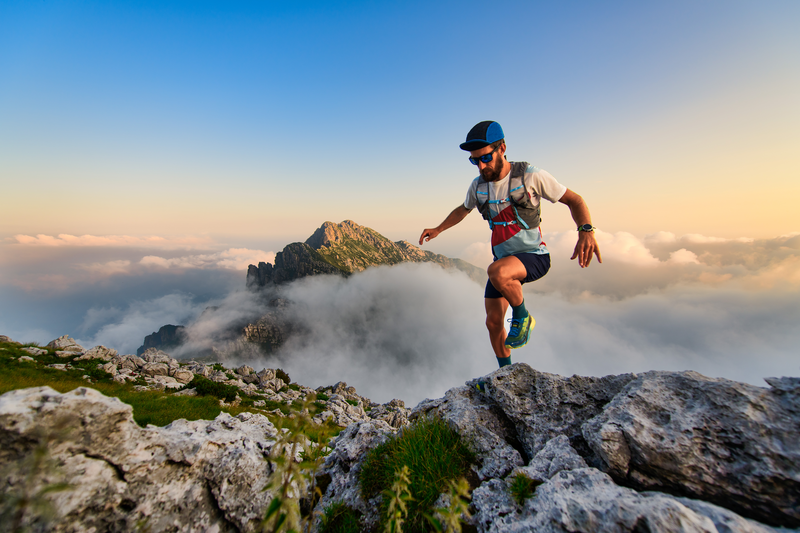
94% of researchers rate our articles as excellent or good
Learn more about the work of our research integrity team to safeguard the quality of each article we publish.
Find out more
REVIEW article
Front. Immunol. , 06 December 2024
Sec. Multiple Sclerosis and Neuroimmunology
Volume 15 - 2024 | https://doi.org/10.3389/fimmu.2024.1460072
This article is part of the Research Topic Microglia and tissue macrophages in pain View all 3 articles
Neuropathic pain (NP) is an ineffectively treated, debilitating chronic pain disorder that is associated with maladaptive changes in the central nervous system, particularly in the spinal cord. Murine models of NP looking at the mechanisms underlying these changes suggest an important role of microglia, the resident immune cells of the central nervous system, in various stages of disease progression. However, given the number of different NP models and the resource limitations that come with tracking longitudinal changes in NP animals, many studies fail to truly recapitulate the patterns that exist between pain conditions and temporal microglial changes. This review integrates how NP studies are being carried out in murine models and how microglia changes over time can affect pain behavior in order to inform better study design and highlight knowledge gaps in the field. 258 peer-reviewed, primary source articles looking at spinal microglia in murine models of NP were selected using Covidence. Trends in the type of mice, statistical tests, pain models, interventions, microglial markers and temporal pain behavior and microglia changes were recorded and analyzed. Studies were primarily conducted in inbred, young adult, male mice having peripheral nerve injury which highlights the lack of generalizability in the data currently being collected. Changes in microglia and pain behavior, which were both increased, were tested most commonly up to 2 weeks after pain initiation despite aberrant microglia activity also being recorded at later time points in NP conditions. Studies using treatments that decrease microglia show decreased pain behavior primarily at the 1- and 2-week time point with many studies not recording pain behavior despite the involvement of spinal microglia dysfunction in their development. These results show the need for not only studying spinal microglia dynamics in a variety of NP conditions at longer time points but also for better clinically relevant study design considerations.
Pain, while evolved to serve a protective purpose, can become persistent and lead to maladaptive changes in the central nervous system (CNS). Neuropathic pain (NP) is one such debilitating, chronic pain condition resulting from damage to the somatosensory nervous system, which affects 7%–10% of the population (1). This chronic pain is characterized by symptoms including increased sensitivity to stimuli (hyperalgesia) and a pain response to non-painful stimuli (allodynia) (1). The treatments for NP are largely ineffective and are associated with harmful side effects (1), due to which there is a growing interest in better characterizing this disease and discovering new therapeutic targets.
Microglia, the resident CNS immune cells, are one such target that are garnering popularity and are being studied in murine models of NP due to their involvement in pain progression (2). While inhibition of microglia can suppress mechanical allodynia in NP models (3), globally targeting microglia can be detrimental, particularly due to their role in maintaining CNS homeostasis. This has led to an increase in the study of microglia-mediated pathways in pain conditions. Researchers have found that several dynamic changes can occur in these cells during the different stages of NP development (2). These include the microglia being activated after nerve injury and promoting NP through mechanisms such as the activation of brain-derived neurotrophic factor (BDNF)/TrkB signaling, leading to a disrupted neuron chloride homeostasis and the subsequent decrease in inhibitory transmission (4) or synaptic pruning (5).
This scoping review characterizes the changes in spinal microglia over time in murine models of chronic pain by consolidating the findings on how these microglial changes over time after NP are induced. This study also evaluates the importance of the time points that different studies used to measure chronic pain and the relation of potential pain treatments to spinal microglia and pain development. Overall, this review examines the temporal changes in microglial dynamics and the relationships that exist between pain conditions and microglial activation.
A literature search to investigate the changes in the spinal microglia over time in murine models of NP was conducted. A comprehensive review of four minimally overlapping databases (i.e., EMBASE, Web of Science, SCOPUS, and PsycInfo) was completed to ensure that no relevant sources were excluded by this investigation.
Sources were required to meet the following inclusion criteria: 1) must have been conducted using a mouse model of pain; 2) must have included investigation of microglia in the spinal cord; 3) must be published in the English language in a peer-reviewed journal; and 4) must be a primary source article.
Studies were excluded if they were gray literature sources (i.e., conference proceedings, poster abstracts, etc.). The articles retrieved in the search were also excluded if they were review articles or if the full text was not available.
After the initial search of these four databases, 4,146 non-duplicate sources were identified for the screening of titles and abstracts. Following the title and abstract screening of these sources according to the above inclusion and exclusion criteria, 339 sources were identified for a review of the full texts (6–263). Of these sources, only 258 studies were deemed eligible for inclusion in the review based on a dual-reviewer screening process. The reviewers were the first two authors (Figure 1). Conflicts at each stage were discussed between reviewers before consensus was reached. Wrong immune cell activation was chosen as a reason for exclusion if the study looked at macrophages, indirect microglia activation markers such as inflammatory cytokines, or only showed colocalization with microglia and not microglial change. Multiple sclerosis (MS) and amyotrophic lateral sclerosis (ALS), disabling chronic CNS diseases affecting spinal cord neurons (264), were included in the study, while studies looking at temporal microglial changes only in rats or cell lines were excluded.
Figure 1. PRISMA flowchart of the study selection, review criteria, and reasons for study exclusion at various phases of the scoping review.
Data were extracted from the full-text articles using Covidence’s non-intervention data extracting tool (265). The study characteristics including the publication year, publishing journal, year of publication, sex, sample size, strain, age (distributed into five categories) (266), and the statistical test used were recorded. The type of pain model (267), the treatment, the spinal cord region, the spinal cord section, and the microglial markers were documented. The change in microglia and the pain-related behavior before and after treatment, as well as the time point and the region of the spinal cord where the change occurred, were separated according to the sex of the mice studied. Inter-rater reliability for the full text and title and abstract screening was calculated by Covidence using Cohen’s kappa statistic. The data were exported into Microsoft Excel, where each time point was treated as a separate experiment. The male and female data were separated if both sexes of mice were used in the study and showed different results. The sorting and count functions were then used to separate the data according to pain condition, sex, microglial changes pre- and post-treatment, and mechanical allodynia change post-treatment. All data were presented as counts and percentages. Further information can be found in the Supplementary Excel File.
Moderate inter-rater reliability (268) was found for the full-text and title and abstract screening, with Cohen’s kappa values of 0.53804 and 0.66311, respectively.
China was the highest producer of articles (33%), while the second highest, Japan, produced less than half of the percentage of studies conducted in China, which was then followed by the United States of America (Figure 2B; Supplementary Table S1).
Figure 2. Characteristics of the publications that met the inclusion criteria. (A) Year of publication of the included studies. (B) Country where the study was conducted.
Articles were found in various journals, with Pain, The Journal of Neuroscience, and Molecular Pain being the top 3 publishing sources, but cumulatively only producing 18% of all the included sources.
Despite the literature search within each database not including any limitations on the year of publication, 65.89% of the sources that met the final inclusion criteria were published between 2017 and 2023 (Figure 2A).
Only 6% of the studies mentioned testing for normality in their statistical analysis section, while approximately 5% of the studies used non-parametric tests without mentioning a normal distribution test. The average sample size was six mice in each group. Of the studies, 6% had undefined statistics.
More than twice the number of studies were conducted only in male mice (48%) as compared with female mice (21%), with only 16% studying both sexes. Of the studies, 14% did not specify the sex of the mice used (Figure 3B).
Figure 3. Characteristics of the mice in the studies that met the inclusion criteria. (A) Mouse strain. (B) Sex. (C) Age of mice. (D) Pain models applied.
Approximately 75% of the studies were carried out using young adult mice aged 1–4 months, while 20% of the studies did not mention the age of the mice used (Figure 3C). Of the studied mice, 78% were inbred strains, with 70% of the studies including mice with a C57BL/6 background (Figure 3A).
Peripheral nerve injury (44%), spinal cord injury (SCI; 22%), and MS (9%) were the top 3 pain conditions studied (Figure 3D). For a full list of the various models of pain used, see Supplementary Table S2.
Ionized calcium-binding adapter molecule 1 (Iba-1) was the most prominent microglial marker studied (63%), followed by cluster of differentiation receptor 11b (CD11b; 17%) and fractalkine receptor (CX3CR1; 12%) (Table 1). These microglial markers were primarily assessed using immunofluorescence assays.
Table 1. Distribution of studies based on the microglial markers used (total of 339 as some studies used more than one microglial marker).
An increase in microglia post-pain initiation was observed in 73% of the experiments, with 21% not specifying any change to microglial presence (Figure 4A). The most used time points were days 7, 14, 3, 21, and 28 post-lesion (Supplementary Table S4). At every time point, the studies showed an increase in microglia (Figure 4B) and mechanical allodynia (Figure 4C), with the highest number of studies being peripheral nerve injury experiments conducted within 2 weeks of pain development.
Figure 4. Changes to microglial presence in the spinal cord over time in relation to pain model induction. (A) Global (all pain conditions grouped) changes to microglial presence following induction of the pain condition. (B) Distribution of studies depending on microglia increase at particular time points. (C) Distribution of studies depending on mechanical allodynia increase at time points.
Post-treatment, spinal microglia decreased at every time point (Figure 5A). Up to 2 weeks after injury, mechanical allodynia was primarily decreased post-spinal microglial depletion (Figure 5B). After 2 weeks, a similar number of experiments showed decreased mechanical allodynia and unspecified changes to pain behavior despite spinal microglial decrease post-treatment. For a full figure illustrating the various treatments summarized in these figures, see Supplementary Figure S1.
Figure 5. Changes to microglial presence and pain behavior in the spinal cord over time post-treatment (all treatments grouped). (A) Distribution of experiments depending on microglial change after treatment. (B) Distribution of studies depending on microglial decrease at particular time points.
The role of spinal microglia in murine NP conditions has recently been the focus of widespread interest from the pain research community given the increasing trend of using mouse models, instead of rats, in pain research (269). This is likely motivated by the advent of using transgenic mouse lines (270) to study the various aspects of chronic pain. An overwhelming majority of the sources that met the eligibility criteria for this review were published within the last 5 years, further reinforcing that this is an area of heightened research interest in the field of preclinical chronic pain research.
Analysis of the characteristics of experimental mice showed that over three quarters of the studies were conducted on inbred mice despite evidence showing that the use of outbred mice is more efficient, generalizable, and clinically relevant (271). Most of the included studies were conducted using only a single mouse strain despite evidence suggesting strain-specific immune responses in NP murine models (92). Young adult mice within 4 months of age, corresponding to approximately 15–20 human years (272), were the most used in these studies, and 2 weeks post-chronic pain initiation was the most studied time point. This can lead to an incomplete understanding of immune cell-mediated pain mechanisms due to mice not being studied for longer time points and at more mature ages (270), especially when microglia have been shown to cause time-dependent changes in NP conditions (5).
Moreover, more than twice the number of studies used only male mice as compared with females, despite chronic pain being more prevalent in female individuals (273). The studies that did use only female mice were skewed toward MS pain models, where female mice better recapitulated the disease phenotype (274). Proliferation of microglia occurred in both sexes following nerve injury (2, 275, 276). Moreover, in this study, we found that, after chronic pain initiation, there is a predominant consensus of an increase in spinal microglia. The role of spinal microglia in chronic pain progression is still contentious, especially with the emerging understanding of the heterogeneity in the microglial phenotypes and their involvement in different pain conditions. Some studies showed that microglia-mediated pain hypersensitivity post-nerve injury is observed primarily in male rodents (275), with other studies finding that microglia are involved in the progression of chronic pain in both sexes (200). This further highlighted the need to include both sexes of mice in chronic pain studies and to properly report the strain, age, and sex of the animals used in the experiments.
The top 3 microglial markers—intracellular protein Iba-1 and the microglial membrane proteins CD11b and CX3CR1—are activated microglial markers that mediate phagocytosis and pathogen recognition, respectively. However, these are general microglial markers that do not differentiate between resident CNS microglia and peripheral macrophages. The use of more specific markers for microglia, such as the transmembrane protein 119 (TMEM119) and purinergic receptor P2Y12R, or co-staining with specific resident macrophage markers, including the high expression of cluster of differentiation receptor (CD44 and CD206), will be required in future studies to properly parse out which immune cells are changing in NP (277). Moreover, microglia can undergo drastic morphological and functional changes when activated by different signals (278). These changes include the M1-like phenotype characterized by the release of inflammatory cytokines and the M2-like phenotype associated with anti-inflammation mediators (278). In addition to NP, many CNS disorders, such as stroke, Alzheimer’s disease, and MS, have shown how specific microglia can be associated with particular stages of disease progression (278). Moreover, transcriptomics data have shown phenotypic profiles of microglia that go beyond the simple M1- and M2-like classification and how some microglia can express multiple markers simultaneously (278). The metrics often applied in the existing literature, including microglial increase/decrease, are crude measures of the nuanced roles that microglia play in the progression of NP. Future studies thus require in-depth analysis of the changes to microglial morphology and function with respect to time and disease progression. Follow-up experiments using specific microglial markers, including the enzymes inducible nitric oxide synthase (iNOS) and arginase-1 (Arg-1), to identify pro- and anti-inflammatory states, respectively, and the accompanying morphological changes are paramount to properly characterize the role of microglia in NP (277, 278).
