- 1Department of General Surgery, The Third Hospital of Mianyang, Sichuan Mental Health Center, Mianyang, Sichuan, China
- 2School of Pharmacy, Southwest Medical University, Luzhou, Sichuan, China
- 3Department of Pharmacy, The Third Hospital of Mianyang, Sichuan Mental Health Center, Mianyang, Sichuan, China
Immunotherapy has profoundly changed the treatment of gastric cancer, but only a minority of patients benefit from immunotherapy. Therefore, numerous studies have been devoted to clarifying the mechanisms underlying resistance to immunotherapy or developing biomarkers for patient stratification. However, previous studies have focused mainly on the tumor microenvironment. Systemic immune perturbations have long been observed in patients with gastric cancer, and the involvement of the peripheral immune system in effective anticancer responses has attracted much attention in recent years. Therefore, understanding the distinct types of systemic immune organization in gastric cancer will aid personalized treatment designed to pair with traditional therapies to alleviate their detrimental effects on systemic immunity or to directly activate the anticancer response of systemic immunity. Herein, this review aims to comprehensively summarize systemic immunity in gastric cancer, including perturbations in systemic immunity induced by cancer and traditional therapies, and the potential clinical applications of systemic immunity in the detection, prediction, prognosis and therapy of gastric cancer.
1 Introduction
Although its incidence has decreased in recent decades, gastric cancer (GC) remains the most common cause of cancer-related death worldwide, especially in regions with high Helicobacter pylori infection, such as East Asia, South America and the Middle East (1). In 2022, both the number of new cases of GC worldwide and the number of GC-related deaths ranked fifth, with estimated values of 968350 and 659853, respectively (2). Except in some countries and regions with well-established screening programs, such as Japan, Korea and some areas in China, the majority of GC patients are diagnosed at late stages, leading to dismal long-term survival (3).
Traditionally, the main strategy for curing GC is radical gastrectomy, and in some cases, chemotherapy and/or radiotherapy are needed to reduce recurrence. However, in unresectable advanced patients, systemic chemotherapy combined with targeted therapy is the standard treatment, aiming to prolong survival and improve quality of life (3). The participation of the immune system in tumorigenesis has long been heavily investigated, resulting in the impressive success of immunotherapy in the past decade. Immunotherapy, including immune checkpoint inhibitors (ICIs), cell-based therapy and vaccines, has revolutionized cancer therapy, and several recently published phase 3 clinical trials have proven the encouraging effects of immunotherapy in patients with GC in first- or late-line settings (4–7). Nevertheless, patients who achieve a durable response are limited, while the majority of patients with GC are primarily or secondarily resistant to immunotherapy. Therefore, numerous efforts have been devoted to elucidating the mechanisms underlying the responsiveness of GC to immunotherapy. However, most of these studies are limited to the tumor microenvironment (TME), such as programmed cell death-ligand 1 (PD-L1) expression, the tumor neoantigen load and the profile of infiltrating immune cells (8, 9).
Although perturbations in systemic immunity have long been observed in cancer patients, how they influence the progression of tumors and the effects of cancer therapies, especially immunotherapy, have not received much attention until recently (10). Inspired by these findings, this review aimed to focus on systemic immunity in GC. We first summarize the perturbations of systemic immunity induced by GCs and then outline the effects of traditional therapies, including radical gastrectomy and chemotherapy, on systemic immunity. Finally, we address the potential clinical applications of systemic immunity in the detection, prediction, prognosis and therapy of GC.
2 Perturbations in systemic immunity induced by GC
Through the disruption of hematopoiesis or direct effects on peripheral immune cells, both human cancers and animal tumor models have been shown to induce extensive perturbations in systemic immunity, manifesting as alterations in circulating cytokines, the expansion of immunosuppressive myeloid populations and a decrease in immune cells with antitumor ability (11, 12). Progenitors with myeloid differentiation potential have been found to increase in the bone marrow of mouse models, leading to elevated frequencies of neutrophils and monocytes, along with reductions in dendritic cell (DC) and lymphocyte populations, which can be reversed by resection of cancer or cytokine blockage, suggesting that circulating cytokines secreted by cancer cells drive the remodeling of systemic immunity (12). Currently, no studies have investigated the changes in hematopoiesis in bone marrow induced by GCs; however, numerous studies have reported perturbations in cytokines and major immune lineages in peripheral blood (Figure 1; Supplementary Table S1).
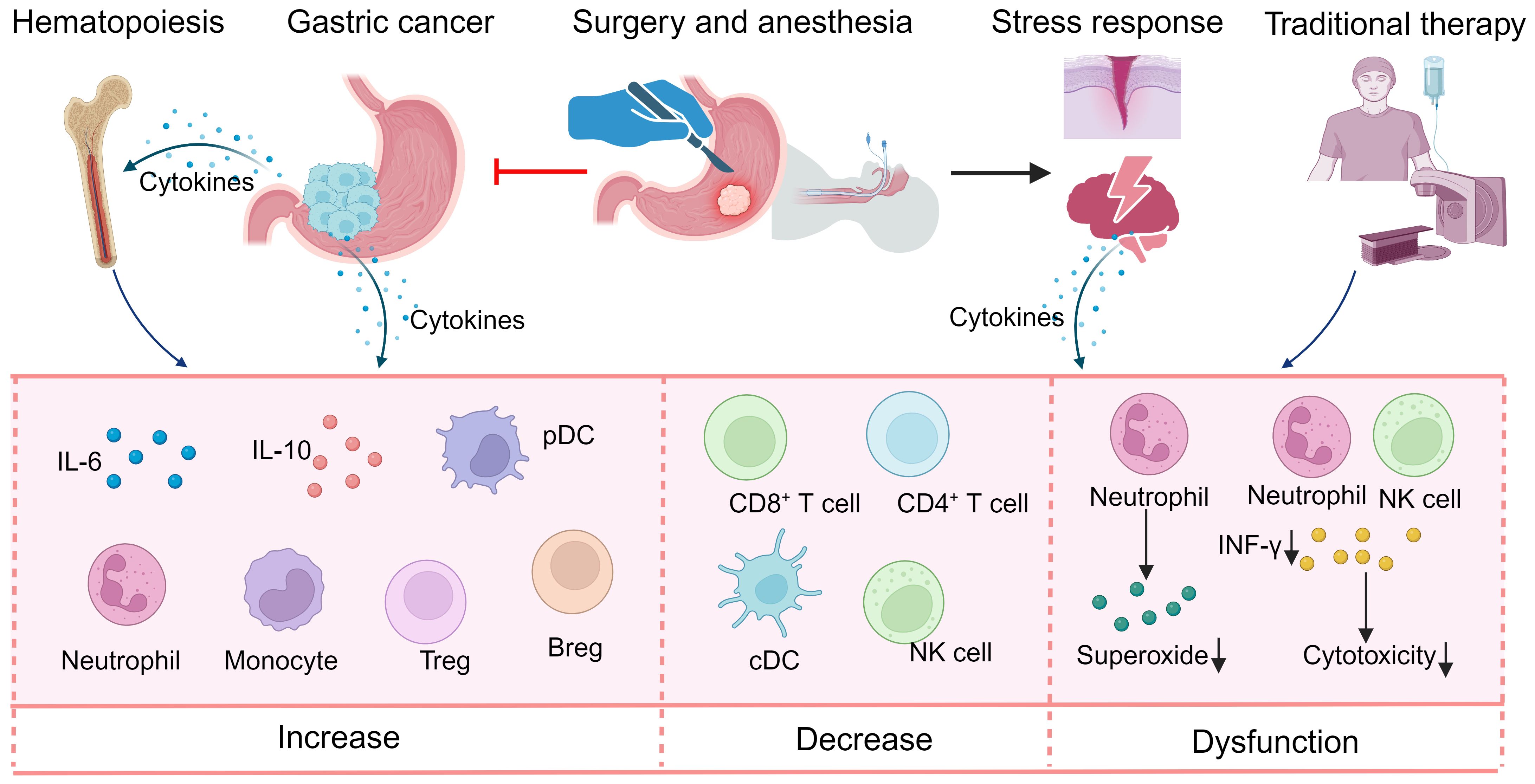
Figure 1. Perturbations in systemic immunity in gastric cancer. Gastric cancer itself and traditional therapy can induce reorganization of systemic immunity, manifesting most prominently in alterations in circulating cytokines, expansion of immunosuppressive myeloid populations and a decrease in immune cells with antitumor ability. Breg, regular B cell; cDC, classical dendritic cell; INF, interferon; NK, natural killer; pDC, plasmacytoid dendritic cell; Treg, regular T cell.
2.1 Circulating cytokines
Cytokines are a collection of molecules that participate in almost every step of tumorigenesis and immunology (13). Numerous studies have investigated the perturbations of circulating cytokines in GC patients (14). In general, the concentrations of cytokines, including interleukin-1β (IL-1β), IL-6, IL-10, IL-17, interferon-γ (IFN-γ) and tumor necrosis factor-α (TNF-α), in the peripheral blood of GC patients are commonly greater than those in healthy subjects, whereas the concentration of macrophage chemotactic protein (MCP)-1 has been found to be lower in several studies (15–19). However, cytokines in the circulation can be secreted by nearly all cells, including immune cells and GC cells themselves, and their levels are strongly affected by various factors, such as age, sex, lifestyle and genetic background (20–23). Therefore, inconsistent findings are common across studies, and accurately interpreting the perturbations in circulating cytokines induced by GCs is difficult. For individual cytokines, the peripheral changes in GC patients may be context dependent rather than a regular phenomenon. For example, although IL-6 is one of the most common cytokines whose peripheral levels are increased in GC patients, several studies have not shown significant differences between GC patients and controls (16, 24–26). Furthermore, the serum levels of several cytokines are associated with clinicopathological features. For example, IL-6 levels are elevated only in intestinal GC patients, whereas MCP-1 levels are lower only in diffuse GC patients (16). Nevertheless, a general finding is that the degree of alterations in peripheral cytokines increases with disease progression (21, 27, 28).
