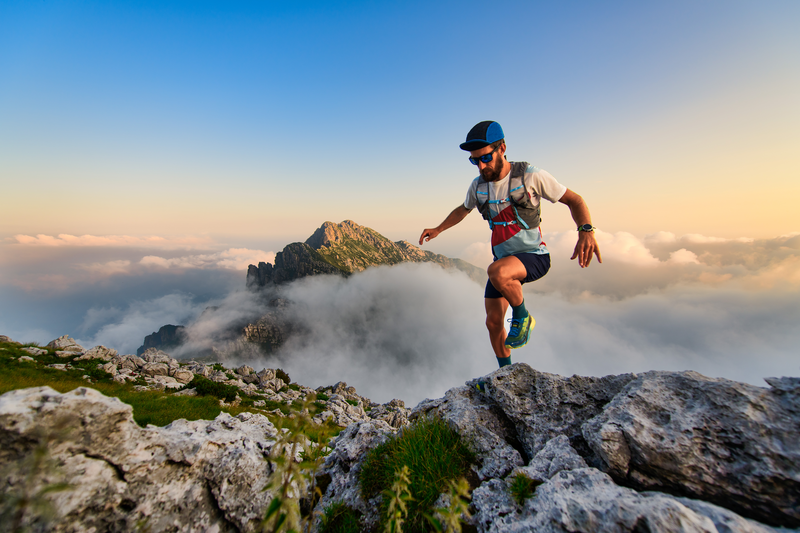
95% of researchers rate our articles as excellent or good
Learn more about the work of our research integrity team to safeguard the quality of each article we publish.
Find out more
MINI REVIEW article
Front. Immunol. , 17 September 2024
Sec. Cancer Immunity and Immunotherapy
Volume 15 - 2024 | https://doi.org/10.3389/fimmu.2024.1455469
This article is part of the Research Topic Community Series in Adenosine Pathways in Cancer Immunity and Immunotherapy: Volume II View all 5 articles
Purines and pyrimidines are signaling molecules in the tumor microenvironment that affect cancer immunity. The purinergic signaling pathways have been shown to play an important role in the development and progression of cancer. CD39 and CD73 are ectonucleotidases responsible for breaking down ATP or ADP into adenosine, which regulates immunosuppression in various types of cancer. These enzymes have been studied as a potential therapeutic target in immunotherapy, and recent research suggests a correlation between ectonucleotidases and clinical outcomes in cancer.Prostate cancer is the most diagnosed cancer in men, after non-melanoma skin tumors, and is the second leading cause of death in men in the world. Despite having long survival periods, patients often receive excessive or insufficient treatment. Within this complex landscape, the adenosine/CD73 pathway plays a crucial role. Therefore, this review aims to highlight new findings on the potential role of purinergic signaling in cancer treatment and emphasizes the importance of anti-CD73 as a pharmacological strategy for prostate cancer therapy.
Prostate cancer (PC) is the most frequently diagnosed urological neoplasm in males and it has high incidence today, being the second most common type of cancer and one of the leading causes of cancer-related death (1). Among the risk factors for the development of PC: are advanced age, family history, and genetic mutations such as BRCA1/2 mutations. According to a study published by the World Cancer Research Fund International, certain lifestyles such as excessive exposure to tobacco, obesity, a high-fat diet, and a sedentary lifestyle also contribute to unfavorable outcomes (2).
PC treatment is a challenge for biomedical sciences and has a great impact on public health, as it presents a high incidence and prevalence today (3, 4). Despite having long survival periods, patients often receive excessive or insufficient treatment due to inadequate risk stratification (5, 6).
The purinergic signaling pathways have been shown to play an important role in the development and progression of cancer (7). Purines and pyrimidines are signaling molecules in the tumor microenvironment that affect cancer immunity (8). Ectonucleotidases, such as CD39 and CD73, hydrolyze ATP or ADP into AMP and adenosine, respectively, regulating the nucleosides’ impact on immunosuppression in various tumor types (7). Besides, CD73 and CD39 are overexpression of several cancers (7, 9). Studies have suggested that the hydrolysis of nucleotides, which produce adenosine in the bloodstream, may be associated with prostate cancer progression and promote cancer cell growth (10). Targeting the ATP-adenosine pathway and CD39 reduces immunosuppressive adenosine accumulation and increases pro-inflammatory ATP levels (11).
Additionally, the role of CD73 has been investigated as a potential target for cancer immunotherapy (12). Monoclonal antibodies and small molecule inhibitors that interfere with the adenosine/CD73 pathway are being investigated in clinical trials (13). According to a previous study, it was demonstrated that there is a difference in the nucleotide hydrolysis profiles between patients with PC and healthy controls (10). However, further research is required to determine the association between purinergic signaling modulation and cancer. Understanding the factors that influence tumor progression and the correlation between CD39 and CD73 expression and clinical outcomes of prostate cancer is crucial. Therefore, establishing new strategies to improve the overall survival of PC patients is essential. In this review, we specifically focus on purinergic signaling, particularly anti-CD73, as a treatment for prostate cancer.
