-γ-positive T cells. (C, D) Kinetic changes of the percentages of IFN-γ-positive CD4 and CD8 T cells in the spleen of unimmunized and BK61-immunized mice at 4, 7, 14, 21 and 28 dpi. Data shown are mean ± SD from four mice per group. ns, no statistical significance; *P < 0.05, ***P < 0.001, ****P < 0.0001.
PRV BK61-primed CD4 but not CD8 T cells exhibit characteristics of multi-functional T cells
Among activated T cells, polyfunctional T cells that are able to simultaneously produce multiple cytokines (IFN-γ, TNF-α and IL-2) have been shown to correlate with protective immunity, and are equivalent to central memory T cells (42, 43). We further analyzed the functionalities of those PRV-specific T cells by ICS of multiple cytokines though their frequency was low. As shown in Figures 3A, B, compared to the unimmunized mice, BK61-immunized mice have more PRV-specific IFN-γ-producing CD4 T cells, which also simultaneously co-expressed TNF-α (IFN-γ+TNF-α+) or IL-2 (IFN-γ+IL-2+) or TNF-α and IL-2 (IFN-γ+TNF-α+IL-2+), with higher proportion in IFN-γ single positive (61.2%) and IFN-γ+TNF-α+IL-2+ CD4 T cells (19.8%) (Figures 3A, B). In addition, there were also slightly more IFN-γ-negative TNF-α+, IL-2+ and TNF-α+IL-2+ CD4 T cells in BK61-immunized mice (Figure 3A). These results demonstrated that BK61 immunization induced multi-functional CD4 T cells. Further analysis of the kinetics of PRV-specific CD4 T cells showed that all three subsets of double-cytokine-producing CD4 T cells peaked at 14 dpi and then decreased by 28 dpi (Figure 3C). In contrast, very few of IFN-γ+TNF-α+CD8 T cells were induced in BK61-immunized mice. These cells showed similar kinetics to that of cytokine-producing CD4 T cells but no triple cytokine-producing CD8 T cells were detected after immunization (Figures 3D, E). These results showed that PRV BK61-primed CD4 but not CD8 T cells showed characteristics of multi-functional T cells.
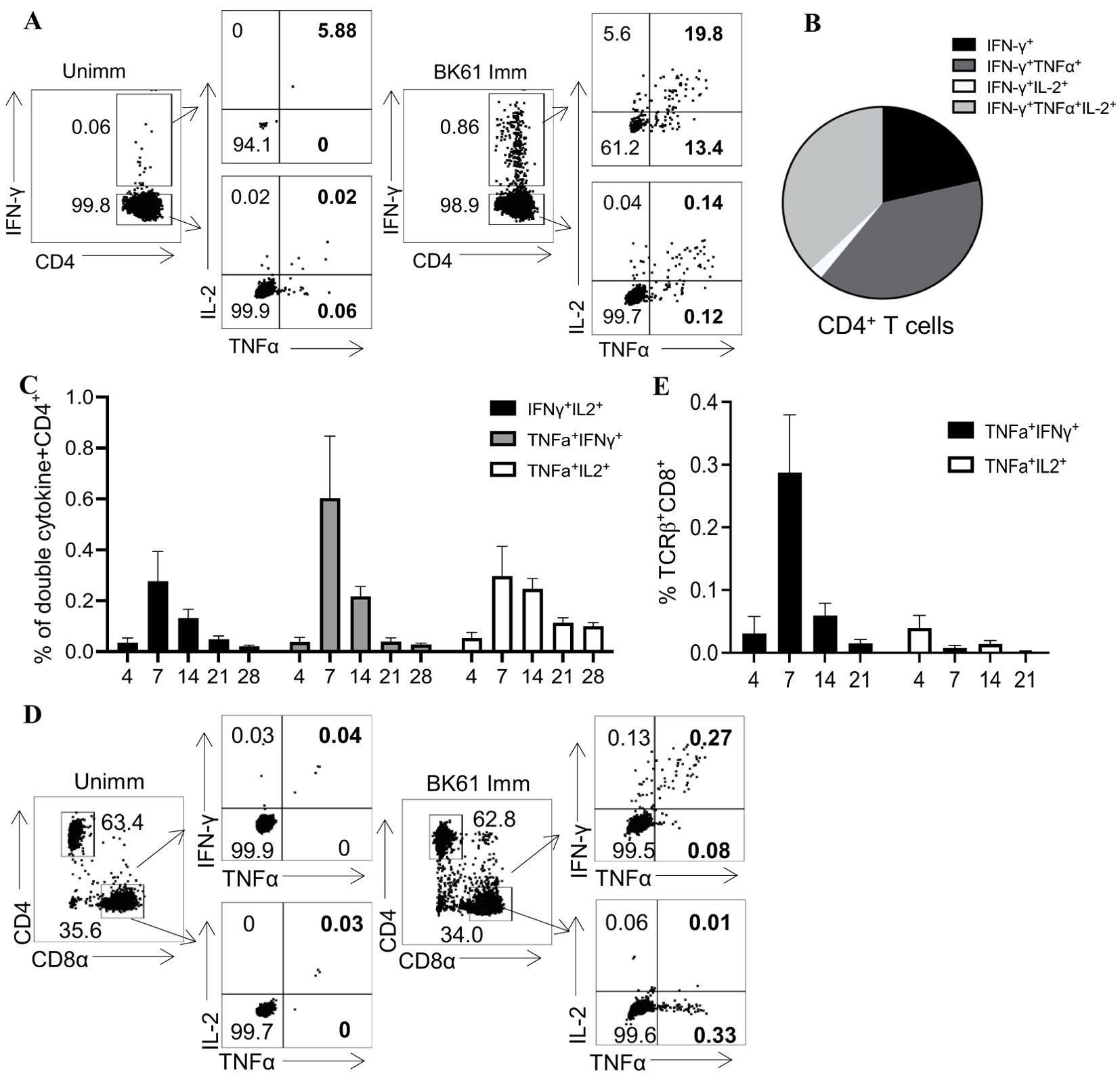
Figure 3. PRV BK61-primed CD4 but not CD8 T cells exhibit characteristics of multi-functional T cells. Splenocytes from unimmunized and BK61-immunized C57BL/6 mice were stimulated ex vivo for 18h with live BK61 at MOI of 1 at indicated time-points after immunization and then harvested for intracellular staining of IFN-γ, TNF-α and IL-2. (A) Representative dot-plots of multiple cytokine-producing CD4 T cells in the spleens of unimmunized and immunized mice at 14 dpi. (B) Pie graphs show relative proportion of TNF-α+, IL-2+ and TNF-α+IL-2+ CD4+ T cells among IFN-γ+CD4+ T cells from the spleens of immunized mice at 14 dpi. (C) The changes of percentage of PRV-specific double-cytokine (IFN-γ+TNF-α+, IFN-γ+IL-2+ and TNF-α+IL-2+)-producing CD4+ T cells detected in BK61-immunized mice at different time-points after immunization. (D) Representative dot-plots of double cytokine (IFN-γ+TNF-α+, TNF-α+IL-2+)-producing CD8 T cells in the unimmunized and immunized mice at 14 dpi. (E) The changes of percentage of PRV-specific double-cytokine (IFN-γ+TNF-α+ and TNF-α+IL-2+)-producing CD8 T cells detected in BK61-immunized mice after immunization. Data shown are mean ± SD from four mice per group.