2.2 Myeloid lineages
Several cancers have been demonstrated to promote hematopoiesis toward monocytic and granulocytic lineages through cancer-derived factors (10). Although such hematopoietic alterations have not been validated in GC, many studies have reported that both neutrophils and monocytes are extensively perturbed in GC patients. However, these perturbations are commonly represented by ratios between different immune cells, while studies on their functions are limited. In general, patients with GC have a greater percentage of neutrophils in the peripheral blood than healthy donors do, and with increasing tumor burden, the percentage of neutrophils in the periphery significantly increases (29, 30). Although disparities in phenotype and function have been found between neutrophils obtained from cancer tissues and peripheral blood, these differences between neutrophils from GC patients and those from healthy subjects have not been addressed (29). In terms of function, peripheral neutrophils from GC patients exhibit normal phagocytic activity but reduced superoxide generation (31). Furthermore, a subset of myeloid-derived suppressor cells (MDSCs), which highly express neutrophil markers, is dramatically increased in the circulation of GC patients and has the ability to suppress the activity of CD8+ T cells (32). In addition, neutrophil extracellular traps (NETs), one of the main contributors to the cancer-promoting ability of neutrophils, were found to be more abundant in the blood of GC patients, especially those with late-stage disease (33, 34). Another cell type of myeloid origin, monocytes, was also found to be increased in the peripheral blood of GC patients with decreased chemotactic responsiveness and upregulated T-cell immunoglobulin and mucin-domain containing-3 (TIM-3) expression, which may be an important mechanism in GC progression (35, 36). In addition to these findings, few studies have investigated GC-induced dysfunction of neutrophils and monocytes. As both neutrophils and monocytes are composed of heterogeneous cell populations with pro- and anticancer abilities, determining their alterations is important for clinical applications.
Dendritic cells, which participate in antigen presentation and T-cell priming and proliferation, are critical orchestrators of innate and adaptive immunity in cancer (37). Therefore, perturbation of DCs from the peripheral circulation has long been observed in many cancers, including GC (10). Compared with healthy individuals, patients with GC have lower circulating DC counts and percentages, with reduced cytotoxicity and TNF-α, IL-2 and CD40 expression, indicating impaired function and immature status (38, 39). However, similar to other immune cells, DCs are composed of various heterogeneous subsets, including plasmacytoid DCs (pDCs), classical DCs (cDCs) and inflammatory DCs, which play different roles in human diseases (40). Therefore, despite the overall decrease in peripheral DCs, subsets with tumor-promoting effects, such as pDCs and DC-10 cells, were found to be elevated in the periphery of GC patients (41–43). In patients with GC, the frequency and mean fluorescence intensity of DC-10 in the peripheral blood are dramatically increased and strongly associated with tumor grade (41). The levels of other tolerogenic DCs, pDCs, are also significantly increased in the blood of GC patients, and these pDCs are proposed to be recruited to the TME through chemokine receptor 9 (CCR9) and C-C motif chemokine (CCL25) interactions, leading to an immunosuppressive microenvironment in GC (42). Therefore, subsets and functional states should be taken into consideration when peripheral DCs are utilized for clinical application.
2.3 Lymphoid lineage
As the main participants in antitumor immunity, lymphocytes have long been the focus of cancer immunology. In terms of peripheral immune cells in GC, substantially more studies have investigated alterations in lymphocytes. Despite a few inconsistent findings, lymphopenia is common in GC patients (15, 44). However, circulating lymphocytes are composed of complicated subsets with both cancer-promoting and cancer-inhibiting activities. Therefore, in addition to the total lymphocyte population, individual subsets have also been extensively studied. CD8+ T cells are the main effector cells involved in tumor cell killing, and their functions are strongly impaired in the peripheral blood of GC patients (45, 46). Under some conditions, CD8+ T cells can be induced by GCs to express IL-10, PD-1 and TIM-3, which inhibit the effector function of CD8+ T cells (46, 47). In contrast, the levels of suppressive lymphocytes, such as regulatory T (Treg) cells, regulatory B (Breg) cells and IL-17-producing T cells, are typically greater in GC patients than in normal controls (48–52). These cell types synergize with each other to establish an immunosuppressive environment in GC patients. For example, increased Breg cells in the blood of GC patients inhibited the production of T-cell cytokines and converted T cells to Treg cells, leading to immune escape in GC (52).
Natural killer (NK) cells are a type of innate immune cell that differentiates from common lymphoid progenitors and participates in cancer immunosurveillance through direct cancer cell killing and orchestrates the functions of other players in the immune system (53). The phenotype of circulating NK cells from GC patients differed from that from healthy controls, characterized by a decrease in the number of NK cells expressing activating molecules, including NKp30, NKp46, NKG2D and DNAM-1, and an increase in the number of NK cells expressing the inhibitory molecules KIR3DL1 and TIM-3; these perturbations are significantly associated with cancer progression (54–56). Additionally, the anticancer capacity of these NK cells has been shown to be impaired in GC patients, manifesting as decreased IFN-γ production and cytotoxic function (21, 57, 58).
2.4 Indices derived from multiple immune components
The human immune system is an intricate network with complex synergistic and/or antagonistic interactions among individual immune components. Therefore, various indices derived from multiple immune components have been established to reflect the peripheral immune state more precisely. Owing to their low cost and noninvasive accessibility, numerous studies have investigated alterations in these indices in patients with GC. In general, compared with healthy individuals, patients with cancer present with a greater neutrophil-to-lymphocyte ratio (NLR), platelet-to-lymphocyte ratio (PLR), and systemic immune-inflammation index (SII), and lower lymphocyte-to-monocyte ratio (LMR), and the severity of perturbations increases with disease progression (15, 59–61).
Overall, these findings strongly indicate that systemic immunity, which results from the effects of cancer cells and participates in the progression of cancer, is reduced in GC patients. Further efforts are warranted to fully clarify the systemic immune landscape of patients with GC and its associations with disease stage and patient demographics. The mechanisms underlying these perturbations are also largely unknown in GC, and elucidating these mechanisms is critical for therapeutic development.
3 Changes in systemic immunity during traditional therapy for GC
Traditional therapies for GC, including surgery, chemotherapy, and radiotherapy, have long been known to positively or negatively affect systemic immunity, which may determine the efficacy of treatment and oncological outcomes (Supplementary Table S2). In contrast, although targeted therapy has been developed as a standardized treatment for GC in various settings and research on its combination with immunotherapy has been increasing, few studies have been conducted to investigate the effects of targeted therapy on systemic immunity in patients with GC. Elucidating the changes in systemic immunity during traditional GC therapy is critical for optimizing these strategies to strengthen rather than impair anticancer immunity.
3.1 Radical gastrectomy and perioperative events
Radical gastrectomy is the main method for achieving complete disease control and long-term disease-free survival in GC patients. As mentioned above, systemic immune perturbations are induced mainly by various factors derived from the primary tumor; therefore, radical gastrectomy can eliminate these factors and restore normal peripheral immunity, which has been demonstrated in breast and colon cancer (12). However, wound healing and the stress response following gastrectomy also have detrimental effects on systemic immunity.
After radical gastrectomy, wound healing programs remodel systemic immunity, characterized by elevated circulating IL-2, IL-6, IL-10, TNF-α and IFN-γ, ultimately driving peripheral immune cells to immunosuppressive states (62–64). In addition to gastrectomy, perioperative events, including anesthesia, analgesia, postoperative complications, intraoperative blood loss and blood transfusion, all activate or prolong the surgical stress response, leading to the activation of neural signaling and systemic inflammation (65). More extended surgery and an eventful postoperative course are associated with elevated serum catecholamines, which have been shown to suppress anticancer immunity (66). Therefore, the peripheral IL-6 concentration is greater in GC patients who have undergone longer operations (62). In patients who underwent gastrectomy in combination with splenectomy, the T-cell subsets were decreased, and their functions were significantly suppressed (64). The number and cancer cell-killing potential of NK cells are also decreased by abdominal laparotomy, which leads to lung metastasis (67). In addition to the direct suppression of anticancer effector immune cells, surgical stress also increases the levels of immune inhibitory molecules and cells in the periphery. For example, in mouse models, gastrectomy can induce the accumulation of γδT cells in mesenteric lymph nodes, which suppresses the cell-mediated response by transforming growth factor-β (TGF-β) (68). Although some studies have shown that increased proinflammatory cytokines return to normal levels immediately after gastrectomy, long-term functional suppression of immune cells in the blood has been demonstrated in breast cancer models (62, 63, 69). Collectively, these findings suggest that gastrectomy and perioperative events can induce systemic immune perturbations.
3.2 Cytotoxic therapy
The majority of chemotherapeutics and radiation kill cancer cells through direct damage and induction of apoptosis. Although the latter mechanism may cause immunogenic death, which enhances anticancer immunity, acute cancer cell death and the stress response of cells within the TME release various proinflammatory molecules into the circulation to modulate the function of peripheral immune cells (70). Currently, although chemotherapy and radiotherapy are known to cause granulopenia and lymphopenia in clinical practice, studies investigating the effects of cytotoxic therapy on systemic immunity in GC patients are scarce. Few studies have suggested that following chemotherapy, the levels of immune-enhancing cytokines, including IL-2, IL-4, IL-10, and IFN-γ, decrease in the serum of GC patients (15, 71). Nevertheless, the most commonly used cytotoxic drugs in GC, such as oxaliplatin and 5-fluorouracil (5-FU), were found to reorganize systemic immunity in patients with cancer. For example, oxaliplatin induces the systemic release of ectoenzyme-expressing extracellular vesicles (EVs) from B cells and ATP from cancer cells, leading to the production of adenosine, which contributes to CD8+ T cell dysfunction (72). In addition, in response to 5-FU, circulating proinflammatory factors secreted by myeloid and CD4+ T cells promote tumor progression (73, 74). Whether these impairments also occur in patients with GC needs further clinical study.
4 Potential for clinical application
4.1 Roles of systemic immunity in cancer progression
Multiple decades of research have demonstrated that the immune system has both cancer-promoting and cancer-inhibiting functions, the process of which is referred to as cancer immunoediting and comprises three phases: elimination, equilibrium and escape (75). However, the conceptual development has been based mainly on the TME, whereas the role of systemic immunity has been less considered. For successful cancer cell elimination by natural and therapeutically induced anticancer immunity, intact peripheral immunity is a critical determinant, as the majority of steps of the tumor-immune cycle, including tumor antigen presentation, effector cell priming, proliferation and trafficking, occur outside the TME (76). Therefore, when the progression of effector cells is blocked or cancer drainage lymph nodes are resected, immunotherapeutic efficacy is abrogated (77, 78). On the other hand, through various secreted factors, many cancers can disrupt hematopoiesis extensively and drive circulating immune cells toward accomplices to facilitate tumor progression (10). In addition to preresident cells, the majority of cancer-infiltrated immune components circulate from the periphery and participate in the development of an immunosuppressive TME. For example, a strong association was observed between the levels of peripheral and intratumoral neutrophils, indicating that the expanded immature neutrophils in the peripheral blood of GC patients also infiltrate cancer tissues, resulting from the high expression of molecules involved in neutrophil recruitment and plasticity modulation (79). Furthermore, perturbed systemic immunity is involved in many steps of the cancer invasion-metastasis cascade. For example, cancer-edited immune cells induce the formation of premetastatic niches that are conducive to the survival and proliferation of cancers before their arrival (80). During trafficking in the circulation, cancer cells are protected and supported by many immune components, including neutrophils and platelets (81, 82) (Figure 2).
