- 1Immunomodulation and Vaccines Focus Area, Vaccine Research Theme, Medical Research Council/Uganda Virus Research Institute and London School of Hygiene and Tropical Medicine (MRC/UVRI and LSHTM) Uganda Research Unit, Entebbe, Uganda
- 2Medical Research Council (MRC) International Statistics and Epidemiology Group, Department of Infectious Disease Epidemiology, London School of Hygiene and Tropical Medicine, London, United Kingdom
- 3Department of Infection Biology, London School of Hygiene and Tropical Medicine, London, United Kingdom
- 4Department of Cell and Chemical Biology, Leiden University Medical Center, Leiden, Netherlands
- 5Department of Parasitology, Leiden University Medical Center, Leiden, Netherlands
- 6Department of Clinical Research, London School of Hygiene and Tropical Medicine, London, United Kingdom
Background: Variations in vaccine responses have been observed between populations. A role for helminth infections has been proposed due to their immunomodulatory properties. In a secondary analysis of data from a randomised trial assessing effects of anthelminthic treatment on vaccine responses, we examined associations between helminth infections at baseline prior to vaccine administration, and vaccine responses among adolescents (9-17 years) in Koome Islands, Lake Victoria, Uganda.
Methods: Participants received BCG [week 0], yellow fever (YF-17D), oral typhoid (Ty21a), HPV-prime [week 4], and HPV-boost, tetanus/diphtheria [week 28]. Outcomes were BCG-specific interferon-γ ELISpot responses and antibody responses to yellow-fever-, typhoid-, HPV-, tetanus- and diphtheria-specific antigens measured at two time points post vaccination. S. mansoni infection was determined as positive if either the plasma Circulating Anodic Antigen (CAA) assay or stool PCR were positive. Hookworm and Strongyloides were determined by stool PCR. Linear mixed effects regression was used to assess associations.
Results: Among 478 adolescents, 70% were Schistosoma mansoni (Sm) infected and 23% hookworm infected at baseline. Sm was associated with lower Salmonella Typhi O:LPS-specific IgG responses (adjusted geometric mean ratio (aGMR) 0.69 (0.57-0.83)), and hookworm with higher diphtheria-specific IgG (aGMR 1.16 (1.02, 1.31)) and lower HPV-16-specific IgG (aGMR 0.70 (0.55, 0.90)) post-vaccination. High Sm intensity was associated with lower BCG-specific interferon-γ and S. Typhi O:LPS-specific IgG.
Conclusions: We found inverse associations between Sm and responses to two live vaccines, whereas hookworm was positively associated with diphtheria-specific IgG. These findings support the hypothesis that helminth infections can modulate vaccine responses, while also highlighting potential heterogeneity in the direction of these effects.
Introduction
Vaccination is an essential tool in the prevention of infectious diseases. However, the efficacy and immunogenicity of some commonly used vaccines vary greatly by population, with low and middle income as well as rural populations often exhibiting impaired responses (1, 2). Several vaccines are affected, notably the Bacillus Calmette-Guérin (BCG) vaccine, whose efficacy against pulmonary tuberculosis varies around the world, with lower efficacy observed in regions near the equator (2). These settings are characterised by high exposure to immunomodulating infections, including parasites such as helminths (3). Alongside other factors, parasitic infections have been implicated as a potential cause of impaired efficacy and immunogeneicity.
Parasitic helminths infect both humans and animals. The most common types of helminths that infect humans are soil-transmitted helminths (3), which are transmitted via contaminated soil and can cause intestinal morbidity, anaemia and impaired growth, and schistosomes (4), which are a type of flatworm that are transmitted via freshwater snails and can cause a disease called schistosomiasis. The prevalence and distribution of these parasites varies by region and population (3, 5).
It is estimated that approximately 24% of the global population is infected with one or more soil-transmitted helminths, most commonly hookworm, Ascaris, and whipworm. Most of these infections are found in sub-Saharan Africa, the Americas, and East Asia (3). Schistosomiasis is estimated to affect nearly 240 million people globally (5). The majority of people affected by schistosomiasis due to Schistosoma mansoni infection live in Africa, the Middle East, the Caribbean, Brazil, Venezuela, or Suriname (5). The distribution of schistosomiasis is highly focal, being driven by proximity to fresh water. For example, a national representative survey conducted in Uganda in 2017 estimated the prevalence of schistosomiasis to be around 29% (6). However, within the country, the prevalence of helminths varies by region. In the islands of Lake Victoria, helminths, primarily S. mansoni, affect nearly 70% of the population (7). Despite the use of mass drug administration with praziquantel, the only widely-used treatment option for schistosomiasis, prevalence remains high often due to high reinfection rates.
One way in which helminths may impact vaccine responses is through modulation of immune responses. Helminths secrete a range of molecules that can modulate the activity of immune cells, including T cells, B cells, and macrophages (8). For example, helminths have been shown to alter the balance of T helper cell subsets, leading to a shift in Th2 and regulatory T cell responses (8). They also affect antigen presenting cells (APCs); for example, by driving an alternatively activated macrophage phenotype (9) or dendritic cell-mediated induction of regulatory responses (10). This may be important when encountering a new vaccine antigen thereby potentially affecting the ability of vaccines to elicit an effective immune response. Why some vaccine responses appear to be more affected than others is not fully known; however, helminths, for example, may modify the immune system’s reaction to live vaccines by modifying innate immune response profiles (11).
Reviews found that, in animal models, helminths generally impair priming and accelerate waning of vaccine responses (12, 13). Studies in humans have found more mixed results when investigating the effect of helminths on vaccine responses (12). For example, one study conducted in Ethiopia found that anthelminthic treatment improved IFN-γ and IL-12 cellular responses to purified protein derivative (PPD) post-BCG vaccination, while the untreated group had higher PPD-specific TGF-β responses, implying a role for helminth-induced immunosuppression in impaired BCG responses (14) However, another study conducted in Ecuador did not find a similar effect on responses to BCG (15). Our recent meta-analysis on the effect of helminths on vaccine responses found that generally helminths are associated with lower responses and that the effects are vaccine specific, and subject to a high level of heterogeneity (12).
The mixed findings from available studies suggest that the effect of helminths on vaccine responses may vary depending on the specific vaccine and the host immune response. In this secondary analysis of data from a randomised trial, we sought to investigate the association of helminth infections with vaccine immune responses to a panel of vaccines administered to Ugandan adolescents living in a helminth-endemic setting. The portfolio of licenced vaccines assessed comprised live and inert, oral, and parental, priming, and boosting vaccines.
Materials and methods
Study setting, design, participants, data
This was a secondary analysis of data from the longitudinal ‘POPVAC A’ trial. The trial assessed the effect of intensive treatment of schistosomiasis on vaccine responses (16, 17). It was conducted among adolescents (9-17 years) living in the schistosomiasis-endemic Koome islands of Lake Victoria, Uganda. The study setting is located approximately 60 minutes from the closest mainland by powered boat and comprises mainly fishing communities (Figure 1). Participants were recruited from eight selected primary schools in the islands.
