- 1Department of General Surgery, Affiliated Hospital of Nantong University & Medical School of Nantong University, Nantong, China
- 2Clinical and Translational Research Center, Affiliated Hospital of Nantong University & Department of Oncology, Medical School of Nantong University, Nantong, Jiangsu, China
Background: Breast cancer (BC) remains a significant health issue globally and most common cause of mortality in women. Enhancing our understanding on biomarkers may greatly improve both diagnostic and therapeutic approaches to this disease.
Methods: We retrospectively assessed tumor samples from 228 BC cases and 51 normal samples, alongside relevant clinical data. Neuronal vesicle trafficking associated 2(NSG2) expression was evaluated through bioinformatics and multiplex immunohistochemistry. Associations between NSG2 expression, tumor-infiltrating immune cells (TIICs), immune checkpoints, and clinical outcomes were investigated.
Results: NSG2 was present in both breast cancer cells and adjacent stromal cells. Increased NSG2 expression in cancer cells correlated with greater tumor size, distant metastasis, and more advanced clinical stages. Kaplan-Meier survival and multivariate analyses identified NSG2 expression in both cancer and stromal cells as an independent prognostic factor for breast cancer survival. Elevated NSG2 levels both in cancer and stroma cells were linked to increased CD4+ T, CD8+ T, and Lamp3+ dendritic cells infiltration in stromal regions (P < 0.05). Conversely, the expression of NSG2 in the stroma was negatively correlated with CD20+ B cells (P < 0.05). Additionally, NSG2 expression was found to be associated with CTLA-4 levels (P < 0.05).
Conclusion: NSG2 seems to be a significant component of the BC immune microenvironment and may serve as an important prognostic marker.
1 Introduction
Breast cancer (BC) is the most common cancer and a leading cause of cancer-related deaths in women worldwide (1, 2), with treatment decisions often based on molecular profiling of BC (3, 4). BC classification has traditionally been based on its receptors, and Her-2 expression to guide treatment choices (5). However, issues like drug resistance continue to pose significant challenges, underscoring the need for new strategies to enhance long-term patient outcomes (6).
Immune cells were traditionally considered inhibitors of cancer progression (7). However, emerging evidence reveals a more complex role for the immune microenvironment, which can both suppress tumor growth and facilitate tumor escape (8, 9). This evolving understanding has shifted focus towards immunotherapy as a promising strategy for enhancing patient outcomes (10). Tumor microenvironment (TME) is essential for cancer development and clinical prognosis (11). Analysis of tumor-infiltrating immune cells (TIICs) offers insights into mechanisms of immune evasion and opens avenues for novel therapeutic strategies (12). Therefore, integrating immune infiltration characteristics with molecular and histologic criteria is essential for advancing BC classification (13).
Immune checkpoint inhibitors can reverse the immune suppression induced by tumors, thereby restoring the immune system’s ability to target cancer cells (14), and have shown promise for patients who are resistant to conventional treatments or have poor prognoses.
Cancer neuroscience is an emerging field within cancer biology that seeks to elucidate the interactions between the nervous system, malignancy, and its microenvironment (15). Neural elements and BC exhibit a complex and interdependent relationship, with nerves significantly impacting patient outcomes (16). Recent studies have highlighted the involvement of neuronal cell vesicles in various cancers, including gastric cancer, glioma, and head and neck squamous cell carcinoma (15, 17, 18). Moreover, vesicle trafficking has been shown to impact cancer cell drug resistance by modulating the immune microenvironment (19–21). Neuronal vesicle trafficking associated 2(NSG2), also known as HMP19, is localized in the Golgi apparatus of neural and neuroendocrine cells and plays a role in nerve signal transmission (22). Overexpression of NSG2 in primary bone marrow cells has been linked to the proliferation of immature cells and shows promise in inhibiting the growth and spread of pancreatic cancer (23).
This study investigates NSG2’s role in BC by analyzing its immunological and prognostic significance through multiplex immunofluorescence, exploring its correlation with clinical features, outcomes, TIICs, and immune checkpoints, and suggesting its potential as promising indicator for prognosis and immunotherapy target in this disease.
2 Materials and methods
2.1 Genomic data assessment
Sangerbox database (http://sangerbox.com/Tool) was used for assessing NSG2 expression levels in human BC tissues with those in unpaired normal tissues (24), and Kaplan-Meier Plotter (http://kmplot.com/analysis/) was used to determine NSG2 expression prognostic significance, which determines optimal cutoff values for group categorization.
2.2 Clinical tissue samples
BC tissue samples (n = 228) and non-cancerous tissue samples (n = 51) were collected from Nantong Tumor Hospital Affiliated to Nantong University, between 2010 and 2016. Clinical data were retrieved from the hospital’s electronic records, and tissue microarrays (TMAs) were constructed by the Department of Clinical Biobank. No cases underwent preoperative therapy. OS was considered the time from surgery to death or last follow-up. Ethics approval (number 2024-031) was provided by the local hospital’s Human Research Ethics Committee.
2.3 Tissue microarray and multiplex immunohistochemical staining
TMA slides were de-paraffinized, rehydrated, and subjected to antigen retrieval before being stained with primary and secondary antibodies using the PerkinElmer Opal 7-Color Technology Kit, DAPI, and scanned at 20× magnification with Vectra 3.0 and analyzed using inForm software.