PRV-specific CD4 T cells showed weak memory response upon challenge with PRV DX strain
It has been reported that PRV vaccine-induced T-cell immunity played a critical role in early protection against PRV in pigs and repeated vaccination are required clinically for long-term protection (28, 29), suggesting that PRV attenuated vaccine may induce weak memory T cells response. Therefore, we examined the secondary response of PRV-specific T cells after challenge with PRV DX strain. Both BK61-immunized and unimmunized mice were challenged at 28 dpi or left unchallenged and IFN-γ- and TNF-α-producing T cells were detected at 7 dpc upon restimulation with BK61. As shown in Figure 4, when comparing the percentage and kinetic change of total IFN-γ-producing CD4 T cells, there was no significant difference between the immunized and unimmunized mice after DX challenge (Figures 4A–C). However, when comparing the percentage of IFN-γ+TNF-α+ CD4 T cells, BK61-immunized mice have more IFN-γ+TNF-α+ CD4 T cells than the unimmunized mice at 4 and 7 days after DX challenge (Figures 4D–F), which were detected as early as 4 dpc (Figure 4F), suggesting BK61 immunization had induced antigen-specific memory CD4 T cells, thus have rapid secondary response after challenge. Similarly, we also analyzed BK61-induced memory CD8 T cells by comparing the percentage of IFN-γ+TNF-α+ CD8 T cells between the two groups after challenge. We found no significant increase in secondary CD8 T cells response, compared to primary CD8 T cells response in terms of percentage (Figures 4F–H). Of note, the percentages of both PRV-specific secondary CD4 and CD8 T cells were very low though BK61-primed T cells were pre-existed before challenge. These results indicated that BK61 immunization mainly induced a weak memory response of CD4 T cells.
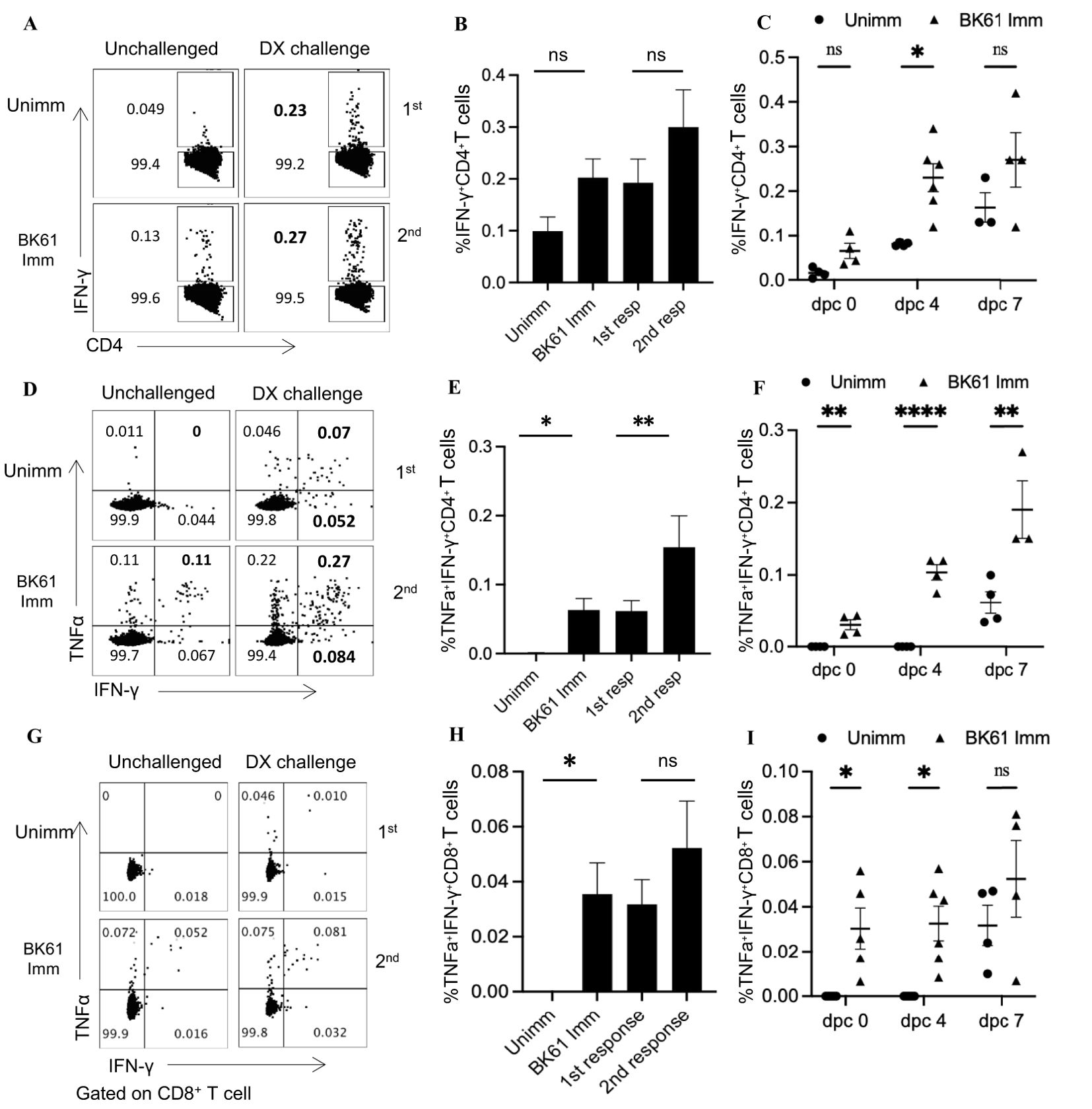
Figure 4. PRV-specific CD4 T cells showed weak memory response upon challenge with PRV DX strain. C57BL/6 mice were immunized with or without PRV Bartha K61 and rested for 28 days, then the immunized and age-matched naïve mice were challenged with or without PRV DX strain. The primary and secondary response of IFN-γ- or IFN-γ and TNF-α-producing CD4 and CD8 T cells in the naïve and prior immunized mice were compared by flow cytometry. (A) Representative dot-plots of IFN-γ-positive CD4 T cells in the spleens of indicated mice challenged with or without DX strain. Numbers indicate the percentages of IFN-γ+CD4+ T cells. (B) Bar graph shows the percentages of IFN-γ+CD4+ T cells in the spleens of indicated mice at 7 days post-challenge (dpc). (C) The kinetic changes of the percentages of primary and secondary IFN-γ+CD4+ T cells from the naïve and previously immunized mice at 0, 4 and 7 dpc. (D) Representative dot-plots of IFN-γ+TNF-α+CD4 T cells in the spleens of indicated mice challenged with or without DX strain. (E) The comparison of the percentages of IFN-γ+TNF-α+CD4 T cells in the spleens of indicated mice at 7 dpc. (F) The kinetic changes of the percentages of primary and secondary IFN-γ+TNF-α+CD4 T cells from the naïve and previously immunized mice at 0, 4 and 7 dpc. (G) Representative dot-plots depict IFN-γ+TNF-α+CD8 T cells in the spleens of indicated mice challenged with or without DX strain. (H) The comparison of the percentages of IFN-γ+TNF-α+CD8 T cells in the spleens of indicated mice at 7 dpc. (I) The kinetic changes of the percentages of primary and secondary IFN-γ+TNF-α+CD8 T cells from the naïve and previously immunized mice at 0, 4 and 7 dpc. Data shown are mean ± SD from four mice per group. ns, no statistical difference, *P < 0.05, **P < 0.01, ****P < 0.0001.