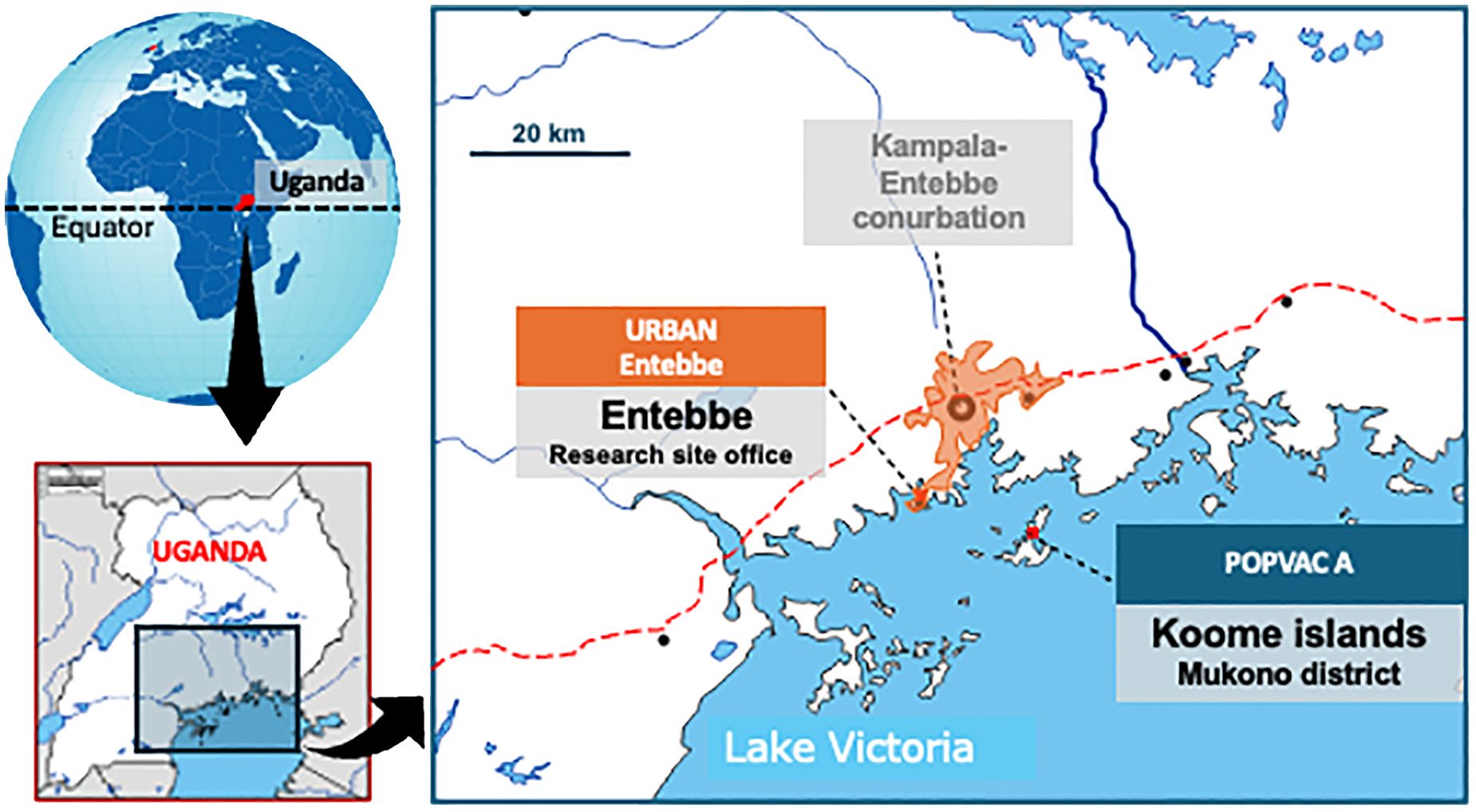
Figure 1. POPVAC A study site: The map of the study site shows Koome islands in Lake Victoria, where the study took place. These Islands are approximately 60 minutes from the nearest urban setting of Entebbe by a powered boat.
Socio-demographic characteristics of participants were collected using a questionnaire at baseline (six weeks prior to the first vaccination). For vaccinations, we selected a portfolio that would provide insights into the effects of both live and inert, oral and parenteral vaccines, while also being valuable to the age-group and communities under study. Six vaccines were administered: live parenteral BCG (Serum Institute of India, 0.1ml intradermally, right upper arm), live yellow fever (YF-17D, Sanofi Pasteur, France 0.5ml intramuscularly [IM], left upper arm), live oral typhoid (Ty21a, Vivotif, PaxVax, UK: three capsules, one per day taken every other day), virus-like particle Human Papillomavirus vaccine (HPV; Gardasil, Merck & Co, USA, 0.5ml IM, left upper arm) and toxoid vaccines (Tetanus/Diphtheria, Td, Serum Institute of India, 0.5ml IM, left upper arm), according to the schedule shown in Figure 2, i.e. BCG at week 0; YF-17D, Ty21a, and HPV at week 4; and Td at week 28. The trial enrolled 478 participants, half received intensive treatment for schistosomiasis (n=239) and the other half received standard treatment (n=239). Participants in the intensive arm received three doses of praziquantel (PZQ), 40mg/kg, at -6, -4 and -2 weeks, prior to the first vaccination, BCG at week 0, followed by PZQ at 8 weeks (after samples were taken for assessing vaccine responses at this time point) and then quarterly (weeks 20, 44 and 52) PZQ until the end of the 12-month follow-up. Participants in the standard arm received a dose of PZQ after week 8 and 52 samples had been taken; this is equivalent to standard of care which recommends annual treatment in schistosomiasis-endemic communities in Uganda.
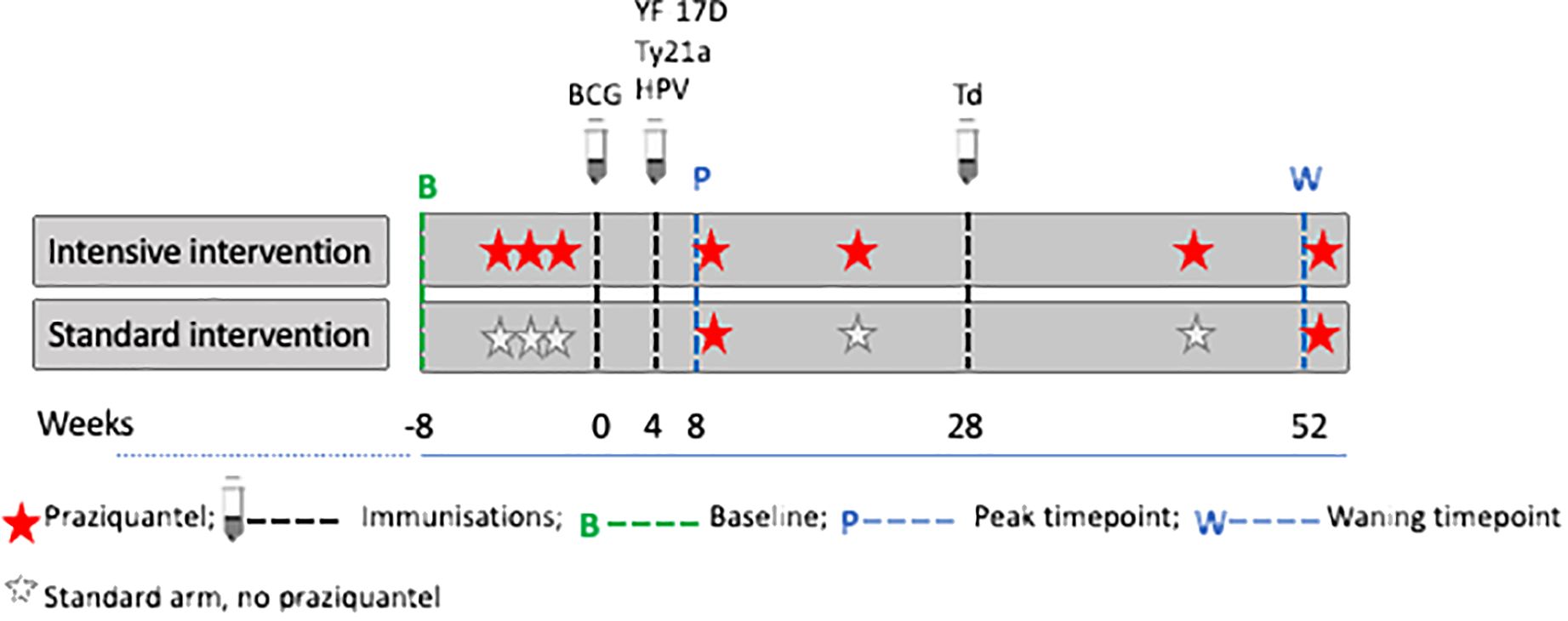
Figure 2. Praziquantel treatment, vaccine administration and response measurement time points. Figure adapted from (16). Peak timepoints were at 8 weeks post BCG and 4 weeks post yellow fever (YF- 17D), oral typhoid (Ty21a), human papilloma virus (HPV) and 24 weeks post tetanus/diphtheria (Td) vaccination. Waning timepoints were at 52 weeks post BCG and 48 weeks post YF- 17D, Ty21a, and HPV.
