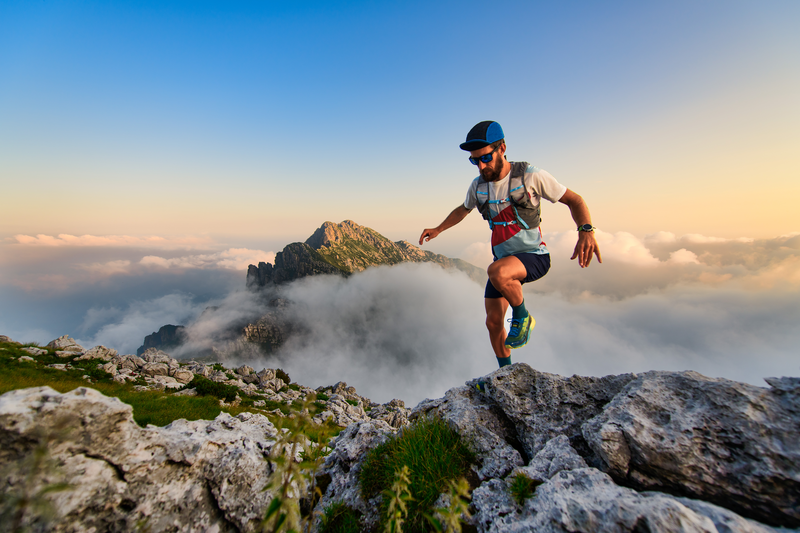
94% of researchers rate our articles as excellent or good
Learn more about the work of our research integrity team to safeguard the quality of each article we publish.
Find out more
ORIGINAL RESEARCH article
Front. Immunol. , 14 August 2024
Sec. Vaccines and Molecular Therapeutics
Volume 15 - 2024 | https://doi.org/10.3389/fimmu.2024.1451538
This article is part of the Research Topic Advancements in Understanding Zoonotic Parasitic Diseases View all 17 articles
Background: Echinococcus granulosus is a widespread zoonotic parasitic disease, significantly impacting human health and livestock development; however, no vaccine is currently available for humans. Our preliminary studies indicate that recombinant antigen P29 (rEg.P29) is a promising candidate for vaccine.
Methods: Sheep were immunized with rEg.P29, and venous blood was collected at various time points. Serum was isolated, and the presence of specific antibodies was detected using ELISA. We designed and synthesized a total of 45 B cell monopeptides covering rEg.P29 using the overlap method. ELISA was employed to assess the serum antibodies of the immunized sheep for recognition of these overlapping peptides, leading to the preliminary identification of B cell epitopes. Utilizing these identified epitopes, new single peptides were designed, synthesized, and used to optimize and confirm B-cell epitopes.
Results: rEg.P29 effectively induces a sustained antibody response in sheep, particularly characterized by high and stable levels of IgG. Eight B-cell epitopes of were identified, which were mainly distributed in three regions of rEg.P29. Finally, three B cell epitopes were identified and optimized: rEg.P2971-90, rEg.P29151-175, and rEg.P29211-235. These optimized epitopes were well recognized by antibodies in sheep and mice, and the efficacy of these three epitopes significantly increased when they were linked in tandem.
Conclusion: Three B-cell epitopes were identified and optimized, and the efficacy of these epitopes was significantly enhanced by tandem connection, which indicated the feasibility of tandem peptide vaccine research. This laid a solid foundation for the development of epitope peptide vaccine for Echinococcus granulosus.
Echinococcus granulosus is a zoonotic parasitic disease caused by the larvae of the Echinococcus tapeworm, which parasitizes animals, including humans. It is globally distributed and prevalent in regions like Eastern Europe, East Africa, the Middle East, and Central Asia, particularly in areas with advanced animal husbandry (1, 2). This disease not only poses a severe threat to human health but also adversely affects the development of animal husbandry, leading to substantial medical and economic losses (3–5). Vaccines are a crucial and effective method for the prevention and control of epidemics, offering benefits such as high safety, no residue, and no withdrawal period for animals (6). The main vaccine types researched for Echinococcus granulosus include traditional, genetically engineered, nucleic acid, and peptide vaccines. Peptide vaccines are immunogenic vaccines designed and synthesized based on the amino acid sequence of an epitope from a known or predicted effective protective antigen (7, 8). Their simplicity in preparation, relatively stable structure, and absence of infection risk makes them a focal point in new vaccine research.
Screening and identifying dominant epitopes are essential for developing epitope-based vaccines. Optimizing antigen screening at the epitope level can induce a more effective immune response, ensuring immune specificity and safety (9, 10). Our group successfully cloned and constructed the recombinant antigen P29 (rEg.P29) earlier, which induced superior cellular and humoral immune responses in mice and sheep, providing 96.6% and 94.8% immune protection, respectively. These findings suggest that rEg.P29 is a promising candidate vaccine against Echinococcus granulosus (11, 12). We conducted rEg.P29 epitope peptide vaccine studies in mice, identifying T-cell and B-cell epitopes (13, 14), that elicited strong cellular and humoral immune responses in mice (15). However, data on peptide vaccines for sheep, the most suitable hosts for Echinococcus granulosus, are lacking. Developing and promoting the rEg.P29 peptide vaccine for sheep holds significant practical value for disease prevention and control.