Purinergic signaling was initially proposed as a complex network that could regulate various cellular processes, such as proliferation, differentiation, and cell death (14). Later, the purinergic receptors were identified and classified into two groups: adenosine or P1 receptors and ATP or P2 receptors (15). The P1 receptors are G protein-coupled and can be further divided into four types (A1, A2A, A2B, and A3) based on their molecular structure, biochemistry, and pharmacology (14, 15). On the other hand, P2 receptors can be divided into P2Y receptors, which are activated by ATP and ADP and are also G protein-coupled. There are eight subunits of P2Y receptors (1, 2, 4, 6, 11, 12, 13, and 14) (14, 16). Additionally, there are P2X receptors, which are ATP-responsive ion channels that consist of trimeric forms of different subunits. The P2X subunits are classified from P2X1 to P2X7 according to their historical order of cloning, and each subunit has unique pharmacological and physiological properties (17, 18).
The ectonucleotidases play a crucial role in regulating the effects of extracellular nucleotides. These enzymes form a family that works in a highly coordinated manner to break down nucleotides and convert them into nucleosides, which is essential for extracellular purinergic regulation (15, 19). This family of enzymes includes the following members: Ecto-nucleoside triphosphate di phosphohydrolases (NTPDases –EC 3.6.1.5); Ecto-nucleoside pyrophosphatase/phosphodiesterases (NPPs – EC 3.6.1.9/EC 3.1.4.1); Alkaline Phosphatase (AP – EC 3.1.3.1); Ecto-5’-nucleotidase/CD73 (ecto-5’NT/CD73 - Ec 3.1.3.5). E-NTPDases hydrolyze ATP to ADP and ADP to AMP, while ecto-5’-nucleotidase acts on the conversion of AMP to ADO. Alkaline phosphatases hydrolyze monophosphate nucleotides and NPPs act in the extracellular catabolism of dinucleotides (20).
The NTPDase1 (E.C. 3.6.1.5) is also called CD39, while ecto-5’-nucleotidase can also be called CD73 (E.C. 3.1.3.5), as they are markers of B and T lymphocyte maturation. CD39 is a member of the NTPDase group and is situated on the surface of the plasma membrane (PM). It is responsible for the conversion of ATP or ADP into AMP extracellularly, which subsequently binds to purinergic system receptors, activating them. In contrast, CD73, also known as ecto-5’-nucleotidase, extracellularly dephosphorylates AMP to ADO, which has a pro-tumor effect and binds to P1 receptors (14, 15, 19, 20).
Maintaining a balance between ATP and ADO is crucial to prevent uncontrolled tissue destruction and excessive inflammatory response. In stressful situations such as inflammation, neoplasms, and ischemia, there is an accumulation of ATP in the extracellular environment (15, 21). However, in healthy tissues, the accumulation of extracellular nucleotides and nucleosides is insignificant except in the synaptic clefts or muscle interstitium during exercise (22). The accumulated extracellular ATP (eATP) can stimulate P2 purinergic receptors (P2X and P2Y), leading to the activation of immune cell inflammation activity (14). Meanwhile, the accumulated extracellular ADO (eADO) can bind to downstream P1 purinergic receptors, which have immunosuppressive effects in many tumor types, including urological tumors, primarily PC (23).
The tumor microenvironment (TME) is a dynamic environment characterized by biochemical and cellular features that play a crucial role in regulating the metabolism, proliferation, and dissemination of tumor cells (23, 24). The TME may exhibit both pro-inflammatory and pro-tumor characteristics, facilitating neoplastic progression. The biochemical composition of the TME results from interactions between host cells and tumor cells, leading to the release of damage-associated molecular patterns (DAMPs) and activation of innate immunity, including the recruitment of immune cells such as macrophages and dendritic cells through the NOD-like receptor (25). The TME is a complex milieu that includes cytokines, growth factors, cytotoxic molecules, proteases, and DAMPs. This environment significantly impairs the host’s immune system’s ability to combat the tumor (24, 25).
In TME, ATP is released through various mechanisms, such as passive efflux due to cell death, active transport via calcium channels, and microvesicles of plasma membranes (25). The TME is a crucial site for producing and releasing extracellular ATP (eATP) and adenosine (ADO), and this pathway’s modulatory actions have become a focal point for targeted cancer therapies (26, 27). The antineoplastic activity of ATP was first described by Rapaport (1983), who demonstrated that the addition of exogenous ATP caused cells to rest in the S cell cycle phase, and cancer cells were treated with ADO which promoted tumor growth (28).
Besides, neoplastic cells show high expression of CD39 as well as immune cells, particularly NK cells, Treg cells, macrophages, and tumor-specific effector T cells in TME (29). Tumor-associated macrophages co-express CD39 and P2X7 receptors, consequently the activation of these receptors by eATP stimulates specific actions in the inflammasome that lead to the release of cytosolic caspases (30). Including the release of microvesicles from monocytes and macrophages, resulting in the rapid release of interleukin-1β and interleukin-18 (31). An in vivo study has shown that eATP release promotes the rapid recruitment of neutrophils through the caspase activation mechanism, in addition to binding to P2Y2 receptors, enhancing this recruitment (32). Elevated CD39 expression promotes its pro-inflammatory actions by stimulating the regulatory function of Th17 cells, causing the exhaustion of CD8+ T cells, stimulating neutrophil chemotaxis, suppressing the function of NK cells, stimulating the presentation of dendritic cells (DC) and inhibiting platelet aggregation (33).