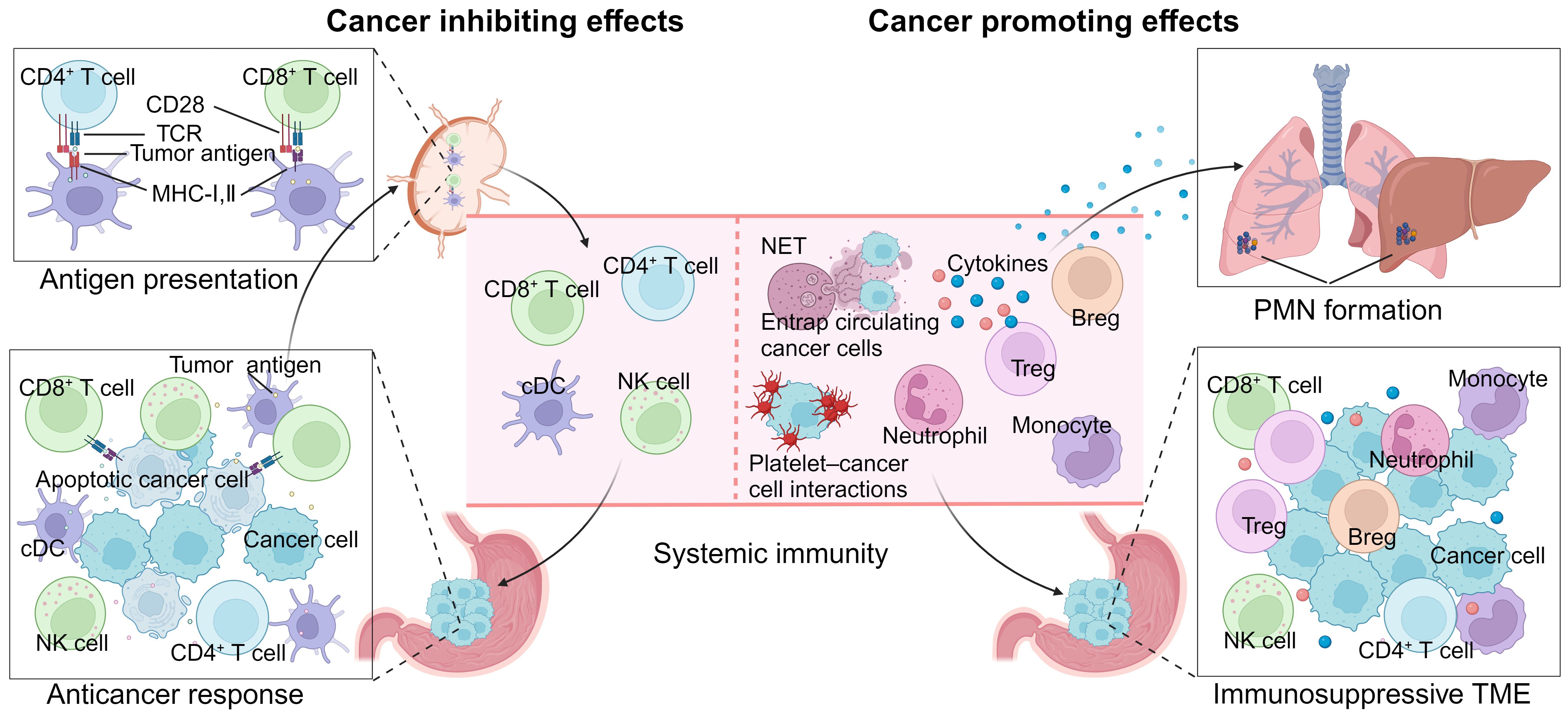
Figure 2. Roles of systemic immunity in gastric cancer progression. On the one hand, intact peripheral immunity is essential for the anticancer immune response, as the majority of steps of the tumor-immune cycle occur outside the tumor microenvironment (TME). On the other hand, dysregulated systemic immunity promotes cancer progression through immunosuppressive TME development, premetastatic niche (PMN) formation and circulating tumor cell protection. Breg, regular B cell; cDC, classical dendritic cell; MHC, major histocompatibility complex; NET, neutrophil extracellular trap; NK, natural killer; PMN, premetastatic niche; TME, tumor microenvironment; TCR, T-cell receptor; Treg, regular T cell.
Because of its critical roles and extensive perturbations that occur during cancer progression, the potential of systemic immunity for GC detection, efficacy prediction, prognosis and therapy has been extensively investigated (Figure 3).
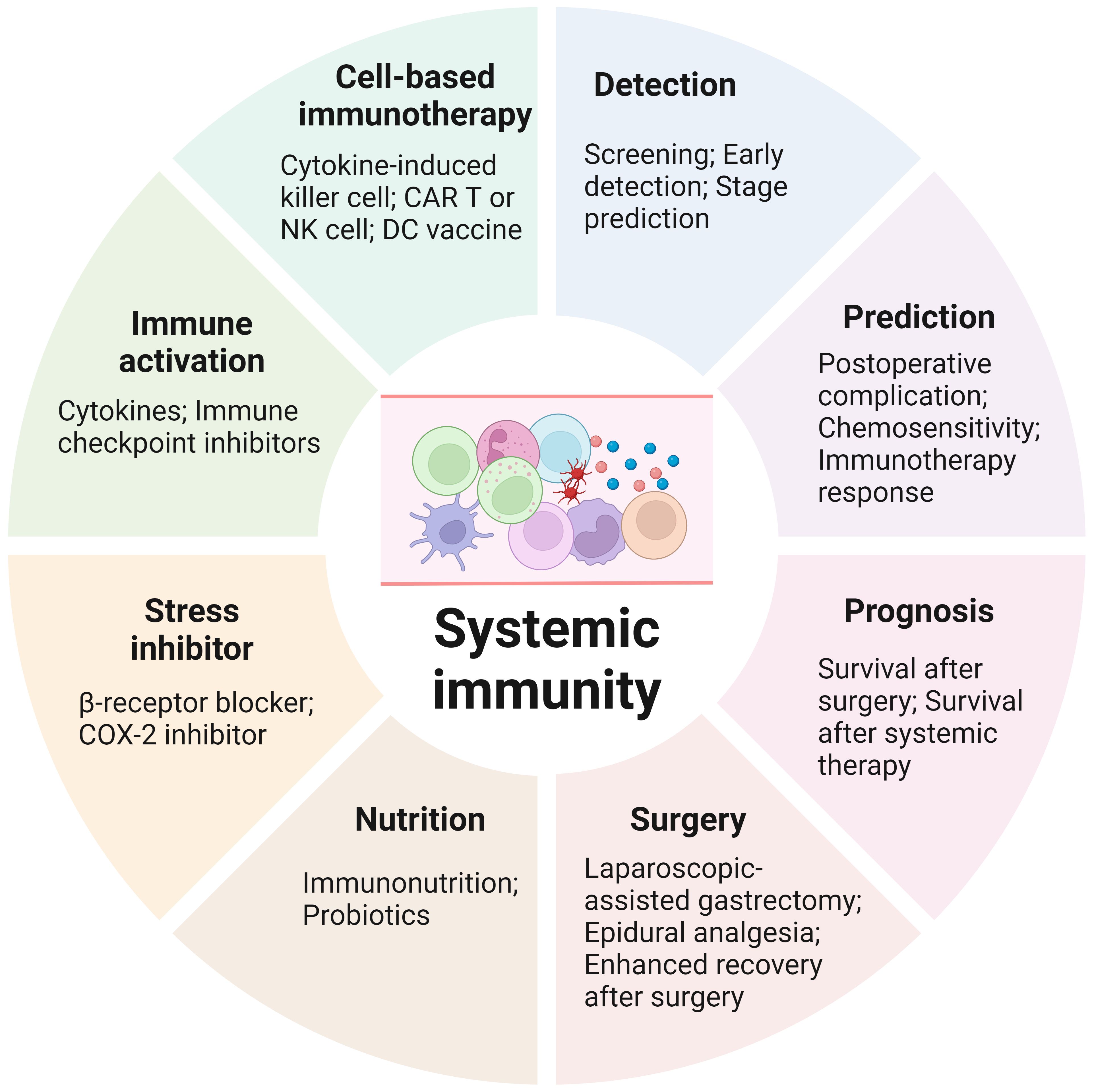
Figure 3. Clinical applications of systemic immunity in gastric cancer. Biomarkers based on systemic immunity can be utilized for gastric cancer detection, therapy response prediction and prognosis. Various strategies have been shown to preserve or activate systemic immunity in patients with gastric cancer. Furthermore, cells from systemic immunity are the main sources of cell-based immunotherapies. CAR, chimeric antigen receptor; COX-2, cyclooxygenase; DC, dendritic cell; NK, natural killer.
4.2 Detection
As mentioned above, compared with healthy subjects, GC patients exhibit extensive alterations in peripheral immune components. Owing to their low cost and noninvasive accessibility, the potential of these alterations as biomarkers for GC detection has been explored. For example, the serum MIC-1 is significantly elevated in early GC, and the performance of early GC detection was 72.9% (83). A diagnostic model including CEA, CA724, IL-6, IL-8, and TNF-α showed the potential to screen for GC, including patients with early-stage disease (84). Peripheral immune cells and indices derived from them also have diagnostic value for GC (41, 59, 85). In addition, the preoperative NLR is significantly correlated with the presence of peritoneal metastasis, especially for type 4 or diffuse type 3 cancers, which may have potential in decision-making regarding staging laparoscopy (86). Despite these promising findings, the application of these biomarkers for the screening and identification of high-risk populations for GC is still lacking. First, all the data were obtained from retrospective cohorts, the majority of which had small sample sizes and were not validated prospectively or externally. Second, the cut-offs used to define high or low levels varied across studies, impeding the development of an optimal method for generalization. Third, peripheral immune components are widely affected by various factors, leading to low specificity for the detection of GC. Therefore, systemic immunity-related biomarkers may be utilized as supplements rather than methods to screen for GC independently.
4.3 Efficacy prediction
Treatment for GC, including surgery, chemotherapy, radiotherapy, targeted therapy and immunotherapy, puts patients at risk for complications and adverse effects. Therefore, exactly predicting the efficacy and possible adverse effects has long been a goal in the management of GC. Postoperative complications following radical gastrectomy, especially infections, including anastomotic leakage, pneumonia and intraabdominal infections, are significantly associated with oncological outcomes (65, 87). Therefore, various prediction models, including indices based on components of systemic immunity, have been established to assess the risk of postoperative complications. For example, preoperative peripheral T cells, B cells, NK cells, the NLR, the PLR and the LMR are predictive of prolonged hospital stays and infectious complications (88, 89). Furthermore, the postoperative systemic immune state is also a predictor of infectious complications (90). Nevertheless, whether preoperative strategies based on these prediction models will improve the short-term outcomes of GC patients has not been investigated. In terms of chemotherapy and immunotherapy alone or in combination, which are used to reduce recurrence following radical gastrectomy or to prolong the survival of patients with advanced disease, the predictive value of biomarkers based on peripheral immune components has also been suggested. The PLR obtained prior to chemotherapy might be a useful indicator for predicting chemosensitivity, whereas the baseline IL-6 concentration and progressive decrease in the PLR during treatment can predict the therapeutic benefits of immunochemotherapy (91–94). Furthermore, baseline circulating Treg cell levels can predict the probability of the occurrence of immunotherapy-related adverse events (95). In the future, if these predictive biomarkers can be regularly and dynamically monitored, personalized therapy for GC can optimize therapeutic effects while reducing adverse events.
