- 1School of Pharmacy, Changchun University of Chinese Medicine, Changchun, Jilin, China
- 2The First Hospital of Jilin University, Changchun, China
Hepatocellular carcinoma (HCC) is one of the most common primary neoplasms of the liver and one of the most common solid tumors in the world. Its global incidence is increasing and it has become the third leading cause of cancer-related deaths. There is growing evidence that chemokines play an important role in the tumor microenvironment, regulating the migration and localization of immune cells in tissues and are critical for the function of the immune system. This review comprehensively analyses the expression and activity of chemokines in the TME of HCC and describes their interrelationship with hepatocarcinogenesis and progression. Special attention is given to the role of chemokine-chemokine receptors in the regulation of immune cell accumulation in the TME. Therapeutic strategies targeting tumor-promoting chemokines or the induction/release of beneficial chemokines are reviewed, highlighting the potential value of natural products in modulating chemokines and their receptors in the treatment of HCC. The in-depth discussion in this paper provides a theoretical basis for the treatment of HCC. It is an important reference for new drug development and clinical research.
1 Introduction
Hepatocellular carcinoma (HCC) is one of the most common primary neoplasms of the liver and one of the most common solid tumors in the world (1). More so than other cancers, chronic inflammation is a hallmark of HCC, with 90% of diagnoses occurring in the context of chronic liver disease (2). The incidence of HCC is on the rise worldwide and has become the third most common cause of cancer-related deaths (3). The main risk factors are infection with the hepatitis B virus (HBV) or the hepatitis C virus (HCV) (4), metabolic-associated fatty liver disease (MAFLD) (5), smoking (6), obesity and type 2 diabetes mellitus(T2DM) (7), etc. Inflammation is a major contributor to the pathogenesis of HCC (8).
The tumor microenvironment (TME) is a key determinant of tumor growth and consists of tumor cells, numerous immune cells, vascular and stromal cells, etc (9). Chemokines, vital cytokines capable of altering the TME, constitute the largest subset of cell factors (10). During inflammatory responses, chemokines are regarded as primary drivers for immune cell infiltration into the liver, including macrophages, neutrophils, and other cells (11). To date, more than 50 chemokines have been identified in humans and mice. Chemokines are categorized into families according to the structural motifs of their N-terminal cysteines: C-C motif chemokine ligands (CC), C-X-C motif chemokine ligands (CXC), X-C motif chemokine ligands (XC), and CX3C motif chemokine ligands (CX3C) (12), where C represents cysteine and X denotes any amino acid residue (13). These chemotactic factors signal through seven-transmembrane chemokine receptors coupled with cell surface G-proteins, thereby stimulating directed cell migration (14). Chemokine receptors are classified into four subfamilies based on their binding to different ligands: CC Chemokine Receptors (CCR), CXC chemokine receptors (CXCR), XC chemokine receptors (XCR), and CX3C chemokine receptors (CX3CR) (15). Constitutive chemokines are expressed under physiological conditions and play roles in cell migration and homing (16), while inflammatory chemokines are rapidly secreted at sites of inflammation to recruit effector cells to inflamed tissues (17). In TME, tumor cells and immune cells express multiple chemokine receptors on their surfaces. This diversity leads to differential responses to chemokines, which in turn affects their migration and function, regulating the tumor immune response, as well as tumor progression and prognosis, significantly influencing tumor therapy (18). A substantial body of research substantiates that CC chemokines (e.g., CCL2, CCL5) and CXC chemokines (e.g., CXCL1, CXCL2, CXCL5) recruit diverse immune cells, such as CCR2+ monocytes and CXCR2+ neutrophils, to tumor sites. These cells subsequently differentiate into tumor-associated macrophages (TAMs) and tumor-associated neutrophils (TANs), thereby exerting either promotive or inhibitory effects on tumorigenesis (19, 20). For instance, studies in HCC have demonstrated that the deletion of CCL5 enhances CXCL1 expression in neutrophils. This upregulation activates the CXCL1-CXCR2 axis, promoting neutrophil infiltration into the liver and consequently exacerbating inflammatory liver damage (21). CCL15 recruits CCR1+CD14+ monocytes to the invasive front of HCC, thereby suppressing antitumor immune responses, promoting angiogenesis, and accelerating the metastasis of HCC cells. Notably, blockade of the CCL15-CCR1 axis has been shown to reduce the proliferation and migration of HCC cells in vivo (22). In contrast, Brandt et al. elucidated that the chemokine receptor CXCR3 orchestrates the polarization of TAMs, effectively constraining tumor growth and angiogenesis in murine hepatocellular carcinoma (HCC) (23). In conclusion, chemokines and their receptors are promising biomarkers and therapeutic targets for the diagnosis and immunotherapy of hepatocellular carcinoma, and an in-depth understanding of the regulatory mechanisms of chemokines is essential for the development of novel therapeutic strategies (24).
Currently, natural products has been demonstrated to play a significant role in the prevention of HCC, as well as in the control of its metastasis and recurrence (25). These natural products modulate chemokine expression and function, as well as the interaction between chemokines and their receptors, to influence the immune system and microenvironment in the body, thereby suppressing the progression of HCC (26). Reportedly, the natural product 747 from Abies georgei exhibits sensitivity and selectivity as a CCR2 antagonist in HCC mouse models. It effectively blocks tumor-infiltrating macrophage-mediated immune suppression, increases the number of CD8+ T cells within tumors, and suppresses the growth of both in situ and subcutaneous tumors in a CD8+ T cell-dependent manner (27). Triptolide, derived from Tripterygium wilfordii Hook.f. extract, is a monomeric compound renowned for its diverse pharmacological properties. It functions as an inhibitor of the miR-532-5p/CXCL2 axis, effectively suppressing the migration of HCC cells and inhibiting the initiation and metastasis of HCC tumors (28). Aconite alkaloid extract (AAE) induces the upregulation of CCL2 via activation of c-Jun N-terminal kinase (JNK), thereby enhancing natural killer (NK) cell infiltration to suppress the growth of HCC (29). These findings suggest that leveraging natural products to modulate chemokine actions and adjust the tumor microenvironment composition can bolster immune responses in patients, presenting a promising and clinically valuable therapeutic strategy for HCC (30). This article provides a comprehensive review of the effects of metabolites derived from natural products on the regulation of chemokines and their receptors in the HCC tumor microenvironment, revealing how natural products regulate chemokines through specific molecular pathways, thereby affecting the tumor microenvironment and the development of HCC. We summarizes the research progress of plant metabolites in the treatment of hepatocellular carcinoma by affecting chemokines and discusses their potential developmental trends and shortcomings.
2 Tumor microenvironment and chemokines
TME constitutes a complex ecosystem comprising tumor cells, immune cells, stromal cells, and vascular networks. Beyond supporting tumor cell growth, the TME plays a pivotal role in tumor immune evasion and drug resistance (10). TME exhibits dynamic changes, wherein the distribution and functional status of immune cells evolve continuously with tumor progression (31). Chemokines are a class of cytokines that direct immune cell migration by binding to specific receptors. Within the TME, chemokines not only govern the recruitment and directional migration of immune cells but also impact their activation states, thus playing pivotal roles in tumor immune surveillance and evasion (32).
2.1 Chemokines and tumor-associated macrophages
TAMs constitute a critical component of the immune microenvironment in HCC (33), primarily recruited and polarized into an M2 phenotype by various cytokines (34, 35). It is widely accepted that TAMs originate from peripheral blood monocytes (PBMCs) and are recruited to the tumor vicinity by chemokines secreted within the tumor microenvironment, such as CCL2. Once within the tumor microenvironment, monocytes undergo polarization into M2-like macrophages (36). These macrophages secrete anti-inflammatory cytokines, promote angiogenesis, exert anti-inflammatory effects, and contribute to stromal remodeling. This polarization process leads to apoptosis of CD8+ T lymphocytes, suppression of Th1-type immune responses, reshaping of the microenvironment, alteration of immune homeostasis, and ultimately facilitates tumor growth, invasion, and metastasis (37). Concurrently, TAMs stimulate the secretion of CXCL1, promoting the polarization of M2 macrophages and thereby influencing the migration and invasion of HCC cells (38). Targeting the CCL2/CCR2 axis and CXCL1/CXCR2 blockade therapy can inhibit the recruitment and M2 polarization of inflammatory monocytes/infiltrative TAMs, disrupting immune suppression within the TME (39). Consequently, reshaping the immunosuppressive TME and repolarizing TAMs from an M2 to an anti-tumor phenotype emerges as a promising cancer immunotherapy strategy. This approach can activate anti-tumor immune responses, enhance the efficacy of cancer immunotherapy, and thereby restrict tumor progression (40).
2.2 Chemokines and tumor-associated neutrophils
Within the TME, neutrophils undergo moderate activation to become TANs, releasing reactive oxygen species (ROS), neutrophil elastase (NE), and other bioactive substances that promote tumor growth and invasion (41). TANs display two distinct phenotypes categorized as the anti-tumor N1 and pro-tumor N2 phenotypes (42, 43). Research has revealed that TANs enhance stem cell-like characteristics in HCC through activation of the miR-301b-3p/LSAMP/CYLD pathway. These stem cell-like HCC cells secrete the chemokine CXCL5, which in turn recruits intratumoral TANs, thereby facilitating the invasion and metastasis of HCC (44). Xu et al. explored the role of CCL21 in the TME of HCC using in vitro and in vivo HCC subcutaneous tumor models with neutrophils. Their findings indicate that CCL21 inhibits the polarization of N2 neutrophils by suppressing the activation of the nuclear factor κB (NF-κB) pathway (45). In the future, targeting the recruitment, migration, or activation of neutrophils may offer promising avenues for anti-tumor therapy.
2.3 Chemokines and myeloid-derived suppressor cells
Myeloid-derived suppressor cells (MDSCs) are highly heterogeneous suppressive immune cells distributed across the bone marrow, spleen, peripheral blood, and tumor tissues (46). MDSCs can be categorized into two subsets: monocytic MDSCs (M-MDSCs) and polymorphonuclear or granulocytic MDSCs (PMN-MDSCs) (47). In HCC, reduced RIP3 expression has been observed in patients, validating its capacity to upregulate CXCL1 expression within HCC cells. This upregulation promotes the recruitment of MDSCs through the CXCL1-CXCR2 axis, thereby fostering the progression of HCC. Targeting the CXCL1-CXCR2 chemotactic pathway presents a potential immunotherapeutic strategy to impede the advancement of RIP3-deficient HCC (48).In H22 orthotopic HCC mice, PMN-MDSCs exhibit increased prevalence compared to M-MDSCs, predominating in the spleen, peripheral blood, and tumor tissue. CCL9 and CCL2 play a role in mobilizing PMN-MDSCs from the spleen to peripheral blood, thereby facilitating tumor initiation and growth (49). Hence, therapeutic strategies aimed at modulating MDSCs accumulation and activation hold promise as treatments for malignant liver diseases.
2.4 Chemokines and cancer-associated fibroblasts
One of the hallmark features of HCC is liver fibrosis, observed in approximately 80% to 90% of clinical cases (50). The development of fibrosis often involves the infiltration of immune cells and stromal cells, including hepatic stellate cells (HSCs), a significant source of cancer-associated fibroblasts (CAFs) (51). Luo et al. demonstrated that CAFs promote HCC stem-like characteristics by inducing the expression of Forkhead box Q1 (FOXQ1) in HCC cells and activating N-myc downstream-regulated gene 1 (NDRG1). Furthermore, activated HCC cells can secrete chemokine ligand 26 (CCL26) to recruit additional CAFs, thereby driving HCC progression (52). In HCC, CAFs secrete higher levels of chemokines, including CCL2, CCL5, CCL7, and CXCL16, compared to neighboring fibroblasts. These chemokines play pivotal roles in recruiting immune cells, thus facilitating HCC metastasis and invasion (53). This body of evidence underscores the involvement of CAFs in immune suppression through the modulation of interactions among diverse immune cell types via chemokine signaling.
