- 1Hebei Medical University-National University of Ireland Galway Stem Cell Research Center, Hebei Medical University, Shijiazhuang, Hebei, China
- 2Department of Clinical Laboratory, The Second Hospital of Hebei Medical University, Shijiazhuang, Hebei, China
- 3Hebei Key Laboratory of Laboratory Medicine, The Second Hospital of Hebei Medical University, Shijiazhuang, Hebei, China
- 4Department of Medicine, University of California Los Angeles, Los Angeles, CA, United States
- 5Department of Medicina Molecolare e Biotecnologie Mediche, Federico II University, Naples, Italy
- 6Department of Rheumatology and Immunology, The Second Hospital of Hebei Medical University, Shijiazhuang, Hebei, China
- 7Hebei Research Center for Stem Cell Medical Translational Engineering, Shijiazhuang, Hebei, China
In recent years, the use of chimeric antigen receptor (CAR)-T cells has emerged as a promising immunotherapy in multiple diseases. CAR-T cells are T cells genetically modified to express a surface receptor, known as CAR, for the targeting of cognate antigens on specific cells. The effectiveness of CAR-T cell therapy in hematologic malignancies including leukemia, myeloma, and non-Hodgkin’s lymphoma has led to consider its use as a potential avenue of treatment for autoimmune diseases. However, broadening the use of CAR-T cell therapy to a large spectrum of autoimmune conditions is challenging particularly because of the possible development of side effects including cytokine release syndrome and neurotoxicity. The design of CAR therapy that include additional immune cells such as double-negative T cells, γδ T cells, T regulatory cells and natural killer cells has shown promising results in preclinical studies and clinical trials in oncology, suggesting a similar potential utility in the treatment of autoimmune diseases. This review examines the mechanisms, efficacy, and safety of CAR approaches with a focus on their use in autoimmune diseases including systemic lupus erythematosus, Sjögren’s syndrome, systemic sclerosis, multiple sclerosis, myasthenia gravis, lupus nephritis and other autoimmune diseases. Advantages and disadvantages as compared to CAR-T cell therapy will also be discussed.
Introduction
Chimeric Antigen Receptor (CAR)-T cell technology is a cutting-edge immunotherapy approach designed to harness a patient’s own immune system by customizing autologous T-cells to express a CAR, which allows T cell to recognize and eliminate target cells bearing the cognate antigen. Costimulatory molecules and CAR immunoreceptors can further enhance targeted elimination of cells (Figure 1). Compared to antibody therapies, CAR-T cells act faster and in a more sustained fashion.
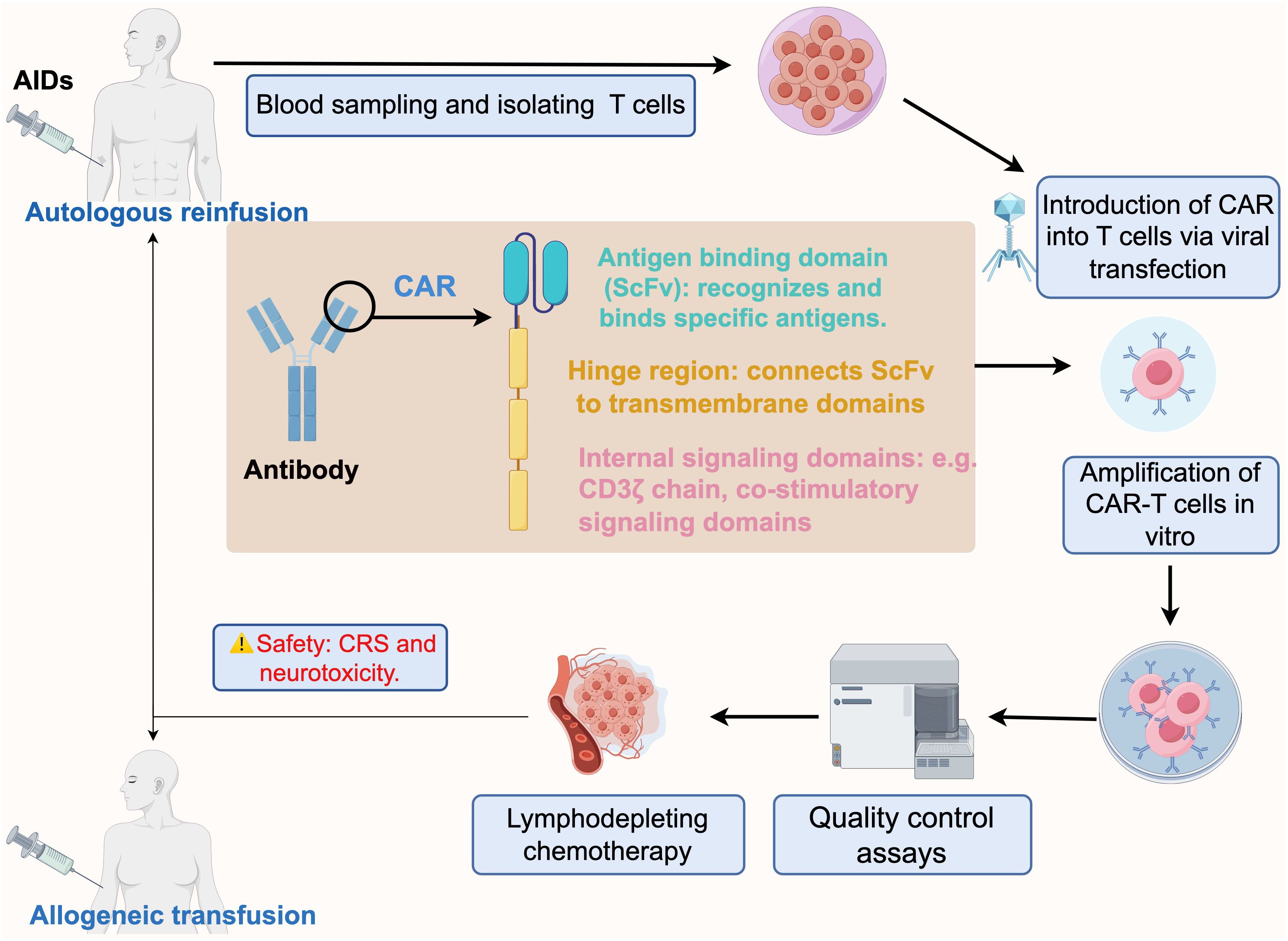
Figure 1. Schematic process of CAR-T immunotherapy for autoimmune diseases, from design to infusion. The CAR design initially incorporates a tumor antigen-specific ScFv that is fused to a major activation signaling component (usually CD3ζ). Blood is taken from patients with autoimmune diseases and T-cells are isolated, and the designed CAR is combined with T-cells via lentiviral transfection to expand CAR-T cells in vitro. The prepared CAR-T cells are subjected to several quality control assays, including sterility, cell activity, CAR expression level, cytotoxicity and specificity tests. Prior to CAR-T cell infusion, patients are usually required to undergo chemotherapy for lymphatic clearance to make space for incoming CAR-T cells. Finally, the qualified CAR-T cells are transfused back into the patient intravenously via autologous or allogeneic cell infusion. CAR-T Chimeric antigen receptor T cells, AIDs autoimmune diseases, ScFv single-chain variable fragment, CRS cytokine release syndrome. By figdraw.