4.4 Prognosis
A precise prognosis is critical in the management of GC patients, as more intensive therapy may be needed for patients with negative prognostic factors. Therefore, numerous host and cancer features, including biomarkers based on systemic immunity, have been explored as prognostic factors. In general, higher levels of peripheral immune components involved in anticancer responses indicate better survival. For example, IL-2 and INF-γ levels are positively associated with overall survival (OS), whereas IL-6, IL-10 and IL-17A levels are negatively associated with OS (15, 24, 96). C-reactive protein (CRP), an indicator of ongoing proinflammatory response, was negatively associated with OS in a meta-analysis (97). In addition, ICOS+Foxp3+ Treg cells and pDCs in the peripheral blood could predict poor clinical outcomes in GC patients (98). In the literature, the most studied systemic immune biomarkers with prognostic value in GC are indices derived from multiple immune components, such as the NLR, PLR, LMR and prognostic nutritional index (PNI). Table 1 summarizes the results of the meta-analyses on the prognostic value of these indices in patients with GC receiving different treatment strategies. These meta-analyses consistently suggest that higher levels of neutrophils, monocytes and platelets and lower levels of lymphocytes are significantly associated with poor OS (Table 2) (91, 97, 99–122).
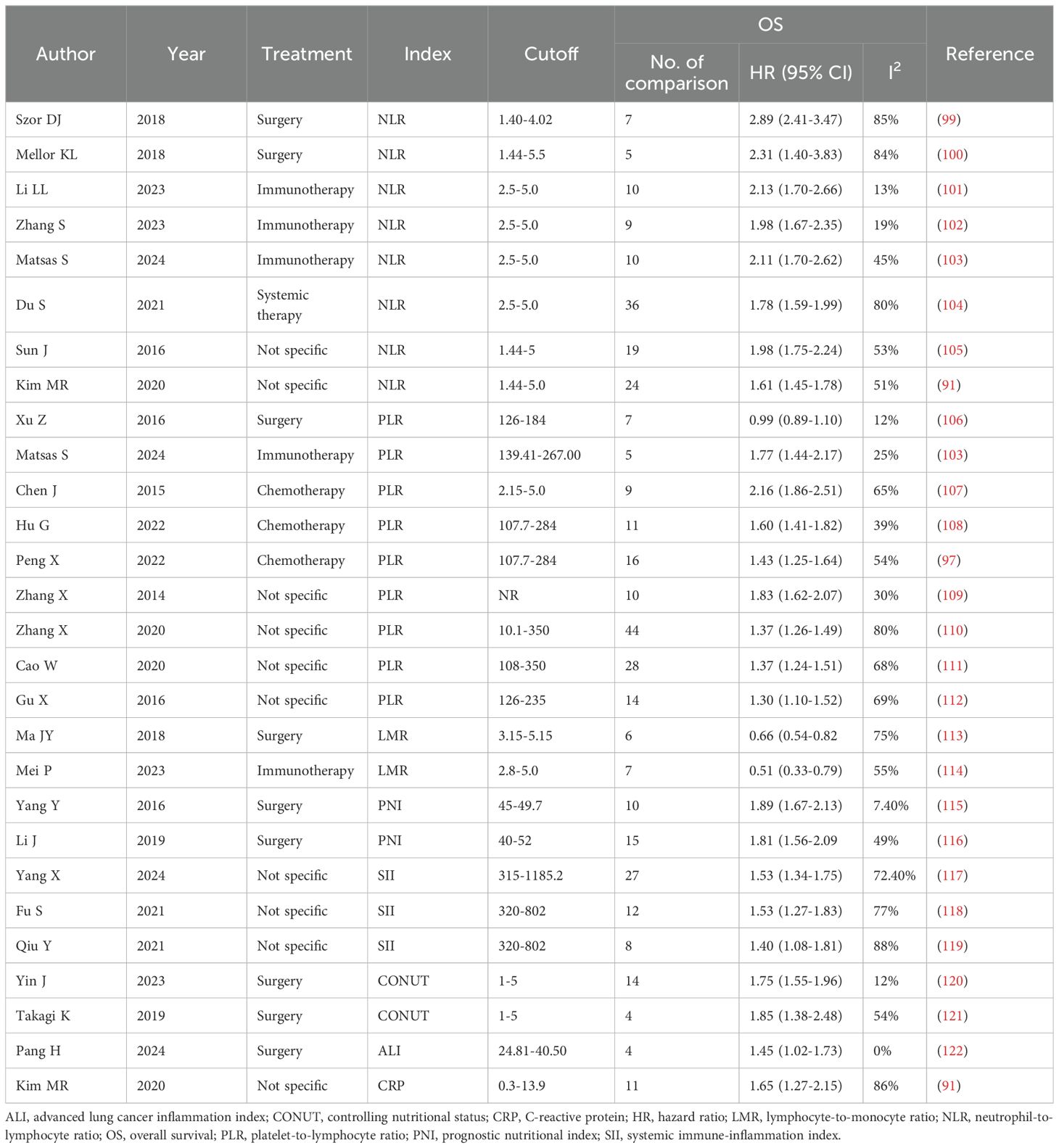
Table 1. Meta-analyses investigating the prognostic value of systemic immune biomarkers in gastric cancer.
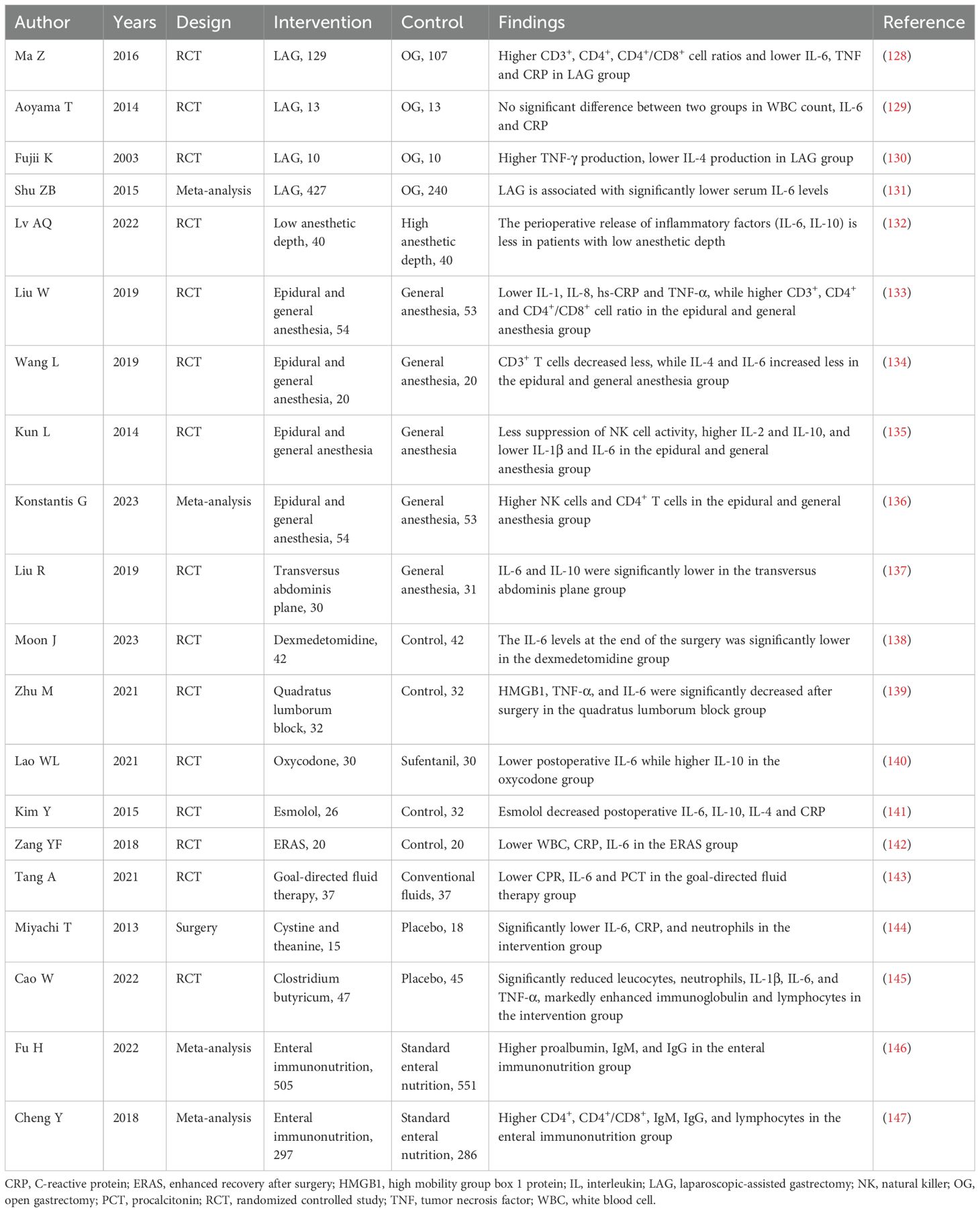
Table 2. Randomized controlled studies and meta-analyses investigating strategies to preserve or enhance systemic immunity following radical gastrectomy.
Nevertheless, despite tremendous interest in the development of predictive and prognostic biomarkers derived from peripheral immunity, no such biomarkers have shown sufficient ability to guide bedside decision-making. First, because of the wide range of cutoff values used across studies, the optimal values of these indices as prognostic factors are unknown and need to be standardized through multicenter and international studies. Second, systemic immunity is continuously influenced by various factors, including cancer treatment. Although changes in some indices during therapy have shown better prognostic value, studies dedicated to monitoring the dynamics of systemic immune biomarkers in GC are limited (15, 24). Finally, the predictive and prognostic values of systemic immune biomarkers may be context dependent. For example, the prognostic value of circulating cytokines was exclusive to patients receiving immunotherapy in combination with chemotherapy but not to patients receiving chemotherapy alone (15). Therefore, further studies are needed to fully understand why the prognostic value of systemic immune components in GC patients is inconsistent across different contexts.