A majority of the NP studies included in this review were conducted using peripheral nerve injury models, specifically those involving the sciatic nerve. This is likely a result of this model being easily reproducible and having highly localized mechanical allodynia (270). However, there are a plethora of other clinically observed NP conditions (279) caused by drugs, other diseases such as cancer and diabetes, and CNS injury, in which microglial changes and their influence on pain behavior are understudied. In this review, SCI studies frequently examined locomotor dysfunction, while MS models primarily focused on the physical indicators of disease progression, with minimal inclusion of pain behavioral assays, which could provide useful insights into neurodegenerative conditions with a pain component. In the studies in which pain behavior was explicitly quantified, many included measures of evoked mechanical allodynia (primarily via the Von Frey test), choosing to omit clinically relevant measures of other forms of pain, including heat hypersensitivity, cold allodynia, and spontaneous measures of pain (280).
Increased microgliosis and mechanical allodynia was observed in the majority of studies within 1 month of NP initiation, which is consistent with the emerging role of spinal microglia promoting increased pain behavior through the activation, proliferation, and the release of inflammatory immunomodulators post-pain initiation (2). Studies using treatments that decrease spinal microglia were mostly associated with decreased mechanical allodynia up to 2 weeks. After the 2-week time point, the number of studies examining spinal microglial dynamics and studies examining pain behavior declined. This is important to consider because, in conditions such as SCI and peripheral nerve injury, 6 weeks post-injury is considered chronic (281) and microgliosis can occur 20 days after the injury (5), respectively. Moreover, further work must be done to study the long-term potential of microglia-modifying treatments in the reduction of pain behavior.
While microglial involvement in chronic pain has garnered much preclinical focus mechanistically and therapeutically, the manipulation of microglia for chronic pain treatment is not exclusive to animal studies. Human clinical trials using drugs that prevent microglia activation and that reduce inflammation are underway to treat chronic pain diseases such as fibromyalgia (282–286). Animal studies, thus, have the potential to provide a basis for discovering therapeutic targets that can be translated to human research, and the clinical translatability increases with well-designed preclinical studies. In addition, research further elucidating the complexity of microglia-mediated cell signaling during chronic pain continues to provide new methods of targeting microglia, and this can be seen not only through the latest papers being published in the field (287–292) but also through the variety of microglial treatments used in these studies, which included genetic modifications using gene silencing drugs, direct pharmacological microglial ablation, indirect anti-inflammatory drugs, and physical interventions (Supplementary Figure S1).
With regard to the evaluation of statistical testing, a very low percentage of studies tested for normality. Only one study explicitly stated that normality was assumed (198). One study went as far as to use a non-parametric test, but, in the same sentence, stated that a normal distribution was assumed (72). This highlighted a lack of understanding of statistical tests and the assumptions associated with them, particularly when dealing with low sample sizes (293) and variable number of animals in each group (294). This can have long-term consequences on the reproducibility and the generalizability of studies, especially when some studies did not even mention which statistical tests were conducted.
The limitations of this review include generalizing the analyses to all chronic pain conditions, particularly when looking at treatment effects. This was due to the low numbers of studies looking at each subclass of chronic pain models (Supplementary Table S3) at various time points, further highlighting the opportunity for future research to look at microglial temporal dynamics in specific subtypes of chronic pain models. Moreover, treatments were generalized based on the microglial changes they caused in the spinal cord and not on the drug class due to the widespread heterogeneity in the treatment types (Supplementary Figure S1). For the same reasons, the time point of administration and the dose information were not integrated in this review, but are available in the Supplementary Excel Master Sheet. While moderate inter-rater reliability scores were obtained, all conflicts were resolved by both reviewers, and the exploratory nature of the scoping review made it such that conditions like MS were included at the conflict resolution stage along with indirect studies of microglial colocalization being excluded from the analysis. Simplifications for compiling the variable data were made, including generalizing mechanical allodynia to pain behavior. In addition, inflammatory microglial increases were included in microglial increase, and one article showing non-inflammatory microglial increase was deemed as microglial decrease due to the lack of distinction of the microglial phenotypes in a majority of papers, as well as the association of inflammatory microglia with disease progression and non-inflammatory microglia with disease resolution (278). Lastly, the literature search for this review was conducted in December 2023, with the analyses being limited to articles published within that time frame.
Temporal changes of spinal microglia are thus becoming increasingly recognized for their key roles in the genesis and maintenance of chronic pain conditions. The importance of microglia is highlighted by their increase at various time points after chronic pain initiation, with most studies in this review showing corresponding mechanical allodynia increases. The decrease in microglia after treatment is related to the decreased mechanical allodynia in some studies; however, many chronic pain studies using SCI and MS pain models did not explicitly study pain behavior, leaving a major gap in pain-relevant studies that could enrich the understanding of the role of microglial dynamics in other chronic pain conditions. This gap in the research, along with the lack of long-term longitudinal studies in specific pain models, highlighted the need for better study design considerations. This includes, but is not limited to, the sex, age, and strain of mice included, the specific microglial markers used, the statistical tests applied, the pain models constructed, the time points assessed, and the pain tests conducted (Figure 6).
Figure 6. Narrowing down of the factors studied in the majority of research relevant to clinical neuropathic pain.
SD: Conceptualization, Data curation, Formal analysis, Investigation, Methodology, Writing – original draft, Writing – review & editing. HD: Conceptualization, Investigation, Methodology, Visualization, Writing – original draft, Writing – review & editing. AR-D-S: Funding acquisition, Project administration, Supervision, Writing – original draft, Writing – review & editing.
The author(s) declare that no financial support was received for the research, authorship, and/or publication of this article.
Authors would like to thank McGill Librarian Andrea Miller-Nesbitt for guidance and Dr. Ji Zhang for helpful discussions. Hannah Derue recognizes the support of an Edwards PhD Fellowship in Chronic Pain Research from the Louise and Alan Edwards Foundation. All figures were made using BioRender.
The authors declare that the research was conducted in the absence of any commercial or financial relationships that could be construed as a potential conflict of interest.
All claims expressed in this article are solely those of the authors and do not necessarily represent those of their affiliated organizations, or those of the publisher, the editors and the reviewers. Any product that may be evaluated in this article, or claim that may be made by its manufacturer, is not guaranteed or endorsed by the publisher.
The Supplementary Material for this article can be found online at: https://www.frontiersin.org/articles/10.3389/fimmu.2024.1460072/full#supplementary-material
1. Cavalli E, Mammana S, Nicoletti F, Bramanti P, Mazzon E. The neuropathic pain: An overview of the current treatment and future therapeutic approaches. Int J Immunopathology Pharmacol. (2019) 33:2058738419838383. doi: 10.1177/2058738419838383
2. Karavis MY, Siafaka I, Vadalouca A, Georgoudis G. Role of microglia in neuropathic pain. Cureus. (2023) 15:e43555. doi: 10.7759/cureus.43555
3. Sorge RE, Mapplebeck JC, Rosen S, Beggs S, Taves S, Alexander JK, et al. Different immune cells mediate mechanical pain hypersensitivity in male and female mice. Nat Neurosci. (2015) 18:1081–3. doi: 10.1038/nn.4053
4. Coull JA, Beggs S, Boudreau D, Boivin D, Tsuda M, Inoue K, et al. BDNF from microglia causes the shift in neuronal anion gradient underlying neuropathic pain. Nature. (2005) 438:1017–21. doi: 10.1038/nature04223
5. Yousefpour N, Locke S, Deamond H, Wang C, Marques L, St-Louis M, et al. Time-dependent and selective microglia-mediated removal of spinal synapses in neuropathic pain. Cell Rep. (2023) 42:112010. doi: 10.1016/j.celrep.2023.112010
6. Agalave NM, Rudjito R, Farinotti AB, Khoonsari PE, Sandor K, Nomura Y, et al. Sex-dependent role of microglia in disulfide high mobility group box 1 protein-mediated mechanical hypersensitivity. Pain. (2021) 162:446–58. doi: 10.1097/j.pain.0000000000002033
7. Akagi T, Matsumura Y, Yasui M, Minami E, Inoue H, Masuda T, et al. Interferon regulatory factor 8 expressed in microglia contributes to tactile allodynia induced by repeated cold stress in rodents. J Pharmacol Sci. (2014) 126:172–6. doi: 10.1254/jphs.14143SC
8. Al-HadlaQ MW, Masocha W. Sex differences in the expression of neuroimmune molecules in the spinal cord of a mouse model of antiretroviral-induced neuropathic pain. Biomedicines. (2023) 11. doi: 10.3390/biomedicines11030875
9. Alhadlaq MW, Masocha W. Microglia and p38 MAPK inhibitors suppress development of mechanical allodynia in both sexes in a mouse model of antiretroviral-induced neuropathic pain. Int J Mol Sci. (2023) 24. doi: 10.3390/ijms241612805
10. Almeida C, Demaman A, Kusuda R, Cadetti F, Ravanelli MI, Queiroz AL, et al. Exercise therapy normalizes BDNF upregulation and glial hyperactivity in a mouse model of neuropathic pain. Pain. (2015) 156:504–13. doi: 10.1097/01.j.pain.0000460339.23976.12
11. Amo-Aparicio J, Garcia-Garcia J, Francos-Quijorna I, Urpi A, Esteve-Codina A, Gut M, et al. Interleukin-4 and interleukin-13 induce different metabolic profiles in microglia and macrophages that relate with divergent outcomes after spinal cord injury. Theranostics. (2021) 11:9805–20. doi: 10.7150/thno.65203
12. Ando S, Osanai D, Takahashi K, Nakamura S, Shimazawa M, Hara H. Survival motor neuron protein regulates oxidative stress and inflammatory response in microglia of the spinal cord in spinal muscular atrophy. J Pharmacol Sci. (2020) 144:204–11. doi: 10.1016/j.jphs.2020.09.001
13. Apolloni S, Amadio S, Parisi C, Matteucci A, Potenza RL, Armida M, et al. Spinal cord pathology is ameliorated by P2X7 antagonism in a SOD1-mutant mouse model of amyotrophic lateral sclerosis. DMM Dis Models Mech. (2014) 7:1101–9. doi: 10.1242/dmm.017038
14. Bai J, Geng B, Wang XW, Wang SH, Yi Q, Tang YC, et al. Exercise facilitates the M1-to-M2 polarization of microglia by enhancing autophagy via the BDNF/AKT/mTOR pathway in neuropathic pain. Pain Physician. (2022) 25:E1137–E51.
