- 1Nutrition and Seafood Laboratory (NuSea.Lab), School of Life and Environmental Sciences, Deakin University, Queenscliff, VIC, Australia
- 2Department of Fisheries Biology and Genetics, Patuakhali Science and Technology University, Patuakhali, Bangladesh
- 3School of Environmental and Life Sciences, The University of Newcastle, Callaghan, NSW, Australia
Introduction: Extreme water temperature affects the well-being of all aquatic animals, including fish. Higher temperatures can lead to the generation of reactive oxygen species (ROS), which can induce oxidative stress and negatively impact fish health and well-being. This study investigated the protective effects of seaweed extract on growth, antioxidant status, inflammatory responses, and gut microbiota to gain a better understanding of the acclimatization ability of Nile tilapia, Oreochromis niloticus in response to oxidative stress caused by high water temperatures.
Methods: Red-seaweed, Gracilaria tenuistipitata rich in polyphenols (i.e., total phenolics and flavonoids content) was considered for the preparation of the Gracilaria extract (GE) for the study. Nile tilapia were fed the GE supplemented diet along with a control diet for 42 days, followed by 14 days of temperature ramping at a rate of 1°C every two days to the desired target (35°C) and 14 days of holding at 32°C for acclimatation.
Results: Nile tilapia fed the GE had a significantly higher growth performance attributed to increased muscle fiber size compared to control (p < 0.05) after the 70 days of feeding trial. Fish fed the GE diet also showed a significantly lower lipid peroxidation by decreased malondialdehyde level when compared to control (p < 0.05). Furthermore, GE diet exhibited increased red blood cell counts with the decreased number of cellular and nuclear abnormalities. The gene expression of tight junction (i.e., occludin, claudin1, ZO-1) and nrf2 (antioxidant biomarker) were upregulated, while hsp70 (related to stress response) was downregulated in fish fed the GE diet. Additionally, GE supplementation led to an increase in bacterial diversity and the abundance of phylum Firmicutes, order Lactobacillales, and genera Sphingobacterium and Prevotella in the distal gut of Nile tilapia, which are mostly considered as beneficial for fish.
Conclusion: The findings suggest that GE has the potential to be used as a dietary supplement to improve health, particularly as a stress-resistant supplement in the diet for Nile tilapia. This study may help make more informed decisions for tailoring the nutrient requirements of fish in the face of climate warming.
1 Introduction
Climate change has emerged as a serious threat in global aquaculture production, particularly due to changes in temperature patterns around the world (1). Increased water temperatures can result in the generation of reactive oxygen species (ROS), which could increase oxidative stress and negatively impact fish by disrupting their growth, reproduction, and overall wellbeing (2). Elevated water temperature is also known to result in a reduction of dissolved oxygen leading to hypoxia, causing further negative effects in the farmed fish (3). According to Huang et al. (1), every fish species has specific temperature ranges in which they thrive, where slight deviations from these ranges can negatively impact feed intake, growth, reproduction, metabolic activity, energy requirement, and utilization, as well as the overall fitness of ectothermic fish. Furthermore, acute and chronic stress resulting from temperature alterations have been shown to manifest in several metabolic (4), immunological (5), and neuroendocrinological (6) disturbances in fish. To uphold standard physiological performance, the inclusion of functional ingredients to aquafeeds is common place, with investigations into probiotics, prebiotics, functional amino acids, fatty acids, vitamins, and organic acids featured widely in studies evaluating the increase of temperature stress (7, 8). Moreover, the ability of seaweed-supplemented diets to strengthen the fish immune system and increase resilience to stress brought on by temperature has recently attracted the attention of the aquaculture industry.
Nutrient rich red-seaweeds such as Gracilaria sp. and Asparagopsis sp. have proven health benefits in fish (9, 10). Proteins, peptides, polysaccharides, and polyphenols are among the biologically active components in red-seaweeds, and their supplementation in aquafeeds have been reported to enhance growth, boost immune response, and increase disease resistance in fish (11). Notable polyphenols present in various red-seaweeds include phenolics, flavonoids, and carotenoids (12). These compounds are reported to have antioxidative, anti- inflammatory, and immunostimulatory effects when administered in animal nutrition (13, 14). Antioxidant properties in red-seaweeds help to neutralize harmful free radicals, reducing oxidative stress (15). Lowering oxidative stress may improve fish ability to cope with environmental stressors. For instance, Silva-Brito et al. (11) discovered that incorporating a 2.5% extract of Gracilaria gracilis in the diets of gilthead seabream, Sparus aurata, enhanced the immune response and reduced cortisol levels when they were subjected to the stress of overcrowding.
Red-seaweeds also contain specific carbohydrates (e.g., agar, agarose, and carrageenans) that can function as prebiotics in promoting host health (16). Prebiotics stimulate the growth of beneficial gut bacteria, which help maintain a healthy gut microbiome, promoting better health and improving disease resistance in fish. For example, Chen et al. (17) investigated the prebiotic effect of polysaccharides (e.g., total sugar, sulfate, and monosaccharides), extracted from red-seaweeds Grateloupia filicina and Eucheuma spinosum, which enhanced the growth of Bifidobacterium. Additionally, Ferreira et al. (18) reported that the inclusion of G. gracilis in the diet of European seabass, Dicentrarchus labrax, increased the abundance of Sulfitobacter and Methylobacterium. These bacteria are known to produce short- and medium-chain fatty acids that help reduce intestinal pH, playing a vital role in controlling the growth of pathogenic bacteria in fish (19, 20). In addition to red-seaweed, Zhang et al. (21) reported that dietary supplementation of green-seaweed, Ulva pertusa, in the diet of white-spotted rabbitfish, Siganus canaliculatus, led to an increase in the abundance of certain Firmicutes bacteria, notably Ruminococcus, Clostridium, and Lachnospiraceae. These bacteria play a key role in breaking down non-starch polysaccharides in the host gut, helping to maintain gut health, strengthen the intestinal barrier, and reduce the risk of intestinal inflammation, especially during times of stress.
The results mentioned above suggest that bioactive substances found in seaweeds have positive effects on immune function, especially in enhancing resilience against different bacterial diseases. Nevertheless, the impacts of seaweed-based diets to mitigate climate-induced stress such as temperature, have not been explored in aquaculture. Nile tilapia, Oreochromis niloticus, is one of the most commonly farmed fish across the world (22). Given the sensitivity of aquaculture to the effects of climate change, Nile tilapia could be assessed as a model species due to its higher thermal tolerance and wide distribution (23). A study conducted by Islam et al. (24) found that Nile tilapia had normal growth at 31°C but produce lower growth and physiological imbalance (i.e., erythrocytic cellular abnormalities and nuclear abnormalities) when cultured at high temperature (34°C). Therefore, the current study aimed to investigate the effects of dietary supplementation with red-seaweed (Gracilaria extract) on the potential mitigation of temperature-induced oxidative stress in Nile tilapia reared under high temperature. More precisely, it was examined how supplementing the feed of Nile tilapia with a Gracilaria extract containing bioactive compounds (i.e., total phenolic and flavonoid content) can regulate the immunological status and antioxidant response to cope at high temperature.
2 Materials and methods
2.1 Seaweed collection, processing, and experimental diet
Gracilaria tenuistipitata extract was prepared following the protocol described by Thépot et al. (25). The red-seaweed sample was collected in May 2022 from the Sonadia Sea Beach coast (21°29′N 91°54′E), Moheskhali, Bangladesh [water salinity ~32 ppt, temperature ~26°C] and thoroughly washed with tap water to remove unwanted contaminants (i.e., salt, sediments, sands, invertebrates, and epiphytes). The clean seaweed samples were then kept in a freeze drier at −80°C for 72 h. Once dried, the sample was grounded and sieved (200 µm mesh size) to produce a fine powder that was then vacuum-sealed and stored at −20°C for further processing. The Gracilaria extract (GE) was made with 70% methanol in a 1:10 (m:v) ratio under dark conditions about 12 h in each time. The crude extract was filtered (Whatman® no. 2) and then evaporated slowly in a rotary evaporator (IKA® RV3 Eco). Once the methanol had completely evaporated, the extract was then stored at −20°C for use. The various steps involved in making the GE are depicted in Figure 1. A representative amount of GE (triplicated) was dried using a freeze dryer at −80°C. The dried GE was then assessed for its nutrient composition (%) including protein (12.47 ± 1.57), lipid (1.35 ± 0.14), and ash (27.02 ± 1.50) based on a standard protocol (AOAC, 2023). The bioactive compounds, including total phenolic content (TPC) and total flavonoid content (TFC), were determined using the procedure described by Sobuj et al. (26). The results showed a TPC of 63.46 ± 1.77 mg GAE/g (gallic acid equivalents per gram) and a TFC of 29.71 ± 1.09 mg QE/g (quercetin equivalent per gram) using methanol as the solvent. The GE was added to the feed mixture along with all the other ingredients during the water addition step to make dough. Afterward, the dough was pelletized measuring 1.0–3.0 mm using a laboratory pelletizer. The pellets were dried at 50°C for 12 h and stored in airtight polythene bags in a refrigerator at 4°C until used. The feed formulation and chemical composition of the diet are presented in Table 1.
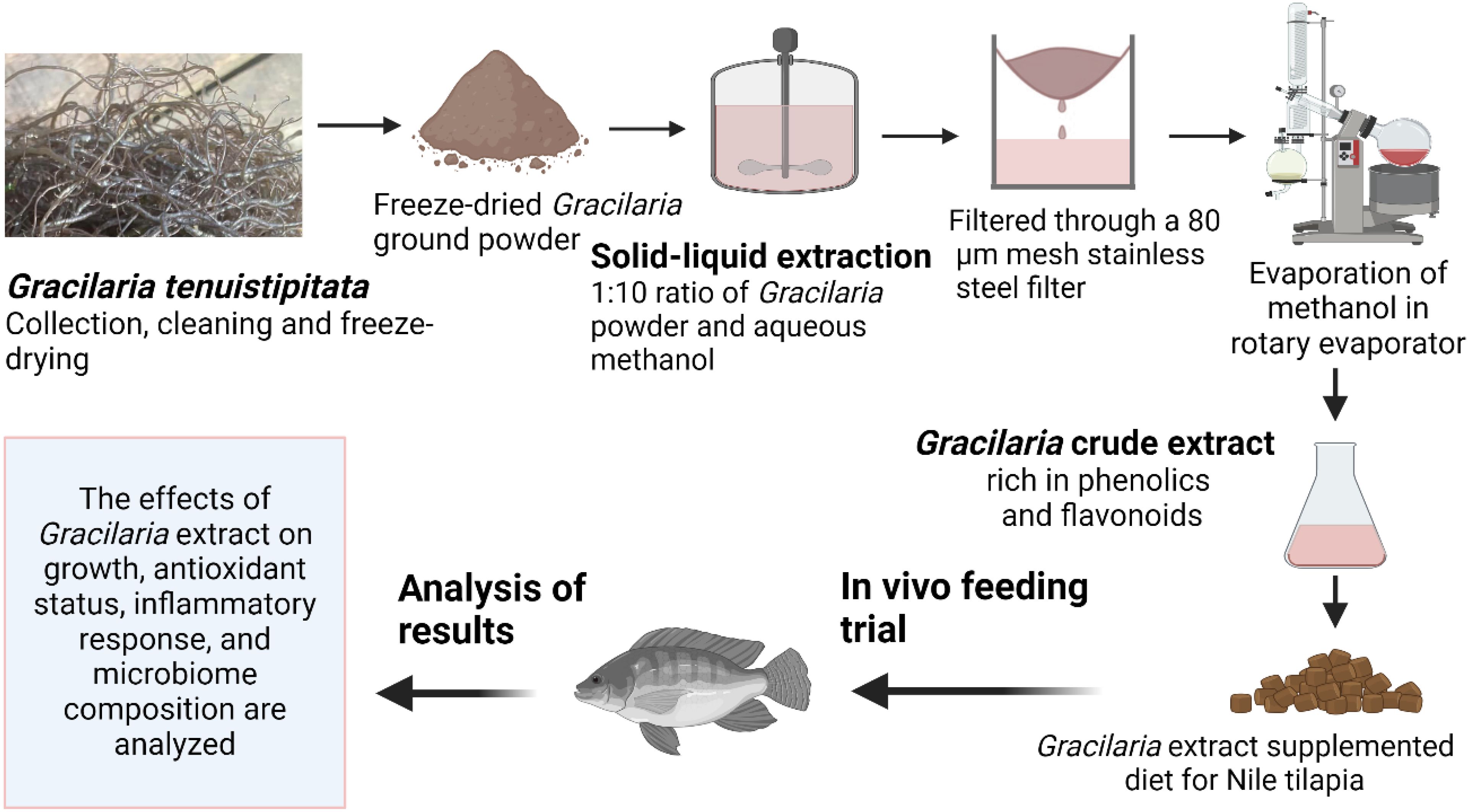
Figure 1. Production and utilization of Gracilaria extract from red-seaweed, Gracilaria tenuistipitata, for Nile tilapia.