CAR-T cells have been engineered to target CD19 and other B-cell surface antigens with notable success in the treatment of relapsed or refractory B-cell cancers (1).
Inspired by the success in oncology, researchers are exploring the use of CAR approaches in autoimmune diseases, in which current treatments primarily rely on broad-spectrum immunosuppressive drugs for the suppression of self-reactive T cells and B cells that attack the host tissues causing inflammation and damage ultimately leading to loss of organ function.
The success of B-cell-targeted therapies and compelling data from preclinical models of systemic lupus erythematosus (SLE) (2) have supported the use of CAR-T cell therapy in the disease, and clinical trials have demonstrated that CD19 CAR-T cell therapy rapidly induced and sustained remission in refractory SLE without the adverse events typically associated with CAR-T therapy (3).
In addition to SLE, CAR-T cell therapy has also been shown to have considerable potential for type 1 diabetes (T1D), multiple sclerosis, autoimmune arthritis, graft-versus-host disease (GvHD), and host versus graft reaction (HvG). Table 1 summarizes the ongoing clinical trials with CAR-T cell therapy in autoimmune diseases. These trials also include CAR-T cell therapy targeting CD19 and BCMA, the latter expressed by mature autoantibody-producing plasma cells resistant to immunosuppressive regimens.
Despite the promise, significant challenges hinder broader applications of CAR-T therapy in autoimmune diseases. Side effects of CAR-T therapy include cytokine release syndrome (CRS), neurotoxicity, and hematocytopenia (4). Additionally, the economic burden of CAR-T therapy is substantial, largely due to manufacturing expenses and the cost of pre-treatment for lymphocyte depletion and the management of possible adverse events, thus limiting access to these therapies (5).
More recently, CAR engineering of selected immune cells for immunotherapy against tumors and autoimmune diseases has broadened the focus to include double-negative T (DNT) cells, T regulatory cells (Tregs), γδ T cells, and natural killer (NK) cells (Figure 2). This review examines the development, current status, challenges and prospects of CAR-DNT cell, CAR-Tregs, CAR-γδ T cell and CAR-NK cell therapies for the treatment of autoimmune diseases, as well as their potential advantages and limitations as compared to the use of CAR-T cells.
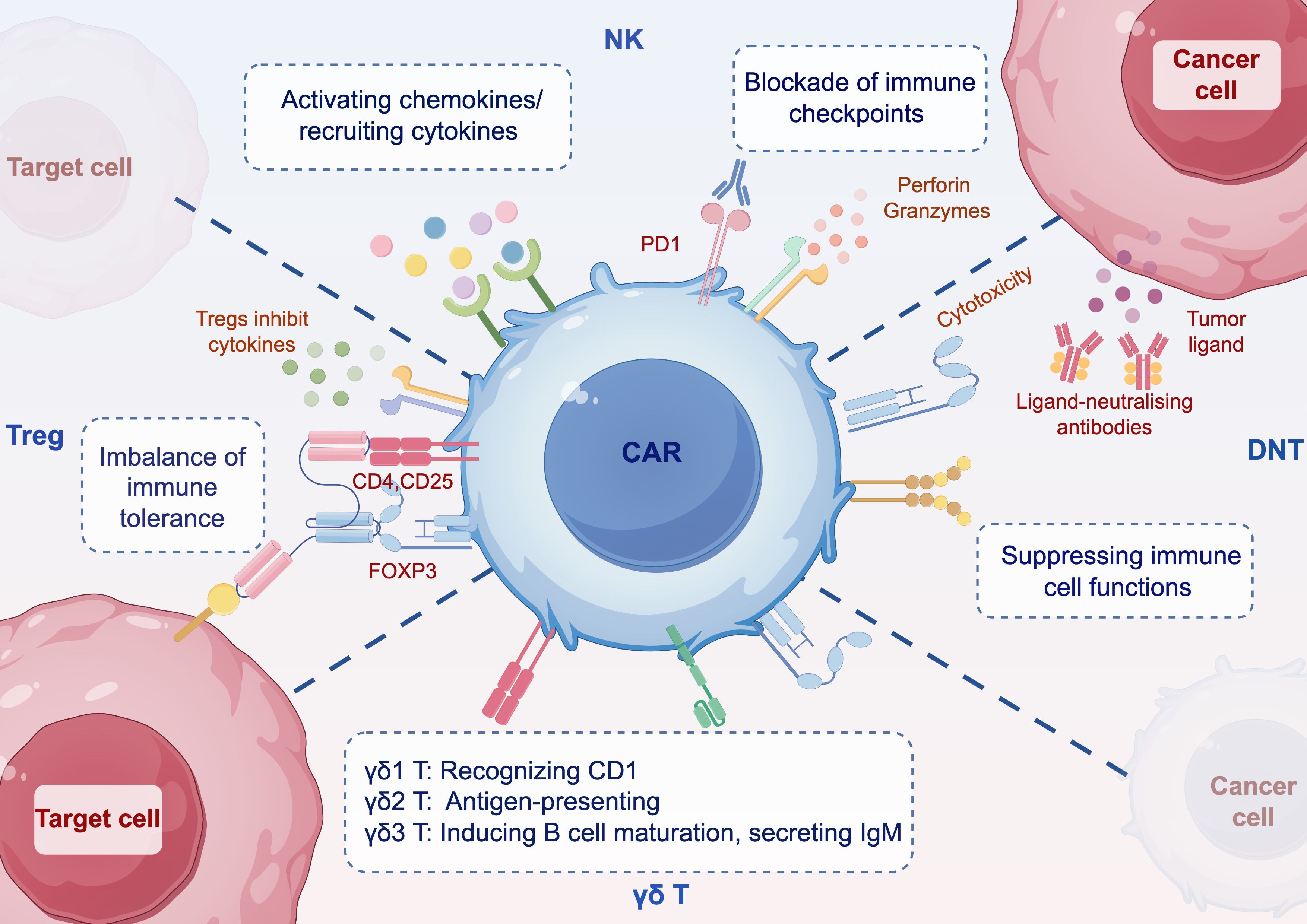
Figure 2. Schematic mechanisms of immune cells with CAR. DNT can inhibit the function of immune cells such as CD4+ and CD8+ T cells, B cells, dendritic cells, NK cells, etc. CAR-DNT cells also have potent antitumor properties. Tregs have immunosuppressive capacity and express CD4, CD25, and the master transcription factor FOXP3. CAR-Treg can modulate the imbalance of immune tolerance. γδ T cells are divided into three main classes in vivo, and targeting γδ T cells can activate or inhibit their functional responses. CAR-γδ T is unaffected by antigen processing and can directly kill tumor cells or target cells. CAR-NK cells can induce an early onset of antitumor activity. Blocking immune checkpoints, CAR-T cells are recruited through chemokines. CAR Chimeric antigen receptor, DNT double negative T cell, Treg regulatory T cell, NK nature killers. By figdraw.