15. Bao JP, Yang S. ScRNA analysis and ferroptosis-related ceRNA regulatory network investigation in microglia cells at different time points after spinal cord injury. J Orthopaedic Surg Res. (2023) 18:14. doi: 10.1186/s13018-023-04195-5
16. Basso L, Lapointe TK, Iftinca M, Marsters C, Hollenberg MD, Kurrasch DM, et al. Granulocyte-colony-stimulating factor (G-CSF) signaling in spinal microglia drives visceral sensitization following colitis. Proc Natl Acad Sci United States America. (2017) 114:11235–40. doi: 10.1073/pnas.1706053114
17. Bellver-Landete V, Bretheau F, Mailhot B, Vallières N, Lessard M, Janelle ME, et al. Microglia are an essential component of the neuroprotective scar that forms after spinal cord injury. Nat Commun. (2019) 10:18. doi: 10.1038/s41467-019-08446-0
18. Benedek G, Zhang J, Bodhankar S, Nguyen H, Kent G, Jordan K, et al. Estrogen induces multiple regulatory B cell subtypes and promotes M2 microglia and neuroprotection during experimental autoimmune encephalomyelitis. J Neuroimmunology. (2016) 293:45–53. doi: 10.1016/j.jneuroim.2016.02.009
19. Biber K, Tsuda M, Tozaki-Saitoh H, Tsukamoto K, Toyomitsu E, Masuda T, et al. Neuronal CCL21 up-regulates microglia P2X4 expression and initiates neuropathic pain development. EMBO J. (2011) 30:1864–73. doi: 10.1038/emboj.2011.89
20. Bobinski F, Teixeira JM, Sluka KA, Santos ARS. Interleukin-4 mediates the analgesia produced by low-intensity exercise in mice with neuropathic pain. Pain. (2018) 159:437–50. doi: 10.1097/j.pain.0000000000001109
21. Bogacka J, Ciapała K, Pawlik K, Kwiatkowski K, Dobrogowski J, Przeklasa-Muszynska A, et al. CCR4 antagonist (C021) administration diminishes hypersensitivity and enhances the analgesic potency of morphine and buprenorphine in a mouse model of neuropathic pain. Front Immunol. (2020) 11. doi: 10.3389/fimmu.2020.01241
22. Borgonetti V, Galeotti N. Intranasal delivery of an antisense oligonucleotide to the RNA-binding protein HuR relieves nerve injury-induced neuropathic pain. Pain. (2021) 162:1500–10. doi: 10.1097/j.pain.0000000000002154
23. Borgonetti V, Galeotti N. Microglia senescence is related to neuropathic pain-associated comorbidities in the spared nerve injury model. Pain. (2023) 164:1106–17. doi: 10.1097/j.pain.0000000000002807
24. Borgonetti V, Galeotti N. Posttranscriptional regulation of gene expression participates in the myelin restoration in mouse models of multiple sclerosis: antisense modulation of HuR and HuD ELAV RNA binding protein. Mol Neurobiology. (2023) 60:2661–77. doi: 10.1007/s12035-023-03236-8
25. Borgonetti V, Governa P, Manetti F, Galeotti N. Zingiberene, a non-zinc-binding class I HDAC inhibitor: A novel strategy for the management of neuropathic pain. Phytomedicine. (2023) 111. doi: 10.1016/j.phymed.2023.154670
26. Borgonetti V, Meacci E, Pierucci F, Romanelli MN, Galeotti N. Dual HDAC/BRD4 inhibitors relieves neuropathic pain by attenuating inflammatory response in microglia after spared nerve injury. Neurotherapeutics. (2022) 19:1634–48. doi: 10.1007/s13311-022-01243-6
27. Borgonetti V, Mugnaini C, Corelli F, Galeotti N. The selective CB2 agonist COR167 reduced symptoms in a mice model of trauma-induced peripheral neuropathy through HDAC-1 inhibition. Biomedicines. (2023) 11. doi: 10.3390/biomedicines11061546
28. Borgonetti V, Roberts AJ, Bajo M, Galeotti N, Roberto M. Chronic alcohol induced mechanical allodynia by promoting neuroinflammation: A mouse model of alcohol-evoked neuropathic pain. Br J Pharmacol. (2023) 180:2377–92. doi: 10.1111/bph.v180.18
29. Cai Y, Kong H, Pan YB, Jiang L, Pan XX, Hu L, et al. Procyanidins alleviates morphine tolerance by inhibiting activation of NLRP3 inflammasome in microglia. J Neuroinflammation. (2016) 13:14. doi: 10.1186/s12974-016-0520-z
30. Cai Y, Xu J, Cheng Q. Proto-oncogene tyrosine-protein kinase SRC (Src) inhibition in microglia relieves neuroinflammation in neuropathic pain mouse models. Bioengineered. (2021) 12:11390–8. doi: 10.1080/21655979.2021.2008694
31. Cao L, Beaulac H, Eurich A. Differential lumbar spinal cord responses among wild type, CD4 knockout, and CD40 knockout mice in spinal nerve L5 transection-induced neuropathic pain. Mol Pain. (2012) 8:88. doi: 10.1186/1744-8069-8-88
32. Chen G, Luo X, Qadri MY, Berta T, Ji RR. Sex-dependent glial signaling in pathological pain: distinct roles of spinal microglia and astrocytes. Neurosci Bulletin. (2018) 34:98–108. doi: 10.1007/s12264-017-0145-y
33. Chen J, Chen YQ, Shi YJ, Ding SQ, Shen L, Wang R, et al. VX-765 reduces neuroinflammation after spinal cord injury in mice. Neural Regeneration Res. (2021) 16:1836–47. doi: 10.4103/1673-5374.306096
34. Chen L, Hu Y, Wang S, Cao K, Mai W, Sha W, et al. mTOR-neuropeptide Y signaling sensitizes nociceptors to drive neuropathic pain. JCI Insight. (2022) 7. doi: 10.1172/jci.insight.159247
35. Chen Y, Willcockson HH, Valtschanoff JG. Influence of the vanilloid receptor TRPV1 on the activation of spinal cord glia in mouse models of pain. Exp Neurology. (2009) 220:383–90. doi: 10.1016/j.expneurol.2009.09.030
36. Chen Y, Zhang ZX, Zheng LP, Wang L, Liu YF, Yin WY, et al. The adenosine A2A receptor antagonist SCH58261 reduces macrophage/microglia activation and protects against experimental autoimmune encephalomyelitis in mice. Neurochemistry Int. (2019) 129:10. doi: 10.1016/j.neuint.2019.104490
37. Chen YY, Shi YQ, Wang GX, Li Y, Cheng LZ, Wang Y. Memantine selectively prevented the induction of dynamic allodynia by blocking Kir2.1 channel and inhibiting the activation of microglia in spinal dorsal horn of mice in spared nerve injury model. Mol Pain. (2019) 15:11. doi: 10.1177/1744806919838947
38. Cheng H, Zhang Y, Lu W, Gao X, Xu C, Bao H. Caffeic acid phenethyl ester attenuates neuropathic pain by suppressing the p38/NF-κB signal pathway in microglia. J Pain Res. (2018) 11:2709–19. doi: 10.2147/JPR.S166274
39. Choi B, Soh M, Manandhar Y, Kim D, Han SI, Baik S, et al. Highly selective microglial uptake of ceria-zirconia nanoparticles for enhanced analgesic treatment of neuropathic pain. Nanoscale. (2019) 11:19437–47. doi: 10.1039/C9NR02648G
40. Choi SR, Beitz AJ, Lee JH. Inhibition of cytochrome P450c17 reduces spinal astrocyte activation in a mouse model of neuropathic pain via regulation of p38 MAPK phosphorylation. Biomedicine Pharmacotherapy. (2019) 118. doi: 10.1016/j.biopha.2019.109299
41. Chu ZM, Lu Y, Qin RJ, Dong YF. LncRNA KCNQ1OT1 promotes the apoptosis and inflammatory response of microglia by regulating the miR-589-5p/NPTN axis after spinal cord injury. Anais Da Academia Bras Ciencias. (2022) 94:13. doi: 10.1590/0001-3765202220210188
42. Chuang TY, Guo Y, Seki SM, Rosen AM, Johanson DM, Mandell JW, et al. LRP1 expression in microglia is protective during CNS autoimmunity. Acta Neuropathologica Commun. (2016) 4:12. doi: 10.1186/s40478-016-0343-2
43. Ciapała K, Pawlik K, Ciechanowska A, Mika J, Rojewska E. Effect of pharmacological modulation of the kynurenine pathway on pain-related behavior and opioid analgesia in a mouse model of neuropathic pain. Toxicol Appl Pharmacol. (2023) 461. doi: 10.1016/j.taap.2023.116382
44. Dalenogare DP, Souza Monteiro de Araújo D, Landini L, Titiz M, De Siena G, De Logu F, et al. Neuropathic-like nociception and spinal cord neuroinflammation are dependent on the TRPA1 channel in multiple sclerosis models in mice. Cells. (2023) 12. doi: 10.3390/cells12111511
45. Damo E, Agarwal A, Simonetti M. Activation of β2-adrenergic receptors in microglia alleviates neuropathic hypersensitivity in mice. Cells. (2023) 12:28. doi: 10.3390/cells12020284
46. de Oliveira LRC, Mimura LAN, Fraga-Silva TFD, Ishikawa LLW, Fernandes AAH, Zorzella-Pezavento SFG, et al. Calcitriol prevents neuroinflammation and reduces blood-brain barrier disruption and local macrophage/microglia activation. Front Pharmacol. (2020) 11:15. doi: 10.3389/fphar.2020.00161
47. DeLeo JA, Rutkowski MD, Stalder AK, Campbell IL. Transgenic expression of TNF by astrocytes increases mechanical allodynia in a mouse neuropathy model. NeuroReport. (2000) 11:599–602. doi: 10.1097/00001756-200002280-00033
48. Ding Y, Hu L, Wang X, Sun Q, Hu T, Liu J, et al. The contribution of spinal dorsal horn astrocytes in neuropathic pain at the early stage of EAE. Neurobiol Dis. (2022) 175. doi: 10.1016/j.nbd.2022.105914
49. Djelloul M, Popa N, Pelletier F, Raguénez G, Boucraut J. RAE-1 expression is induced during experimental autoimmune encephalomyelitis and is correlated with microglia cell proliferation. Brain Behav Immunity. (2016) 58:209–17. doi: 10.1016/j.bbi.2016.07.147
50. Dolci S, Mannino L, Bottani E, Campanelli A, Di Chio M, Zorzin S, et al. Therapeutic induction of energy metabolism reduces neural tissue damage and increases microglia activation in severe spinal cord injury. Pharmacol Res. (2022) 178:13. doi: 10.1016/j.phrs.2022.106149
51. Doolen S, Iannitti T, Donahue RR, Shaw BC, Grachen CM, Taylor BK. Fingolimod reduces neuropathic pain behaviors in a mouse model of multiple sclerosis by a sphingosine-1 phosphate receptor 1-dependent inhibition of central sensitization in the dorsal horn. Pain. (2018) 159:224–38. doi: 10.1097/j.pain.0000000000001106
52. Evans TA, Barkauskas DS, Myers JT, Hare EG, You JQ, Ransohoff RM, et al. High-resolution intravital imaging reveals that blood-derived macrophages but not resident microglia facilitate secondary axonal dieback in traumatic spinal cord injury. Exp Neurology. (2014) 254:109–20. doi: 10.1016/j.expneurol.2014.01.013
53. Fang Q, Li J, Wang YP, Liu XL, Shi Y, Chen JL, et al. AdipoRon engages microglia to antinociception through the AdipoR1/AMPK pathway in SNI mice. Mediators Inflammation. (2023) 2023:11. doi: 10.1155/2023/7661791
54. Fang Y, Cui H, Liu F, Su S, Wang T, Yuan B, et al. Astrocytic phosphatase and tensin homolog deleted on chromosome 10 regulates neuropathic pain by facilitating 3-hydroxy-3-methylglutaryl-CoA reductase-dependent cholesterol biosynthesis. Pain. (2022) 163:E1192–E206. doi: 10.1097/j.pain.0000000000002682
55. Fenn AM, Hall JCE, Gensel JC, Popovich PG, Godbout JP. IL-4 signaling drives a unique arginase+/IL-1β+ Microglia phenotype and recruits macrophages to the inflammatory CNS: consequences of age-related deficits in IL-4Rα after traumatic spinal cord injury. J Neurosci. (2014) 34:8904–17. doi: 10.1523/JNEUROSCI.1146-14.2014
56. Fernandez-Zafra T, Gao TL, Jurczak A, Sandor K, Hore Z, Agalave NM, et al. Exploring the transcriptome of resident spinal microglia after collagen antibody-induced arthritis. Pain. (2019) 160:224–36. doi: 10.1097/j.pain.0000000000001394
57. Ferraz CR, Carvalho TT, Fattori V, Saraiva-Santos T, Pinho-Ribeiro FA, Borghi SM, et al. Jararhagin, a snake venom metalloproteinase, induces mechanical hyperalgesia in mice with the neuroinflammatory contribution of spinal cord microglia and astrocytes. Int J Biol Macromolecules. (2021) 179:610–9. doi: 10.1016/j.ijbiomac.2021.02.178
58. Finocchiaro A, Marinelli S, De Angelis F, Vacca V, Luvisetto S, Pavone F. Botulinum toxin b affects neuropathic pain but not functional recovery after peripheral nerve injury in a mouse model. Toxins. (2018) 10. doi: 10.3390/toxins10030128
59. Foley JC, McIver SR, Haydon PG. Gliotransmission modulates baseline mechanical nociception. Mol Pain. (2011) 7:93. doi: 10.1186/1744-8069-7-93
60. Freria CM, Brennan FH, Sweet DR, Guan Z, Hall JC, Kigerl KA, et al. Serial systemic injections of endotoxin (LPS) elicit neuroprotective spinal cord microglia through IL-1-dependent cross talk with endothelial cells. J Neurosci. (2020) 40:9103–20. doi: 10.1523/JNEUROSCI.0131-20.2020
61. García-Magro N, Martin YB, Negredo P, Zafra F, Avendaño C. Microglia and inhibitory circuitry in the medullary dorsal horn: laminar and time-dependent changes in a trigeminal model of neuropathic pain. Int J Mol Sci. (2021) 22:16. doi: 10.3390/ijms22094564