Outcomes and exposures
Except for Td, responses to vaccines for each participant were measured at three time points, i.e., pre-vaccination (at week 0, i.e. when BCG was administered); the second measurements were taken at 8 weeks post-BCG and 4 weeks post-YF, Ty21a, and HPV (peak timepoint); the third measurements were taken at 52 weeks post-BCG i.e. 48 weeks post-YF, Ty21a and HPV (waning timepoint). Responses to tetanus and diphtheria were measured at week 28 (pre-vaccination for Td) and at week 52 (24 weeks after Td vaccination). The treatment, vaccine administration and response measurement time points are shown in Figure 2.
Vaccine response measurements were BCG-specific interferon gamma (IFN-γ) ELISpot responses, YF-17D 50% and 90% neutralising antibody titres (assessed using plaque reduction neutralising reference tests, PRNTs), S. Typhi O-lipopolysaccharide (O:LPS)-specific IgG, HPV type 16 and type 18 L1 protein-specific IgG concentration, tetanus toxoid-specific IgG, and diphtheria toxoid-specific IgG. Assay details were previously reported (17) and are provided in the Supplementary Information.
Exposures were S. mansoni, hookworm, and Strongyloides stercoralis infections, assessed at baseline (before any intervention). S. mansoni infection was determined as positive if either the plasma Circulating Anodic Antigen (CAA) assay or stool PCR were positive. Hookworm and Strongyloides were determined by stool PCR. Helminth infection assessments were previously reported (17) and are further detailed below.
Plasma detection of Schistosoma circulating anodic antigen
Baseline S. mansoni infection was determined retrospectively through measurement of plasma Circulating Anodic Antigen (CAA), using the up-converting phosphor lateral flow (UCP-LF) assay with a SCAA20 test format (30 pg/ml positivity threshold) (18). Briefly, Human negative serum (obtained from the Uganda blood bank, Nakasero) was spiked with a known concentration of CAA standard (100,000 pg/ml) and diluted up to eight standard points, with two negative controls. These were used to generate a standard curve to quantify the plasma sample CAA levels. Plasma and standards (50 µl) were extracted with an equal volume of 4% w/v trichloroacetic acid (TCA; Merck Life Science NV, the Netherlands), vortexed and incubated at ambient temperature and centrifuged. The resulting supernatant (20µl) was added to the wells containing 100 ng dry UCP particles (19) (400 nm Y2O2S:Yb3+, Er3+) coated with mouse monoclonal anti-CAA antibodies (20) hydrated with 100µl of high salt lateral flow buffer (HSLF: 200 mM Tris pH8, 270 mM NaCl, 0.5% (v/v) Tween-20, 1% (w/v) BSA). These were incubated for one hour at 37°C while shaking at 900 rpm. The CAA lateral flow strips (21) were labelled with the standard and sample identifications and then placed in the wells on the UCP plate. The samples and standards were allowed to flow and left to dry overnight. The strips were then analysed using the Labrox Upcon reader (Labrox Oy, Turku, Finland).
Stool PCR detection of Schistosoma mansoni, Necator americanus and Strongyloides stercoralis DNA
Stool samples stored at -800C in 95% molecular grade ethanol were retrieved and thawed at room temperature. Total DNA (and hence helminth DNA, if present) was extracted from the stool samples using the Fast DNA Spin Kit for Feces (catalogue number 116570200, MP Biomedicals Germany GmbH). The multiplex real-time PCR was adapted from existing procedures (22, 23). The following are the specific forward (F) and reverse (R) primers and TaqMan® probes that were used to simultaneously detect DNA from three helminth species: Necator americanus (Na58F: 5’-CTGTTTGTCGAACGGTACTTGC-3’; Na158R: 5’- ATAACAGCGTGCACATGTTGC-3’; Nec-2-FAM (MGB): FAM-5’-CTGTACTACGCATTGTATAC-3’-XS; Nec-3-FAM (MGB): 5’-CTGTACTACGCATTGTATGT-3’), Schistosoma mansoni (Ssp48F: 5’-GGTCTAGATGACTTGATYGAGATGCT-3’; Ssp124R: 5’-TCCCGAGCGYGTATAATGTCATTA-3’; Ssp78T-RT: Texas Red-5’-TGGGTTGTGCTCGAGTCGTGGC-3’-BHQ2), Strongyloides stercoralis (Stro18S-1530F: 5’-GAATTCCAAGTAAACGTAAGTCATTAGC-3’; Stro18S-1630R: 5’-TGCCTCTGGATATTGCTCAGTTC-3’; Stro-4-TRBhq2-VIC- 5’-ACACACCGSCCGTCGCTGC-3’). Phocine herpes virus (PhHV) DNA, extracted from the Phocine herpes virus (kindly provided by Dr. Martin Schutten, Erasmus Medical Center, Rotterdam, the Netherlands), was included in the PCR master mix, thus distributed to all reaction wells as an internal control to check for PCR inhibition. The PhHV forward primer PhHV-267s (5’-GGGCGAATCACAGATTGAATC-3’), reverse primer PhHV-337as (5’-GCGGTTCCAAACGTACCAA-3’) and probe PhHV-305tq (Cy5-5’ TTTTTATGTGTCCGCCACCATCTGGATC-3’-BHQ2) were used for Phocin herpes virus DNA detection. A positive pool was included on the plate for every run as a test control. The positive pool was made up of a mixture of DNA from samples (from among the study samples) that were highly positive for S. mansoni and N. americanus on Kato-Katz, and for S. stercoralis by PCR (conducted on samples from a previous study). The amplification conditions were 15 minutes at 95°C, 50 cycles of 15 seconds at 95°C, 30s at 60°C and 30s at 72°C. DNA amplification, detection and data analysis were attained with the ABI 7500 Fast Real time machine and 7500 Fast systems software version 1.5.1.
Statistical methods
The current analysis aimed to assess associations between baseline helminth infection status and responses to BCG, YF, HPV, Ty21a and Td, following vaccination with these vaccines. Baseline characteristics at enrolment prior to any interventions were summarised by S. mansoni and hookworm infection statuses and Chi-square tests (for categorical variables) and Wilcoxon rank sum tests (for continuous variables) were performed to examine if there were differences in the baseline characteristics between the groups.
