- 1Guangdong VTR Bio-tech Co., Ltd, R&D Center, Zhuhai, China
- 2South China University of Technology, School of Biology and Biological Engineering, Guangzhou, China
- 3National Engineering Laboratory of Biological Feed Safety and Pollution Prevention and Control, Key Laboratory of Animal Nutrition and Feed, Ministry of Agriculture, Key Laboratory of Animal Nutrition and Feed Science of Zhejiang Province, Institute of Feed Science, Zhejiang University, Hangzhou, Zhejiang, China
Various problems and obstacles are encountered during pig farming, especially the weaning phase when switching from liquid to solid feed. Infection by pathogenic bacteria causes damage to the intestinal barrier function of piglets, disrupts the balance of the intestinal microbiota, and destroys the chemical, mechanical, and immune barriers of the intestinal tract, which is one of the main causes of gut inflammation or gut diseases in piglets. The traditional method is to add antibiotics to piglet diets to prevent bacterial infections. However, long-term overuse of antibiotics leads to bacterial resistance and residues in animal products, threatening human health and causing gut microbiota dysbiosis. In this context, finding alternatives to antibiotics to maintain pre- and post-weaning gut health in piglets and prevent pathogenic bacterial infections becomes a real emergency. The utilization of probiotics in piglet nutrition has emerged as a pivotal strategy to promote gut health and defend against pathogenic infections, offering a sustainable alternative to traditional antibiotic usage. This review introduces recent findings that underscore the multifaceted roles of probiotics in enhancing piglet welfare, from fortifying the gut barrier to mitigating the impacts of common bacterial pathogens. Meanwhile, this study introduces the functions of probiotics from different perspectives: positive effects of probiotics on piglet gut health, protecting piglets against pathogen infection, and the mechanisms of probiotics in preventing pathogenic bacteria.
Introduction
The global livestock industry is undergoing a profound transformation, with the promotion of antibiotic-free husbandry emerging as a key trend in the development of the industry (1). The use of probiotics has been proven to significantly improve the gut health of piglets, both before and after weaning (2, 3). Weaning marks a significant turning point in the early life of piglets, signifying the transition from liquid to solid food and accompanying vulnerability to pathogenic microorganisms, which leads to the temporary disruption of gut barrier, digestive and absorptive disorder, and inflammation risks (4).
The gut microbiota plays a pivotal role in digestion, nutrient absorption, and immune function. Maintaining a balanced gut microbiota, intact gut barrier function, and a well-regulated immune system are hallmarks of a healthy gut in piglets. However, the immature microbiota of piglets cannot support them to defend the pathogens (5). Probiotics have emerged as a promising strategy to maintain gut health and combat bacterial infections, and the definition of probiotics had been revised in 2014 by the International Scientific Association for Probiotics and Prebiotics (ISAPP)—”live microorganisms that, when administered in adequate amounts, confer a health benefit on the host”. The roles of probiotics include enhancing nutrient absorption (6), reducing the incidence of diarrhea, promoting the development of a healthy gut microbiota (7), stimulating the production of beneficial metabolites (8), and supporting gut barrier and immune function integrity (9). In recent years, with the deepening understanding of the gut microbiome, probiotics have shown great potential in preventing and treating diseases in piglets caused by Escherichia coli, Salmonella, Clostridium perfringens, etc., which poses a serious threat to piglet health.
This review aims to provide a comprehensive overview of the probiotic application in the piglet stage, analyzing the mechanisms of action, functional characteristics, and positive effects of probiotics on the gut microbiota of piglets, and providing a scientific basis for the rational application of probiotics in pig farming, laying a theoretical foundation for promoting piglet health, improving farming efficiency, and ensuring food safety.
The gut health of piglets
Gut health is a multifaceted concept that encompasses the maintenance of a balanced gut microbiota, an intact gut barrier, and a well-regulated immune system (10). A balanced gut microbiota is characterized by a diverse community of microorganisms that coexist in a stable state, contributing to the digestion of feed, the development of the immune system, and the overall health of the piglet (11).
Microbiota balance
The pig’s gut hosts a diverse array of microorganisms, which include bacteria, fungi, and viruses. There are scholars who want to measure the gut microbiota of pigs on a temporal and spatial scale. For instance, analyzing the relative abundances of gut microbes in pigs, Hu et al. identified three core-predominant species: Phascolarctobacterium succinatutens, Prevotella copri, and Oscillibacter valericigenes (12). Niu et al. identified a total of 22 phyla and 249 genera from fecal samples of pig, Firmicutes and Bacteroidetes were consistently the most dominant phyla, and as age increased, there was a notable rise in the proportion of TM7 and Tenericutes, while Lentisphaerae and Synergistetes showed a decline (13). Another research reported that consistent with prior studies, Bacteroidetes and Firmicutes were the predominant bacterial phyla, with Prevotella and Roseburia being the most abundant genera (14). Among these studies, Firmicutes, Bacteroidetes, Actinobacteria, and Proteobacteria are the core phyla for pig microbiota.
In general, the compositional structure of intestinal microbiota corresponds to different functions, including maintaining nutritional, physiological, and immunological functions. Firstly, microbiota in pig gut help in the breakdown and absorption of nutrients, particularly complex carbohydrates that the host cannot digest alone. Microbiota across different segments of the intestine exhibit distinct metabolic capabilities for various nutrients. For instance, the microbiota in the proximal intestine primarily engages in amino acid metabolism, while that in the distal intestine is predominantly involved in fermenting dietary fiber (DF) (15). DF plays a crucial role in sustaining normal intestinal function. Its biological effects are primarily realized through microbial fermentation, predominantly in the distal small intestine and the large intestine, and short-chain fatty acids (SCFAs), the principal products of this fermentation process, serve as the primary energy source for intestinal cells (16). Secondly, the microbiota influences physiology by regulating metabolism, and this process is interactive (17). For example, microbial enzymes can modify bile acids, affecting lipid digestion and absorption, and certain bacteria produce amino acids and their derivatives, which can influence gut health and systemic processes or even the brain (18). Include compounds like bacteriocins, which have antimicrobial properties, and other bioactive molecules that may affect the host’s physiology (19). In conclusion, the gut microbiota of the pig is intimately linked to its functions, and its functioning is also related to its metabolites.
Gut barrier function
The gut barrier function is a critical component of piglet gut health, which includes physical, chemical, biological, and immune barriers (20). The physical barrier is primarily composed of the intestinal epithelium and the tight junctions (TJs) between epithelial cells, which prevent the translocation of harmful substances and pathogens from the lumen into the bloodstream (21). Cells include the absorptive enterocytes (intestinal mucosal epithelial cell) and secretory enterocytes (enteroendocrine cell, goblet cell, and paneth cell), and intercellular junction complexes between intestinal epithelial cells, including TJs, adherens junctions, gap junctions, and bridges, are also important in maintaining the integrity of the epithelial barrier. The chemical barrier consists of secretions such as mucus, antimicrobial peptides (AMPs), and immunoglobulins that protect against pathogens. The mucus has many mucins including secretory mucin and binding mucin, covering the surface of the intestinal tract and defending against pathogens and other harmful substances, and AMPs in the chemical barrier are a class of peptides with antimicrobial activity, including defensins and lysozyme (10). Moreover, immunoglobulins such as secretory immunoglobulin A (sIgA) are essential for maintaining intestinal health; they not only defend against foreign pathogens, but also reduce pathogen attack on the intestinal epithelium by enhancing the intestinal barrier function (22). The biological barrier refers to the symbiotic relationship between the host and the commensal microbes that inhibit the growth of harmful bacteria (23). Studies have shown that the composition of intestinal microorganisms and the health of the body are closely related, such as some fiber-utilizing bacteria, which can break down indigestible fibers and insoluble proteins into monosaccharides and small peptides that can be absorbed by the animal to provide energy and nutrients for the organism, and a part of the bacteria that can produce SCFAs that can directly supply the body with energy and, at the same time, lower the pH in the intestine and inhibit the growth of pathogenic bacteria (24). With the intestinal epithelium, the mucus layer and innate immune cells constitute the immune barrier (25). A well-regulated immune system is capable of mounting an appropriate response to eliminate pathogens without causing excessive inflammation that could damage the gut tissue (26).