Vaccination with BK61-infected dendritic cells transiently enhanced PRV-specific T cell response but not protective efficacy
Previous studies showed that antigen-specific CD4 and CD8 T cells response can be rapidly induced and potently enhanced by dendritic cell (DC) immunization (33, 44). To further demonstrate whether the weak T cells response induced by BK61 is an intrinsic property of PRV BK61. We employed DC immunization to enhance BK61-induced T cells response. BMDCs were pulsed with live or inactivated PRV BK61 at MOI of 0.5 (DC-BK61 or DC-iBK61) and then used to immunize mice. PRV-specific CD4 and CD8 T cells were examined and compared after immunization as aforementioned protocol. As shown in Figures 5A, B, PRV-specific IFN-γ-producing CD4 and CD8 T cells response were significantly enhanced at 7 dpi by DC-BK61 immunization as DC-BK61-immunized mice have the highest percentage (1%) of IFN-γ-producing CD4 and CD8 T cells, compared to the other three groups (DMEM, DC alone and BK61). However, by 14 dpi, this difference was no longer observed between BK61 and DC-BK61 group (Figures 5C, D). To further test whether DC-BK61-enhanced T cell response was translated into better protection against PRV DX challenge. The survival rates of each group were compared after challenge. As a result, DC vaccination with BK61 or inactivated BK61 did not provide better protection than BK61 immunization (Figure 5F). These results indicated that although the weak T cells response induced by BK61 were transiently enhanced by dendritic cell vaccination, the eventual protective immunity was not increased, suggesting that there is an intrinsic drawback for PRV BK61 in inducing cellular immunity to some extent.
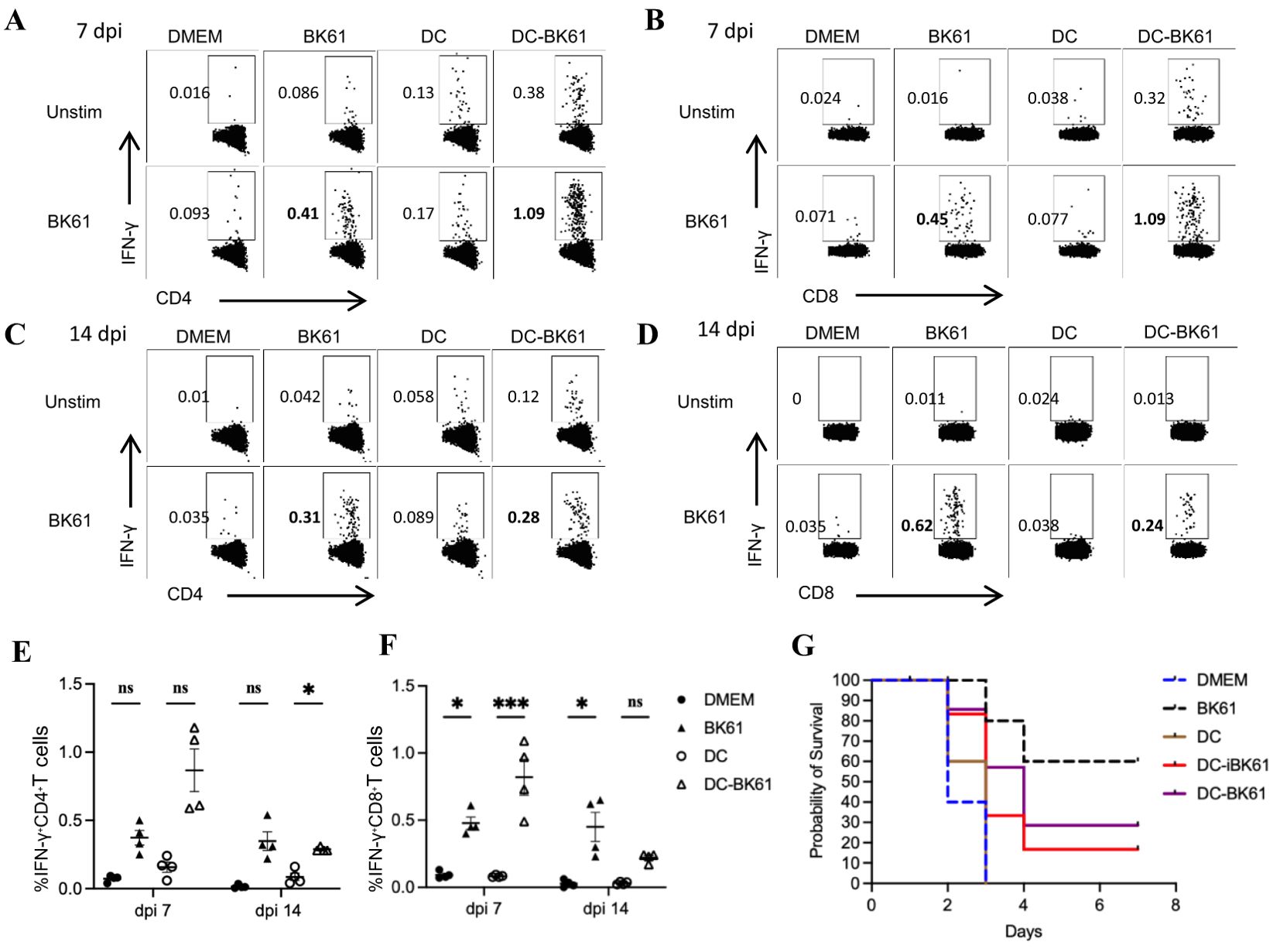
Figure 5. Immunization with BK61-infected dendritic cells transiently enhanced PRV-specific T cell response but not protective efficacy against PRV DX challenge. C57BL/6 mice were immunized with DMEM, PRV Bartha K61, bone marrow-derived dendritic cells (DCs) and BK61-pulsed DCs, respectively, and euthanized at 7 and 14 days after immunization or challenged with PRV DX strain at 28 dpi. Splenocytes from each group were prepared at indicated time-points and restimulated with or without live BK61 for 18 hours and followed by intracellular staining of IFN-γ and analyzed by flow cytometry. Survival rates of each group were recorded after PRV DX challenge. (A–D) Representative dot-plots of PRV-specific IFN-γ-producing CD4 (A, C) and CD8 T cells (B, C) in the spleens of indicated mice at 7 (A, B) and 14 days (C, D) after vaccination. (E, F) The kinetic changes of the percentages of IFN-γ-producing CD4 (E) and CD8 (F) T cells from the spleens of mock-, BK61-, DC only- and DC-BK61-immunized mice at 7 and 14 dpi. (G) The survival curves of mock-, BK61-, DC only- and DC-BK61-immunized mice after PRV DX challenge. Data shown are mean ± SD from four mice per group. ns, no statistical difference, *P < 0.05, ***P < 0.01.