4.5 Harnessing systemic immunity for GC therapy
Although the exact mechanisms underlying the contribution of systemic immunity to GC progression remain unknown, its distinct perturbations during carcinogenesis and prognostic value suggest that systemic immunity has the potential to be harnessed for GC therapy. As surgery and anesthesia are two of the strongest inducers of the stress response, studies have focused on exploring strategies to alleviate their effects on systemic immune function and inflammation (123). Compared with open gastrectomy, laparoscopic-assisted gastrectomy (LAG) has a weaker inflammatory response and less impact on the immune system. Furthermore, in recent years, the noninferiority of LAG in long-term survival has been strongly established for both early and locally advanced GC patients (124–127). Therefore, LAG is recommended for GC patients without contraindications to reduce the detrimental effects of surgery on systemic immunity. In terms of anesthesia and analgesia, the available evidence supports the combination of epidural analgesia and general anesthesia, which has the potential to improve systemic immunity while inhibiting the inflammatory response. As discussed above, activation of the sympathetic nervous system contributes the most to postoperative immune suppression; therefore, intraoperative administration of esmolol, a β-receptor blocker, decreases the inflammatory response and CRP production in a dose-dependent manner. In addition to these examples, various other perioperative strategies, including analgesics, anesthesia at low depths, goal-directed fluid therapy, enhanced recovery after surgery (ERAS), probiotics and enteral immunonutrition, have also shown beneficial effects on systemic immunity, and their regular administration is worthy of further exploration (Table 2) (128–147).
In addition to these strategies aimed at preserving systemic immunity through a reduction in the stress response and inflammation following radical gastrectomy, another means to harness systemic immunity for GC therapy is to directly enhance the anticancer response. One unsophisticated strategy involves the use of recombinant cytokines, such as IL-2, type I IFNs and granulocyte-macrophage colony-stimulating factor (GM-CSF), which can activate peripheral lymphocytes and improve survival (148–151). However, cytokine therapy has been discontinued in recent years, largely owing to severe systemic adverse events. Cell-based immunotherapies, such as cytokine-induced killer cell (CIK) therapy, dendritic cell-based vaccines, and chimeric antigen receptor (CAR) T or NK cell therapy, mainly involve obtaining therapeutic cells from the periphery and have shown promising results in both preclinical and clinical trials in GC (152–157). Another class of immunotherapy, ICIs, has revolutionized the field of oncology in the past decade. Many clinical trials have demonstrated the efficacy of ICIs in patients with GC (4–7). Although many studies have focused on the TME to investigate the mechanisms underlying the anticancer effects of ICIs, the involvement of systemic immunity has also been noted in recent years. As persistence in the TME rapidly drives dysfunctional differentiation of T cells, effective immunotherapies need continuous new effector T-cell infiltration. Several studies have shown that the de novo immune response mainly occurs in the periphery (158–160). In GC patients, ICIs have been shown to increase the levels of circulating IL-2 and IFN-γ and enhance the activation of central/effector memory and effector subsets of CD4+/CD8+ T cells (15, 161). As discussed above, traditional therapies for GC have detrimental effects on systemic immunity; therefore, pairing chemotherapy with ICIs significantly improved the oncological outcomes of GC patients with GC (4–7). In contrast, the application of immunotherapy during the perioperative timeframe has been limited by the established and theoretical risks pertinent around the time of surgery. However, as perioperative immune preservation or stimulation have various advantages, further investigations are needed to develop potential strategies.
5 Conclusions and future perspectives
With the progression of GC, not only the local but also the systemic immune landscape are strongly perturbed by cancer. In addition, traditional treatments for GC, including radical gastrectomy and chemotherapy, also have detrimental effects on systemic immunity. Although effector immune cells in the TME are the key executors, the localized anticancer immune response cannot persist without continuous communication with the periphery. As systemic immunity closely participates in the progression of GC and is a critical determinant of the efficacy of other therapeutic methods, systemic immunity can be widely applied as a biomarker for GC detection, prediction and prognosis and can be harnessed for GC treatment.
Nevertheless, the majority of currently available data are limited to exploring the perturbations and their associations with therapeutic efficacy and oncological outcomes, and few studies have elucidated the underlying mechanisms involved. Although some critical progress has been made in other cancer types, because systemic immune alterations vary across cancer tissue origins, disease stages and patient characteristics, more studies are needed to clarify the distinct immune states and critical mechanisms involved in directing treatment development to restore an anticancer immune macroenvironment. In recent years, the progress of single-cell technologies has provided many impressive transcriptomic, epigenomic and proteomic data on the immune microenvironment, which can also be applied to assess alterations in systemic immunity. In addition to traditional measurement methods, such as peripheral blood cell counts, circulating molecule detection and flow cytometry, these single-cell technologies can inform the distinct types of systemic immune organization in GC, which will aid personalized treatment designed to pair with traditional therapies to alleviate their detrimental effects on systemic immunity or to directly activate the anticancer response of systemic immunity. Although various strategies have been shown to improve the function of systemic immunity in GC patients during traditional therapy, the translation of these effects into survival benefits is limited, and further studies are needed to determine the underlying mechanisms involved. On the other hand, immunotherapy has achieved impressive success in patients with GC; however, the majority of patients obtained no benefit from this therapeutic strategy. Previous studies on the mechanisms underlying resistance to immunotherapy or biomarkers for patient stratification have focused mainly on the TME. Owing to the close associations with effective anticancer immune responses, strategies harnessing systemic immunity to improve the oncological outcomes of patients with GC warrant further research.
Author contributions
YZ: Data curation, Formal analysis, Writing – original draft. JFL: Writing – original draft. JL: Conceptualization, Funding acquisition, Methodology, Supervision, Visualization, Writing – review & editing. JW: Resources, Supervision, Writing – review & editing.
Funding
The author(s) declare financial support was received for the research, authorship, and/or publication of this article. This work is supported by the Medical Research Project of The Third Hospital of Mianyang (202222) and the Scientific Research Projects of Health Commission of Mianyang City (202223).
Acknowledgments
The authors would like to thank the Health Commission of Mianyang City for their support. We apologize to authors whose papers could not be cited due to space limitations.
Conflict of interest
The authors declare that the research was conducted in the absence of any commercial or financial relationships that could be construed as a potential conflict of interest.
Publisher’s note
All claims expressed in this article are solely those of the authors and do not necessarily represent those of their affiliated organizations, or those of the publisher, the editors and the reviewers. Any product that may be evaluated in this article, or claim that may be made by its manufacturer, is not guaranteed or endorsed by the publisher.
Supplementary material
The Supplementary Material for this article can be found online at: https://www.frontiersin.org/articles/10.3389/fimmu.2024.1450128/full#supplementary-material
References
1. Zhang T, Chen H, Zhang Y, Yin X, Man J, Yang X, et al. Global changing trends in incidence and mortality of gastric cancer by age and sex, 1990-2019: Findings from Global Burden of Disease Study. J Cancer. (2021) 12:6695–705. doi: 10.7150/jca.62734
2. Bray F, Laversanne M, Sung H, Ferlay J, Siegel RL, Soerjomataram I, et al. Global cancer statistics 2022: GLOBOCAN estimates of incidence and mortality worldwide for 36 cancers in 185 countries. CA Cancer J Clin. (2024) 74:229–63. doi: 10.3322/caac.21834
3. Joshi SS, Badgwell BD. Current treatment and recent progress in gastric cancer. CA Cancer J Clin. (2021) 71:264–79. doi: 10.3322/caac.21657
4. Chen LT, Satoh T, Ryu MH, Chao Y, Kato K, Chung HC, et al. A phase 3 study of nivolumab in previously treated advanced gastric or gastroesophageal junction cancer (ATTRACTION-2): 2-year update data. Gastric Cancer. (2020) 23:510–9. doi: 10.1007/s10120-019-01034-7
5. Shitara K, Van Cutsem E, Bang YJ, Fuchs C, Wyrwicz L, Lee KW, et al. Efficacy and safety of pembrolizumab or pembrolizumab plus chemotherapy vs chemotherapy alone for patients with first-line, advanced gastric cancer: the KEYNOTE-062 phase 3 randomized clinical trial. JAMA Oncol. (2020) 6:1571–80. doi: 10.1001/jamaoncol.2020.3370
6. Shitara K, Ajani JA, Moehler M, Garrido M, Gallardo C, Shen L, et al. Nivolumab plus chemotherapy or ipilimumab in gastro-oesophageal cancer. Nature. (2022) 603:942–8. doi: 10.1038/s41586-022-04508-4
7. Janjigian YY, Kawazoe A, Yañez P, Li N, Lonardi S, Kolesnik O, et al. The KEYNOTE-811 trial of dual PD-1 and HER2 blockade in HER2-positive gastric cancer. Nature. (2021) 600:727–30. doi: 10.1038/s41586-021-04161-3
8. Kono K, Nakajima S, Mimura K. Biomarker-oriented chemo-immunotherapy for advanced gastric cancer. Int J Clin Oncol. (2024) 29:865–72. doi: 10.1007/s10147-024-02525-z
9. Mou P, Ge QH, Sheng R, Zhu TF, Liu Y, Ding K. Research progress on the immune microenvironment and immunotherapy in gastric cancer. Front Immunol. (2023) 14:1291117. doi: 10.3389/fimmu.2023.1291117
10. Hiam-Galvez KJ, Allen BM, Spitzer MH. Systemic immunity in cancer. Nat Rev Cancer. (2021) 21:345–59. doi: 10.1038/s41568-021-00347-z
11. Gabrilovich DI, Ostrand-Rosenberg S, Bronte V. Coordinated regulation of myeloid cells by tumours. Nat Rev Immunol. (2012) 12:253–68. doi: 10.1038/nri3175
12. Allen BM, Hiam KJ, Burnett CE, Venida A, DeBarge R, Tenvooren I, et al. Systemic dysfunction and plasticity of the immune macroenvironment in cancer models. Nat Med. (2020) 26:1125–34. doi: 10.1038/s41591-020-0892-6