62. Gerber YN, Saint-Martin GP, Bringuier CM, Bartolami S, Goze-Bac C, Noristani HN, et al. CSF1R inhibition reduces microglia proliferation, promotes tissue preservation and improves motor recovery after spinal cord injury. Front Cell Neurosci. (2018) 12:17. doi: 10.3389/fncel.2018.00368
63. Greenhalgh AD, David S. Differences in the phagocytic response of microglia and peripheral macrophages after spinal cord injury and its effects on cell death. J Neurosci. (2014) 34:6316–22. doi: 10.1523/JNEUROSCI.4912-13.2014
64. Gu N, Peng J, Murugan M, Wang X, Eyo UB, Sun D, et al. Spinal microgliosis due to resident microglial proliferation is required for pain hypersensitivity after peripheral nerve injury. Cell Rep. (2016) 16:605–14. doi: 10.1016/j.celrep.2016.06.018
65. Gu N, Yi MH, Murugan M, Xie ML, Parusel S, Peng JY, et al. Spinal microglia contribute to sustained inflammatory pain via amplifying neuronal activity. Mol brain. (2022) 15:19. doi: 10.1186/s13041-022-00970-3
66. Guida F, Lattanzi R, Boccella S, Maftei S, Romano R, Marconi R, et al. PC1, a non-peptide PKR1-preferring antagonist, reduces pain behavior and spinal neuronal sensitization in neuropathic mice. Pharmacol Res. (2014) 91:36–46. doi: 10.1016/j.phrs.2014.11.004
67. Guo Y, Zhang H, Yang J, Liu S, Bing L, Gao J, et al. Granulocyte colony-stimulating factor improves alternative activation of microglia under microenvironment of spinal cord injury. Neuroscience. (2013) 238:1–10. doi: 10.1016/j.neuroscience.2013.01.047
68. Haight ES, Johnson EM, Carroll IR, Tawfik VL. Of mice, microglia, and (wo)men: a case series and mechanistic investigation of hydroxychloroquine for complex regional pain syndrome. Pain Rep. (2020) 5:9. doi: 10.1097/PR9.0000000000000841
69. Hakim R, Zachariadis V, Sankavaram SR, Han JM, Harris RA, Brundin L, et al. Spinal cord injury induces permanent reprogramming of microglia into a disease-associated state which contributes to functional recovery. J Neurosci. (2021) 41:8441–59. doi: 10.1523/JNEUROSCI.0860-21.2021
70. Halder SK, Milner R. A critical role for microglia in maintaining vascular integrity in the hypoxic spinal cord. Proc Natl Acad Sci United States America. (2019) 116:26029–37. doi: 10.1073/pnas.1912178116
71. Han DX, Yu ZW, Liu WL, Yin D, Pu YY, Feng JF, et al. Plasma Hemopexin ameliorates murine spinal cord injury by switching microglia from the M1 state to the M2 state. Cell Death Disease. (2018) 9:16. doi: 10.1038/s41419-017-0236-8
72. Han J, Cho HJ, Park D, Han S. DICAM in the extracellular vesicles from astrocytes attenuates microglia activation and neuroinflammation. Cells. (2022) 11:18. doi: 10.3390/cells11192977
73. Hansmann F, Zhang N, Herder V, Leitzen E, Baumgärtner W. Delayed astrogliosis associated with reduced M1 microglia activation in matrix metalloproteinase 12 knockout mice during Theiler's murine encephalomyelitis. Int J Mol Sci. (2019) 20:14. doi: 10.3390/ijms20071702
74. Herder V, Iskandar CD, Kegler K, Hansmann F, Elmarabet SA, Khan MA, et al. Dynamic changes of microglia/macrophage M1 and M2 polarization in theiler's murine encephalomyelitis. Brain Pathology. (2015) 25:712–23. doi: 10.1111/bpa.2015.25.issue-6
75. Hervera A, Leanez S, Motterlini R, Pol O. Treatment with carbon monoxide-releasing molecules and an HO-1 inducer enhances the effects and expression of mu-opioid receptors during neuropathic pain. Anesthesiology. (2013) 118:1180–97. doi: 10.1097/ALN.0b013e318286d085
76. Hirai T, Uchida K, Nakajima H, Guerrero AR, Takeura N, Watanabe S, et al. The prevalence and phenotype of activated microglia/macrophages within the spinal cord of the hyperostotic mouse (twy/twy) changes in response to chronic progressive spinal cord compression: implications for human cervical compressive myelopathy. PloS One. (2013) 8:14. doi: 10.1371/journal.pone.0064528
77. Hong S, Niu M, Meng DM, Li AQ, Dong QZ, Zhang J, et al. High-density lipoprotein reduces microglia activation and protects against experimental autoimmune encephalomyelitis in mice. Int Immunopharmacology. (2022) 105:14. doi: 10.1016/j.intimp.2022.108566
78. Hong X, Jiang F, Li Y, Fang L, Qian ZY, Chen HT, et al. Treatment with 5-methoxytryptophan attenuates microglia-induced neuroinflammation in spinal cord trauma. Int Immunopharmacology. (2020) 88:8. doi: 10.1016/j.intimp.2020.106988
79. Honjoh K, Nakajima H, Hirai T, Watanabe S, Matsumine A. Relationship of inflammatory cytokines from M1-type microglia/macrophages at the injured site and lumbar enlargement with neuropathic pain after spinal cord injury in the CCL21 knockout (plt) mouse. Front Cell Neurosci. (2019) 13:10. doi: 10.3389/fncel.2019.00525
80. Hozumi T, Sawai S, Jitsuishi T, Kitajo K, Inage K, Eguchi Y, et al. Gene expression profiling of the spinal cord at the chronic pain phase identified CDKL5 as a candidate gene for neural remodeling. Neurosci Lett. (2021) 749. doi: 10.1016/j.neulet.2021.135772
81. Hu T, Sun Q, Gou Y, Zhang Y, Ding Y, Ma Y, et al. Salidroside alleviates chronic constriction injury-induced neuropathic pain and inhibits of TXNIP/NLRP3 pathway. Neurochemical Res. (2022) 47:493–502. doi: 10.1007/s11064-021-03459-y
82. Hu TT, Wang RR, Tang YY, Wu YX, Yu J, Hou WW, et al. TLR4 deficiency abrogated widespread tactile allodynia, but not widespread thermal hyperalgesia and trigeminal neuropathic pain after partial infraorbital nerve transection. Pain. (2018) 159:273–83. doi: 10.1097/j.pain.0000000000001100
83. Hu X, Du L, Liu S, Lan Z, Zang K, Feng J, et al. A TRPV4-dependent neuroimmune axis in the spinal cord promotes neuropathic pain. J Clin Invest. (2023) 133. doi: 10.1172/JCI161507
84. Hu XY, Huang JX, Li YT, Dong L, Chen YH, Ouyang FR, et al. TAZ induces migration of microglia and promotes neurological recovery after spinal cord injury. Front Pharmacol. (2022) 13:14. doi: 10.3389/fphar.2022.938416
85. Hu Z, Yu X, Chen P, Jin K, Zhou J, Wang G, et al. BDNF-TrkB signaling pathway-mediated microglial activation induces neuronal KCC2 downregulation contributing to dynamic allodynia following spared nerve injury. Mol Pain. (2023) 19. doi: 10.1177/17448069231185439
86. Huang YL, Hao J, Yang XL, Xu L, Liu Y, Sun YE, et al. Pretreatment of the ROS inhibitor phenyl-N-tert-butylnitrone alleviates sleep deprivation-induced hyperalgesia by suppressing microglia activation and NLRP3 inflammasome activity in the spinal dorsal cord. Neurochemical Res. (2023) 48:305–14. doi: 10.1007/s11064-022-03751-5
87. Hunyady Á, Hajna Z, Gubányi T, Scheich B, Kemény Á, Gaszner B, et al. Hemokinin-1 is an important mediator of pain in mouse models of neuropathic and inflammatory mechanisms. Brain Res Bulletin. (2019) 147:165–73. doi: 10.1016/j.brainresbull.2019.01.015
88. Huo W, Liu Y, Lei Y, Zhang Y, Huang Y, Mao Y, et al. Imbalanced spinal infiltration of Th17/Treg cells contributes to bone cancer pain via promoting microglial activation. Brain Behavior Immunity. (2019) 79:139–51. doi: 10.1016/j.bbi.2019.01.024
89. Huo W, Zhang Y, Liu Y, Lei Y, Sun R, Zhang W, et al. Dehydrocorydaline attenuates bone cancer pain by shifting microglial M1/M2 polarization toward the M2 phenotype. Mol Pain. (2018) 14. doi: 10.1177/1744806918781733
90. Hutchinson JM, Isaacson LG. Elimination of microglia in mouse spinal cord alters the retrograde CNS plasticity observed following peripheral axon injury. Brain Res. (2019) 1721:11. doi: 10.1016/j.brainres.2019.146328
91. Imari K, Harada Y, Zhang J, Mori Y, Hayashi Y. KCNMB3 in spinal microglia contributes to the generation and maintenance of neuropathic pain in mice. Int J Mol Med. (2019) 44:1585–93. doi: 10.3892/ijmm.2019.4279
92. Isami K, Imai S, Sukeishi A, Nagayasu K, Shirakawa H, Nakagawa T, et al. The impact of mouse strain-specific spatial and temporal immune responses on the progression of neuropathic pain. Brain Behavior Immunity. (2018) 74:121–32. doi: 10.1016/j.bbi.2018.08.013
93. Ishii H, Tanabe S, Ueno M, Kubo T, Kayama H, Serada S, et al. ifn-γ-dependent secretion of IL-10 from Th1 cells and microglia/macrophages contributes to functional recovery after spinal cord injury. Cell Death Disease. (2013) 4:9. doi: 10.1038/cddis.2013.234
94. Jeffrey-Gauthier R, Bouyer J, Piché M, Côté MP, Leblond H. Locomotor deficits induced by lumbar muscle inflammation involve spinal microglia and are independent of KCC2 expression in a mouse model of complete spinal transection. Exp Neurology. (2021) 338. doi: 10.1016/j.expneurol.2020.113592
95. Jeong H, Na YJ, Lee K, Kim YH, Lee Y, Kang M, et al. High-resolution transcriptome analysis reveals neuropathic pain gene-expression signatures in spinal microglia after nerve injury. Pain. (2016) 157:964–76. doi: 10.1097/j.pain.0000000000000470
96. Ji XB, Song ZW, He JS, Guo SW, Chen YW, Wang HH, et al. NIMA-related kinase 7 amplifies NLRP3 inflammasome pro-inflammatory signaling in microglia/macrophages and mice models of spinal cord injury. Exp Cell Res. (2021) 398:9. doi: 10.1016/j.yexcr.2020.112418
97. Jiang JH, Xu LF, Yang L, Liu S, Wang Z. Mitochondrial-derived peptide MOTS-c ameliorates spared nerve injury-induced neuropathic pain in mice by inhibiting microglia activation and neuronal oxidative damage in the spinal cord via the AMPK pathway. ACS Chem Neurosci. (2023) 14:2362–74. doi: 10.1021/acschemneuro.3c00140
98. Jiang S, Baba K, Okuno T, Kinoshita M, Choong CJ, Hayakawa H, et al. Go-sha-jinki-Gan Alleviates Inflammation in Neurological Disorders via p38-TNF Signaling in the Central Nervous System. Neurotherapeutics. (2021) 18:460–73. doi: 10.1007/s13311-020-00948-w
99. Jones M, Wen J, Selvaraj P, Tanaka M, Moran S, Zhang Y. Therapeutic effect of the substrate-selective COX-2 inhibitor IMMA in the animal model of chronic constriction injury. Front Pharmacol. (2018) 9. doi: 10.3389/fphar.2018.01481
100. Khan N, Gordon R, Woodruff TM, Smith MT. Antiallodynic effects of alpha lipoic acid in an optimized RR-EAE mouse model of MS-neuropathic pain are accompanied by attenuation of upregulated BDNF-TrkB-ERK signaling in the dorsal horn of the spinal cord. Pharmacol Res Perspectives. (2015) 3. doi: 10.1002/prp2.137
101. Kim D, You B, Jo EK, Han SK, Simon MI, Lee SJ. NADPH oxidase 2-derived reactive oxygen species in spinal cord microglia contribute to peripheral nerve injury-induced neuropathic pain. Proc Natl Acad Sci United States America. (2010) 107:14851–6. doi: 10.1073/pnas.1009926107
102. Kim H, Choi B, Lim H, Min H, Oh JH, Choi S, et al. Polyamidoamine dendrimer-conjugated triamcinolone acetonide attenuates nerve injury-induced spinal cord microglia activation and mechanical allodynia. Mol Pain. (2017) 13:11. doi: 10.1177/1744806917697006
103. Kim JH, Park JS, Park D. Anti-allodynic effect of interleukin 10 in a mouse model of complex regional pain syndrome through reduction of NKI receptor expression of microglia in the spinal cord. J Pain Res. (2018) 11:1729–41. doi: 10.2147/JPR.S166624
104. Kim JW, Park HS, You MJ, Yang B, Jang KB, Kwon MS. Time course of peripheral and central immune system alterations in paclitaxel-treated mice: possible involvement of dysfunctional microglia. Neurochemical J. (2020) 14:204–14. doi: 10.1134/S1819712420020063
105. Kitayama M, Ueno M, Itakura T, Yamashita T. Activated microglia inhibit axonal growth through RGMa. PloS One. (2011) 6:9. doi: 10.1371/journal.pone.0025234
106. Kobashi S, Terashima T, Katagi M, Nakae Y, Okano J, Suzuki Y, et al. Transplantation of M2-deviated microglia promotes recovery of motor function after spinal cord injury in mice. Mol Ther. (2020) 28:254–65. doi: 10.1016/j.ymthe.2019.09.004
107. Kobashi S, Terashima T, Katagi M, Urushitani M, Kojima H. Bone marrow-derived inducible microglia-like cells ameliorate motor function and survival in a mouse model of amyotrophic lateral sclerosis. Cytotherapy. (2022) 24:789–801. doi: 10.1016/j.jcyt.2022.02.001