The weak Th1-biased immunity may be an intrinsic property of PRV LAVs
PRV BK61 strain originally contains deletions of the US2, gE, gI, and US9 genes. To test whether other gene-deleted attenuated PRV strain also induce similar T cell responses in mice, we examined PRV-specific IFN-γ-producing T cells elicited by PRV HD/c strain, a gE/TK-deleted PRV variant strain constructed in our lab (21). As shown in Figure 6, PRV HD/c immunization induced similar kinetics and magnitude of IFN-γ-producing CD4 and CD8 T cells as well as IFN-γ+TNF-α+IL-2+CD4 T cells before 14 dpi and more IFN-γ+CD4 T cells from 21 to 35 dpi, compared to the BK61 immunization, suggesting that the magnitude of T cell response (less than 0.5%) may be determined by the PRV LAVs itself but not by the difference of gene deletions in the LAVs. To further confirm whether attenuated PRV vaccine induce similar magnitude of T cells responses in pigs as well, we used HD/c to immunize piglets and examined the kinetics and magnitude of IFN-γ-expressing T cells in PBMCs of the immunized pigs. As shown in Figure 7, HD/c immunization barely induced phenotypic activation (CD69+) of CD4 T cells at 21 dpi and CD8 T cells at 7 dpi with the percentages less than 0.6% in the PBMCs (Figures 7A, B). PRV-specific IFN-γ+ or IFN-γ+TNF-α+ CD4 T cells and CD8 T cells were significantly elicited in the PBMCs of pigs after PRV HD/c immunization but with low frequency (less than 0.6%) as well (Figures 7C–F). No PRV-specific triple cytokine-producing T cells were detected in the immunized pigs (data not shown). These results demonstrated that attenuated PRV vaccine strain also induced weak Th1 immunity in pigs, similar to that observed in mice, which may be an intrinsic property of PRV LAVs.
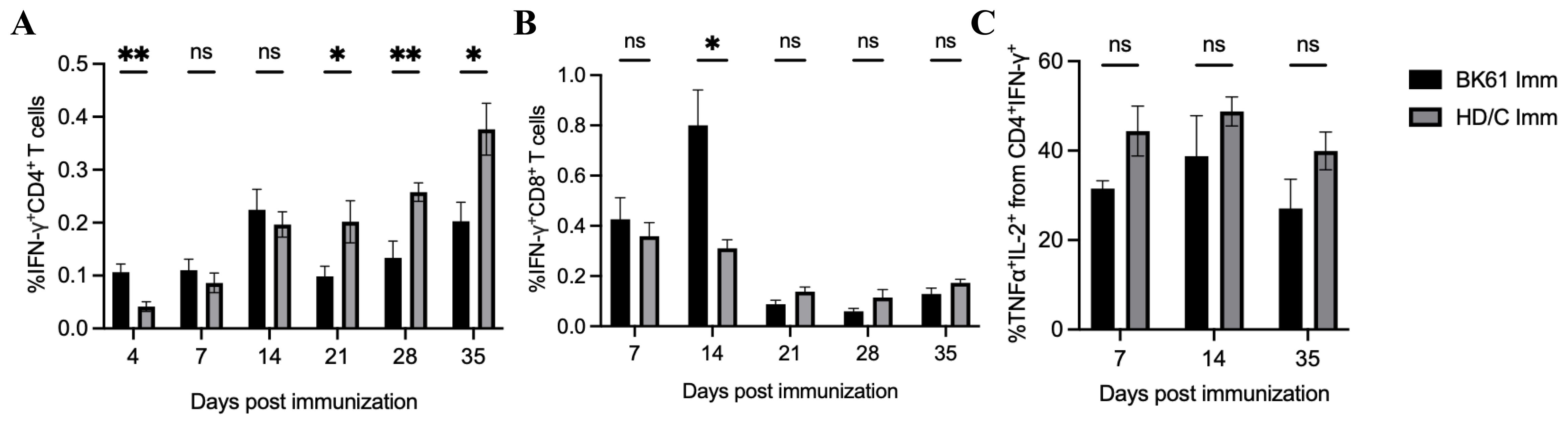
Figure 6. Low magnitude of antigen-specific CD4 and CD8 T cells were induced by another PRV attenuated strain HD/c in mice. C57BL/6 mice were immunized with PRV Bartha K61 and HD/c, respectively. Splenocytes from both groups were prepared at indicated time-points after immunization and restimulated with or without live BK61 or HD/c strain, respectively, for 18 hours and followed by intracellular staining of IFN-γ and compared by flow cytometry. The percentages of IFN-γ+CD4 T cells (A), IFN-γ+TNF-α+IL-2+CD4 T cells (B) and IFN-γ+CD8 T cells (C) from the spleens of BK61- and HD/c-immunized mice (n=8) were compared at indicated time-points after immunization. Data shown are mean ± SEM pooled from eight mice from two independent experiments. ns, no statistical difference, *P < 0.05, **P < 0.01.