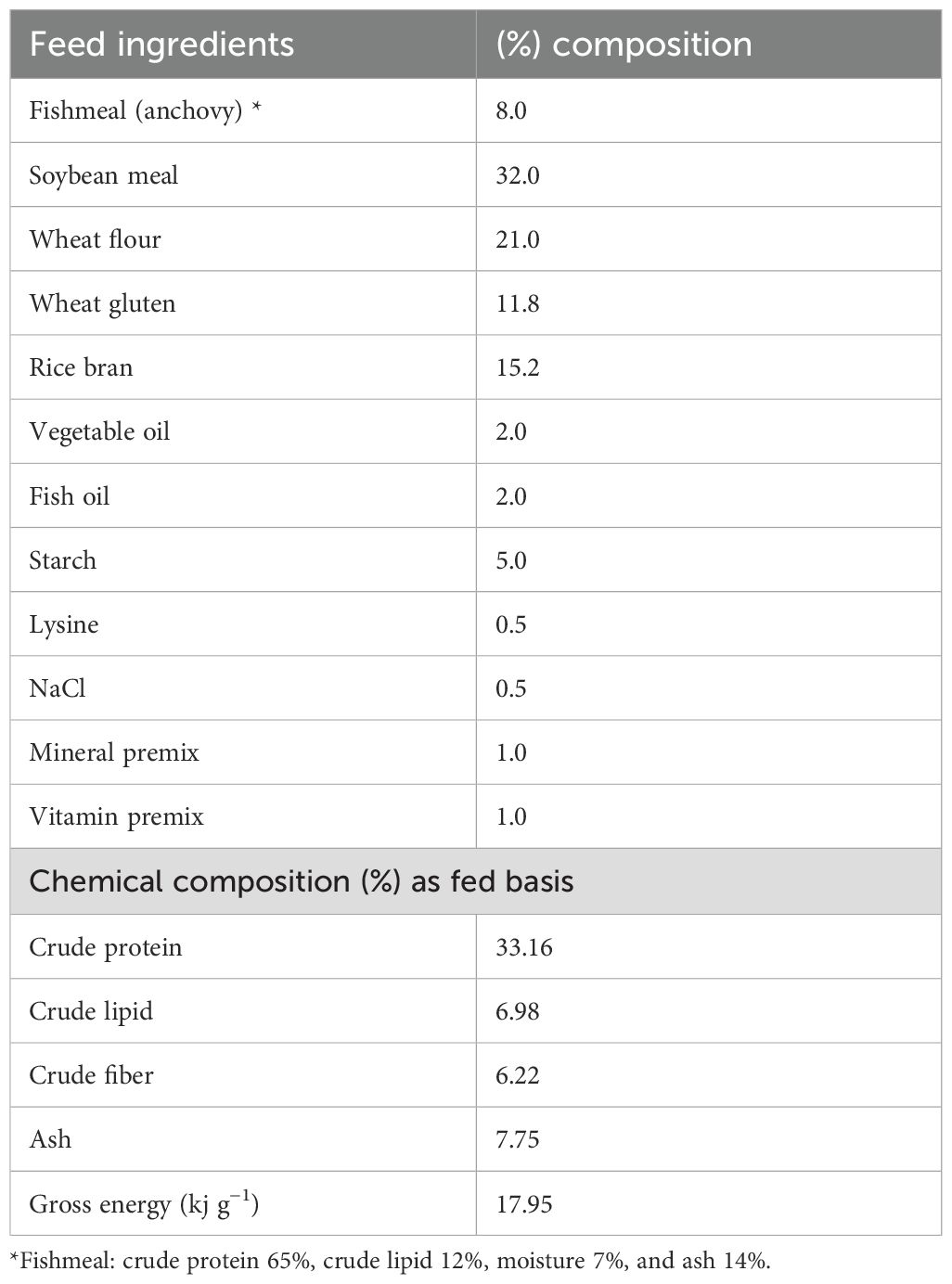
Table 1. Feed ingredients and proximate chemical composition of the basal Nile tilapia diet (% dry weight basis).
2.2 Fish and feeding trial
Monosex Nile tilapia, O. niloticus, fry were purchased from a local fish hatchery (BRAC Fisheries, Khulna, Bangladesh). Nile tilapia fry were placed in eight experimental tanks, each with a 100-L water holding capacity, continuous aeration, and a water temperature of 28°C. They were then acclimatized in tanks for a period of 2 weeks where fish were fed the commercial tilapia diet (Quality Feeds Limited, Bangladesh) twice daily. After 2 weeks of acclimatization, fish were fasted for 24 h, then 200 similar-sized (1.61 ± 0.15 g) fry were randomly assigned into the same eight tanks divided into two dietary groups (four tanks per diet with 25 fish per tank). One quadruplicate group served as a control termed CON, while another group was fed the CON diet added with 1% Gracilaria extract (dry weight basis of feed) named GE. The concentration of GE (~1% of feed) was determined based on previous findings (27). The experiment duration was 10 weeks in which fish were kept under ambient temperature conditions (28°C) for the first 6 weeks. In the following 2 weeks, the water temperature was gradually increased by 1°C every 2 days from the initial temperature to 35°C to test the fish ability to withstand temperature changes. Subsequently, it was maintained at 32°C for 2 weeks to acclimatize the fish to a temperature 4°C higher than their original ambient temperature of 28°C. An overview of the experimental time chart is illustrated in Figure 2. Aerators were used in every tank to ensure enough oxygen (>5 mg/L). Fish were fed twice daily, at 08:00 and 16:00 h, until they appeared to be satisfied. The amount of feed delivered and the bulk weight of each replication tank were assessed every 2 weeks to monitor fish performance over time. To keep the water quality favorable for fish, regular siphoning was ensured to take out the unused feed and feces. Every morning, one-third of the tank water was replaced with temperature-controlled reservoir tank water to maintain the desired water temperature for fish.
2.3 Growth, survival, and feed utilization
Following the feeding trial, fish were counted and bulk weighed in each tank. Several performance parameters such as final body weight (FBW), specific growth rate (SGR), feed intake (FI), feed conversion ratio (FCR), hepatosomatic index (HSI), viscerasomatic index (VSI), and survival rate (SR) were calculated using the formula mentioned in an earlier study (28).
2.4 Histology of muscle, liver, and fat tissues
Eight randomly selected fish (two fish per each replicate) from each dietary group were sampled for liver and intraperitoneal fat histology. The collected samples were cleaned with normal saline solution to remove blood and other unwanted substances and were preserved immediately in 10% buffered formalin and stored at 4°C until further processing. The muscle and fat samples were preserved in 70% alcohol at 4°C until histological analysis. Assessment of lipid accumulation in the liver was performed using Oil Red O staining, whereas muscle and intraperitoneal fat samples were stained with hematoxylin and eosin (H&E). The histology was performed based on standard protocol. Briefly, fixed samples were dehydrated by passing through a graded series of alcohol. After dehydration, the samples were molted with wax and kept in cool storage. The samples were then sectioned (5 μm) using a microtome. The sectioned tissues were stained with H&E and observed under a light microscope (Olympus, Germany) at 400× magnification with photos captured with an onboard camera (BX40F4, Olympus, Tokyo, Japan) connected to the microscope. Muscle microstructure such as fiber diameter and density were assessed by nonparametric statistical procedures according to Rowlerson et al. (29). The adipocyte number and diameter were determined from 30 intact cells from each dietary group according to Chaklader et al. (30). All images were captured using a light digital microscope connected to a camera and ImageJ software (version: 1.53). The protocol used to calculate lipid droplet accumulation in the liver was described in Supplementary Figure S1).
2.5 Serum biochemical responses
At the end of the feeding trial, 4 fish from each replicate tank (16 fish/dietary group) were chosen at random, and blood samples were taken by puncturing the caudal vein and kept in non-heparinized tubes, left to settle for 6 h, and then centrifuged at 4°C for 10 min to extract serum that was kept at −20°C. The lysozyme activity of serum was analyzed following Siddik et al. (31). The levels of aspartate aminotransferase (AST), alanine transaminase (ALT), cholesterol, triglycerides, and glycogen were analyzed by an automated blood analyzer (SLIM; SEAC Inc., Florence, Italy) following Blanc et al. (32).
2.6 Antioxidant response
Fish that were being considered for blood collection were dissected to obtain liver tissue for measuring the antioxidant response. The activities of antioxidant enzymes including malondialdehyde (MDA), superoxide dismutase (SOD), and catalase (CAT) were assessed using commercial kits from ZellBio GmbH, Lonsee, Germany.
2.7 Real-time quantitative PCR
Total RNA from frozen hind gut samples of GE and control-fed fish was isolated using RNeasy Mini Plus Kit (Qiagen, Hilden, Germany) following the manufacturer’s instructions. The quality and quantity of the RNA were evaluated through agarose gel electrophoresis and spectrophotometry (NanoDrop® ND-2000), respectively. cDNA synthesis was performed using a PrimeScript™ RT reagent kit (Takara, Japan). The real-time quantitative PCR (RT-qPCR) primers used in this study for Nile tilapia are listed in Table 2. The melting curve of the amplicon from the primers was obtained using uMELT software. Gene expression levels were measured by RT-qPCR with PowerUp™ SYBR Green Master Mix (Thermo Scientific, USA) on the 7500 Real-Time PCR System (Applied Biosystems, USA). The following conditions were used for real-time PCR: initial denaturation for 2 min at 95°C, followed by 40 cycles of amplification stating 30 s denaturation at 95°C, annealing for 1 min at 60°C, and extension at 72°C for 30 s. The melting stage in PCR began with the 95°C heating step for 15 s and cycled to 70°C cooling for 1 minute, with the continuous increase of 0.015°C per second. The analysis of RT-qPCR data for relative gene expression levels was normalized to the β-actin content in each sample and quantified using the 2−ΔΔCt method as outlined by Livak and Schmittgen (33).
2.8 Blood RBC level and cellular and nuclear abnormalities
Blood red blood cell (RBC) level and its cellular and nuclear abnormalities in fish were measured in Nile tilapia at three different temperatures across the feeding trial in D42 (pre-temperature stress at 28°C), D56 (post-temperature stress at 35°C), and D70 (post-temperature adaptation at 32°C). Two fish from each replicate tank were selected for blood sampling and euthanized after recording their weights for biomass calculations. RBC counts were determined using a hemocytometer. The blood smear slides were prepared immediately after collecting the blood. The blood was spread along the edge of the slide and tilted at a 45° angle to create a thin, even smear. After air-drying for 5 min, the smear was fixed in methanol for approximately 2 min. Once dry and fixed, it was stained with a 5% Giemsa solution. The slides were then air-dried overnight and mounted with dibutylphthalate polystyrene xylene (DPX). The samples were examined under an optical microscope (G-206, Italy) using a 100× objective lens to assess RBC cellular and nuclear abnormalities. RBC cellular abnormalities were categorized as elongated (significantly longer than wider), fusion (joining of more than two cells to create a larger mass), and twin (two cells connected by their surfaces). RBC nuclear abnormalities consisted of micronucleus (circular chromatin bodies resembling the central nucleus), nuclear bridge (a strip of nuclear material connecting two nuclei within separate erythrocytes or within a single erythrocyte), and nuclear degeneration (nuclear condensation and extrusion leading to the creation of a pyrenocyte structure, which is subsequently engulfed and broken down by macrophages).
2.9 Amplicon sequencing
Eight fish from each dietary treatment were randomly selected to obtain hind gut samples, following appropriate biosafety measures. The gut samples, which included mucosa and digesta, were homogenized using a TissueLyser II (Qiagen, Hilden, Germany). Genomic DNA extraction was then carried out using the DNeasy PowerSoil Pro Kit (Qiagen, Hilden, Germany) following the instructions provided by the manufacturer. The DNA was quantified using a NanoDrop 2000c (Thermo Fisher Scientific, USA), and its quality was assessed by running it on a 1% agarose gel. To prepare the final master mix, 50 μL of Hot Start 2× Master mix (New England BioLabs Inc., USA), as per the manufacturer’s instructions, was combined with 2 μL of template DNA, 1 μL each of V3V4 primers (10 µM) with Illumina overhang adapter, and 21 μL of nuclease-free treated water. The mixture was then subjected to 35 cycles of amplification using a BioRad S1000 Thermal Cycler (BioRad Laboratories Inc., USA). Positive amplicons were purified using AMPure beads and indexed according to the Illumina 16S Sequencing Library Preparation protocol (Part # 15044223 Rev. B). Finally, the equimolar amplicons were pooled and sequenced on an Illumina MiSeq platform (Illumina Inc., San Diego, California, USA) using the paired-end, v3 kit with 600 cycles.
2.10 Processing of Illumina data
Paired-end amplicon sequence data (gz format) were imported in qiime2 (v2021.11) for further processing. The denoising of reads was performed using DADA2 followed by trimming of demultiplexed reads with parameters such as -p-trim-left-f l0; -p-trunc-len-f 260; -p-trim-left-r 10; -p-trunc-len-r 220. Chimeric sequences with >0.05% error rates were removed and non-chimeric reads (82%) indicating biological features were tabulated for feature frequency amplicon sequence variants (ASVs). The lowest non-zero frequency of 10 was set to filter the feature ASV table. Phylogenetic classification of ASVs into different taxa levels was performed using the qiime2 “classify consensus- blast” plug-in against SILVA 138 release (34). The feature table collapsed with taxonomy and subsequently removed mitochondrial and chloroplast sequences (<1% of reads). There were variations in Clostridium classification, and as a result, these variations were classified as “Clostridium sensu stricto 1-9” throughout the dataset. To make a homogeneous dataset for Clostridium, we renamed all “Clostridium sensu stricto 1-9” as “Clostridium”, and other non-classified reads into the “Unclassified” bacterial group. The final set of data was normalized (also called “rarefaction”) at an even depth of 15,236 for bacterial community analysis. Metagenome prediction of functional features from 16S rRNA data was performed with the PICRUSt2 pipeline (35).
2.11 Diversity and composition analysis
The ASV table, taxonomy, and metadata files were imported into R software (v4.22) for diversity and composition analysis (36). The number of shared and unique taxa were calculated using the MicEco package (37). Species richness (observed), Chao1, Shannon, and Simpson diversity were considered for alpha-diversity measurements. Beta-diversity was performed as a UniFrac distance metric (Unweighted and Weighted) and PERMANOVA was conducted for the visualization of feeding effects on beta-dispersion with 1,000 permutations using vegan and phyloseq (38) R packages. The distance between samples for a group was calculated as the “Bray–Curtis “ distance. We considered 1% taxa abundance per sample as a threshold for composition analysis at the phylum and genus level (39). Further analysis of differential abundance between groups was performed as Linear Discriminant Analysis (LDA) using the MicrobiomeMarker R package (40). An LDA cutoff value of 2.0 and a p-value of <0.05 were considered as statistically significant for compositional difference analysis.