In this study, we designed and synthesized single amino acid peptides covering rEg.P29 using the overlap method. We used enzyme linked immunosorbent assay (ELISA) to detect antibody recognition of overlapping peptides and initially screened B-cell epitopes. Following this, new peptides were designed and synthesized, with the B cell epitopes being finalized through further optimization and characterization.
rEg.P29 was prepared using a recombinant expression plasmid stored in our laboratory, following the specific protocol previously described (16). Briefly, sterile LB liquid medium was prepared, containing 0.1 mM isopropyl β-D-thiogalactoside (IPTG, Invitrogen, Waltham, USA). The preserved strain was inoculated into the LB liquid medium and incubated at 37°C for 10 h. rEg.P29 was then purified using a Ni-NTA His-Tag purification kit (Merck, Kenilworth, USA), and finally the protein was eluted and dissolved by Elution Buffer containing urea, and endotoxins were eliminated with an endotoxin removal kit (Genscript, Nanjing, China). The endotoxin-free purified rEg.P29 underwent protein concentration assessment using a BCA kit (KeyGen Biotech, Nanjing, China), and protein purity was confirmed by sodium dodecyl sulfate polyacrylamide gel electrophoresis (SDS-PAGE).
Thirty-six female Chinese Yan chi Tan sheep, aged 4-6 months, were randomly divided into four groups. Each group received subcutaneous immunizations with primary and booster doses at 4-week intervals: PBS group (1 mL PBS), Quil A adjuvant group (1 mg Quil A, InvivoGen, San Diego, USA), rEg.P29 group (50 μg rEg.P29), and rEg.P29+Quil A group (50 μg rEg.P29 with 1 mg Quil A). Sheep peripheral blood was collected via the jugular vein at different time points, and serum was isolated. Fifteen 6–8-week-old female C57BL/6 mice were randomly divided into three groups: PBS group (1 mL PBS), Freund’s adjuvant group (Freund’s adjuvant), and rEg.P29+Freund’s adjuvant group (20 μg rEg.P29 with Freund’s adjuvant). Mice received booster immunizations two weeks after the initial dose, using Freund’s complete and incomplete adjuvants (Sigma-Aldrich, St. Louis, USA). Mice were anesthetized with sodium pentobarbital via tail vein injection for serum collection. Details of animal immunization and sample collection are illustrated in Figures 1A, B.
Figure 1. Animal immune grouping and epitope peptide design strategies. (A) Sheep (n=9/group) were immunized by subcutaneous injection in the abdomen, 4 weeks between initial and booster vaccination. Peripheral blood was collected at the designated times. (B) Mice were immunized subcutaneously via the abdomen, with an interval of 4 weeks between the initial and booster immunizations, and serum was collected two weeks after the booster immunization. (C) The 45 overlapping single peptides were designed with a length of 15 amino acids, a step of 5 amino acids and an overlap of 10 amino acids, covering 238 amino acids of rEg.P29.
Following the overlapping peptides design principle, each designed single peptide spanned 15 amino acids, with a step size of 5 amino acids and an overlap of 10 amino acids. We designed a total of 45 overlapping single peptides to encode the 238 amino acids of rEg.P29 (Figure 1C; Table 1). To aid in the screening of single peptides, three adjacent single peptides were mixed in equal proportions, resulting in 15 mixed peptide groups and one comprehensive mix of all single peptides. Sangon Biotech (Shanghai, China) synthesized the designed peptides with a purity of ≥ 98%, ensuring they were sterile and endotoxin-free. According to the buffer recommended in the peptide synthesis report, the peptides were dissolved in a 1 mg/mL solution and stored at -80°C for use.
rEg.P29 (recognition antibody, positive control), along with single or mixed peptides, were diluted to 5 μg/mL by carbonate buffer (pH 9.6) and incubated overnight at 4°C in enzyme-labeled plates for encapsulation. The plates were then washed five times with PBST (PBS with 0.05% Tween-20) and subsequently blocked with 5% skim milk powder for 2 h at 37°C. After washing, diluted sheep or mouse serum, serving as the primary antibody, was added to the plate, and incubated for 1 hour at 37°C. Horseradish peroxidase (HRP)-conjugated anti-sheep immunoglobulin G (IgG), IgM, IgA, IgE, IgG1, IgG2 (ABD Serotec, Kidlington, UK), or anti-mouse IgG, IgM, IgA, IgE, IgG1, IgG2a, IgG2b, IgG2c, and IgG3 (Abcam, Cambridge, USA) were added and incubated for another hour at 37°C. Following this, the plate was washed, and 3,3’,5,5’-Tetramethylbenzidine (TMB) was introduced. The reaction was terminated with 2 M H2SO4. Absorbance was measured at 450 nm using a Multiskan SkyHigh Microplate Spectrophotometer (Thermo Fisher Scientific, MA, USA).