A linear regression analysis was used to determine whether there was an association between baseline helminth infection status and vaccine responses at the peak and waning timepoints separately. Because vaccine responses had skewed distributions, they were log10 transformed for analysis. Geometric means and Geometric mean ratios (GMR) with 95% confidence intervals (CI) were obtained and presented. Both univariable and multivariable results, adjusted for confounders, are presented. Confounders were included in the multivariable models based on their plausible relationship with exposures and outcomes as identified in the literature. Multivariable models controlled for the following potential confounders: age, sex, body mass index, history of anthelminthic treatment, immunisation history, malaria infection and antibodies, the environment (village, town/city) where the participant lived at birth and five years and diet at baseline. Diet information was collected as the number of days in a week when fruits, vegetables, fish, and meat are consumed. For analysis, a diet score was calculated as the sum of days of consumption. The score ranged between 0 and 28, with a score of 28 representing daily consumption of the four food items every week i.e. greater variety of foods consumed.
For BCG, yellow fever, oral typhoid and HPV where responses after vaccinations were measured at the peak and waning timepoints, a linear mixed model with random intercepts was used to examine the associations of S. mansoni and hookworm infections with vaccine responses while controlling for confounders. In this analysis, an interaction between infection status and trial arm was explored. This was to determine whether associations between infections and vaccine responses differed depending on what treatment participants received after baseline infection status was determined. The analysis included responses at peak and waning time points. The use of a linear mixed model allowed us to account for dependencies in the data, arising from repeated measurements of the vaccine responses for each participant.
The analyses described above were repeated, but this time focusing on S. mansoni intensity levels as the main exposure rather than infection status. The intensity categories were based on tertiles for high, medium, and low intensity. This was done for S. mansoni only because the other helminths were less prevalent.
Stata 18.0 (College Station, Texas, USA) and R version 4.2.0 (R Foundation for Statistical Computing, Vienna, Austria) were used to analyse the data. For all analyses, a 5% significance level was used.
Ethics approval
Ethics approval was granted by the Uganda Virus Research Institute Research Ethics Committee (references: GC/127/18/09/680, GC/127/19/05/664), the London School of Hygiene and Tropical Medicine Observational/Interventions Research Ethics Committee (reference: 16032), the Uganda National Council for Science and Technology (reference: HS2486) and the Uganda National Drug Authority (reference: CTA0093).
Results
Participants’ characteristics
The study enrolled 478 adolescents, of whom 335 (70.1%) were S. mansoni positive. Among 475 participants with baseline information on other helminth species (excluding 3 for not having a stool sample), 111 (23.4%) were Necator americanus (hookworm) positive and 38 (8.0%) were Strongyloides stercoralis positive. Baseline characteristics of all study participants, as well as categorised by helminth infection status, are presented in Table 1. The median age of participants at enrolment was 11 years (interquatile range (IQR) 10.0–13.0) with 57.7% being male. S. mansoni-positive participants were more likely to be born in a village setting, have a lower diet score, come from a household without a toilet and rely on lake or spring water for drinking rather than well or piped water. Hookworm-positive participants were more likely to be male and have a higher diet score. Other characteristics were similar between the helminth infection status groups.
Pre-vaccination responses to vaccine antigens
Responses to selected vaccine antigens may be detected before adolescent vaccination (pre-vaccination) due to previous receipt of the same vaccines (for example in infancy for BCG, tetanus and diphtheria) or due to exposure to the target pathogen or cross-reactive organisms. Prior to vaccination during the study, S. mansoni positive (CAA/PCR) participants had lower BCG-specific IFN-γ responses (median 58.3 (IQR: 33.3-103.3) compared to 89.2 (IQR: 36.7-150.0), p = 0.018). Conversely, hookworm-positive participants had higher BCG-specific IFN-γ responses (91.7 (IQR:50.0-123.3) versus 61.7 (IQR: 31.7-105.0), p = 0.039) and higher HPV-16-specific IgG responses (4.0 (IQR: 2.8-6.9) versus 3.5 (IQR: 2.3 5.4), p = 0.048). S. mansoni and hookworm infected participants had comparable yellow fever PRNT50 and PRNT90 titres, S. Typhi O:LPS-specific IgG, HPV-18 IgG, tetanus toxoid-specific IgG, and diphtheria toxoid-specific IgG responses pre-vaccination compared to negative participants (Table 2).
Association between pre-vaccination characteristics and vaccine responses
Supplementary Tables 1, 2 present the results of analyses focusing on the crude associations between vaccine responses at each time point and various baseline characteristics, including exposures of interest (with focus on the most prevalent baseline helminths, S. mansoni and hookworm). Except for yellow fever titres four weeks after vaccination, post-vaccination responses to all vaccines investigated exhibited significant positive associations with their corresponding pre-vaccination responses (Figure 3).

Figure 3. Crude associations between pre and post vaccination responses. The dots represent geometric mean ratios, and the bars 95% confidence intervals. The horizontal dashed line shows the null value of 1. IFN-g: interferon-γ; YF50: Yellow fever titres based on plaque reduction neutralizing reference tests at 50% neutralisation; YF90: Yellow fever titres based on plaque reduction neutralizing reference tests at 90% neutralisation; HPV-16: Human Papillomavirus type 16; HPV-18: Human Papillomavirus type 18.
At the peak timepoint, we observed that S. mansoni infection did not show a crude association with any vaccine responses. However, hookworm infection was inversely associated with HPV-16 responses, with a geometric mean ratio of 0.70 (95% CI 0.52, 0.93). Higher age was inversely associated with HPV 16 and 18-specific IgG responses, while displaying positive associations with tetanus and diphtheria toxoid-specific IgG responses (Supplementary Table 1).
We also observed sex related differences, with males exhibiting significantly lower HPV and tetanus toxoid-specific antibody responses compared to females. Participants who reported not receiving immunisation as babies showed lower tetanus toxoid-specific IgG responses, while those who reported receiving immunisation after starting school displayed lower yellow fever PRNT50 and PRNT90 titres and higher HPV responses. Participants in the intensive arm of the study had higher BCG-specific IFN-γ responses as reported elsewhere (Supplementary Table 1) (17).
Interestingly, participants who reported treatment of worm infection within 12 months prior to enrolment exhibited higher diphtheria toxoid-specific IgG responses. Current malaria infection and higher malaria-specific antibodies were associated with lower HPV responses. Moreover, participants from households that reported toilet ownership had higher BCG-specific IFN-γ, yellow fever PRNT50 and PRNT90 responses. Similarly, individuals whose drinking water source was from a well or piped source demonstrated higher BCG-specific IFN-γ responses but lower S. Typhi O:LPS-specific IgG responses. Lastly, a more varied diet was associated with higher BCG-specific IFN-γ responses (Supplementary Table 1).
At the waning timepoint, S. mansoni infection at baseline was inversely associated with S. Typhi O:LPS-specific IgG, while hookworm infection was inversely associated with tetanus toxoid-specific IgG. There were lower BCG-specific IFN-γ and yellow fever PRNT50 responses among participants with heavy S. mansoni infection compared to uninfected participants. Participants with medium infection intensity had lower HPV-18 responses compared to the uninfected participants. Males had significantly lower yellow fever and HPV titres. A higher body mass index was crudely associated with higher S. Typhi O:LPS-specific IgG responses. Participants who reported not being immunised as babies had significantly higher yellow fever PRNT50 and PRNT90 titres and lower HPV 16 responses (Supplementary Table 2). Plots of individual vaccine response profiles at each time point (pre-vaccination (week 0) and post vaccination (weeks 8 and 52)) by S. mansoni infection status and by S. mansoni intensity at baseline are shown in Supplementary Figures S1, S2.