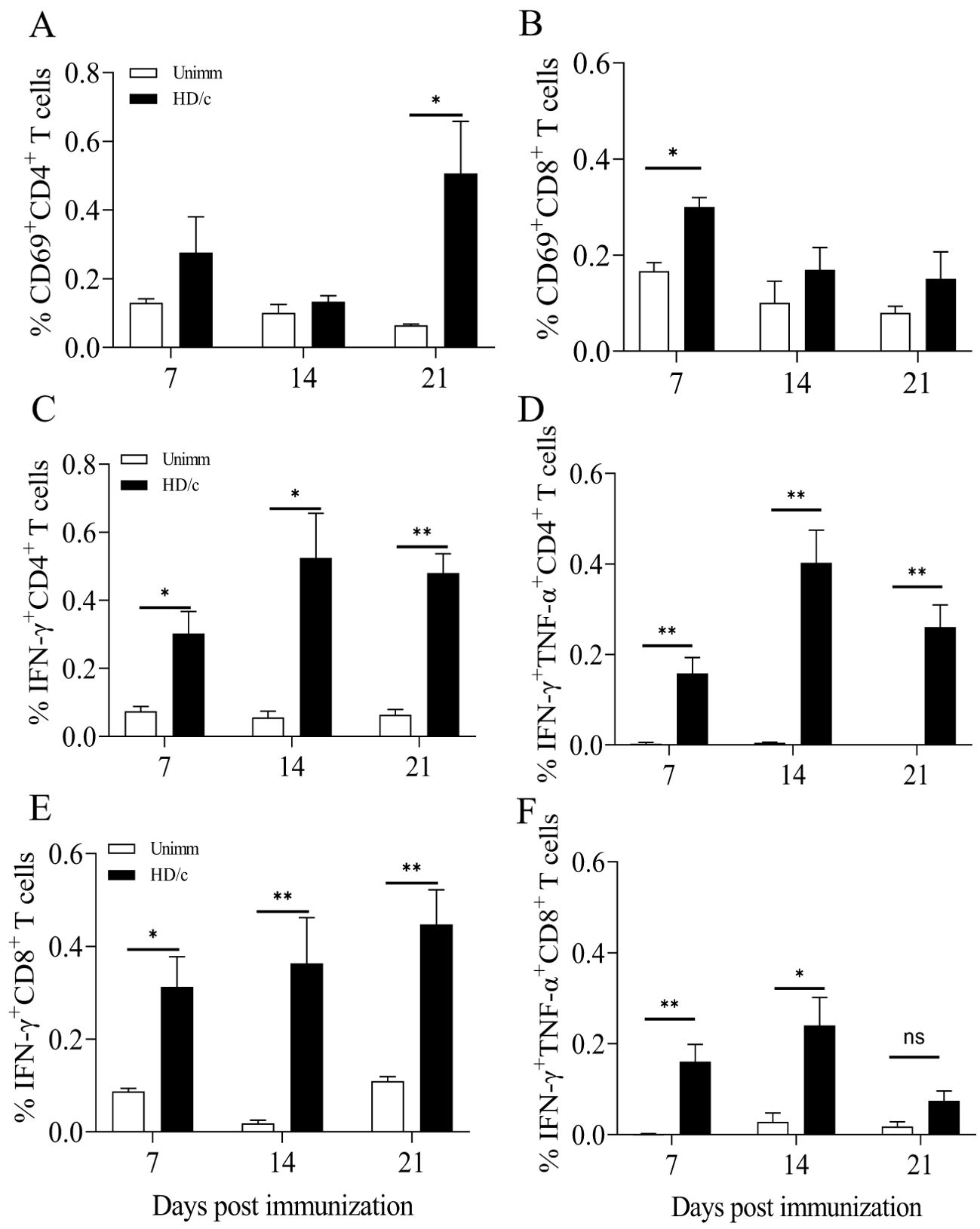
Figure 7. The attenuated PRV vaccine also induced a weak Th1-biased immunity in pigs. Six-week-old piglets were immunized with or without PRV HD/c and PBMCs from the unimmunized and immunized pigs were isolated at indicated time-points after immunization. The expression of CD69 and IFN-γ and/or TNF-α by CD4 and CD8 T cells were detected by flow cytometry. (A, B) Representative dot-plots depict CD69 expression on CD4 (A) and CD8 (B) T cells from the unimmunized and immunized pigs at 7, 14 and 21 dpi. Numbers indicate the percentages of CD69-positive cells. (C, D) The kinetic changes of the percentages of IFN-γ-positive (C) or IFN-γ and TNF-α double-producing CD4 T cells (D) in the PBMCs of the unimmunized and immunized pigs upon ex vivo restimulation with live HD/c at 7, 14, 21 dpi. (E, F) The kinetic changes of the percentages of IFN-γ-positive (E) or IFN-γ and TNF-α double-producing CD8 T cells (F) in the PBMCs of the unimmunized and immunized pigs upon ex vivo restimulation with live HD/c at 7, 14, 21 dpi. Data shown are mean ± SD from four pigs per group. ns, no statistical significance; *P < 0.05, **P < 0.01.
Discussion
Aujeszky’s disease caused by PRV has substantial impact on the pig industry in China (1). Although this disease has been controlled by the use of vaccination with PRV LAVs, especially the Bartha K61 (BK61) strain (7), the magnitude and kinetics of PRV BK61-induced cellular immunity has not been fully examined. In this study, through a comprehensive analysis of the phenotypic and functional changes and the magnitude of PRV-specific T cell response in vivo after vaccination, we found that PRV BK61 induced a weak cellular immunity in mice and pigs, phenotypically manifested by undetectable T cell activation and expansions in terms of CD69 and CD44 expression, low frequency of PRV-specific CD4 T cells that can be temporarily enhanced by a strategy of DC immunization, suggesting that this might be the intrinsic property or drawbacks of PRV LAVs.
Modified live virus vaccines are generally believed to elicit potent cellular immune responses and antibody responses (9, 37–39). For instance, influenza LAV (DelNS1-LAIV) and a mouse coronavirus LAV (N7-MTase-mutated MHV) induced significant increase of effector and memory T cells after challenge (37) and about 2% IFN-γ-producing T cells in mice (38). It was surprisingly unexpected that PRV BK61 induced such a weak cellular immunity in this study. Initially, we directly compared the change of T cell frequency before and after BK61 immunization, but no difference was observed. Therefore, we chose to detect the very early activation marker CD69 (40) and antigen-experienced T cells marker CD44 (41), but still no apparent activation of T cells was observed (Figure 1). Lastly, we detected PRV-specific IFN-γ-producing T cells by in vitro stimulation with live BK61. Indeed, we successfully detected PRV-specific CD4 and CD8 T cells, but their percentages are unexpectedly low as well (less than 0.5%) (Figure 2). Live BK61 was supposed to encode many antigens that were presented to all BK61-primed T cells to stimulate them producing cytokines during in vitro co-culture. To increase the detection rate of IFN-γ-producing T cells, we had tried to use BK61-pulsed BMDCs as stimuli for intracellular cytokine staining assay because antigen-pulsed BMDCs have been shown to be able to stimulate the primed T cells to produce more IFN-γ (36). However, we still cannot detect higher percentage of PRV-specific IFN-γ-producing T cells (data no shown). These results indicate that PRV BK61 immunization truly induce a low magnitude of PRV-specific T cells response. In addition, when comparing the percentage of PRV-specific IFN-γ+CD4+ T cells, no memory response was detected after challenge (Figures 4A–C), only a small portion of IFN-γ+TNF-α+ CD4 T cells showed significant secondary response after challenge (Figures 4D–F), suggesting that PRV BK61-primed T cells truly have a weak memory response. Of note, PRV HD/c also induced a low magnitude of PRV-specific T cells response as did BK61 (Figure 6) even though PRV HD/c was inoculated at a high dose compared to BK61 (107.25 VS 105.0 TCID50). All these results implied that PRV BK61-induced weak Th1-biased immunity is likely an intrinsic property of PRV LAVs.