2.5 Chemokines and tumor-infiltrating lymphocytes
Tumor-infiltrating lymphocytes (TILs) are a pivotal component of the HCC microenvironment, encompassing regulatory T cells (Tregs), NK cells, cytotoxic T lymphocytes (CTLs), and B cells (54). Tregs are a subset of immune cells known for their potent immunosuppressive effects (55). Studies have reported a significant increase in CD4+ CD25+ Tregs in the peritumoral regions of HCC, with this increase being correlated with tumor size (56). TAMs secrete the chemokine CCL22, which recruits T cells to the tumor site, thereby enhancing the accumulation of Tregs and fostering an immunosuppressive microenvironment that impedes cytotoxic T cell activation (57). NK cells mediate innate immune responses and possess the ability to directly exert cytotoxic effects without prior sensitization. However, their functionality is often compromised within the tumor microenvironment (58).Recent studies have elucidated that miR-561-5p, which is upregulated in HCC, directly targets and downregulates CX3CL1 expression, thereby inhibiting the chemotactic migration of CX3CR1+ NK cells, promoting tumor cell survival, and facilitating pulmonary metastasis (59).
In conclusion, chemokines play a pivotal role in the TME, orchestrating the recruitment, migration, and activation of immune cells by binding to specific receptors. This regulatory process significantly impacts tumor progression and immune evasion mechanisms. In HCC, chemokines not only drive the polarization and functionality of TAMs, TANs, MDSCs, and CAFs, but also influence the distribution and activity of TILs. Collectively, these roles contribute to the establishment of an immunosuppressive TME that supports tumor growth, invasion, and metastasis.
By modulating chemokines and their associated signaling pathways, the immunosuppressive TME can be reshaped to activate antitumor immune responses. Growing evidence indicates that natural products has unique potential in regulating chemokines and their signaling pathways. Modulating the TME through natural products can effectively inhibit tumor progression. Numerous studies have investigated the mechanisms by which these active components of natural products exert their effects in HCC, thereby advancing the development of immunotherapy for this malignancy (Figure 1).
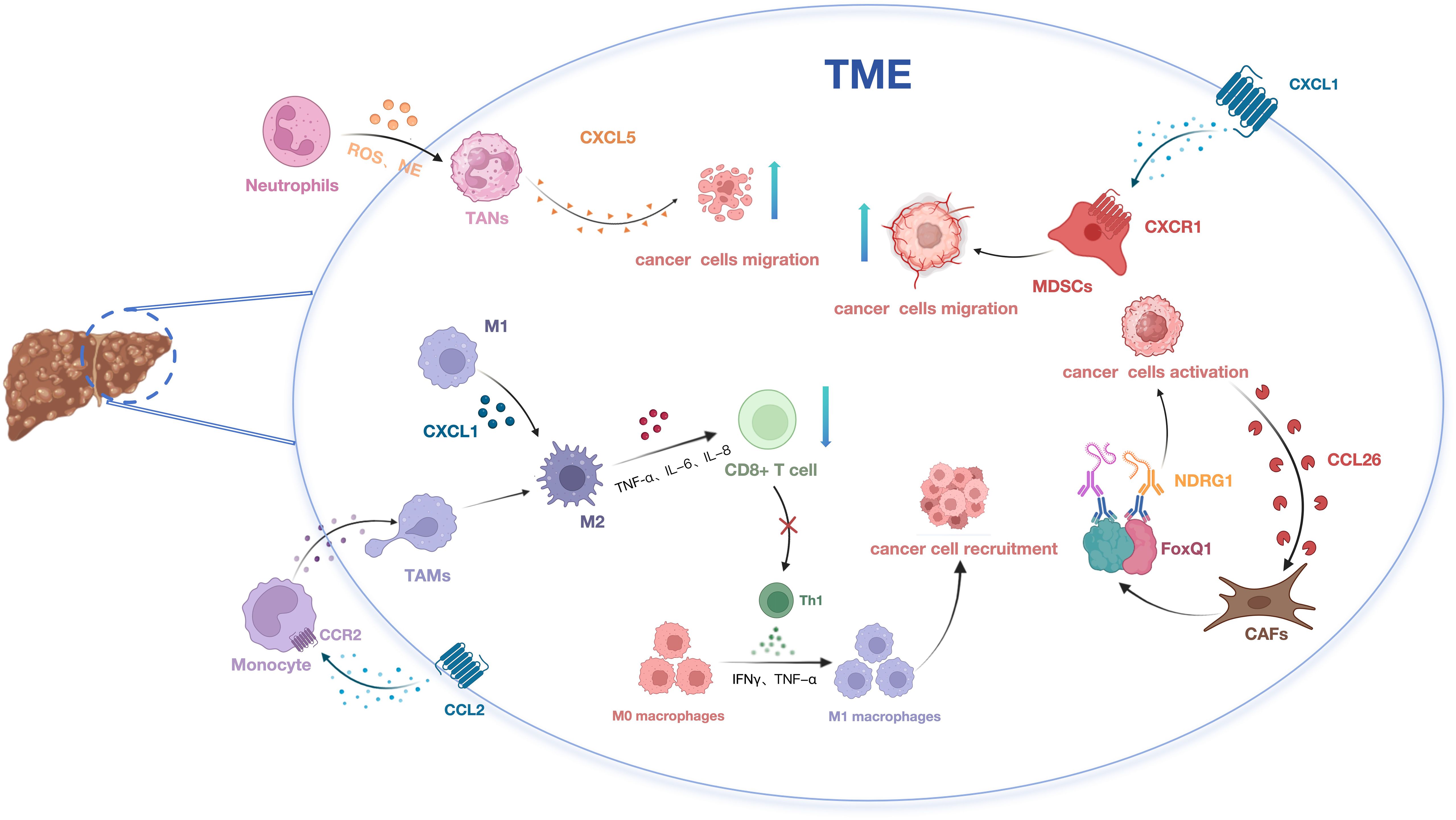
Figure 1. The impact of chemokines on HCC development via modulation of immune cell migration and recruitment.
3 Role of natural products and their extracts in hepatocellular carcinoma
Natural products are extensively utilized in cancer treatment due to their inherent characteristics. Unlike conventional drugs that target specific symptoms or diseases, natural products often regulate overall bodily functions (60). Compared to chemical drugs, natural products tend to have fewer side effects, which is particularly advantageous for HCC patients with impaired liver function and low drug tolerance. Furthermore, natural products possess unique potential and mechanisms for regulating chemokines to mediate immune cell activity and treat HCC (61). Various natural components, such as phenolics, flavonoids, terpenes, and alkaloids, exhibit notable antitumor activity (Table 1).
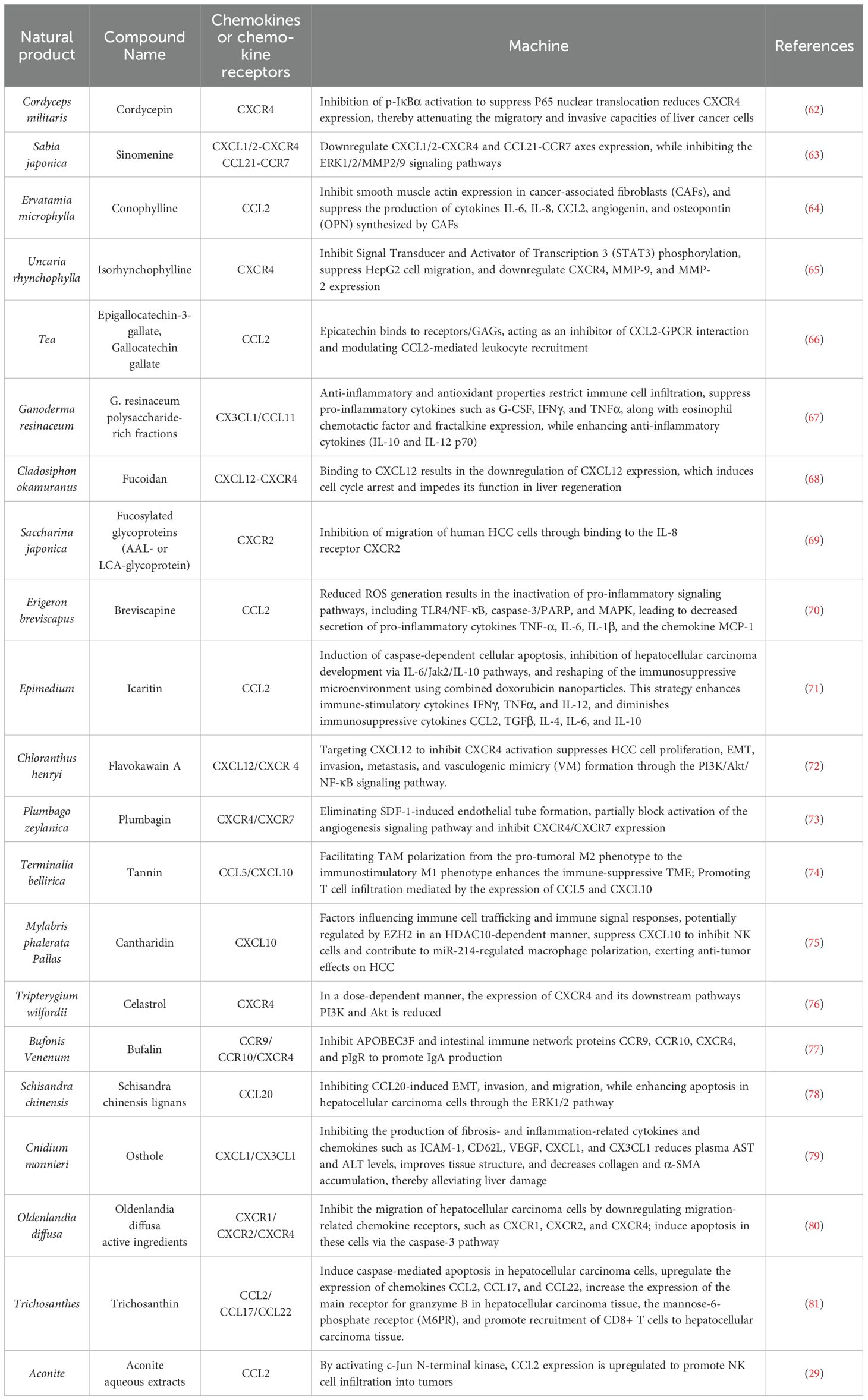
Table 1. Mechanism of natural products and their extracts in the treatment of HCC through the chemokines.
3.1 Alkaloids
Alkaloids, a class of natural compounds extracted from natural products, have been shown to possess significant anti-hepatocellular carcinoma effects (82). Current research indicates that alkaloids exert their anticancer effects by reducing cancer cell migration and invasion through mechanisms such as downregulating the expression of CXCR4, MMP-9, and MMP-2, or inhibiting the CXCL12-CXCR4 and CCL21-CCR7 axes.
3.1.1 Cordycepin
Cordyceps sinensis (BerK.) Sacc. exhibits potent anti-hepatocellular carcinoma properties and enhances immune function (83). Key components such as cordycepin, cordyceps polysaccharides, and cordyceps peptides possess anti-inflammatory, antitumor, antiviral, and immunomodulatory effects (84). These components exert anticancer effects by interfering with cancer cell signal transduction, regulating the cell cycle, and modulating the expression of apoptosis-related proteins. Guo’s research investigated the mechanisms by which cordycepin inhibits HepG2 and Huh7 liver cancer cells using migration, invasion, and chemotaxis assays. They found that the invasion and migration rates of cells treated with 10 µM cordycepin were significantly lower than those of the control group, with a concomitant downregulation of CXCR4 expression. Consequently, the migration and invasion abilities of liver cancer cells were impaired, indicating a significant inhibitory effect of cordycepin on liver cancer. Furthermore, the inhibitory effect of cordycepin was markedly enhanced when used in combination with JSH-23, suggesting that cordycepin may have potential to prevent liver cancer metastasis when combined with other therapeutic compounds (62). Li et al. treated HepG2 cells with various concentrations of cordycepin and doxorubicin, either alone or in combination, for 48 hours. Both cordycepin and doxorubicin downregulated CCL2 expression in a dose-dependent manner, effectively inhibiting HCC cell proliferation and migration, and inducing apoptosis. The combined treatment showed more pronounced effects (85).