Single- and mixed-peptide screening was conducted using sheep serum samples exhibiting the highest IgG antibody levels. Plates were coated with either peptides or rEg.P29, and the serum acted as the primary antibody. B-cell mixed peptides were identified by assessing IgG antibodies’ recognition using the ELISA method, as previously described. Subsequently, the corresponding single peptides of these mixed peptides were screened to pinpoint the B-cell epitopes.
Based on the locations and amino acid sequences of the initially identified epitopes, new peptides were methodically designed, optimized, and subsequently synthesized. ELISA plates were coated with these newly synthesized peptides alongside rEg.P29, employing the same ELISA procedure as described previously for the screening of B-cell epitopes. Ultimately, this process led to the identification and optimization of sheep B-cell epitopes.
Data analysis and graphing were conducted using the Statistical Package for the GraphPad Prism 8.0 graphing software (SPSS) version 22.0. Comparisons between two groups were executed using an unpaired t-test, while comparisons involving two or more groups employed one-way ANOVA. Data are presented as either mean or mean ± standard deviation (SD). P < 0.05 is considered statistically significant.
Analysis of serum antigen-specific antibodies in sheep at various time points post-immunization revealed that immunization with rEg.P29, particularly when supplemented with the adjuvant QuilA, elicited high levels of specific IgG, IgM, IgE, IgG1, and IgG2 (Figures 2A, B, D–F). A modest amount of IgA was also detected (Figure 2C), with IgG showing the highest and most rapid increase. Notably, immunization with rEg.P29 alone also induced some level of IgG production (Figure 2A). All antibody types demonstrated a rapid increase following immunization, reaching a peak two weeks post-booster immunization. Over time, antibody levels gradually declined, with IgA and IgM decreasing more rapidly compared to a slower decline in IgG.
Figure 2. Serum antibody expression in rEg.P29-immunized sheep. (A) Expression of serum specific antibody IgG. (B) Expression of serum specific antibody IgM. (C) Expression of serum specific antibody IgA. (D) Expression of serum specific antibody IgE. (E) Expression of serum specific antibody IgG1. (F) Expression of serum specific antibody IgG2. 0 weeks represents primary immunization. Results presented as mean ± SD (*P < 0.05; **P < 0.01; ***P < 0.001; ****P < 0.0001).
Sera collected at weeks 4, 6, and 8 were diluted from 1:1,000 to 1:256,000. Remarkably, even at a 256,000-fold dilution, high IgG titers were maintained (Figures 3A–C), particularly evident at week 6 (Figure 3B), which corresponds to two weeks post-booster immunization. At a 64,000-fold dilution of the week 6 serum, the levels of IgG subtypes IgG1 and IgG2 remained relatively high, with IgG1 levels surpassing those of IgG2 (Figures 3D–F). These findings strongly suggest that rEg.P29 effectively induces a sustained antibody response in sheep, particularly characterized by high and stable levels of IgG.
Figure 3. Serum IgG antibody titers determination in immunized sheep. (A) IgG antibody expression after doubling dilution of serum samples at week 4. (B) IgG antibody expression after doubling dilution of serum samples at week 6. (C) IgG antibody expression after doubling dilution of serum samples at week 8. (D) IgG1 antibody expression after doubling dilution of serum samples at week 6. (E) IgG2 antibody expression after doubling dilution of serum samples at week 6. (F) Comparison of IgG1 and IgG2 antibody expression after doubling dilution of serum samples at week 6. Results presented as mean ± SD (ns, P > 0.05; *P < 0.05; **P < 0.01; ***P < 0.001; ****P < 0.0001).
In this phase, fifteen pools of 3 epitope peptides (Table 1), each comprising three adjacent single peptides, were screened. Five pools of 3 epitope peptides were identified: ID13-15, ID16-18, ID31-33, ID34-36, and ID43-45 (Figure 4A). Notably, ID13-15 and ID16-18 elicited higher responses, significantly differing from the other pools of 3 epitope peptides. These five pools of 3 epitope peptides collectively encompass fifteen single peptides: ID13, ID14, ID15, ID16, ID17, ID18, ID31, ID32, ID33, ID34, ID35, ID36, ID43, ID44, and ID45. A subsequent screening was conducted on these 15 peptides.
Figure 4. Preliminary screening of B-cell mixed peptides and single peptides. (A) Screening of B-cell mixed peptides by recognition of IgG antibodies. “Mix” means pools of 15 epitope peptides. (B) Screening of B-cell monopeptides by recognition with IgG antibodies. Results presented as mean ± SD (*P < 0.05; **P < 0.01; ***P < 0.001; ****P < 0.0001).
Further analysis revealed that eight peptides were recognized in the immunoserum: ID15, ID16, ID31, ID32, ID33, ID43, ID44, ID45 (Figure 4B). These peptides correspond to the 71-85AA, 76-90AA, 151-165AA, 156-170AA, 161-175AA, 211-225AA, 216-230AA, and 221-235AA regions of rEg.P29, initially considered as potential linear B-cell epitopes. The identified epitopes were in three distinct regions of rEg.P29: ID15 and ID16 in the 71-90AA region, ID31, ID32, and ID33 in the 151-175AA region, and ID43, ID44, and ID45 in the 211-235AA region. The question arose whether these three regional peptides are more effective than the corresponding single peptides. To address this, further optimization, verification, and identification were undertaken.