DC immunization has shown great potency in enhancing CD4 and CD8 T cells response and immune protection (33, 44). In this study, BK61-BMDC vaccination indeed enhanced BK61-specific IFN-γ-producing CD4 and CD8 T cells at 7 dpi but did not improve the protective efficacy after DX challenge (Figure 5). The reason behind this might be that the cellular immunity elicited by BK61-DC immunization was not strong enough or long-lasting. Attenuated PRV BK61 was shown to be neurotropic in mice and can transmit to the PNS via sensory and motor neuronal pathways (45). It was reported that PRV virion captured cellular kinesin motor in epithelial cells for its subsequent invasion of neuronal cells (46). If so, once DC-carrying PRV BK61 gets into the body, the virus may quickly and eventually infect neuronal cells, leading to insufficient antigen presentation by APCs to prime T cells. This may also explain why attenuated PRV induces such a weak cellular immune response. But further investigation is needed.
Although PRV-specific IFN-γ-producing memory CD4 T helper cells and CD8 CTL had been detected in DBA/2 mice and pigs in previous studies (22, 25–27), most of those findings were from in vitro long-term culture and enrichment of lymphocytes upon restimulation with BK61 or lymphoproliferative assay. The kinetic changes and magnitude of PRV-specific T cells were lacking after immunization. In this study, through multicolor flow cytometry, we demonstrated that PRV BK61 mainly induced a CD4 Th1-biased response in both mice and pigs that peaked at 14 dpi with low frequency (Figures 2, 7) and IFN-γ+CD8 T cells seemed to play a dispensable role, consistent with a previous study (30). As previous studies showed that the magnitude of the IFN-γ response in PBMCs of pigs was correlated best with protection against virus shedding (28) and in vivo depletion of CD4 T cells and IFN-γ led to less protection of the BK61-immunized mice from challenge (30). These results highlighted the importance of IFN-γ-producing CD4 T cells in protective immunity against PRV infection. Of note, due to that PRV-induced Th1-biased immunity is more favorable to help antibody secretion by B cells (27), PRV may not be an ideal viral vector for developing a vectored-vaccine against PRRSV and ASFV whose immune protection are believed to largely rely on cell-mediated immunity (32, 47, 48).
Taken together, through a comprehensive analysis of PRV-specific T cells response, we demonstrated that attenuated PRV vaccine induced a Th1-biased cellular immunity in mice and pigs that is relatively weak, which might be an intrinsic property or drawbacks of PRV LAVs. Our findings provide a rationale for the design of PRV-vectored vaccines and may also be implicative for the selection of other viral vectors for vaccine development.
Data availability statement
The original contributions presented in the study are included in the article/supplementary material. Further inquiries can be directed to the corresponding authors.
Ethics statement
This study was carried out in accordance with the recommendations in the Guide for the Care and Use of Laboratory Animals of Zhejiang University. All animal experiments were approved by the Animal Welfare and Ethics Committee at the College of animal science of Zhejiang University with reference number ZJU20230315.
Author contributions
GX: Funding acquisition, Investigation, Methodology, Resources, Writing – original draft, Data curation, Formal analysis, Validation, Visualization. HL: Data curation, Formal analysis, Investigation, Methodology, Validation, Visualization, Writing – original draft, Software. CL: Data curation, Formal analysis, Investigation, Methodology, Visualization, Writing – original draft. HML: Data curation, Formal analysis, Investigation, Methodology, Writing – original draft. YJ: Data curation, Formal analysis, Investigation, Methodology, Writing – original draft, Visualization. YY: Methodology, Writing – original draft, Project administration, Resources. SS: Methodology, Project administration, Resources, Writing – original draft, Conceptualization, Funding acquisition, Investigation, Supervision, Writing – review & editing. JZ: Conceptualization, Funding acquisition, Project administration, Resources, Supervision, Writing – original draft, Writing – review & editing.
Funding
The author(s) declare financial support was received for the research, authorship, and/or publication of this article. This study was financially supported by National Natural Science Foundation of China (3192454), the National Key Research and Development Program of China (2022YFD1800804), the Fundamental Research Funds for the Central Universities (2022-KYY-517101-0005), Hangzhou Chengxi Sci-tech innovation Corridor Management Committee, and a project funded by the Priority Academic Program Development of Jiangsu Higher Education Institutions (PAPD) and JSSCTD202224.
Conflict of interest
The authors declare that the research was conducted in the absence of any commercial or financial relationships that could be construed as a potential conflict of interest.
Publisher’s note
All claims expressed in this article are solely those of the authors and do not necessarily represent those of their affiliated organizations, or those of the publisher, the editors and the reviewers. Any product that may be evaluated in this article, or claim that may be made by its manufacturer, is not guaranteed or endorsed by the publisher.