2.12 Statistical analysis
Data were assessed for normality using the Shapiro–Wilk test and then analyzed with two-tailed Student’s t-tests and two-way analysis of variance (ANOVA). Two-tailed Student’s t-tests were applied to compare the effects of GE diet to control. The total RBC count, along with its cellular and nuclear abnormalities, was analyzed using two-way ANOVA. Additionally, alpha-diversity, beta-diversity, and compositional log-fold changes between the two dietary groups in microbiome composition were analyzed using two-tailed t-tests. Gut microbiome analysis was carried out using the R software. A p-value of <0.05, <0.01, and <0.001were considered statistically significant between the two dietary groups of GE and CON.
3 Results
3.1 Growth performances, feed utilization, and muscle health
Nile tilapia fed the GE diet showed a significant improvement in growth performance and feed utilization compared to the CON (Figures 3A–E, p < 0.05). However, the somatic indices such as hepatosomatic index and viscerasomatic index were not influenced by the GE supplementation (Figures 3F, G, p > 0.05). Additionally, there was no significant difference in the survival rate between the two dietary groups (Figure 3H). The higher growth performance was supported by the enhanced dorsal muscle fiber diameter in fish fed the GE diet (Figures 3I–K).
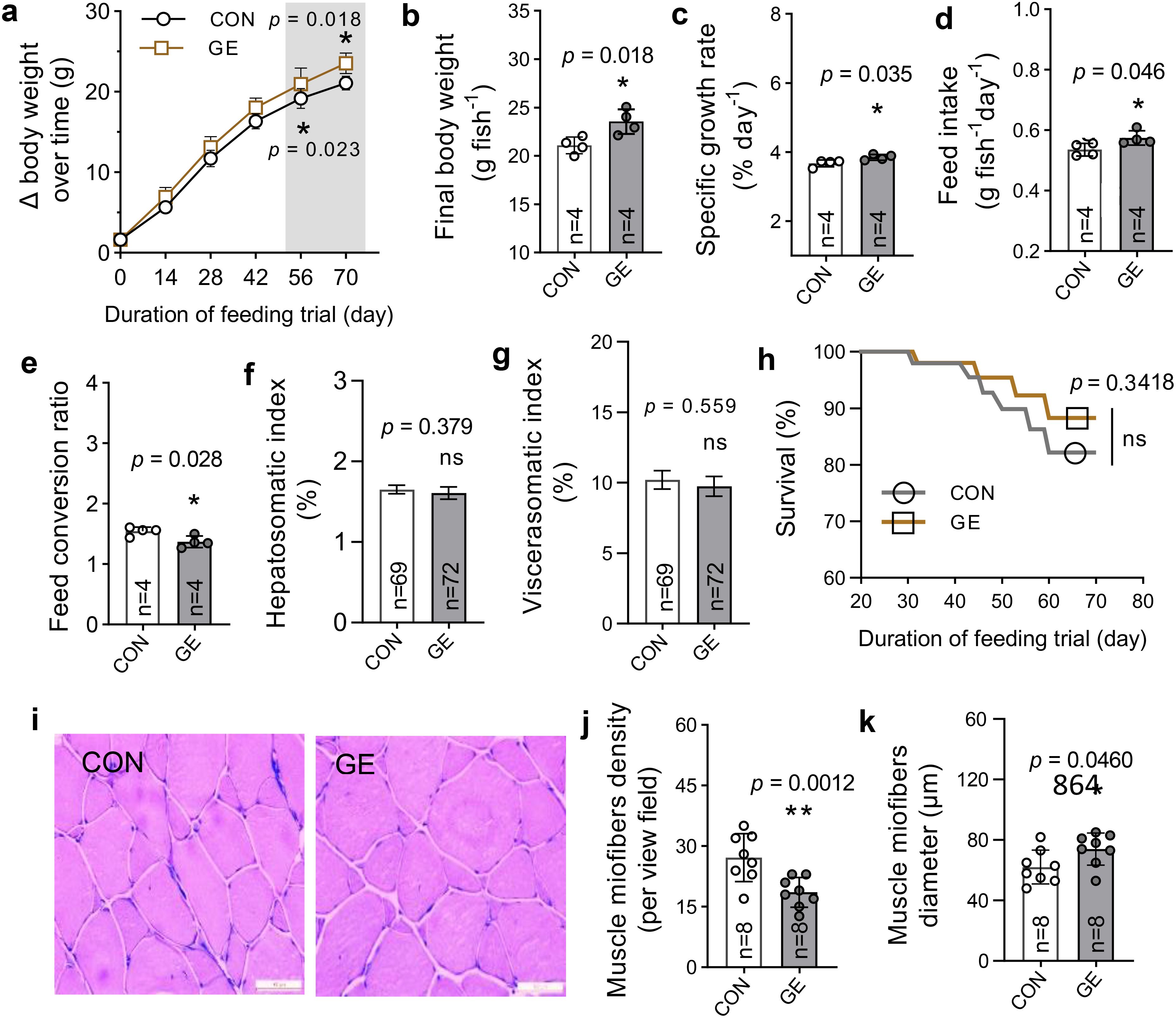
Figure 3. Growth and muscle health of Nile tilapia fed Gracilaria tenuistipitata extract for 70 days. (A–H) Growth performance, feed utilization, somatic indices, and survival of fish. (I) Transverse section of dorsal muscle microstructure (×200, H&E). (J, K) The myofiber development in fish in terms of fiber diameter and density. The data are presented as mean ± SD. Asterisks * and ** above the bars indicate significant differences between the two dietary groups of GE and CON, as determined by an unpaired t-test at p < 0 <.05 and p < 0.01, respectively. ns, non-significant; GE, Gracilaria extract; CON, control.
3.2 Liver health and adipocyte distribution
Hepatic lipid droplets significantly reduced in the GE-fed fish compared to the control, while liver weight exhibited no significant difference between the dietary groups (Figures 4A–C). Likewise, the hepatic enzymes AST and ALT were lower in GE-fed fish compared to control-fed fish, whereas cholesterol, triglyceride, and glycogen levels were not affected by seaweed supplementation (Figures 4D–H). Adipocyte numbers and fatty tissue diameter in fish fed the control diet were significantly decreased in comparison to fish fed the control diet (Figures 4I–K). The frequency of adipocyte diameter increased in GE-fed fish up to 50 μm, while adipocytes in fish fed the CON diet were greater in diameter (>50 to 200 μm) (Figure 4L).
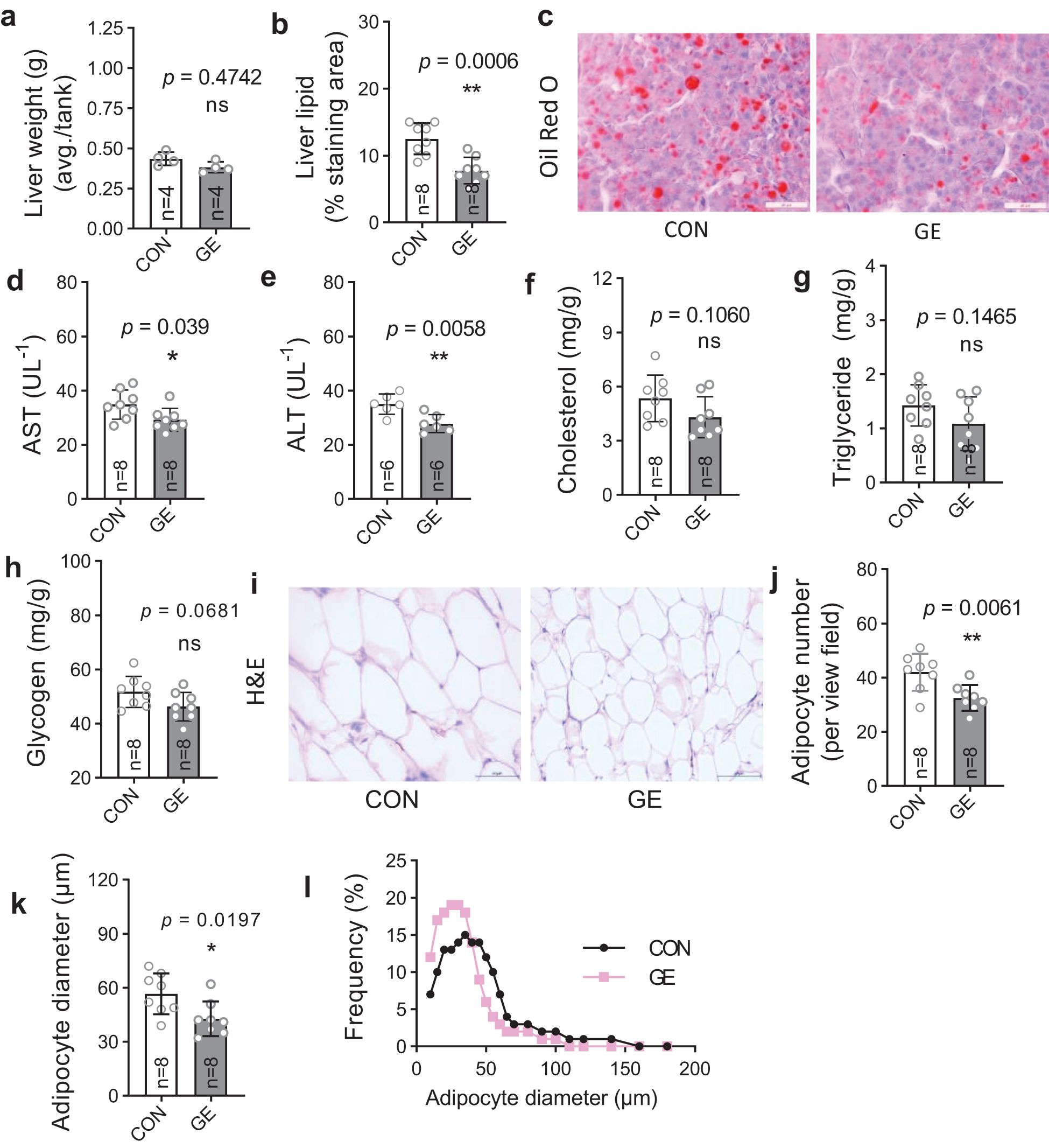
Figure 4. Liver health and intraperitoneal fat distribution in Nile tilapia fed Gracilaria tenuistipitata extract in varying water temperatures for 70 days. (A, B) Liver weight and quantification of lipid droplet area in liver of Nile tilapia. (C) Representative Oil Red O-stained liver histology showing lipid droplets (n = 8, magnification 50×). (D-H) Quantification of serum AST, ALT, cholesterol, triglyceride, and glycogen levels as liver health indicator. (I) Histomorphometry of intestinal adipocytes (n = 8, 10 fields per section, magnification 50×, H&E). (J–L) Quantitative image analysis for adipocyte distribution in terms of average adipocyte numbers, diameter (μm), and frequency (%). Data are the mean ± SD (standard deviation). An unpaired t-test is used to compare the results from GE-fed fish to control at *p < 0.05 and **p < 0.01. ns, non-significant; GE, Gracilaria extract; CON, control.
3.3 Antioxidant status, immunity, and gene expression
The level of MDA significantly reduced in fish fed the GE-supplemented diet while CAT and SOD levels exhibited no difference between the two dietary groups (Figures 5A–C, p < 0.05). The lysozyme activity was enhanced in the GE group compared to the CON group (Figure 5D). Dietary GE supplementation significantly upregulated the expression of tight junction proteins (occludin, claudin1, and ZO-1) and the antioxidant gene, nrf2, while a downregulation of hsp70 was observed in the GE group compared to the CON group. The pro-inflammatory cytokines tnf-α and il-1β were found unaffected by seaweed supplementation (Figure 5E, p > 0.05).