Figure 4 shows associations between helminth infection and vaccine responses after adjusting for confounders. The analysis was first conducted separately for responses at each timepoint. S. mansoni infection at baseline was associated with lower S. Typhi O:LPS-specific IgG responses four weeks (aGMR 0.67 (95% CI 0.52, 0.86)) and 48 weeks (aGMR 0.74 (0.60-0.92)) after oral typhoid vaccination. Infection with hookworm at baseline was associated with lower HPV-16 responses (aGMR 0.66 (0.51, 0.86)) 44 weeks after vaccination and higher diphtheria-specific IgG responses 24 weeks after vaccination (aGMR 1.157 (1.02-1.31)). Detailed adjusted associations of helminth infection status and S. mansoni intensity with vaccine responses are shown in Supplementary Tables 3, 4.
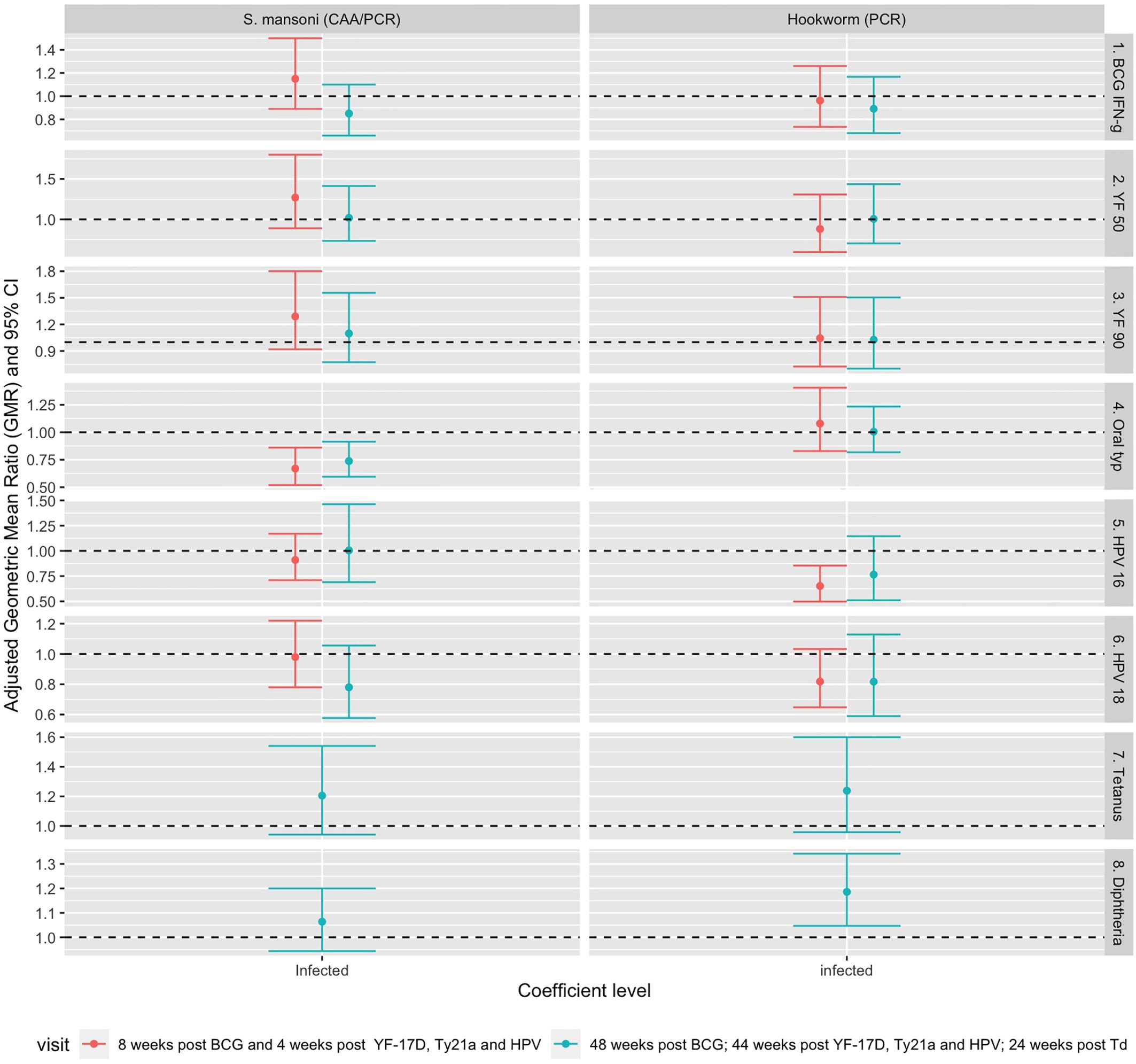
Figure 4. Adjusted association between helminth infections and vaccine responses. Shown are geometric mean rations (dots) and 95% CI (bars). The horizontal dashed line shows the null value of 1. S. mansoni: Schistosoma mansoni; CAA, circulating anodic antigen; PCR, Polymerase Chain Reaction; IFN-g, interferon-γ; YF50, Yellow fever titres based on plaque reduction neutralizing reference tests at 50% neutralisation; YF90, Yellow fever titres based on plaque reduction neutralizing reference tests at 90% neutralisation; HPV-16, Human Papillomavirus type 16; HPV-18, Human Papillomavirus type 18.
Finally, to combine vaccine response data from multiple time points into a single analysis, a linear mixed model was fitted to the data to account for correlation between responses of each individual at various timepoints. This analysis was done for responses that had measurements at two post-vaccination timepoints i.e. BCG-specific IFN-γ, yellow fever PRNT50, yellow fever PRNT90, S. Typhi O:LPS-specific IgG and HPV. The results adjusted for confounders are presented in Table 3. In this analysis, we investigated the interaction between infection status and trial arm to see if the association between S. mansoni and hookworm infection and vaccine responses differed by the study trial arm. There was no significant interaction between infection status and trial arm for any of the vaccine responses. S mansoni infection was inversely associated with S. Typhi O:LPS-specific IgG responses (aGMR 0.69 (0.57- 0.83)) whereas hookworm infection was inversely associated with HPV-16 IgG responses (aGMR 0.702 (0.549, 0.899)). Supplementary Figures S3, S4 show the predicted responses by S mansoni and hookworm infections.