Immune system
Specifically, the immune system plays a crucial role in gut health by recognizing and responding to both harmless commensal microbes and potential pathogens (27). Therefore, the function of the immune system is closely related to the gut microbes. Microbiota has the ability to regulate the immune function of host; it can stimulate the development and maturation of the immune system, including the gut-associated lymphoid tissue (GALT) (28). GALT is an important component of the mucosal immune system and plays a key role in regulating the immune response in the gut. It consists of components such as Peyer’s patches (PPs), mesenteric lymphoid nodes (MLNs), isolated lymphoid follicles, and lamina propria (29). As with long-term microbial colonization, sustained microbial exposure during the development of piglets is crucial for maintaining a balanced immune cell population (30). The functions of microorganisms in regulating the body’s immunity are also interconnected, for example, sIgA concentrations show a positive correlation with the abundance of Prevotella and are associated with enhanced animal growth (31). Meanwhile, SCFAs have immunomodulatory effects, which help prevent inflammation and microbial infections.
In piglets, the development of gut health is particularly important due to their underdeveloped immune systems and the significant changes in diet and environment they experience, especially during the weaning period. At this stage, the piglets are more susceptible to pathogens. Hence, the maintenance of gut health is a critical aspect of piglet rearing and overall health .
Definition, classification, and function of probiotics
The definition of probiotics was introduced in 2014 and clearly defines the range of probiotics and non-probiotics (2), including three key attributes: live microorganisms, adequate quantities, and health benefits to the host. Recent developments have imposed stricter criteria on probiotic use, emphasizing safety and the absence of transferable antibiotic resistance genes or pathogenic virulence factors. Probiotics must also demonstrate resilience under normal gastrointestinal conditions, such as pH tolerance, exposure to proteolytic enzymes like trypsin, and bile salt tolerance.
Three types of probiotics are used as feed additives: Lactic acid bacteria (LAB) are characterized by their ability to ferment carbohydrates into lactic acid. According to Bergey’s Manual of Systematic Bacteriology, LAB is categorized into several genera including Lactobacillus, Streptococcus, Bifidobacterium, Leuconostoc, and Pediococcus (32). These bacteria are typically acid-tolerant and bile-resistant and adhere strongly to surfaces (33). LAB prevent pathogen infection by lowering pH and competing for binding sites on the intestinal epithelium, while stimulating the immune system and preserving the gut barrier (34, 35). Bacillus species are Gram-positive, spore-forming rods capable of surviving under aerobic or anaerobic conditions. Their spores provide resistance to harsh environmental conditions. Known for heat tolerance, rapid germination, and enzyme secretion, Bacillus spores are used in processing, storage, and transportation, germinating into vegetative cells (36). Commonly used species in animal production include Bacillus subtilis, Bacillus amyloliquefaciens, Bacillus natto, Bacillus licheniformis, and Bacillus coagulans (37). They maintain an anaerobic gut environment, inhibit pathogen proliferation, and produce organic acids and secrete enzymes. Additionally, they secrete secondary metabolites like bacteriocins and stimulate the host’s immune response (38).
Yeast, primarily Saccharomyces species, are heterotrophic, facultative anaerobic fungi that ferment sugars into ethanol and carbon dioxide, used widely in brewing and food fermentation. As probiotics, yeasts offer nutritional benefits, rich in proteins and polysaccharides (39), and have antagonistic effects against pathogens, improving gut ecology (40). Yeast cell wall extracts, containing mannans and glucans, boost gut immunity (41). However, the efficacy of live yeast and yeast culture varies significantly in animal production, influenced by factors such as species and rearing conditions. The left part of Figure 1 overviewed the probiotics knowledge, introducing the main characteristics of three types of probiotics.
Effects of probiotics on piglet gut health
The strategic use of probiotics can effectively address common challenges faced during the piglet stage, such as stress, diarrhea, disrupted gut microbiota, and reduced growth rates. Table 1 summarized the impact of probiotics on the intestinal health of piglets. Liu et al. demonstrate the effectiveness of a combination of Lactobacillus casei and Enterococcus faecalis (in a 3:1 ratio, 1×109 CFU/mL) administered orally to nursing piglets on day 1 (1 mL), day 7 (2 mL), day 14 (3 mL), and day 21 (4 mL). This probiotic mix was found to decrease the numbers of E. coli and C. perfringens in the intestines compared to the control group (42). Similarly, using B. amyloliquefaciens 1 mL (1×108 CFU/mL) continuously for 15 days resulted in a significant reduction of Escherichia, Shigella, and Streptococcus, while increasing the abundance of SCFA-producing bacteria and enhancing the antioxidant capacity of the piglets (43). Varun et al. administered Pediococcus FT28 1 mL (1×109 CFU/mL) to piglets from day 7 to day 21, observing an improvement in intestinal integrity and a significant reduction in mortality rates (44). Richard et al. indicated that early supplementation of probiotics (Bifidobacterium animalis, Lactobacillus acidophilus, and L. casei, 5×109 CFU/3 mL), can alleviate susceptibility to necrotizing enterocolitis (NEC) in piglets, as well as reduce C. perfringens (45). E. faecalis (2.5×106 CFU/g diet) was used to treat the weaned piglet for 28 days, which decreased diarrhea rate and improved the growth performance (46).
Probiotics also offer a promising solution to mitigate the challenges following weaning, such as intestinal dysfunction and diarrhea. Hu et al. administered Rhodotorula mucilaginosa at a concentration of 1×1010 CFU/mL/day for 4 weeks to weaned piglets, and results were shown to significantly improve the piglets’ performance, promote gut development, protect against harmful bacteria, and enhance antioxidant capacity (47). Yu et al. fed piglets a diet supplemented with B. licheniformis (5×105–1 × 106 CFU/g of feed) for 28 days. The results indicated that B. licheniformis significantly reduced diarrhea in weaned piglets, improved their antioxidant and immune functions, and maintained the structure of the gut microbiota (48). Du et al. provided piglets with a diet containing 2×105 CFU/g of B. amyloliquefaciens SC06 for 28 days. Compared to the antibiotic group, the SC06 group exhibited a significant enhancement in the piglets’ gut barrier and immune functions (49). Sun et al. fed piglets with a diet containing 600 g/ton of B. coagulans for 26 days. The results showed that, compared to the antibiotic group, B. coagulans (4.8×106 CFU/g of feed) significantly reduced the diarrhea rate in piglets, improved their performance, and optimized the gut microbiota structure (50). Wang et al. added a mixture of probiotics (Lactobacillus reuteri, Lactobacillus fermentum, and L. casei) and probiotics-derived antimicrobial compounds (reuterin) to the piglet feed at concentrations ranging from 7.74 ± 0.4 to 8.4 ± 0.5 log (CFU/g). The addition of probiotics significantly reduced the abundance of E. coli and C. perfringens at weaning, whereas the reuterin had less pronounced effects (51). Jenna et al. added yeast-based probiotics yeast (0.05% in phase 1, 0.025% in phase 2, and 0% in phase 3) to the piglet diet and observed, after continuous feeding for 24 days, a decrease in the antibiotic resistance of E. coli in the feces and an increase in the growth performance (52). Zhu et al. fed weaning pigs with Saccharomyces cerevisiae (12.9×107 CFU/g of feed) for 21 days, and they found that the E. coli decreased, and SOD activity and sIgA secretions increased (53). One research utilized the three types of mixed bacteria including LAB, Bacillus, and yeast (>1×108 CFU/g for each strain), which increased the growth performance and fecal acetic and propionic acid of weaned piglets, and reduced the diarrhea (54).
Moreover, probiotics can also have an impact on GALT, which has important implications for the host immune system and gut health. In weaned piglets, DNA from B. animalis was detected in MLN and PPs, with concentrations increasing proportionally to the administered probiotic dose (55). Consumption of Lactobacillus plantarum Lp6 was found to modulate gene expression within the jejunal PPs, and this modulation affected genes associated with immune response, cell differentiation, etc (56). One research used fermented milk with Lactobacillus bulgaricus and Streptococcus thermophilus to test its function, with results indicating that probiotics modulated the gut microbiota but did not clearly exert beneficial effects on GALT lymphocyte cell numbers and mucosal IgA levels (57). In addition to these direct effects, there is some evidence that probiotics stimulate the expression of inflammatory factors through GALT (58). For instance, O’Mahony et al. reported that commensal bacteria, such as Lactobacillus and Bifidobacterium, induced the production of regulatory cytokines, including IL-10, by MLN and MLN-derived dendritic cells (DCs); in contrast, pathogenic bacteria like Salmonella induced the production of Th1-polarizing cytokines (IL-12 and TNF-α) (59). Bifidobacteria from VSL#3 have the most pronounced anti-inflammatory effects during the maturation process of DCs, and they up-regulate the production of IL-10 by DCs, reduce the expression of costimulatory molecules such as CD80 and CD40, and decrease the production of IFN-γ by T cells (60). Using Lactobacillus jensenii also modulated the GALT-associated part, increasing the expression of T cell-related mRNA (CD3, IL-2, and IFN-γ) (61). Furthermore, some probiotics and commensal bacteria can activate local antigen-presenting cells, thereby enhancing antigen presentation to B lymphocytes and boosting the production of sIgA both locally and systemically (62). The whole activation of GALT strengthens the host’s defenses against pathogens and improve the gut health.