13. Propper DJ, Balkwill FR. Harnessing cytokines and chemokines for cancer therapy. Nat Rev Clin Oncol. (2022) 19:237–53. doi: 10.1038/s41571-021-00588-9
14. Yang E, Chua W, Ng W, Roberts TL. Peripheral cytokine levels as a prognostic indicator in gastric cancer: A review of existing literature. Biomedicines. (2021) 9:1916. doi: 10.3390/biomedicines9121916
15. Hou Y, Li X, Yang Y, Shi H, Wang S, Gao M. Serum cytokines and neutrophil-to-lymphocyte ratio as predictive biomarkers of benefit from PD-1 inhibitors in gastric cancer. Front Immunol. (2023) 14:1274431. doi: 10.3389/fimmu.2023.1274431
16. Sánchez-Zauco N, Torres J, Gómez A, Camorlinga-Ponce M, Muñoz-Pérez L, Herrera-Goepfert R, et al. Circulating blood levels of IL-6, IFN-γ, and IL-10 as potential diagnostic biomarkers in gastric cancer: a controlled study. BMC Cancer. (2017) 17:384. doi: 10.1186/s12885-017-3310-9
17. Lippitz BE. Cytokine patterns in patients with cancer: a systematic review. Lancet Oncol. (2013) 14:e218–28. doi: 10.1016/S1470-2045(12)70582-X
18. Ashizawa T, Okada R, Suzuki Y, Takagi M, Yamazaki T, Sumi T, et al. Clinical significance of interleukin-6 (IL-6) in the spread of gastric cancer: role of IL-6 as a prognostic factor. Gastric Cancer. (2005) 8:124–31. doi: 10.1007/s10120-005-0315-x
19. Tonouchi H, Miki C, Tanaka K, Kusunoki M. Profile of monocyte chemoattractant protein-1 circulating levels in gastric cancer patients. Scand J Gastroenterol. (2002) 37:830–3. doi: 10.1080/gas.37.7.830.833
20. Amedei A, Della Bella C, Silvestri E, Prisco D, D'Elios MM. T cells in gastric cancer: friends or foes. Clin Dev Immunol. (2012) 2012:690571. doi: 10.1155/2012/690571
21. Szkaradkiewicz A, Karpiński TM, Drews M, Borejsza-Wysocki M, Majewski P, Andrzejewska E. Natural killer cell cytotoxicity and immunosuppressive cytokines (IL-10, TGF-beta1) in patients with gastric cancer. J BioMed Biotechnol. (2010) 2010:901564. doi: 10.1155/2010/901564
22. Jiang P, Zhang Y, Ru B, Yang Y, Vu T, Paul R, et al. Systematic investigation of cytokine signaling activity at the tissue and single-cell levels. Nat Methods. (2021) 18:1181–91. doi: 10.1038/s41592-021-01274-5
23. Cárdenas DM, Sánchez AC, Rosas DA, Rivero E, Paparoni MD, Cruz MA, et al. Preliminary analysis of single-nucleotide polymorphisms in IL-10, IL-4, and IL-4Rα genes and profile of circulating cytokines in patients with gastric Cancer. BMC Gastroenterol. (2018) 18:184. doi: 10.1186/s12876-018-0913-9
24. Qi Q, Peng Y, Zhu M, Zhang Y, Bao Y, Zhang X, et al. Association between serum levels of 12 different cytokines and short-term efficacy of anti-PD-1 monoclonal antibody combined with chemotherapy in advanced gastric cancer. Int Immunopharmacol. (2023) 114:109553. doi: 10.1016/j.intimp.2022.109553
25. Guo GH, Xie YB, Zhang PJ, Jiang T. Blood index panel for gastric cancer detection. World J Gastrointest Surg. (2022) 14:1026–36. doi: 10.4240/wjgs.v14.i9.1026
26. Epplein M, Xiang YB, Cai Q, Peek RM Jr, Li H, Correa P, et al. Circulating cytokines and gastric cancer risk. Cancer Causes Control. (2013) 24:2245–50. doi: 10.1007/s10552-013-0284-z
27. Zou Z, Zhao L, Su S, Liu Q, Yu L, Wei J, et al. The plasma levels of 12 cytokines and growth factors in patients with gastric cancer. Med (Baltimore). (2018) 97:e0413. doi: 10.1097/MD.0000000000010413
28. Liang J, Li Y, Liu X, Xu X, Zhao Y. Relationship between cytokine levels and clinical classification of gastric cancer. Asian Pac J Cancer Prev. (2011) 12:1803–6.
29. Wang TT, Zhao YL, Peng LS, Chen N, Chen W, Lv YP, et al. Tumour-activated neutrophils in gastric cancer foster immune suppression and disease progression through GM-CSF-PD-L1 pathway. Gut. (2017) 66:1900–11. doi: 10.1136/gutjnl-2016-313075
30. Chen J, Liu Z, Gao G, Mo Y, Zhou H, Huang W, et al. Efficacy of circulating microRNA-130b and blood routine parameters in the early diagnosis of gastric cancer. Oncol Lett. (2021) 22:725. doi: 10.3892/ol.2021.12986
31. Arii K, Tanimura H, Iwahashi M, Tsunoda T, Tani M, Noguchi K, et al. Neutrophil functions and cytokine production in patients with gastric cancer. Hepatogastroenterology. (2000) 47:291–7.
32. Mao FY, Zhao YL, Lv YP, Teng YS, Kong H, Liu YG, et al. CD45(+)CD33(low)CD11b(dim) myeloid-derived suppressor cells suppress CD8(+) T cell activity via the IL-6/IL-8-arginase I axis in human gastric cancer. Cell Death Dis. (2018) 9:763. doi: 10.1038/s41419-018-0803-7
33. Ross DS, Neer RM, Ridgway EC, Daniels GH. Subclinical hyperthyroidism and reduced bone density as a possible result of prolonged suppression of the pituitary-thyroid axis with L-thyroxine. Am J Med. (1987) 82:1167–70. doi: 10.1016/0002-9343(87)90219-1
34. Zhu T, Zou X, Yang C, Li L, Wang B, Li R, et al. Neutrophil extracellular traps promote gastric cancer metastasis by inducing epithelial−mesenchymal transition. Int J Mol Med. (2021) 48:127 [pii]. doi: 10.3892/ijmm.2021.4960
35. Yamane T, Sakita M, Kasuga M, Nishioka B, Fujita Y, Majima S. Monocyte count, monocyte chemotaxis and chemotactic factor inactivator in gastric cancer patients. Jpn J Surg. (1981) 11:422–7. doi: 10.1007/BF02469025
36. Wang Z, Yin N, Zhang Z, Zhang Y, Zhang G, Chen W. Upregulation of T-cell immunoglobulin and mucin-domain containing-3 (Tim-3) in monocytes/macrophages associates with gastric cancer progression. Immunol Invest. (2017) 46:134–48. doi: 10.1080/08820139.2016.1229790
37. Gardner A, Ruffell B. Dendritic cells and cancer immunity. Trends Immunol. (2016) 37:855–65. doi: 10.1016/j.it.2016.09.006
38. Chen J, Yang J, Jiang J, Zhuang Y, He W. Function and subsets of dendritic cells and natural killer cells were decreased in gastric cancer. Int J Clin Exp Pathol. (2014) 7:8304–11.
39. Chang LL, Wang SW, Wu IC, Yu FJ, Su YC, Chen YP, et al. Impaired dendritic cell maturation and IL-10 production following H. pylori stimulation in gastric cancer patients. Appl Microbiol Biotechnol. (2012) 96:211–20. doi: 10.1007/s00253-012-4034-z
40. Coutant F, Miossec P. Altered dendritic cell functions in autoimmune diseases: distinct and overlapping profiles. Nat Rev Rheumatol. (2016) 12:703–15. doi: 10.1038/nrrheum.2016.147
41. Xu DP, Shi WW, Zhang TT, Lv HY, Li JB, Lin A, et al. Elevation of HLA-G-expressing DC-10 cells in patients with gastric cancer. Hum Immunol. (2016) 77:800–4. doi: 10.1016/j.humimm.2016.01.003
42. Yu H, Mei Y, Dong Y, Chen C, Lin X, Jin H, et al. CCR9-CCL25 mediated plasmacytoid dendritic cell homing and contributed the immunosuppressive microenvironment in gastric cancer. Transl Oncol. (2023) 33:101682. doi: 10.1016/j.tranon.2023.101682
43. Huang XM, Liu XS, Lin XK, Yu H, Sun JY, Liu XK, et al. Role of plasmacytoid dendritic cells and inducible costimulator-positive regulatory T cells in the immunosuppression microenvironment of gastric cancer. Cancer Sci. (2014) 105:150–8. doi: 10.1111/cas.12327
44. Li J, Chen Z, Li Q, Liu R, Zheng J, Gu Q, et al. Study of miRNA and lymphocyte subsets as potential biomarkers for the diagnosis and prognosis of gastric cancer. PeerJ. (2024) 12:e16660. doi: 10.7717/peerj.16660
45. Xu J, Guo R, Jia J, He Y, He S. Activation of Toll-like receptor 2 enhances peripheral and tumor-infiltrating CD8(+) T cell cytotoxicity in patients with gastric cancer. BMC Immunol. (2021) 22:67. doi: 10.1186/s12865-021-00459-z
46. Zhong C, Song Z, Li M. Gastric cancer patients display a distinctive population of IFNg(+)IL10(+) double positive CD8 T cells, which persists longer during prolonged activation. Exp Cell Res. (2019) 382:111487. doi: 10.1016/j.yexcr.2019.06.032
47. Takano S, Saito H, Ikeguchi M. An increased number of PD-1+ and Tim-3+ CD8+ T cells is involved in immune evasion in gastric cancer. Surg Today. (2016) 46:1341–7. doi: 10.1007/s00595-016-1305-9
48. Zhong F, Cui D, Tao H, Du H, Xing C. IL-17A-producing T cells and associated cytokines are involved in the progression of gastric cancer. Oncol Rep. (2015) 34:2365–74. doi: 10.3892/or.2015.4246
49. Zhuang Y, Peng LS, Zhao YL, Shi Y, Mao XH, Chen W, et al. CD8(+) T cells that produce interleukin-17 regulate myeloid-derived suppressor cells and are associated with survival time of patients with gastric cancer. Gastroenterology. (2012) 143:951–62.e8. doi: 10.1053/j.gastro.2012.06.010
50. Li H, Cao GM, Gu GL, Li SY, Yan Y, Fu Z, et al. Expression characteristics of peripheral lymphocyte programmed death 1 and FoxP3(+) Tregs in gastric cancer during surgery and chemotherapy. World J Gastroenterol. (2023) 29:5582–92. doi: 10.3748/wjg.v29.i40.5582
51. Zhang Y, Wu J, Zhang H, Wu C. The regulation between CD4(+)CXCR5(+) follicular helper T (Tfh) cells and CD19(+)CD24(hi)CD38(hi) regulatory B (Breg) cells in gastric cancer. J Immunol Res. (2022) 2022:9003902. doi: 10.1155/2022/9003902
52. Wang WW, Yuan XL, Chen H, Xie GH, Ma YH, Zheng YX, et al. CD19+CD24hiCD38hiBregs involved in downregulate helper T cells and upregulate regulatory T cells in gastric cancer. Oncotarget. (2015) 6:33486–99. doi: 10.18632/oncotarget.5588
53. Shimasaki N, Jain A, Campana D. NK cells for cancer immunotherapy. Nat Rev Drug Discovery. (2020) 19:200–18. doi: 10.1038/s41573-019-0052-1
54. Han B, Mao FY, Zhao YL, Lv YP, Teng YS, Duan M, et al. Altered NKp30, NKp46, NKG2D, and DNAM-1 expression on circulating NK cells is associated with tumor progression in human gastric cancer. J Immunol Res. (2018) 2018:6248590. doi: 10.1155/2018/6248590
55. Wang Z, Zhu J, Gu H, Yuan Y, Zhang B, Zhu D, et al. The clinical significance of abnormal Tim-3 expression on NK cells from patients with gastric cancer. Immunol Invest. (2015) 44:578–89. doi: 10.3109/08820139.2015.1052145
56. Peng YP, Zhu Y, Zhang JJ, Xu ZK, Qian ZY, Dai CC, et al. Comprehensive analysis of the percentage of surface receptors and cytotoxic granules positive natural killer cells in patients with pancreatic cancer, gastric cancer, and colorectal cancer. J Transl Med. (2013) 11:262. doi: 10.1186/1479-5876-11-262
57. de la Torre JC, Goldsmith HS. Increased blood flow enhances axon regeneration after spinal transection. Neurosci Lett. (1988) 94:269–73. doi: 10.1016/0304-3940(88)90029-8
58. Lindgren Å, Yun CH, Sjöling Å, Berggren C, Sun JB, Jonsson E, et al. Impaired IFN-γ production after stimulation with bacterial components by natural killer cells from gastric cancer patients. Exp Cell Res. (2011) 317:849–58. doi: 10.1016/j.yexcr.2011.01.006
59. Zhang J, Zhang L, Duan S, Li Z, Li G, Yu H. Single and combined use of the platelet-lymphocyte ratio, neutrophil-lymphocyte ratio, and systemic immune-inflammation index in gastric cancer diagnosis. Front Oncol. (2023) 13:1143154. doi: 10.3389/fonc.2023.1143154
60. Uzunoglu H, Kaya S. Does systemic immune inflammation index have predictive value in gastric cancer prognosis. North Clin Istanb. (2023) 10:24–32. doi: 10.14744/nci.2021.71324
61. Zhao Q, Dong L, Liang H, Pang K, Wang P, Ge R, et al. Evaluation of multiple biological indicators for combined diagnosis of gastric cancer: A retrospective analysis. Med (Baltimore). (2022) 101:e31904. doi: 10.1097/MD.0000000000031878
62. Servis D, Busic Z, Stipancic I, Patrlj L, Gagro A. Serum cytokine changes after gastric resection or gastrectomy for gastric cancer. Hepatogastroenterology. (2008) 55:1868–72.