CAR-DNT
T cells express either αβ or γδ chain receptors, in addition to CD4 or CD8 (6). However, a specialized subpopulation of T cells characterized by the absent expression of CD4 and/or CD8, the CD3+CD4-CD8- double-negative T (DNT) cells, accounts for approximately 1-5% of peripheral blood mononuclear cells (PBMCs), both in humans and in mice (7). Like all T cells, DNT cells express either the TCR αβ chain or the γδ chain, enabling them to recognize and respond to antigenic peptides in the adaptive immune response. The precise origin of human DNT cells remains uncertain, although it has been suggested that they may also derive from tissues and organs other than the thymus (8, 9). Functionally, DNT cells have inhibitory effects on CD4+ and CD8+ T cells, B cells, dendritic cells (DCs) and NK cells. This inhibitory capacity contributes to their suppressive role in GvHD and HvG reactions (10). Additionally, DNT cells have potent anti-tumor properties, reducing cancer cell proliferation and infiltration through mechanisms such as elevated production of interferon-γ (IFN-γ) and the expression of Fas ligand (FasL) (11, 12). Notably, DNTs are not restricted by the MHC and can be efficiently expanded in vitro, killing allogeneic and autologous leukemic cells through perforin- and granzyme-dependent processes (13–15).
In addition to their use in oncology, DNTs hold considerable potential for cellular immunotherapy. They can effectively inhibit CD4+ T cell proliferation and selectively suppress mammalian target of rapamycin (mTOR) signaling in these cells, altering metabolic patterns and functions to mimic long-lived central memory T cells and enhancing cell survival (16). Moreover, DNT cells can proliferate in inflamed tissues and contribute to the pathogenesis of autoimmune inflammatory diseases including SLE (17, 18), in which interleukin (IL)-17-producing DNT cells associate with progression of kidney disease, making them potential targets in lupus nephritis (19). Of interest, transfer of DNT cells into psoriatic mice associated with improvement of the inflammatory skin condition (20).
In 2000, Zhang et al. demonstrated antigen-specific inhibitory function of DNT cells in graft rejection in mice (21). In 2022, the same team successfully engineered DNT cells with an anti-CD19-CAR and assessed safety and efficacy in a mouse tumor xenograft model (22). The work confirmed the potential of CAR-DNT cells to target hematologic and solid tumors and reduce/delay acute GvHD (23). Recent studies have assessed the safety and anti-tumor effectiveness of a novel CAR-DNT cell therapy, RJMty19, in patients with relapsed/refractory large B-cell lymphoma (LBCL). This first human, open-label, single-dose, phase 1 study indicated that CD19-CAR-DNT cells are well-tolerated in patients with LBCL and show robust anti-tumor activity. Importantly, neither severe CRS or neurotoxicity was observed, nor were any cases of GvHD or dose-limiting toxicities (DLTs) reported (24). These findings suggest that CAR-DNT therapy may offer a better safety profile compared to conventional CAR-T cell therapies. Furthermore, DNT cells expanded from healthy volunteers under good manufacturing practice (GMP) conditions can be cryopreserved, maintaining their functionality over time both in vitro and in vivo.
The advancement in cellular formulation—from fresh to cryopreserved—holds significant potential, positioning cellular therapies to become readily available as traditional pharmaceuticals. While further investigations into the specific roles and mechanisms of DNT cells in inflammatory or tumor environments are needed, there are currently four CAR-DNT studies registered on Clinicaltrials.gov, two of which focusing on safety and efficacy of CD19-CAR-DNT in the treatment of autoimmune diseases (Table 2).
CAR-Tregs
Tregs are a subset of T cells known for their immunosuppressive capabilities and express CD4, CD25, and the master transcription factor FOXP3. They can be classified into natural Tregs (nTregs), which develop in the thymus, and peripherally induced Tregs (pTregs), the latter differentiating from peripheral naïve CD4+CD25- T cells in the presence of tolerogenic cytokines (25). Since Tregs inhibit T effector cells, in the tumor microenvironment they contribute to cancer immune evasion and represent therefore a major barrier to cancer immunotherapy. On the contrary, a defective Treg number and/function promotes T effector responses to self-antigens and autoimmune disease (26, 27).