3.1.2 Sinomenine
Sinomenine is an alkaloid compound primarily extracted from the roots and stems of the Menispermaceae plant, Sabia japonica Maxim., commonly used in its hydrochloride salt form (86). It exhibits a broad range of pharmacological activities, including antitumor, anti-inflammatory, neuroprotective, and immunosuppressive properties, making it highly effective against HCC (87). Shen et al. investigated the inhibitory effects of sinomenine hydrochloride on the growth, invasion, and metastasis of mouse liver cancer cells (Hepa1-6). They analyzed its impact on the expression of apoptosis-related and metastasis/invasion-related genes at the mRNA level. The study found that sinomenine hydrochloride inhibits the CXCL12-CXCR4 and CCL21-CCR7 axes in HCC cells, thereby suppressing HCC cell growth and invasion while promoting apoptosis (63).
3.1.3 Conophylline
Conophylline (CnP), a vinca alkaloid derived from the tropical plant Ervatamia microphylla Pit., has shown promising therapeutic potential for liver disease (88). Utilizing a primary CAFs model established from surgically resected HCC tissues, it was revealed that CnP effectively inhibits the activation of rat HSCs. Further investigations demonstrated that CnP primarily exerts its anti-HCC effects by suppressing the activation of CAFs and reducing the production of pro-tumorigenic factors, particularly interleukin-6 (IL-6), interleukin-8 (IL-8), CCL2, angiopoietin, and osteopontin (OPN) (64).
3.1.4 Isorhynchophylline
Isorhynchophylline (Rhy), a major oxindole alkaloid isolated from Uncaria rhynchophylla (Miq.) Miq. ex Havil., has demonstrated notable anticancer properties. Lee et al. employed HepG2 cells to elucidate the detailed mechanisms underlying Rhy’s anticancer effects. Utilizing Annexin V assay, scratch assay, and Transwell assay, their findings revealed that Rhy inhibits HepG2 cell migration, invasion, and the constitutive expression of CXCR4. Furthermore, the study showed that Rhy modulates multiple cellular signaling pathways by inhibiting the phosphorylation of p38, ERK, JNK, CREB, c-Jun, Akt, and STAT3, while enhancing the phosphorylation of p53 at the Ser15 residue. These actions collectively induce apoptosis and exert antimetastatic effects in HepG2 cells (65).
3.2 Phenols
Phenolic compounds, a class of organic molecules featuring one or more hydroxyl groups (-OH) directly attached to an aromatic ring, are abundant in various natural products, including Scutellaria baicalensis Georgi, Salvia miltiorrhiza Bunge, and catechins. The CCL2/CCR2 axis has been implicated in HCC by promoting the recruitment of monocytes/macrophages. Deepak et al. conducted a systematic investigation into the inhibitory mechanisms of epigallocatechin-3-gallate (EGCG) and gallocatechin gallate (GCG) in HCC. Their findings demonstrated that both EGCG and GCG inhibit CCL2 chemokine-mediated monocyte recruitment. Notably, EGCG reduces CCL2-induced macrophage migration, whereas GCG functions as an effective protein-protein interaction (PPI) inhibitor, regulating CCL2-directed leukocyte recruitment to ameliorate inflammation and immune dysregulation (66).
3.3 Polysaccharide
Polysaccharides are polymeric carbohydrates consisting of ten or more monosaccharide units linked by glycosidic bonds. They are prevalent in animals, plants, microorganisms, and other organisms. Owing to their low toxicity and minimal side effects, polysaccharides have found extensive applications in the food and pharmaceutical industries (89). Research has demonstrated their antitumor effects through mechanisms including the regulation of the CXCR4/CXCL12 axis, downregulation of CXCL12 expression, and reduction in the production of eosinophil chemotactic factors (90).
3.3.1 Ganoderma resinaceum polysaccharide
Ganoderma resinaceum polysaccharide-rich fractions (GRP) exhibits chemopreventive properties by inhibiting cell proliferation, promoting liver structural restoration, enhancing antioxidant enzymes, and modulating cytokine/chemokine levels (67). Data from the treatment groups reveal that GRP II effectively reduces immune cell infiltration into subcutaneous tissues, suppresses the expression of pro-inflammatory cytokines Interferon-γ (IFN-γ), tumor necrosis factor-α (TNF-α) and chemokines (eosinophil chemotactic factor, fractalkine), and increases levels of anti-inflammatory cytokines (IL-10 and IL-12p70) in Wistar rats with N-nitrosodiethylamine-induced HCC, highlighting its potent in vivo anti-inflammatory activity (67).
3.3.2 Fucoidan
Fucoidan, also known as brown algae polysaccharide, is a natural active polysaccharide uniquely found in brown algae and containing sulfate groups (91). It primarily resides in the cell wall matrix, intercellular spaces, and secreted mucilage of seaweeds such as kelp, wakame, giant kelp, and bladderwrack. Fucoidan exhibits essential functions including antimicrobial, moisturizing, and radioprotective effects on algae themselves (92). Recent research has extensively documented multiple physiological benefits of fucoidan, including enhancement of gastrointestinal health, anti-tumor properties, treatment of chronic kidney failure, immune modulation, and anticoagulant activity. Studies have demonstrated that fucoidan modulates the CXCL12/CXCR4 axis, exerting a dose-dependent inhibitory effect on Huh7 liver cancer cells by reducing CXCL12 expression (68). Fucosylated AAL-polysaccharides and LCA-glycoproteins similarly significantly decrease IL-8-induced migration of HCC cells. This effect may be attributed to their capacity to bind IL-8 receptors, particularly CXCR2, which likely retains sugar residues linked with Fuc α1-2 and/or Galβ1-4 (Fucα1-3) GlcNAc. This interaction competitively inhibits IL-8 binding to CXCR2, thereby suppressing the migration of human liver cancer cells (69).
3.4 Flavonoids
Flavonoids, secondary metabolites naturally found in plants, demonstrate diverse biological activities (93). In anticancer research, several flavonoids have been identified to modulate the expression or activity of chemokines and their receptors to exert their effects.
3.4.1 Breviscapine
Breviscapine, a flavonoid isolated from the traditional Chinese herb Erigeron breviscapus (Vant.) Hand.-Mazz., is renowned for its diverse pharmacological effects, including anti-inflammatory, antioxidant, anti-apoptotic, vasodilatory, antiplatelet, and anticoagulant properties. Recent studies have demonstrated that breviscapine protects against CCl4-induced liver injury by reducing the secretion of pro-inflammatory cytokines and oxidative stress. Specifically, breviscapine attenuates the secretion of TNF-α, IL-6, IL-1β, and the chemokine monocytechemoattractantprotein-1 (MCP-1) in serum, as well as their expression in liver tissues (70).
3.4.2 Icaritin
Icaritin (ICT), an active flavonoid compound derived from Epimedium Linn., has demonstrated clinical efficacy in extending the survival of HCC patients through immune modulation (94). Several studies have shown that ICT induces autophagy and apoptosis in cancer cells while enhancing the anti-tumor effects of doxorubicin. However, the precise mechanisms underlying these effects remain to be elucidated. Yu et al. first reported that ICT inhibits the proliferation of Hepa1-6 and Huh7 liver cancer cells by promoting caspase-mediated mitochondrial apoptosis. Furthermore, they demonstrated that the combination of ICT and doxorubicin synergistically reduces the immunosuppressive functions of MDSCs, Treg cells, and M2 macrophages, leading to decreased release of immunosuppressive cytokines, including chemokine CCL2, transforming growth factor β (TGF-β), IL-4, IL-6, and IL-10 (95). Additional research has revealed that ICT significantly blocks the immunosuppressive activity of bone marrow cells, modulates immunosuppressive MDSC cells and inflammation-associated cytokines and chemokines, and inhibits the expression of programmed death ligand-1 (PD-L1), thereby enhancing the efficacy of immunotherapy and anti-cancer treatments (71).
3.4.3 Flavokawain A
Xiao’s research isolated a chalcone compound, flavokawain A (FKA), from Chloranthus henryi Hemsl. Utilizing SMMC-7721, Huh7, PANC-1, HepG2, HeLa, and Hep3B cell lines, they conducted Transwell invasion assays to investigate cell invasiveness. Their findings indicate that FKA inhibits the migration, invasion, VM formation, and EMT progression of HCC cells by targeting CXCL12, thereby suppressing the PI3K/Akt/HIF-1α/Twist1 pathway (72).
3.5 Plumbagin
Zhong et al. utilized SDF-1 to induce proliferation, invasion, and growth factor activation in the HCC cell line SMMC-7721, revealing that SDF-1 enhances the secretion of angiogenic factors IL-8 and VEGF. Plumbagin (PL) was found to significantly inhibit SDF-1-induced angiogenesis in co-cultured SMMC-7721 and HUVECs, and it downregulated the mRNA expression of CXCR4 and CXCR7, thereby suppressing angiogenesis in HCC (73).
3.6 Tannic
Terminalia bellirica (Gaertn.) Roxb. (TB-TF) tannin extract promotes T cell infiltration mediated by the chemokines CCL5 and CXCL10. Chemokine CCL5 and its receptor CCR5, as well as CXCL9, CXCL10, and their receptor CXCR3, are involved in the recruitment of CD8+ T cells within the TME (96). Immunohistochemical (IHC) staining with CD68, a pan-macrophage marker, was employed to evaluate macrophage recruitment. The results showed that TB-TF treatment significantly increased the number of tumor-infiltrating macrophages in Hepa 1-6 orthotopic mice and markedly enhanced macrophage-mediated recruitment of CD8+T cells. Restoring the ability of tumor-trained macrophages to recruit CD8+T cells inhibited HCC growth and reversed tumor-conditioned media-induced M2 polarization of macrophages. However, the underlying mechanisms by which TB-TF reverses this tumor cell suppression remain unclear and warrant further investigation (74).
3.7 Terpenes
Terpenoids are a class of natural products commonly found in plants, known for their diverse biological activities, including anti-inflammatory, antibacterial, and antitumor properties. In the realm of antitumor research, the antitumor potential of terpenoids has been extensively investigated. Current studies suggest that cantharidin and triptolide may exert their antitumor effects by modulating chemokines and their receptor signaling pathways, thereby influencing the activities of immune cells and tumor cells.
3.7.1 Cantharidin
Cantharidin (CTD), the primary component of an anticancer drug derived from Mylabris phalerata Pallas, has shown significant antitumor activity in various cancers, particularly HCC (97). In vivo treatment with CTD resulted in increased expression of CXC and CCL chemokines involved in the immune response. Flow cytometry analysis of mouse blood indicated an elevated proportion of CD4+/CD8+ T cells and B cells, while the proportion of T lymphocytes decreased. These findings suggest that CTD may inhibit HCC progression by modulating chemokines that regulate immune cell trafficking and immune signaling responses (75).
3.7.2 Celastrol
Celastrol, a compound extracted from the traditional Chinese herb Tripterygium wilfordii Hook. f., exhibits notable antitumor activity. Its antitumor mechanisms primarily include inhibiting tumor cell proliferation, promoting tumor cell apoptosis, suppressing tumor cell migration, and inhibiting tumor angiogenesis. Chan et al. discovered that triptolide dose-dependently reduces CXCR4 expression by inhibiting the CXCR4-mediated pathway. This inhibition also downregulated downstream pathways, including PI3K and Akt. Their experimental results demonstrated that triptolide significantly inhibits the proliferation and migration of HCC cells and induces apoptosis by targeting CXCR4-related signaling pathways (76).
3.8 Steroids
Current research indicates that steroid compounds have potential therapeutic effects in HCC treatment through modulation of chemokines (98). Yang et al. observed elevated expression of APOBEC3F in HCC tumors compared to adjacent tissues based on proliferation and migration experiments, suggesting it as a potential tumor protein influencing HCC invasiveness. Further confirmation through APOBEC3F siRNA experiments showed that siAPOBEC3F reduced the expression levels of intestinal immune network IgA generation pathway proteins, including CCR9, CCR10, CXCR4, and pIgR. Subsequently, in SK-Hep1 and Bel-7404 cell lines, the effects of bufalin on cell proliferation and migration were assessed via CCK-8 assay, wound healing assay, and transwell assay. Bufalin was found to inhibit the expression of CCR9, CCR10, CXCR4, and pIgR proteins, thereby suppressing IgA production and impeding cancer cell proliferation and migration (77).