Moreover, targeting CD73 or A2A receptor (A2AR) is necessary for the activation of effector T cells and natural killer (NK) cells in the tumor. Through inhibition of A2BR or CD73, cancer cell survival is significantly hampered (34). Moreover, the understanding of the TME has led to the identification of metabolic pathways essential for tumor cell survival. Tumor hypoxia induces a wide network of metabolic and immunological changes that favor tumor growth and progression, with proangiogenic and immunosuppressive effects. The consequence is the promotion of a strong selection among tumor cells with an increase in their aggressiveness instead of an antitumor response (35). Figure 1 illustrates the mechanisms that encompass purinergic signaling, particularly the adenosine pathway, and their impact on the tumor microenvironment.
Figure 1. Promising mechanism of purinergic signaling pathway in cancer progression. ATP in TME is released by passive efflux due to cell death, so this ATP is converted into adenosine by upregulated CD39 and CD73. Adenosine promotes cancer progression by acting through P1 receptors (A1, A2A, A2B e A3) and activates the immunosuppressive action of Treg cells. This process enhances the production of pro-angiogenic factors (β-FGF, VEGF, and IL-8). So, the adenosine generated by CD39/CD73 expressed either on primary neoplastic cells or on cancer exosomes generates an immunosuppressive environment by acting on macrophages, neutrophils, dendritic cells, and T cells. IL-8, interleukin-8; VEGF, vascular endothelial growth factor; TGF- β, transforming growth factor beta.
CD73 is expressed in different body tissues and on various cell types, such as B lymphocytes, some T lymphocytes, endothelial, epithelial, and tumor cells (34). Tumor cells exhibit several evasion mechanisms to evade the surveillance of the immune system, mainly with the help of inhibitory immune cells and immunosuppressive cytokines. Among immunosuppressive cells, the actions of regulatory T cells (Tregs), macrophages, and natural killer cells (NK) stand out, which together have suppressed antitumor activities and consequent pro-tumor actions (35).
The expression of CD73 by such immune cells creates an immunosuppressive TME, which affects the basic functions of these cells, facilitating the development of tumor cells (36). The Treg cells in humans express low levels of CD73, but it has been documented that their expression may be elevated in cancer patients, consequently, inhibition of CD73 on Treg cells inhibits their immunosuppressive action (37). On the other hand, anergic or exhausted effector T cells exhibit high levels of CD73, consequently, the reduction of this expression in these cells leads to antitumor effects, with potentiation of their effector functions (38). It is already documented that NK cells in TME exhibit high expression of CD73, consequently, the ADO released from AMP dephosphorylation in TME suppresses the functions of NK cells, through the activation of A2A receptors, mainly (39).
Several studies have already shown that CD73 is overexpressed in various types of solid tumors, especially in more aggressive tumors with greater potential for metastasis (40). CD73-deficient mice are resistant to prostate carcinogenesis, suggesting that in the future therapies based on anti-CD73 monoclonal antibodies could dramatically reduce the growth of prostate tumor cells, inhibiting the process of metastasis and unfavorable outcomes (36). Therefore, its high expression in tumor cells is associated with a worse prognosis (36, 37).
However, CD73 can also be under-expressed and will not necessarily lead to a good prognosis. A study showed that when analyzing the expressions of CD73 in immunohistochemical studies of 136 patients with non-metastatic breast cancer and correlating with the clinical evolution, he noticed that patients with CD73 overexpression had a longer disease-free interval and longer global survival (41). Another study also demonstrated that the high expression of CD73 will lead to a better prognosis in patients with epithelial ovarian carcinoma (42). Lung cancer has high expressions of CD73, which promotes tumor growth via the EGFR pathway, consequently, these patients tend to be more resistant to chemotherapy in general (43). Regarding colon cancer, CD73 overexpression correlates with more aggressive behavior/metastasis and poor response to chemotherapy regimens containing 5-fluorouracil (44).
Many factors may contribute to the modulation of CD73 expression in tumor cells (40). Based on this theory, several studies confirmed that the use of APCP (a-b-methyl adenosine-5-diphosphatase), an unhydrolyzed analog of ADP, a selective inhibitor of CD73, inhibits cell proliferation, controlling the advance of the disease (45). Blocking CD73 using APCP has been associated with tumor regression, potentiation of the antitumor T-cell response, and increased survival in animal models (46).
Although blocking with anti-CD73 agents is not curative, inhibition of this pathway improves the antitumor activity of other treatments, such as those related to the CTLA-4 and PD-1 pathways (47). The expectation is that the combination of anti-PD-1 and anti-CD73, or A2A receptor antagonists, will result in more effective immune responses mediated by antitumor T cells (48). Another relevant data regarding CD73 is its solubility, so its activity can be evaluated by its expression in plasma (49). Patients with metastatic melanoma show high basal levels of CD73 expression in their plasma, but after starting treatment with nivolumab, they show a sharp drop, suggesting a possible marker of response to treatment (50).