In this study, we collectively highlight the potential benefits of probiotics in the gut health and growth performance with piglets, demonstrating their roles in reducing diarrhea, enhancing immune and antioxidant functions, and optimizing gut microbiota composition. The right part of Figure 1 demonstrates the main functions of probiotics: (1) regulation of the host microbiota, improving the beneficial bacteria; (2) improvement of intestinal barrier function and competitive exclusion of pathogens; (3) modulation of immunity, accelerating the maturation of gut microbiota; and (4) secretion of metabolites or neurotransmitters, producing antimicrobial substances.
Probiotics and prevention of pathogenic bacterial infections
Probiotics have been shown to regulate gut health, but are they also effective during pathogen infections, especially during the formative “window period” of gut microbiota establishment in piglets? This query now stands as a focal point for scholarly investigation.
Table 2 summarizes the impact of probiotics on preventing pathogens. Qin et al. utilized L. casei to prevent enterotoxigenic E. coli (ETEC) K88 infection in piglets. They revealed that probiotics effectively alleviated symptoms associated with ETEC infection, such as intestinal damage and increased diarrhea rates, and activated mucosal and humoral immune responses in the piglets (63). Prasert et al. fed piglets a diet supplemented with 3 mL of L. plantarum and Lactococcus lactis (1×109 CFU/mL). After ETEC challenge (5×109 CFU/mL) 14 days post-weaning, they observed that probiotics improved gut microbiota composition, reduced antibiotic resistance in the gut microbiota, and mitigated oxidative stress (64). Guevara Ordaz et al. continuously fed piglets with L. plantarum (2×1010 CFU/animal/day) from 4 to 6 weeks of age. Following single 6-mL oral dose ETEC K88 (2×109 CFU/mL) challenge from days 23 to 27, they noted an increased abundance of L. plantarum, along with a reduction in diarrhea (65). Yang et al. administered a mixture of 10 mL of B. subtilis and B. licheniformis (3.9×107–7.8×107 CFU/mL) to piglets for 36 days. Post-challenge with 10 mL ETEC F4 (1.0×109 CFU/mL) on day 21, they observed a significant decrease of E. coli (66). Zhang et al. investigated the preventive effects of Lactobacillus rhamnosus GG 10 mL dose (1×1010 CFU/mL) against ETEC K88 10 mL dose (1×1010 CFU/mL) infection in weaned piglets. They found that the incidence of diarrhea was significantly lower in the probiotic group, and enhanced secretion of sIgA (67). Bhandari et al. evaluated the efficacy of B. subtilis (1.2×106 CFU/g of feed) as a direct-fed microbial in preventing ETEC K88 infection in weaned piglets (challenged at day 24, with 6 mL, 6.4×109 CFU/mL). Their results indicated that probiotics were equally effective as antibiotics in preventing diarrhea in piglets (68). Ahmed et al. conducted a study using Lactobacillus and Bacillus as probiotics (3.2×106 CFU/g of feed) to prevent 5-mL Salmonella KCTC 2515 (5.9×108 CFU/mL) and E. coli KCTC 2571 (2.3×108 CFU/mL) infections in piglets, and results showed that probiotic prevention led to a significant reduction in E. coli and Streptococcus (69). Emili Barba et al. utilized a Bifidobacterium longum subsp. infantis CECT 7210 2-mL dose (1×109 CFU) to prevent Salmonella typhimurium 2-mL dose (5×108 CFU) infection in weaned piglets. They found that probiotics resulted in reduced shedding of Salmonella, lower diarrhea scores, and enhanced gut immunity (70). Casey et al. administered a probiotic mixture (4×109 CFU) to weaned piglets for 30 consecutive days, followed by a Salmonella typhimurium (1×108 CFU) challenge on the sixth day. The study revealed that animals treated with probiotics had significantly reduced incidence, severity, and duration of diarrhea (71). Upadhaya et al. found, in a 3-week probiotic intervention using Bacillus (1×10 9 CFU/g) in weaned piglets challenged with Salmonella typhimurium 1 mL (1×1011 CFU/mL), that probiotic increased Lactobacillus and exerted immunomodulatory effects (72). Trevisi et al. fed piglets S. cerevisiae (5×107 CFU/g of feed) at weeks 4 and 7. After the ETEC F4 1.5 mL (1×108 CFU/mL) challenge on day 24, they found a substantial reduction of ETEC (73). S. cerevisiae boulardii was used to prevent the lipopolysaccharide (LPS, 25 μg/kg BW) challenge in weaning pigs, and results showed that the growth performance increased and the LPS-induced mortality was reduced obviously (74). Weaning piglets were fed with S. cerevisiae to defend the ETEC K88 6 mL (5×1010 CFU/mL), and a study illustrated that it significantly reduces the diarrhea scores and increases the IgA levels (75).
In summary, the application of probiotics can alleviate disturbances caused by bacterial diseases such as those from E. coli and Salmonella. The studies mentioned here illustrate the ability of probiotics to mitigate the impacts of bacterial pathogens on piglets, emphasizing their roles in enhancing gut barrier integrity, regulating the gut microbiota, and modulating immune responses. How exactly do probiotics prevent pathogenic bacterial infections (which is also currently the dominant line of research)?
Molecular mechanisms of probiotics against pathogenic bacteria
On a macro level, probiotics can counteract the negative consequences caused by pathogenic bacteria. At the molecular level, studies demonstrate that these beneficial microbes wield their effects through various mechanisms. For instance, they can modulate the host’s immune system, and probiotics also directly influence other microorganisms in the gut. Additionally, they are able to secrete small molecules or metabolites that inhibit the proliferation and virulence of pathogens.
Probiotics exert regulatory influence on both the innate and adaptive immunity of the host, affecting diverse cell types including macrophages, DCs, and T and B lymphocytes. They stimulate immune responses, enhancing overall host immunity and protecting against intestinal diseases (27). Probiotics prompt DCs to secrete anti-inflammatory cytokines, such as IL-10, triggering anti-inflammatory responses (76). The reduction in pro-inflammatory cytokine release by immune cells is attributed to probiotics’ interference with inflammatory signaling pathways, notably NF-κB, MAPK, and MLCK (77). Activation of these pathways leads to excessive release of pro-inflammatory cytokines, compromising the integrity of the gut barrier. Pathogenic bacteria activate the NF-κB and MAPK pathways, promoting the release of cytokines like IL-1β, IL-6, and IL -8, which recruit neutrophils to infected sites, causing inflammatory tissue damage (78). Xie et al. demonstrated that L. reuteri CO21 increases the anti-inflammatory capacity in piglets by inhibiting NF-κB and MLCK pathways, mitigating inflammation induced by ETEC K88 (79). Zhou et al. found that L. plantarum attenuates the inflammation mediated by C. perfringens through the modulation of MAPK and NF-κB pathways (80). B. subtilis BS29784 was shown to prevent the nuclear translocation of NF-κB by blocking IκB degradation, thereby limiting the accumulation of pro-inflammatory cytokines like IL-1β (38). L. reuteri was found to alleviate ETEC K88-induced inflammation by inhibiting the activation of the MLCK pathway, thus reducing inflammation and enhancing epithelial function (81). Probiotics also play a role in stimulating the production of gut antibodies, impacting the gut barrier by promoting IgA from B cells (82). Gut IgA interferes with pathogen adherence to the epithelium (83), and studies have shown that Saccharomyces boulardii and L. rhamnosus enhance sIgA levels or immunoglobulin secretion (84, 85). Moreover, probiotics can modulate the host’s immune response by influencing macrophage phagocytosis of pathogens. For example, L. casei exhibits enhanced inhibition of pathogenic Pseudomonas aeruginosa and Listeria monocytogenes in the gut, correlated with increased macrophage activity (86).