3.9 Phenylpropanoids
Schisandra chinensis (Turcz.) Baill is a perennial deciduous woody vine in the Magnoliaceae family (99). A recent study by Jiang et al. revealed that the combination of the Schisandra chinensis lignans and acteoside effectively suppressed the expression of CCL20. This is noteworthy because the CCL20-CCR6 axis is implicated in promoting invasion and metastasis of hepatocellular carcinoma cells by influencing regulatory T cells within the tumor microenvironment. The study suggests that the Schisandra chinensis lignans and acteoside may counteract CCL20-induced epithelial-mesenchymal transition (EMT), invasion, and migration of hepatocellular carcinoma cells via the ERK1/2 pathway, while also promoting apoptosi (78). Osthole is a natural coumarin compound extracted from the dried mature fruits of the Umbelliferae plant Cnidium monnieri. Liu and colleagues utilized an SD rat model of thioacetamide (TAA)-induced liver fibrosis to demonstrate osthol’s significant reduction in fibrosis-related gene expression induced by TAA. Analysis of various extracellular matrix (ECM) formation mediators via cytokine arrays revealed that osthol treatment markedly decreases levels of ICAM-1, CD62L, VEGF, and the chemokine CX3CL1. These findings highlight osthol’s potent inhibitory effects on the inflammatory response associated with liver fibrosis (79).
3.10 Other natural product extracts
Natural product extracts have shown promising potential in regulating chemokine expression for the treatment of HCC. Specific natural product extracts have been identified for their ability to modulate chemokine expression, thereby impeding the progression of HCC. These extracts exert their effects by targeting specific signaling pathways or molecular targets involved in the generation and secretion of chemokines, thus modifying the tumor microenvironment to suppress tumor growth and metastasis. For instance, Oldenlandia diffusa (Willd.) Roxb. (OD) extract, a natural product, inhibits HCC metastasis by downregulating crucial migration-related chemokine receptors such as CXCR1, CXCR2, and CXCR4. Scratch wound healing assays conducted with Huh7 and HepG2 cells have demonstrated that OD inhibits the migration of liver cancer cells through the inhibition of OA and UA migration receptors. Moreover, OD enhances the expression of the apoptosis-related enzyme caspase-3, thereby suppressing the growth of liver cancer cells (80). Trichosanthin (TCS) is a single-chain ribosome-inactivating protein extracted from the rhizomes of the natural product Trichosanthes Linn (100). Wang et al. explored the in vitro and in vivo anticancer effects of TCS in HCC by administering it to HCC cell cultures and xenograft models in BALB/c mice with intact immune systems. They investigated how TCS modulates T cell recruitment in host anti-HCC immune responses. Treatment with varying concentrations of TCS demonstrated dose-dependent inhibition of HCC cell line and xenograft tumor growth. Further investigations showed that TCS upregulates the expression of chemokines CCL2, CCL17, and CCL22, facilitating the accumulation of CD8+ T cells in HCC tissues (81). Additionally, TCS-induced GrzB secretion by T cells, transported via M6PR into HCC cells, promotes cell apoptosis (101). These findings suggest that TCS enhances T cell-mediated immunity in anti-tumor immunotherapy by potentially augmenting chemokine secretion and facilitating GrzB entry into HCC cell (81). Cisplatin (CDDP), a platinum-based anticancer drug, markedly upregulates the expression of chemokine CKLF1 in HepG2 cells. Functional assays reveal that CKLF1 potentially enhances metastasis in HCC. Kanglaite (KLT), derived from Coix lacryma-jobi L.var.mayuen(Roman.) Stapf seed, mitigates CDDP-induced CKLF1-mediated NF-κB pathway activation, thereby synergizing with CDDP in the combined therapy of HCC (102). Yang et al. established a subcutaneous tumor model in mice and found that treatment with AAE significantly reduced tumor size and weight. Immunohistochemical staining revealed that AAE intervention significantly decreased the proliferative capacity of tumor cells and increased the expression of multiple chemokines, including CCL2, CCL5, and CCL10. Notably, CCL2 was the most upregulated chemokine following AAE treatment, significantly enhancing NK cell infiltration into tumors. The knockdown of CCL2 via viral transfection attenuated AAE’s effects on NK cell infiltration and tumor growth inhibition, further validating these findings. The authors conducted in vitro studies using HuH7, HepG2, and Hepa1-6 cell lines, elucidating that the upregulation of CCL2 expression is mediated through JNK activation. Viral transfection-mediated knockdown of CCL2 diminished AAE’s effects on NK cell infiltration and tumor growth inhibition (29). Cisplatin (CDDP) is a widely employed chemotherapeutic agent for treating hepatocellular carcinoma. While elevating its concentration enhances cancer cell apoptosis rates, it concurrently poses risks of toxicity and resistance, thereby constraining its clinical utility (103). Kanglaite (KLT) is a biologically active compound derived from Coix lacryma-Jobi, recognized as a biphasic broad-spectrum anticancer agent (104). KLT augmented the antitumor efficacy of CDDP in HepG2 cells. While CDDP notably upregulated the chemokine-like factor 1 (CKLF1)-mediated NF-κB pathway in HepG2 cells, KLT effectively suppressed CDDP-induced NF-κB activation. This synergistic effect possibly stems from its ability to modulate inflammation and combat chemotherapy resistance (102). The mechanism underlying the combined therapy of KLT and CDDP against hepatocellular carcinoma remains unclear, necessitating comprehensive studies. Nevertheless, these findings advance our understanding of the pharmacological interactions between traditional Chinese and Western medicines, highlighting their potential in treating hepatocellular carcinoma.
4 Role of botanical drugs remedies in hepatocellular carcinoma
Botanical drugs remedies are a pivotal aspect of traditional medicine, distinguished by their multi-component and multi-target characteristics (105). These formulations regulate the body’s internal environment and enhance immune function, thereby achieving therapeutic effects (106). Recent studies have increasingly highlighted the efficacy of natural product formulations in treating HCC (Table 2).
The use of Astragalus mongholicus Bunge—Curcuma aromatica Salisb. (AC) is a classic pairing in Chinese medicine for the treatment of HCC disease (118). AC modulates the CXCL8/CXCR2 chemokine axis and inhibits the PI3K/Akt/mTOR pathway in colorectal cancer cells and orthotopic mouse models, thereby exerting anti-metastatic effects on HCC (107). Biejiajian Pill (BJJP), originally documented in the “Jin Gui Yao Lue”. Research indicates that BJJP inhibits tumor cell growth in an immune-dependent manner, modulating CCL5 expression and promoting CD8+ T cell infiltration into HCC tumors in H22 tumor-bearing mice (108). Cheng et al. demonstrated that Gehua Jiecheng Decoction (GHJCD) has therapeutic effects on diethylnitrosamine (DEN)-induced HCC in mice. Their findings elucidate that GHJCD counteracts the immunosuppressive tumor microenvironment of HCC by downregulating the proportions of Tregs, TAMs, and MDSCs (109). Zhang et al. utilized bioinformatics and network pharmacology to identify that the regulator of cell cycle gene (RGCC) and atypical chemokine receptor 3 (ACKR3) are implicated in the progression from non-alcoholic liver disease to hepatocellular carcinoma and impact the prognosis of hepatocellular carcinoma. They discovered that the Shipi-Xiaoji recipe (SPXJF) effectively inhibited the proliferation and invasion of HEPG2 cells in a dose-dependent manner. Furthermore, SPXJF was observed to dose-dependently suppress the expression of RGCC and ACKR3 proteins, highlighting its potential therapeutic relevance (110). The Chinese herbal decoction Kuan-Sin-Yin (KSY) reduces the expression of CCL2, which is associated with cell migration, and downregulates the mRNA levels of phosphoinositide-3-kinase regulatory subunit 3 (PIK3R3) and CEA cell adhesion molecule 1 (CEACAM1), thereby inhibiting the migration and invasion of HCC cells (111). Wang et al. explored the mechanisms by which Jiedu Huayu granules prevent liver injury in a rat model induced by D-galactosamine and lipopolysaccharide, demonstrating that these granules mitigate liver damage through T cell-mediated suppression of inflammation (119). Tianhuang formula (THF) downregulates the macrophage marker CD68 and improves liver injury, inflammation, and fibrosis by inhibiting the CCL2-CCR2 axis and its downstream MAPK/NF-κB signaling pathways (112). Dahuang Zhechong Pill (DZP) reduces the expression of CCL2 and its receptor CCR2 in the liver, decreasing M2 macrophage polarization. By remodeling the hepatic microenvironment, it inhibits colorectal cancer liver metastasis, providing strong evidence for the role of traditional Chinese medicine in reshaping the premetastatic niche and preventing liver metastasis (113). The Wan-Nian-Qing prescription (WNQP), a traditional Chinese medicine formulation containing Ornithogalum caudatum, has shown promising immunomodulatory effects. Antibody chip screening in immunosuppressed BALB/c mice revealed that WNQP modulates serum levels of interleukins and chemokines, leading to IL-2 activation of NK cells and subsequent secretion of CCL28. This mechanism enhances the targeted cytotoxicity against tumor cells, forming an immunoregulatory network that inhibits tumor growth and regulates tumor progression (114). When it comes to treating liver diseases, the Qizhu Anticancer Prescription (QZACP) has shown promise as a therapeutic approach (120). In DEN-induced hepatocellular carcinoma mice, QZACP significantly reduced both the number and size of intrahepatic nodules. It effectively mobilized NK and CD8+ T cells to combat tumor invasion and stimulated the release of interferon-γ. Furthermore, QZACP induced irreversible cell cycle arrest in stressed tumor cells by up-regulating the expression of p21 and promoting the secretion of PASP factors (decorin, CXCL14, and Wnt family member 2) within tumor cells after recruiting NK and CD8+ T cells (115). Yang et al. demonstrated that the binding of SDF-1 to CXCR4 activates downstream signaling pathways that regulate tumor cell proliferation, adhesion, and migration. The traditional Chinese medicine formulation Chanling Gao (CLG) was found to inhibit the expression of SDF-1α and CXCR4 in the liver. Results indicate that CLG effectively suppresses the growth and liver metastasis of colorectal cancer xenografts in nude mice, potentially through the HIF-1α/SDF-1α-CXCR4/PI3K-Akt signaling pathway (116). JC-001 is a traditional Chinese medicine formulation used for the treatment of liver diseases. Clinical studies have demonstrated that JC-001 inhibits Hepa 1-6 tumors in immunocompetent models through immunomodulation. It increases TNF-αlevels in the tumor microenvironment, thereby enhancing the inflammatory response. Additionally, JC-001 induces an elevation in IL-12p70 levels within the tumor microenvironment. IL-12 enhances the cytotoxicity, survival, and proliferation of CD8+ T cells by producing CCL1 and CCL17. It also aids in the differentiation of naive CD4+ T cells into Th1 cells, promoting the secretion of TNF-α and IFN-γ by T cells and NK cells (117). Botanical drugs remedies have shown efficacy and advantages in the treatment of HCC, involving multiple mechanisms. However, current research on their use for HCC remains in the early stages, and further in-depth investigation and validation of their mechanisms of action and efficacy are required.
5 Discussion and prospect
Currently, there are numerous treatments for HCC, including surgical resection, liver transplantation, radio frequencyablation (RFA), transcatheter arterial chemoembolization (TACE), targeted therapy, and immunotherapy (121). Due to the subtle symptoms of early-stage HCC, early detection is often challenging, making surgical eradication difficult at diagnosis. Surgical interventions, particularly liver transplantation, are theoretically effective but limited in practice by donor shortages and eligibility criteria (122). Liver resection also has drawbacks, including lower recurrence-free survival rates and high risks for patients with advanced cancer and cirrhosis (123). Radio frequencyablation techniques are minimally invasive, safe, simple, and relatively inexpensive, but they require repeated treatments to be effective and are limited by tumor size and location (124). TACE is an interventional procedure that delivers chemotherapeutic drugs directly to liver tumors via the hepatic artery, effectively targeting the tumor tissue (125). Although TACE can improve survival rates in intermediate-stage patients, the complex arterial supply of HCC means that tumors may continue to grow via collateral circulation even after main vessel embolization, leading to postoperative recurrence (126). Chemotherapy for HCC also carries risks. For instance, sorafenib, a multi-kinase inhibitor, can block up to 40 kinases, extending the survival time of patients with advanced HCC from 7.9 months to 10.7 months (127). However, its adverse effects, such as hand-foot skin reactions, diarrhea, liver function abnormalities, hypertension, and rash, as well as the potential for drug resistance within six months of treatment initiation, limit its clinical efficacy in treating HCC (128).