It appears that anti-CD73 monoclonal antibody (mAb) treatment not only stops tumor growth but also limits the metastasis process through direct inhibition of tumor cell adhesion to endothelial cells. Therefore, the antitumor effects of anti-CD73 mAb or APCP are not limited to blocking CD73 enzymatic activity alone (36). On the other hand, the possible toxicity of anti-CD73 mAbs is still unknown, since there is the expression of CD73 in normal cells in various tissues, so its enzymatic action is also necessary for several physiological processes, which may be a limiting factor to its use (51). Interestingly, Morello et al. (2017) demonstrated that patients with metastatic melanoma who have higher CD73 activities have worse prognoses (50). Besides, researchers found that exosomal CD73 from patients with metastatic melanoma was associated with dampening T-cell activity and potentially influencing the effectiveness of anti-PD-1 immunotherapy (52). Targeting multiple immunosuppressive pathways can synergistically enhance anti-tumor immune responses (29). Inhibition of adenosine signaling has been shown to synergize with anti-PD-1 or anti-CTLA-4 mAbs in preclinical studies (47, 48).
As described in Table 1, 34 studies were found, including phases I, II, and III, of which 41% of the studies are only phase I, and 38.3% are phase II, while studies that include phases I and II are present in 17.7%. Only 3% of the studies are only phase III. Among these studies, lung cancer is the most researched clinical study, followed by breast cancer and prostate cancer. In the studies, combinations of different classes are tested together with the main agent, to verify the effectiveness of a combined therapy, the most frequent associations in the studies are, oleclumab, durvalumab, oleclumab + durvalumab and monalizumab (Table 1).
The purinergic system, which includes ATP and adenosine signaling pathways, has been shown to play an important role in the development and progression of prostate cancer. Studies have revealed that the activation of purinergic receptors, such as P2R, can enhance the proliferation, migration, and invasion of prostate cancer cells. Studies have shown that ATP can inhibit the growth of prostate cancer cells, likely through P2 receptors (53). As a result, many studies have described the antineoplastic effects of extracellular nucleotides and ectonucleotidases in different types of tumors (54, 55).
CD73 is an independent prognostic factor in prostate cancer, as the expression of CD73 in the prostate epithelium suppresses immunosurveillance by CD8+ T cells, while the expression of CD73 in the tumor stroma reduces NF-kB signaling in tumor cells via adenosine A2B receptor. CD73 expression, even on the normal adjacent prostate epithelium, can thus effectively discriminate between aggressive and indolent forms of prostate cancer (54).
The presence of tumor-infiltrating CD8+ T cells has been associated with a favorable prognosis in various types of cancer (55) and CD73 deficiency is associated with a significant reduction in prostate tumor growth and increased infiltration of CD8+ T cells so CD73 may be associated with prostate cancer progression and decreased antitumor immunity (36). The exosomes are a distinct population of extracellular vesicles, these exosomes CD73+ can directly impair the capacity of CD14+ monocytes to differentiate into functional dendritic cells (DC), thus having a potential effect on both antigen presentation and subsequent T cell responses (56). Consequently, the dominant effect of DC function by prostate cancer exosomes is immunosuppression and not antigen delivery (57).
Currently, there is a focus on understanding the impact of CD39 enzymatic function on immunity, and how therapeutic modulation of this pathway alters its functional potential within the tumor microenvironment (27). Increased levels of CD39 expression have been observed in various solid tumors (23), and extracellular vesicles derived from these cell types also contain CD39 and CD73 are believed to generate adenosine (58). However, further studies are needed to evaluate this association in the context of prostate cancer.
Some studies in patients with metastatic castrate-resistant prostate cancer (mCRPC) showed that the inhibition of the adenosine 2A receptor (A2AR) diminishes the immunosuppressive effects of adenosine and may complement immune-targeting drugs such as durvalumab and oleclumab anti-CD73. However, in this heavily pretreated population, AZD4635 with durvalumab or oleclumab demonstrated minimal antitumor activity. Safety profiles of the treatment combinations were manageable and consistent with the known profiles of the individual agents. They concluded that exploring alternative dosing in less heavily treated patients may be beneficial (59).
Most interestingly, synergistic effects of combination therapy associating CD39 inhibition with immune checkpoint blockade, MAPK inhibitors, or immunogenic chemotherapy were recently reported (60). Furthermore, Gardani et al. (10) reported that the hydrolysis of ATP, ADP, and AMP is increased in the plasma of patients diagnosed with PC. Higher levels of ATP hydrolysis were observed in patients with clinical stage IIA, compared to those with locally more advanced disease, such as in clinical stages IIB and III. In contrast, higher levels of AMP hydrolysis were observed in patients with stages IIB and III, suggesting that the coordinated action of CD39/CD73, phosphodiesterase, and alkaline phosphatase can modulate the immune system, creating a favorable environment for tumor development (10). Besides, another study investigated the expression of CD39 and CD73 enzymes as potential biomarkers in prostate cancer. By analyzing tissue and blood samples from PC patients, the findings suggest that CD39 and CD73 could serve as valuable biomarkers for prostate cancer, aiding in diagnosis and treatment strategies (61).