Cytokeratin (CK)-positive tumor areas were identified to distinguish cancer cells from stromal cells. TIIC levels were quantified using a machine-learning algorithm, and NSG2 protein expression was compared with immune cell types in the BC TMA. The antibodies used for mIHC analysis are shown in Supplementary Table S1.
2.4 Statistical analysis
GraphPad Prism (version 5.0, USA) and SPSS (version 24.0, USA) were used for data analysis. Pearson χ² tests assessed correlations between NSG2 expression and clinicopathologic features, Cox regression models and Kaplan-Meier curves analyzed survival, and the Spearman test evaluated relationships between TIIC abundance, immune checkpoint expression, and NSG2 levels, using P < 0.05 for denoting significant difference.
3 Results
3.1 NSG2 in BC and prognostic implications
NSG2 mRNA expression was remarkedly increased in BC tissues (mean ± SEM: -3.35 ± 3.03) than in non-cancerous tissues (mean ± SEM: -4.81 ± 2.71; P < 0.05) (Figure 1A). Kaplan-Meier survival analysis revealed high tumoral NSG2 expression associated with poorer prognosis (Figure 1B).
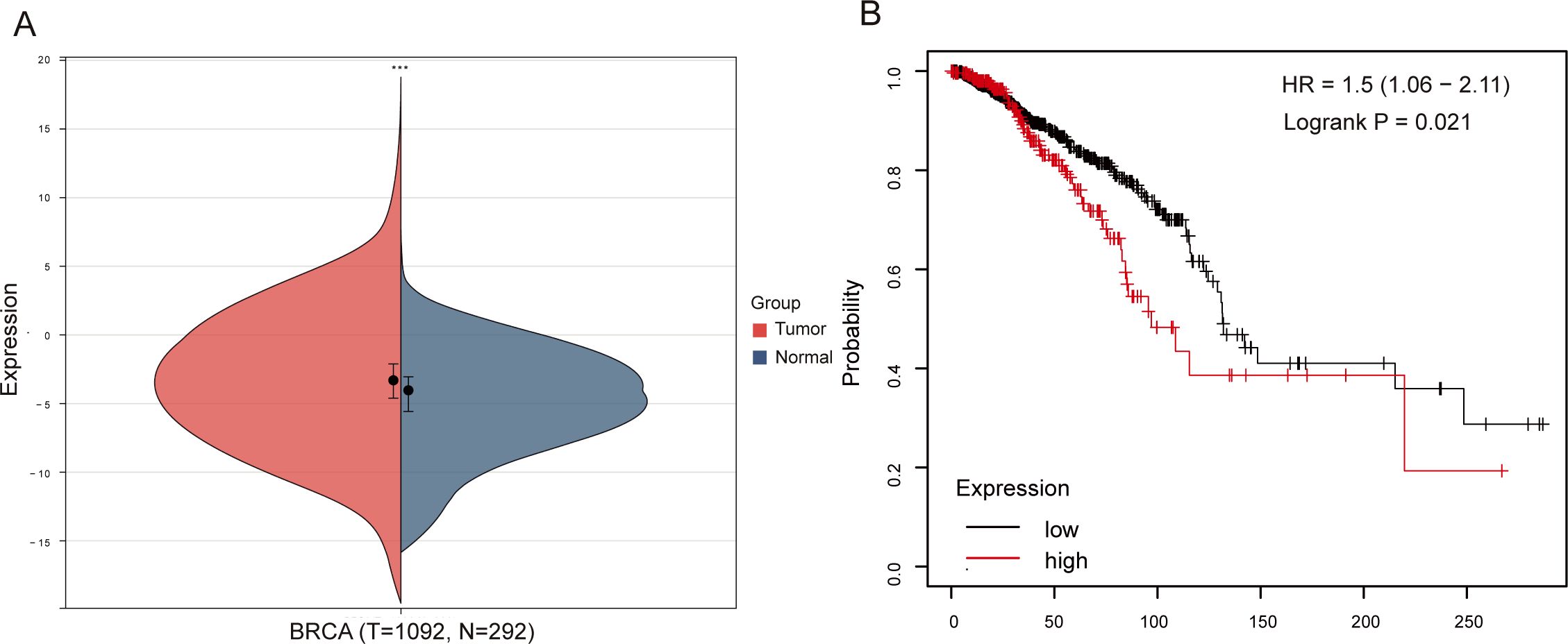
Figure 1. Association between NSG2 expression and OS in breast cancer. (A) NSG2 mRNA expression in breast carcinoma versus normal tissues. (B) Kaplan-Meier survival curves for high- and low-expression groups.
3.2 NSG2 protein expression in BC and non-cancer tissues
Due to post-transcriptional regulatory mechanisms, mRNA expression does not always coincide with the expression of the corresponding protein (25). To evaluate NSG2 protein expression, multiplex immunohistochemistry was used, revealing higher levels in cancer cells (36.47 ± 13.61) compared to ductal epithelial cells (32.36 ± 10.59; Z = -2.003, P = 0.045) (Figures 2A, B) and greater expression in breast cancer tissues than benign tissues (Figure 2C). NSG2 protein was also more prevalent in intratumoral cells than in stromal cells (36.47 ± 13.61 vs. 10.25 ± 8.18; P < 0.001) (Figure 2D).