HCC resides within a complex immune microenvironment, where the TME often exerts functional suppression on immune cells, thereby playing a pivotal role in cancer progression (129). The genesis and dynamic alterations of this TME encompass diverse cell types and intricate signaling pathways, reminiscent of the multi-faceted and bidirectional immune modulation emphasized in traditional Chinese medicine (130, 131). Natural products leverage their advantages such as comprehensive regulation and minimal toxicity, complementing conventional therapies by ameliorating patient symptoms, bolstering immune function, and enhancing overall quality of life during HCC treatment. Natural products, enriched with multiple active constituents, confers diverse mechanisms of action and targets, thus influencing the pathological processes of HCC through multifaceted approaches concurrently (132). Based on current research, we are increasingly clarifying the mechanisms through which natural products exert therapeutic effects in HCC by modulating chemokine function. Certain active ingredients of natural products inhibit chemokine production, thereby reducing immune cell aggregation and inflammation. Conversely, other active ingredients can stimulate chemokine expression, enhancing immune system function and suppressing tumor growth and metastasis (133). Chemokines, a significant research focus in biomedicine, play recognized roles in immune and inflammation-related diseases (134). Chemokine-based therapies in immunotherapy generally fall into two categories: targeting pro-tumor chemokines and increasing anti-tumor chemokine levels. Both can be used as standalone therapies or in combination with other treatment strategies. There have been successful clinical applications of chemokine therapies, such as the CXCR4 antagonist AMD3100 for treating relapsed or refractory acute myeloid leukemia (AML) and the CCL2 inhibitor CNTO 888 for metastatic prostate cancer (135). These developments provide new treatment options for cancer patients and lay a solid foundation for further advancement in chemokine therapies. Furthermore, data suggest that combining natural products with chemotherapy drugs significantly improves chemotherapy sensitivity, enhances tumor suppression effects, and notably ameliorates cancer-related fatigue, bone marrow suppression, and other adverse reactions (136). Natural products typically exhibit multi-target and multi-pathway characteristics in cancer treatment, effectively impeding HCC development by modulating various physiological processes (137). However, despite some successes, natural products in HCC treatment face challenges. The development and progression of HCC involve abnormalities in multiple genes and pathways, making it difficult for a single drug to address all aspects. Additionally, the complex composition and unclear mechanisms of natural products limit their application in HCC treatment. Chemokine therapies still face many challenges and opportunities. The complexity of chemokines and their receptors makes selecting and validating therapeutic targets particularly difficult. Nonetheless, with the continuous development and innovation of biotechnology, techniques like gene editing and the combined application of immunotherapy and chemokine therapies could allow more precise regulation of chemokine expression and function, achieving targeted disease treatment.
In summary, our comprehensive investigation into the role of chemokines within the immune microenvironment of HCC offers a novel perspective on understanding the disease’s pathogenesis (138). Concurrently, the exploration of natural product-derived active ingredients targeting chemokines in HCC treatment presents promising new therapeutic avenues (Figure 2). However, while natural products offer distinct advantages in HCC treatment, they also come with certain limitations (139). Thus, it is crucial to elucidate the precise mechanisms through which natural products modulate chemokines to enhance therapeutic outcomes in HCC. This effort aims to provide enhanced theoretical support and practical guidance for precision medicine in HCC treatment. As our understanding of HCC pathogenesis advances, future research may uncover additional therapeutic targets involving chemokines, potentially leading to more effective treatment strategies for HCC patients.
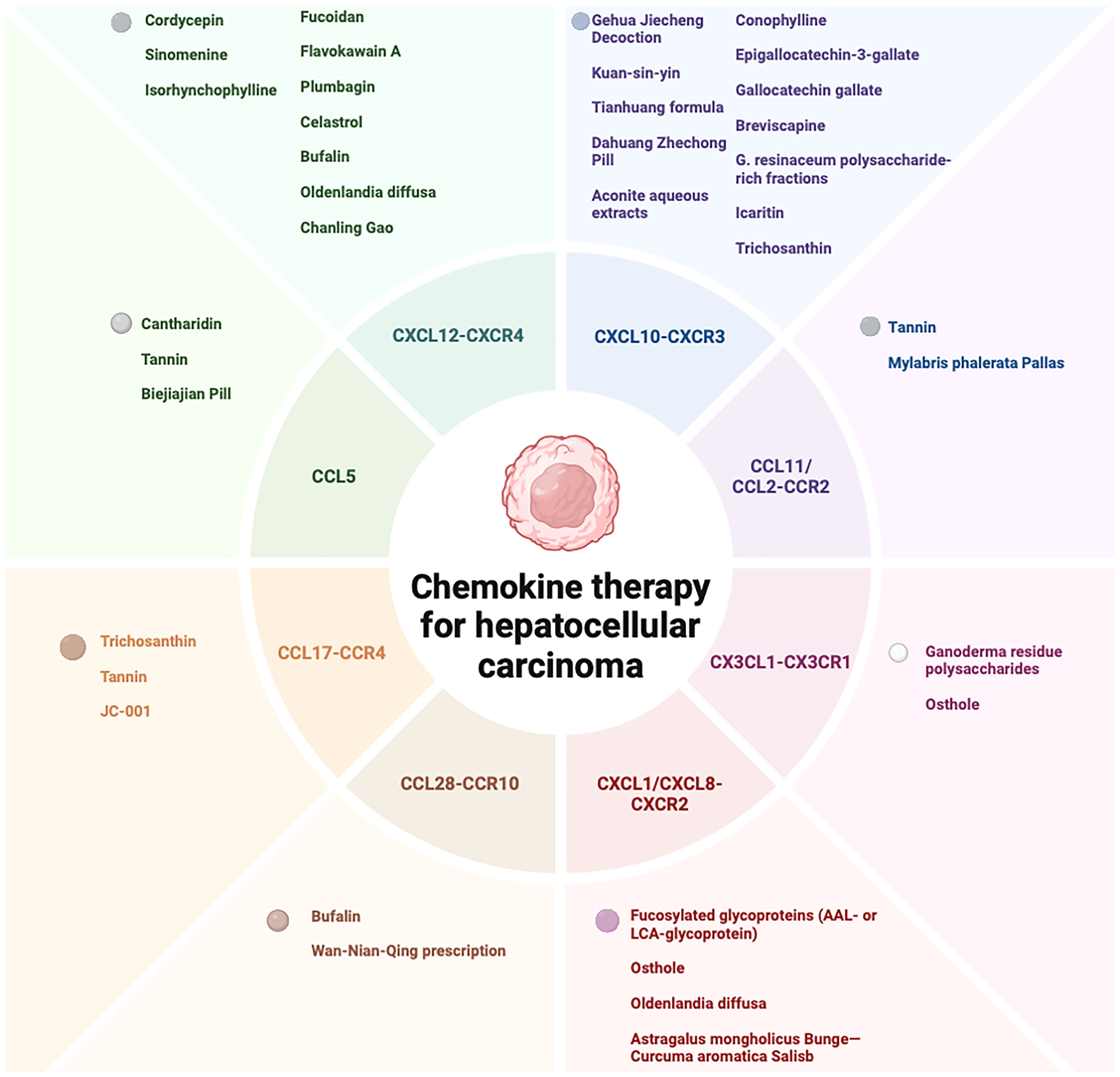
Figure 2. Therapeutic potential of natural products and its extracts, and formulations in HCC treatment via chemokine modulation.
Author contributions
XR: Writing – original draft. XL: Writing – original draft. BY: Writing – original draft. YW: Writing – original draft. ZX: Writing – original draft. FX: Writing – original draft. ZD: Writing – original draft. LX: Writing – review & editing. ZM: Writing – review & editing. LH: Writing – review & editing.
Funding
The author(s) declare financial support was received for the research, authorship, and/or publication of this article. This work was supported by the National Natural Science Foundation of China (Grant number: 82204785 and 82304913), the Jilin Province Science and Technology Development Plan Item (Grant Numbers: JJKH20241086KJ, YDZJ202401110ZYTS, 20240601005RC and 20220204001YY), the Jilin Provincial Administration of Traditional Chinese Medicine (Grant number: 2023115).
Conflict of interest
The authors declare that the research was conducted in the absence of any commercial or financial relationships that could be construed as a potential conflict of interest.
Publisher’s note
All claims expressed in this article are solely those of the authors and do not necessarily represent those of their affiliated organizations, or those of the publisher, the editors and the reviewers. Any product that may be evaluated in this article, or claim that may be made by its manufacturer, is not guaranteed or endorsed by the publisher.
References
1. Zhang HJ, Zhang WY, Jiang LY, Chen YH. Recent advances in systemic therapy for hepatocellular carcinoma. biomark Res. (2022) 10:3. doi: 10.1186/s40364-021-00350-4
2. Bishayee A. The role of inflammation in liver cancer. Adv Exp Med Biol. (2014) 816:401–35. doi: 10.1007/978.3-0348-0837-8-16
3. Sung H, Ferlay J, Siegel RL, Laversanne M, Soerjomataram I, Jemal A, et al. Global cancer statistics 2020: GLOBOCAN estimates of incidence and mortality worldwide for 36 cancers in 185 countries. CA: A Cancer J Clin. (2021) 71:209–49. doi: 10.3322/caac.21660
4. Russo FP, Zanetto A, Pinto E, Battistella S, Penzo B, Burra P, et al. Hepatocellular carcinoma in chronic viral hepatitis: where do we stand? Int J Mol Sci. (2022) 23:500. doi: 10.3390/ijms23010500
5. Deng Y, Huang J, Wong MCS. Associations of non-alcoholic fatty liver disease and cirrhosis with liver cancer in European and East Asian populations: A Mendelian randomization study. Cancer Rep. (2023) 7:e1913. doi: 10.1002/cnr2.1913
6. Jain D, Chaudhary P, Varshney N, Bin Razzak KS, Verma D, Khan Zahra TR, et al. Tobacco smoking and liver cancer risk: potential avenues for carcinogenesis. J Oncol. (2021) 2021:1–11. doi: 10.1155/2021/5905357
7. Hartke J, Johnson M, Ghabril M. The diagnosis and treatment of hepatocellular carcinoma. Semin Diagn Pathology. (2017) 34:153–9. doi: 10.1053/j.semdp.2016.12.011
8. Huang DQ, Singal AG, Kono Y, Tan DJH, El-Serag HB, Loomba R. Changing global epidemiology of liver cancer from 2010 to 2019: NASH is the fastest growing cause of liver cancer. Cell Metab. (2022) 34:969–77.e2. doi: 10.1016/j.cmet.2022.05.003
9. Xiao Y, Yu D. Tumor microenvironment as a therapeutic target in cancer. Pharmacol Ther. (2021) 221:107753. doi: 10.1016/j.pharmthera.2020.107753
10. Marcuzzi E, Angioni R, Molon B, Calì B. Chemokines and chemokine receptors: orchestrating tumor metastasization. Int J Mol Sci. (2018) 20:96. doi: 10.3390/ijms20010096
11. Cao S, Liu M, Sehrawat TS, Shah VH. Regulation and functional roles of chemokines in liver diseases. Nat Rev Gastroenterol Hepatology. (2021) 18:630–47. doi: 10.1038/s41575-021-00444-2
12. Legler DF, Thelen M. Chemokines: chemistry, biochemistry and biological function. Chimia. (2016) 70(12). doi: 10.2533/chimia.2016.856
13. Baggiolini M, Dewald B, Moser B. Human chemokines: an update. Annu Rev Immunol. (1997) 15:675–705. doi: 10.1146/annurev.immunol.15.1.675
14. Li BH, Garstka MA, Li ZF. Chemokines and their receptors promoting the recruitment of myeloid-derived suppressor cells into the tumor. Mol Immunol. (2020) 117:201–15. doi: 10.1016/j.molimm.2019.11.014
15. Wasmuth HE, Tacke F, Trautwein C. Chemokines in liver inflammation and fibrosis. Semin Liver Disease. (2010) 30:215–25. doi: 10.1055/s-0030-1255351
16. Chen KQ, Bao ZY, Tang P, Gong WH, Yoshimura T, Wang JM. Chemokines in homeostasis and diseases. Cell Mol Immunol. (2018) 15:324–34. doi: 10.1038/cmi.2017.134
17. Mikolajczyk TP, Szczepaniak P, Vidler F, Maffia P, Graham GJ, Guzik TJ. Role of inflammatory chemokines in hypertension. Pharmacol Ther. (2021) 223:107799. doi: 10.1016/j.pharmthera.2020.107799
18. Sprinzl MF, Galle PR. Current progress in immunotherapy of hepatocellular carcinoma. J Hepatology. (2017) 66:482–4. doi: 10.1016/j.jhep.2016.12.009
19. Do HTT, Lee CH, Cho J. Chemokines and their receptors: multifaceted roles in cancer progression and potential value as cancer prognostic markers. Cancers. (2020) 12(2):287. doi: 10.3390/cancers12020287
20. Xu M, Wang Y, Xia R, Wei Y, Wei X. Role of the CCL2-CCR2 signalling axis in cancer: Mechanisms and therapeutic targeting. Cell Proliferation. (2021) 54:e13115. doi: 10.1111/cpr.13115