This review has focused on the role of purinergic signaling in cancer, especially in prostate cancer. We have discussed how modulations of the ectonucleotidases can potentially impact antitumor immunity. Prostate cancer is one of the most common neoplasms and one of the main causes of death in males worldwide. Most patients die due to complications caused by metastases; however, they have long survival periods.
Upon carefully reviewing the scenario of cancer, we recognize the significance of the purinergic system in this process. ATP and adenosine act synchronously, sometimes exhibiting anti-tumor and at other times pro-tumor actions. It is believed that modulating CD39 could have a substantial impact on antitumor immunity by acting in the ATP/ADO cascade and can act in two ways: initially preventing ATP phosphorylation and subsequently preventing the accumulation of ADO in the TME. It is crucial to correlate the actions of ectonucleotidases (CD39/CD73), as the conversion of eATP to AMP by CD39 leads to increased production of ADO, resulting from enhanced stimulation of the CD73 pathway.
However, regarding prostate cancer, we have not yet obtained sufficient data in the literature to conclusively define their behavior. Due to their critical roles as regulators of extracellular adenosine levels, ectonucleotidases are uniquely positioned to regulate cancer growth. Within this complex landscape, the adenosine/CD73 pathway plays a crucial role. Additionally, CD73 has been demonstrated to be an independent prognostic factor in prostate cancer, and several clinical trials using anti-CD73 immunotherapy are currently underway. There is also growing interest in the potential pharmacological manipulation of the adenosine/CD73 pathway in prostate cancer patients.
CG: Writing – original draft, Writing – review & editing, Data curation, Visualization. FD: Writing – original draft, Writing – review & editing, Visualization. LD: Writing – original draft, Data curation. LR: Writing – original draft, Writing – review & editing, Data curation. SL: Writing – review & editing, Visualization. FM: Conceptualization, Writing – original draft, Writing – review & editing, Data curation, Visualization.
The author(s) declare financial support was received for the research, authorship, and/or publication of this article. The authors thank Coordenação de Aperfeiçoamento de Pessoal de Nivel Superior – Brasil (CAPES, Finance Code 001) and Conselho Nacional de Desenvolvimento Científico e Tecnológico (CNPq, Project N° 314687/2021-1) CAPES - Catedra Tubingen (88881.915282/2023-01) for financial support.
The authors declare that the research was conducted in the absence of any commercial or financial relationships that could be construed as a potential conflict of interest.
All claims expressed in this article are solely those of the authors and do not necessarily represent those of their affiliated organizations, or those of the publisher, the editors and the reviewers. Any product that may be evaluated in this article, or claim that may be made by its manufacturer, is not guaranteed or endorsed by the publisher.
1. Siegel RL, Miller KD, Fuchs HE. Cancer statistics, 2022. CA Cancer J Clin. (2022) 72:7–33. doi: 10.3322/caac21708
2. Friedenreich CM, Ryder-Burbidge C, McNeil J. Physical activity, obesity and sedentary behavior in cancer etiology: epidemiologic evidence and biologic mechanisms. Mol Oncol. (2021) 15:790–800. doi: 10.1002/1878-0261.12772
3. Center MM, Jemal A, Lortet-Tieulent J, Ward E, Ferlay J, Brawley O, et al. International variation in prostate cancer incidence and mortality rates. Eur Urol. (2012) 61:1079–92. doi: 10.1016/j.eururo.2012.02.054
4. Wolf AM, Wender RC, Etzioni RB, Thompson IM, D’Amico AV, Volk RJ, et al. American Cancer Society guideline for early detection of prostate cancer: update 2010. CA Cancer J Clin. (2010) 60:70–98. doi: 10.3322/caac.20066
5. Preventive Services Task Force US, Grossman DC, Curry SJ, Owens DK, Bibbins-Domingo K, Caughey AB, et al. Screening for prostate cancer: US preventive services task force recommendation statement. JAMA. (2018) 319:1901–13. doi: 10.1001/jama.2018.3710