108. Komori T, Morikawa Y, Inada T, Hisaoka T, Senba E. Site-specific subtypes of macrophages recruited after peripheral nerve injury. NeuroReport. (2011) 22:911–7. doi: 10.1097/WNR.0b013e32834cd76a
109. Kong ER, Li YC, Ma P, Zhang YX, Ding RF, Hua T, et al. Lyn-mediated glycolysis enhancement of microglia contributes to neuropathic pain through facilitating IRF5 nuclear translocation in spinal dorsal horn. J Cell Mol Med. (2023) 27:1664–81. doi: 10.1111/jcmm.v27.12
110. Kosaka Y, Yafuso T, Shimizu-Okabe C, Kim J, Kobayashi S, Okura N, et al. Development and persistence of neuropathic pain through microglial activation and KCC2 decreasing after mouse tibial nerve injury. Brain Res. (2020) 1733:146718. doi: 10.1016/j.brainres.2020.146718
111. Koshimizu H, Ohkawara B, Nakashima H, Ota K, Kanbara S, Inoue T, et al. Zonisamide ameliorates neuropathic pain partly by suppressing microglial activation in the spinal cord in a mouse model. Life Sci. (2020) 263. doi: 10.1016/j.lfs.2020.118577
112. Lee J, Hwang H, Lee SJ. Distinct roles of GT1b and CSF-1 in microglia activation in nerve injury-induced neuropathic pain. Mol Pain. (2021) 17:8. doi: 10.1177/17448069211020918
113. Lee JY, Choi HY, Yune TY. MMP-3 secreted from endothelial cells of blood vessels after spinal cord injury activates microglia, leading to oligodendrocyte cell death. Neurobiol Disease. (2015) 82:141–51. doi: 10.1016/j.nbd.2015.06.002
114. Lee S, Shi XQ, Fan AN, West B, Zhang J. Targeting macrophage and microglia activation with colony stimulating factor 1 receptor inhibitor is an effective strategy to treat injury-triggered neuropathic pain. Mol Pain. (2018) 14:12. doi: 10.1177/1744806918764979
115. Lee S, Wu Y, Shi XQ, Zhang J. Characteristics of spinal microglia in aged and obese mice: potential contributions to impaired sensory behavior. Immun Ageing. (2015) 12:10. doi: 10.1186/s12979-015-0049-5
116. Li H, Wang PF, Tang L, Sun JJ, Zhang YL, Luo W, et al. Distinct polarization dynamics of microglia and infiltrating macrophages: A novel mechanism of spinal cord ischemia/reperfusion injury. J Inflammation Res. (2021) 14:5227–39. doi: 10.2147/JIR.S335382
117. Li J, Wei GH, Huang H, Lan YP, Liu B, Liu H, et al. Nerve injury-related autoimmunity activation leads to chronic inflammation and chronic neuropathic pain. Anesthesiology. (2013) 118:416–29. doi: 10.1097/ALN.0b013e31827d4b82
118. Li J, Wei YY, Zhou JL, Zou HL, Ma LL, Liu CX, et al. Activation of locus coeruleus-spinal cord noradrenergic neurons alleviates neuropathic pain in mice via reducing neuroinflammation from astrocytes and microglia in spinal dorsal horn. J Neuroinflammation. (2022) 19:19. doi: 10.1186/s12974-022-02489-9
119. Li JL, Fu GQ, Wang YY, Bian MM, Xu YM, Zhang L, et al. The polarization of microglia and infiltrated macrophages in the injured mice spinal cords: a dynamic analysis. Peerj. (2023) 11:23. doi: 10.7717/peerj.14929
120. Li Q, Li R, Zhu X, Chu X, An X, Chen M, et al. EphA1 aggravates neuropathic pain by activating CXCR4/RhoA/ROCK2 pathway in mice. Hum Cell. (2023) 36:1416–28. doi: 10.1007/s13577-023-00911-9
121. Li XY, Kang JN, Lv H, Liu RH, Chen JA, Zhang YN, et al. CircPrkcsh, a circular RNA, contributes to the polarization of microglia towards the M1 phenotype induced by spinal cord injury and acts via the JNK/p38 MAPK pathway. FASEB J. (2021) 35:18. doi: 10.1096/fj.202100993R
122. Li XY, Wu GZ, Li MY, Zhang Z. Oleanolic acid administration alleviates neuropathic pain after a peripheral nerve injury by regulating microglia polarization-mediated neuroinflammation. Rsc Advances. (2020) 10:12920–8. doi: 10.1039/C9RA10388K
123. Liao YC, Wang JW, Zhang JL, Guo C, Xu XL, Wang K, et al. Component-target network and mechanism of Qufeng Zhitong capsule in the treatment of neuropathic pain. J Ethnopharmacology. (2022) 283. doi: 10.1016/j.jep.2021.114532
124. Lim H, Lee H, Noh K, Lee SJ. IKK/NF-B-dependent satellite glia activation induces spinal cord microglia activation and neuropathic pain after nerve injury. Pain. (2017) 158:1666–77. doi: 10.1097/j.pain.0000000000000959
125. Liu R, Li Y, Wang ZY, Chen P, Xie Y, Qu WS, et al. Regulatory T cells promote functional recovery after spinal cord injury by alleviating microglia inflammation via STAT3 inhibition. CNS Neurosci Ther. (2023) 29:2129–44. doi: 10.1111/cns.14161
126. Liu Y, Kano F, Hashimoto N, Xia L, Zhou Q, Feng X, et al. Conditioned medium from the stem cells of human exfoliated deciduous teeth ameliorates neuropathic pain in a partial sciatic nerve ligation model. Front Pharmacol. (2022) 13. doi: 10.3389/fphar.2022.745020
127. Liu Y, Zhou LJ, Wang J, Li D, Ren WJ, Peng JY, et al. TNF-α Differentially regulates synaptic plasticity in the hippocampus and spinal cord by microglia-dependent mechanisms after peripheral nerve injury. J Neurosci. (2017) 37:871–81. doi: 10.1523/JNEUROSCI.2235-16.2016
128. Livni L, Keating BA, Fiore NT, Lees JG, Goldstein D, Moalem-Taylor G. Effects of combined chemotherapy and anti-programmed cell death protein 1 treatment on peripheral neuropathy and neuroinflammation in mice. Pain. (2022) 163:110–24. doi: 10.1097/j.pain.0000000000002384
129. Lopes DM, Malek N, Edye M, Jager SB, McMurray S, McMahon SB, et al. Sex differences in peripheral not central immune responses to pain-inducing injury. Sci Rep. (2017) 7. doi: 10.1038/s41598-017-16664-z
130. Lopes FSR, Giardini AC, Sant'Anna MB, Kimura LF, Bufalo MC, Vigerelli H, et al. Crotalphine modulates microglia M1/M2 phenotypes and induces spinal analgesia mediated by opioid-cannabinoid systems. Int J Mol Sci. (2022) 23:16. doi: 10.3390/ijms231911571
131. Lu X, Chen LH, Jiang C, Cao KL, Gao ZH, Wang Y. Microglia and macrophages contribute to the development and maintenance of sciatica in lumbar disc herniation. Pain. (2023) 164:362–74. doi: 10.1097/j.pain.0000000000002708
132. Lu Y, Zhang J, Zeng F, Wang P, Guo X, Wang H, et al. Human PMSCs-derived small extracellular vesicles alleviate neuropathic pain through miR-26a-5p/Wnt5a in SNI mice model. J Neuroinflamm. (2022) 19. doi: 10.1186/s12974-022-02578-9
133. Luongo L, Petrelli R, Gatta L, Giordano C, Guida F, Vita P, et al. 5'-Chloro-5'-deoxy-(±)-ENBA, a potent and selective adenosine A1 receptor agonist, alleviates neuropathic pain in mice through functional glial and microglial changes without affecting motor or cardiovascular functions. Molecules. (2012) 17:13712–26. doi: 10.3390/molecules171213712
134. Ma DZ, Zhao YN, Huang L, Xiao ZF, Chen B, Shi Y, et al. A novel hydrogel-based treatment for complete transection spinal cord injury repair is driven by microglia/macrophages repopulation. Biomaterials. (2020) 237:14. doi: 10.1016/j.biomaterials.2020.119830
135. Ma L, Zhou YH, Khan MAS, Yasuhara S, Martyn JAJ. Burn-induced microglia activation is associated with motor neuron degeneration and muscle wasting in mice. Shock. (2019) 51:569–79. doi: 10.1097/SHK.0000000000001300
136. Ma XR, Wang S, Li CL, Jia XH, Wang TT, Leng Z, et al. Baicalein inhibits the polarization of microglia/macrophages to the M1 phenotype by targeting STAT1 in EAE mice. Int Immunopharmacology. (2022) 113:12. doi: 10.1016/j.intimp.2022.109373
137. Masuda T, Iwamoto S, Yoshinaga R, Tozaki-Saitoh H, Nishiyama A, Mak TW, et al. Transcription factor IRF5 drives P2X4R+-reactive microglia gating neuropathic pain. Nat Commun. (2014) 5:11. doi: 10.1038/ncomms4771
138. Masuda T, Tsuda M, Yoshinaga R, Tozaki-Saitoh H, Ozato K, Tamura T, et al. IRF8 is a critical transcription factor for transforming microglia into a reactive phenotype. Cell Rep. (2012) 1:334–40. doi: 10.1016/j.celrep.2012.02.014
139. Matsuo H, Uchida K, Nakajima H, Guerrero AR, Watanabe S, Takeura N, et al. Early transcutaneous electrical nerve stimulation reduces hyperalgesia and decreases activation of spinal glial cells in mice with neuropathic pain. Pain. (2014) 155:1888–901. doi: 10.1016/j.pain.2014.06.022
140. Mika J, Osikowicz M, Rojewska E, Korostynski M, Wawrzczak-Bargiela A, Przewlocki R, et al. Differential activation of spinal microglial and astroglial cells in a mouse model of peripheral neuropathic pain. Eur J Pharmacol. (2009) 623:65–72. doi: 10.1016/j.ejphar.2009.09.030
141. Mishra MK, Rawji KS, Keough MB, Kappen J, Dowlatabadi R, Vogel HJ, et al. Harnessing the benefits of neuroinflammation: generation of macrophages/microglia with prominent remyelinating properties. J Neurosci. (2021) 41:3366–85. doi: 10.1523/JNEUROSCI.1948-20.2021
142. Miskimon M, Han S, Lee JJ, Ringkamp M, Wilson MA, Petralia RS, et al. Selective expression of Narp in primary nociceptive neurons: Role in microglia/macrophage activation following nerve injury. J Neuroimmunology. (2014) 274:86–95. doi: 10.1016/j.jneuroim.2014.06.016
143. Montilla A, Zabala A, Er-Lukowiak M, Rissiek B, Magnus T, Rodriguez-Iglesias N, et al. Microglia and meningeal macrophages depletion delays the onset of experimental autoimmune encephalomyelitis. Cell Death Disease. (2023) 14:13. doi: 10.1038/s41419-023-05551-3
144. Morimoto SI, Ito M, Oda S, Sugiyama A, Kuroda M, Adachi-Akahane S. Spinal mechanism underlying the antiallodynic effect of gabapentin studied in the mouse spinal nerve ligation model. J Pharmacol Sci. (2012) 118:455–66. doi: 10.1254/jphs.11102FP
145. Moura CCV, dos Santos RS, Duarte LP, Galdino G. Cannabinoid CB2 receptors and spinal microglia are implicated in tingenone-mediated antinociception in mice. Asian Pacific J Trop Biomedicine. (2021) 11:141–7. doi: 10.4103/2221-1691.310200
146. Mukaino M, Nakamura M, Yamada O, Okada S, Morikawa S, Renault-Mihara F, et al. Anti-IL-6-receptor antibody promotes repair of spinal cord injury by inducing microglia-dominant inflammation. Exp Neurology. (2010) 224:403–14. doi: 10.1016/j.expneurol.2010.04.020
147. Nakajima H, Honjoh K, Watanabe S, Kubota A, Matsumine A. Distribution and polarization of microglia and macrophages at injured sites and the lumbar enlargement after spinal cord injury. Neurosci Letters. (2020) 737:8. doi: 10.1016/j.neulet.2020.135152
148. Nakamura Y, Fukuta A, Miyashita K, Zhang FF, Wang DL, Liu KY, et al. Perineural high-mobility group box 1 induces mechanical hypersensitivity through activation of spinal microglia: Involvement of glutamate-NMDA receptor dependent mechanism in spinal dorsal horn. Biochem Pharmacol. (2021) 186:9. doi: 10.1016/j.bcp.2021.114496
149. Narita M, Yoshida T, Nakajima M, Narita M, Miyatake M, Takagi T, et al. Direct evidence for spinal cord microglia in the development of a neuropathic pain-like state in mice. J Neurochemistry. (2006) 97:1337–48. doi: 10.1111/j.1471-4159.2006.03808.x
150. Nent E, Nozaki C, Schmöle AC, Otte D, Zimmer A. CB2 receptor deletion on myeloid cells enhanced mechanical allodynia in a mouse model of neuropathic pain. Sci Rep. (2019) 9. doi: 10.1038/s41598-019-43858-4
151. Nicol LS, Dawes JM, Russa FL, Didangelos A, Clark AK, Gentry C, et al. The role of G-protein receptor 84 in experimental neuropathic pain. J Neurosci. (2015) 35:8959–69. doi: 10.1523/JNEUROSCI.3558-14.2015
152. Nishi R, Ohyagi M, Nagata T, Mabuchi Y, Yokota T. Regulation of activated microglia and macrophages by systemically administered DNA/RNA heteroduplex oligonucleotides. Mol Ther. (2022) 30:2210–23. doi: 10.1016/j.ymthe.2022.02.019
153. Fiore NT, Yin Z, Guneykaya D, Gauthier CD, Hayes JP, D'Hary A, et al. Sex-specific transcriptome of spinal microglia in neuropathic pain due to peripheral nerve injury. Glia. (2022) 71:675–96. doi: 10.1002/glia.24320
154. Noristani HN, Boukhaddaoui H, Saint-Martin G, Auzer P, Sidiboulenouar R, Lonjon N, et al. A Combination of Ex vivo Diffusion MRI and Multiphoton to Study Microglia/Monocytes Alterations after Spinal Cord Injury. Front Aging Neurosci. (2017) 9:12. doi: 10.3389/fnagi.2017.00230