References
1. Tan L, Yao J, Yang Y, Luo W, Yuan X, Yang L, et al. Current status and challenge of pseudorabies virus infection in China. Virol Sin. (2021) 36:588–607. doi: 10.1007/s12250-020-00340-0
2. Fan S, Yuan H, Liu L, Li H, Wang S, Zhao W, et al. Pseudorabies virus encephalitis in humans: a case series study. J Neurovirol. (2020) 26:556–64. doi: 10.1007/s13365-020-00855-y
3. Ai JW, Weng SS, Cheng Q, Cui P, Li YJ, Wu HL, et al. Human endophthalmitis caused by pseudorabies virus infection, China, 2017. Emerg Infect Dis. (2018) 24:1087–90. doi: 10.3201/eid2406.171612
4. An TQ, Peng JM, Tian ZJ, Zhao HY, Li N, Liu YM, et al. Pseudorabies virus variant in Bartha-K61-vaccinated pigs, China, 2012. Emerg Infect Dis. (2013) 19:1749–55. doi: 10.3201/eid1911.130177
5. Tong W, Liu F, Zheng H, Liang C, Zhou YJ, Jiang YF, et al. Emergence of a Pseudorabies virus variant with increased virulence to piglets. Vet Microbiol. (2015) 181:236–40. doi: 10.1016/j.vetmic.2015.09.021
6. Steiner I, Kennedy PG, Pachner AR. The neurotropic herpes viruses: herpes simplex and varicella-zoster. Lancet Neurol. (2007) 6:1015–28. doi: 10.1016/S1474-4422(07)70267-3
7. Freuling CM, Muller TF, Mettenleiter TC. Vaccines against pseudorabies virus (PrV). Vet Microbiol. (2017) 206:3–9. doi: 10.1016/j.vetmic.2016.11.019
8. Laval K, Vernejoul JB, Van Cleemput J, Koyuncu OO, Enquist LW. Virulent pseudorabies virus infection induces a specific and lethal systemic inflammatory response in mice. J Virol. (2018) 92:e01614–18. doi: 10.1128/JVI.01614-18
9. Travieso T, Li J, Mahesh S, Mello J, Blasi M. The use of viral vectors in vaccine development. NPJ Vaccines. (2022) 7:75. doi: 10.1038/s41541-022-00503-y
10. Liu Z, Kong ZJ, Chen M, Shang YL. Design of live-attenuated animal vaccines based on pseudorabies virus platform. Anim Dis. (2022) 2:10. doi: 10.1186/s44149-022-00044-w
11. Ren J, Madera R, Cunningham C, Shi J, Wang L. An easy method to generate recombinant pseudorabies virus expressing the capsid protein of Porcine circovirus type 2d. Front Microbiol. (2023) 14:1206021. doi: 10.3389/fmicb.2023.1206021
12. Zheng LL, Guo XQ, Zhu QL, Chao AJ, Fu PF, Wei ZY, et al. Construction and immunogenicity of a recombinant pseudorabies virus co-expressing porcine circovirus type 2 capsid protein and interleukin 18. Virus Res. (2015) 201:8–15. doi: 10.1016/j.virusres.2015.02.010
13. Lu C, Li H, Chen W, Li H, Ma J, Peng P, et al. Immunological characteristics of a recombinant alphaherpesvirus with an envelope-embedded Cap protein of circovirus. Front Immunol. (2024) 15:1438371. doi: 10.3389/fimmu.2024.1438371
14. Tong W, Zheng H, Li GX, Gao F, Shan TL, Zhou YJ, et al. Recombinant pseudorabies virus expressing E2 of classical swine fever virus (CSFV) protects against both virulent pseudorabies virus and CSFV. Antiviral Res. (2020) 173:104652. doi: 10.1016/j.antiviral.2019.104652
15. Zhou M, Abid M, Cao S, Zhu S. Recombinant pseudorabies virus usage in vaccine development against swine infectious disease. Viruses. (2023) 15:370. doi: 10.3390/v15020370
16. Deng L, Gu S, Huang Y, Wang Y, Zhao J, Nie M, et al. Immunogenic response of recombinant pseudorabies virus carrying B646L and B602L genes of African swine fever virus in mice. Vet Microbiol. (2023) 284:109815. doi: 10.1016/j.vetmic.2023.109815
17. Wittmann G, Bartenbach G, Jakubik J. Cell-mediated immunity in Aujeszky disease virus infected pigs. I. Lymphocyte stimulation. Arch Virol. (1976) 50:215–22. doi: 10.1007/BF01320575
18. Martin S, Wardley RC, Donaldson AI. Functional antibody responses in pigs vaccinated with live and inactivated Aujeszky's disease virus. Res Vet Sci. (1986) 41:331–5. doi: 10.1016/S0034-5288(18)30625-8
19. Wittmann G, Leitzke I, Hohn U. Cell-mediated cytotoxicity and lymphocyte stimulation in Aujeszky's disease. II. Following vaccination of swine and subsequent infection. Zentralbl Veterinarmed B. (1985) 32:181–96. doi: 10.1111/j.1439-0450.1985.tb01955.x
20. Kimman TG, De Bruin TM, Voermans JJ, Peeters BP, Bianchi AT. Development and antigen specificity of the lymphoproliferation responses of pigs to pseudorabies virus: dichotomy between secondary B- and T-cell responses. Immunology. (1995) 86:372–8.
21. Jin YL, Yin D, Xing G, Huang YM, Fan CM, Fan CF, et al. The inactivated gE/TK gene-deleted vaccine against pseudorabies virus type II confers effective protection in mice and pigs. Front Microbiol. (2022) 13:943707. doi: 10.3389/fmicb.2022.943707
22. Zuckermann FA, Husmann RJ, Schwartz R, Brandt J, Mateu de Antonio E, Martin S. Interleukin-12 enhances the virus-specific interferon gamma response of pigs to an inactivated pseudorabies virus vaccine. Vet Immunol Immunopathol. (1998) 63:57–67. doi: 10.1016/S0165-2427(98)00082-8
23. De Bruin MG, De Visser YE, Kimman TG, Bianchi AT. Time course of the porcine cellular and humoral immune responses in vivo against pseudorabies virus after inoculation and challenge: significance of in vitro antigenic restimulation. Vet Immunol Immunopathol. (1998) 65:75–87. doi: 10.1016/S0165-2427(98)00175-5
24. Martin S, Wardley RC. Local humoral and cellular responses in Aujeszky's disease virus infection in pigs. Res Vet Sci. (1987) 42:170–4. doi: 10.1016/S0034-5288(18)30681-7
25. de Bruin TG, van Rooij EM, de Visser YE, Voermans JJ, Samsom JN, Kimman TG, et al. Discrimination of different subsets of cytolytic cells in pseudorabies virus-immune and naive pigs. J Gen Virol. (2000) 81:1529–37. doi: 10.1099/0022-1317-81-6-1529
26. Zuckermann FA, Zsak L, Mettenleiter TC, Ben-Porat T. Pseudorabies virus glycoprotein gIII is a major target antigen for murine and swine virus-specific cytotoxic T lymphocytes. J Virol. (1990) 64:802–12. doi: 10.1128/jvi.64.2.802-812.1990
27. Ober BT, Summerfield A, Mattlinger C, Wiesmuller KH, Jung G, Pfaff E, et al. Vaccine-induced, pseudorabies virus-specific, extrathymic CD4+CD8+ memory T-helper cells in swine. J Virol. (1998) 72:4866–73. doi: 10.1128/JVI.72.6.4866-4873.1998