6. Andriole GL, Crawford ED, Grubb RL, Buys SS, Chia D, Church TR, et al. Mortality results from a randomized prostate cancer screening trial. N Engl J Med. (2009)0) 360:1310–9. doi: 10.1056/NEJMoa0810696
7. Di Virgilio F, Adinolfi E. Extracellular purines, purinergic receptors and tumor growth. Oncogene. (2017) 36:293–303. doi: 10.1038/onc.2016.206
8. Wu Hl, Gong Y, Ji P, Xie Y-F, Jiang Y-Z, Liu G-Y. Targeting nucleotide metabolism: a promising approach to enhance cancer immunotherapy. J Hematol Oncol. (2022) 15:45. doi: 10.1186/s13045-022-01263-x
9. Xia C, Yin S, To KKW, Xia C, Yin S, To KKW, et al. CD39/CD73/A2AR pathway and cancer immunotherapy. Mol Cancer. (2023) 22:44. doi: 10.1186/s12943-023-01733-x
10. Gardani CFF, Cappellari AR, Souza JB, da Silva BT, Engroff P, Moritz CEJ, et al. Hydrolysis of ATP, ADP and AMP is increased in blood plasma of prostate cancer patients. Purinergic Signal. (2019) 15:95–105. doi: 10.1007/s11302-018-9642-3
11. Liu Y, Li Z, Zhao X, Bi J, Li X-Y, Chen G, et al. Review immune response of targeting CD39 in cancer. biomark Res. (2023) 11:63. doi: 10.1186/s40364-023-00500-w
12. Scheffel TB, Grave N, Vargas P, Diz FM, Rockenbach L, Morrone FB. Immunosuppression in gliomas via PD-1/PD-L1 axis and adenosine pathway. Front Oncol. (2020) 10:617385. doi: 10.3389/fonc.2020.617385
13. Harvey JB, Phan LH, Villarreal OE, Bowser JL. CD73’s potential as an immunotherapy target in gastrointestinal cancers. Front Immunol. (2020) 11:508. doi: 10.3389/fimmu.2020.00508
14. Burnstock G. Purinergic signalling. Br J Pharmacol. (2006) Suppl 1):S172–81. doi: 10.1038/sj.bjp.0706429
15. Huang Z, Xie N, Illes P, Ulrich H, Semyanov A, Verkhratsky A, et al. From purines to purinergic signalling: molecular functions and human diseases. Sig Transduct Target Ther. (2021) 6:162. doi: 10.1038/s41392-021-00553-z
16. Morrone FB, Vargas P, Rockenbach L, Scheffel TB. P2Y12 purinergic receptor and brain tumors: implications on glioma microenvironment. Molecules. (2021) 26:6146. doi: 10.3390/molecules26206146
17. Di Virgilio F, Dal Ben D, Sarti AC, Giuliani AL, Falzoni S. The P2X7 receptor in infection and inflammation. Immunity. (2017) 47:15–31. doi: 10.1016/j.immuni.2017.06.020
18. White N, Butler PEM, Burnstock G. Human melanomas express functional P2X(7) receptors. Cell Tissue Res. (2005) 321:411–8. doi: 10.1007/s00441-005-1149-x
19. Zimmermann H, Zebisch M, Sträter N. Cellular function and molecular structure of ecto-nucleotidases. Purinergic Signal. (2012) 8:437–502. doi: 10.1007/s11302-012-9309-4
20. Stagg J, Smyth MJ. Extracellular adenosine triphosphate and adenosine in cancer. Oncogene. (2010) 29:5346–58. doi: 10.1038/onc.2010.292
21. Bastid J, Regairaz A, Bonnefoy N, Déjou C, Giustiniani J, Laheurte C, et al. Inhibition of CD39 enzymatic function at the surface of tumor cells alleviates their immunosuppressive activity. Cancer Immunol Res. (2015) 3:254–65. doi: 10.1158/2326-6066.CIR-14-0018
22. Forrester T. A case of serendipity*. Purinergic Signal. (2008) 4:93–100. doi: 10.1007/s11302-007-9090-y
23. Guo S, Han F, Zhu W. CD39 – a bright target for cancer immunotherapy. Biomed e Pharmacother. (2022) 151:113066. doi: 10.1016/j.biopha.2022.113066
24. McAllister SS, Weinberg RA. Tumor-host interactions: a far-reaching relationship. J Clin. (2010) 28:4022–8. doi: 10.1200/JCO.2010.28.4257
25. Corbet C, Feron O. Tumour acidosis: from the passenger to the driver’s seat. Nat Rev Cancer. (2017) 17:577–93. doi: 10.1038/nrc.2017.77
26. Baghbani E, Noorolyai S, Shanehbandi D, Mokhtarzadeh A, Aghebati-Maleki L, Shahgoli VK, et al. Regulation immune responses through CD39 and CD73 in cancer: Novel checkpoints. Life Sci. (2021) 282:119826. doi: 10.1016/j.lfs.2021.119826
27. Moesta AK, Li XY, Smyth MJ. Targeting CD39 in cancer. Nat Rev Immunol. (2020) 20:739–55. doi: 10.1038/s41577-020-0376-4
28. Rapaport E. Treatment of human tumor cell with ADP or ATP yields arrest of growth in the S phase of cell cycle. J Cell Physiol. (1983) 114:279–83. doi: 10.1002/jcp.1041140305
29. Allard B, Longhi MS, Robson SC, Stagg J. The ectonucleotidases CD39 and CD73: Novel checkpoint inhibitor targets. Immunol Rev. (2017) 276:121–44. doi: 10.1111/imr.12528