New single peptides corresponding to the regions 71-90AA, 151-175AA, and 211-235AA were synthesized. Additionally, three peptides were created by tandemly connecting two regions each, with GSGSGS tandem sequences inserted between them. This process resulted in six new single peptides (P1-P6), as depicted in Figure 5A. Antibody recognition tests revealed that P1 (71-90AA), P2 (151-175AA), and P3 (211-235AA) demonstrated markedly enhanced recognition compared to their respective individual peptides within each region. Notably, P3 exhibited superior efficacy (Figures 5B, C). The number of amino acids increased when P1, P2, and P3 were linked in tandem, enhancing their recognition beyond the pre-tandem levels. However, no significant difference in recognition was observed among the three tandem peptides (P4, P5, and P6), as shown in Figure 5B. Consequently, epitope peptides P1, P2, and P3 were identified as the three principal B-cell epitopes. It was also observed that the efficacy of these three epitopes significantly increased when they were linked in tandem.
Figure 5. Optimized design strategies and identification of B-cell epitopes. (A) Three new single peptides were designed and connected in series two by two with GSGSGS in the middle. (B) Optimization and identification of B-cell epitopes by IgG antibody recognition (Statistical analysis was made within and between groups). (C) Doubling dilution determination of IgG antibodies to six B epitopes. Data from 9 sheep, results presented as mean ± SD (****P < 0.0001).
The recognition of six single peptides, P1-P6, by IgM, IgA, IgE, IgG1, and IgG2 antibodies in sheep serum was observed. The results indicated that these peptides could not recognize IgM and IgA antibodies (Figures 6A, B), but they effectively recognized IgE, IgG1, and IgG2 antibodies. Notably, peptides P4, P5, and P6 showed superior recognition effects compared to P1, P2, and P3, with P5 demonstrating the most significant recognition impact on the three antibodies (Figures 6C–E).
Figure 6. Recognition of sheep antibodies by optimized B cell epitopes. (A) Recognition of lgM by B cell epitopes. (B) Recognition of lgA by B cell epitopes. (C) Recognition of lgE by B cell epitopes. (D) Recognition of lgG1 by B cell epitopes. (E) Recognition of lgG2 by B cell epitopes. Results presented as mean ± SD (ns, P > 0.05; **P < 0.01; ****P < 0.0001).
Similarly, the interaction of these six single peptides, P1-P6, with various antibodies in mouse serum was examined. The findings revealed that they could recognize IgG, IgM, IgG1, and IgG2b antibodies (Figures 7A, B, E, G), but failed to recognize IgA, IgE, and IgG3 antibodies (Figures 7C, D, I). Peptides P4, P5, and P6 exhibited enhanced recognition effects compared to P1, P2, and P3. Additionally, P4, P5, and P6 were able to recognize IgG2a and IgG2c antibodies, unlike P1, P2, and P3 (Figures 7F, H). These results demonstrate that the three B-cell epitopes, P4, P5, and P6, optimized through this study, can effectively recognize antibodies in both sheep and mice, qualifying them as dominant B-cell epitopes.
Figure 7. Recognition of mouse antibodies by optimized B cell epitopes. (A) Recognition of lgG by B cell epitopes. (B) Recognition of lgM by B cell epitopes. (C) Recognition of lgA by B cell epitopes. (D) Recognition of lgE by B cell epitopes. (E) Recognition of lgG1 by B cell epitopes. (F) Recognition of lgG2a by B cell epitopes. (G) Recognition of lgG2b by B cell epitopes. (H) Recognition of lgG2c by B cell epitopes. (I) Recognition of lgG3 by B cell epitopes. Results presented as mean ± SD (ns, P > 0.05; *P < 0.05; **P < 0.01; ***P < 0.001; ****P < 0.0001).
Antigen-specific antibodies are crucial in antiparasitic infections and serve as a key index for evaluating humoral immunity (17, 18). Post-immunization of sheep with rEg.P29, the serum exhibited high titers of IgG, IgG1, and IgG2 antibodies, with levels rapidly increasing after booster immunization and remaining elevated until the 20th week. In earlier stages, our group employed rEg.P29 in conjunction with Freund’s complete/incomplete adjuvant for sheep immunization and infection (12), which is consistent with the current research results. Lalramhluna et al. infected two types of sheep with Haemonchus contortus and noted a significant increase in IgG1 and IgG2 levels in the serum of resistant sheep, indicating a more robust humoral immune response (19). Valizadeh et al. used the envelope antigen extracted from live Protocercaria for sheep immunization and observed a notable elevation in IgG antibody titer in the immunized group (20). Heath et al. established the correlation between IgG antibody levels and immune protection in organisms (21). The high and sustained levels of IgG, IgG1, and IgG2 antibodies induced by the vaccine are vital for resistance to parasitic infections (22, 23), which is corroborated by the antibody responses observed in this study. These findings suggest that rEg.P29 is effective in inducing humoral immune responses in sheep.