Several studies have shown that Tregs are promising for treating autoimmune diseases and preventing GvHD. In 2009, in vitro-expanded autologous polyclonal Tregs were successfully transferred to patients with acute or chronic GvHD (28). In autoimmune diseases, antigen-specific Tregs show higher immunosuppressive potency in various preclinical murine models as compared to classical polyclonal Tregs (29–31). Importantly, antigen specificity of the Tregs can achieved by engineering them to express transgenic TCRs or CARs. These Tregs predominantly localize to sites where target antigens are expressed, reducing risks of systemic immunosuppression. While TCR-engineered Tregs are MHC-dependent, CAR Tregs are MHC-independent and less reliant on IL-2 (32). CARs can also recognize non-protein targets like carbohydrate and glycolipid molecules (33), and CAR Tregs show considerable potential for various immune disorders. For example, CD19-CAR Tregs inhibit antibody production and B cell differentiation, effectively suppressing B cell proliferation and thus a key immune activity implicated in the pathogenesis of SLE. In murine models of SLE, a single infusion of FOXP3-overexpressing anti-CD19 CAR Tregs limited autoantibody production, reduced lymphopenia, and helped restore immune cell compartments in lymphoid organs without toxicity (34). Another study showed that injecting immunodeficient mice reconstituted with human PBMCs with CD19-CAR Tregs suppressed antibody production and reduced GvHD risk (35).
Importantly, in SLE patients, CAR-Tregs efficiently restored immune homeostasis without adverse side effects (3, 34, 35).
The importance of antigen-specific stimulation for CAR-Tregs in CEA transgenic mice with colitis also showed potential in ameliorating ulcerative colitis and hindering colorectal cancer progression (36). Another study designed CAR-Tregs specific for myelin oligodendrocyte glycoprotein to inhibit experimental autoimmune encephalomyelitis (37), a model of multiple sclerosis (MS). In this model, co-expression of FOXP3 and CAR promoted Treg differentiation from naïve CD4 T cells, and the engineered Tregs suppressed ongoing encephalomyelitis (38). Also CAR-Tregs for type 1 diabetes (T1D) localized to the pancreas and regulated localized cellular damage (39).
So far, all phase I/II clinical studies have demonstrated safety of CAR-Treg immunotherapy (40), with no added risk of infection or cancer. Similar to clinical trials on polyclonal Tregs (41), CAR-Tregs show limited persistence. Adjusting treatment regimens, such as combining CAR-Tregs with low-dose IL-2 or removing lymphocytes beforehand might improve viability and persistence (42).
CAR-γδ T
γδ T cells represent a small fraction of T cells, about 1-5% of PBMC. Unlike traditional CD4+ and CD8+ αβ T cells, γδ T cells have innate features such as MHC-independent antigen recognition similar to NK cells and cytotoxic activity. Additionally, they produce chemokines, cytokines, and inflammatory and cytotoxic mediators that enhance inflammatory responses and regulate the differentiation and apoptosis of damaged cells, forming a first line of defense against tumors and infection (43). Human γδ T cells are categorized into three main types based on their δ-chain expression: Vδ1, Vδ2, and Vδ3 T cells. The majority (50-90%) of γδ T cells in the human blood express the Vδ2 TCR. Activated Vδ2 T cells function as professional antigen-presenting cells (APCs), presenting antigens and expressing co-stimulatory molecules and adhesion molecules such as MHC II, CD80, and CD86 (44). The αβ TCRs are restricted to the recognitions of antigen-derived peptides presented by MHC/human leukocyte antigens (HLA) molecules, thus distinguishing them from γδ T cells-which are not constrained by antigen processing and MHC/HLA restriction (45).
In preclinical studies, CAR-γδ T cells showed antitumor efficacy in leukemia models. Transduction of polyclonal γδ T cells with CD19-targeted CAR enhanced IFN-γ and tumor necrosis factor (TNF)-α responses and significantly reduced leukemic load (with comparable transduction efficacy and cytotoxicity to standard CAR-T in vivo) (46, 47). However, for the aggressive ALL cell line in mice, it was impossible to completely clear it, because of the limited persistence of CAR-γδ T cells. Yet, repeated infusions of CAR-γδ T cells improved the anti-leukemic effects (47).
For solid tumors, CAR-γδ T cells may act as professional APCs, inducing endogenous immunity in response to antigenic heterogeneity (48).
In autoimmune diseases, specifically in rheumatoid arthritis, γδ T cells contribute to inflammation by secreting cytokines and inducing inflammatory cells. Several studies have shown that γδ T cells strongly regulate Th17 autoimmune responses in experimental autoimmune uveitis (49) and interact with DCs (50). Higher numbers of γδ T cells have also been observed in the MS white matter plaques and cerebrospinal fluid as compared to peripheral blood (51). An increase in circulating γδ T cells can also be seen in relapsing-remitting MS (RRMS) patients (52). However, numbers of total γδ and γδ2 T cells were significantly lower in MS patients compared to healthy controls, possibly due to apoptosis or migration of γδ2 T cells to the central nervous system (CNS) (53).
γδ T cells help control inflammation and promote disease repair in the CNS through Fas/FasL-induced apoptosis of encephalitogenic T cells. Timely resolution of inflammation is crucial to prevent irreversible CNS damage in chronic diseases (54). Thus, the clinical potential of CAR-modified γδ T cell is enormous, also because CAR-γδ T cells contribute to humoral immunity by targeting and promoting B cell maturation, antibody production, and class switching. They also indirectly regulate αβ T cell activity by activating NK cells, DCs, and B cells. Although CAR γδ T cell immunotherapy is still at early stages, it has shown promise in the field of oncology as well as autoimmune diseases. A total of eight studies on CAR-γδ T have been registered on Clinicaltrials.gov, including two on autoimmune diseases, one for refractory/moderately severe SLE and one for lupus nephritis (Table 3).