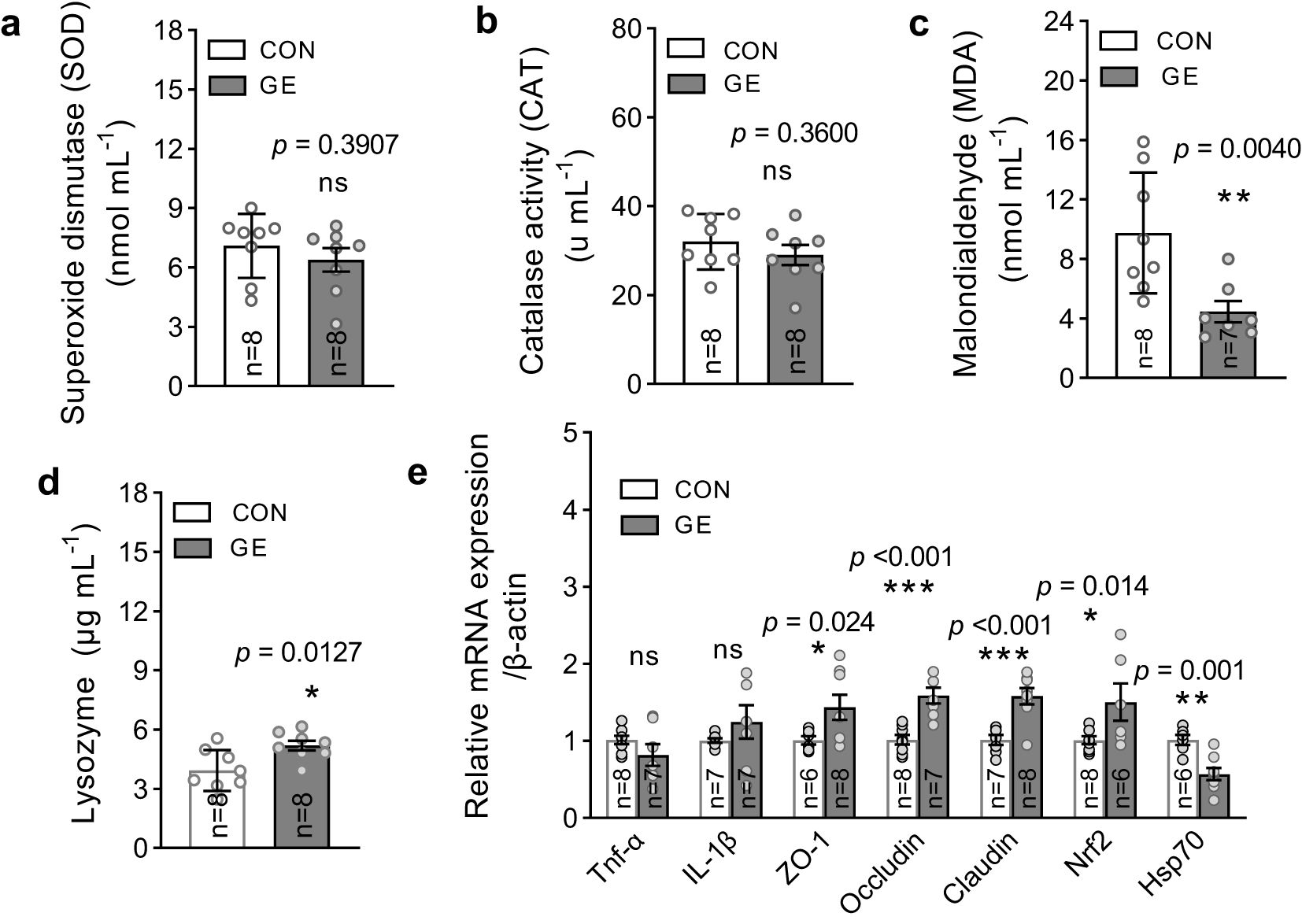
Figure 5. Antioxidant enzymes, lysozyme activity, and mRNA expression of Nile tilapia fed a diet supplemented with Gracilaria tenuistipitata extract in varying water temperatures for 70 days. (A–C) Quantification of oxidative stress through MDA, SOD, and CAT activities in fish liver after 10 weeks of feeding trial. (D) Immune response in terms of lysozyme activity in blood serum. (E) The relative quantification of mRNA expression of pro-inflammatory cytokines (tnf-α and il-1β), antioxidant gene (nrf2), tight junction genes (ZO-1, occludin, and claudin1), and heat shock protein 70 (hsp70) in the hind gut of fish. The data are presented as mean ± SD. Asterisks (*, **, and ***) indicate significant differences between the two dietary groups, control, and GE (Gracilaria extract), as determined by an unpaired t-test at p < 0 <.05, p < 0 <.01, and p < 0 <.001, respectively. ns, non-significant; GE, Gracilaria extract; CON, control.
3.4 Blood RBC level and cellular and nuclear abnormalities in response to temperature stress
RBC count and cellular and nuclear abnormalities were found significantly different by both the dietary groups and temperatures (Figure 6, p < 0.05). Fish fed the GE-supplemented diet significantly enhanced the RBC level when compared to the control. Likewise, the total frequencies of RBC cellular and nuclear abnormalities were found significantly lowered in the control group when compared to the control. However, fish at 35°C post-temperature stress produced more cellular and nuclear abnormalities compared to pre-temperature stress and post- temperature adaptation at 28°C and 32°C, respectively.
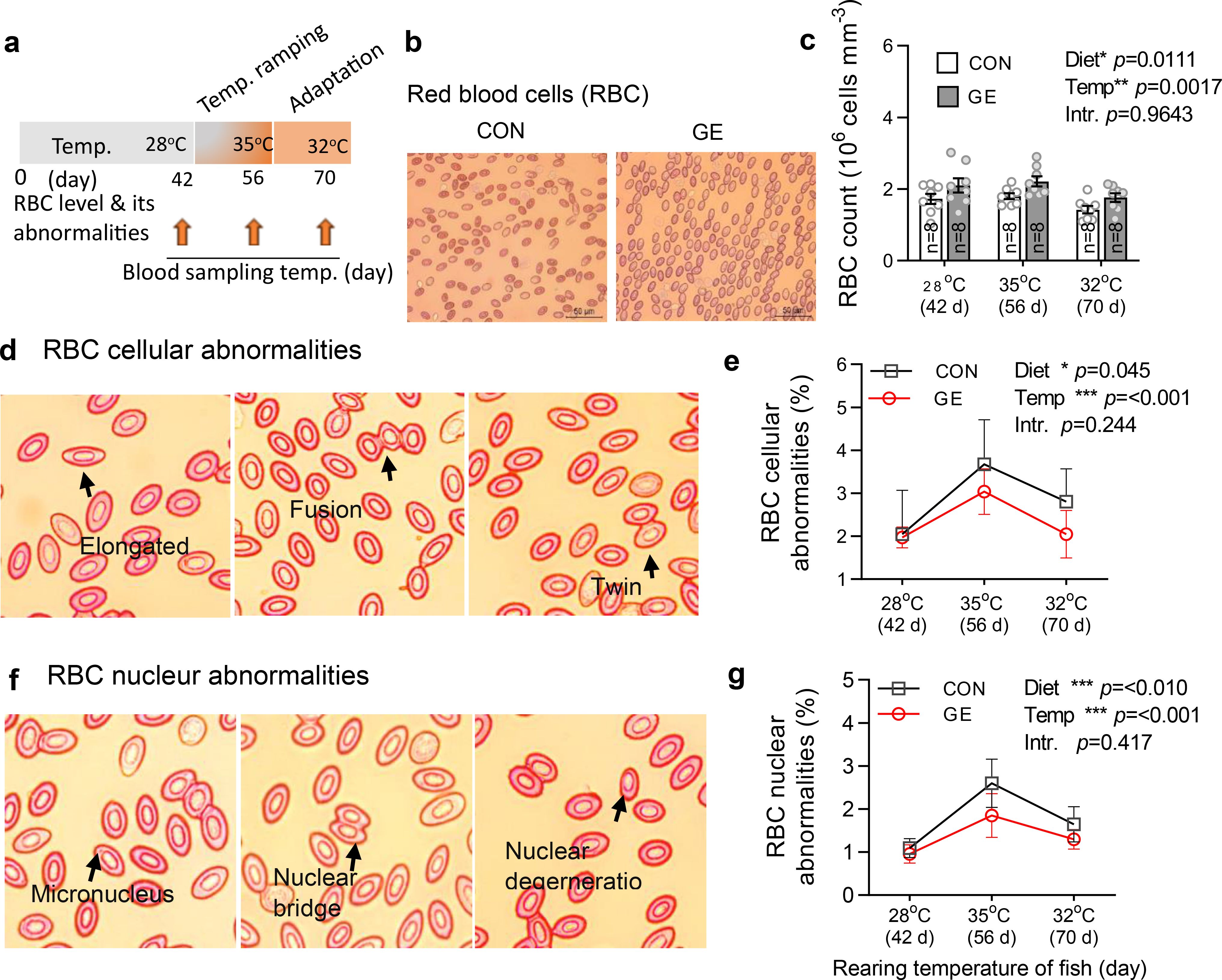
Figure 6. Effects of Gracilaria extract (GE)-supplemented diet on blood RBC level and its cellular and nuclear abnormalities in Nile tilapia in varying temperatures of 28°C, 35°C, and 32°C at days 42, 56, and 70, respectively. (A) Timeline of blood sampling at various temperatures during the feeding trial. (B, C) RBC level and its cellular and nuclear abnormalities in fish at 42 days (pre-temperature stress at 28°C), 56 days (post-temperature stress at 35°C), and 70 days (adaptation at 32°C). (D, E) representative H&E-stained blood histology showing RBC cellular abnormalities (elongated, fusion, and twin) and their quantification in GE and CON fed fish. (F, G) RBC nuclear abnormalities (micronucleus, nuclear bridge, and nuclear degeneration) and their quantification in fish fed GE and CON. Data are presented as mean ± SD. Asterisks (*, **, and ***) indicate significant differences between dietary groups and temperatures, determined by two-way ANOVA at p < 0.05, p < 0.01, and p < 0.001, respectively. GE, Gracilaria extract; CON, control; Temp, temperature; Intr, interaction.
3.5 Sequence statistics and diversity of gut microbiota
A total of 322, 761-bp quality reads were obtained after trimming, with an average of 20,172.6 ± 941.7 and ranging from 16,860 to 30,244 bp that were classified into 1,503 ASVs, six phyla, and 455 genera. The average good’s coverage index value of 0.998 and plateaued rarefaction curve were observed, indicating adequate depth and saturation level of all study sequences (Figure 7A, Supplementary Figure S2). Only 63 ASVs were shared between CON and GE diet groups while the latter generated 548 additional unique ASVs compared to 218 in CON (Figure 7B). Consistent with ASVs, species diversity (observed ASVs and Chao index) and Shannon and Simpson diversities reflecting richness and evenness of top abundant taxa were significantly higher in the GE group, compared to CON (Figure 7C). Distinct bacterial communities in terms of bacterial presence–absence and relative abundance were observed between CON and GE diet groups in the beta ordination PCoA plot. Significant R and p-values in PERMANOVA indicate the influence of dietary GE in the gut bacterial diversity in terms of shifting in relative abundance of top abundant taxa and augmenting rare bacterial communities compared to the CON group (Figures 7D, E). The Bray–Curtis distance showed more balance variation in abundance for bacterial communities in the GE diet compared to CON feed (Figure 7F).
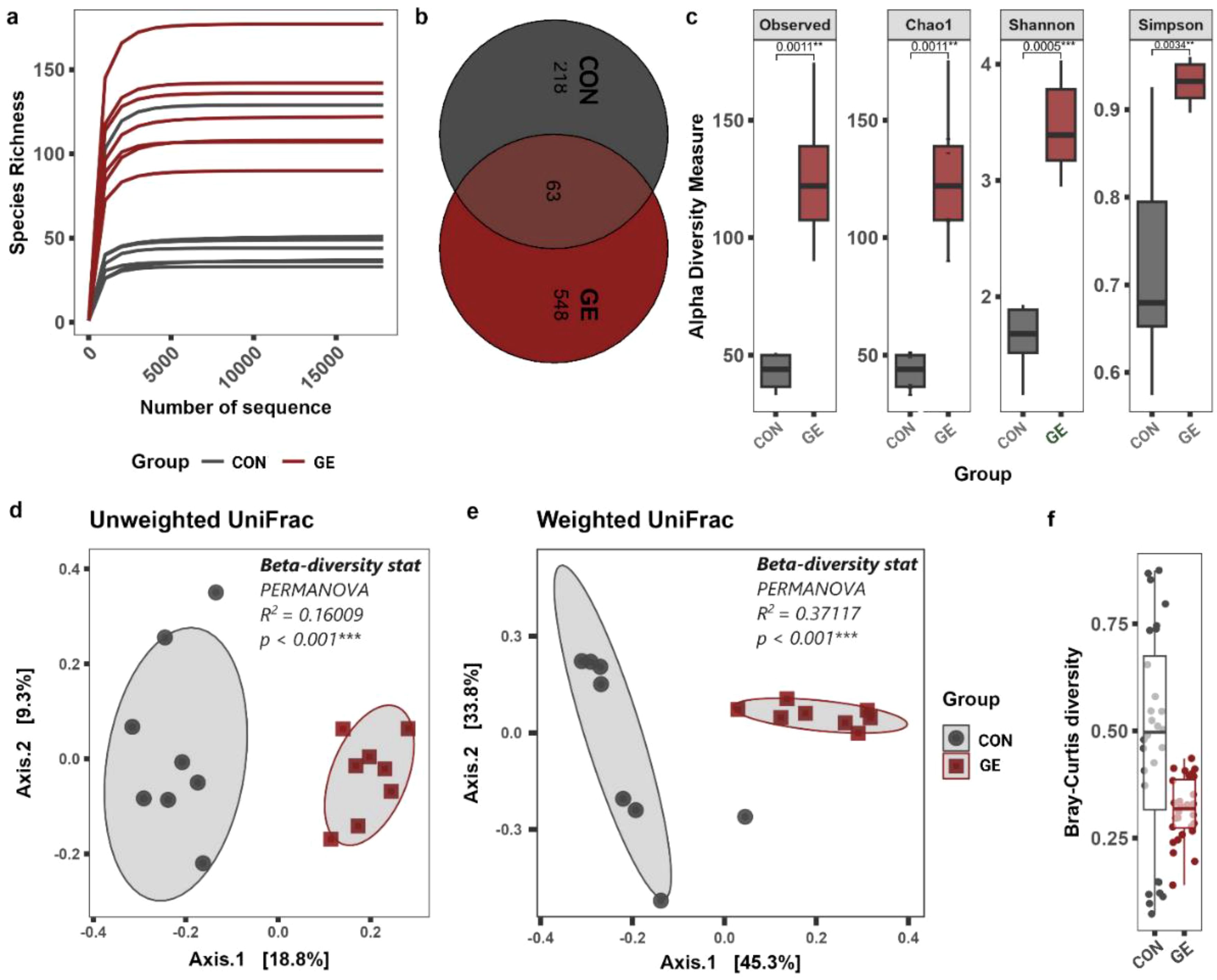
Figure 7. Effects of seaweed extract-supplemented diet on alpha-/beta-diversity of gut microbiota in Nile tilapia. (A) Rarefaction curve showing the depth and saturation level of study samples. (B) Number of shared and unique taxa. (C) Alpha-diversity measurements in terms of observed species, Chao1, Shannon, and Simpson diversity index. (D, E) Beta- diversity PCoA plot based on unweighted and weighted UniFrac distance matric. (F) The normalized pattern of Bray–Curtis distance represents the patterns of community distributions for CON and GE diets. Asterisks (** and ***) above the bars indicate significant differences between the two dietary groups of CON and GE determined by an unpaired t-test at p < 0 <.01, and p < 0 <.001, respectively. GE, Gracilaria extract; CON, control.