The direct impact of probiotics on commensal and pathogenic bacteria is another mechanism by which they resist pathogen infection, as probiotics can directly influence the gut microbiota. Gordon et al. showed that introducing probiotics into the mouse gut alters the metabolic pathways of its indigenous microbiome (87). Certain bacteria may find their ability to compete for nutritive or non-nutritive substance, such as iron, altered by the presence of probiotic strains, preventing pathogenic microorganisms from proliferating. For instance, L. acidophilus can utilize iron in the gut, thereby limiting its availability for other pathogens (88). Gordon et al. also demonstrated that treating germ-free mice with Bifidobacteria and Lactobacillus resulted in shifts in gene expression patterns, showing enhanced carbohydrate utilization capabilities by the bacteria. Interestingly, the sets of genes that responded to each probiotic did not overlap, indicating distinct effects on the microbiome (87). Probiotics can also antagonize pathogens directly, co-aggregating with them to prevent adhesion and colonization. Studies have shown that LAB can enhance their own colonization ability by self-aggregation and co-aggregation, forming a barrier against pathogen colonization (89, 90). Research has found that Bifidobacterium lactis or L. rhamnosus GG can reduce the attachment of pathogens like Salmonella, C. perfringens, and E. coli to porcine intestinal mucus (9). This anti-adhesive effect is achieved by competing for the same receptors as the pathogens.
Probiotics combat pathogenic bacteria through the secretion of various small-molecule substances that exhibit antimicrobial properties. These include bacteriocins, SCFAs, organic acid, and hydrogen peroxide. Bacteriocins, ribosomally synthesized AMPs, bind to microbial cells and disrupt phospholipid membranes, causing cellular leakage and inhibiting DNA/RNA synthesis and cell wall protein production (91). The probiotic B. subtilis PB6 produces surfactin, which restrains C. perfringens colonization. B. amyloliquefaciens RX7 has been shown to produce antibiotics with broad-spectrum antibacterial and antifungal activities, effectively curbing the growth of Candida albicans (92). Bacteriocins produced by Bifidobacterium NCFB exhibit activity against a range of Gram-positive bacteria, owing to their ability to adhere to bacterial surfaces (93, 94). Piewngam et al. observed that Bacillus could successfully defend against Staphylococcus aureus infection, primarily by reducing pathogen numbers through bacteriocin production (95). Secondary metabolites from bacteria can also inhibit quorum sensing (QS) in pathogens, disrupting their communication and virulence generation. For instance, pathogens equipped with QS systems, such as S. aureus and C. perfringens, communicate via the Agr system. Bacillus species capable of producing lipopeptides like fengycins (e.g., B. subtilis and B. amyloliquefaciens) can interfere with Agr signaling in S. aureus, disrupting QS system and preventing colonization in the gut or nasal cavity (96). Similar findings were reported by Piewngam et al. in a murine study, where oral administration of B. subtilis spores inhibited E. faecalis translocation from the gut to the bloodstream and systemic infection, with the mechanism involving fengycins and surfactant protein lipopeptides (97). Organic acids, particularly acetic and lactic acid, produced by probiotics can inhibit the growth of many enteric pathogens. Undissociated lactic acid acts as a permeabilizer for Gram-negative bacterial membranes, leading to cell disruption upon entry into the cytoplasm. It can also lower the intracellular pH, potentiating the effects of other antimicrobial compounds (98, 99). Hydrogen peroxide (H2O2) is another antimicrobial mechanism utilized by commensal bacteria. H2O2 can attenuate pathogen virulence, reduce invasion of epithelial cells, and alter gene transcription and signal transduction (100). Probiotic strains with H2O2-producing traits have been shown to significantly inhibit Salmonella typhimurium and S. aureus in vitro.
The mechanism of probiotics in preventing pathogens is summarized in Figure 2. Firstly, probiotics are able to compete directly with pathogenic bacteria for ecological niches and reduce by pathogen colonization. Secondly, appropriate probiotics can be the immune activators to prevent pathogen-related inflammation via multiple signaling pathways. Thirdly, the surface molecules and metabolites of probiotics may modulate piglets’ gut barrier function or directly reduce the load of pathogenic bacteria, for example, by inhibiting the QS system, disrupting the bacterial cell membrane structure, or lowering intestinal pH.
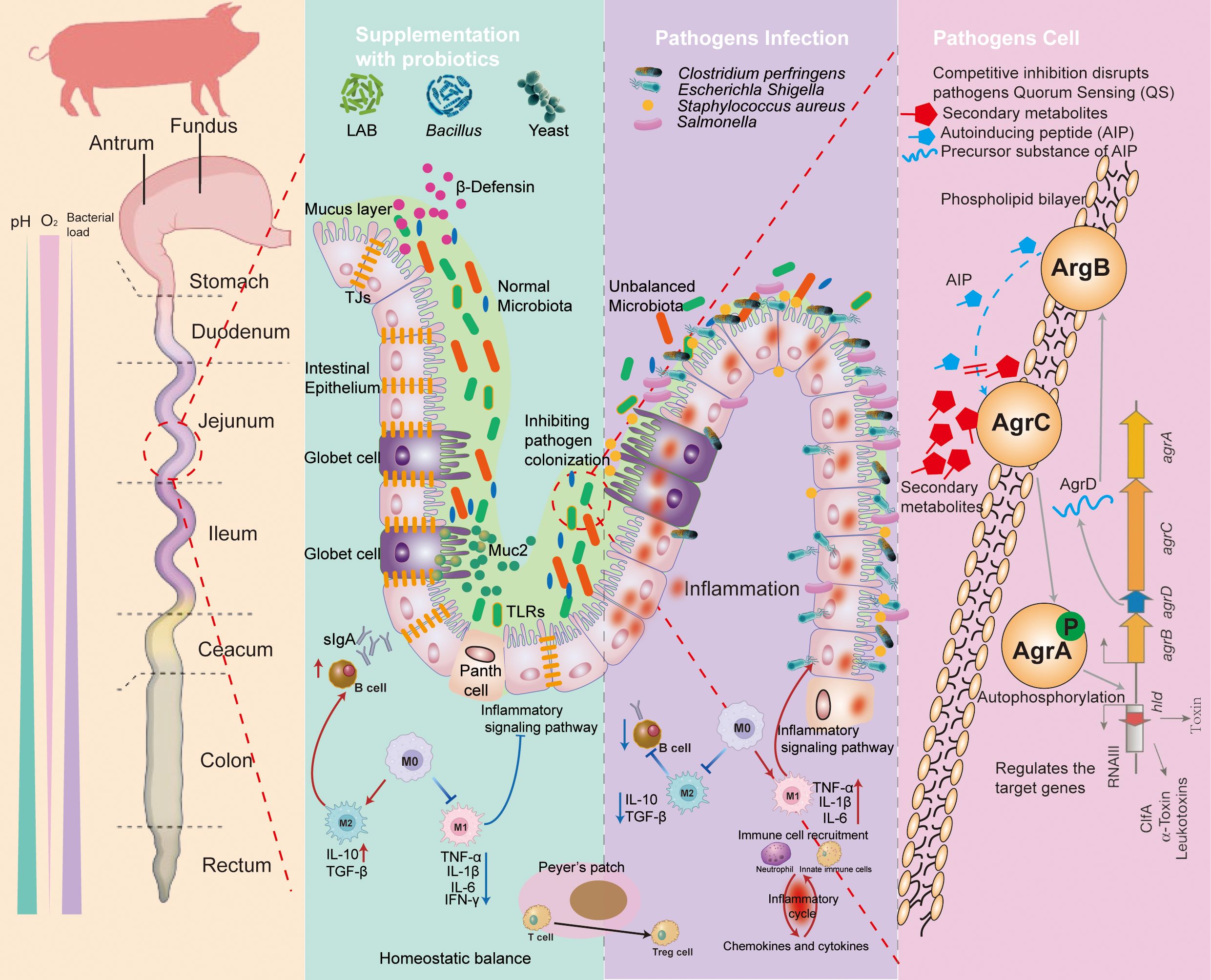
Figure 2. The mechanisms of probiotic prevention against the pathogen infection. Supplementation with probiotics: (1) manipulation of microbiota; (2) competitive inhibition of pathogen adhesion; (3) producing the metabolites and antimicrobial substance (such as bacteriocins, SCFAs, antimicrobial peptides, and organic acids); and (4) regulating the immune system. Pathogen infection: (1) causing microbiota disruption; (2) resulting in damage to the intestinal barrier; (3) generation of toxic substances into the host; and (4) triggering inflammatory responses. Pathogen cell: probiotics prevent the colonization of pathogens by producing secondary metabolites that block the transmission of quorum sensing (QS) systems of pathogens, leading to a reduction in the proliferation. TLRs, toll-like receptors; MUC2, mucin 2; TJs, tight junctions; DC, dendritic cell; TGF-b, transforming growth factor-b; TNF, tumor necrosis factor; IL, interleukin; sIgA, secretory immunoglobulin A; AMPs, antimicrobial peptides; AIP, autoinducing peptide.