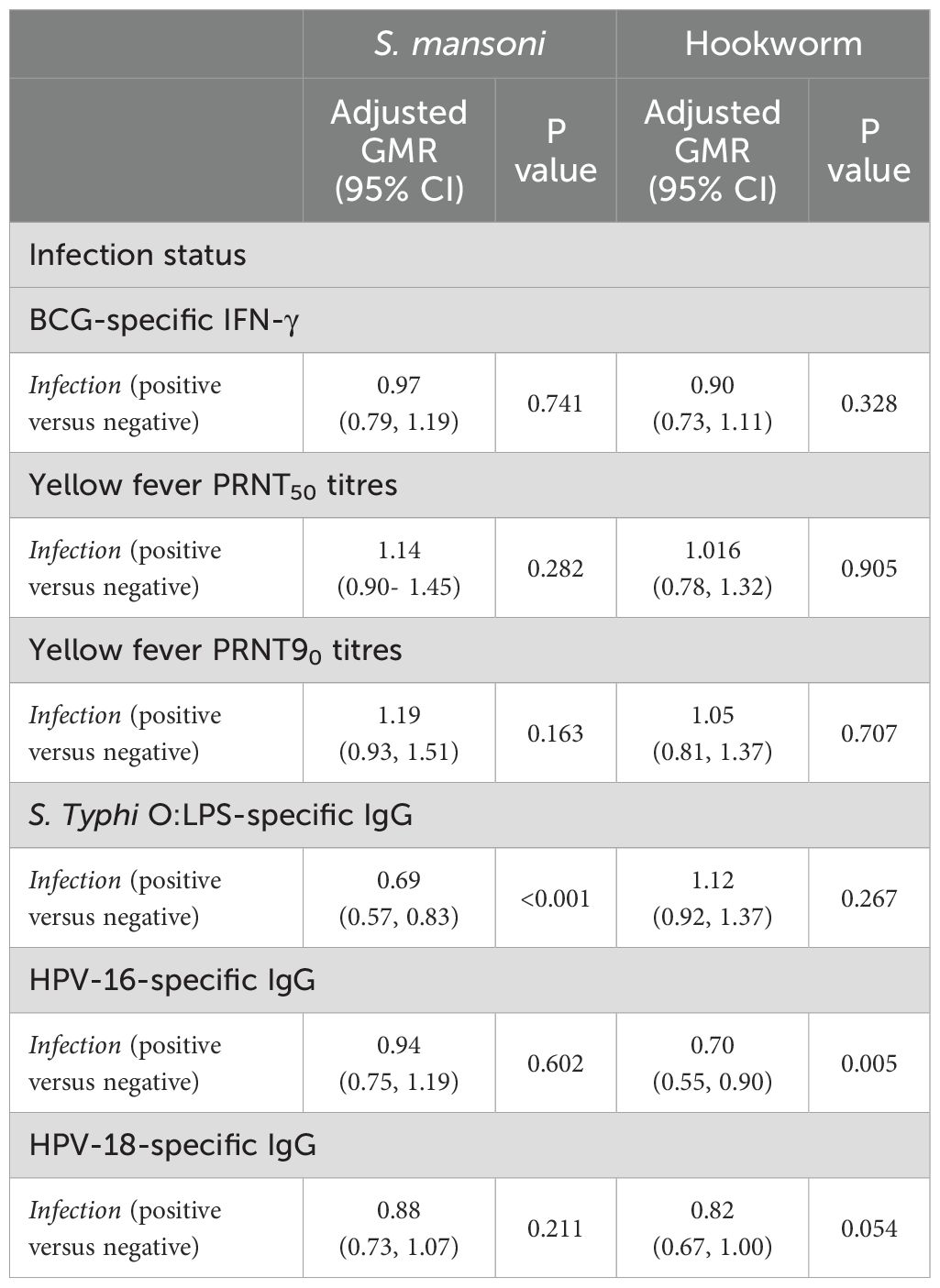
Table 3. Adjusted associations between helminth infections and vaccine responses for weeks 8 and 52 timepoints.
Using the same modelling approach, we found a significant interaction between S. mansoni infection intensity and trial arm. S. mansoni infection intensity was associated with responses to BCG and oral typhoid with high intensity being associated with lower responses to BCG among standard arm participants only (aGMR 0.65 (0.46-0.91)) and high and medium S. mansoni infection intensities being associated with lower response to oral typhoid compared to S. mansoni negatives (aGMR 0.74 (0.59-0.94)) and (aGMR 0.70 (0.55-0.88)) respectively. Detailed results of associations of S. mansoni infection intensity and vaccine responses are presented in Supplementary Table 5.
Discussion
We conducted an observational analysis to evaluate associations between baseline helminth infections and subsequent study-administered vaccine responses among Ugandan adolescents living in Koome Islands of Lake Victoria. In these fishing communities, where helminth infections, particularly S. mansoni, are highly prevalent, S. mansoni infection prior to vaccination was associated with lower S. Typhi O:LPS-specific IgG responses post vaccination. On the other hand, hookworm infection was associated with higher diphtheria toxoid-specific IgG responses and lower HPV-16 responses post vaccination. We also found that higher S. mansoni infection intensity was associated with lower BCG-specific IFN-γ and S. Typhi O:LPS-specific IgG responses. There was no significant association observed between helminth infections and responses to tetanus or yellow fever vaccines. These findings partly support the hypothesis that helminth infections may impair vaccine responses, although these effects were not consistent across all vaccines. In our study, negative effects were observed mainly for responses to BCG and oral typhoid vaccines both of which are live attenuated bacterial vaccines.
In the parent trial, we observed a significant reduction in schistosomiasis infection intensity following intensive treatment with praziquantel. Intensive treatment showed evidence of improved BCG-specific cellular response and no statistically significant improvement in the response to oral typhoid or responses to other studied vaccines (17). In the current observational analysis, we found an association between high S. mansoni infection intensity and lower BCG-specific IFN-γ responses in individuals who had not received treatment prior to vaccination. These findings are in line with a review that showed generally lower BCG-specific IFN-γ responses among helminth infected individuals (12).
We found that hookworm infection was associated with higher diphtheria-specific IgG and somewhat higher tetanus toxoid-specific IgG responses, consistent with findings from other studies that some worms can boost responses to some vaccines. Other studies that have shown this boosting effect include one for a malaria vaccine candidate, which found that hookworm and malaria coinfection resulted in higher IgG responses to the GMZ2 malaria candidate vaccine antigen and that hookworm treatment resulted in lower IgG levels (24). Adjuvant effects of helminths have also been observed for Onchocerca volvulus in mice (25) and human (26) studies. Interestingly, these effects have also been observed in studies investigating the effect of maternal helminth infection on vaccines given routinely in infancy. These boosting effects have been observed for orally administered polio and rotavirus vaccines, with a higher vaccine-specific response level observed in children born to helminth infected compared to uninfected mothers (27).
The lack of association between helminths, especially S. mansoni infection, and toxoid vaccines in our study was not unexpected, given that our recent meta-analysis of data from several studies found no association between responses to tetanus toxoid and helminth infection status prior to or at the time of vaccination (12). However, Riner and colleagues showed that among people who needed an immediate tetanus toxoid boost based on their baseline titres being less than 0.01 mIU/ml, those infected with S. mansoni had significantly lower tetanus responses than negative controls at six and eight months after the boost. In our study, we explored the association between helminths and vaccine responses in this subgroup of people for both tetanus and diphtheria but found no evidence of association.
Our research had some limitations. There was significant missing data for some exposures, such as prior treatment for worms and information on this, and other variables such as vaccinations received in infancy, were based on self-report and could be subject to measurement error. The study setting is highly endemic for S. mansoni and thus among participants who were negative at baseline, infections and reinfections may occur at short intervals, this might have inhibited us from seeing the full effect of Sm infection on vaccine responses and might not be typical of what happens in other settings. Also, as demonstrated elsewhere, the CAA test format (SCAA20) used to assess S. mansoni infection in our study was not the format with ultimate (single worm) sensitivity (28), implying that some individuals who were categorised as negative could carry a low worm burden infection. Following this observation, we investigated the association between S. mansoni intensity and vaccine responses, and the results supported what was observed for S. mansoni infection status and oral typhoid responses. Finally, given its low prevalence at baseline, Strongyloides was not included in the association analysis.
Overall, helminths have a complex effect on vaccine responses in humans. Certain species can impair the immunogenicity of some vaccinations, while others may have a neutral or positive effect (12). Our data show that being infected with S. mansoni prior to vaccination jeopardizes responses to some vaccines. The vaccines we tested generally produce robust protection, and a reduction in responses due to infection may not necessarily translate into individuals being unprotected. However, for some vaccines where benefits are more marginal, or for new vaccines, the reduction in responses among those infected or with heavy infection may be of public health concern. Although further research is needed to increase our understanding of the mechanisms behind these effects and their implications for public health, helminths can have both positive and negative effects on the effectiveness of certain vaccinations.