155. Okuda H, Ishikawa T, Hori K, Kwankaew N, Ozaki N. Hedgehog signaling plays a crucial role in hyperalgesia associated with neuropathic pain in mice. J Neurochemistry. (2022) 162:207–20. doi: 10.1111/jnc.v162.2
156. Olechowski CJ, Truong JJ, Kerr BJ. Neuropathic pain behaviours in a chronic-relapsing model of experimental autoimmune encephalomyelitis (EAE). Pain. (2009) 141:156–64. doi: 10.1016/j.pain.2008.11.002
157. Omoto H, Matsumura S, Kitano M, Miyazaki S, Minami T, Ito S. Comparison of mechanisms of allodynia induced by acromelic acid A between early and late phases. Eur J Pharmacol. (2015) 760:42–8. doi: 10.1016/j.ejphar.2015.03.075
158. Osikowicz M, Skup M, Mika J, Makuch W, Czarkowska-Bauch J, Przewlocka B. Glial inhibitors influence the mRNA and protein levels of mGlu2/3, 5 and 7 receptors and potentiate the analgesic effects of their ligands in a mouse model of neuropathic pain. Pain. (2009) 147:175–86. doi: 10.1016/j.pain.2009.09.002
159. Pan J, Zhao Y, Sang R, Yang R, Bao J, Wu Y, et al. Huntington-associated protein 1 inhibition contributes to neuropathic pain by suppressing Cav1.2 activity and attenuating inflammation. Pain. (2023) 164:E286–302. doi: 10.1097/j.pain.0000000000002837
160. Papa S, Caron I, Erba E, Panini N, De Paola M, Mariani A, et al. Early modulation of pro-inflammatory microglia by minocycline loaded nanoparticles confers long lasting protection after spinal cord injury. Biomaterials. (2016) 75:13–24. doi: 10.1016/j.biomaterials.2015.10.015
161. Papa S, Rossi F, Ferrari R, Mariani A, De Paola M, Caron I, et al. Selective nanovector mediated treatment of activated proinflammatory microglia/macrophages in spinal cord injury. ACS Nano. (2013) 7:9881–95. doi: 10.1021/nn4036014
162. Pavao-de-Souza GF, Zarpelon AC, Tedeschi GC, Mizokami SS, Sanson JS, Cunha TM, et al. Acetic acid- and phenyl-p-benzoquinone-induced overt pain-like behavior depends on spinal activation of MAP kinases, PI(3)K and microglia in mice. Pharmacol Biochem Behavior. (2012) 101:320–8. doi: 10.1016/j.pbb.2012.01.018
163. Peng J, Gu N, Zhou L, B Eyo U, Murugan M, Gan WB, et al. Microglia and monocytes synergistically promote the transition from acute to chronic pain after nerve injury. Nat Commun. (2016) 7. doi: 10.1038/ncomms12029
164. Peng JY, Yi MH, Jeong H, McEwan PP, Zheng JY, Wu GX, et al. The voltage-gated proton channel Hv1 promotes microglia-astrocyte communication and neuropathic pain after peripheral nerve injury. Mol brain. (2021) 14:18. doi: 10.1186/s13041-021-00812-8
165. Perez JC, Poulen G, Cardoso M, Boukhaddaoui H, Gazard CM, Courtand G, et al. CSF1R inhibition at chronic stage after spinal cord injury modulates microglia proliferation. Glia. (2023) 71:2782–98. doi: 10.1002/glia.v71.12
166. Poulen G, Bartolami S, Noristani HN, Perrin FE, Gerber YN. Unlike brief inhibition of microglia proliferation after spinal cord injury, long-term treatment does not improve motor recovery. Brain Sci. (2021) 11:13. doi: 10.3390/brainsci11121643
167. Poznanski P, Giebultowicz J, Durdzinska J, Kocki T, Sacharczuk M, Bujalska-Zadrozny M, et al. Mechanisms underlining inflammatory pain sensitivity in mice selected for high and low stress-induced analgesia-the role of endocannabinoids and microglia. Int J Mol Sci. (2022) 23:17. doi: 10.3390/ijms231911686
168. Qian ZY, Chen HT, Xia MJ, Chang J, Li XY, Ye SH, et al. Activation of glucagon-like peptide-1 receptor in microglia attenuates neuroinflammation-induced glial scarring via rescuing Arf and Rho GAP adapter protein 3 expressions after nerve injury. Int J Biol Sci. (2022) 18:1328–46. doi: 10.7150/ijbs.68974
169. Quarta S, Mitrić M, Kalpachidou T, Mair N, Schiefermeier-Mach N, Andratsch M, et al. Impaired mechanical, heat, and cold nociception in a murine model of genetic TACE/ADAM17 knockdown. FASEB J. (2019) 33:4418–31. doi: 10.1096/fj.201801901R
170. Reda HM, Zaitone SA, Moustafa YM. Effect of levetiracetam versus gabapentin on peripheral neuropathy and sciatic degeneration in streptozotocin-diabetic mice: Influence on spinal microglia and astrocytes. Eur J Pharmacol. (2016) 771:162–72. doi: 10.1016/j.ejphar.2015.12.035
171. Rolls A, Shechter R, London A, Segev Y, Jacob-Hirsch J, Amariglio N, et al. Two faces of chondroitin sulfate proteoglycan in spinal cord repair: A role in microglia/macrophage activation. PloS Med. (2008) 5:1262–77. doi: 10.1371/journal.pmed.0050171
172. Rotterman TM, Akhter ET, Lane AR, MacPherson KP, García VV, Tansey MG, et al. Spinal motor circuit synaptic plasticity after peripheral nerve injury depends on microglia activation and a CCR2 mechanism. J Neurosci. (2019) 39:3412–33. doi: 10.1523/JNEUROSCI.2945-17.2019
173. Ru WJ, Liu X, Bae C, Shi YQ, Walikonis R, Chung JM, et al. Microglia mediate HIV-1 gp120-induced synaptic degeneration in spinal pain neural circuits. J Neurosci. (2019) 39:8408–21. doi: 10.1523/JNEUROSCI.2851-18.2019
174. Rueda-Carrasco J, Martin-Bermejo MJ, Pereyra G, Mateo MI, Borroto A, Brosseron F, et al. SFRP1 modulates astrocyte-to-microglia crosstalk in acute and chronic neuroinflammation. EMBO Rep. (2021) 22:17. doi: 10.15252/embr.202051696
175. Saika F, Matsuzaki S, Kobayashi D, Ideguchi Y, Nakamura TY, Kishioka S, et al. Chemogenetic regulation of CX3CR1-expressing microglia using Gi-DREADD exerts sex-dependent anti-allodynic effects in mouse models of neuropathic pain. Front Pharmacol. (2020) 11. doi: 10.3389/fphar.2020.00925
176. Sánchez-Ventura J, Amo-Aparicio J, Navarro X, Penas C. BET protein inhibition regulates cytokine production and promotes neuroprotection after spinal cord injury. J Neuroinflamm. (2019) 16. doi: 10.1186/s12974-019-1511-7
177. Santos Passos FR, Pereira EWM, Heimfarth L, Monteiro BS, Barbosa Gomes de Carvalho YM, Siqueira-Lima PS, et al. Role of peripheral and central sensitization in the anti-hyperalgesic effect of hecogenin acetate, an acetylated sapogenin, complexed with β-cyclodextrin: Involvement of NFκB and p38 MAPK pathways. Neuropharmacology. (2021) 186. doi: 10.1016/j.neuropharm.2020.108395
178. Santos-Nogueira E, Lopez-Serrano C, Hernandez J, Lago N, Astudillo AM, Balsinde J, et al. Activation of lysophosphatidic acid receptor type 1 contributes to pathophysiology of spinal cord injury. J Neurosci. (2015) 35:10224–35. doi: 10.1523/JNEUROSCI.4703-14.2015
179. Savarin C, Hinton DR, Valentin-Torres A, Chen ZH, Trapp BD, Bergmann CC, et al. Astrocyte response to IFN-γ limits IL-6-mediated microglia activation and progressive autoimmune encephalomyelitis. J Neuroinflammation. (2015) 12:14. doi: 10.1186/s12974-015-0293-9
180. Sawicki CM, Kim JK, Weber MD, Faw TD, McKim DB, Madalena KM, et al. Microglia Promote Increased Pain Behavior through Enhanced Inflammation in the Spinal Cord during Repeated Social Defeat Stress. J Neurosci. (2019) 39:1139–49. doi: 10.1523/JNEUROSCI.2785-18.2018
181. Sayo A, Konishi H, Kobayashi M, Kano K, Kobayashi H, Hibi H, et al. GPR34 in spinal microglia exacerbates neuropathic pain in mice. J Neuroinflamm. (2019) 16. doi: 10.1186/s12974-019-1458-8
182. Schampel A, Volovitch O, Koeniger T, Scholz CJ, Jörge S, Linker RA, et al. Nimodipine fosters remyelination in a mouse model of multiple sclerosis and induces microglia-specific apoptosis. Proc Natl Acad Sci United States America. (2017) 114:E3295–E304. doi: 10.1073/pnas.1620052114
183. Schonfeld E, Johnstone TM, Haider G, Shah A, Marianayagam NJ, Biswal S, et al. Sigma-1 receptor expression in a subpopulation of lumbar spinal cord microglia in response to peripheral nerve injury. Sci Rep. (2023) 13:8. doi: 10.1038/s41598-023-42063-8
184. Shao S, Xu CB, Chen CJ, Shi GN, Guo QL, Zhou Y, et al. Divanillyl sulfone suppresses NLRP3 inflammasome activation via inducing mitophagy to ameliorate chronic neuropathic pain in mice. J Neuroinflamm. (2021) 18. doi: 10.1186/s12974-021-02178-z
185. Shi CY, He XB, Zhao C, Wang HJ. Luteoloside exerts analgesic effect in a complete freund's adjuvant-induced inflammatory model via inhibiting interleukin-1β Expression and macrophage/microglia activation. Front Pharmacol. (2020) 11:10. doi: 10.3389/fphar.2020.01158
186. Shi XQ, Lim TKY, Lee S, Zhao YQ, Zhang J. Statins alleviate experimental nerve injury-induced neuropathic pain. Pain. (2011) 152:1033–43. doi: 10.1016/j.pain.2011.01.006
187. Sideris-Lampretsas G, Oggero S, Zeboudj L, Silva R, Bajpai A, Dharmalingam G, et al. Galectin-3 activates spinal microglia to induce inflammatory nociception in wild type but not in mice modelling Alzheimer's disease. Nat Commun. (2023) 14:19. doi: 10.1038/s41467-023-39077-1
188. Song WY, Ding H, Dunn T, Gao JL, Labastida J, Schlagal C, et al. Low-dose metformin treatment in the subacute phase improves the locomotor function of a mouse model of spinal cord injury. Neural Regeneration Res. (2021) 16:2234–42. doi: 10.4103/1673-5374.310695
189. Spiller KJ, Restrepo CR, Khan T, Dominique MA, Fang TC, Canter RG, et al. Microglia-mediated recovery from ALS-relevant motor neuron degeneration in a mouse model of TDP-43 proteinopathy. Nat Neurosci. (2018) 21:329–40. doi: 10.1038/s41593-018-0083-7
190. Staikopoulos V, Qiao S, Liu J, Song X, Yang X, Luo Q, et al. Graded peripheral nerve injury creates mechanical allodynia proportional to the progression and severity of microglial activity within the spinal cord of male mice. Brain Behavior Immunity. (2021) 91:568–77. doi: 10.1016/j.bbi.2020.11.018
191. Sun JJ, Tang L, Zhao XP, Xu JM, Xiao Y, Li H. Infiltration of blood-derived macrophages contributes to the development of diabetic neuropathy. J Immunol Res. (2019) 2019. doi: 10.1155/2019/7597382
192. Sun L, Li H, Tai LW, Gu P, Cheung CW. Adiponectin regulates thermal nociception in a mouse model of neuropathic pain. Br J Anaesthesia. (2018) 120:1356–67. doi: 10.1016/j.bja.2018.01.016
193. Sun S, Chen D, Lin F, Chen M, Yu H, Hou L, et al. Role of interleukin-4, the chemokine CCL3 and its receptor CCR5 in neuropathic pain. Mol Immunol. (2016) 77:184–92. doi: 10.1016/j.molimm.2016.08.006
194. Sun W, Wang QP, Zhang RY, Zhang N. Ketogenic diet attenuates neuroinflammation and induces conversion of M1 microglia to M2 in an EAE model of multiple sclerosis by regulating the NF-κB/NLRP3 pathway and inhibiting HDAC3 and P2X7R activation. Food Funct. (2023) 14:7247–69. doi: 10.1039/D3FO00122A
195. Suzuki N, Hasegawa-Moriyama M, Takahashi Y, Kamikubo Y, Sakurai T, Inada E. Lidocaine attenuates the development of diabetic-induced tactile allodynia by inhibiting microglial activation. Anesth Analgesia. (2011) 113:941–6. doi: 10.1213/ANE.0b013e31822827a2
196. Takamura H, Terashima T, Mori K, Katagi M, Okano J, Suzuki Y, et al. Bone-marrow-derived mononuclear cells relieve neuropathic pain after spinal nerve injury in mice. Mol Ther - Methods Clin Dev. (2020) 17:657–65. doi: 10.1016/j.omtm.2020.03.020
197. Takeura N, Nakajima H, Watanabe S, Honjoh K, Takahashi A, Matsumine A. Role of macrophages and activated microglia in neuropathic pain associated with chronic progressive spinal cord compression. Sci Rep. (2019) 9:12. doi: 10.1038/s41598-019-52234-1
198. Tan WL, Su PYP, Leff J, Gao X, Chen J, Guan AK, et al. Distinct phases of adult microglia proliferation: a Myc-mediated early phase and a Tnfaip3-mediated late phase. Cell Discovery. (2022) 8:34. doi: 10.1038/s41421-022-00377-3
199. Tanaka K, Okada Y, Kanno T, Otomo A, Yanagisawa Y, Shouguchi-Miyata J, et al. A dopamine receptor antagonist L-745,870 suppresses microglia activation in spinal cord and mitigates the progression in ALS model mice. Exp Neurology. (2008) 211:378–86. doi: 10.1016/j.expneurol.2008.02.004
200. Tansley S, Gu N, Guzmán AU, Cai WH, Wong C, Lister KC, et al. Microglia-mediated degradation of perineuronal nets promotes pain. Science. (2022) 377:80–6. doi: 10.1126/science.abl6773