63. Sun HL, Dong YC, Wang CQ, Qian YN, Wang ZY. Effects of postoperative analgesia with the combination of tramadol and lornoxicam on serum inflammatory cytokines in patients with gastric cancer. Int J Clin Pharmacol Ther. (2014) 52:1023–9. doi: 10.5414/CP202190
64. Okuno K, Tanaka A, Shigeoka H, Hirai N, Kawai I, Kitano Y, et al. Suppression of T-cell function in gastric cancer patients after total gastrectomy with splenectomy: implications of splenic autotransplantation. Gastric Cancer. (1999) 2:20–5. doi: 10.1007/s101200050016
65. Zhi X, Kuang X, Li J. The impact of perioperative events on cancer recurrence and metastasis in patients after radical gastrectomy: A review. Cancers (Basel). (2022) 14:3496. doi: 10.3390/cancers14143496
66. Hiller JG, Perry NJ, Poulogiannis G, Riedel B, Sloan EK. Perioperative events influence cancer recurrence risk after surgery. Nat Rev Clin Oncol. (2018) 15:205–18. doi: 10.1038/nrclinonc.2017.194
67. Tai LH, de Souza CT, Bélanger S, Ly L, Alkayyal AA, Zhang J, et al. Preventing postoperative metastatic disease by inhibiting surgery-induced dysfunction in natural killer cells. Cancer Res. (2013) 73:97–107. doi: 10.1158/0008-5472.CAN-12-1993
68. Gryglewski A, Majcher P, Bryniarski K, Konturek S, Ptak M, Ptak W, et al. Mesenteric lymph node Tgammadelta cells induced by gastrectomy in mice suppress cell-mediated immune response in vitro via released TGF-beta. J Surg Res. (2007) 142:66–71. doi: 10.1016/j.jss.2006.10.050
69. Bosiljcic M, Cederberg RA, Hamilton MJ, LePard NE, Harbourne BT, Collier JL, et al. Targeting myeloid-derived suppressor cells in combination with primary mammary tumor resection reduces metastatic growth in the lungs. Breast Cancer Res. (2019) 21:103. doi: 10.1186/s13058-019-1189-x
70. Shaked Y. The pro-tumorigenic host response to cancer therapies. Nat Rev Cancer. (2019) 19:667–85. doi: 10.1038/s41568-019-0209-6
71. Zhang M, Fan Y, Che X, Hou K, Zhang C, Li C, et al. 5-FU-induced upregulation of exosomal PD-L1 causes immunosuppression in advanced gastric cancer patients. Front Oncol. (2020) 10:492. doi: 10.3389/fonc.2020.00492
72. Zhang F, Li R, Yang Y, Shi C, Shen Y, Lu C, et al. Specific decrease in B-cell-derived extracellular vesicles enhances post-chemotherapeutic CD8(+) T cell responses. Immunity. (2019) 50:738–50.e7. doi: 10.1016/j.immuni.2019.01.010
73. Bruchard M, Mignot G, Derangère V, Chalmin F, Chevriaux A, Végran F, et al. Chemotherapy-triggered cathepsin B release in myeloid-derived suppressor cells activates the Nlrp3 inflammasome and promotes tumor growth. Nat Med. (2013) 19:57–64. doi: 10.1038/nm.2999
74. Takeuchi S, Baghdadi M, Tsuchikawa T, Wada H, Nakamura T, Abe H, et al. Chemotherapy-derived inflammatory responses accelerate the formation of immunosuppressive myeloid cells in the tissue microenvironment of human pancreatic cancer. Cancer Res. (2015) 75:2629–40. doi: 10.1158/0008-5472.CAN-14-2921
75. Schreiber RD, Old LJ, Smyth MJ. Cancer immunoediting: integrating immunity's roles in cancer suppression and promotion. Science. (2011) 331:1565–70. doi: 10.1126/science.1203486
76. Garner H, de Visser KE. Immune crosstalk in cancer progression and metastatic spread: a complex conversation. Nat Rev Immunol. (2020) 20:483–97. doi: 10.1038/s41577-019-0271-z
77. Spitzer MH, Carmi Y, Reticker-Flynn NE, Kwek SS, Madhireddy D, Martins MM, et al. Systemic immunity is required for effective cancer immunotherapy. Cell. (2017) 168:487–502.e15. doi: 10.1016/j.cell.2016.12.022
78. Fransen MF, Schoonderwoerd M, Knopf P, Camps MG, Hawinkels LJ, Kneilling M, et al. Tumor-draining lymph nodes are pivotal in PD-1/PD-L1 checkpoint therapy. JCI Insight. (2018) 3:e124507. doi: 10.1172/jci.insight.124507
79. Ruan DY, Chen YX, Wei XL, Wang YN, Wang ZX, Wu HX, et al. Elevated peripheral blood neutrophil-to-lymphocyte ratio is associated with an immunosuppressive tumour microenvironment and decreased benefit of PD-1 antibody in advanced gastric cancer. Gastroenterol Rep (Oxf). (2021) 9:560–70. doi: 10.1093/gastro/goab032
80. Peinado H, Zhang H, Matei IR, Costa-Silva B, Hoshino A, Rodrigues G, et al. Pre-metastatic niches: organ-specific homes for metastases. Nat Rev Cancer. (2017) 17:302–17. doi: 10.1038/nrc.2017.6
81. Hedrick CC, Malanchi I. Neutrophils in cancer: heterogeneous and multifaceted. Nat Rev Immunol. (2022) 22:173–87. doi: 10.1038/s41577-021-00571-6
82. Gaertner F, Massberg S. Patrolling the vascular borders: platelets in immunity to infection and cancer. Nat Rev Immunol. (2019) 19:747–60. doi: 10.1038/s41577-019-0202-z
83. Ge X, Zhang X, Ma Y, Chen S, Chen Z, Li M. Diagnostic value of macrophage inhibitory cytokine 1 as a novel prognostic biomarkers for early gastric cancer screening. J Clin Lab Anal. (2021) 35:e23568. doi: 10.1002/jcla.23568
84. Li J, Xu L, Run ZC, Feng W, Liu W, Zhang PJ, et al. Multiple cytokine profiling in serum for early detection of gastric cancer. World J Gastroenterol. (2018) 24:2269–78. doi: 10.3748/wjg.v24.i21.2269
85. Fang T, Wang Y, Yin X, Zhai Z, Zhang Y, Yang Y, et al. Diagnostic sensitivity of NLR and PLR in early diagnosis of gastric cancer. J Immunol Res. (2020) 2020:9146042. doi: 10.1155/2020/9146042
86. Nakamura N, Kinami S, Fujii Y, Miura S, Fujita J, Kaida D, et al. The neutrophil/lymphocyte ratio as a predictor of peritoneal metastasis during staging laparoscopy for advanced gastric cancer: a retrospective cohort analysis. World J Surg Oncol. (2019) 17:108. doi: 10.1186/s12957-019-1651-3
87. Li J, Zhang Y, Hu DM, Gong TP, Xu R, Gao J. Impact of postoperative complications on long-term outcomes of patients following surgery for gastric cancer: A systematic review and meta-analysis of 64 follow-up studies. Asian J Surg. (2020) 43:719–29. doi: 10.1016/j.asjsur.2019.10.007
88. Dan Zeng CD, Tong YX, Xiao AT, Gao C, Zhang S. Peripheral lymphocyte subsets absolute counts as feasible clinical markers for predicting surgical outcome in gastric cancer patients after laparoscopic D2 gastrectomy: A prospective cohort study. J Inflammation Res. (2021) 14:5633–46. doi: 10.2147/JIR.S335847
89. Gülmez S, Senger A, Uzun O, Ozduman O, Ofluoglu C, Subasi İ, et al. Comparative analysis of preoperative ratio based markers in predicting postoperative infectious complications after gastrectomy. Pol Przegl Chir. (2022) 95:1–5. doi: 10.5604/01.3001.0015.9662
90. Hwang SH, Kim DJ. Nomogram for predicting infectious complications following curative gastrectomy using clinical and laboratory parameters. Anticancer Res. (2024) 44:1781–90. doi: 10.21873/anticanres.16978
91. Peng X, Zeng W, Tang B, He A, Zhang M, Luo R. Utility of pretreatment blood platelet-to-lymphocyte ratio in prediction of clinical outcomes and chemosensitivity in patients with advanced gastric cancer: A meta-analysis. Med Sci Monit. (2022) 28:e933449. doi: 10.12659/MSM.933449
92. Liu J, Mao Y, Mao C, Wang D, Dong L, Zhu W. An on-treatment decreased trend of serum IL-6 and IL-8 as predictive markers quickly reflects short-term efficacy of PD-1 blockade immunochemotherapy in patients with advanced gastric cancer. J Immunol Res. (2024) 2024:3604935. doi: 10.1155/2024/3604935
93. Lee CK, Lee JB, Park SJ, Che J, Kwon WS, Kim HS, et al. Second-line chemoimmunotherapy with nivolumab and paclitaxel in immune-related biomarker-enriched advanced gastric cancer: a multicenter phase Ib/II study. Gastric Cancer. (2024) 27:118–30. doi: 10.1007/s10120-023-01435-9
94. Ohe Y, Fushida S, Yamaguchi T, Kinoshita J, Saito H, Okamoto K, et al. Peripheral blood platelet-lymphocyte ratio is good predictor of chemosensitivity and prognosis in gastric cancer patients. Cancer Manag Res. (2020) 12:1303–11. doi: 10.2147/CMAR.S241069
95. He XX, Du B, Wu T, Shen H. Prognostic analysis of related factors of adverse reactions to immunotherapy in advanced gastric cancer and establishment of a nomogram model. World J Gastrointest Oncol. (2024) 16:1268–80. doi: 10.4251/wjgo.v16.i4.1268
96. Szaflarska A, Szczepanik A, Siedlar M, Czupryna A, Sierzega M, Popiela T, et al. Preoperative plasma level of IL-10 but not of proinflammatory cytokines is an independent prognostic factor in patients with gastric cancer. Anticancer Res. (2009) 29:5005–12.