It is recommended that optimal vaccine response monitoring take place in helminth endemic settings, particularly for vaccines that have been shown to be negatively affected by helminths, allowing for potential boosting. Additionally, vaccine manufacturers should consider assessing the effect of helminths on candidate vaccines in early phase trials, especially for vaccines designed to be used in regions where helminths are endemic.
Further research should be conducted to investigate the mechanisms underlying the lower oral typhoid responses among those infected with S. mansoni as well as the potential boosting effects of hookworm infection on diphtheria toxoid-specific IgG responses. This will inform strategies to optimise vaccine effectiveness in communities at high risk of illness and death from infectious diseases.
Data availability statement
The de-identified individual participant data that underlie the results reported in this article are stored in a non-publicly available repository (LSHTM Data Compass), together with a data dictionary. Data are available on request via https://doi.org/10.17037/DATA.00003862. Researchers who would like to access the data may submit a request through LSHTM Data Compass, detailing the data requested, the intended use for the data, and evidence of relevant experience and other information to support the request. The request will be reviewed by the Principal Investigator in consultation with the MRC/UVRI and LSHTM data management committee, with oversight from the UVRI and LSHTM ethics committees. In line with the MRC policy on Data Sharing, there will have to be a good reason for turning down a request. Patient Information Sheets and consent forms specifically referenced making anonymised data available and this has been approved by the relevant ethics committees. Researchers given access to the data will sign data sharing agreements which will restrict the use to answering pre-specified research questions.
Ethics statement
The studies involving humans were approved by the Uganda Virus Research Institute Research Ethics Committee (references: GC/127/18/09/680, GC/127/19/05/664), the London School of Hygiene and Tropical Medicine Observational/Interventions Research Ethics Committee (reference: 16032), the Uganda National Council for Science and Technology (reference: HS2486) and the Uganda National Drug Authority (reference: CTA0093). The studies were conducted in accordance with the local legislation and institutional requirements. Written informed consent for participation in this study was provided by the participants’ legal guardians/next of kin.
Author contributions
AN: Conceptualization, Data curation, Formal analysis, Writing – original draft. LZ: Project administration, Writing – review & editing. GN: Writing – review & editing. JN: Writing – review & editing. RN: Writing – review & editing. AM: Data curation, Writing – review & editing. PC: Writing – review & editing. GD: Writing – review & editing. AE: Conceptualization, Funding acquisition, Investigation, Supervision, Writing – review & editing. EW: Conceptualization, Supervision, Writing – review & editing.
Funding
The author(s) declare financial support was received for the research, authorship, and/or publication of this article. The POPVAC programme of work was supported primarily by the Medical Research Council (MRC) of the United Kingdom (grant # MR/R02118X/1 and MC_PC_21034). This award is jointly funded by the UK Medical Research Council (MRC) and the UK Foreign Commonwealth and Development Office (FCDO) under the MRC/FCDO Concordat agreement and is also part of the EDCTP2 programme supported by the European Union. JN was also supported by the Makerere University – Uganda Virus Research Institute Centre of Excellence for Infection and Immunity Research and Training (MUII-plus), funded under the DELTAS Africa Initiative (grant # DEL-15-004). The DELTAS Africa Initiative was an independent funding scheme of the African Academy of Sciences, Alliance for Accelerating Excellence in Science in Africa and supported by the New Partnership for Africa’s Development Planning and Coordinating Agency with funding from the Wellcome Trust and the UK Government. AN, LZ, EW, and AE received additional support from the UK National Institute of Health and Care Research (NIHR; grant # NIHR134531) using UK aid from the UK Government to support global health research; GN received additional support from the EDCTP2 programme supported by the European Union (grant # TMA2019PF-2707) and from the Wellcome Trust (grant # 224263/Z/21/Z); EW also received funding through the International Statistics and Epidemiology Group (ISEG) from the UK MRC grant # MR/R010161/1. The work was conducted at the MRC/UVRI and LSHTM Uganda Research Unit; both ISEG and the MRC/UVRI and LSHTM Uganda Research Unit are jointly funded by the UK MRC part of UK Research and Innovation and the UK Foreign, Commonwealth and Development Office (FCDO) under the MRC/FCDO Concordat agreement and are also part of the EDCTP2 programme supported by the European Union.
Acknowledgments
We thank the trial participants, their parents and communities for participation, and the Koome sub-county local council and Mukono District authorities for supporting the work. We thank Troy Kemp and Ligia Pinto (Frederick National Laboratory for Cancer Research, USA) for guidance in conducting HPV IgG assays; Elizabeth Jones, Andrew Pollard, the Oxford Vaccine Centre Biobank and their study participants for kindly providing control sera for the S. Typhi O:LPS-specific IgG assays; Claudia de Dood (Leiden University Medical Center) for production of reagents and support in undertaking the CAA assays.
Conflict of interest
The authors declare that the research was conducted in the absence of any commercial or financial relationships that could be construed as a potential conflict of interest.
Publisher’s note
All claims expressed in this article are solely those of the authors and do not necessarily represent those of their affiliated organizations, or those of the publisher, the editors and the reviewers. Any product that may be evaluated in this article, or claim that may be made by its manufacturer, is not guaranteed or endorsed by the publisher.
Supplementary material
The Supplementary Material for this article can be found online at: https://www.frontiersin.org/articles/10.3389/fimmu.2024.1460183/full#supplementary-material
References
1. Barreto ML, Pilger D, Pereira SM, Genser B, Cruz AA, Cunha SS, et al. Causes of variation in BCG vaccine efficacy: Examining evidence from the BCG REVAC cluster randomized trial to explore the masking and the blocking hypotheses. Vaccine. (2014) 32:3759–64. doi: 10.1016/j.vaccine.2014.05.042
2. Fine PEM. Variation in protection by BCG: implications of and for heterologous immunity. Lancet. (1995) 346:1339–45. doi: 10.1016/S0140-6736(95)92348-9
3. World Health Organization. Soil-transmitted helminth infections (2022). Available online at: https://www.who.int/news-room/fact-sheets/detail/soil-transmitted-helminth-infections (accessed February 20, 2024).
4. Colley DG, Bustinduy AL, Secor WE, King CH. Human schistosomiasis. Lancet. (2014). 383:2253–64. doi: 10.1016/S0140-6736(13)61949-2
5. World Health Organization. Schistosomiasis (2022). Available online at: https://www.who.int/news-room/fact-sheets/detail/schistosomiasis (accessed February 20, 2024).