201. Tansley S, Uttam S, Guzmán AU, Yaqubi M, Pacis A, Parisien M, et al. Single-cell RNA sequencing reveals time- and sex-specific responses of mouse spinal cord microglia to peripheral nerve injury and links ApoE to chronic pain. Nat Commun. (2022) 13:16. doi: 10.1038/s41467-022-28473-8
202. Tashima R, Mikuriya S, Tomiyama D, Shiratori-Hayashi M, Yamashita T, Kohro Y, et al. Bone marrow-derived cells in the population of spinal microglia after peripheral nerve injury. Sci Rep. (2016) 6:8. doi: 10.1038/srep23701
203. Tateda S, Kanno H, Ozawa H, Sekiguchi A, Yahata K, Yamaya S, et al. Rapamycin suppresses microglial activation and reduces the development of neuropathic pain after spinal cord injury. J Orthopaedic Res. (2017) 35:93–103. doi: 10.1002/jor.23328
204. Tawfik MK, Helmy SA, Badran DI, Zaitone SA. Neuroprotective effect of duloxetine in a mouse model of diabetic neuropathy: Role of glia suppressing mechanisms. Life Sci. (2018) 205:113–24. doi: 10.1016/j.lfs.2018.05.025
205. Teixeira-Santos L, Martins S, Sousa T, Teixeira AA, Pinho D. The pro-resolving lipid mediator Maresin 1 ameliorates pain responses and neuroinflammation in the spared nerve injury-induced neuropathic pain: A study in male and female mice. PloS One. (2023) 18. doi: 10.1371/journal.pone.0287392
206. Tenorio G, Kulkarni A, Kerr BJ. Resident glial cell activation in response to perispinal inflammation leads to acute changes in nociceptive sensitivity: Implications for the generation of neuropathic pain. Pain. (2013) 154:71–81. doi: 10.1016/j.pain.2012.09.008
207. Tonkin RS, Bowles C, Perera CJ, Keating BA, Makker PGS, Duffy SS, et al. Attenuation of mechanical pain hypersensitivity by treatment with Peptide5, a connexin-43 mimetic peptide, involves inhibition of NLRP3 inflammasome in nerve-injured mice. Exp Neurology. (2018) 300:1–12. doi: 10.1016/j.expneurol.2017.10.016
208. Toth CC, Jedrzejewski NM, Ellis CL, Frey Ii WH. Cannabinoid-mediated modulation of neuropathic pain and microglial accumulation in a model of murine type I diabetic peripheral neuropathic pain. Mol Pain. (2010) 6. doi: 10.1186/1744-8069-6-16
209. Tozaki-Saitoh H, Masuda J, Kawada R, Kojima C, Yoneda S, Masuda T, et al. Transcription factor MafB contributes to the activation of spinal microglia underlying neuropathic pain development. Glia. (2019) 67:729–40. doi: 10.1002/glia.23570
210. Tozaki-Saitoh H, Tsuda M, Miyata H, Ueda K, Kohsaka S, Inoue K. P2Y12 receptors in spinal microglia are required for neuropathic pain after peripheral nerve injury. J Neurosci. (2008) 28:4949–56. doi: 10.1523/JNEUROSCI.0323-08.2008
211. Tsuruta K, Shidara T, Miyagishi H, Nango H, Nakatani Y, Suzuki N, et al. Anti-inflammatory effects of miyako bidens pilosa in a mouse model of amyotrophic lateral sclerosis and lipopolysaccharide-stimulated BV-2 microglia. Int J Mol Sci. (2023) 24:14. doi: 10.3390/ijms241813698
212. Tu YS, Muley MM, Beggs S, Salter MW. Microglia-independent peripheral neuropathic pain in male and female mice. Pain. (2022) 163:E1129–E44. doi: 10.1097/j.pain.0000000000002643
213. Ueda H, Neyama H, Nagai J, Matsushita Y, Tsukahara T, Tsukahara R. Involvement of lysophosphatidic acid-induced astrocyte activation underlying the maintenance of partial sciatic nerve injury-induced neuropathic pain. Pain. (2018) 159:2170–8. doi: 10.1097/j.pain.0000000000001316
214. Ulmann L, Hatcher JP, Hughes JP, Chaumont S, Green PJ, Conquet F, et al. Up-regulation of P2X4 receptors in spinal microglia after peripheral nerve injury mediates BDNF release and neuropathic pain. J Neurosci. (2008) 28:11263–8. doi: 10.1523/JNEUROSCI.2308-08.2008
215. Umebayashi D, Natsume A, Takeuchi H, Hara M, Nishimura Y, Fukuyama R, et al. Blockade of gap junction hemichannel protects secondary spinal cord injury from activated microglia-mediated glutamate exitoneurotoxicity. J Neurotrauma. (2014) 31:1967–74. doi: 10.1089/neu.2013.3223
216. Vacca V, Marinelli S, Pieroni L, Urbani A, Luvisetto S, Pavone F. Higher pain perception and lack of recovery from neuropathic pain in females: A behavioural, immunohistochemical, and proteomic investigation on sex-related differences in mice. Pain. (2014) 155:388–402. doi: 10.1016/j.pain.2013.10.027
217. Wang L, Wu JP, He XJ. Butylphthalide has an Anti-Inflammatory Role in Spinal Cord Injury by Promoting Macrophage/Microglia M2 Polarization via p38 Phosphorylation. Spine. (2020) 45:E1066–E76. doi: 10.1097/BRS.0000000000003503
218. Wang LJ, Yin C, Xu XY, Liu TY, Wang B, Abdul MN, et al. Pellino1 contributes to morphine tolerance by microglia activation via MAPK signaling in the spinal cord of mice. Cell Mol Neurobiology. (2020) 40:1117–31. doi: 10.1007/s10571-020-00797-3
219. Wilkerson JL, Alberti LB, Kerwin AA, Ledent CA, Thakur GA, Makriyannis A, et al. Peripheral versus central mechanisms of the cannabinoid type 2 receptor agonist AM1710 in a mouse model of neuropathic pain. Brain Behav. (2020) 10. doi: 10.1002/brb3.v10.12
220. Wlaschin JJ, Gluski JM, Nguyen E, Silberberg H, Thompson JH, Chesler AT, et al. Dual leucine zipper Kinase is required for mechanical allodynia and microgliosis after nerve injury. eLife. (2018) 7. doi: 10.7554/eLife.33910
221. Wu J, Zhao Z, Zhu X, Renn CL, Dorsey SG, Faden AI. Cell cycle inhibition limits development and maintenance of neuropathic pain following spinal cord injury. Pain. (2016) 157:488–503. doi: 10.1097/j.pain.0000000000000393
222. Wu WF, Tan XJ, Dai YB, Krishnan V, Warner M, Gustafsson J. Targeting estrogen receptor β in microglia and T cells to treat experimental autoimmune encephalomyelitis. Proc Natl Acad Sci United States America. (2013) 110:3543–8. doi: 10.1073/pnas.1300313110
223. Xia LK, Qi JH, Tang MM, Liu J, Zhang D, Zhu YB, et al. Continual deletion of spinal microglia reforms astrocyte scar favoring axonal regeneration. Front Pharmacol. (2022) 13:10. doi: 10.3389/fphar.2022.881195
224. Xiao YC, Xu JW, Wang S, Mao CM, Jin M, Ning G, et al. Genetic ablation of steroid receptor coactivator-3 promotes PPAR-β-mediated alternative activation of microglia in experimental autoimmune encephalomyelitis. Glia. (2010) 58:932–42. doi: 10.1002/glia.20975
225. Xie WJ, Xie WQ, Jiang CC, Kang ZM, Liu NZ. Apigenin alleviates allodynia and hyperalgesia in a mouse model of chemotherapy-induced peripheral neuropathy via regulating microglia activation and polarization. J Integr Neurosci. (2023) 22:8. doi: 10.31083/j.jin2203064
226. Xu AH, Yang Y, Shao Y, Jiang MY, Sun YX. Poly(ADP-ribose) polymerase family member 14 promotes functional recovery after spinal cord injury through regulating microglia M1/M2 polarization via STAT1/6 pathway. Neural Regeneration Res. (2023) 18:1809–17. doi: 10.4103/1673-5374.357909
227. Xu AH, Yang Y, Shao Y, Jiang MY, Sun YX, Feng B. FHL2 regulates microglia M1/M2 polarization after spinal cord injury via PARP14-depended STAT1/6 pathway. Int Immunopharmacology. (2023) 124:16. doi: 10.1016/j.intimp.2023.110853
228. Xu C, Zheng H, Liu T, Zhang Y, Feng Y. Bioinformatics analysis identifies CSF1R as an essential gene mediating Neuropathic pain - Experimental research. Int J Surgery. (2021) 95. doi: 10.1016/j.ijsu.2021.106140
229. Xu S, Zhu W, Shao MH, Zhang F, Guo J, Xu HC, et al. Ecto-5′-nucleotidase (CD73) attenuates inflammation after spinal cord injury by promoting macrophages/microglia M2 polarization in mice. J Neuroinflammation. (2018) 15:14. doi: 10.1186/s12974-018-1183-8
230. Yaguchi M, Ohta S, Toyama Y, Kawakami Y, Toda M. Functional recovery after spinal cord injury in mice through activation of microglia and dendritic cells after IL-12 administration. J Neurosci Res. (2008) 86:1972–80. doi: 10.1002/jnr.21658
231. Yang L, Han XY, Xing FP, Wu H, Shi HL, Huang F, et al. Total flavonoids of astragalus attenuates experimental autoimmune encephalomyelitis by suppressing the activation and inflammatory responses of microglia via JNK/AKT/NFκB signaling pathway. Phytomedicine. (2021) 80:11. doi: 10.1016/j.phymed.2020.153385
232. Yang PP, Chueh SH, Shie HL, Chen CC, Lee LY, Chen WP, et al. Effects of hericium erinaceus mycelium extracts on the functional activity of purinoceptors and neuropathic pain in mice with L5 spinal nerve ligation. Evidence-Based Complementary Altern Med. (2020) 2020. doi: 10.1155/2020/2890194
233. Yang PP, Yeh GC, Huang EYK, Law PY, Loh HH, Tao PL. Effects of dextromethorphan and oxycodone on treatment of neuropathic pain in mice. J Biomed Sci. (2015) 22. doi: 10.1186/s12929-015-0186-3
234. Yao AH, Liu FF, Chen K, Tang L, Liu L, Zhang K, et al. Programmed death 1 deficiency induces the polarization of macrophages/microglia to the M1 phenotype after spinal cord injury in mice. Neurotherapeutics. (2014) 11:636–50. doi: 10.1007/s13311-013-0254-x
235. Yao Y, Echeverry S, Shi XQ, Yang M, Yang QZ, Wang GYF, et al. Dynamics of spinal microglia repopulation following an acute depletion. Sci Rep. (2016) 6:12. doi: 10.1038/srep22839
236. Yin JX, Tu JL, Lin HJ, Shi FD, Liu RL, Zhao CB, et al. Centrally administered pertussis toxin inhibits microglia migration to the spinal cord and prevents dissemination of disease in an EAE mouse model. PloS One. (2010) 5:14. doi: 10.1371/journal.pone.0012400
237. Yip PK, Bowes AL, Hall JCE, Burguillos MA, Ip THR, Baskerville T, et al. Docosahexaenoic acid reduces microglia phagocytic activity via miR-124 and induces neuroprotection in rodent models of spinal cord contusion injury. Hum Mol Genet. (2019) 28:2427–48. doi: 10.1093/hmg/ddz073
238. Yoshikawa M, Ishikawa C, Li H, Kudo T, Shiba D, Shirakawa M, et al. Comparing effects of microgravity and amyotrophic lateral sclerosis in the mouse ventral lumbar spinal cord. Mol Cell Neurosci. (2022) 121. doi: 10.1016/j.mcn.2022.103745
239. Yu L, Song HX, Fang XT, Hu YL. Role of MK2 signaling pathway mediating microglia/macrophages polarization in chronic compression injury of cervical spinal cord. Ann Palliative Med. (2021) 10:1304–12. doi: 10.21037/apm-20-396
240. Yu SS, Li ZY, Xu XZ, Yao F, Luo Y, Liu YC, et al. M1-type microglia can induce astrocytes to deposit chondroitin sulfate proteoglycan after spinal cord injury. Neural Regeneration Res. (2022) 17:1072–9. doi: 10.4103/1673-5374.324858
241. Yuan YM, Zhu F, Pu YY, Wang D, Huang AJ, Hu X, et al. Neuroprotective effects of nitidine against traumatic CNS injury via inhibiting microglia activation. Brain Behav Immunity. (2015) 48:287–300. doi: 10.1016/j.bbi.2015.04.008
242. Zajączkowska R, Pawlik K, Ciapała K, Piotrowska A, Ciechanowska A, Rojewska E, et al. Mirogabalin decreases pain-like behaviors by inhibiting the microglial/macrophage activation, p38MAPK signaling, and pronociceptive CCL2 and CCL5 release in a mouse model of neuropathic pain. Pharmaceuticals. (2023) 16. doi: 10.3390/ph16071023
243. Zajaczkowska R, Popiolek-Barczyk K, Pilat D, Rojewska E, Makuch W, Wordliczek J, et al. Involvement of microglial cells in the antinociceptive effects of metamizol in a mouse model of neuropathic pain. Pharmacology Biochem Behavior. (2018) 175:77–88. doi: 10.1016/j.pbb.2018.09.008
244. Zeng YZ, Wang NW, Guo TT, Zheng QY, Wang S, Wu SS, et al. Snx27 deletion promotes recovery from spinal cord injury by neuroprotection and reduces macrophage/microglia proliferation. Front Neurology. (2018) 9:11. doi: 10.3389/fneur.2018.01059
245. Zha Z, Gao YF, Ji J, Sun YQ, Li JL, Qi F, et al. Bu Shen Yi Sui Capsule Alleviates Neuroinflammation and Demyelination by Promoting Microglia toward M2 Polarization, Which Correlates with Changes in miR-124 and miR-155 in Experimental Autoimmune Encephalomyelitis. Oxid Med Cell Longevity. (2021) 2021:26. doi: 10.1155/2021/5521503
246. Zhang F, Vadakkan KI, Kim SS, Wu L-J, Shang Y, Zhuo M. Selective activation of microglia in spinal cord but not higher cortical regions following nerve injury in adult mouse. Mol Pain. (2008) 4:15. doi: 10.1186/1744-8069-4-15
247. Zhang G, Liu N, Zhu C, Ma L, Yang J, Du J, et al. Antinociceptive effect of isoorientin against neuropathic pain induced by the chronic constriction injury of the sciatic nerve in mice. Int Immunopharmacol. (2019) 75. doi: 10.1016/j.intimp.2019.105753