3.6 Gut microbial composition
At the phylum level, Proteobacteria represented approximately half of the classified reads (45%) in both groups —37.8% in CON and 53.8% in GE, while Fusobacteriota (28%) and Actinobacteria (28.7%) composed 56.7% of reads in the CON diet. Firmicutes (13.8%) had a higher abundance than Fusobacteria (14.8%) and Actinobacteria (12.7%) in the fish gut-fed GE diet. Firmicutes abundance was only 2.6% in the CON diet, indicating the influential impact of GE diet on this phylum. Alongside Firmicutes and Bacteroidota, abundance also increased from <0.5% in CON to 3.8% in the GE diet (Figure 8A). At the genus level, Aeromicrobium, Escherichia-Shigella, and Cetobacterium comprised 84.6% of the total reads in fish fed the CON diet while Pseudomonas (32.3%) was the most abundant bacterial group in fish fed the GE diet, followed by Escherichia-Shigella (14.5%), Cetobacterium (13.3%), Aeromicrobium (4.9%), Cutibacterium (3.6%), and Sphingobacterium (2.2%) (Figure 8B). Dietary GE provision significantly promoted the abundance of Bacteroidota, Campilobactorota, Desulfobacterota, and Firmicutes at the phylum level compared to Nitrospirota in the CON diet (Figure 9A). At the order and genus level, Sphingobacterium, Prevotella, Lactobacilliles, Lactobacillus, Roseburia, Bifidobacterium, and Ruminococcus showed significantly higher abundance in the GE diet compared to Rhodopirellula and Rhizobiales in CON (Figure 9B, Supplementary Figure S3). The GE diet also increased abundance for some opportunistic pathogens including Streptococcus, Staphylococcus, and Corynebacterium (Figure 9C). A shift in bacterial abundance in the gut also modulates metabolic pathways in the dietary GE group, specifically amino acid biosynthesis and metabolism compared to glucose–sucrose metabolism in the CON group (Supplementary Figure S4). The microbial interaction based on ASV correlation showed the dominance of Proteobacteria and Firmicutes in the network. Some of the Proteobacteria and Firmicutes communities were found to be self-interactive (Figure 10A). Despite their high abundance, Actinobacteria and Fusobacteria were less interactive. Microbial co-occurrence network analysis revealed that Firmicutes led interactions in the community, despite the Proteobacteria-rich environment and their dominance in strong interactions. Firmicutes were associated with over 70% of medium and 50% of weak interactions, mostly with Bacteroidetes and Actinobacteria. Proteobacteria led the strong interactions and showed no involvement with Firmicutes (Figure 10B).
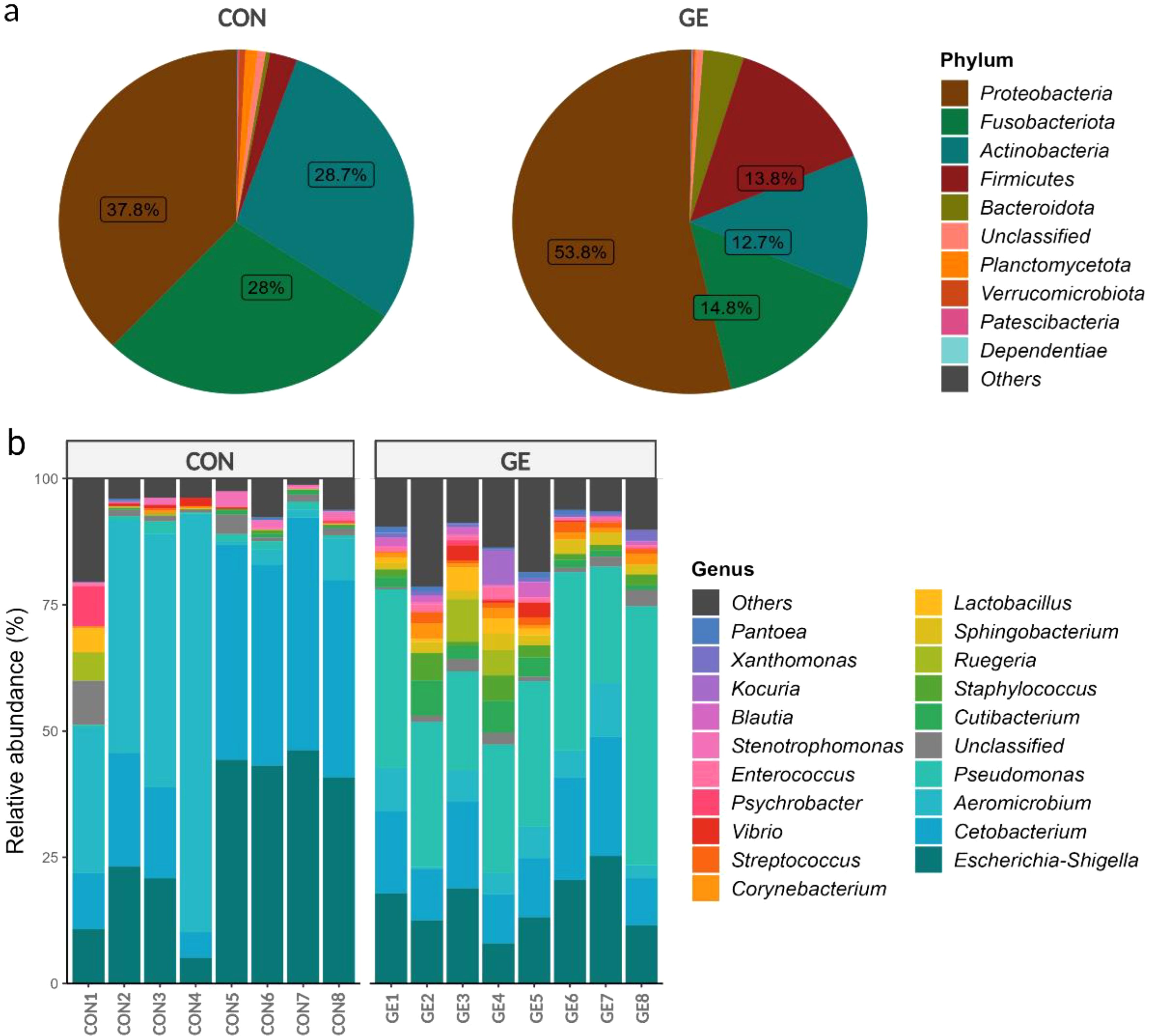
Figure 8. Relative abundance (≥1%) of bacteria in the hind gut of Nile tilapia. (A) Pie chart representing gut bacteria at the phylum level. (B) Bar plot representing gut bacteria at the genus level. GE, Gracilaria extract; CON, control.
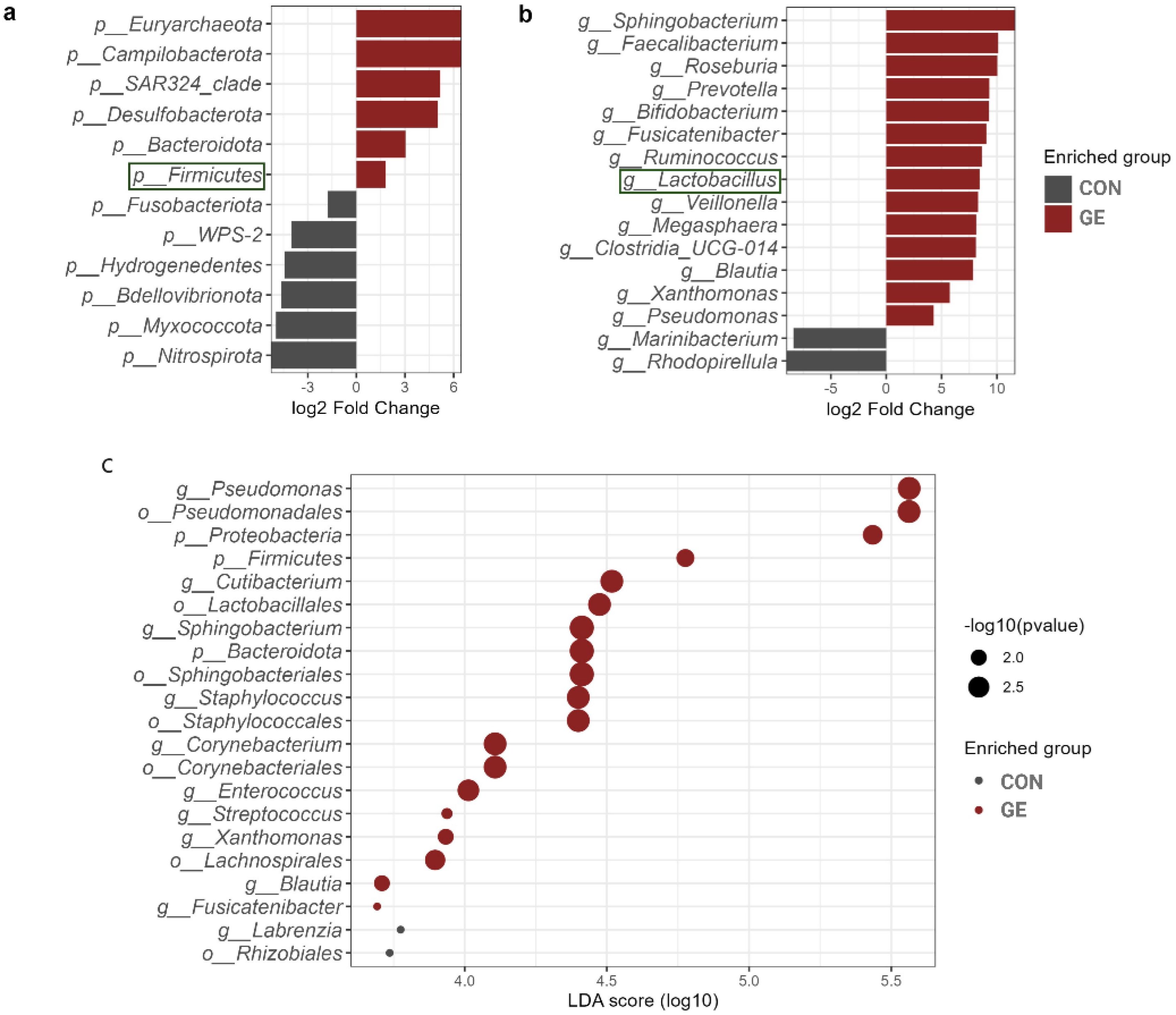
Figure 9. Differential abundant taxa in the hind gut of Nile tilapia. (A) Comparison of different phyla in the two dietary groups of GE and control. (B) Comparison of different genera in the two dietary groups of GE and control. The health beneficial genus is marked with green color. (C) Enriched phyla, order, and genera with log10 p-value. The X-axis indicates the LDA score with dots representing the significance level of p-values. Bigger dots indicate more significant p-value. GE, Gracilaria extract; CON, control.
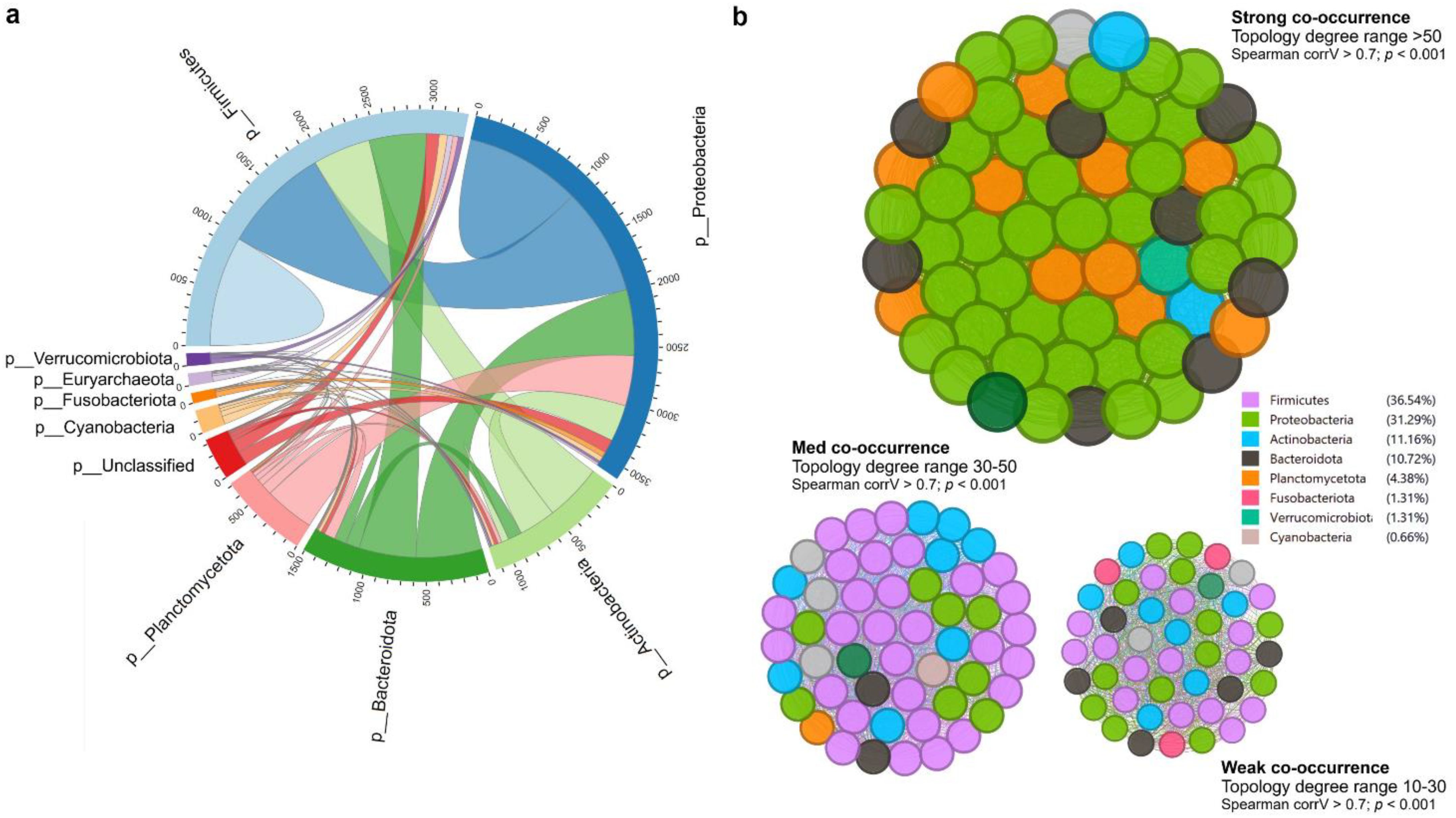
Figure 10. Microbial interaction and co-occurrence network. (A) Cladogram representing the correlation of amplicon sequence variants (ASVs) between two diets in terms of interactions. The number in the outer circular part indicates the average ASV counts per sample. (B) Co-occurrence network of bacterial community regarding the degree of interactions. The network was segregated based on topology wherein the size of the nodes represents the degree of interaction (10%–100%). The color code on the right side indicates the phyla and contributions to the interaction.