On the surface of an antigenic molecule, certain specific chemical groups determine the antigen’s specificity. These groups are known as antigenic determining groups or antigenic epitopes (24, 25), which act as functional units for antigen-receptor binding and play various roles in eliciting humoral and cellular immune responses (26, 27). In vaccine-induced protective immune responses, antigen-specific epitopes are predominantly involved, with the body’s immune response primarily targeting antigen-dominant epitopes (28, 29). Peptide vaccines, based on antigenic epitopes, are crucial in disease prevention (30, 31). Identifying antigenically dominant epitopes with protective effects is fundamental for developing epitope-based peptide vaccines, making the study of antigenic epitopes a critical methodology. The overlapping synthetic peptide method is commonly used for identifying cellular epitopes (32, 33), which is adopted in this study.
Peptide vaccines designed to effectively elicit humoral and/or cellular immune responses must incorporate epitopes capable of triggering the desired immune reaction. B-cell epitopes are typically categorized as either linear or conformational epitopes (34, 35). Due to the challenges in identifying conformational epitopes and the widespread use of linear epitopes, the latter have garnered more attention. In dogs, fine-grained Echinococcus granulosus tapeworm infections are chiefly mediated by antibody-specific B-cell antibodies (36), making protective B-cell epitope peptides critical for peptide vaccine development (37). Researchers have conducted extensive studies on echinococcosis peptide vaccines, focusing primarily on informatics analysis and the epitope peptides of the Eg95 gene. Woollard et al. synthesized four epitope peptides of Eg95, demonstrating their strong immunogenicity in inducing IgG1 and IgG2 antibodies in sheep. However, these peptides did not confer immune protection in sheep, indicating a need for further investigation into the mechanism of immune protection by epitope peptides (38). Esmaelizad et al. integrated five T-cell epitopes into a multicell epitope antigen, which induced mice achieved a protection rate of 99.6% (39). Currently, peptide vaccines for echinococcosis remain in the stages of informatics prediction and laboratory validation (40–42), with no mature peptide vaccines available yet for the prevention and treatment of echinococcosis. Our efforts are directed towards developing a multi-epitope peptide vaccine with effective immune-protective properties.
In this study, we screened and optimized B-cell epitopes by examining the interaction of overlapping peptides with specific IgG antibodies using ELISA. The dominant B-cell epitopes were identified as rEg.P2971-90 (P1), rEg.P29151-175 (P2), and rEg.P29211-235 (P3). It was confirmed that these dominant B-cell epitopes could recognize sheep-specific IgE, IgG1, and IgG2 antibodies, but they did not bind to specific IgM and IgA antibodies. This may be attributed to the relatively low levels of these two antibodies in the serum. When the three dominant epitopes were linked in tandem, their peptide recognition efficacy significantly exceeded that of the individual dominant epitopes. This improved recognition is likely due to the broader range of epitopes presented by a larger number of amino acids, suggesting the potential for developing multi-epitope peptide vaccines. Moreover, various peptides and peptides in tandem also identified mouse-specific IgG, IgM, IgG1, and IgG2b antibodies. Additionally, the tandem peptides were able to recognize mouse-specific IgG2a and IgG2c. This indicates that the B-cell epitopes screened and identified using sheep might also be effectively applicable in mice.
Screening of the few peptides identified did produce high OD values, but we must be concerned that the coating efficiency of peptides affects the results of peptide identification. This requires us to use certain methods to make the peptide encapsulation as homogeneous as possible, such as the use of labelling and antibody capture methods. Inhibition experiments can be performed to control the binding of rEg.P29 antibody bound to the enzyme labelled plate, allowing better control of the encapsulation efficiency. Antibodies that are highly reactive to peptides in ELISA are not necessarily neutralizing antibodies and may not be immunoprotective. At the same time, some of the peptides identified by the screen may be aggregated, resulting in obtaining peptides that may not be the results we desire. Through the mouse animal model, our research group screened and confirmed the dominant epitopes of T cells and B cells of rEg.P29. Immunizing mice with combined epitopes produced strong humoral and cellular immune effects, especially B cell epitopes (15). The protective effect of combined epitope vaccine on mouse infection model is being studied. Screening and identifying peptides with good immunoprotective effects is our goal. The binding of peptide-inducing antibodies to natural rEg.P29 would be a good indicator of potential efficacy. rEg.P29 is known to bind tightly to lipids, which may affect its ability to bind to peptide-inducing antibodies. This provides a better reference for our subsequent studies. Therefore, combined with the results of the mouse animal model, in the follow-up study, we will carry out the peptide vaccine protection effect study targeting B-cell epitopes.