Conclusion and future perspectives
This review has provided an extensive examination of the role of probiotics in maintaining and enhancing piglet gut health and discussed on their potential for defending against pathogenic bacterial infections and their mechanisms of action. We reviewed the studies that underscore the multifaceted benefits of different probiotics, including the modulation of gut microbiota, enhancement of gut barrier functions, stimulation of the immune system, and the production of antimicrobial compounds. Different from antibiotics, the molecular mechanisms of probiotics are complex and involve direct interactions with pathogens and modulation of host immune responses. Therefore, probiotics not only have considerable ability in the application of pathogen infection prevention in piglets, but also have unique advantages for antibiotic substitution.
From the available research, probiotics for prevention, treatment, or a combination may be the way forward. There are several areas of research and development that promise to advance the application of probiotics in piglet gut health. Strain Selection and Development: continued research is needed to identify and develop novel probiotic strains with enhanced efficacy against a broad spectrum of pathogens. In Vitro and In Vivo Experiments: screened probiotics that can stably colonize and efficiently display various functions (such as secreting antibacterial substances, improving the host immune system, and competing for ecological niches with pathogens) need to be verified. Personalized Probiotic Therapies: exploring the potential for personalized probiotic therapies tailored to the specific needs of individual piglets based on their unique gut microbiota profiles. Synergistic Approaches: investigating the synergistic effects of probiotics when combined with other interventions such as prebiotics, postbiotics, and phytobiotics to enhance gut health. Additionally, using the metabolites produced by probiotics directly may be a viable and efficient strategy to prevent or treat pathogen infection in piglets.
Author contributions
ZJ: Conceptualization, Data curation, Formal analysis, Funding acquisition, Investigation, Methodology, Project administration, Resources, Software, Supervision, Validation, Visualization, Writing – original draft, Writing – review & editing. MY: Resources, Writing – review & editing. WS: Methodology, Writing – review & editing. LM: Investigation, Writing – review & editing. YQL: Investigation, Methodology, Writing – review & editing. YG: Investigation, Writing – review & editing. YYL: Project administration, Writing – review & editing. WL: Methodology, Writing – review & editing. BY: Methodology, Project administration, Writing – review & editing. ZH: Resources, Writing – review & editing. YW: Funding acquisition, Methodology, Project administration, Supervision, Validation, Writing – review & editing.
Funding
The author(s) declare financial support was received for the research, authorship, and/or publication of this article. This research was supported by the 2024 Zhuhai Science and Technology Programme Key Funding Projects in the Social Development Field——Enzyme–probiotics combination on the pre-digestion treatment of feed ingredients and its effect on the intestinal health of piglets and the mechanism research (Project number: 2420004000196). This research was also supported by the earmarked fund for the Modern Agro-industry Technology Research System (CARS-35)
Conflict of interest
Authors ZJ, LM, YQL, YG, YYL, WL, and ZH were employed by the company Guangdong VTR Bio-tech Co., Ltd.
The remaining authors declare that the research was conducted in the absence of any commercial or financial relationships that could be construed as a potential conflict of interest.
Publisher’s note
All claims expressed in this article are solely those of the authors and do not necessarily represent those of their affiliated organizations, or those of the publisher, the editors and the reviewers. Any product that may be evaluated in this article, or claim that may be made by its manufacturer, is not guaranteed or endorsed by the publisher.
References
1. Wright GD, Cook MA. The past, present, and future of antibiotics. Sci Trans Med. (2022) 14. doi: 10.1126/scitranslmed.abo7793
2. Hill C, Guarner F, Reid G, Gibson GR, Merenstein DJ, Pot B, et al. Expert consensus document. The International Scientific Association for Probiotics and Prebiotics consensus statement on the scope and appropriate use of the term probiotic. Nat Rev Gastroenterol Hepatol. (2014) 11:506–14. doi: 10.1038/nrgastro.2014.66
3. Larsen N, Thorsen L, Kpikpi EN, Stuer-Lauridsen B, Cantor MD, Nielsen B, et al. Characterization of Bacillus spp. strains for use as probiotic additives in pig feed. Appl Microbiol Biotechnol. (2014) 98:1105–18. doi: 10.1007/s00253-013-5343-6
4. Su W, Gong T, Jiang Z, Lu Z, Wang Y. The role of probiotics in alleviating postweaning diarrhea in piglets from the perspective of intestinal barriers. Front Cell Infection Microbiol. (2022) 12:883107. doi: 10.3389/fcimb.2022.883107
6. Jha R, Das R, Oak S, Mishra P. Probiotics (Direct-fed microbials) in poultry nutrition and their effects on nutrient utilization, growth and laying performance, and gut health: A systematic review. Anim (Basel). (2020) 10:1863. doi: 10.3390/ani10101863
7. Butel MJ. Probiotics, gut microbiota and health. Med Mal Infect. (2014) 44:1–8. doi: 10.1016/j.medmal.2013.10.002
8. Sanders ME, Merenstein DJ, Reid G, Gibson GR, Rastall RA. Probiotics and prebiotics in intestinal health and disease: from biology to the clinic. Nat Rev Gastroenterol Hepatol. (2019) 16:605–16. doi: 10.1038/s41575-019-0173-3
9. Collado MC, Grzeskowiak L, Salminen S. Probiotic strains and their combination inhibit in vitro adhesion of pathogens to pig intestinal mucosa. Curr Microbiol. (2007) 55:260–5. doi: 10.1007/s00284-007-0144-8
10. Vancamelbeke M, Vermeire S. The intestinal barrier: a fundamental role in health and disease. Expert Rev Gastroenterol Hepatol. (2017) 11:821–34. doi: 10.1080/17474124.2017.1343143
11. Round JL, Mazmanian SK. The gut microbiota shapes intestinal immune responses during health and disease. Nat Rev Immunol. (2009) 9:313–23. doi: 10.1038/nri2515
12. Hu J, Chen J, Ma L, Hou Q, Zhang Y, Kong X, et al. Characterizing core microbiota and regulatory functions of the pig gut microbiome. ISME J. (2024) 18:wrad037. doi: 10.1093/ismejo/wrad037
13. Niu Q, Li P, Hao S, Zhang Y, Kim SW, Li H, et al. Dynamic distribution of the gut microbiota and the relationship with apparent crude fiber digestibility and growth stages in pigs. Sci Rep. (2015) 5. doi: 10.1038/srep09938
14. Ramayo-Caldas Y, Mach N, Lepage P, Levenez F, Denis C, Lemonnier G, et al. Phylogenetic network analysis applied to pig gut microbiota identifies an ecosystem structure linked with growth traits. ISME J. (2016) 10:2973–7. doi: 10.1038/ismej.2016.77
15. Puiman P, Stoll B, Mølbak L, de Bruijn A, Schierbeek H, Boye M, et al. Modulation of the gut microbiota with antibiotic treatment suppresses whole body urea production in neonatal pigs. Am J Physiology-Gastrointestinal Liver Physiol. (2013) 304:G300–10. doi: 10.1152/ajpgi.00229.2011
16. Hu R, Li S, Diao H, Huang C, Yan J, Wei X, et al. The interaction between dietary fiber and gut microbiota, and its effect on pig intestinal health. Front Immunol. (2023) 14:1095740. doi: 10.3389/fimmu.2023.1095740