21. Chen L, Gu J, Qian Y, Li M, Qian Y, Xu M, et al. Deletion of C-C motif chemokine ligand 5 worsens invariant natural killer T-cell–mediated hepatitis via compensatory up-regulation of CXCR2–related chemokine activity. Cell Mol Gastroenterol Hepatology. (2019) 7:623–39. doi: 10.1016/j.jcmgh.2018.12.009
22. Liu LZ, Zhang Z, Zheng BH, Shi Y, Duan M, Ma LJ, et al. CCL15 recruits suppressive monocytes to facilitate immune escape and disease progression in hepatocellular carcinoma. Hepatology. (2018) 69:143–59. doi: 10.1002/hep.30134
23. Brandt EF, Fischer P, Wirtz T, Sahin H, Berres ML. Chemokine receptor CXCR3 modulates polarization of tumor associated macrophages limiting tumor growth and angiogenesis in murine hepatocellular carcinoma. J Hepatology. (2018) 68:S663–S4. doi: 10.1016/S0168-8278(18)31583-6
24. Zajkowska M, Mroczko B. Chemokines in primary liver cancer. Int J Mol Sci. (2022) 23(16):8846. doi: 10.3390/ijms23168846
25. Li Y, Li Y, Zhang J, Ji L, Li M, Sun X, et al. Current perspective of traditional chinese medicines and active ingredients in the therapy of hepatocellular carcinoma. J Hepatocellular Carcinoma. (2022) 9:41–56. doi: 10.2147/JHC.S346047
26. Fu K, Wang C, Ma C, Zhou H, Li Y. The potential application of chinese medicine in liver diseases: A new opportunity. Front Pharmacol. (2021) 12. doi: 10.3389/fphar.2021.771459
27. Yao W, Ba Q, Li X, Li H, Zhang S, Yuan Y, et al. A natural CCR2 antagonist relieves tumor-associated macrophage-mediated immunosuppression to produce a therapeutic effect for liver cancer. EBioMedicine. (2017) 22:58–67. doi: 10.1016/j.ebiom.2017.07.014
28. Jiang ZT, Han Y, Liu XY, Lv LY, Pan JH, Liu CD. Tripterine Restrains the Aggressiveness of Hepatocellular Carcinoma Cell via Regulating miRNA-532-5p/CXCL2 Axis. OncoTargets Ther. (2020) 13:2973–85. doi: 10.2147/OTT.S238074
29. Yang K-d, Zhang X, Shao M-c, Wang L-n. Aconite aqueous extract inhibits the growth of hepatocellular carcinoma through CCL2-dependent enhancement of natural killer cell infiltration. J Integr Medicine-Jim. (2023) 21:575–83. doi: 10.1016/j.joim.2023.10.002
30. Mollica Poeta V, Massara M, Capucetti A, Bonecchi R. Chemokines and chemokine receptors: new targets for cancer immunotherapy. Front Immunol. (2019) 10. doi: 10.3389/fimmu.2019.00379
31. Nagarsheth N, Wicha MS, Zou W. Chemokines in the cancer microenvironment and their relevance in cancer immunotherapy. Nat Rev Immunol. (2017) 17:559–72. doi: 10.1038/nri.2017.49
32. Zhou Z, Hu Y, Wu Y, Qi Q, Wang J, Chen L, et al. The immunosuppressive tumor microenvironment in hepatocellular carcinoma-current situation and outlook. Mol Immunol. (2022) 151:218–30. doi: 10.1016/j.molimm.2022.09.010
33. Hirano R, Okamoto K, Shinke M, Sato M, Watanabe S, Watanabe H, et al. Tissue-resident macrophages are major tumor-associated macrophage resources, contributing to early TNBC development, recurrence, and metastases. Commun Biol. (2023) 6:144. doi: 10.1038/s42003-023-04525-7
34. Chávez-Galán L, Olleros ML, Vesin D, Garcia I. Much More than M1 and M2 Macrophages, There are also CD169+ and TCR+ Macrophages. Front Immunol. (2015) 6. doi: 10.3389/fimmu.2015.00263
35. Frontiers Production O. Erratum: Construction of a tumor immune infiltration macrophage signature for predicting prognosis and immunotherapy response in liver cancer. Front Mol Biosci. (2022) 9. doi: 10.3389/fmolb.2022.1094902
36. Ovais M, Guo M, Chen C. Tailoring nanomaterials for targeting tumor-associated macrophages. Advanced Materials. (2019) 31:1808303. doi: 10.1002/adma.201808303
37. Zhu W, Guo L, Zhang B, Lou L, Lin Z, Zhu X, et al. Combination of osteopontin with peritumoral infiltrating macrophages is associated with poor prognosis of early-stage hepatocellular carcinoma after curative resection. Ann Surg Oncol. (2013) 21:1304–13. doi: 10.1245/s10434-013-3445-0
38. Zhao H, Wei S, Zhou D, Liu Y, Guo Z, Fang C, et al. Blocking the CXCL1-CXCR2 axis enhances the effects of doxorubicin in HCC by remodelling the tumour microenvironment via the NF-κB/IL-1β/CXCL1 signalling pathway. Cell Death Discovery. (2023) 9:120. doi: 10.1038/s41420-023-01424-y
39. Li X, Yao W, Yuan Y, Chen P, Li B, Li J, et al. Targeting of tumour-infiltrating macrophages via CCL2/CCR2 signalling as a therapeutic strategy against hepatocellular carcinoma. Gut. (2017) 66:157–67. doi: 10.1136/gutjnl-2015-310514
40. Li C, Xu X, Wei S, Jiang P, Xue L, Wang J. Tumor-associated macrophages: potential therapeutic strategies and future prospects in cancer. J ImmunoTherapy Cancer. (2021) 9:e001341. doi: 10.1136/jitc-2020-001341
41. Peng Z-P, Jiang Z-Z, Guo H-F, Zhou M-M, Huang Y-F, Ning W-R, et al. Glycolytic activation of monocytes regulates the accumulation and function of neutrophils in human hepatocellular carcinoma. J Hepatology. (2020) 73:906–17. doi: 10.1016/j.jhep.2020.05.004
42. Andzinski L, Kasnitz N, Stahnke S, Wu CF, Gereke M, von Köckritz-Blickwede M, et al. Type IIFNs induce anti-tumor polarization of tumor associated neutrophils in mice and human. Int J Cancer. (2015) 138:1982–93. doi: 10.1002/ijc.29945
43. Fridlender ZG, Sun J, Kim S, Kapoor V, Cheng GJ, Ling LN, et al. Polarization of tumor-associated neutrophil phenotype by TGF-β: “N1” versus “N2” TAN. Cancer Cell. (2009) 16:183–94. doi: 10.1016/j.ccr.2009.06.017
44. Zhou SL, Yin D, Hu ZQ, Luo CB, Zhou ZJ, Xin HY, et al. A positive feedback loop between cancer stem-like cells and tumor-associated neutrophils controls hepatocellular carcinoma progression. Hepatology. (2019) 70:1214–30. doi: 10.1002/hep.30630
45. Xu W, Weng J, Xu M, Zhou Q, Liu S, Hu Z, et al. Chemokine CCL21 determines immunotherapy response in hepatocellular carcinoma by affecting neutrophil polarization. Cancer Immunology Immunotherapy. (2024) 73:56. doi: 10.1007/s00262-024-03650-4
46. Gabrilovich DI, Nagaraj S. Myeloid-derived suppressor cells as regulators of the immune system. Nat Rev Immunol. (2009) 9:162–74. doi: 10.1038/nri2506
47. Wang Y, Zhang T, Sun M, Ji X, Xie M, Huang W, et al. Therapeutic values of myeloid-derived suppressor cells in hepatocellular carcinoma: facts and hopes. Cancers. (2021) 13(20):5127. doi: 10.3390/cancers13205127
48. Li YM, Liu ZY, Wang JC, Yu JM, Li ZC, Yang HJ, et al. Receptor-interacting protein kinase 3 deficiency recruits myeloid-derived suppressor cells to hepatocellular carcinoma through the chemokine (C-X-C motif) ligand 1–chemokine (C-X-C motif) receptor 2 axis. Hepatology. (2019) 70:1564–81. doi: 10.1002/hep.30676
49. Li B-H, Jiang W, Zhang S, Huang N, Sun J, Yang J, et al. The spleen contributes to the increase in PMN-MDSCs in orthotopic H22 hepatoma mice. Mol Immunol. (2020) 125:95–103. doi: 10.1016/j.molimm.2020.07.002
50. Baglieri J, Brenner D, Kisseleva T. The role of fibrosis and liver-associated fibroblasts in the pathogenesis of hepatocellular carcinoma. Int J Mol Sci. (2019) 20(7):1723. doi: 10.3390/ijms20071723
51. Ying F, Chan MSM, Lee TKW. Cancer-associated fibroblasts in hepatocellular carcinoma and cholangiocarcinoma. Cell Mol Gastroenterol Hepatology. (2023) 15:985–99. doi: 10.1016/j.jcmgh.2023.01.006
52. Luo Q, Wang C-Q, Yang L-Y, Gao X-M, Sun H-T, Zhang Y, et al. FOXQ1/NDRG1 axis exacerbates hepatocellular carcinoma initiation via enhancing crosstalk between fibroblasts and tumor cells. Cancer Letters. (2018) 417:21–34. doi: 10.1016/j.canlet.2017.12.021
53. Liu J, Chen S, Wang W, Ning B-F, Chen F, Shen W, et al. Cancer-associated fibroblasts promote hepatocellular carcinoma metastasis through chemokine-activated hedgehog and TGF-β pathways. Cancer Letters. (2016) 379:49–59. doi: 10.1016/j.canlet.2016.05.022
54. Unitt E, Marshall A, Gelson W, Rushbrook SM, Davies S, Vowler SL, et al. Tumour lymphocytic infiltrate and recurrence of hepatocellular carcinoma following liver transplantation. J Hepatology. (2006) 45:246–53. doi: 10.1016/j.jhep.2005.12.027
55. Pedroza-Gonzalez A, Verhoef C, Ijzermans JNM, Peppelenbosch MP, Kwekkeboom J, Verheij J, et al. Activated tumor-infiltrating CD4+ regulatory T cells restrain antitumor immunity in patients with primary or metastatic liver cancer. Hepatology. (2013) 57:183–94. doi: 10.1002/hep.26013
56. Lee WC, Wu TJ, Chou HS, Yu MC, Hsu PY, Hsu HY, et al. The impact of CD4+ CD25+ T cells in the tumor microenvironment of hepatocellular carcinoma. Surgery. (2012) 151(2):213–22. doi: 10.1016/j.surg.2011.07.029
57. Wang D, Yang L, Yue D, Cao L, Li L, Wang D, et al. Macrophage-derived CCL22 promotes an immunosuppressive tumor microenvironment via IL-8 in Malignant pleural effusion. Cancer Letters. (2019) 452:244–53. doi: 10.1016/j.canlet.2019.03.040
58. Choucair K, Duff JR, Cassidy CS, Albrethsen MT, Kelso JD, Lenhard A, et al. Natural killer cells: a review of biology, therapeutic potential and challenges in treatment of solid tumors. Future Oncol. (2019) 15:3053–69. doi: 10.2217/fon-2019-0116
59. Chen E-B, Zhou Z-J, Xiao K, Zhu G-Q, Yang Y, Wang B, et al. The miR-561-5p/CX3CL1 signaling axis regulates pulmonary metastasis in hepatocellular carcinoma involving CX3CR1+ Natural killer cells infiltration. Theranostics. (2019) 9:4779–94. doi: 10.7150/thno.32543
60. Nan Y, Su H, Zhou B, Liu S. The function of natural compounds in important anticancer mechanisms. Front Oncol. (2023) 12. doi: 10.3389/fonc.2022.1049888
61. Wei L, Wang Z, Jing N, Lu Y, Yang J, Xiao H, et al. Frontier progress of the combination of modern medicine and traditional Chinese medicine in the treatment of hepatocellular carcinoma. Chin Med. (2022) 17:90. doi: 10.1186/s13020-022-00645-0
62. Guo Z, Chen W, Dai G, Huang Y. Cordycepin suppresses the migration and invasion of human liver cancer cells by downregulating the expression of CXCR4. Int J Mol Med. (2019) 45:141–50. doi: 10.3892/ijmm