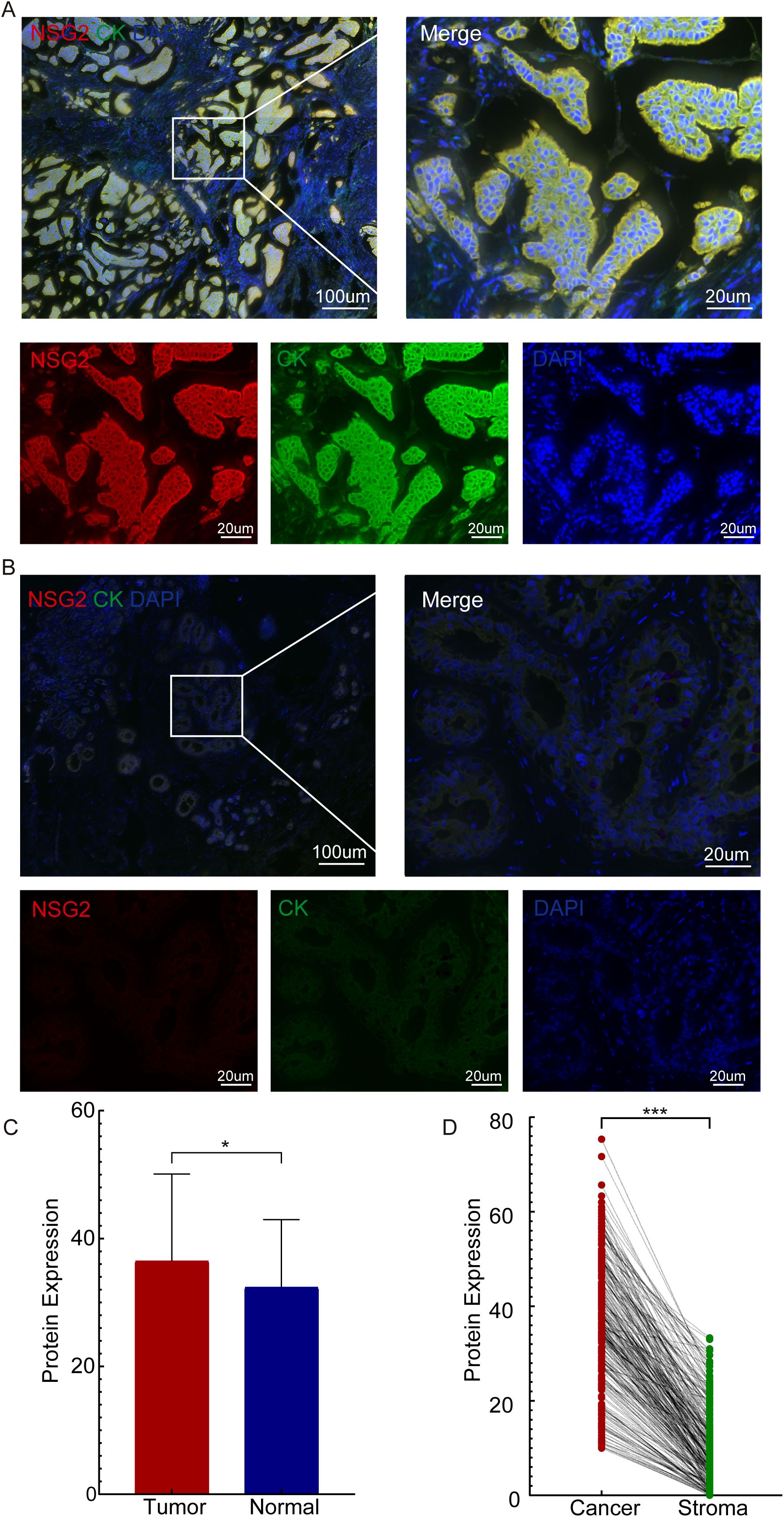
Figure 2. NSG2 protein expression. (A) In breast carcinoma tissues. (B) In non-cancerous samples. (C) Comparison between breast carcinoma and normal tissues. (D) Comparison between intratumoral and stromal cells.
3.3 Correlation of NSG2 protein expression with clinical features in breast cancer
We determined the optimal cutoff value of NSG2 protein expression in cancer cells using the R package MaxStat. Based on this, we categorized patients into two groups: high NSG2 expression (≥ 33.33, n = 86) and low NSG2 expression (< 33.33, n = 142). For NSG2 protein expression in stromal cells, a cutoff of 16 was used, dividing patients into lower expression (≤ 5.33, n = 83) and higher expression (> 5.33, n = 145) groups. Pearson χ² tests indicated that high NSG2 expression in cancer cells was associated with larger tumor size (P = 0.034), distant metastasis (P = 0.036), and advanced clinical stage (P = 0.032). Conversely, NSG2 expression in TIICs was specifically associated with distant metastasis (P = 0.042) (Table 1).
Univariate Cox regression of 228 patients showed that high NSG2 protein expression in both cancer and stromal cells, along with T stage, N stage, M stage, and clinical stage, significantly correlated with OS. Multivariate analysis confirmed NSG2 expression in cancer and stromal cells as independent prognostic factors for poor outcomes (Table 2). Kaplan-Meier curves indicated that patients with elevated NSG2 in both intratumoral cells and TIICs had notably worse outcomes (Figures 3A, B).