17. Wang H, Xu R, Zhang H, Su Y, Zhu W. Swine gut microbiota and its interaction with host nutrient metabolism. Anim Nutr. (2020) 6:410–20. doi: 10.1016/j.aninu.2020.10.002
18. Patil Y, Gooneratne R, Ju X-H. Interactions between host and gut microbiota in domestic pigs: a review. Gut Microbes. (2019) 11:310–34. doi: 10.1080/19490976.2019.1690363
19. Lu S, Na K, Li Y, Zhang L, Fang Y, Guo X. Bacillus-derived probiotics: metabolites and mechanisms involved in bacteria-host interactions. Crit Rev Food Sci Nutr. (2022) 64:1–14. doi: 10.1080/10408398.2022.2118659
20. Ohland CL, Macnaughton WK. Probiotic bacteria and intestinal epithelial barrier function. Am J Physiol Gastrointest Liver Physiol. (2010) 298:G807–819. doi: 10.1152/ajpgi.00243.2009
21. Katherine R. Groschwitz SPH. Intestinal barrier function: Molecular regulation and disease pathogenesis. J Allergys Clin Immunol. (2009) 124(1):3–20. doi: 10.1016/j.jaci.2009.05.038
22. Guo J, Ren C, Han X, Huang W, You Y, Zhan J. Role of IgA in the early-life establishment of the gut microbiota and immunity: Implications for constructing a healthy start. Gut Microbes. (2021) 13:1–21. doi: 10.1080/19490976.2021.1908101
23. Aziz Q, Dore J, Emmanuel A, Guarner F, Quigley EM. Gut microbiota and gastrointestinal health: current concepts and future directions. Neurogastroenterol Motil. (2013) 25:4–15. doi: 10.1111/nmo.12046
24. van Zyl WF, Deane SM, Dicks LMT. Molecular insights into probiotic mechanisms of action employed against intestinal pathogenic bacteria. Gut Microbes. (2020) 12:1831339. doi: 10.1080/19490976.2020.1831339
25. Konig J, Wells J, Cani PD, Garcia-Rodenas CL, MacDonald T, Mercenier A, et al. Human intestinal barrier function in health and disease. Clin Transl Gastroenterol. (2016) 7:e196. doi: 10.1038/ctg.2016.54
26. Maynard CL, Elson CO, Hatton RD, Weaver CT. Reciprocal interactions of the intestinal microbiota and immune system. Nature. (2012) 489:231–41. doi: 10.1038/nature11551
27. D’Amelio P, Sassi F. Gut microbiota, immune system, and bone. Calcif Tissue Int. (2018) 102:415–25. doi: 10.1007/s00223-017-0331-y
28. Fouhse JM, Zijlstra RT, Willing BP. The role of gut microbiota in the health and disease of pigs. Anim Front. (2016) 6:30–6. doi: 10.2527/af.2016-0031
29. Mörbe UM, Jørgensen PB, Fenton TM, von Burg N, Riis LB, Spencer J, et al. Human gut-associated lymphoid tissues (GALT); diversity, structure, and function. Mucosal Immunol. (2021) 14:793–802. doi: 10.1038/s41385-021-00389-4
30. Inman CF, Haverson K, Konstantinov SR, Jones PH, Harris C, Smidt H, et al. Rearing environment affects development of the immune system in neonates. Clin Exp Immunol. (2010) 160:431–9. doi: 10.1111/j.1365-2249.2010.04090.x
31. Mach N, Berri M, Estellé J, Levenez F, Lemonnier G, Denis C, et al. Early-life establishment of the swine gut microbiome and impact on host phenotypes. Environ Microbiol Rep. (2015) 7:554–69. doi: 10.1111/1758-2229.12285
32. Mathur H, Beresford TP, Cotter PD. Health benefits of lactic acid bacteria (LAB) fermentates. Nutrients. (2020) 12:1679. doi: 10.3390/nu12061679
33. Raman J, Kim JS, Choi KR, Eun H, Yang D, Ko YJ, et al. Application of lactic acid bacteria (LAB) in sustainable agriculture: advantages and limitations. Int J Mol Sci. (2022) 23:7784. doi: 10.3390/ijms23147784
34. Yasui H, Shida K, Matsuzaki T, Yokokura T. Immunomodulatory function of lactic acid bacteria. Lactic Acid Bacteria: Genetics Metab Appl. (1999) 76:383–9. doi: 10.1007/978-94-017-2027-4_24
35. Kongo J. M.. Ed., ‘Lactic Acid Bacteria-R&D for Food, Health and Livestock Purposes’. InTech (2013). doi: 10.5772/2825
36. Leser TD, Knarreborg A, Worm J. Germination and outgrowth of Bacillus subtilis and Bacillus licheniformis spores in the gastrointestinal tract of pigs. J Appl Microbiol. (2008) 104:1025–33. doi: 10.1111/j.1365-2672.2007.03633.x
37. Chen KL, Kho WL, You SH, Yeh RH, Tang SW, Hsieh CW. Effects of Bacillus subtilis var. natto and Saccharomyces cerevisiae mixed fermented feed on the enhanced growth performance of broilers. Poult Sci. (2009) 88:309–15. doi: 10.3382/ps.2008-00224
38. Rhayat L, Maresca M, Nicoletti C, Perrier J, Brinch KS, Christian S, et al. Effect of bacillus subtilis strains on intestinal barrier function and inflammatory response. Front Immunol. (2019) 10:564. doi: 10.3389/fimmu.2019.00564
39. Polyorachbr S, Wanapat M. Enrichment of protein content in cassava (Manihot esculenta Crantz) bysupplementing with yeast for use as animal feed. Emirates J Food Agric. (2013) 25. doi: 10.9755/ejfa.v25i2.10649
40. Shurson GC. Yeast and yeast derivatives in feed additives and ingredients: Sources, characteristics, animal responses, and quantification methods. Anim Feed Sci Technol. (2018) 235:60–76. doi: 10.1016/j.anifeedsci.2017.11.010
41. Robinson PH, Erasmus LJ. Effects of analyzable diet components on responses of lactating dairy cows to Saccharomyces cerevisiae based yeast products: A systematic review of the literature. Anim Feed Sci Technol. (2009) 149:185–98. doi: 10.1016/j.anifeedsci.2008.10.003
42. Liu C, Zhu Q, Chang J, Yin Q, Song A, Li Z, et al. Effects of Lactobacillus casei and Enterococcus faecalis on growth performance immune function and gut microbiota of suckling piglets. Arch Anim Nutr. (2017) 71:120–33. doi: 10.1080/1745039X.2017.1283824
43. Jiang Z, Su W, Li W, Wen C, Du S, He H, et al. Bacillus amyloliquefaciens 40 regulates piglet performance, antioxidant capacity, immune status and gut microbiota. Anim Nutr. (2022) 12:116–27. doi: 10.1016/j.aninu.2022.09.006
44. Sarkar VK, De UK, Kala A, Verma AK, Chauhan A, Paul BR, et al. Early-life intervention of lactoferrin and probiotic in suckling piglets: effects on immunoglobulins, intestinal integrity, and neonatal mortality. Probiotics Antimicrob Proteins. (2022) 15:149–59. doi: 10.1007/s12602-022-09964-y
45. Siggers RH, Siggers J, Boye M, Thymann T, Mølbak L, Leser T, et al. Early administration of probiotics alters bacterial colonization and limits diet-induced gut dysfunction and severity of necrotizing enterocolitis in preterm pigs. J Nutr. (2008) 138:1437–44. doi: 10.1093/jn/138.8.1437
46. Suchodolski JS, Hu Y, Dun Y, Li S, Zhang D, Peng N, et al. Dietary enterococcus faecalis LAB31 improves growth performance, reduces diarrhea, and increases fecal lactobacillus number of weaned piglets. PloS One. (2015) 10:e0116635. doi: 10.1371/journal.pone.0116635
47. Hu P, Mao J, Zeng Y, Sun Z, Deng H, Chen C, et al. Isolation, identification, and function of rhodotorula mucilaginosa TZR(2014) and its effects on the growth and health of weaned piglets. Front Microbiol. (2022) 13:922136. doi: 10.3389/fmicb.2022.922136
48. Yu X, Cui Z, Qin S, Zhang R, Wu Y, Liu J, et al. Effects of bacillus licheniformis on growth performance, diarrhea incidence, antioxidant capacity, immune function, and fecal microflora in weaned piglets. Anim (Basel). (2022) 12:1609. doi: 10.3390/ani12131609
49. Du W, Xu H, Mei X, Cao X, Gong L, Wu Y, et al. Probiotic Bacillus enhance the intestinal epithelial cell barrier and immune function of piglets. Benef Microbes. (2018) 9:743–54. doi: 10.3920/BM2017.0142
50. Sun T, Miao H, Zhang C, Wang Y, Liu S, Jiao P, et al. Effect of dietary Bacillus coagulans on the performance and intestinal microbiota of weaned piglets. Animal. (2022) 16:100561. doi: 10.1016/j.animal.2022.100561