63. Shen C, Li J, Li R, Ma Z, Tao Y, Zhang Q, et al. Effects of tumor-derived DNA on CXCL12-CXCR4 and CCL21-CCR7 axes of hepatocellular carcinoma cells and the regulation of sinomenine hydrochloride. Front Oncol. (2022) 12. doi: 10.3389/fonc.2022.901705
64. Yamanaka T, Harimoto N, Yokobori T, Muranushi R, Hoshino K, Hagiwara K, et al. Conophylline inhibits hepatocellular carcinoma by inhibiting activated cancer-associated fibroblasts through suppression of G protein–coupled receptor 68. Mol Cancer Ther. (2021) 20:1019–28. doi: 10.1158/1535-7163.MCT-20-0150
65. Lee H, Baek S, Lee J, Kim C, Ko J-H, Lee S-G, et al. Isorhynchophylline, a potent plant alkaloid, induces apoptotic and anti-metastatic effects in human hepatocellular carcinoma cells through the modulation of diverse cell signaling cascades. Int J Mol Sci. (2017) 18(5):1095. doi: 10.3390/ijms18051095
66. Tripathi DK, Nagar N, Kumar V, Joshi N, Roy P, Poluri KM. Gallate moiety of catechin is essential for inhibiting CCL2 chemokine-mediated monocyte recruitment. J Agric Food Chem. (2023) 71:4990–5005. doi: 10.1021/acs.jafc.3c01283
67. Sipping MTK, Mediesse FK, Kenmogne LV, Kanemoto JEN, Njamen D, Boudjeko T. Polysaccharide-rich fractions from Ganoderma resinaceum (Ganodermataceae) as chemopreventive agents in N-diethylnitrosamine-induced hepatocellular carcinoma in wistar rats. Evidence-Based Complementary Altern Med. (2022) 2022:8198859. doi: 10.1155/2022/8198859
68. Nagamine T, Hayakawa K, Kusakabe T, Takada H, Nakazato K, Hisanaga E, et al. Inhibitory effect of fucoidan on Huh7 hepatoma cells through downregulation of CXCL12. Nutr Cancer. (2009) 61:340–7. doi: 10.1080/01635580802567133
69. Wu LH, Shi BZ, Zhao QL, Wu XZ. Fucosylated glycan inhibition of human hepatocellular carcinoma cell migration through binding to chemokine receptors. Glycobiology. (2009) 20:215–23. doi: 10.1093/glycob/cwp168
70. Liu Y, Wen PH, Zhang XX, Dai Y, He Q. Breviscapine ameliorates CCl4−induced liver injury in mice through inhibiting inflammatory apoptotic response and ROS generation. Int J Mol Med. (2018) 42:755–68. doi: 10.3892/ijmm
71. Fan Y, Li S, Ding X, Yue J, Jiang J, Zhao H, et al. First-in-class immune-modulating small molecule Icaritin in advanced hepatocellular carcinoma: preliminary results of safety, durable survival and immune biomarkers. BMC Cancer. (2019) 19:279. doi: 10.1186/s12885-019-5471-1
72. Xiao T, Bao J, Tian J, Lin R, Zhang Z, Zhu Y, et al. Flavokawain A suppresses the vasculogenic mimicry of HCC by inhibiting CXCL12 mediated EMT. Phytomedicine. (2023) 112:154687. doi: 10.1016/j.phymed.2023.154687
73. Zhong J, Li JX, Wei JX, Huang DT, Huo LN, Zhao C, et al. Plumbagin restrains hepatocellular carcinoma angiogenesis by stromal cell-derived factor (SDF-1)/CXCR4-CXCR7 axis. Med Sci Monitor. (2019) 25:6110–9. doi: 10.12659/MSM.915782
74. Chang Z, Zhang Q, Hu Q, Liu Y, Zhang L, Liu R. Tannins in Terminalia bellirica inhibits hepatocellular carcinoma growth via re-educating tumor-associated macrophages and restoring CD8+T cell function. Biomedicine Pharmacotherapy. (2022) 154:113543. doi: 10.1016/j.biopha.2022.113543
75. Yan J, Deng XL, Ma SQ, Hui Li Y, Gao YM, Shi GT, et al. Cantharidin suppresses hepatocellular carcinoma development by regulating EZH2/H3K27me3-dependent cell cycle progression and antitumour immune response. BMC Complementary Med Therapies. (2023) 23:160. doi: 10.1186/s12906-023-03975-0
76. Kun-Ming C, Chih-Hsien C, Chen-Fang L, Ting-Jung W, Hong-Shiue C, Wei-Chen L. Potential anticancer effect of celastrol on hepatocellular carcinoma by suppressing CXCR4-related signal and impeding tumor growth in vivo. Arch Med Res. (2020) 51:297–302. doi: 10.1016/j.arcmed.2020.03.001
77. Yang Z, Tao Y, Xu X, Cai F, Yu Y, Ma L. Bufalin inhibits cell proliferation and migration of hepatocellular carcinoma cells via APOBEC3F induced intestinal immune network for IgA production signaling pathway. Biochem Biophys Res Commun. (2018) 503:2124–31. doi: 10.1016/j.bbrc.2018.07.169
78. Jiang JJ, Cheng R, Song AQ, Lou YF, Fan GR. Multi-omics analysis reveals mechanism of Schisandra chinensis lignans and acteoside on EMT in hepatoma cells via ERK1/2 pathway. Funct Integr Genomics. (2024) 24:112. doi: 10.1007/s10142-024-01351-w
79. Liu YW, Chiu YT, Fu SL, Huang YT. Osthole ameliorates hepatic fibrosis and inhibits hepatic stellate cell activation. J Biomed Sci. (2015) 22:63. doi: 10.1186/s12929-015-0168-5
80. Sunwoo Y-Y, Lee J-H, Jung HY, Jung YJ, Park M-S, Chung Y-A, et al. Oldenlandia diffusaPromotes antiproliferative and apoptotic effects in a rat hepatocellular carcinoma with liver cirrhosis. Evidence-Based Complementary Altern Med. (2015) 2015:1–11. doi: 10.1155/2015/501508
81. Wang K, Wang X, Zhang M, Ying Z, Zhu Z, Tam KY, et al. Trichosanthin promotes anti-tumor immunity through mediating chemokines and granzyme B secretion in hepatocellular carcinoma. Int J Mol Sci. (2023) 24(2):1416. doi: 10.3390/ijms24021416
82. Liu C, Yang S, Wang K, Bao X, Liu Y, Zhou S, et al. Alkaloids from Traditional Chinese Medicine against hepatocellular carcinoma. Biomedicine Pharmacotherapy. (2019) 120:109543. doi: 10.1016/j.biopha.2019.109543
83. Tuli HS, Sandhu SS, Sharma AK. Pharmacological and therapeutic potential of Cordyceps with special reference to Cordycepin. 3 Biotech. (2013) 4:1–12. doi: 10.1007/s13205-013-0121-9
84. Lee C-T, Huang K-S, Shaw J-F, Chen J-R, Kuo W-S, Shen G, et al. Trends in the immunomodulatory effects of cordyceps militaris: total extracts, polysaccharides and cordycepin. Front Pharmacol. (2020) 11. doi: 10.3389/fphar.2020.575704
85. Li X, Wu W, Cao F, Hu X, Wu X, Fu J. Mechanism of cordycepin enhancing doxorubicin against hepatocellular carcinoma in vitro and in vivo. J Funct Foods. (2022) 98:105268. doi: 10.1016/j.jff.2022.105268
86. Hou W, Huang L, Huang H, Liu S, Dai W, Tang J, et al. Bioactivities and mechanisms of action of sinomenine and its derivatives: A comprehensive review. Molecules. (2024) 29(2):540. doi: 10.3390/molecules29020540
87. Yang S, Peng L-Y, Peng W, Huang C, Wei D-N, Mou M-T, et al. Anticancer potentials of sinomenine from Sinomenium acutum: A mini-review. Trop J Pharm Res. (2019) 17(12):2519–26. doi: 10.4314/tjpr.v17i12.30
88. Liao XZ, Gao Y, Zhao HW, Zhou M, Chen DL, Tao LT, et al. Cordycepin reverses cisplatin resistance in non-small cell lung cancer by activating AMPK and inhibiting AKT signaling pathway. Front Cell Dev Biol. (2021) 8. doi: 10.3389/fcell.2020.609285
89. Zhang R, Ma C, Wei Y, Wang X, Jia J, Li J, et al. Isolation, purification, structural characteristics, pharmacological activities, and combined action of Hedyotis diffusa polysaccharides: A review. Int J Biol Macromolecules. (2021) 183:119–31. doi: 10.1016/j.ijbiomac.2021.04.139
90. Liu Y, Wu J, Hao H. Antitumor immunostimulatory activity of the traditional Chinese medicine polysaccharide on hepatocellular carcinoma. Front Immunol. (2024) 15. doi: 10.3389/fimmu.2024.1369110
91. Zvyagintseva TN, Usoltseva RV, Shevchenko NM, Surits VV, Imbs TI, Malyarenko OS, et al. Structural diversity of fucoidans and their radioprotective effect. Carbohydr Polymers. (2021) 273:118551. doi: 10.1016/j.carbpol.2021.118551
92. Ayrapetyan ON, Obluchinskaya ED, Zhurishkina EV, Skorik YA, Lebedev DV, Kulminskaya AA, et al. Antibacterial properties of fucoidans from the brown algae Fucus vesiculosus L. of the barents sea. Biology-Basel. (2021) 10:67. doi: 10.3390/biology10010067
93. Joshi N, Kumar D, Poluri KM. Elucidating the molecular interactions of chemokine CCL2 orthologs with flavonoid baicalin. ACS Omega. (2020) 5:22637–51. doi: 10.1021/acsomega.0c03428
94. Reyes-Hernández OD, Figueroa-González G, Quintas-Granados LI, Hernández-Parra H, Peña-Corona SI, Cortés H, et al. New insights into the anticancer therapeutic potential of icaritin and its synthetic derivatives. Drug Dev Res. (2024) 85:e22175. doi: 10.1002/ddr.22175
95. Yu Z, Guo J, Hu M, Gao Y, Huang L. Icaritin exacerbates mitophagy and synergizes with doxorubicin to induce immunogenic cell death in hepatocellular carcinoma. ACS Nano. (2020) 14:4816–28. doi: 10.1021/acsnano.0c00708
96. Gajewski TF, Schreiber H, Fu Y-X. Innate and adaptive immune cells in the tumor microenvironment. Nat Immunol. (2013) 14:1014–22. doi: 10.1038/ni.2703
97. Naz F, Wu YX, Zhang N, Yang Z, Yu CY. Anticancer attributes of cantharidin: involved molecular mechanisms and pathways. Molecules. (2020) 25(14):3279. doi: 10.3390/molecules25143279
98. Dey P, Kundu A, Chakraborty HJ, Kar B, Choi WS, Lee BM, et al. Therapeutic value of steroidal alkaloids in cancer: Current trends and future perspectives. Int J Cancer. (2019) 145:1731–44. doi: 10.1002/ijc.31965
99. He XL, Chen JM, Mu YP, Zhang H, Chen GF, Liu P, et al. The effects of inhibiting the activation of hepatic stellate cells by lignan components from the fruits of Schisandra chinensis and the mechanism of schisanhenol. J Natural Medicines. (2020) 74:513–24. doi: 10.1007/s11418-020-01394-w
100. Mondal A. A novel extraction of trichosanthin fromTrichosanthes kirilowiiroots using three-phase partitioning and its in vitro anticancer activity. Pharm Biol. (2014) 52:677–80. doi: 10.3109/13880209.2013.864684
101. Li CM, Zeng MQ, Chi HJ, Shen J, Ng TB, Jin GY, et al. Trichosanthin increases Granzyme B penetration into tumor cells by upregulation of CI-MPR on the cell surface. Oncotarget. (2017) 8:26460–70. doi: 10.18632/oncotarget.v8i16