28. van Rooij EM, de Bruin MG, de Visser YE, Middel WG, Boersma WJ, Bianchi AT. Vaccine-induced T cell-mediated immunity plays a critical role in early protection against pseudorabies virus (suid herpes virus type 1) infection in pigs. Vet Immunol Immunopathol. (2004) 99:113–25. doi: 10.1016/j.vetimm.2004.01.004
29. Pomorska-Mol M, Markowska-Daniel I, Pejsak Z. Evaluation of humoral and antigen-specific T-cell responses after vaccination of pigs against pseudorabies in the presence of maternal antibodies. Vet Microbiol. (2010) 144:450–4. doi: 10.1016/j.vetmic.2010.01.015
30. Bianchi AT, Moonen-Leusen HW, van Milligen FJ, Savelkoul HF, Zwart RJ, Kimman TG. A mouse model to study immunity against pseudorabies virus infection: significance of CD4+ and CD8+ cells in protective immunity. Vaccine. (1998) 16:1550–8. doi: 10.1016/S0264-410X(98)00044-9
31. Grob P, Schijns VE, van den Broek MF, Cox SP, Ackermann M, Suter M. Role of the individual interferon systems and specific immunity in mice in controlling systemic dissemination of attenuated pseudorabies virus infection. J Virol. (1999) 73:4748–54. doi: 10.1128/JVI.73.6.4748-4754.1999
32. Tian Y, Wang D, He S, Cao Z, Li W, Jiang F, et al. Immune cell early activation, apoptotic kinetic, and T-cell functional impairment in domestic pigs after ASFV CADC_HN09 strain infection. Front Microbiol. (2024) 15:1328177. doi: 10.3389/fmicb.2024.1328177
33. Mu S, Chen L, Dong H, Li S, Zhang Y, Yin S, et al. Enhanced antigen-specific CD8 T cells contribute to early protection against FMDV through swine DC vaccination. J Virol. (2024) 98:e0200223. doi: 10.1128/jvi.02002-23
34. Hao X, Li S, Li J, Yang Y, Qin A, Shang S. An anti-tumor vaccine against marek's disease virus induces differential activation and memory response of gammadelta T cells and CD8 T cells in chickens. Front Immunol. (2021) 12:645426. doi: 10.3389/fimmu.2021.645426
35. Shang S, Kats D, Cao L, Morgun E, Velluto D, He Y, et al. Induction of mycobacterium tuberculosis lipid-specific T cell responses by pulmonary delivery of mycolic acid-loaded polymeric micellar nanocarriers. Front Immunol. (2018) 9:2709. doi: 10.3389/fimmu.2018.02709
36. Shang S, Siddiqui S, Bian Y, Zhao J, Wang CR. Nonclassical MHC ib-restricted CD8+ T cells recognize mycobacterium tuberculosis-derived protein antigens and contribute to protection against infection. PLoS Pathog. (2016) 12:e1005688. doi: 10.1371/journal.ppat.1005688
37. Lee AC, Zhang AJ, Li C, Chen Y, Liu F, Zhao Y, et al. Intradermal vaccination of live attenuated influenza vaccine protects mice against homologous and heterologous influenza challenges. NPJ Vaccines. (2021) 6:95. doi: 10.1038/s41541-021-00359-8
38. Zhang Z, Liu Q, Sun Y, Li J, Liu J, Pan R, et al. Live attenuated coronavirus vaccines deficient in N7-Methyltransferase activity induce both humoral and cellular immune responses in mice. Emerg Microbes Infect. (2021) 10:1626–37. doi: 10.1080/22221751.2021.1964385
39. Hoft DF, Babusis E, Worku S, Spencer CT, Lottenbach K, Truscott SM, et al. Live and inactivated influenza vaccines induce similar humoral responses, but only live vaccines induce diverse T-cell responses in young children. J Infect Dis. (2011) 204:845–53. doi: 10.1093/infdis/jir436
40. Tian Y, Hao Y, Dong M, Li S, Wang D, Jiang F, et al. Development of a monoclonal antibody to pig CD69 reveals early activation of T cells in pig after PRRSV and ASFV infection. Viruses. (2022) 14:1343. doi: 10.3390/v14061343
41. Baaten BJ, Tinoco R, Chen AT, Bradley LM. Regulation of antigen-experienced T cells: lessons from the quintessential memory marker CD44. Front Immunol. (2012) 3:23. doi: 10.3389/fimmu.2012.00023
42. Han Q, Bagheri N, Bradshaw EM, Hafler DA, Lauffenburger DA, Love JC. Polyfunctional responses by human T cells result from sequential release of cytokines. Proc Natl Acad Sci U.S.A. (2012) 109:1607–12. doi: 10.1073/pnas.1117194109
43. Seder RA, Darrah PA, Roederer M. T-cell quality in memory and protection: implications for vaccine design. Nat Rev Immunol. (2008) 8:247–58. doi: 10.1038/nri2274
44. Badovinac VP, Messingham KA, Jabbari A, Haring JS, Harty JT. Accelerated CD8+ T-cell memory and prime-boost response after dendritic-cell vaccination. Nat Med. (2005) 11:748–56. doi: 10.1038/nm1257
45. Brittle EE, Reynolds AE, Enquist LW. Two modes of pseudorabies virus neuroinvasion and lethality in mice. J Virol. (2004) 78:12951–63. doi: 10.1128/JVI.78.23.12951-12963.2004
46. Pegg CE, Zaichick SV, Bomba-Warczak E, Jovasevic V, Kim D, Kharkwal H, et al. Herpesviruses assimilate kinesin to produce motorized viral particles. Nature. (2021) 599:662–6. doi: 10.1038/s41586-021-04106-w
47. Li S, Li J, Tian Y, Liu J, Zhu J, Chen N, et al. A potent CD8 T-cell response may be associated with partial cross-protection conferred by an attenuated Chinese HP-PRRSV vaccine against NADC30-like PRRSV challenge. J Gen Virol. (2023) 104:001850. doi: 10.1099/jgv.0.001850
Keywords: Pseudorabies virus, live attenuated vaccines, Bartha K61, cellular immunity, T cells
Citation: Xing G, Li H, Lu C, Li H, Jin Y, Yan Y, Shang S and Zhou J (2024) The attenuated Pseudorabies virus vaccine Bartha K61 induces a weak cellular immunity: implications for the development of PRV-vectored vaccines. Front. Immunol. 15:1489268. doi: 10.3389/fimmu.2024.1489268
Received: 31 August 2024; Accepted: 30 November 2024;
Published: 23 December 2024.
Edited by:
Lei Tan, Chinese Academy of Agricultural Sciences, ChinaReviewed by:
Parul Sharma, University of Liverpool, United KingdomLi Huang, Chinese Academy of Agricultural Sciences, China
Copyright © 2024 Xing, Li, Lu, Li, Jin, Yan, Shang and Zhou. This is an open-access article distributed under the terms of the Creative Commons Attribution License (CC BY). The use, distribution or reproduction in other forums is permitted, provided the original author(s) and the copyright owner(s) are credited and that the original publication in this journal is cited, in accordance with accepted academic practice. No use, distribution or reproduction is permitted which does not comply with these terms.
*Correspondence: Shaobin Shang, c2hhb2JpbnNoYW5nQHl6dS5lZHUuY24=; Jiyong Zhou, anl6aG91QHpqdS5lZHUuY24=