30. Li X, Moesta AK, Xiao C, Nakamura K, Nakamura K, Casey M, et al. Targeting CD39 in cancer reveals an extracellular ATP- and inflammasome- driven tumor immunity. Cancer Discovery. (2019) 9:1754–73. doi: 10.1158/2159-8290.CD-19-0541
31. MacKenzie A, Wilson HL, Kiss-Toth E, Dower SK, North RA, Surprenant A. Rapid secretion of interleukin 1β by microvesicle shedding. Immunity. (2001) 15:825–35. doi: 10.1016/s1074-7613(01)00229-1
32. Chen Y, Corriden R, Inoue Y, Yip L, Hashiguchi N, Zinkernagel A, et al. ATP release guides neutrophil chemotaxis via P2Y2 and A3 receptors. Science. (2006) 314:1792–5. doi: 10.1126/science.1132559
33. Zeng J, Ning Z, Wang Y, Xiong H. Implications of CD39 in immune-related diseases. Int Immunopharmacol. (2020) 89:107055. doi: 10.1016/j.intimp.2020.107055
34. Vijayan D, Young A, Teng MWL, Smyth MJ. Targeting immunosuppressive adenosine in cancer. Nat Rev Cancer. (2017) 17:765. doi: 10.1038/nrc.2017.110
35. Biswas SK. Metabolic reprogramming of immune cells in cancer progression. Immunity. (2015) 43:435–49. doi: 10.1016/j.immuni.2015.09.001
36. Stagg J, Beavis PA, Divisekera U, Liu MC, Möller A, Darcy PK, et al. CD73-deficient mice are resistant to carcinogenesis. Cancer Res. (2012) 72:2190–6. doi: 10.1158/0008-5472.CAN-12-0420
37. Di Gennaro P, Gerlini G, Caporale R, Sestini S, Brandani P, Urso C, et al. T regulatory cells mediate immunosuppression by adenosine in peripheral blood, sentinel lymph node and tils from melanoma patients. Cancer Lett. (2018) 417:124–30. doi: 10.1016/j.canlet.2017.12.032
38. Deng WW, Li YC, Ma SR, Mao L, Yu GT, Bu LL, et al. Specific blockade CD73 alters the “exhausted” phenotype of T cells in head and neck squamous cell carcinoma. Int J Cancer. (2018) 143:1494–504. doi: 10.1002/ijc.31534
39. Wang J, Matosevic S. Adenosinergic signaling as a target for natural killer cell immunotherapy. J Mol Med (Berl). (2018) 96:903–13. doi: 10.1007/s00109-018-1679-9
40. Chen S, Wainwright DA, Wu JD, Wan Y, Matei DE, Zhang Y, et al. CD73: an emerging checkpoint for cancer immunotherapy. Immunotherapy. (2019) 11:983–97. doi: 10.2217/imt-2018-0200
41. Supernat A, Markiewicz A, Welnicka-Jaskiewicz M, Seroczyńska B, Skokowski J MD, Sejda A, et al. CD73 expression as a potential marker of good prognosis in breast carcinoma. Appl Immunohistochem Mol Morphol. (2012) 20:103–7. doi: 10.1097/pai.0b013e3182311d82
42. Oh HK, Sin JI, Choi J, Park SH, Lee TS, Choi YS. Overexpression of CD73 in epithelial ovarian carcinoma is associated with better prognosis, lower stage, better differentiation, and lower regulatory t cell infiltration. J Gynecol Oncol. (2012) 23:274–81. doi: 10.3802/jgo.2012.23.4.274
43. Li J, Wang L, Chen X, Li L, Li Y, Ping Y, et al. CD39/CD73 upregulation on myeloid-derived suppressor cells via TGF- β-mTOR-HIF-1 signaling in patients with non-small cell lung cancer. Oncoimmunology. (2017) 6:e1320011. doi: 10.1080/2162402X.2017.1320011
44. Messaoudi N, Cousineau I, Arslanian E, Henault D, Stephen D, Vandenbroucke-Menu F, et al. Prognostic value CD73 expression in resected colorectal cancer liver metastasis. Oncoimmunology. (2020) 9:1746138. doi: 10.1080/2162402X.20201746138
45. Rockenbach L, Bavaresco L, Fernandes Farias P, Cappellari AR, Barrios CH, Bueno Morrone F, et al. Alterations in the extracellular catabolism of nucleotides are involved in the antiproliferative effect of quercetin in human bladder cancer T24 cells. Urol Oncol. (2013) 31:1204–11. doi: 10.1016/j.urolonc.2011.10.009
46. Jin D, Fan J, Wang L, Thompson LF, Liu A, Daniel BJ, et al. CD73 on tumor cells impairs antitumor T-cell responses: a novel mechanism of tumor-induced immune suppression. Cancer Res. (2010) 70:2245–55. doi: 10.1158/0008-5472.CAN-09-3109
47. Allard B, Pommey S, Smyth MJ, Stagg J. Targeting CD73 enhances the antitumor activity of anti-PD-1 and anti-CTLA-4 mAbs. Clin Cancer Res. (2013) 19:5626–35. doi: 10.1158/1078-0432.CCR-13-0545
48. Beavis PA, Milenkovski N, Henderson MA, John LB, Allard B, Loi S, et al. Adenosine receptor 2A blockade increases the efficacy of anti-PD-1 through enhanced antitumor T-cell responses. Cancer Immunol. (2015) 3:506–17. doi: 10.1158/2326-6066.CIR-14-0211
49. Vaara ST, Hollmén M, Korhonen AM, Maksimow M, Ala-Kokko T, Salmi M, et al. Soluble CD73 in critically ill septic patients - data from the prospective FINNAKI study. PloS One. (2016) 11:e0164420. doi: 10.1371/journal.pone.0164420