248. Zhang J, Shi XQ, Echeverry S, Mogil JS, De Koninck Y, Rivest S. Expression of CCR2 in both resident and bone marrow-derived microglia plays a critical role in neuropathic pain. J Neurosci. (2007) 27:12396–406. doi: 10.1523/JNEUROSCI.3016-07.2007
249. Zhang Q, Bian GL, Chen P, Liu L, Yu CY, Liu FF, et al. Aldose reductase regulates microglia/macrophages polarization through the cAMP response element-binding protein after spinal cord injury in mice. Mol Neurobiology. (2016) 53:662–76. doi: 10.1007/s12035-014-9035-8
250. Zhang R, Xu BA, Zhang N, Niu JD, Zhang MN, Zhang QQ, et al. Spinal microglia-derived TNF promotes the astrocytic JNK/CXCL1 pathway activation in a mouse model of burn pain. Brain Behav Immunity. (2022) 102:23–39. doi: 10.1016/j.bbi.2022.02.006
251. Zhang SH, Yu J, Lou GD, Tang YY, Wang RR, Hou WW, et al. Widespread pain sensitization after partial infraorbital nerve transection in MRL/MPJ mice. Pain. (2016) 157:740–9. doi: 10.1097/j.pain.0000000000000432
252. Zhang T, Sun K, Shen W, Qi L, Yin W, Wang L-W. SOCS1 regulates neuropathic pain by inhibiting neuronal sensitization and glial activation in mouse spinal cord. Brain Res Bulletin. (2016) 124:231–7. doi: 10.1016/j.brainresbull.2016.05.012
253. Zhang X, Xia L, Xie A, Liao O, Ju F, Zhou Y. Low concentration of Bupivacaine ameliorates painful diabetic neuropathy by mediating miR-23a/PDE4B axis in microglia. Eur J Pharmacol. (2021) 891. doi: 10.1016/j.ejphar.2020.173719
254. Zhang ZL, Wang ZT, Shi J, Pu XP, Zhai SD. Tetrandrine attenuates SNI-induced mechanical allodynia by inhibiting spinal CKLF1. Neuropharmacology. (2023) 238. doi: 10.1016/j.neuropharm.2023.109673
255. Zhang ZY, Sun XF, Zhao GQ, Ma YC, Zeng GL. LncRNA embryonic stem cells expressed 1 (Lncenc1) is identified as a novel regulator in neuropathic pain by interacting with EZH2 and downregulating the expression of Bai1 in mouse microglia. Exp Cell Res. (2021) 399:9. doi: 10.1016/j.yexcr.2020.112435
256. Zhao J, Gao QY. TMEM16F inhibition limits pain-associated behavior and improves motor function by promoting microglia M2 polarization in mice. Biochem Biophys Res Commun. (2019) 517:603–10. doi: 10.1016/j.bbrc.2019.07.070
257. Zhou F, Wang X, Han BY, Tang XH, Liu R, Ji Q, et al. Short-chain fatty acids contribute to neuropathic pain via regulating microglia activation and polarization. Mol Pain. (2021) 17:11. doi: 10.1177/1744806921996520
258. Zhou HJ, Wang LQ, Zhan RY, Zheng XJ, Zheng JS. lncRNA MEG3 restrained the M1 polarization of microglia in acute spinal cord injury through the HuR/A20/NF-κB axis. Brain Pathology. (2022) 32:16. doi: 10.1111/bpa.13070
259. Zhou LJ, Peng JY, Xu YN, Zeng WJ, Zhang J, Wei X, et al. Microglia are indispensable for synaptic plasticity in the spinal dorsal horn and chronic pain. Cell Rep. (2019) 27:3844–59. doi: 10.1016/j.celrep.2019.05.087
260. Zhou X, Tao L, Zhao M, Wu S, Obeng E, Wang D, et al. Wnt/β-catenin signaling regulates brain-derived neurotrophic factor release from spinal microglia to mediate HIV1 gp120-induced neuropathic pain. Mol Pain. (2020) 16. doi: 10.1177/1744806920922100
261. Zhu B, Gao J, Ouyang Y, Hu Z, Chen X. Overexpression of mir138 ameliorates spared sciatic nerve injury-induced neuropathic pain through the anti-inflammatory response in mice. J Pain Res. (2019) 12:3135–45. doi: 10.2147/JPR.S219462
262. Zhu MD, Zhao LX, Wang XT, Gao YJ, Zhang ZJ. Ligustilide inhibits microglia-mediated proinflammatory cytokines production and inflammatory pain. Brain Res Bulletin. (2014) 109:54–60. doi: 10.1016/j.brainresbull.2014.10.002
263. Zychowska M, Rojewska E, Piotrowska A, Kreiner G, Mika J. Microglial inhibition influences XCL1/XCR1 expression and causes analgesic effects in a mouse model of diabetic neuropathy. Anesthesiology. (2016) 125:573–89. doi: 10.1097/ALN.0000000000001219
264. Wijesekera LC, Leigh PN. Amyotrophic lateral sclerosis. Orphanet J Rare Dis. (2009) 4:3. doi: 10.1186/1750-1172-4-3
265. Covidence Systematic Review Software. Melbourne, Australia: Veritas Health Innovation. (2024). Available at: www.covidence.org.
266. Radulescu CI, Cerar V, Haslehurst P, Kopanitsa M, Barnes SJ. The aging mouse brain: cognition, connectivity and calcium. Cell Calcium. (2021) 94:102358. doi: 10.1016/j.ceca.2021.102358
267. Anand KS, DeMarco GJ, Dubner R, Green S, Hawk CT, Lascelles BDX. Recognition and Alleviation of Pain in Laboratory Animals. Washington, DC: National Research Council (US) Committee on Recognition and Alleviation of Pain in Laboratory Animals (2009).
268. Landis JR, Koch GG. The measurement of observer agreement for categorical data. Biometrics. (1977) 33:159–74. doi: 10.2307/2529310
269. Mogil JS. Animal models of pain: progress and challenges. Nat Rev Neurosci. (2009) 10:283–94. doi: 10.1038/nrn2606
270. Sadler KE, Mogil JS, Stucky CL. Innovations and advances in modelling and measuring pain in animals. Nat Rev Neurosci. (2022) 23:70–85. doi: 10.1038/s41583-021-00536-7
271. Tuttle AH, Philip VM, Chesler EJ, Mogil JS. Comparing phenotypic variation between inbred and outbred mice. Nat Methods. (2018) 15:994–6. doi: 10.1038/s41592-018-0224-7
272. Fox JG, Barthold S, Davisson M, Newcomer CE, Quimby FW, Smith A. “History, wild mice, and genetics”, In: Fox JG, Quimby FW, Barthold SW, Newcomer CE, Smith AL, editors. The Mouse in Biomedical Research, (2nd ed., Vol. 1). Cambridge, Massachusetts: American Press. (2006).
273. Osborne NR, Davis KD. “Chapter Eight - Sex and gender differences in pain”. In: Moro E, Arabia G, Tartaglia MC, Ferretti MT, editors. International Review of Neurobiology, vol. 164. Cambridge, Massachusetts: Academic Press (2022). p. 277–307.
274. Rahn EJ, Iannitti T, Donahue RR, Taylor BK. Sex differences in a mouse model of multiple sclerosis: neuropathic pain behavior in females but not males and protection from neurological deficits during proestrus. Biol Sex Differ. (2014) 5:4. doi: 10.1186/2042-6410-5-4
275. Mapplebeck JCS, Beggs S, Salter MW. Sex differences in pain: a tale of two immune cells. PAIN. (2016) 157. doi: 10.1097/j.pain.0000000000000389
276. Taves S, Berta T, Liu DL, Gan S, Chen G, Kim YH, et al. Spinal inhibition of p38 MAP kinase reduces inflammatory and neuropathic pain in male but not female mice: Sex-dependent microglial signaling in the spinal cord. Brain Behav Immun. (2016) 55:70–81. doi: 10.1016/j.bbi.2015.10.006
277. Jurga AM, Paleczna M, Kuter KZ. Overview of general and discriminating markers of differential microglia phenotypes. Front Cell Neurosci. (2020) 14. doi: 10.3389/fncel.2020.00198
278. Qin J, Ma Z, Chen X, Shu S. Microglia activation in central nervous system disorders: A review of recent mechanistic investigations and development efforts. Front Neurol. (2023) 14:1103416. doi: 10.3389/fneur.2023.1103416
279. VanDenKerkhof EG, Mann EG, Torrance N, Smith BH, Johnson A, Gilron I. An epidemiological study of neuropathic pain symptoms in Canadian adults. Pain Res Management. (2016) 2016:9815750. doi: 10.1155/2016/9815750
280. Deuis JR, Dvorakova LS, Vetter I. Methods used to evaluate pain behaviors in rodents. Front Mol Neurosci. (2017) 10:284. doi: 10.3389/fnmol.2017.00284
281. Kwon BK, Soril LJJ, Bacon M, Beattie MS, Blesch A, Bresnahan JC, et al. Demonstrating efficacy in preclinical studies of cellular therapies for spinal cord injury — how much is enough? Exp Neurol. (2013) 248:30–44. doi: 10.1016/j.expneurol.2013.05.012
282. Younger J, Mackey S. Fibromyalgia symptoms are reduced by low-dose naltrexone: A pilot study. Pain Med. (2009) 10:663–72. doi: 10.1111/j.1526-4637.2009.00613.x
283. Miwa K. Oral minocycline therapy improves symptoms of myalgic encephalomyelitis, especially in the initial disease stage. Internal Med. (2021) 60:2577–84. doi: 10.2169/internalmedicine.6082-20
284. Parkitny L, Younger J. Reduced pro-inflammatory cytokines after eight weeks of low-dose naltrexone for fibromyalgia. Biomedicines. (2017) 5:16. doi: 10.3390/biomedicines5020016
285. Mueller C, Ness TJ, Younger JW. Low-dose dextromethorphan for the treatment of fibromyalgia pain: Results from a longitudinal, single-blind, placebo-controlled pilot trial. J Pain Res. (2021) 14:189–200. doi: 10.2147/JPR.S285609
286. Ortega E, Bote ME, Giraldo E, García JJ. Aquatic exercise improves the Monocyte pro- and anti-inflammatory cytokine production balance in fibromyalgia patients. Scandinavian J Med Sci Sports. (2012) 22:104–12. doi: 10.1111/j.1600-0838.2010.01132.x
287. Shan L, Xu K, Ji L, Zeng Q, Liu Y, Wu Y, et al. Injured sensory neurons-derived galectin-3 contributes to neuropathic pain via programming microglia in the spinal dorsal horn. Brain Behavior Immunity. (2024) 117:80–99. doi: 10.1016/j.bbi.2024.01.002
288. Kong E, Hua T, Li J, Li Y, Yang M, Ding R, et al. HSV-1 reactivation results in post-herpetic neuralgia by upregulating Prmt6 and inhibiting CGAS-sting. Brain. (2024) 147:2552–65. doi: 10.1093/brain/awae053
289. Lu Y, Liu S, Wang P, Guo X, Qin Z, Hou H, et al. A novel microglia-targeting strategy based on nanoparticle-mediated delivery of Mir-26a-5p for long-lasting analgesia in chronic pain. J Nanobiotechnology. (2024) 22. doi: 10.1186/s12951-024-02420-9
290. Fiore NT, Hayes JP, Williams SI, Moalem-Taylor G. Interleukin-35 alleviates neuropathic pain and induces an anti-inflammatory shift in spinal microglia in nerve-injured male mice. Brain Behavior Immunity. (2024) 122:287–300. doi: 10.1016/j.bbi.2024.07.043
291. Silveira Prudente A, Hoon Lee S, Roh J, Luckemeyer DD, Cohen CF, Pertin M, et al. Microglial Sting activation alleviates nerve injury-induced neuropathic pain in male but not female mice. Brain Behavior Immunity. (2024) 117:51–65. doi: 10.1016/j.bbi.2024.01.003
292. Hua T, Kong E, Zhang H, Lu J, Huang K, Ding R, et al. PRMT6 deficiency or inhibition alleviates neuropathic pain by decreasing glycolysis and inflammation in microglia. Brain Behavior Immunity. (2024) 118:101–14. doi: 10.1016/j.bbi.2024.02.027
293. Ghasemi A, Zahediasl S. Normality tests for statistical analysis: a guide for non-statisticians. Int J Endocrinol Metab. (2012) 10:486–9. doi: 10.5812/ijem.3505
Keywords: microglia, neuropathic pain (NP), mouse model, spinal cord, temporal changes
Citation: Dhir S, Derue H and Ribeiro-da-Silva A (2024) Temporal changes of spinal microglia in murine models of neuropathic pain: a scoping review. Front. Immunol. 15:1460072. doi: 10.3389/fimmu.2024.1460072
Received: 05 July 2024; Accepted: 18 November 2024;
Published: 06 December 2024.
Edited by:
Wulin Tan, The First Affiliated Hospital of Sun Yat-sen University, ChinaReviewed by:
Giovane Galdino, Federal University of Alfenas, BrazilCopyright © 2024 Dhir, Derue and Ribeiro-da-Silva. This is an open-access article distributed under the terms of the Creative Commons Attribution License (CC BY). The use, distribution or reproduction in other forums is permitted, provided the original author(s) and the copyright owner(s) are credited and that the original publication in this journal is cited, in accordance with accepted academic practice. No use, distribution or reproduction is permitted which does not comply with these terms.
*Correspondence: Alfredo Ribeiro-da-Silva, YWxmcmVkby5yaWJlaXJvZGFzaWx2YUBtY2dpbGwuY2E=
Disclaimer: All claims expressed in this article are solely those of the authors and do not necessarily represent those of their affiliated organizations, or those of the publisher, the editors and the reviewers. Any product that may be evaluated in this article or claim that may be made by its manufacturer is not guaranteed or endorsed by the publisher.
Research integrity at Frontiers
Learn more about the work of our research integrity team to safeguard the quality of each article we publish.