CAR-NK
NK cells are innate immune cells that act as the first line of defense against tumors or virally infected cells independently of MHC molecules. NK cell function is modulated by a complex array of activating and inhibitory receptors that determines whether NK cells initiate killing activity against abnormal cells or maintain tolerance to healthy cells (55). At least 90% of peripheral blood NK cells are CD56dimCD16bright, representing the ultimate stage of NK cell maturation. Conversely, CD56bright NK cells are immature populations that more closely resemble helper cells and secrete large amounts of cytokines such as IFN-γ, TNF-β, and granulocyte-macrophage colony-stimulating factor (56, 57). CAR significantly enhances the specificity and potency of NK cells. Due to their unique features, CAR NK cells possess both CAR-mediated targeted killing ability and inherent anti-tumor properties. They can recognize and kill tumor cells even when CAR targets are dowregulated or absent, reducing the chances that tumor cells escape detection, thereby increasing therapeutic efficacy (58). Additionally, NK cells have a lower risk of inducing GvHD (59) and are less likely to cause CRS and severe neurotoxicity than activated T cells (60). Interestingly, NK cells have a limited lifespan, averaging about two weeks (61). This means that any potential toxicity from CAR-NK cells can be self-limiting as the cells naturally disappear. However, this also implies that repeated infusions of CAR-NK cells might be necessary to maintain remission. In 2020, Liu et al. published a phase I clinical trial of CAR-NK cells against CD19-positive lymphoid tumors (62). In this trial, 11 patients with B-lymphoid malignancies were treated, of which 7 achieved complete remission, 6 tested negatives for minimal residual disease, and none experienced increased inflammatory cytokines or adverse effects such as CRS, neurotoxicity, hemophagocytic lymphohistiocytosis, or GvHD. In another phase I trial, NK-92-derived CD33-CAR-NK cells showed good tolerability in three patients with acute myeloid leukemia (NCT02944162). These results demonstrate that CAR-NK cells are a safe strategy for cancer treatment. In autoimmune diseases, Meng et al. developed a chimeric autoantibody receptor (CAAR) targeting the autoantigen La/SSB (63), which is associated with several autoimmune diseases. They introduced this CAAR into NK92MI cells, enabling them to selectively target and destroy La/SSB-reactive autoreactive B-cell clones, providing a new approach to autoimmune disease therapy. Additionally, King et al. showed that treating SLE mice with CAR NK-92 cells reduced splenomegaly and the numbers of CD4+ T cells (64). While CAR NK therapy for autoimmune diseases shows promise, more clinical studies are needed to support and explore its potential. Considering the lifespan of mature NK cells and their transient nature in vivo, persistence will be a major issue affecting clinical outcomes. Of note, it remains controversial whether CAR-NK cell transfusion requires the same lymphocyte-clearing chemotherapy with fludarabine and cyclophosphamide as CAR-T therapy. To date, all published clinical trials have administered lymphocyte-clearing chemotherapy prior to CAR-NK treatment, which may be due to the fact the enrolled patients with refractory disease had received other targeted therapies, such as CAR-T therapy, prior to CAR-NK (65). Alternatively, the investigators administered uniform lymphatic clearance prior to clinical trial in order to observe the effects of different doses of CAR-NK on patients (66). In all, whether eliminating lymphatic chemotherapy is imperative during CAR-NK cell therapy still needs to be explored. Currently, there are 104 CAR-NK programs registered on Clinicaltrials.gov, with 10 focused on autoimmune diseases (Table 4).
Discussion
CAR-T cell therapy has been a landmark achievement in oncology yet still faces obstacles including the immunosuppressive and immunoexclusive tumor microenvironment, tumor antigen heterogeneity, and poor T cell trafficking. For autoimmune diseases, CAR therapy is still in its infancy. Although only a few autoimmune diseases may be suitable for CAR cell therapy, the large number of patients affected by autoimmune diseases means that as the number of approved clinical therapies increases, so will the total number of patients eligible for CAR cell therapy.
Importantly, combinations of CAR immune cells supporting conventional CAR-T cells are overcoming current challenges in oncology treatment. For example, CAR-DNT are not restricted by MHC and efficiently expand in vitro; CAR-Tregs act as naturally inhibitory; and CAR-γδ T cells act as APCs, cross-presenting antigens to conventional αβ CAR-T and endogenous T cells. Similar to CAR-γδ T cells, CAR-NK cells can be used in combination with conventional CAR-T cells or as a bridging therapy prior to CAR-T therapy. Therefore, combining various types of CAR cells to provide synergistic effects is a promising direction for future research.
While some CAR-T products for treating lymphomas and leukamia have been approved by the FDA and have shown impressive anti-tumor effects, CAR-T therapies remain expensive. Cells derived from induced pluripotent stem cells(iPSCs) induced by genetic factors or chemical reagents provide a solution for constructing large-scale standardized CAR cells that can significantly reduce costs. However, inducing functional, modulable immune cells from iPSCs remains a technical challenge.
Overall, despite the many challenges in the development of CAR cell therapy, next generations of CAR beyond CAR-T are emerging with improvement on manufacturing and storage process of CARs, more targeted design, applications in solid tumors, and reduced side effects including CRS, neurotoxicity and thrombocytopenia. As CAR will target diverse immune cells, durable disease remission and more safety will improve prospects of therapeutic management of tumors and autoimmune diseases.
Author contributions
JY: Conceptualization, Data curation, Formal analysis, Investigation, Methodology, Resources, Visualization, Writing – original draft. YY: Data curation, Formal analysis, Methodology, Writing – review & editing, Investigation. ZG: Data curation, Writing – review & editing, Formal analysis, Investigation. MS: Data curation, Methodology, Writing – review & editing. ALC: Data curation, Methodology, Writing – review & editing, Formal analysis, Validation. AL: Conceptualization, Data curation, Funding acquisition, Project administration, Supervision, Validation, Writing – review & editing.
Funding
The author(s) declare financial support was received for the research, authorship, and/or publication of this article. This work was supported by Natural Science Foundation of Hebei Province (H2023206264), S&T Program of Hebei (19277721D) and Medical Science and Research Program of Hebei Province (20240127, 20220999).
Conflict of interest
The authors declare that the research was conducted in the absence of any commercial or financial relationships that could be construed as a potential conflict of interest.
The author(s) declared that they were an editorial board member of Frontiers, at the time of submission. This had no impact on the peer review process and the final decision.
Publisher’s note
All claims expressed in this article are solely those of the authors and do not necessarily represent those of their affiliated organizations, or those of the publisher, the editors and the reviewers. Any product that may be evaluated in this article, or claim that may be made by its manufacturer, is not guaranteed or endorsed by the publisher.