Three dominant B-cell epitopes of rEg.P29 were successfully identified: rEg.P2971-90, rEg.P29151-175, and rEg.P29211-235. The efficacy of these epitopes was notably enhanced through tandem linkage, indicating the feasibility of conducting research on tandem peptide vaccines. This advancement lays a solid groundwork for the development of epitope-based peptide vaccine.
The original contributions presented in the study are included in the article/supplementary material. Further inquiries can be directed to the corresponding authors.
The animal study was approved by Ethics Committee of Ningxia Medical University. The study was conducted in accordance with the local legislation and institutional requirements.
JY: Writing – original draft, Writing – review & editing. YL: Writing – original draft. YZZ: Writing – original draft. JS: Writing – original draft. MZ: Writing – review & editing. CW: Writing – review & editing. YF: Writing – original draft. WZ: Writing – review & editing. YQZ: Writing – review & editing.
The author(s) declare financial support was received for the research, authorship, and/or publication of this article. The authors declare that the research and publication of this article was supported by the School Scientific Research Projects of Ningxia Medical University (No. XT2023027), the Key R&D Projects of Ningxia Hui Autonomous Region (No. 2019BCG01001), and the National Natural Science Foundation of China (31960708).
We thank for the Ningxia Medical University Science and Technology Center and Ningxia Key Laboratory of Prevention and Control of Common Infectious Diseases providing the research conditions for this study. In addition, we thank Editage for providing language editing work.
The authors declare that the research was conducted in the absence of any commercial or financial relationships that could be construed as a potential conflict of interest.
All claims expressed in this article are solely those of the authors and do not necessarily represent those of their affiliated organizations, or those of the publisher, the editors and the reviewers. Any product that may be evaluated in this article, or claim that may be made by its manufacturer, is not guaranteed or endorsed by the publisher.
1. Woolsey ID, Miller AL. Echinococcus granulosus sensu lato and Echinococcus multilocularis: A review. Res Vet Sci. (2021) 135:517–22. doi: 10.1016/j.rvsc.2020.11.010
2. Sanchez L, Mayta H, Jara LM, Verástegui M, Gilman RH, Gómez-Puerta LA, et al. Echinococcus granulosus sensu stricto and E. canadensis are distributed in livestock of highly endemic area in the Peruvian highlands. Acta Trop. (2022) 225:106178. doi: 10.1016/j.actatropica.2021.106178
3. Larrieu E, Gavidia CM, Lightowlers MW. Control of cystic echinococcosis: Background and prospects. Zoonoses Public Health. (2019) 66:889–99. doi: 10.1111/zph.12649
4. Wen H, Vuitton L, Tuxun T, Li J, Vuitton DA, Zhang W, et al. Echinococcosis: advances in the 21st century. Clin Microbiol Rev. (2019) 32:e00075–18. doi: 10.1128/CMR.00075-18
5. Hou X, Shi Y, Kang X, Rousu Z, Li D, Wang M, et al. Echinococcus granulosus: The establishment of the metacestode in the liver is associated with control of the CD4(+) T-cell-mediated immune response in patients with cystic echinococcosis and a mouse model. Front Cell Infect Microbiol. (2022) 12:983119. doi: 10.3389/fcimb.2022.983119
6. Guo M, Liu X, Chen X, Li Q. Insights into new-onset autoimmune diseases after COVID-19 vaccination. Autoimmun Rev. (2023) 22:103340. doi: 10.1016/j.autrev.2023.103340
7. Pourseif MM, Moghaddam G, Daghighkia H, Nematollahi A, Omidi Y. A novel B- and helper T-cell epitopes-based prophylactic vaccine against Echinococcus granulosus. BioImpacts: BI. (2018) 8:39–52. doi: 10.15171/bi.2018.06
8. Zhao X, Zhang F, Li Z, Wang H, An M, Li Y, et al. Bioinformatics analysis of EgA31 and EgG1Y162 proteins for designing a multi-epitope vaccine against Echinococcus granulosus. Infect Genet Evol. (2019) 73:98–108. doi: 10.1016/j.meegid.2019.04.017
9. Manavalan B, Govindaraj RG, Shin TH, Kim MO, Lee G. iBCE-EL: A new ensemble learning framework for improved linear B-cell epitope prediction. Front Immunol. (2018) 9:1695. doi: 10.3389/fimmu.2018.01695
10. Gong W, Pan C, Cheng P, Wang J, Zhao G, Wu X. Peptide-based vaccines for tuberculosis. Front Immunol. (2022) 13:830497. doi: 10.3389/fimmu.2022.830497