4 Discussion
Extreme temperature events caused by climate change have substantial impacts on aquatic ecosystems and can affect the aquaculture industry in various ways. These include changes in water temperature, reduced dissolved oxygen levels, and facilitating the spread of pathogens and parasites in farming environments (2). These challenges lead to increased stress and disease susceptibility and reduced growth rates in fish. Sustainable and responsible aquaculture practices, coupled with a broader commitment to mitigating temperature-induced stress, are essential for ensuring the resilience of fish farming in the face of global warming. Nutritional modulation and enrichment of aquafeeds can play a crucial role in mitigating the negative effects of acute temperature stress on aquaculture systems. Nowadays, the application of seaweeds and seaweed -based functional metabolites in aquafeeds has greatly attracted worldwide attention, demonstrating positive effects on stress reduction and improved disease resistance and overall health (14, 41). The findings of the present study demonstrate the potential of using GE in aquafeeds to counteract stress and improve growth performance while rearing at high temperature via the modulation of antioxidant status and gut microbiota composition in Nile tilapia.
Fish fed the GE-supplemented diet in the present study significantly improved the growth performance and feed utilization. These findings align with previous studies that have demonstrated the positive effects of incorporating seaweeds such as Gracilaria persica into fish diets, benefitting the growth performance of Atlantic salmon (42) and Persian sturgeon, Acipenser persicus (43), respectively. The improved growth realized in the present study may be due to the availability of nutrients (polyunsaturated fatty acids, favorable amino acids, minerals, and vitamins) and bioactive metabolites (phenolic, flavonoid, and β-carotene) in the seaweed extract that stimulated the digestion and absorption by fish (44, 45). Furthermore, the growth increment shown in this study may also be attributed to the presence of large amounts of polysaccharides and oligosaccharides present in seaweeds that act as prebiotics, thus positively increasing the digestion and assimilation of nutrients by enhanced activity of the beneficial bacteria (46). Moreover, the growth of fish has a close connection with the development of muscle that is attributed to its hyperplastic and hypertrophic fiber production (47, 48). Quantitative observations of muscle fiber morphology have been considered to be essential to evaluate muscle growth in nutritional research. Production of new muscle (hyperplasia) and the increment of existing muscle (hypertrophy) have been greatly stimulated by dietary composition (47). The increment of size and consequent decrease in the number of muscle fibers of Nile tilapia fed the GE diet in this study revealed the positive impacts of dietary GE, assisting in growth enhancement. In the current study, the FCR of Nile tilapia significantly improved due to the dietary supplementation of GE. This result is consistent with the study on Indian major carp, Labeo rohita, fed fucoidan-rich Sargassum wightii extract (49). The improved FCR could be attributed to the enhanced feed palatability and feed intake, which may have resulted from the activity of phenolic and flavonoid bioactive compounds in seaweed (50). Moreover, these biologically active compounds stimulate the secretion of important digestive enzymes such as amylase, lipase, and protease that significantly improve digestion and improve feed utilization (51).
The liver is an important organ that regulates the metabolism of essential nutrients and controls energy storage and utilization to maintain homeostasis (52). Aquafeeds high in fat and carbohydrates can lead to an increase in liver fat as well as larger adipocyte size (hypertrophy), causing the accumulation of body fat, while optimum feeding and proper nutrition can reduce adipocyte size (atrophy) as excess fat is utilized for energy (53). Therefore, understanding liver health and adipocyte tissue and their regulation is essential in studying dietary nutrition and related metabolic issues in fish. The significant reduction in liver fat coupled with the reduction of adipocyte size in fish fed the GE diet could be related to the reduction of AST and ALT enzyme activity levels compared to the control diet. This reduction in liver fat and adipocyte size in GE-fed fish may also be related to the abundance of intestinal microbiota, which may influence fat absorption by producing metabolites such as short-chain fatty acids and secondary bile acids, as well as pro-inflammatory bacterially derived factors such as lipopolysaccharides (54, 55). However, more research is needed to gain a comprehensive understanding of how GE affects liver lipid accumulation and adipose tissue quantity and size in fish.
Lysozyme activity is a vital component of fish innate immune system to defend against infections (56). The current study revealed higher lysozyme activity in fish fed GE, suggesting a boosting immunity possibly triggered by active compounds including polyphenols and polysaccharides present in Gracilaria sp. (57). Moreover, this finding can be attributed to the seaweed’s role in activating the immune response and scavenging excessive reactive oxygen metabolites (ROS). In accordance with the current study, the administration of Gracilaria sp. extract increased lysozyme activity in European seabass (58). Fish under stressful conditions often experience oxidative stress, which is caused by an imbalance between the production and elimination of ROS within cells (59). The disruption of scavenging capacity results in lipid peroxidation, ROS buildup, and rupturing the lipid membrane of body cells (60). The DNA of immune cells is likewise harmed by this interruption. In this instance, the body secretes SOD, CAT, and GPx, which are enzymatic and non-enzymatic antioxidative responses that reduce ROS and increase antioxidative capacity (61). However, failing to do so leads to elevated lipid peroxidation and the generation of MDA (62). The current study revealed that dietary GE-based diet significantly reduced the MDA level in fish confirming the stress-inhibitory role of dietary GE. These results align with other studies that have found dietary supplementation of Sargassum horneri, and a mixture of seaweed (i.e., Ulva lactuca, Jania rubens, and Pterocladia capillacea) extract induced significantly lower MDA levels in black sea bream, Acanthopagrus schlegelii, and striped catfish, Pangasianodon hypophthalmus (63, 64). The ameliorative capacity of the seaweed extract was associated with the high content of bioactive substances such as flavonoids and phenolic substances and could act as natural antioxidants and play an important role to neutralize free radicals and improve stress resilience (65, 66). In line with the present results, we also observed an increased upregulation of tight junction proteins and antioxidant gene in the hind gut of GE-fed Nile tilapia. These data suggested that GE supplementation protects gut permeability and translocation of pathogens in fish while improving nrf2 pathway and strengthening antioxidant defense. Likewise, in the present study, hsp70 protein expression was downregulated in the GE group compared to the CON group, indicating stress-mediating effects of GE in fish under adverse conditions.
RBCs play a crucial role in oxygen transport throughout the body, and an increase in their count can lead to higher levels of haemoglobin (67). This allows fish to efficiently transport oxygen from the gills to various tissues and organs. As a result, fish are likely to cope better with stressors, such as changes in water temperature, pollution, or other environmental challenges. In the present study, a significant increase in RBC levels was found in fish fed a GE diet. Similar findings have been observed in striped catfish, where a mixture of seaweeds (U. lactuca, J. rubens, and P. capillacea) increased the RBC count (63). Another study showed that a Sargassum angustifolium algae extract-supplemented diet enhanced the number of RBCs in rainbow trout (68). These results suggest that supplementing the diet with seaweed may enhance erythropoietin production and erythrocytic stability, which may increase the RBC level in fish (69). Furthermore, fish fed the GE diet in the present study demonstrated a significant decrease in RBC cellular and nuclear abnormalities, indicating the beneficial effects of seaweed in mitigating stress during extreme warm exposure. Warm stress generally results in transmembrane alterations and metabolic inhibition that trigger the DNA-damaging mitochondrial caspase-3, which leads to erythrocytic cellular and nuclear anomalies (70–73). Furthermore, elevated water temperatures can also damage DNA by causing the release of DNase enzymes from lysosomes and thermally inactivating the enzymes that repair DNA, which can change the shape of cells (74). Significantly higher RBC cellular and nuclear abnormalities observed in fish fed the control diet could be due to the excess lipid peroxidation that considerably increased the permeability and decreased the symmetry of the erythrocyte cell membrane, which is also responsible for higher abnormalities in erythrocytes (75). Nevertheless, the higher RBC abnormalities with the control diet are justified by the much higher overexpression of hsp70 identified in these fish when compared to GE-fed fish.
The present study found that Nile tilapia fed a diet supplemented with GE exhibited a healthier gut, as indicated by the higher diversity and abundance of bacterial populations and enrichment of phylum Firmicutes, family Lactobacillales, and genus Lactobacillus. These bacteria are mostly considered beneficial for fish and other aquatic animals (76). A study by Cui et al. (77) has shown that supplementing the diet with fucoidan, a polysaccharide extracted from brown-seaweed Undaria pinnatifida, enhanced the activity of digestive enzymes and regulated the microbial populations and increased beneficial bacteria in fish. The increased abundance of Lactobacillus is of considerable interest since an enrichment in these bacteria is associated with improved health (76). The increased bacterial diversity and higher number of Lactobacillus in Nile tilapia fed GE may be due to the presence of bioactive compounds (e.g., short-chain peptides, polyphenols, and polysaccharides) in seaweed, which might have induced the colonization of health-promoting bacteria. Polysaccharides in seaweed are a preferential substrate for lactic acid bacteria (78), as demonstrated in the gut of zebrafish, Danio rerio (79), and barramundi, Lates calcarifer (80). Additionally, Lactobacillus has been linked to various protective mechanisms in the host against pathogens, such as producing bacteriocin to remove pathogens from the gut epithelium (81). In the present study, feeding fish with seaweed extract decreased the abundance of opportunistic pathogen Escherichia-Shigella (p < 0.05, LDA < 2.0) in Nile tilapia gut. This result is supported by Sargassum dentifolium extract that reduced the abundance of Escherichia coli in the gut of king prawn, Litopenaeus vannamei (82). Studies indicate that increasing environmental temperatures have adverse effects on fish, leading to reduced digestive efficiency, lower gut bacterial abundance, and higher pathogenic bacteria with negative implications for the host’s health and physiology (83, 84). Despite the augmentation of Firmicutes and Lactobacillus, GE also influenced the colonization of some opportunistic clinical pathogens including Streptococcus, Staphylococcus, and Corynebacterium that needs further investigation. Nevertheless, the higher diversity of bacteria in the GE-fed group compared to the control group indicates the effects of seaweed even at high temperatures. Future research should focus on understanding the impact of temperature variations on the fish microbiome and the consequences of microbial changes on fish performance.
5 Conclusion
This study demonstrates that dietary Gracilaria extract (1% of feed) supplementation could counteract the adverse effects of temperature-induced oxidative stress in fish through (1) enhancing the antioxidant capacity indicated by the upregulation of nrf2 expression and reduction of MDA production; (2) protecting gut permeability by improving the expression of tight junction proteins (occludin and claudin1); (3) increasing the RBC count, which could indicate a higher blood flow in the circulatory system; (4) reduction of stress levels indicated by the reduction of hsp70 mRNA expression; and (5) increasing the number of potentially health-promoting bacteria in fish gut. A summary of the beneficial role of seaweed extract in the gut–liver axis and its underlying mechanisms is depicted in Figure 11. The present findings reinforce the prospect of seaweed extracts as an effective supplement in aquafeeds to attenuate temperature-induced stress in aquaculture production during summer in the face of global warming.