References
1. Schuster SJ, Svoboda J, Chong EA, Nasta SD, Mato AR, Anak Ö, et al. Chimeric antigen receptor T cells in refractory B-cell lymphomas. N Engl J Med. (2017) 377:2545–54. doi: 10.1056/NEJMoa1708566
2. Kansal R, Richardson N, Neeli I, Khawaja S, Chamberlain D, Ghani M, et al. Sustained B cell depletion by CD19-targeted CAR T cells is a highly effective treatment for murine lupus. Sci Transl Med. (2019) 11:eaav1648. doi: 10.1126/scitranslmed.aav1648
3. Mougiakakos D, Krönke G, Völkl S, Kretschmann S, Aigner M, Kharboutli S, et al. CD19-targeted CAR T cells in refractory systemic lupus erythematosus. N Engl J Med. (2021) 385:567–9. doi: 10.1056/NEJMc2107725
4. Miao L, Zhang Z, Ren Z, Li Y. Reactions related to CAR-T cell therapy. Front Immunol. (2021) 12:663201. doi: 10.3389/fimmu.2021.663201
5. Lyman GH, Nguyen A, Snyder S, Gitlin M, Chung KC. Economic evaluation of chimeric antigen receptor T-cell therapy by site of care among patients with relapsed or refractory large B-cell lymphoma. JAMA Netw Open. (2020) 3:e202072. doi: 10.1001/jamanetworkopen.2020.2072
6. Liu C, Lan Y, Liu B, Zhang H, Hu H. T cell development: old tales retold by single-cell RNA sequencing. Trends Immunol. (2021) 42:165–75. doi: 10.1016/j.it.2020.12.004
7. Fischer K, Voelkl S, Heymann J, Przybylski GK, Mondal K, Laumer M, et al. Isolation and characterization of human antigen-specific TCR αβ+ CD4-CD8- double-negative regulatory T cells. Blood. (2005) 105:2828–35. doi: 10.1182/blood-2004-07-2583
8. Palathumpat V, Dejbakhsh-Jones S, Holm B, Strober S. Different subsets of T cells in the adult mouse bone marrow and spleen induce or suppress acute graft-versus-host disease. J Immunol. (1992) 149:808–17. doi: 10.4049/jimmunol.149.3.808
9. Sadasivam M, Noel S, Lee SA, Gong J, Allaf ME, Pierorazio P, et al. Activation and proliferation of PD-1+ kidney double-negative T cells is dependent on nonclassical MHC proteins and IL-2. J Am Soc Nephrol. (2019) 30:277–92. doi: 10.1681/ASN.2018080815
10. Ford MS, Young KJ, Zhang Z, Ohashi PS, Zhang L. The immune regulatory function of lymphoproliferative double negative T cells in vitro and in vivo. J Exp Med. (2002) 196:261–7. doi: 10.1084/jem.20020029
11. Chen B, Lee JB, Kang H, Minden MD, Zhang L. Targeting chemotherapy-resistant leukemia by combining DNT cellular therapy with conventional chemotherapy. J Exp Clin Cancer Res. (2018) 37:88. doi: 10.1186/s13046-018-0756-9
12. Alspach E, Lussier DM, Schreiber RD. Interferon γ and its important roles in promoting and inhibiting spontaneous and therapeutic cancer immunity. Cold Spring Harb Perspect Biol. (2019) 11:a028480. doi: 10.1101/cshperspect.a028480
13. Merims S, Li X, Joe B, Dokouhaki P, Han M, Childs RW, et al. Anti-leukemia effect of ex vivo expanded DNT cells from AML patients: a potential novel autologous T-cell adoptive immunotherapy. Leukemia. (2011) 25:1415–22. doi: 10.1038/leu.2011.99
14. Lee J, Minden MD, Chen WC, Streck E, Chen B, Kang H, et al. Allogeneic human double negative T cells as a novel immunotherapy for acute myeloid leukemia and its underlying mechanisms. Clin Cancer Res. (2018) 24:370–82. doi: 10.1158/1078-0432.CCR-17-2228
15. Voelkl S, Gary R, Mackensen A. Characterization of the immunoregulatory function of human TCR-αβ+ CD4- CD8- double-negative T cells. Eur J Immunol. (2011) 41:739–48. doi: 10.1002/eji.201040982
16. Haug T, Aigner M, Peuser MM, Strobl CD, Hildner K, Mougiakakos D, et al. Human double-negative regulatory T-cells induce a metabolic and functional switch in effector T-cells by suppressing mTOR activity. Front Immunol. (2019) 10:883. doi: 10.3389/fimmu.2019.00883
17. Li H, Tsokos GC. Double-negative T cells in autoimmune diseases. Curr Opin Rheumatol. (2021) 33:163–72. doi: 10.1097/BOR.0000000000000778
18. Bleesing JJ, Brown MR, Novicio C, Guarraia D, Dale JK, Straus SE, et al. A composite picture of TcR αβ+ CD4-CD8- T Cells (αβ-DNTCs) in humans with autoimmune lymphoproliferative syndrome. Clin Immunol. (2002) 104:21–30. doi: 10.1006/clim.2002.5225
19. Qiao G, Yang L, Li Z, Williams JW, Zhang J. A77 1726, the active metabolite of leflunomide, attenuates lupus nephritis by promoting the development of regulatory T cells and inhibiting IL-17-producing double negative T cells. Clin Immunol. (2015) 157:166–74. doi: 10.1016/j.clim.2015.01.006
20. Wei Y, Sun G, Yang Y, Li M, Zheng S, Wang X, et al. Double-negative T cells ameliorate psoriasis by selectively inhibiting IL-17A-producing γδlow T cells. J Transl Med. (2024) 22:328. doi: 10.1186/s12967-024-05132-8
21. Zhang ZX, Yang L, Young KJ, DuTemple B, Zhang L. Identification of a previously unknown antigen-specific regulatory T cell and its mechanism of suppression. Nat Med. (2000) 6:782–9. doi: 10.1038/77513
22. Vasic D, Lee JB, Leung Y, Khatri I, Na Y, Abate-Daga D, et al. Allogeneic double-negative CAR-T cells inhibit tumor growth without off-tumor toxicities. Sci Immunol. (2022) 7:eabl3642. doi: 10.1126/sciimmunol.abl3642
23. Achita P, Dervovic D, Ly D, Lee JB, Haug T, Joe B, et al. Infusion of ex-vivo expanded human TCR-αβ+ double-negative regulatory T cells delays onset of xenogeneic graft-versus-host disease. Clin Exp Immunol. (2018) 193:386–99. doi: 10.1111/cei.13145