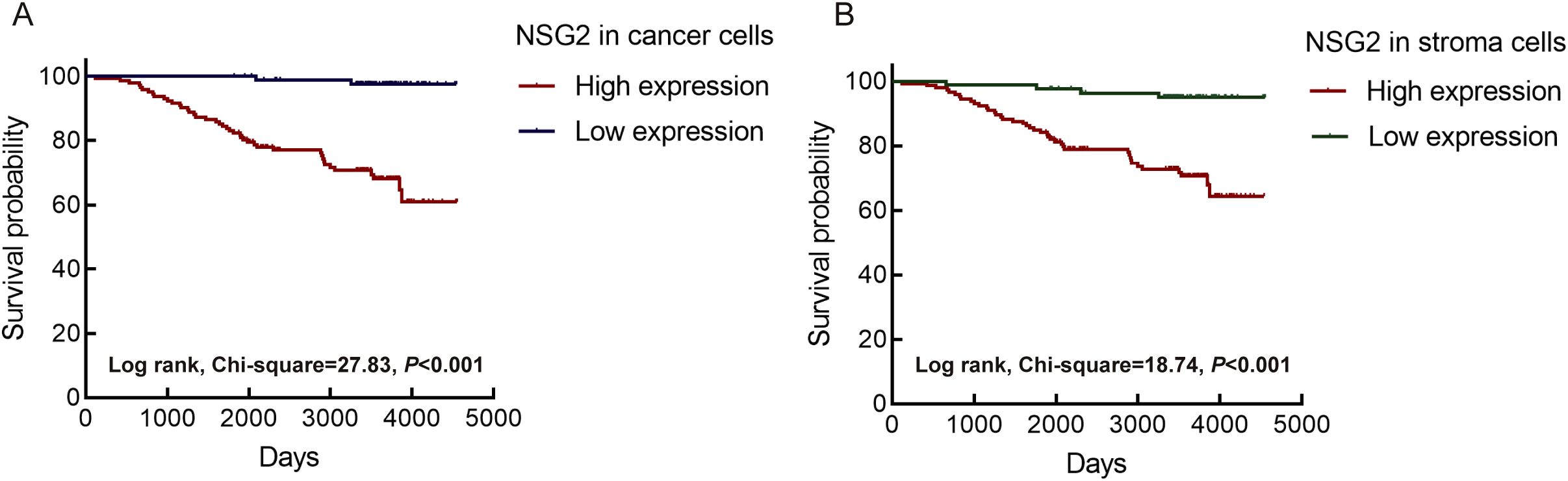
Figure 3. Association between NSG2 protein expression and overall survival. (A) Intratumoral cells. (B) Stroma cells.
3.4 NSG2 expression is associated with the abundance of TIICs and immune checkpoints in breast cancer
NSG2 expression positively correlated with CD4+ T cells, CD8+ T cells, and Lamp3+ dendritic cells in both cancer and stromal compartment, but negatively correlated with CD20+ B cells in stromal cells (Figure 4; Table 3). Additionally, NSG2 levels positively correlated with CTLA-4, but showed no significant associations with PD-1 or PD-L1 (Figure 4; Table 3).
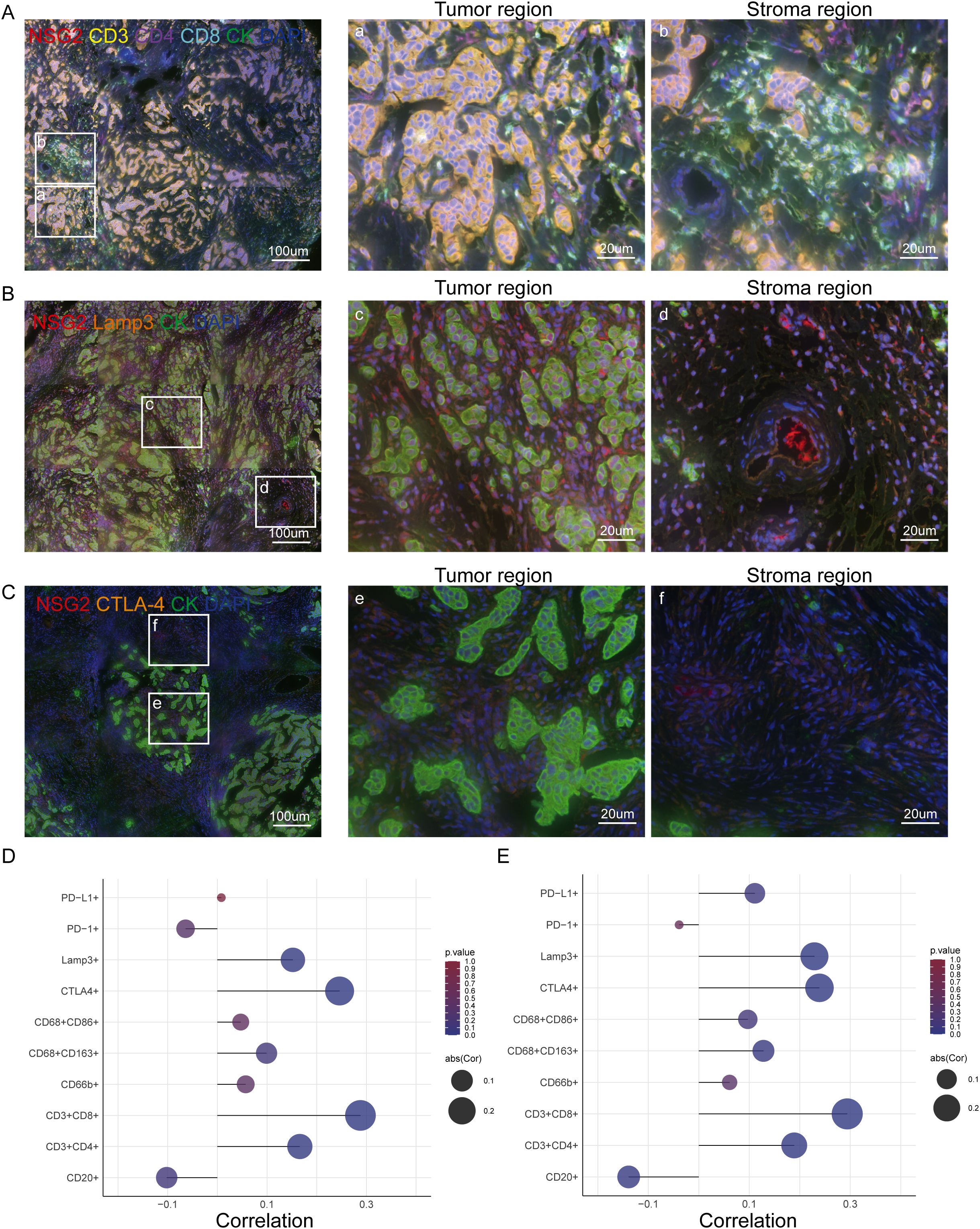
Figure 4. NSG2 expression and its correlation with TIICs and immune checkpoints in BC. (A) Six-color multispectral composite images of NSG2, CD3, CD4, CD8, CK and DAPI. (B) Four-color multispectral composite images of NSG2, LAMP3, CK and DAPI. (C) Four-color multispectral composite images of NSG2, CTLA-4, CK and DAPI. (D) Correlation of NSG2 protein expression in cancer cells with immune markers. (E) Correlation of NSG2 protein expression in stroma cells with immune markers.