11. Shi Z, Wang Y, Li Z, Li Z, Bo Y, Ma R, et al. Cloning, expression, and protective immunity in mice of a gene encoding the diagnostic antigen P-29 of Echinococcus granulosus. Acta Biochim Biophys Sin (Shanghai). (2009) 41:79–85. doi: 10.1093/abbs/gmn009
12. Wang H, Li Z, Gao F, Zhao J, Zhu M, He X, et al. Immunoprotection of recombinant Eg.P29 against Echinococcus granulosus in sheep. Vet Res Commun. (2016) 40:73–9. doi: 10.1007/s11259-016-9656-7
13. Lv Y, Zhu Y, Chang L, Yang J, Zhao Y, Zhao J, et al. Identification of a dominant murine T-cell epitope in recombinant protein P29 from Echinococcus granulosus. Acta Biochim Biophys Sin (Shanghai). (2022) 54:482–93. doi: 10.3724/abbs.2022036
14. Lv Y, Li S, Zhang T, Zhu Y, Tao J, Yang J, et al. Identification of B-cell dominant epitopes in the recombinant protein P29 from Echinococcus granulosus. Immunity Inflamm Dis. (2022) 10:e611. doi: 10.1002/iid3.611
15. Lv Y, Chang L, Yang J, Wen J, Zhao Y, Zhu M, et al. Immunogenicity of peptide-based vaccine composed of epitopes from Echinococcus granulosus rEg.P29. FASEB J. (2023) 37:e22819. doi: 10.1096/fj.202201636R
16. Wang C, Yang SH, Niu N, Tao J, Du XC, Yang JH, et al. lncRNA028466 regulates Th1/Th2 cytokine expression and associates with Echinococcus granulosus antigen P29 immunity. Parasites vectors. (2021) 14:295. doi: 10.1186/s13071-021-04795-2
17. Xu W, Guo L, Dong X, Li X, Zhou P, Ni Q, et al. Detection of viruses and mycoplasma pneumoniae in hospitalized patients with severe acute respiratory infection in northern China, 2015-2016. Jpn J Infect Dis. (2018) 71:134–9. doi: 10.7883/yoken.JJID.2017.412
18. Tas JMJ, Koo JH, Lin YC, Xie Z, Steichen JM, Jackson AM, et al. Antibodies from primary humoral responses modulate the recruitment of naive B cells during secondary responses. Immunity. (2022) 55:1856–71.e6. doi: 10.1016/j.immuni.2022.07.020
19. Lalramhluna M, Bordoloi G, Pandit S, Baidya S, Joardar SN, Patra AK, et al. Parasitological and immunological response to Haemonchus contortus infection: Comparison between resistant Garole and susceptible Sahabadi sheep. Vet Parasitol Reg Stud Rep. (2020) 22:100477. doi: 10.1016/j.vprsr.2020.100477
20. Valizadeh M, Haghpanah B, Badirzadeh A, Roointan E, Fallahi S, Raeghi S. Immunization of sheep against Echinococcus granulosus with protoscolex tegumental surface antigens. Vet World. (2017) 10:854–8. doi: 10.14202/vetworld.2017.854-858
21. Heath DD, Koolaard J. Serological monitoring of protection of sheep against Echinococcus granulosus induced by the EG95 vaccine. Parasite Immunol. (2012) 34:40–4. doi: 10.1111/j.1365-3024.2011.01341.x
22. Petrone L, Vanini V, Petruccioli E, Ettorre GM, Schininà V, Busi Rizzi E, et al. Polyfunctional specific response to echinococcus granulosus associates to the biological activity of the cysts. PloS Negl Trop Dis. (2015) 9:e0004209. doi: 10.1371/journal.pntd.0004209
23. Li ZD, Mo XJ, Yan S, Wang D, Xu B, Guo J, et al. Multiplex cytokine and antibody profile in cystic echinococcosis patients during a three-year follow-up in reference to the cyst stages. Parasites vectors. (2020) 13:133. doi: 10.1186/s13071-020-4003-9
24. Schroeder SM, Nelde A, Walz JS. Viral T-cell epitopes - Identification, characterization and clinical application. Semin Immunol. (2023) 66:101725. doi: 10.1016/j.smim.2023.101725
25. Xiang Z, Lu J, Rao S, Fu C, Yao Y, Yi Y, et al. Programming peptide-oligonucleotide nano-assembly for engineering of neoantigen vaccine with potent immunogenicity. Theranostics. (2024) 14:2290–303. doi: 10.7150/thno.93395
26. Tang ZM, Tang M, Zhao M, Wen GP, Yang F, Cai W, et al. A novel linear neutralizing epitope of hepatitis E virus. Vaccine. (2015) 33:3504–11. doi: 10.1016/j.vaccine.2015.05.065
27. Nhàn NTT, Yamada T, Yamada KH. Peptide-based agents for cancer treatment: current applications and future directions. Int J Mol Sci. (2023) 24:12931. doi: 10.3390/ijms241612931
28. Akram A, Inman RD. Immunodominance: a pivotal principle in host response to viral infections. Clin Immunol. (2012) 143:99–115. doi: 10.1016/j.clim.2012.01.015
29. Dehghankhold M, Sadat Abolmaali S, Nezafat N, Mohammad Tamaddon A. Peptide nanovaccine in melanoma immunotherapy. Int Immunopharmacol. (2024) 129:111543. doi: 10.1016/j.intimp.2024.111543
30. Wellhausen N, O’Connell RP, Lesch S, Engel NW, Rennels AK, Gonzales D, et al. Epitope base editing CD45 in hematopoietic cells enables universal blood cancer immune therapy. Sci Transl Med. (2023) 15:eadi1145. doi: 10.1126/scitranslmed.adi1145