51. Wang W, Zijlstra RT, Ganzle MG. Feeding Limosilactobacillus fermentum K9-2 and Lacticaseibacillus casei K9-1, or Limosilactobacillus reuteri TMW1.656 Reduces Pathogen Load in Weanling Pigs. Front Microbiol. (2020) 11:608293. doi: 10.3389/fmicb.2020.608293
52. Chance JA, DeRouchey JM, Amachawadi RG, Ishengoma V, Nagaraja TG, Goodband RD, et al. Influence of yeast-based pre- and probiotics in lactation and nursery diets on nursery pig performance and antimicrobial resistance of fecal Escherichia coli. J Anim Sci. (2022) 100:skac166. doi: 10.1093/jas/skac166
53. Zhu C, Wang L, Wei S-Y, Chen Z, Ma X-Y, Zheng C-T, et al. Effect of yeast Saccharomyces cerevisiae supplementation on serum antioxidant capacity, mucosal sIgA secretions and gut microbial populations in weaned piglets. J Integr Agric. (2017) 16:2029–37. doi: 10.1016/s2095-3119(16)61581-2
54. Lu X, Zhang M, Zhao L, Ge K, Wang Z, Jun L, et al. Growth performance and post-weaning diarrhea in piglets fed a diet supplemented with probiotic complexes. J Microbiol Biotechnol. (2018) 28:1791–9. doi: 10.4014/jmb.1807.07026
55. Trevisi P, et al. Effect of fructo-oligosaccharides and different doses of Bifidobacterium animalis in a weaning diet on bacterial translocation and Toll-like receptor gene expression in pigs. Nutrition. (2008) 24:1023–9. doi: 10.1016/j.nut.2008.04.008
56. Chang G, Shi Y, Le G, Xu Z, Sun J, Li J. Effects of Lactobacillus plant arum on genes expression pattern in mice jejunal Peyer’s patches. Cell Immunol. (2009) 258:1–8. doi: 10.1016/j.cellimm.2009.02.005
57. Takayama H, Fukatsu K, Takahashi K, Noguchi M, Watkins A, Matsumoto N, et al. Influences of a fermented milk with Lactobacillus bulgaricus and Streptococcus thermophiles on gut associated lymphoid tissue, mucosal IgA, and gut flora in mice. Clin Nutr Open Sci. (2022) 42:36–48. doi: 10.1016/j.nutos.2022.01.007
58. Sanz Y, De Palma G. Gut microbiota and probiotics in modulation of epithelium and gut-associated lymphoid tissue function. Int Rev Immunol. (2009) 28:397–413. doi: 10.3109/08830180903215613
59. O’Mahony L, O’Callaghan L, McCarthy J, Shilling D, Scully P, Sibartie S, et al. Differential cytokine response from dendritic cells to commensal and pathogenic bacteria in different lymphoid compartments in humans. Am J Physiology-Gastrointestinal Liver Physiol. (2006) 290:G839–45. doi: 10.1152/ajpgi.00112.2005
60. Hart AL. Modulation of human dendritic cell phenotype and function by probiotic bacteria. Gut. (2004) 53:1602–9. doi: 10.1136/gut.2003.037325
61. Sato K, Takahashi K, Tohno M, Miura Y, Kamada T, Ikegami S, et al. Immunomodulation in gut-associated lymphoid tissue of neonatal chicks by immunobiotic diets. Poultry Sci. (2009) 88:2532–8. doi: 10.3382/ps.2009-00291
62. Shi CW, Zeng Y, Yang GL, Jiang YL, Yang WT, Chen YQ, et al. Effect of Lactobacillus rhamnosus on the development of B cells in gut-associated lymphoid tissue of BALB/c mice. J Cell Mol Med. (2020) 24:8883–6. doi: 10.1111/jcmm.15574
63. Qin D, Bai Y, Li Y, Huang Y, Li L, Wang G, et al. Changes in gut microbiota by the lactobacillus casei anchoring the K88 fimbrial protein prevented newborn piglets from clinical diarrhea. Front Cell Infect Microbiol. (2022) 12:842007. doi: 10.3389/fcimb.2022.842007
64. Apiwatsiri P, Pupa P, Sirichokchatchawan W, Sawaswong V, Nimsamer P, Payungporn S, et al. Metagenomic analysis of the gut microbiota in piglets either challenged or not with enterotoxigenic Escherichia coli reveals beneficial effects of probiotics on microbiome composition, resistome, digestive function and oxidative stress responses. PloS One. (2022) 17:e0269959. doi: 10.1371/journal.pone.0269959
65. Guerra-Ordaz AA, Gonzalez-Ortiz G, La Ragione RM, Woodward MJ, Collins JW, Perez JF, et al. Lactulose and Lactobacillus plantarum, a potential complementary synbiotic to control postweaning colibacillosis in piglets. Appl Environ Microbiol. (2014) 80:4879–86. doi: 10.1128/AEM.00770-14
66. Yang GY, Zhu YH, Zhang W, Zhou D, Zhai CC, Wang JF. Influence of orally fed a select mixture of Bacillus probiotics on intestinal T-cell migration in weaned MUC4 resistant pigs following Escherichia coli challenge. Vet Res. (2016) 47:71. doi: 10.1186/s13567-016-0355-8
67. Zhang L, Xu YQ, Liu HY, Lai T, Ma JL, Wang JF, et al. Evaluation of Lactobacillus rhamnosus GG using an Escherichia coli K88 model of piglet diarrhoea: Effects on diarrhoea incidence, faecal microflora and immune responses. Vet Microbiol. (2010) 141:142–8. doi: 10.1016/j.vetmic.2009.09.003
68. Bhandari SK, Xu B, Nyachoti CM, Giesting DW, Krause DO. Evaluation of alternatives to antibiotics using an Escherichia coli K88+ model of piglet diarrhea: effects on gut microbial ecology. J Anim Sci. (2008) 86:836–47. doi: 10.2527/jas.2006-822
69. Sonia TA, Ji H, Hong-Seok M, Chul-Ju Y. Evaluation of Lactobacillus and Bacillus-based probiotics as alternatives to antibiotics in enteric microbial challenged weaned piglets. Afr J Microbiol Res. (2014) 8:96–104. doi: 10.5897/ajmr2013.6355
70. Barba-Vidal E, Castillejos L, Roll VFB, Cifuentes-Orjuela G, Moreno Munoz JA, Martin-Orue SM. The Probiotic Combination of Bifidobacterium longum subsp. infantis CECT 7210 and Bifidobacterium animalis subsp. lactis BPL6 Reduces Pathogen Loads and Improves Gut Health of Weaned Piglets Orally Challenged with Salmonella typhimurium. Front Microbiol. (2017) 8:1570. doi: 10.3389/fmicb.2017.01570
71. Casey PG, Gardiner GE, Casey G, Bradshaw B, Lawlor PG, Lynch PB, et al. A five-strain probiotic combination reduces pathogen shedding and alleviates disease signs in pigs challenged with Salmonella enterica Serovar Typhimurium. Appl Environ Microbiol. (2007) 73:1858–63. doi: 10.1128/AEM.01840-06
72. Upadhaya SD, Shanmugam SK, Kang DK, Kim IH. Preliminary assessment on potentials of probiotic B. subtilis RX7 and B. methylotrophicus C14 strains as an immune modulator in Salmonella-challenged weaned pigs. Trop Anim Health Prod. (2017) 49:1065–70. doi: 10.1007/s11250-017-1278-8
73. Trevisi P, Latorre R, Priori D, Luise D, Archetti I, Mazzoni M, et al. Effect of feed supplementation with live yeast on the intestinal transcriptome profile of weaning pigs orally challenged with Escherichia coli F4. Animal. (2017) 11:33–44. doi: 10.1017/S1751731116001178
74. Collier CT, Carroll JA, Ballou MA, Starkey JD, Sparks JC. Oral administration of Saccharomyces cerevisiae boulardii reduces mortality associated with immune and cortisol responses to Escherichia coli endotoxin in pigs1. J Anim Sci. (2011) 89:52–8. doi: 10.2527/jas.2010-2944
75. Kiarie E, Bhandari S, Scott M, Krause DO, Nyachoti CM. Growth performance and gastrointestinal microbial ecology responses of piglets receiving Saccharomyces cerevisiae fermentation products after an oral challenge with Escherichia coli (K88)1. J Anim Sci. (2011) 89:1062–78. doi: 10.2527/jas.2010-3424
76. Yan F, Polk DB. Probiotics and immune health. Curr Opin Gastroenterol. (2011) 27:496–501. doi: 10.1097/MOG.0b013e32834baa4d
77. Mirpuri J, Sotnikov I, Myers L, Denning TL, Yarovinsky F, Parkos CA, et al. Lactobacillus rhamnosus (LGG) regulates IL-10 signaling in the developing murine colon through upregulation of the IL-10R2 receptor subunit. PloS One. (2012) 7:e51955. doi: 10.1371/journal.pone.0051955