4 Discussion
Breast cancer continues to be the most widespread malignancy affecting women worldwide, representing a major challenge to patient survival (26). The identification of innovative therapeutic targets is essential for improving clinical outcomes (27). TCGA and GTEx data analysis reveal elevated NSG2 mRNA in BC tissues, with Kaplan-Meier analysis linking high NSG2 levels to poor prognosis, highlighting its potential as a prognostic biomarker.
Our mIHC analysis indicated that NSG2 expression correlates with larger tumor size, distant metastasis, and advanced stage, suggesting its potential as a prognostic marker in breast cancer.
TIICs are important for carcinogenesis (28–30). Our study found that NSG2 expression positively correlates with CD4+ T, CD8+ T and Lamp3+ dendritic cells levels in both cancerous and stromal tissues. Increased NSG2 expression and immune cell infiltration had poorer outcomes. Lamp3+ dendritic cells, known for their role in modulating tryptophan metabolism and exerting immunomodulatory effects, contribute to tumor escape and progression (31, 32). High NSG2 expression areas also showed elevated Lamp3+ dendritic cell levels, correlating with worse outcomes. Additionally, CD4+ and CD8+ T cells are often found at tumor margins (33). Our findings indicate that NSG2 overexpression is associated with increased infiltration of CD4+ and CD8+ T cells near the tumor. CD4+ T cells enhance tumor antigen presentation through interactions with antigen-presenting cells like dendritic cells (34, 35), thereby boosting the cytotoxic activity of CD8+ T cells. Although CD8+ T cells are well-documented for their direct tumor-killing abilities (33, 36, 37), our results suggest that NSG2 may amplify tumor immunogenicity, leading to increased CD4+ and CD8+ T cells populations. This hypothesis warrants further investigation.
In addition, the role of tumor-infiltrating B lymphocytes within the tumor immune microenvironment is increasingly recognized (38). B cells function as antigen-presenting cells, activating CD4+ T and CD8+ T cells to directly target tumor cells (39). The enrichment of CD20+ B cells has been correlated with favorable overall survival (OS) in various solid tumors, including esophageal squamous cell carcinoma and ovarian serous cystadenocarcinoma (40). Specifically, in triple-negative breast cancer, higher levels of CD20+ B cells are associated with improved prognosis (41). Our study demonstrated that elevated NSG2 expression in the stroma correlates with reduced CD20+ B cells presence and poorer prognosis, consistent with previous findings. Notably, this association was observed only in stromal cells and not in intratumoral cells. Given that NSG2 expression is significantly higher in cancer cells than in stromal cells, further verification of the relationship between NSG2 and CD20+ B cells is warranted.
Immune checkpoint inhibitors have shown promise in treating breast cancer (42). CTLA-4, expressed on CD4+ and CD8+ T cells, serves as a critical immune checkpoint regulating T cell activation and proliferation (43). Elevated CTLA-4 levels are associated with diminished immune activity. Targeting CTLA-4 with inhibitors such as ipilimumab has garnered attention as a therapeutic strategy in breast cancer (44). By blocking CTLA-4, these inhibitors enhance T cell responses to tumor cells, thereby potentially enhancing antitumor immunity (45). Our findings indicate a positive correlation between NSG2 and CTLA-4 expression, with higher NSG2 levels linked to poorer prognosis, aligning with existing literature. Despite advancements in CTLA-4 inhibitors, low cure rates and emerging challenges underscore the need for novel treatment strategies (46). Despite advancements in CTLA-4 inhibitors, low cure rates and emerging challenges underscore the need for novel treatment strategies. Our study suggests that NSG2 levels could serve as a predictive biomarker for the efficacy of anti-CTLA-4 immunotherapy, highlighting its potential as a target for future immunotherapeutic approaches.
Nevertheless, this study has several limitations. Prospective validation of these findings is essential. The single-center design may limit the generalizability of our conclusions. Additionally, further validation through cytological and preclinical studies is needed to elucidate the mechanisms by which NSG2 influences BC progression.