31. Chen C, Zhang N, Li M, Guo A, Zheng Y, Humak F, et al. Recombinant bacteriophage T4 displaying key epitopes of the foot-and-mouth disease virus as a novel nanoparticle vaccine. Int J Biol Macromol. (2024) 258:128837. doi: 10.1016/j.ijbiomac.2023.128837
32. Jiao C, Wang B, Chen P, Jiang Y, Liu J. Analysis of the conserved protective epitopes of hemagglutinin on influenza A viruses. Front Immunol. (2023) 14:1086297. doi: 10.3389/fimmu.2023.1086297
33. Xi J, Yao L, Li S. Identification of β-conglycinin α’ subunit antigenic epitopes destroyed by thermal treatments. Food Res Int. (2021) 139:109806. doi: 10.1016/j.foodres.2020.109806
34. Lundin SB, Kann H, Fulurija A, Andersson B, Nakka SS, Andersson LM, et al. A novel precision-serology assay for SARS-CoV-2 infection based on linear B-cell epitopes of Spike protein. Front Immunol. (2023) 14:1166924. doi: 10.3389/fimmu.2023.1166924
35. Polyiam K, Ruengjitchatchawalya M, Mekvichitsaeng P, Kaeoket K, Hoonsuwan T, Joiphaeng P, et al. Immunodominant and neutralizing linear B-cell epitopes spanning the spike and membrane proteins of porcine epidemic diarrhea virus. Front Immunol. (2021) 12:785293. doi: 10.3389/fimmu.2021.785293
36. Zhang W, Ross AG, McManus DP. Mechanisms of immunity in hydatid disease: implications for vaccine development. J Immunol. (2008) 181:6679–85. doi: 10.4049/jimmunol.181.10.6679
37. Sharma M, Dixit A. Immune response characterization and vaccine potential of a recombinant chimera comprising B-cell epitope of Aeromonas hydrophila outer membrane protein C and LTB. Vaccine. (2016) 34:6259–66. doi: 10.1016/j.vaccine.2016.10.064
38. Woollard DJ, Heath DD, Lightowlers MW. Assessment of protective immune responses against hydatid disease in sheep by immunization with synthetic peptide antigens. Parasitology. (2000) 121:145–53. doi: 10.1017/s0031182099006186
39. Esmaelizad M, Ahmadian G, Aghaiypour K, Shamsara M, Paykari H, Tebianian M. Induction of prominent Th1 response in C57Bl/6 mice immunized with an E. coli-expressed multi T-cell epitope EgA31 antigen against Echinococcus granulosus. Folia Parasitol (Praha). (2013) 60:28–34. doi: 10.14411/fp.2013.004
40. Li Y, Zhu Y, Sha T, Chen Z, Yu M, Zhang F, et al. A multi-epitope chitosan nanoparticles vaccine of canine against echinococcus granulosus. J BioMed Nanotechnol. (2021) 17:910–20. doi: 10.1166/jbn.2021.3065
41. Yu M, Zhu Y, Li Y, Chen Z, Sha T, Li Z, et al. Design of a novel multi-epitope vaccine against echinococcus granulosus in immunoinformatics. Front Immunol. (2021) 12:668492. doi: 10.3389/fimmu.2021.668492
Keywords: Echinococcus granulosus, sheep, recombinant antigen P29, B cell epitopes, vaccine
Citation: Yang J, Lv Y, Zhu Y, Song J, Zhu M, Wu C, Fu Y, Zhao W and Zhao Y (2024) Optimizing sheep B-cell epitopes in Echinococcus granulosus recombinant antigen P29 for vaccine development. Front. Immunol. 15:1451538. doi: 10.3389/fimmu.2024.1451538
Received: 19 June 2024; Accepted: 01 August 2024;
Published: 14 August 2024.
Edited by:
Rodrigo Morchón García, University of Salamanca, SpainReviewed by:
Alfonso Balmori-de la Puente, University of Salamanca, SpainCopyright © 2024 Yang, Lv, Zhu, Song, Zhu, Wu, Fu, Zhao and Zhao. This is an open-access article distributed under the terms of the Creative Commons Attribution License (CC BY). The use, distribution or reproduction in other forums is permitted, provided the original author(s) and the copyright owner(s) are credited and that the original publication in this journal is cited, in accordance with accepted academic practice. No use, distribution or reproduction is permitted which does not comply with these terms.
*Correspondence: Wei Zhao, d2Vpemhhb0BueG11LmVkdS5jbg==; Yinqi Zhao, NDYwNjQ3MzA5QHFxLmNvbQ==
†These authors have contributed equally to this work
Disclaimer: All claims expressed in this article are solely those of the authors and do not necessarily represent those of their affiliated organizations, or those of the publisher, the editors and the reviewers. Any product that may be evaluated in this article or claim that may be made by its manufacturer is not guaranteed or endorsed by the publisher.
Research integrity at Frontiers
Learn more about the work of our research integrity team to safeguard the quality of each article we publish.