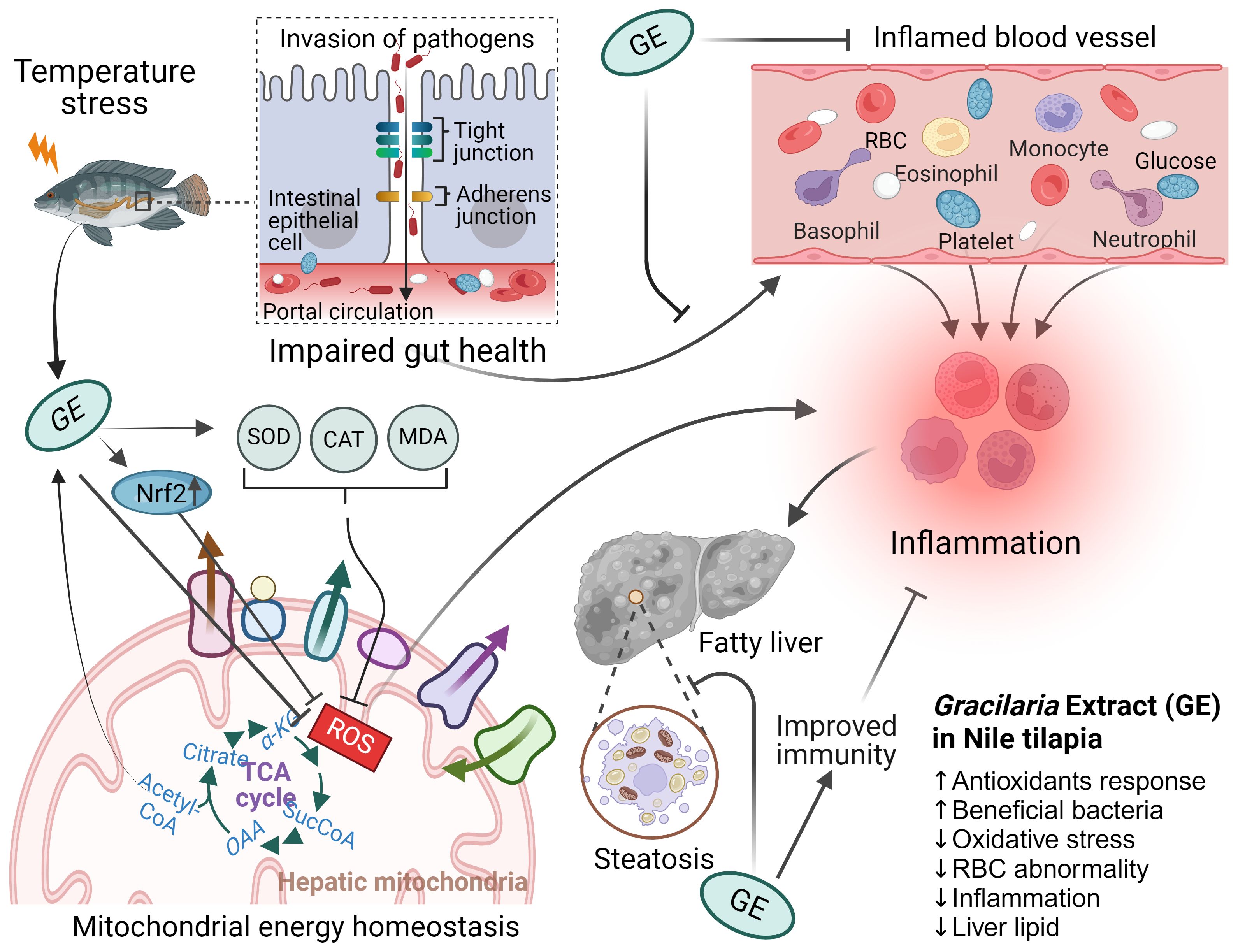
Figure 11. The possible roles of Gracilaria extract (GE) on the gut–liver axis and its underlying mechanisms in Nile tilapia. Nile tilapia under acute temperature stress is susceptible to pathogen invasion through a leaky gut caused inflammation that compromises immune function of fish. Dietary supplementation with GE increases the expression of antioxidant gene (nrf2) and reduces MDA level, which leads to improved mitochondrial function and energy production to help prevent inflammation and subsequent oxidative stress in fish. The GE diet has also been found to reduce liver lipid content, an indication of improved health of fish. The figure includes up and down arrows, denoting increased and decreased cellular functions and systems, respectively.
Data availability statement
The datasets presented in this study can be found in online repositories. The names of the repository/repositories and accession number(s) can be found in the article/Supplementary Material.
Ethics statement
The animal study was approved by The experimental design, husbandry protocol and fish sampling procedures were performed in this study was approved and guided by animal ethics committee (AEC), Patuakhali Science and Technology University, Bangladesh (Approval Number: AEC-FoF/2022/002). The study was conducted in accordance with the local legislation and institutional requirements.
Author contributions
MS: Conceptualization, Data curation, Formal analysis, Funding acquisition, Investigation, Methodology, Project administration, Visualization, Writing – original draft, Writing – review & editing. PF: Conceptualization, Funding acquisition, Project administration, Software, Validation, Visualization, Writing – review & editing. MF: Formal analysis, Methodology, Resources, Software, Validation, Visualization, Writing – review & editing. DF: Conceptualization, Funding acquisition, Project administration, Resources, Software, Supervision, Validation, Writing – review & editing.
Funding
The author(s) declare that financial support was received for the research, authorship, and/or publication of this article. This work was financially supported by the Alfred Deakin Postdoctoral Research Fellowship [RM42943, 2022] to MS from the Faculty of Science, Engineering and Built Environment, Deakin University, Australia.
Conflict of interest
The authors declare that the research was conducted in the absence of any commercial or financial relationships that could be construed as a potential conflict of interest.
Publisher’s note
All claims expressed in this article are solely those of the authors and do not necessarily represent those of their affiliated organizations, or those of the publisher, the editors and the reviewers. Any product that may be evaluated in this article, or claim that may be made by its manufacturer, is not guaranteed or endorsed by the publisher.
Supplementary material
The Supplementary Material for this article can be found online at: https://www.frontiersin.org/articles/10.3389/fimmu.2024.1471261/full#supplementary-material
References
1. Huang M, Ding L, Wang J, Ding C, Tao J. The impacts of climate change on fish growth: A summary of conducted studies and current knowledge. Ecol Indic. (2021) 121:106976. doi: 10.1016/j.ecolind.2020.106976
2. Islam MJ, Kunzmann A, Slater MJ. Responses of aquaculture fish to climate change-induced extreme temperatures: A review. J World Aquac Soc. (2022) 53:314–66. doi: 10.1111/jwas.12853
3. Nowicki R, Heithaus M, Thomson J, Burkholder D, Gastrich K, Wirsing A. Indirect legacy effects of an extreme climatic event on a marine megafaunal community. Ecol Monogr. (2019) 89:e01365. doi: 10.1002/ecm.2019.89.issue-3
4. Fan X, Qin X, Zhang C, Zhu Q, Chen J, Chen P. Metabolic and anti-oxidative stress responses to low temperatures during the waterless preservation of the hybrid grouper (Epinephelus fuscogutatus♀ × Epinephelus lanceolatus♂). Aquaculture. (2019) 508:10–8. doi: 10.1016/j.aquaculture.2019.04.054
5. Feidantsis K, Pörtner HO, Giantsis IA, Michaelidis B. Advances in understanding the impacts of global warming on marine fishes farmed offshore: Sparus aurata as a case study. J Fish Biol. (2021) 98:1509–23. doi: 10.1111/jfb.14611
6. Goikoetxea A, et al. Genetic pathways underpinning hormonal stress responses in fish exposed to short-and long-term warm ocean temperatures. Ecol Indic. (2021) 120:106937. doi: 10.1016/j.ecolind.2020.106937
7. Gupta A, et al. Immunomodulation by dietary supplements: A preventive health strategy for sustainable aquaculture of tropical freshwater fish, Labeo rohita (Hamilton, 1822). Rev Aquac. (2021) 13:2364–94. doi: 10.1111/raq.12581
8. Dawood MA, Koshio S, Abdel-Daim MM, Van Doan H. Probiotic application for sustainable aquaculture. Rev Aquac. (2019) 11:907–24. doi: 10.1111/raq.2019.11.issue-3
9. Thepot V, Campbell AH, Rimmer MA, Paul NA. Meta-analysis of the use of seaweeds and their extracts as immunostimulants for fish: a systematic review. Rev Aquac. (2021) 13:907–33. doi: 10.1111/raq.12504
10. Silva-Brito F, et al. Fish performance, intestinal bacterial community, digestive function and skin and fillet attributes during cold storage of gilthead seabream (Sparus aurata) fed diets supplemented with Gracilaria by-products. Aquaculture. (2021) 541:736808. doi: 10.1016/j.aquaculture.2021.736808
11. Miyashita K, Mikami N, Hosokawa M. Chemical and nutritional characteristics of brown seaweed lipids: A review. J Funct Foods. (2013) 5:1507–17. doi: 10.1016/j.jff.2013.09.019
12. Gupta S, Abu-Ghannam N. Bioactive potential and possible health effects of edible brown seaweeds. Trends Food Sci Technol. (2011) 22:315–26. doi: 10.1016/j.tifs.2011.03.011
13. Čagalj M, Skroza D, Tabanelli G, Özogul F, Šimat V. Maximizing the antioxidant capacity of Padina pavonica by choosing the right drying and extraction methods. Processes. (2021) 9:587. doi: 10.3390/pr9040587
14. Siddik MAB, Francis P, Rohani MF, Azam MS, Mock TS, Francis DS. Seaweed and seaweed-based functional metabolites as potential modulators of growth, immune and antioxidant responses, and gut microbiota in fish. Antioxid (Basel). (2023) 12(12):2066. doi: 10.3390/antiox12122066
15. Michalak I, et al. Antioxidant effects of seaweeds and their active compounds on animal health and production–a review. Vet Q. (2022) 42:48–67. doi: 10.1080/01652176.2022.2061744
16. Cherry P, et al. Prebiotics from seaweeds: an ocean of opportunity? Mar Drugs. (2019) 17(6):327. doi: 10.3390/md17060327
17. Chen X, et al. In vitro prebiotic effects of seaweed polysaccharides. J Oceanol Limnol. (2018) 36:926–32. doi: 10.1007/s00343-018-6330-7
18. Ferreira M, Abdelhafiz Y, Abreu H, Silva J, Valente LMP, Kiron V. Gracilaria gracilis and Nannochloropsis oceanica, singly or in combination, in diets alter the intestinal microbiota of European seabass (Dicentrarchus labrax). Front Mar Sci. (2022) 9:1001942. doi: 10.3389/fmars.2022.1001942
19. Dawood MA, Koshio S. Application of fermentation strategy in aquafeed for sustainable aquaculture. Rev Aquac. (2020) 12:987–1002. doi: 10.1111/raq.12368
20. Wilczynski W, et al. Metagenomic analysis of the gastrointestinal microbiota of Gadus morhua callarias L. originating from a chemical munition dump site. Toxics. (2022) 10:206. doi: 10.3390/toxics10050206
21. Zhang X, et al. Effects of dietary supplementation of Ulva pertusa and non-starch polysaccharide enzymes on gut microbiota of Siganus canaliculatus. J Oceanol Limnol. (2017) 36:438–49. doi: 10.1007/s00343-017-6235-x
22. Naylor RL, et al. A 20-year retrospective review of global aquaculture. Nature. (2021) 591:551–63. doi: 10.1038/s41586-021-03308-6
23. Shahjahan M, Islam MJ, Hossain MT, Mishu MA, Hasan J, Brown C. Blood biomarkers as diagnostic tools: An overview of climate-driven stress responses in fish. Sci Total Environ. (2022) 843:156910. doi: 10.1016/j.scitotenv.2022.156910
24. Islam SM, et al. Elevated temperature affects growth and hemato-biochemical parameters, inducing morphological abnormalities of erythrocytes in Nile tilapia Oreochromis niloticus. Aquac Res. (2020) 51:4361–71. doi: 10.1111/are.v51.10
25. Thépot V, et al. Dietary inclusion of the red seaweed Asparagopsis taxiformis boosts production, stimulates immune response and modulates gut microbiota in Atlantic salmon, Salmo salar. Aquaculture. (2022) 546:737286. doi: 10.1016/j.aquaculture.2021.737286
26. Sobuj MKA, Islam MA, Haque MA, Islam MM, Alam MJ, Rafiquzzaman SM. Evaluation of bioactive chemical composition, phenolic, and antioxidant profiling of different crude extracts of Sargassum coriifolium and Hypnea pannosa seaweeds. J Food Meas Charact. (2020) 15:1653–65. doi: 10.1007/s11694-020-00758-w
27. Peixoto MJ, et al. Role of dietary seaweed supplementation on growth performance, digestive capacity and immune and stress responsiveness in European seabass (Dicentrarchus labrax). Aquac Rep. (2016) 3:189–97. doi: 10.1016/j.aqrep.2016.03.005
28. Siddik MA, Foysal MJ, Fotedar R, Francis DS, Gupta SK. Probiotic yeast Saccharomyces cerevisiae coupled with Lactobacillus casei modulates physiological performance and promotes gut microbiota in juvenile barramundi, Lates calcarifer. Aquaculture. (2022) 546:737346. doi: 10.1016/j.aquaculture.2021.737346
29. Rowlerson A, Mascarello F, Radaelli G, Veggetti A. Differentiation and growth of muscle in the fish Sparus aurata (L): II. Hyperplastic and hypertrophic growth of lateral muscle from hatching to adult. J Muscle Res Cell Motil. (1995) 16:223–36. doi: 10.1007/BF00121131
30. Chaklader MR, Siddik MA, Fotedar R, Howieson J. Insect larvae, Hermetia illucens in poultry by-product meal for barramundi, Lates calcarifer modulates histomorphology, immunity and resistance to Vibrio harveyi. Sci Rep. (2019) 9:16703. doi: 10.1038/s41598-019-53018-3
31. Siddik MAB, Howieson J, Partridge GJ, Fotedar R, Gholipourkanani H. Dietary tuna hydrolysate modulates growth performance, immune response, intestinal morphology and resistance to Streptococcus iniae in juvenile barramundi, Lates calcarifer. Sci Rep. (2018) 8:15942. doi: 10.1038/s41598-018-34182-4
32. Blanc M-C, Neveux N, Laromiguière M, Bérard M-P, Cynober L. Evaluation of a newly available biochemical analyzer: the Olympus AU 600. Clin Chem Lab Med. (2000) 38(5):465–75. doi: 10.1515/CCLM.2000.067
33. Livak KJ, Schmittgen TD. Analysis of relative gene expression data using real-time quantitative PCR and the 2(-Delta Delta C(T)) Method. Methods. (2001) 25:402–8. doi: 10.1006/meth.2001.1262
34. Quast C, et al. The SILVA ribosomal RNA gene database project: improved data processing and web-based tools. Nucleic Acids Res. (2012) 41:D590–6. doi: 10.1093/nar/gks1219
35. Caicedo HH, Hashimoto DA, Caicedo JC, Pentland A, Pisano GP. Overcoming barriers to early disease intervention. Nat Biotechnol. (2020) 38:669–73. doi: 10.1038/s41587-020-0550-z
36. Team RDC. R: A language and environment for statistical computing. (2010). Available online at: http://www.R-project.org/.