In conclusion, this study is the first to identify elevated NSG2 expression in breast cancer, which is linked to poor survival and associated with immune cell abundance and checkpoint expression. NSG2 may thus be a key prognostic and immunological biomarker for breast cancer.
Data availability statement
The raw data supporting the conclusions of this article will be made available by the authors, without undue reservation.
Ethics statement
The studies involving humans were approved by Human Research Ethics Committee of the Nantong Tumor Hospital Affiliated to Nantong University. The studies were conducted in accordance with the local legislation and institutional requirements. The participants provided their written informed consent to participate in this study.
Author contributions
XL: Writing – original draft, Investigation, Formal analysis. QG: Methodology, Formal analysis, Data curation, Writing – original draft, Investigation. PS: Writing – original draft, Software. LY: Writing – original draft, Supervision, Data curation. XZ: Writing – original draft, Validation, Resources. BL: Writing – original draft, Resources. QN: Writing – review & editing, Project administration, Funding acquisition, Conceptualization.
Funding
The author(s) declare financial support was received for the research, authorship, and/or publication of this article. The current study was supported by Jiangsu Province Capability Improvement Project through Science, Technology and Education (ZDXK202234), and Jiangsu Provincial Research Hospital (YJXYY202204), China.
Acknowledgments
The authors wish to appreciate all lab members.
Conflict of interest
The authors declare that the research was conducted in the absence of any commercial or financial relationships that could be construed as a potential conflict of interest.
Publisher’s note
All claims expressed in this article are solely those of the authors and do not necessarily represent those of their affiliated organizations, or those of the publisher, the editors and the reviewers. Any product that may be evaluated in this article, or claim that may be made by its manufacturer, is not guaranteed or endorsed by the publisher.
Supplementary material
The Supplementary Material for this article can be found online at: https://www.frontiersin.org/articles/10.3389/fimmu.2024.1487447/full#supplementary-material
References
1. Siegel RL, Giaquinto AN, Jemal A. Cancer statistics, 2024. CA: Cancer J Clin. (2024) 74:12–49. doi: 10.3322/caac.21820
2. Bray F, Laversanne M, Sung H, Ferlay J, Siegel RL, Soerjomataram I, et al. Global cancer statistics 2022: GLOBOCAN estimates of incidence and mortality worldwide for 36 cancers in 185 countries. CA: Cancer J Clin. (2024) 74:229–63. doi: 10.3322/caac.21834
3. Giannakeas V, Lim DW, Narod SA. Bilateral mastectomy and breast cancer mortality. JAMA Oncol. (2024) 10:1228–36. doi: 10.1001/jamaoncol.2024.2212
4. Bhatia N, Thareja S. Aromatase inhibitors for the treatment of breast cancer: An overview (2019-2023). Bioorganic Chem. (2024) 151:107607. doi: 10.1016/j.bioorg.2024.107607
5. Curigliano G, Burstein HJ, Gnant M, Loibl S, Cameron D, Regan MM, et al. Understanding breast cancer complexity to improve patient outcomes: The St Gallen International Consensus Conference for the Primary Therapy of Individuals with Early Breast Cancer 2023. Ann oncology: Off J Eur Soc Med Oncol. (2023) 34:970–86. doi: 10.1016/j.annonc.2023.08.017
6. Ji G, Liu J, Zhao Z, Lan J, Yang Y, Wang Z, et al. Polyamine anabolism promotes chemotherapy-induced breast cancer stem cell enrichment. Advanced Sci (Weinheim Baden-Wurttemberg Germany). (2024):e2404853. doi: 10.1002/advs.202404853
7. Palmer S, Albergante L, Blackburn CC, Newman TJ. Thymic involution and rising disease incidence with age. Proc Natl Acad Sci US. (2018) 115:1883–8. doi: 10.1073/pnas.1714478115
8. Wang Y, Li C, He J, Zhao Q, Zhou Y, Sun H, et al. Multi-omics analysis and experimental validation of the value of monocyte-associated features in prostate cancer prognosis and immunotherapy. Front Immunol. (2024) 15:1426474. doi: 10.3389/fimmu.2024.1426474
9. Baxevanis CN, Fortis SP, Perez SA. The balance between breast cancer and the immune system: Challenges for prognosis and clinical benefit from immunotherapies. Semin Cancer Biol. (2021) 72:76–89. doi: 10.1016/j.semcancer.2019.12.018
10. Dvir K, Giordano S, Leone JP. Immunotherapy in breast cancer. Int J Mol Sci. (2024) 25:7517. doi: 10.3390/ijms25147517
11. Wang Y, Wang J, Zhang L, He J, Ji B, Wang J, et al. Unveiling the role of YARS1 in bladder cancer: A prognostic biomarker and therapeutic target. J Cell Mol Med. (2024) 28:1–20. doi: 10.1111/jcmm.18213