6. Exum NG, Kibira SPS, Ssenyonga R, Nobili J, Shannon AK, Ssempebwa JC, et al. The prevalence of schistosomiasis in Uganda: A nationally representative population estimate to inform control programs and water and sanitation interventions. PloS Negl Trop Dis. (2019) 13. doi: 10.1371/journal.pntd.0007617
7. Webb EL, Nampijja M, Kaweesa J, Kizindo R, Namutebi M, Nakazibwe E, et al. Helminths are positively associated with atopy and wheeze in Ugandan fishing communities: results from a cross-sectional survey. Allergy: Eur J Allergy Clin Immunol. (2016) 71:1156–69. doi: 10.1111/all.12867
8. McSorley HJ, Maizels RM. Helminth infections and host immune regulation. Clin Microbiol Rev. (2012) 25:585–608. doi: 10.1128/CMR.05040-11
9. Potian JA, Rafi W, Bhatt K, McBride A, Gause WC, Salgame P. Preexisting helminth infection induces inhibition of innate pulmonary anti-tuberculosis defense by engaging the IL-4 receptor pathway. J Exp Med. (2011) 208:1863–74. doi: 10.1084/jem.20091473
10. Hussaarts L, Yazdanbakhsh M, Guigas B. Priming dendritic cells for Th2 polarization: Lessons learned from helminths and implications for metabolic disorders. Front Immunol. (2014) 5. doi: 10.3389/fimmu.2014.00499
11. Stelekati E, Wherry EJ. Chronic bystander infections and immunity to unrelated antigens. Cell Host Microbe. (2012) 12:458–69. doi: 10.1016/j.chom.2012.10.001
12. Natukunda A, Zirimenya L, Nassuuna J, Nkurunungi G, Cose S, Elliott AM, et al. The effect of helminth infection on vaccine responses in humans and animal models: A systematic review and meta-analysis. Parasite Immunol. (2022) 44. doi: 10.1111/pim.12939
13. Sanya RE, Nkurunungi G, Biraro IA, Mpairwe H, Elliott AM. A life without worms. Trans R Soc Trop Med Hyg. (2017) 111:3–11. doi: 10.1093/trstmh/trx010
14. Elias D, Britton S, Aseffa A, Engers H, Akuffo H. Poor immunogenicity of BCG in helminth infected population is associated with increased in vitro TGF-beta production. Vaccine. (2008) 26:3897–902. http://www.ncbi.nlm.nih.gov/pubmed/18554755.
15. Vaca M, Moncayo AL, Cosgrove CA, Chico ME, Castello-Branco LR, Lewis DJ, et al. A single dose of oral BCG Moreau fails to boost systemic IFN-γ responses to tuberculin in children in the rural tropics: Evidence for a barrier to mucosal immunization. J Trop Med. (2012) 2012:132583. doi: 10.1155/2012/132583
16. Nkurunungi G, Zirimenya L, Nassuuna J, Natukunda A, Kabuubi PN, Niwagaba E, et al. Effect of intensive treatment for schistosomiasis on immune responses to vaccines among rural Ugandan island adolescents: Randomised controlled trial protocol A for the POPulation differences in VACcine responses’ (POPVAC) programme. BMJ Open. (2021) 11. doi: 10.1136/bmjopen-2020-040426
17. Nkurunungi G, Nassuuna J, Natukunda A, Zirimenya L, Zziwa C, Ninsiima C, et al. The effect of intensive praziquantel treatment on vaccine-specific responses among schoolchildren in Ugandan schistosomiasis-endemic islands: results of the POPVAC A randomised, controlled trial. Available online at: https://ssrn.com/abstract=4667594 (accessed June 28, 2024).
18. Corstjens PLAM, De Dood CJ, Kornelis D, Tjon Kon Fat EM, Wilson RA, Kariuki TM, et al. Tools for diagnosis, monitoring and screening of Schistosoma infections utilizing lateral-flow based assays and upconverting phosphor labels. Parasitology. (2014) 141:1841–55. doi: 10.1017/S0031182014000626
19. Corstjens PLAM, Li S, Zuiderwijk M, Kardos K, Abrams WR, Niedbala RS, et al. Infrared up-converting phosphors for bioassays. IEE Proc Nanobiotechnol. (2005) p:64–72. doi: 10.1049/ip-nbt:20045014
20. Corstjens PLAM, Van Lieshout L, Zuiderwijk M, Kornelis D, Tanke HJ, Deelder AM, et al. Up-converting phosphor technology-based lateral flow assay for detection of Schistosoma circulating anodic antigen in serum. J Clin Microbiol. (2008) 46:171–6. doi: 10.1128/JCM.00877-07
21. Corstjens PLAM, Hoekstra PT, de Dood CJ, van Dam GJ. Utilizing the ultrasensitive Schistosoma up-converting phosphor lateral flow circulating anodic antigen (UCP-LF CAA) assay for sample pooling-strategies. Infect Dis Poverty. (2017) 6. doi: 10.1186/s40249-017-0368-1
22. Verweij JJ, Brienen EAT, Ziem J, Yelifari L, Polderman AM, Van Lieshout L. Simultaneous Detection and Quantification of Ancylostoma duodenale, Necator americanus, and Oesophagostomum bifurcum in Fecal Samples Using Multiplex Real-Time PCR. Am J Trop Med Hyg. (2007), 685–90. doi: 10.4269/ajtmh.2007.77.685
23. Verweij JJ, Canales M, Polman K, Ziem J, Brienen EAT, Polderman AM, et al. Molecular diagnosis of Strongyloides stercoralis in faecal samples using real-time PCR. Trans R Soc Trop Med Hyg. (2009) 103:342–6. doi: 10.1016/j.trstmh.2008.12.001
24. Amoani B, Gyan B, Sakyi SA, Abu EK, Nuvor SV, Barnes P, et al. Effect of hookworm infection and anthelmintic treatment on naturally acquired antibody responses against the GMZ2 malaria vaccine candidate and constituent antigens. BMC Infect Dis. (2021) 21:332. doi: 10.1186/s12879-021-06027-5
25. MacDonald AJ, Cao L, He Y, Zhao Q, Jiang S, Lustigman S. rOv-ASP-1, a recombinant secreted protein of the helminth Onchocerca volvulus, is a potent adjuvant for inducing antibodies to ovalbumin, HIV-1 polypeptide and SARS-CoV peptide antigens. Vaccine. (2005) 23:3446–52. doi: 10.1016/j.vaccine.2005.01.098
26. MacDonald AJ, Libri NA, Lustigman S, Barker SJ, Whelan MA, Semper AE, et al. A novel, helminth-derived immunostimulant enhances human recall responses to hepatitis C virus and tetanus toxoid and is dependent on CD56+ cells for its action. Clin Exp Immunol. (2008) 152:265–73. doi: 10.1111/j.1365-2249.2008.03623.x
27. Clark CE, Fay MP, Chico ME, Sandoval CA, Vaca MG, Boyd A, et al. Maternal helminth infection is associated with higher infant immunoglobulin A titers to antigen in orally administered vaccines. J Infect Dis. (2016) 213:1996–2004. https://academic.oup.com/jid/article-lookup/doi/10.1093/infdis/jiw066.
Keywords: helminths, Schistosoma mansoni, hookworm, vaccines, immune responses, antibody responses
Citation: Natukunda A, Zirimenya L, Nkurunungi G, Nassuuna J, Nkangi R, Mutebe A, Corstjens PLAM, van Dam GJ, Elliott AM and Webb EL (2024) Pre-vaccination Schistosoma mansoni and hookworm infections are associated with altered vaccine immune responses: a longitudinal analysis among adolescents living in helminth-endemic islands of Lake Victoria, Uganda. Front. Immunol. 15:1460183. doi: 10.3389/fimmu.2024.1460183
Received: 05 July 2024; Accepted: 08 August 2024;
Published: 29 August 2024.
Edited by:
Thiago Almeida Pereira, Stanford University, United StatesReviewed by:
Richard E. Goodman, University of Nebraska-Lincoln, United StatesWannaporn Ittiprasert, United States Army Medical Research Institute of Infectious Diseases (USAMRIID), United States
Copyright © 2024 Natukunda, Zirimenya, Nkurunungi, Nassuuna, Nkangi, Mutebe, Corstjens, van Dam, Elliott and Webb. This is an open-access article distributed under the terms of the Creative Commons Attribution License (CC BY). The use, distribution or reproduction in other forums is permitted, provided the original author(s) and the copyright owner(s) are credited and that the original publication in this journal is cited, in accordance with accepted academic practice. No use, distribution or reproduction is permitted which does not comply with these terms.
*Correspondence: Agnes Natukunda, Agnes.Natukunda@mrcuganda.org; natukundagnes2@gmail.com
†These authors have contributed equally to this work