37. Liu C, Cui Y, Li X, Yao M. microeco: an R package for data mining in microbial community ecology. FEMS Microbiol Ecol. (2021) 97:fiaa255. doi: 10.1093/femsec/fiaa255
38. McMurdie PJ, Holmes S. phyloseq: an R package for reproducible interactive analysis and graphics of microbiome census data. PloS One. (2013) 8:e61217. doi: 10.1371/journal.pone.0061217
39. Siddik MAB, Fotedar R, Chaklader MR, Foysal MJ, Nahar A, Howieson J. Fermented Animal Source Protein as Substitution of Fishmeal on Intestinal Microbiota, Immune-Related Cytokines and Resistance to Vibrio mimicus in Freshwater Crayfish (Cherax cainii). Front Physiol. (2019) 10:1635. doi: 10.3389/fphys.2019.01635
40. Cao Y, Dong Q, Wang D, Zhang P, Liu Y, Niu C. microbiomeMarker: an R/Bioconductor package for microbiome marker identification and visualization. Bioinformatics. (2022) 38:4027–9. doi: 10.1093/bioinformatics/btac438
41. Siddik MAB, Francis DS, Islam SMM, Salini MJ, Fotedar R. Fermentation and fortification of Sargassum linearifolium with multi-strain probiotics improves mucosal barrier status, inflammatory response and resistance to Vibrio harveyi infection in barramundi Lates calcarifer. Aquaculture. (2025) 595:741502. doi: 10.1016/j.aquaculture.2024.741502
42. Kamunde C, Sappal R, Melegy TM. Brown seaweed (AquaArom) supplementation increases food intake and improves growth, antioxidant status and resistance to temperature stress in Atlantic salmon, Salmo salar. PloS One. (2019) 14:e0219792. doi: 10.1371/journal.pone.0219792
43. Adel M, Omidi AH, Dawood MA, Karimi B, Shekarabi SPH. Dietary Gracilaria persica mediated the growth performance, fillet colouration, and immune response of Persian sturgeon (Acipenser persicus). Aquaculture. (2021) 530:735950. doi: 10.1016/j.aquaculture.2020.735950
44. Hashim R, Saat MAM. The utilization of seaweed meals as binding agents in pelleted feeds for snakehead (Channa striatus) fry and their effects on growth. Aquaculture. (1992) 108:299–308. doi: 10.1016/0044-8486(92)90114-Z
45. Lordan S, Ross RP, Stanton C. Marine bioactives as functional food ingredients: potential to reduce the incidence of chronic diseases. Mar Drugs. (2011) 9:1056–100. doi: 10.3390/md9061056
46. O’Sullivan L, et al. Prebiotics from marine macroalgae for human and animal health applications. Mar Drugs. (2010) 8:2038–64. doi: 10.3390/md8072038
47. Jiang Q, et al. Effects of dietary tryptophan on muscle growth, protein synthesis and antioxidant capacity in hybrid catfish Pelteobagrus vachelli♀× Leiocassis longirostris♂. Br J Nutr. (2022) 127:1761–73. doi: 10.1017/S0007114521002828
48. Zhao H, et al. Diet affects muscle quality and growth traits of grass carp (Ctenopharyngodon idellus): a comparison between grass and artificial feed. Front Physiol. (2018) 9:283. doi: 10.3389/fphys.2018.00283
49. Sajina KA, Sahu NP, Varghese T, Jain KK. Fucoidan-rich Sargassum wightii extract supplemented with α-amylase improve growth and immune responses of Labeo rohita (Hamilton, 1822) fingerlings. J Appl Phycol. (2019) 31:2469–80. doi: 10.1007/s10811-019-1742-0
50. Sattanathan G, et al. Effects of Dietary Blend of Algae Extract Supplementation on Growth, Biochemical, Haemato-Immunological Response, and Immune Gene Expression in Labeo rohita with Aeromonas hydrophila Post-Challenges. Fishes. (2022) 8:7. doi: 10.3390/fishes8010007
51. Abdel-Tawwab M, et al. Dietary curcumin nanoparticles promoted the performance, antioxidant activity, and humoral immunity, and modulated the hepatic and intestinal histology of Nile tilapia fingerlings. Fish Physiol Biochem. (2022) 48:585–601. doi: 10.1007/s10695-022-01066-4
52. Choe SS, Huh JY, Hwang IJ, Kim JI, Kim JB. Adipose tissue remodeling: its role in energy metabolism and metabolic disorders. Front Endocrinol (Lausanne). (2016) 7:30. doi: 10.3389/fendo.2016.00030
53. Weil C, Lefèvre F, Bugeon J. Characteristics and metabolism of different adipose tissues in fish. Rev Fish Biol Fish. (2013) 23:157–73. doi: 10.1007/s11160-012-9288-0
54. Schoeler M, Caesar R. Dietary lipids, gut microbiota and lipid metabolism. Rev Endocr Metab Disord. (2019) 20:461–72. doi: 10.1007/s11154-019-09512-0
55. Semova I, et al. Microbiota regulate intestinal absorption and metabolism of fatty acids in the zebrafish. Cell Host Microbe. (2012) 12:277–88. doi: 10.1016/j.chom.2012.08.003
56. Saurabh S, Sahoo PK. Lysozyme: an important defence molecule of fish innate immune system. Aquac Res. (2008) 39:223–39. doi: 10.1111/j.1365-2109.2007.01883.x
57. Xie SP. Ocean warming pattern effect on global and regional climate change. AGU Adv. (2020) 1:e2019AV000130. doi: 10.1029/2019AV000130
58. Peixoto MJ, et al. Effects of dietary supplementation of Gracilaria sp. extracts on fillet quality, oxidative stress, and immune responses in European seabass (Dicentrarchus labrax). J Appl Phycol. (2019) 31:761–70. doi: 10.1007/s10811-018-1519-x
59. Pamplona R, Costantini D. Molecular and structural antioxidant defenses against oxidative stress in animals. Am J Physiol Regul Integr Comp Physiol. (2011) 301:R843–63. doi: 10.1152/ajpregu.00034.2011
60. Burgos-Aceves MA, et al. Modulation of mitochondrial functions by xenobiotic-induced microRNA: from environmental sentinel organisms to mammals. Sci Total Environ. (2018) 645:79–88. doi: 10.1016/j.scitotenv.2018.07.109
61. Goes ESdR, Goes MD, d. Castro PL, d. Lara JAF, Vital ACP. and R. P. Ribeiro, “Imbalance of the redox system and quality of tilapia fillets subjected to pre-slaughter stress. PloS One. (2019) 14:e0210742. doi: 10.1371/journal.pone.0210742
62. Silvestre MA, Yániz JL, Peña FJ, Santolaria P, Castelló-Ruiz M. Role of antioxidants in cooled liquid storage of mammal spermatozoa. Antioxidants. (2021) 10:1096. doi: 10.3390/antiox10071096
63. Abdelhamid AF, Ayoub HF, Abd El-Gawad EA, Abdelghany MF, Abdel-Tawwab M. Potential effects of dietary seaweeds mixture on the growth performance, antioxidant status, immunity response, and resistance of striped catfish (Pangasianodon hypophthalmus) against Aeromonas hydrophila infection. Fish Shellfish Immunol. (2021) 119:76–83. doi: 10.1016/j.fsi.2021.09.043
64. Shi Q, et al. Effects of dietary Sargassum horneri on growth performance, serum biochemical parameters, hepatic antioxidant status, and immune responses of juvenile black sea bream Acanthopagrus schlegelii. J Appl Phycol. (2019) 31:2103–13. doi: 10.1007/s10811-018-1719-4
65. Chew YL, Lim YY, Omar M, Khoo KS. Antioxidant activity of three edible seaweeds from two areas in South East Asia. LWT Food Sci Technol. (2008) 41:1067–72. doi: 10.1016/j.lwt.2007.06.013
66. Ganesan P, Kumar CS, Bhaskar N. Antioxidant properties of methanol extract and its solvent fractions obtained from selected Indian red seaweeds. Bioresour Technol. (2008) 99:2717–23. doi: 10.1016/j.biortech.2007.07.005
67. Wei Z, Xie L, Wang Y, Zhuang J, Niu J, Liu L. Red blood cell lysis pretreatment can significantly improve the yield of Treponema pallidum DNA from blood. Microbiol Spectr. (2024) 11:e05198-22. doi: 10.1128/spectrum.05198-22
68. Zeraatpisheh F, Firouzbakhsh F, Khalili KJ. Effects of the macroalga Sargassum angustifolium hot water extract on hematological parameters and immune responses in rainbow trout (Oncohrynchus mykiss) infected with Yersinia rukeri. J Appl Phycol. (2018) 30:2029–37. doi: 10.1007/s10811-018-1395-4
69. Marques A, et al. Macroalgae-enriched diet protects gilthead seabream (Sparus aurata) against erythrocyte population instability and chromosomal damage induced by aqua-medicines. J Appl Phycol. (2019) 32:1477–93. doi: 10.1007/s10811-019-01996-2
70. Antonopoulou E, Chatzigiannidou I, Feidantsis K, Kounna C, Chatzifotis S. Effect of water temperature on cellular stress responses in meagre (Argyrosomus regius). Fish Physiol Biochem. (2020) 46:1075–91. doi: 10.1007/s10695-020-00773-0
71. Gomes JM, et al. What the erythrocytic nuclear alteration frequencies could tell us about genotoxicity and macrophage iron storage? PloS One. (2015) 10:e0143029. doi: 10.1371/journal.pone.0143029
72. Shuey DL, Gudi R, Krsmanovic L, Gerson RJ. Evidence that oxymorphone-induced increases in micronuclei occur secondary to hyperthermia. Toxicol Sci. (2007) 95:369–75. doi: 10.1093/toxsci/kfl148
73. Zafalon-Silva B, et al. Erythrocyte nuclear abnormalities and leukocyte profile in the Antarctic fish Notothenia coriiceps after exposure to short-and long-term heat stress. Polar Biol. (2017) 40:1755–60. doi: 10.1007/s00300-017-2099-y
74. Davis A, Maney D, Maerz J. The use of leukocyte profiles to measure stress in vertebrates: a review for ecologists. Funct Ecol. (2008) 22:760–72. doi: 10.1111/j.1365-2435.2008.01467.x
75. Hussain R, Khan I, Jamal A, Mohamed BB, Khan A. Evaluation of hematological, oxidative stress, and antioxidant profile in cattle infected with brucellosis in Southern Punjab, Pakistan. BioMed Res Int. (2022) 2022:7140909. doi: 10.1155/2022/7140909
76. Jin W, Jiang L, Hu S, Zhu A. Effects of Lactobacillus plantarum and Bacillus subtilis on growth, immunity and intestinal flora of largemouth bass(Micropterus salmoides). Aquaculture. (2024) 583:740581. doi: 10.1016/j.aquaculture.2024.740581
77. Cui H, et al. Effects of a highly purified fucoidan fromUndaria pinnatifidaon growth performance and intestine health status of gibel carpCarassius auratus gibelio. Aquac Nutr. (2019) 26:47–59. doi: 10.1111/anu.12966
78. Healy LE, et al. Fermentation of brown seaweeds Alaria esculenta and Saccharina latissima for new product development using Lactiplantbacillus plantarum, Saccharomyces cerevisiae and kombucha SCOBY. Algal Res. (2023) 76:103322. doi: 10.1016/j.algal.2023.103322
79. Kim YS, et al. Structural characteristics of sulfated polysaccharides from Sargassum horneri and immune-enhancing activity of polysaccharides combined with lactic acid bacteria. Food Funct. (2022) 13:8214–27. doi: 10.1039/d1fo03946f
80. Nazarudin MF, et al. Chemical, nutrient and physicochemical properties of brown seaweed, sargassum polycystum C. Agardh (Phaeophyceae) collected from Port Dickson, Peninsular Malaysia. Molecules. (2021) 26:5216. doi: 10.3390/molecules26175216
81. Anjana, Tiwari SK. Bacteriocin-producing probiotic lactic acid bacteria in controlling dysbiosis of the gut microbiota. Front Cell Infect Microbiol. (2022) 12:851140. doi: 10.3389/fcimb.2022.851140
82. Sharawy ZZ, et al. Effects of dietary marine microalgae,Tetraselmis suecica, on production, gene expression, protein markers and bacterial count of Pacificwhite shrimp Litopenaeus vannamei. Aquac Res. (2020) 51:2216–28. doi: 10.1111/are.14566
83. Pelusio NF, et al. Interaction between dietary lipid level and seasonal temperature changes in gilthead sea bream sparus aurata: effects on growth, fat deposition, plasma biochemistry, digestive enzyme activity, and gut bacterial community. Front Mar Sci. (2021) 8:664701. doi: 10.3389/fmars.2021.664701
Keywords: Gracilaria tenuistipitata, phenolic and flavonoid compounds, adipocyte tissue, temperature stress, RBC abnormality, tight junction protein, Oreochromis niloticus
Citation: Siddik MAB, Francis P, Foysal MJ and Francis DS (2024) Dietary seaweed extract mitigates oxidative stress in Nile tilapia by modulating inflammatory response and gut microbiota. Front. Immunol. 15:1471261. doi: 10.3389/fimmu.2024.1471261
Received: 27 July 2024; Accepted: 22 October 2024;
Published: 21 November 2024.
Edited by:
Samad Rahimnejad, University of Murcia, SpainReviewed by:
Byron Morales-Lange, Norwegian University of Life Sciences, NorwaySanjay K Gupta, Indian Institute of Agricultural Biotechnology (ICAR), India
Yafei Duan, South China Sea Fisheries Research Institute, China
Copyright © 2024 Siddik, Francis, Foysal and Francis. This is an open-access article distributed under the terms of the Creative Commons Attribution License (CC BY). The use, distribution or reproduction in other forums is permitted, provided the original author(s) and the copyright owner(s) are credited and that the original publication in this journal is cited, in accordance with accepted academic practice. No use, distribution or reproduction is permitted which does not comply with these terms.
*Correspondence: Muhammad A. B. Siddik, bS5zaWRkaWtAZGVha2luLmVkdS5hdQ==