12. Huang F, Wang F, Hu Q, Li Y, Jiang D. PTGR1-mediated immune evasion mechanisms in late-stage triple-negative breast cancer: mechanisms of M2 macrophage infiltration and CD8(+) T cell suppression. Apoptosis: an Int J programmed Cell Death. (2024). doi: 10.1007/s10495-024-01991-0
13. Chu X, Tian Y, Lv C. Decoding the spatiotemporal heterogeneity of tumor-associated macrophages. Mol cancer. (2024) 23:150. doi: 10.1186/s12943-024-02064-1
14. Wang Y, Ji B, Zhang L, Wang J, He J, Ding B, et al. Identification of metastasis-related genes for predicting prostate cancer diagnosis, metastasis and immunotherapy drug candidates using machine learning approaches. Biol direct. (2024) 19:50. doi: 10.1186/s13062-024-00494-x
15. Restaino AC, Walz A, Barclay SM, Fettig RR, Vermeer PD. Tumor-associated genetic amplifications impact extracellular vesicle miRNA cargo and their recruitment of nerves in head and neck cancer. FASEB journal: Off Publ Fed Am Societies Exp Biol. (2024) 38:e23803. doi: 10.1096/fj.202400625rr
16. Liu Y, Yin S, Lu G, Du Y. The intersection of the nervous system and breast cancer. Cancer Lett. (2024) 598:217132. doi: 10.1016/j.canlet.2024.217132
17. Xia Y, Jiang T, Li Y, Gu C, Lv J, Lu C, et al. circVAPA-rich small extracellular vesicles derived from gastric cancer promote neural invasion by inhibiting SLIT2 expression in neuronal cells. Cancer Lett. (2024) 592:216926. doi: 10.1016/j.canlet.2024.216926
18. Yin L, Xu Y, Yin J, Cheng H, Xiao W, Wu Y, et al. Construction and validation of a risk model based on the key SNARE proteins to predict the prognosis and immune microenvironment of gliomas. Front Mol Neurosci. (2023) 16:1304224. doi: 10.3389/fnmol.2023.1304224
19. Kuo CL, Lin YC, Lo YK, Lu YZ, Babuharisankar AP, Lien HW, et al. The mitochondrial stress signaling tunes immunity from a view of systemic tumor microenvironment and ecosystem. iScience. (2024) 27:110710. doi: 10.1016/j.isci.2024.110710
20. Guo Y, Ashrafizadeh M, Tambuwala MM, Ren J, Orive G, Yu G. P-glycoprotein (P-gp)-driven cancer drug resistance: biological profile, non-coding RNAs, drugs and nanomodulators. Drug Discov Today. (2024) 29:104161. doi: 10.1016/j.drudis.2024.104161
21. Zheng S, Hu C, Lin Q, Li T, Li G, Tian Q, et al. Extracellular vesicle-packaged PIAT from cancer-associated fibroblasts drives neural remodeling by mediating m5C modification in pancreatic cancer mouse models. Sci Trans Med. (2024) 16:eadi0178. doi: 10.1126/scitranslmed.adi0178
22. Overby M, Serrano-Rodriguez A, Dadras S, Christiansen AK, Ozcelik G, Lichtenthaler SF, et al. Neuron-specific gene NSG1 binds to and positively regulates sortilin ectodomain shedding via a metalloproteinase-dependent mechanism. J Biol Chem. (2023) 299:105446. doi: 10.1016/j.jbc.2023.105446
23. Kurahara H, Bohl C, Natsugoe S, Nishizono Y, Harihar S, Sharma R, et al. Suppression of pancreatic cancer growth and metastasis by HMP19 identified through genome-wide shRNA screen. Int J cancer. (2016) 139:628–38. doi: 10.1002/ijc.30110
24. Shen W, Song Z, Zhong X, Huang M, Shen D, Gao P, et al. Sangerbox: A comprehensive, interaction-friendly clinical bioinformatics analysis platform. iMeta. (2022) 1:e36. doi: 10.1002/imt2.36
25. Liu Y, Beyer A, Aebersold R. On the dependency of cellular protein levels on mRNA abundance. Cell. (2016) 165:535–50. doi: 10.1016/j.cell.2016.03.014
26. Dizon DS, Kamal AH. Cancer statistics 2024: All hands on deck. CA: Cancer J Clin. (2024) 74:8–9. doi: 10.3322/caac.21824
27. Eliyatkın N, Yalçın E, Zengel B, Aktaş S, Vardar E. Molecular classification of breast carcinoma: from traditional, old-fashioned way to A new age, and A new way. J Breast Health. (2015) 11:59–66. doi: 10.5152/tjbh.2015.1669
28. Shen R, Huang Y, Kong D, Ma W, Liu J, Zhang H, et al. Spatial distribution pattern of immune cells is associated with patient prognosis in colorectal cancer. J Trans Med. (2024) 22:606. doi: 10.1186/s12967-024-05418-x
29. Zhao S, Liu Y, Ding L, Zhang C, Ye J, Sun K, et al. Gastric cancer immune microenvironment score predicts neoadjuvant chemotherapy efficacy and prognosis. J Pathol Clin Res. (2024) 10:e12378. doi: 10.1002/2056-4538.12378
30. Arqueros C, Gallardo A, Vidal S, Osuna-Gómez R, Tibau A, Lidia Bell O, et al. Clinical relevance of tumour-infiltrating immune cells in HER2-negative breast cancer treated with neoadjuvant therapy. Int J Mol Sci. (2024) 25:2627. doi: 10.3390/ijms25052627
31. Qu X, Wang Y, Jiang Q, Ren T, Guo C, Hua K, et al. Interactions of Indoleamine 2,3-dioxygenase-expressing LAMP3(+) dendritic cells with CD4(+) regulatory T cells and CD8(+) exhausted T cells: synergistically remodeling of the immunosuppressive microenvironment in cervical cancer and therapeutic implications. Cancer Commun (London England). (2023) 43:1207–28. doi: 10.1002/cac2.12486