50. Morello S, Capone M, Sorrentino C Giannarelli D, Madonna G, Mallardo D, et al. Soluble CD73 as biomarker in patients with metastatic melanoma patients treated with nivolumab. J Transl Med. (2017) 15:244. doi: 10.1186/s12967-017-1348-8
51. Thompson LF, Eltzschig HK, Ibla JC, Van De Wiele CJ, Resta R, Morote-Garcia JC, et al. Crucial role for ecto-5′- nucleotidase (CD73) in vascular leakage during hypoxia. J Exp Med. (2004) 200:1395–405. doi: 10.1084/jem.20040915
52. Turiello R, Capone M, Morretta E, Monti MC, Madonna G, Azzaro R, et al. Exosomal CD73 from serum of patients with melanoma suppresses lymphocyte functions and is associated with therapy resistance to anti-PD-1 agents. J Immunother Cancer. (2022) 10:e004043. doi: 10.1136/jitc-2021-004043
53. Wang Z, Zhu S, Tan S, Zeng Y, Zeng H. The P2 purinoceptors in prostate cancer. Purinergic Signal. (2023) 19:255–63. doi: 10.1007/s11302-022-09874-2
54. Leclerc BG, Charlebois R, Chouinard G, Allard B, Pommey S, Saad F, et al. CD73 expression. Is an independent prognostic factor in prostate cancer. Clin Cancer Res. (2016) 22:158–66. doi: 10.1158/1078-0432.CCR-15-1181
55. Galon J, Angell HK, Bedognetti D, Marincola FM. The continuum of cancer immunosurveillance: prognostic, predictive, and mechanistic signatures. Immunity. (2013) 39:11–26. doi: 10.1016/j.immuni.2013.07.008
56. Valenti R, Huber V, Iero M, Filipazzi P, Parmiani G, Rivoltini L. Tumor-released microvesicles as vehicles of immunosuprression. Cancer Res. (2007) 67:2912–5. doi: 10.1158/0008-5472.CAN-07-0520
57. Salimu J, Webber J, Gurney M, Al-Taei S, Clayton A, Tabi Z. Dominant immunosuppression of dendritic cell function by prostate-cancer-derived exosomes. J Extracell Vesicles. (2017) 6:1368823. doi: 10.1080/20013078.2017.1368823
58. Clayton A, Al-Taei S, Webber J, Mason MD, Tabi Z. Cancer exosomes express CD39 and CD73, which suppress T cells through adenosine production. J Immunol. (2011) 187:676–83. doi: 10.4049/jimmunol.1003884
59. Lim E, Lim EA, Bendell JC, Falchook GS, Bauer TM, Drake CG, et al. Phase ia/b, open-label, multicenter study of AZD4635 (an adenosine A2A receptor antagonist) as monotherapy or combined with durvalumab, in patients with solid tumors. Clin Cancer Res. (2022) 28:4871–84. doi: 10.1158/1078-0432.CCR-22-0612
60. Zhang H, Vijayan D, Li XY, Robson SC, Geetha N, Teng MWL, et al. The role of NK cells and CD39 in the immunological control of tumor metastases. Oncoimmunology. (2019) 8:e1593809. doi: 10.1080/2162402X.2019.1593809
Keywords: adenosine, CD73, CD39, ectonucleotidases, purinergic system, prostate cancer
Citation: Gardani CFF, Diz FM, Dondé LB, Rockenbach L, Laufer S and Morrone FB (2024) The potential role of purinergic signaling in cancer therapy: perspectives on anti-CD73 strategies for prostate cancer. Front. Immunol. 15:1455469. doi: 10.3389/fimmu.2024.1455469
Received: 26 June 2024; Accepted: 12 August 2024;
Published: 17 September 2024.
Edited by:
Junjiang Fu, Southwest Medical University, ChinaReviewed by:
Fangmin Zhong, Nanchang University, ChinaCopyright © 2024 Gardani, Diz, Dondé, Rockenbach, Laufer and Morrone. This is an open-access article distributed under the terms of the Creative Commons Attribution License (CC BY). The use, distribution or reproduction in other forums is permitted, provided the original author(s) and the copyright owner(s) are credited and that the original publication in this journal is cited, in accordance with accepted academic practice. No use, distribution or reproduction is permitted which does not comply with these terms.
*Correspondence: Fernanda Bueno Morrone, ZmVybmFuZGEubW9ycm9uZUBwdWNycy5icg==; ZmJtb3Jyb25lQGdtYWlsLmNvbQ==
Disclaimer: All claims expressed in this article are solely those of the authors and do not necessarily represent those of their affiliated organizations, or those of the publisher, the editors and the reviewers. Any product that may be evaluated in this article or claim that may be made by its manufacturer is not guaranteed or endorsed by the publisher.
Research integrity at Frontiers
Learn more about the work of our research integrity team to safeguard the quality of each article we publish.