102. Chen C, Ai Q-d, Wei Y-h. Kanglaite enhances the efficacy of cisplatin in suppression of hepatocellular carcinoma via inhibiting CKLF1 mediated NF-κB pathway and regulating transporter mediated drug efflux. J Ethnopharmacology. (2021) 264:113388. doi: 10.1016/j.jep.2020.113388
103. Ghosh S. Cisplatin: The first metal based anticancer drug. Bioorganic Chem. (2019) 88:102925. doi: 10.1016/j.bioorg.2019.102925
104. Lu CC, Wu SL, Ke LX, Liu FM, Shang WR, Deng XX, et al. Kanglaite (Coix seed extract) as adjunctive therapy in cancer: evidence mapping overview based on systematic reviews with meta-analyses. Front Pharmacol. (2022) 13. doi: 10.3389/fphar.2022.901875
105. Chen F, Li J, Wang H, Ba Q. Anti-tumor effects of chinese medicine compounds by regulating immune cells in microenvironment. Front Oncol. (2021) 11. doi: 10.3389/fonc.2021.746917
106. Ma C, Wang X, Zhang J, Zhao Y, Hua Y, Zhang C, et al. Exploring ganweikang tablet as a candidate drug for NAFLD through network pharmacology analysis and experimental validation. Front Pharmacol. (2022) 13. doi: 10.3389/fphar.2022.893336
107. Liu F, Liang Y, Sun R, Yang W, Liang Z, Gu J, et al. Astragalus mongholicus Bunge and Curcuma aromatica Salisb. inhibits liver metastasis of colon cancer by regulating EMT via the CXCL8/CXCR2 axis and PI3K/AKT/mTOR signaling pathway. Chin Med. (2022) 17:91. doi: 10.1186/s13020-022-00641-4
108. Yang X, Sun J, Wen B, Wang Y, Zhang M, Chen W, et al. Biejiajian pill promotes the infiltration of CD8+ T cells in hepatocellular carcinoma by regulating the expression of CCL5. Front Pharmacol. (2021) 12. doi: 10.3389/fphar.2021.771046
109. Cheng C, Shou Q, Lang J, Jin L, Liu X, Tang D, et al. Gehua jiecheng decoction inhibits diethylnitrosamine-induced hepatocellular carcinoma in mice by improving tumor immunosuppression microenvironment. Front Pharmacol. (2020) 11. doi: 10.3389/fphar.2020.00809
110. Zhang JT, Chen HY, Li KX, Peng W, Yang RY, Zeng PH. Bioinformatic analysis and in vitro experimental verification of the intervention effect of shipi-xiaoji recipe on the progression of non-alcoholic fatty liver disease to liver cancer. Natural Product Commun. (2024) 19(4):1934578X241245020. doi: 10.1177/1934578X241245020
111. Chen C-T, Chen C-F, Lin T-Y, Hua W-J, Hua K, Tsai C-Y, et al. Traditional Chinese medicine Kuan-Sin-Yin decoction inhibits cell mobility via downregulation of CCL2, CEACAM1 and PIK3R3 in hepatocellular carcinoma cells. J Ethnopharmacology. (2023) 317:116834. doi: 10.1016/j.jep.2023.116834
112. Lan T, Chen B, Hu X, Cao J, Chen S, Ding X, et al. Tianhuang formula ameliorates liver fibrosis by inhibiting CCL2-CCR2 axis and MAPK/NF-κB signaling pathway. J Ethnopharmacology. (2024) 321:117516. doi: 10.1016/j.jep.2023.117516
113. Chen C, Yao X, Xu Y, Zhang Q, Wang H, Zhao L, et al. Dahuang Zhechong Pill suppresses colorectal cancer liver metastasis via ameliorating exosomal CCL2 primed pre-metastatic niche. J Ethnopharmacology. (2019) 238:111878. doi: 10.1016/j.jep.2019.111878
114. Zhang X, Liu X, Zhang Y, Yang A, Zhang Y, Tong Z, et al. Wan-nian-qing, a herbal composite prescription, suppresses the progression of liver cancer in mice by regulating immune response. Front Oncol. (2021) 11. doi: 10.3389/fonc.2021.696282
115. Hu R, Li J, Huang Q, Zhong X, Sun JL, Yi JY, et al. Qizhu anticancer prescription enhances immunosurveillance of liver cancer cells by regulating p21-dependent secretory phenotypes. J Ethnopharmacology. (2024) 333:118400. doi: 10.1016/j.jep.2024.118400
116. Lebedeva IV, Yang B, Pan C-S, Li Q, Yang Z, Long F-X, et al. Inhibitory effects of Chanling Gao on the proliferation and liver metastasis of transplanted colorectal cancer in nude mice. PloS One. (2019) 14(2):e0201504. doi: 10.1371/journal.pone.0201504
117. Chuang M-H, Chang JT, Hsu L-J, Jan M-S, Lu F-J. Antitumor activity of the chinese medicine JC-001 is mediated by immunomodulation in a murine model of hepatocellular carcinoma. Integr Cancer Therapies. (2016) 16:516–25. doi: 10.1177/1534735416664173
118. Bamodu OA, Kuo K-T, Wang C-H, Huang W-C, Wu ATH, Tsai J-T, et al. Astragalus polysaccharides (PG2) enhances the M1 polarization of macrophages, functional maturation of dendritic cells, and T cell-mediated anticancer immune responses in patients with lung cancer. Nutrients. (2019) 11(10):2264. doi: 10.3390/nu11102264
119. Wang M, Mao D, Li H. Chinese medicine jiedu huayu granules reduce liver injury in rats by regulating T-cell immunity. Evidence-Based Complementary Altern Med. (2019) 2019:1–8. doi: 10.1155/2019/1873541
120. Han ZY, Huang Q, Lv ML, Ma MQ, Zhang W, Feng WX, et al. Qizhu Anti-Cancer Recipe promotes anoikis of hepatocellular carcinoma cells by activating the c-Jun N-terminal kinase pathway. Heliyon. (2023) 9:e22089. doi: 10.1016/j.heliyon.2023.e22089
121. Zhang Z, Zeng X, Wu Y, Liu Y, Zhang X, Song Z. Cuproptosis-related risk score predicts prognosis and characterizes the tumor microenvironment in hepatocellular carcinoma. Front Immunol. (2022) 13. doi: 10.3389/fimmu.2022.925618
122. Llovet JM, De Baere T, Kulik L, Haber PK, Greten TF, Meyer T, et al. Locoregional therapies in the era of molecular and immune treatments for hepatocellular carcinoma. Nat Rev Gastroenterol Hepatology. (2021) 18:293–313. doi: 10.1038/s41575-020-00395-0
123. Xing Y, Liu Z-R, Yu W, Zhang H-Y, Song M-M. Risk factors for post-hepatectomy liver failure in 80 patients. World J Clin Cases. (2021) 9:1793–802. doi: 10.12998/wjcc.v9.i8.1793
124. Kuvshinoff BW, Ota DM. Radiofrequency ablation of liver tumors: Influence of technique and tumor size. Surgery. (2002) 132:605–12. doi: 10.1067/msy.2002.127545
125. Peng ZW, Fan WZ, Zhu BW, Wang GY, Sun JH, Xiao CJ, et al. Lenvatinib combined with transarterial chemoembolization as first-line treatment for advanced hepatocellular carcinoma: A phase III, randomized clinical trial (LAUNCH). J Clin Oncol. (2023) 41:117. doi: 10.1200/JCO.22.00392
126. Kudo M. Atezolizumab plus bevacizumab followed by curative conversion (ABC conversion) in patients with unresectable, TACE-unsuitable intermediate-stage hepatocellular carcinoma. Liver Cancer. (2022) 11:399–406. doi: 10.1159/000526163
127. Forner A, Reig M, Bruix J. Hepatocellular carcinoma. Lancet. (2018) 391:1301–14. doi: 10.1016/S0140-6736(18)30010-2
128. Anwanwan D, Singh SK, Singh S, Saikam V, Singh R. Challenges in liver cancer and possible treatment approaches. Biochim Biophys Acta (BBA) - Rev Cancer. (2020) 1873:188314. doi: 10.1016/j.bbcan.2019.188314
129. Liu Y, Cheng Y, Xu Y, Wang Z, Du X, Li C, et al. Increased expression of programmed cell death protein 1 on NK cells inhibits NK-cell-mediated anti-tumor function and indicates poor prognosis in digestive cancers. Oncogene. (2017) 36:6143–53. doi: 10.1038/onc.2017.209
130. Wang J, Qi F, Wang Z, Zhang Z, Pan N, Huai L, et al. A review of traditional Chinese medicine for treatment of glioblastoma. BioScience Trends. (2019) 13:476–87. doi: 10.5582/bst.2019.01323
131. Tilg H, Adolph TE, Tacke F. Therapeutic modulation of the liver immune microenvironment. Hepatology. (2023) 78:1581–601. doi: 10.1097/HEP.0000000000000386
132. Jia W, Wang L. Using traditional chinese medicine to treat hepatocellular carcinoma by targeting tumor immunity. Evidence-Based Complementary Altern Med. (2020) 2020:1–14. doi: 10.1155/2020/9843486
133. Long H, Wu Z. Immunoregulatory effects of Huaier (Trametes robiniophila Murr) and relevant clinical applications. Front Immunol. (2023) 14. doi: 10.3389/fimmu.2023.1147098
134. Lai WY, Mueller A. Latest update on chemokine receptors as therapeutic targets. Biochem Soc Trans. (2021) 49:1385–95. doi: 10.1042/BST20201114
135. Tavor S, Eisenbach M, Jacob-Hirsch J, Golan T, Petit I, BenZion K, et al. The CXCR4 antagonist AMD3100 impairs survival of human AML cells and induces their differentiation. Leukemia. (2008) 22:2151–8. doi: 10.1038/leu.2008.238
136. Shi Z, Song T, Wan Y, Xie J, Yan Y, Shi K, et al. A systematic review and meta-analysis of traditional insect Chinese medicines combined chemotherapy for non-surgical hepatocellular carcinoma therapy. Sci Rep. (2017) 7:4355. doi: 10.1038/s41598-017-04351-y
137. Gougelet A. Epigenetic modulation of immunity: towards new therapeutic avenues in hepatocellular carcinoma? Gut. (2019) 68:1727–8. doi: 10.1136/gutjnl-2019-319084
138. Morein D, Erlichman N, Ben-Baruch A. Beyond cell motility: the expanding roles of chemokines and their receptors in Malignancy. Front Immunol. (2020) 11. doi: 10.3389/fimmu.2020.00952
Keywords: chemokines, natural products, hepatocellular carcinoma, tumor microenvironment, immune intervention
Citation: Ruishi X, Linyi X, Yunfan B, Wenbo Y, Xiaoying Z, Xiaoxue F, Difu Z, Xintian L, Ming Z and Haoming L (2024) New perspectives on chemokines in hepatocellular carcinoma therapy: a critical pathway for natural products regulation of the tumor microenvironment. Front. Immunol. 15:1456405. doi: 10.3389/fimmu.2024.1456405
Received: 28 June 2024; Accepted: 26 July 2024;
Published: 14 August 2024.
Edited by:
Demin Cai, Yangzhou University, ChinaCopyright © 2024 Ruishi, Linyi, Yunfan, Wenbo, Xiaoying, Xiaoxue, Difu, Xintian, Ming and Haoming. This is an open-access article distributed under the terms of the Creative Commons Attribution License (CC BY). The use, distribution or reproduction in other forums is permitted, provided the original author(s) and the copyright owner(s) are credited and that the original publication in this journal is cited, in accordance with accepted academic practice. No use, distribution or reproduction is permitted which does not comply with these terms.
*Correspondence: Luo Haoming, bHVvLmhhb21pbmdAMTYzLmNvbQ==; Zhu Ming, emh1bWluZzEyNEAxNjMuY29t; Lan Xintian, bGFueGludGlhbjIwMjJAMTYzLmNvbQ==