32. Wang Z, Ji X, Zhang Y, Yang F, Su H, Zhang H, et al. Interactions between LAMP3+ dendritic cells and T-cell subpopulations promote immune evasion in papillary thyroid carcinoma. J Immunother Cancer. (2024) 12:e008983. doi: 10.1136/jitc-2024-008983
33. Kruse B, Buzzai AC, Shridhar N, Braun AD, Gellert S, Knauth K, et al. CD4(+) T cell-induced inflammatory cell death controls immune-evasive tumours. Nature. (2023) 618:1033–40. doi: 10.1038/s41586-023-06199-x
34. Wang X, Zhou Y, Wu Z, Xie C, Xu W, Zhou Q, et al. Single-cell transcriptomics reveals the role of antigen presentation in liver metastatic breast cancer. iScience. (2024) 27:108896. doi: 10.1016/j.isci.2024.108896
35. Chen JH, Nieman LT, Spurrell M, Jorgji V, Elmelech L, Richieri P, et al. Human lung cancer harbors spatially organized stem-immunity hubs associated with response to immunotherapy. Nat Immunol. (2024) 25:644–58. doi: 10.1038/s41590-024-01792-2
36. Tumeh PC, Harview CL, Yearley JH, Shintaku IP, Taylor EJ, Robert L, et al. PD-1 blockade induces responses by inhibiting adaptive immune resistance. Nature. (2014) 515:568–71. doi: 10.1038/nature13954
37. Waldman AD, Fritz JM, Lenardo MJ. A guide to cancer immunotherapy: from T cell basic science to clinical practice. Nat Rev Immunol. (2020) 20:651–68. doi: 10.1038/s41577-020-0306-5
38. Qin Y, Peng F, Ai L, Mu S, Li Y, Yang C, et al. Tumor-infiltrating B cells as a favorable prognostic biomarker in breast cancer: a systematic review and meta-analysis. Cancer Cell Int. (2021) 21:310. doi: 10.1186/s12935-021-02004-9
39. Rastogi I, Jeon D, Moseman JE, Muralidhar A, Potluri HK, McNeel DG. Role of B cells as antigen presenting cells. Front Immunol. (2022) 13:954936. doi: 10.3389/fimmu.2022.954936
40. Zheng M, Li YM, Liu ZY, Zhang X, Zhou Y, Jiang JL, et al. Prognostic landscape of tumor-infiltrating T and B cells in human cancer. Front Immunol. (2021) 12:731329. doi: 10.3389/fimmu.2021.731329
41. Kuroda H, Jamiyan T, Yamaguchi R, Kakumoto A, Abe A, Harada O, et al. Prognostic value of tumor-infiltrating B lymphocytes and plasma cells in triple-negative breast cancer. Breast Cancer (Tokyo Japan). (2021) 28:904–14. doi: 10.1007/s12282-021-01227-y
42. Li X, Li Y, Tuerxun H, Zhao Y, Liu X, Zhao Y. Firing up “cold” tumors: Ferroptosis causes immune activation by improving T cell infiltration. Biomedicine pharmacotherapy = Biomedecine pharmacotherapie. (2024) 179:117298. doi: 10.1016/j.biopha.2024.117298
43. Cillo AR, Kirkwood JM. Assessing clonal changes in T cells over time following immunotherapy is a breeze with Cyclone. Cancer Cell. (2024) 42:1491–3. doi: 10.1016/j.ccell.2024.08.014
44. Vilela T, Valente S, Correia J, Ferreira F. Advances in immunotherapy for breast cancer and feline mammary carcinoma: From molecular basis to novel therapeutic targets. Biochim Biophys Acta Rev cancer. (2024) 1879:189144. doi: 10.1016/j.bbcan.2024.189144
45. Xu W, Zhang T, Zhu Z, Yang Y. The association between immune cells and breast cancer: insights from mendelian randomization and meta-analysis. Int J Surg (London England). (2024). doi: 10.1097/JS9.0000000000001840
46. Barbir EB, Kitchlu A, Herrmann SM. Immune checkpoint inhibitor-associated nephritis - treatment standard. OXFORD UNIV PRESS, GREAT CLARENDON ST, OXFORD, ENGLAND, OX2 6DP: Nephrology, dialysis, transplantation: official publication of the European Dialysis and Transplant Association - European Renal Association (2024).
Keywords: NSG2, breast cancer, tumor microenvironment, prognosis, biomarker
Citation: Li X, Gu Q, Sun P, Yang L, Zhang X, Lu B and Ni Q (2024) NSG2: a promising prognostic marker shaping the immune landscape of breast cancer. Front. Immunol. 15:1487447. doi: 10.3389/fimmu.2024.1487447
Received: 28 August 2024; Accepted: 30 September 2024;
Published: 18 October 2024.
Edited by:
Minghua Ren, First Affiliated Hospital of Harbin Medical University, ChinaReviewed by:
Zhijie Xu, Central South University, ChinaPeng Luo, The University of Hong Kong, Hong Kong SAR, China
Copyright © 2024 Li, Gu, Sun, Yang, Zhang, Lu and Ni. This is an open-access article distributed under the terms of the Creative Commons Attribution License (CC BY). The use, distribution or reproduction in other forums is permitted, provided the original author(s) and the copyright owner(s) are credited and that the original publication in this journal is cited, in accordance with accepted academic practice. No use, distribution or reproduction is permitted which does not comply with these terms.
*Correspondence: Qichao Ni, bnFjdXNlckAxNjMuY29t