24. Xiao X, Liu H, Qiu X, Chen P, Li X, Wang D, et al. CD19-CAR-DNT cells (RJMty19) in patients with relapsed or refractory large B-cell lymphoma: a phase 1, first-in-human study. EClinicalMedicine. (2024) 70 p:102516. doi: 10.1016/j.eclinm.2024.102516
25. La Cava A. Natural tregs and autoimmunity. Front Biosci (Landmark Ed). (2009) 14:333–43. doi: 10.2741/3247
26. Wing K, Sakaguchi S. Regulatory T cells exert checks and balances on self tolerance and autoimmunity. Nat Immunol. (2010) 11:7–13. doi: 10.1038/ni.1818
27. Dominguez-Villar M, Hafler DA. Regulatory T cells in autoimmune disease. Nat Immunol. (2018) 19:665–73. doi: 10.1038/s41590-018-0120-4
28. Trzonkowski P, Bieniaszewska M, Juścińska J, Dobyszuk A, Krzystyniak A, Marek N, et al. First-in-man clinical results of the treatment of patients with graft versus host disease with human ex vivo expanded CD4+CD25+CD127- T regulatory cells. Clin Immunol. (2009) 133:22–6. doi: 10.1016/j.clim.2009.06.001
29. Fu RY, Chen AC, Lyle MJ, Chen CY, Liu CL, Miao CH, et al. CD4+ T cells engineered with FVIII-CAR and murine Foxp3 suppress anti-factor VIII immune responses in hemophilia a mice. Cell Immunol. (2020) 358:104216. doi: 10.1016/j.cellimm.2020.104216
30. Tang Q, Henriksen KJ, Bi M, Finger EB, Szot G, Ye J, et al. In vitro-expanded antigen-specific regulatory T cells suppress autoimmune diabetes. J Exp Med. (2004) 199:1455–65. doi: 10.1084/jem.20040139
31. Sagoo P, Ali N, Garg G, Nestle FO, Lechler RI, Lombardi G, et al. Human regulatory T cells with alloantigen specificity are more potent inhibitors of alloimmune skin graft damage than polyclonal regulatory T cells. Sci Transl Med. (2011) 3:83ra42. doi: 10.1126/scitranslmed.3002076
32. MacDonald KG, Hoeppli RE, Huang Q, Gillies J, Luciani DS, Orban PC, et al. Alloantigen-specific regulatory T cells generated with a chimeric antigen receptor. J Clin Invest. (2016) 126:1413–24. doi: 10.1172/JCI82771
33. Mezzanzanica D, Canevari S, Mazzoni A, Figini M, Colnaghi MI, Waks T, et al. Transfer of chimeric receptor gene made of variable regions of tumor-specific antibody confers anticarbohydrate specificity on T cells. Cancer Gene Ther. (1998) 5:401–7.
34. Doglio M, Ugolini A, Bercher-Brayer C, Camisa B, Toma C, Norata R, et al. Regulatory T cells expressing CD19-targeted chimeric antigen receptor restore homeostasis in Systemic Lupus Erythematosus. Nat Commun. (2024) 15:2542. doi: 10.1038/s41467-024-46448-9
35. Mackensen A, Müller F, Mougiakakos D, Böltz S, Wilhelm A, Aigner M, et al. Anti-CD19 CAR T cell therapy for refractory systemic lupus erythematosus. Nat Med. (2022) 28:2124–32. doi: 10.1038/s41591-022-02017-5
36. Blat D, Zigmond E, Alteber Z, Waks T, Eshhar Z. Suppression of murine colitis and its associated cancer by carcinoembryonic antigen-specific regulatory T cells. Mol Ther. (2014) 22:1018–28. doi: 10.1038/mt.2014.41
37. Fransson M, Piras E, Burman J, Nilsson B, Essand M, Lu B, et al. CAR/FoxP3-engineered T regulatory cells target the CNS and suppress EAE upon intranasal delivery. J Neuroinflamm. (2012) 9:112. doi: 10.1186/1742-2094-9-112
38. De Paula Pohl A, Schmidt A, Zhang AH, Maldonado T, Königs C, Scott DW, et al. Engineered regulatory T cells expressing myelin-specific chimeric antigen receptors suppress EAE progression. Cell Immunol. (2020) 358:104222. doi: 10.1016/j.cellimm.2020.104222
39. Tenspolde M, Zimmermann K, Weber LC, Hapke M, Lieber M, Dywicki J, et al. Regulatory T cells engineered with a novel insulin-specific chimeric antigen receptor as a candidate immunotherapy for type 1 diabetes. J Autoimmun. (2019) 103:102289. doi: 10.1016/j.jaut.2019.05.017
40. Abhishek K, Nidhi M, Chandran S, Shevkoplyas SS, Mohan C. Manufacturing regulatory T cells for adoptive cell therapy in immune diseases: A critical appraisal. Clin Immunol. (2023) 251:109328. doi: 10.1016/j.clim.2023.109328
41. Bluestone JA, Buckner JH, Fitch M, Gitelman SE, Gupta S, Hellerstein MK, et al. Type 1 diabetes immunotherapy using polyclonal regulatory T cells. Sci Transl Med. (2015) 7:315ra189. doi: 10.1126/scitranslmed.aad4134
42. Humrich JY, Morbach H, Undeutsch R, Enghard P, Rosenberger S, Weigert O, et al. Homeostatic imbalance of regulatory and effector T cells due to IL-2 deprivation amplifies murine lupus. Proc Natl Acad Sci U.S.A. (2010) 107:204–9. doi: 10.1073/pnas.0903158107
43. Zhao Y, Niu C, Cui J. γδ T cells: friend or foe in cancer development? J Transl Med. (2018) 16:3. doi: 10.1186/s12967-017-1378-2
44. Moser B, Eberl M. γδ T-APCs: a novel tool for immunotherapy? Cell Mol Life Sci. (2011) 68:2443–52. doi: 10.1007/s00018-011-0706-6
45. Vantourout P, Hayday A. Six-of-the-best: unique contributions of γδ T cells to immunology. Nat Rev Immunol. (2013) 13:88–100. doi: 10.1038/nri3384
46. Deniger DC, Switzer K, Mi T, Maiti S, Hurton L, Singh H, et al. Bispecific T-cells expressing polyclonal repertoire of endogenous γδ T-cell receptors and introduced CD19-specific chimeric antigen receptor. Mol Ther. (2013) 21:638–47. doi: 10.1038/mt.2012.267
47. Rozenbaum M, Meir A, Aharony Y, Itzhaki O, Schachter J, Bank I, et al. Gamma-delta CAR-T cells show CAR-directed and independent activity against leukemia. Front Immunol. (2020) 11:1347. doi: 10.3389/fimmu.2020.01347