78. Llewellyn A, Foey A. Probiotic modulation of innate cell pathogen sensing and signaling events. Nutrients. (2017) 9:1156. doi: 10.3390/nu9101156
79. Xie W, Song L, Wang X, Xu Y, Liu Z, Zhao D, et al. A bovine lactoferricin-lactoferrampin-encoding Lactobacillus reuteri CO21 regulates the intestinal mucosal immunity and enhances the protection of piglets against enterotoxigenic Escherichia coli K88 challenge. Gut Microbes. (2021) 13:1956281. doi: 10.1080/19490976.2021.1956281
80. Zhou Y, Wang B, Wang Q, Tang L, Zou P, Zeng Z, et al. Protective Effects of Lactobacillus plantarum Lac16 on Clostridium perfringens Infection-Associated Injury in IPEC-J2 Cells. Int J Mol Sci. (2021) 22:12388. doi: 10.3390/ijms222212388
81. Gao J, Cao S, Xiao H, Hu S, Yao K, Huang K, et al. Lactobacillus reuteri 1 Enhances Intestinal Epithelial Barrier Function and Alleviates the Inflammatory Response Induced by Enterotoxigenic Escherichia coli K88 via Suppressing the MLCK Signaling Pathway in IPEC-J2 Cells. Front Immunol. (2022) 13:897395. doi: 10.3389/fimmu.2022.897395
82. Azad MAK, Sarker M, Wan D. Immunomodulatory effects of probiotics on cytokine profiles. BioMed Res Int. (2018) 2018:8063647. doi: 10.1155/2018/8063647
83. Liana Viaşu B, Popescu F, Bica. Probiotics C. and their immunomodulatory potential. Biology. (2013) 7(4):539–47. doi: 10.1002/jcp.27559
84. Dogi C, Garcia G, De Moreno de LeBlanc A, Greco C, Cavaglieri L. Lactobacillus rhamnosus RC007 intended for feed additive: immune-stimulatory properties and ameliorating effects on TNBS-induced colitis. Benef Microbes. (2016) 7:539–47. doi: 10.3920/BM2015.0147
85. Rodrigues AC, Cara DC, Fretez SH, Cunha FQ, Vieira EC, Nicoli JR, et al. Saccharomyces boulardii stimulates sIgA production and the phagocytic system of gnotobiotic mice. J Appl Microbiol. (2000) 89:404–14. doi: 10.1046/j.1365-2672.2000.01128.x
86. Tien MT, Girardin SE, Regnault B, Le Bourhis L, Dillies MA, Coppee JY, et al. Anti-inflammatory effect of Lactobacillus casei on Shigella-infected human intestinal epithelial cells. J Immunol. (2006) 176:1228–37. doi: 10.4049/jimmunol.176.2.1228
87. Sonnenburg JL, Chen CT, Gordon JI. Genomic and metabolic studies of the impact of probiotics on a model gut symbiont and host. PloS Biol. (2006) 4:e413. doi: 10.1371/journal.pbio.0040413
88. Elli M, Zink R, Rytz A, Reniero R, Morelli L. Iron requirement of Lactobacillus spp. in completely chemically defined growth media. J Appl Microbiol. (2000) 88:695–703. doi: 10.1046/j.1365-2672.2000.01013.x
89. Janković T, Frece J, Abram M, Gobin I. Aggregation ability of potential probiotic Lactobacillus plantarum strains. Int J Sanitary Eng Res. (2012) 6:19–24.
90. Schachtsiek M, Hammes WP, Hertel C. Characterization of Lactobacillus coryniformis DSM 20001T surface protein Cpf mediating coaggregation with and aggregation among pathogens. Appl Environ Microbiol. (2004) 70:7078–85. doi: 10.1128/AEM.70.12.7078-7085.2004
91. Islam MR, Nagao J, Zendo T, Sonomoto K. Antimicrobial mechanism of lantibiotics. Biochem Soc Trans. (2012) 40:1528–33. doi: 10.1042/BST20120190
92. Lim KB, Balolong MP, Kim SH, Oh JK, Lee JY, Kang DK. Isolation and characterization of a broad spectrum bacteriocin from bacillus amyloliquefaciens RX7. BioMed Res Int. (2016) 2016:8521476. doi: 10.1155/2016/8521476
93. Johnson MG, Yildirim Z. Characterization and antimicrobial spectrum of bifidocin B, a bacteriocin produced by bifidobacterium bifidum NCFB 1454. J Food Prot. (1998) 61:47–51. doi: 10.4315/0362-028X-61.1.47
94. Yildirim Z, Winters DK, Johnson MG. Purification, amino acid sequence and mode of action of bifidocin B produced by Bifidobacterium bifidum NCFB. J Appl Microbiol. (1999) 86:45–54. doi: 10.1046/j.1365-2672.1999.00629.x
95. Pipat Piewngam MO. Probiotics to prevent Staphylococcus aureus disease? Gut Microbes. (2019) 11:94–101. doi: 10.1080/19490976.2019.1591137
96. Piewngam P, Zheng Y, Nguyen TH, Dickey SW, Joo HS, Villaruz AE, et al. Pathogen elimination by probiotic Bacillus via signalling interference. Nature. (2018) 562:532–7. doi: 10.1038/s41586-018-0616-y
97. Piewngam P, Chiou J, Ling J, Liu R, Pupa P, Zheng Y, et al. Enterococcal bacteremia in mice is prevented by oral administration of probiotic Bacillus spores. Sci Trans Med. (2021) 13:eabf4692. doi: 10.1126/scitranslmed.abf4692
98. Alakomi HL, Skyttä E, Saarela M, Mattila-Sandholm T, Latva-Kala K, Helander IM. Lactic acid permeabilizes gram-negative bacteria by disrupting the outer membrane. Appl Environ Microbiol. (2000) 66:2001–5. doi: 10.1128/AEM.66.5.2001-2005.2000
99. Russell JB, Diez-Gonzalez F. The effects of fermentation acids on bacterial growth. Adv Microb Physiol. (1998) 39:205–34. doi: 10.1016/s0065-2911(08)60017-x
100. Fayol-Messaoudi D, Berger CN, Coconnier-Polter MH, Lievin-Le Moal V, Servin AL. pH-, Lactic acid-, and non-lactic acid-dependent activities of probiotic Lactobacilli against Salmonella enterica Serovar Typhimurium. Appl Environ Microbiol. (2005) 71:6008–13. doi: 10.1128/AEM.71.10.6008-6013.2005
101. Nguyen DH, Nyachoti CM, Kim IH. Evaluation of effect of probiotics mixture supplementation on growth performance, nutrient digestibility, faecal bacterial enumeration, and noxious gas emission in weaning pigs. Ital J Anim Sci. (2018) 18:466–73. doi: 10.1080/1828051x.2018.1537726
102. Li H-H, Jiang X-R, Qiao J-Y. Effect of dietary Bacillus subtilis on growth performance and serum biochemical and immune indexes in weaned piglets. J Appl Anim Res. (2021) 49:83–8. doi: 10.1080/09712119.2021.1877717
103. Yang KM, Jiang ZY, Zheng CT, Wang L, Yang XF. Effect of Lactobacillus plantarum on diarrhea and intestinal barrier function of young piglets challenged with enterotoxigenic Escherichia coli K88. J Anim Sci. (2014) 92:1496–503. doi: 10.2527/jas2013-6619
104. Trckova M, Faldyna M, Alexa P, Sramkova Zajacova Z, Gopfert E, Kumprechtova D, et al. The effects of live yeast Saccharomyces cerevisiae on postweaning diarrhea, immune response, and growth performance in weaned piglets. J Anim Sci. (2014) 92:764–74. doi: 10.2527/jas2013-6793
Keywords: piglets, probiotics, pathogens, microbiota, intestinal barrier, mechanisms
Citation: Jiang Z, Yang M, Su W, Mei L, Li Y, Guo Y, Li Y, Liang W, Yang B, Huang Z and Wang Y (2024) Probiotics in piglet: from gut health to pathogen defense mechanisms. Front. Immunol. 15:1468873. doi: 10.3389/fimmu.2024.1468873
Received: 22 July 2024; Accepted: 14 October 2024;
Published: 04 November 2024.
Edited by:
Teleky Bernadette-Emoke, University of Agricultural Sciences and Veterinary Medicine of Cluj-Napoca, RomaniaReviewed by:
Elena Franco Robles, University of Guanajuato, MexicoEric Auclair, Phileo Lesaffre Animal Care, France
Copyright © 2024 Jiang, Yang, Su, Mei, Li, Guo, Li, Liang, Yang, Huang and Wang. This is an open-access article distributed under the terms of the Creative Commons Attribution License (CC BY). The use, distribution or reproduction in other forums is permitted, provided the original author(s) and the copyright owner(s) are credited and that the original publication in this journal is cited, in accordance with accepted academic practice. No use, distribution or reproduction is permitted which does not comply with these terms.
*Correspondence: Zipeng Jiang, MTIxMTcwMDRAemp1LmVkdS5jbg==; Zhiyi Huang, aHVhbmd6aGl5aUB2dHJiaW8uY29t; Yizhen Wang, eXp3YW5nMzIxQHpqdS5lZHUuY24=