48. Capsomidis A, Benthall G, Van Acker HH, Fisher J, Kramer AM, Abeln Z, et al. Chimeric antigen receptor-engineered human γδ T cells: enhanced cytotoxicity with retention of cross presentation. Mol Ther. (2018) 26:354–65. doi: 10.1016/j.ymthe.2017.12.001
49. Ko MK, Shao H, Kaplan HJ, Sun D. CD73+ Dendritic cells in cascading th17 responses of experimental autoimmune uveitis-induced mice. Front Immunol. (2020) 11:601272. doi: 10.3389/fimmu.2020.601272
50. Sun D, Ko MK, Shao H, Kaplan HJ. Augmented Th17-stimulating activity of BMDCs as a result of reciprocal interaction between γδ and dendritic cells. Mol Immunol. (2021) 134:13–24. doi: 10.1016/j.molimm.2021.02.023
51. Freedman MS, Ruijs TC, Selin LK, Antel JP. Peripheral blood γδ T cells lyse fresh human brain-derived oligodendrocytes. Ann Neurol. (1991) 30:794–800. doi: 10.1002/ana.410300608
52. Monteiro A, Cruto C, Rosado P, Martinho A, Rosado L, Fonseca M, et al. Characterization of circulating γδ T cells in relapsing vs remission multiple sclerosis. J Neuroimmunol. (2018) 318:65–71. doi: 10.1016/j.jneuroim.2018.02.009
53. Ramos S, Brenu E, Broadley S, Kwiatek R, Ng J, Nguyen T, et al. Regulatory T, natural killer T and γδ T cells in multiple sclerosis and chronic fatigue syndrome/myalgic encephalomyelitis: a comparison. Asian Pac J Allergy Immunol. (2016) 34:300–5. doi: 10.12932/AP0733
54. Ponomarev ED, Dittel BN. γδ T cells regulate the extent and duration of inflammation in the central nervous system by a Fas ligand-dependent mechanism. J Immunol. (2005) 174:4678–87. doi: 10.4049/jimmunol.174.8.4678
55. Lanier LL. Up on the tightrope: natural killer cell activation and inhibition. Nat Immunol. (2008) 9:495–502. doi: 10.1038/ni1581
56. Wagner JA, Rosario M, Romee R, Berrien-Elliott MM, Schneider SE, Leong JW, et al. CD56bright NK cells exhibit potent antitumor responses following IL-15 priming. J Clin Invest. (2017) 127:4042–58. doi: 10.1172/JCI90387
57. Cooper MA, Fehniger TA, Turner SC, Chen KS, Ghaheri BA, Ghayur T, et al. Human natural killer cells: a unique innate immunoregulatory role for the CD56bright subset. Blood. (2001) 97:3146–51. doi: 10.1182/blood.V97.10.3146
58. Souza-Fonseca-Guimaraes F, Cursons J, Huntington ND. The emergence of natural killer cells as a major target in cancer immunotherapy. Trends Immunol. (2019) 40:142–58. doi: 10.1016/j.it.2018.12.003
59. Xie G, Dong H, Liang Y, Ham JD, Rizwan R, Chen J, et al. CAR-NK cells: A promising cellular immunotherapy for cancer. EBioMedicine. (2020) 59:102975. doi: 10.1016/j.ebiom.2020.102975
60. Pan K, Farrukh H, Chittepu VCSR, Xu H, Pan CX, Zhu Z, et al. CAR race to cancer immunotherapy: from CAR T, CAR NK to CAR macrophage therapy. J Exp Clin Cancer Res. (2022) 41:119. doi: 10.1186/s13046-022-02327-z
61. Zhang Y, Wallace DL, de Lara CM, Ghattas H, Asquith B, Worth A, et al. In vivo kinetics of human natural killer cells: the effects of ageing and acute and chronic viral infection. Immunology. (2007) 121:258–65. doi: 10.1111/j.1365-2567.2007.02573.x
62. Liu E, Marin D, Banerjee P, Macapinlac HA, Thompson P, Basar R, et al. Use of CAR-transduced natural killer cells in CD19-positive lymphoid tumors. N Engl J Med. (2020) 382:545–53. doi: 10.1056/NEJMoa1910607
63. Meng H, Sun X, Song Y, Zou J, An G, Jin Z, et al. La/SSB chimeric autoantibody receptor modified NK92MI cells for targeted therapy of autoimmune disease. Clin Immunol. (2018) 192:40–9. doi: 10.1016/j.clim.2018.04.006
64. King C. CAR NK cell therapy for T follicular helper cells. Cell Rep Med. (2020) 1:100009. doi: 10.1016/j.xcrm.2020.100009
65. Marin D, Li Y, Basar R, Rafei H, Daher M, Dou J, et al. Safety, efficacy and determinants of response of allogeneic CD19-specific CAR-NK cells in CD19 B cell tumors: a phase 1/2 trial. Nat Med. (2024) 30:772–84. doi: 10.1038/s41591-023-02785-8
Keywords: CAR-T cells, autoimmune diseases, immunotherapy, double-negative T cells, γδ T cells, T regulatory cells, natural killer cells
Citation: Yu J, Yang Y, Gu Z, Shi M, La Cava A and Liu A (2024) CAR immunotherapy in autoimmune diseases: promises and challenges. Front. Immunol. 15:1461102. doi: 10.3389/fimmu.2024.1461102
Received: 07 July 2024; Accepted: 30 August 2024;
Published: 01 October 2024.
Edited by:
Michael A. Firer, Ariel University, IsraelReviewed by:
Sun Jung Kim, Northwell Health, United StatesSunil Nagpal, GlaxoSmithKline, United States
Copyright © 2024 Yu, Yang, Gu, Shi, La Cava and Liu. This is an open-access article distributed under the terms of the Creative Commons Attribution License (CC BY). The use, distribution or reproduction in other forums is permitted, provided the original author(s) and the copyright owner(s) are credited and that the original publication in this journal is cited, in accordance with accepted academic practice. No use, distribution or reproduction is permitted which does not comply with these terms.
*Correspondence: Aijing Liu, bGFqMTExQDEyNi5jb20=; YWpsaXVAaGVibXUuZWR1LmNu; Antonio La Cava, QUxhQ2F2YUBtZWRuZXQudWNsYS5lZHU=
†These authors have contributed equally to this work