97. Kim MR, Kim AS, Choi HI, Jung JH, Park JY, Ko HJ. Inflammatory markers for predicting overall survival in gastric cancer patients: A systematic review and meta-analysis. PloS One. (2020) 15:e0236445. doi: 10.1371/journal.pone.0236445
98. Liu X, Yu H, Yan C, Mei Y, Lin C, Hong Y, et al. Plasmacytoid dendritic cells and ICOS(+) regulatory T cells predict poor prognosis in gastric cancer: A pilot study. J Cancer. (2019) 10:6711–5. doi: 10.7150/jca.34826
99. Szor DJ, Dias AR, Pereira MA, Ramos M, Zilberstein B, Cecconello I, et al. Prognostic role of neutrophil/lymphocyte ratio in resected gastric cancer: A systematic review and meta-analysis. Clinics (Sao Paulo). (2018) 73:e360. doi: 10.6061/clinics/2018/e360
100. Mellor KL, Powell A, Lewis WG. Systematic review and meta-analysis of the prognostic significance of neutrophil-lymphocyte ratio (NLR) after R0 gastrectomy for cancer. J Gastrointest Cancer. (2018) 49:237–44. doi: 10.1007/s12029-018-0127-y
101. Li LL, Pan LS. Prognostic value of neutrophil-to-lymphocyte ratio in gastric cancer patients treated with immune checkpoint inhibitors: A meta-analysis. Kaohsiung J Med Sci. (2023) 39:842–52. doi: 10.1002/kjm2.12694
102. Zhang S, Qiu C, Yu H, Xu Y, Xu X. Prognostic value of neutrophil to lymphocyte ratio in gastric cancer patients receiving immune checkpoint inhibitors: a systematic review and meta-analysis. Front Oncol. (2023) 13:1070019. doi: 10.3389/fonc.2023.1070019
103. Matsas S, Aguiar PN Jr, Del Giglio A. Neutrophil-to-lymphocyte ratio and platelet-to-lymphocyte ratio as biomarkers to prognosticate survival in advanced gastric cancer patients in the era of immunotherapy: a systematic review and meta-analysis. J Gastrointest Oncol. (2024) 15:33–51. doi: 10.21037/jgo-23-808
104. Du S, Fang Z, Ye L, Sun H, Deng G, Wu W, et al. Pretreatment neutrophil-to-lymphocyte ratio predicts the benefit of gastric cancer patients with systemic therapy. Aging (Albany NY). (2021) 13:17638–54. doi: 10.18632/aging.203256
105. Sun J, Chen X, Gao P, Song Y, Huang X, Yang Y, et al. Can the neutrophil to lymphocyte ratio be used to determine gastric cancer treatment outcomes? A systematic review and meta-analysis. Dis Markers. (2016) 2016:7862469. doi: 10.1155/2016/7862469
106. Xu Z, Xu W, Cheng H, Shen W, Ying J, Cheng F, et al. The prognostic role of the platelet-lymphocytes ratio in gastric cancer: A meta-analysis. PloS One. (2016) 11:e0163719. doi: 10.1371/journal.pone.0163719
107. Chen J, Hong D, Zhai Y, Shen P. Meta-analysis of associations between neutrophil-to-lymphocyte ratio and prognosis of gastric cancer. World J Surg Oncol. (2015) 13:122. doi: 10.1186/s12957-015-0530-9
108. Hu G, Wang S, Wang S, Huang L. Elevated baseline circulating platelet-to-lymphocyte ratio and survival in initial stage IV gastric cancer patients: A meta-analysis. PloS One. (2022) 17:e0265897. doi: 10.1371/journal.pone.0265897
109. Zhang X, Zhang W, Feng LJ. Prognostic significance of neutrophil lymphocyte ratio in patients with gastric cancer: a meta-analysis. PloS One. (2014) 9:e111906. doi: 10.1371/journal.pone.0111906
110. Zhang X, Zhao W, Yu Y, Qi X, Song L, Zhang C, et al. Clinicopathological and prognostic significance of platelet-lymphocyte ratio (PLR) in gastric cancer: an updated meta-analysis. World J Surg Oncol. (2020) 18:191. doi: 10.1186/s12957-020-01952-2
111. Cao W, Yao X, Cen D, Zhi Y, Zhu N, Xu L. The prognostic role of platelet-to-lymphocyte ratio on overall survival in gastric cancer: a systematic review and meta-analysis. BMC Gastroenterol. (2020) 20:16. doi: 10.1186/s12876-020-1167-x
112. Gu X, Gao XS, Cui M, Xie M, Peng C, Bai Y, et al. Clinicopathological and prognostic significance of platelet to lymphocyte ratio in patients with gastric cancer. Oncotarget. (2016) 7:49878–87. doi: 10.18632/oncotarget.10490
113. Ma JY, Liu Q. Clinicopathological and prognostic significance of lymphocyte to monocyte ratio in patients with gastric cancer: A meta-analysis. Int J Surg. (2018) 50:67–71. doi: 10.1016/j.ijsu.2018.01.002
114. Mei P, Feng W, Zhan Y, Guo X. Prognostic value of lymphocyte-to-monocyte ratio in gastric cancer patients treated with immune checkpoint inhibitors: a systematic review and meta-analysis. Front Immunol. (2023) 14:1321584. doi: 10.3389/fimmu.2023.1321584
115. Yang Y, Gao P, Song Y, Sun J, Chen X, Zhao J, et al. The prognostic nutritional index is a predictive indicator of prognosis and postoperative complications in gastric cancer: A meta-analysis. Eur J Surg Oncol. (2016) 42:1176–82. doi: 10.1016/j.ejso.2016.05.029
116. Li J, Xu R, Hu DM, Zhang Y, Gong TP, Wu XL. Prognostic nutritional index predicts outcomes of patients after gastrectomy for cancer: A systematic review and meta-analysis of nonrandomized studies. Nutr Cancer. (2019) 71:557–68. doi: 10.1080/01635581.2019.1577986
117. Yang X, Wu C. Systemic immune inflammation index and gastric cancer prognosis: A systematic review and meta−analysis. Exp Ther Med. (2024) 27:122. doi: 10.3892/etm.2024.12410
118. Fu S, Yan J, Tan Y, Liu D. Prognostic value of systemic immune-inflammatory index in survival outcome in gastric cancer: a meta-analysis. J Gastrointest Oncol. (2021) 12:344–54. doi: 10.21037/jgo-20-252
119. Qiu Y, Zhang Z, Chen Y. Prognostic value of pretreatment systemic immune-inflammation index in gastric cancer: A meta-analysis. Front Oncol. (2021) 11:537140. doi: 10.3389/fonc.2021.537140
120. Yin J, Qu J, Liang X, Wang M. Prognostic significance of controlling nutritional status score for patients with gastric cancer: A systematic review and meta−analysis. Exp Ther Med. (2023) 25:202. doi: 10.3892/etm.2023.11901
121. Takagi K, Domagala P, Polak WG, Buettner S, Wijnhoven B, Ijzermans J. Prognostic significance of the controlling nutritional status (CONUT) score in patients undergoing gastrectomy for gastric cancer: a systematic review and meta-analysis. BMC Surg. (2019) 19:129. doi: 10.1186/s12893-019-0593-6
122. Pang H, Dai L, Chen L, Chen X, Chen Z, Zhang S, et al. Prognostic value of the advanced lung cancer inflammation index in patients with gastric cancer after radical gastrectomy: a propensity-score matching cohort study and meta-analysis. BMC Cancer. (2024) 24:583. doi: 10.1186/s12885-024-12349-9
123. Liu LB, Li J, Lai JX, Shi S. Harnessing interventions during the immediate perioperative period to improve the long-term survival of patients following radical gastrectomy. World J Gastrointest Surg. (2023) 15:520–33. doi: 10.4240/wjgs.v15.i4.520
124. Katai H, Mizusawa J, Katayama H, Morita S, Yamada T, Bando E, et al. Survival outcomes after laparoscopy-assisted distal gastrectomy versus open distal gastrectomy with nodal dissection for clinical stage IA or IB gastric cancer (JCOG0912): a multicentre, non-inferiority, phase 3 randomised controlled trial. Lancet Gastroenterol Hepatol. (2020) 5:142–51. doi: 10.1016/S2468-1253(19)30332-2
125. Kim HH, Han SU, Kim MC, Kim W, Lee HJ, Ryu SW, et al. Effect of laparoscopic distal gastrectomy vs open distal gastrectomy on long-term survival among patients with stage I gastric cancer: the KLASS-01 randomized clinical trial. JAMA Oncol. (2019) 5:506–13. doi: 10.1001/jamaoncol.2018.6727
126. Yu J, Huang C, Sun Y, Su X, Cao H, Hu J, et al. Effect of laparoscopic vs open distal gastrectomy on 3-year disease-free survival in patients with locally advanced gastric cancer: the CLASS-01 randomized clinical trial. JAMA. (2019) 321:1983–92. doi: 10.1001/jama.2019.5359
127. Hyung WJ, Yang HK, Park YK, Lee HJ, An JY, Kim W, et al. Long-term outcomes of laparoscopic distal gastrectomy for locally advanced gastric cancer: the KLASS-02-RCT randomized clinical trial. J Clin Oncol. (2020) 38:3304–13. doi: 10.1200/JCO.20.01210
128. Ma Z, Bao X, Gu J. Effects of laparoscopic radical gastrectomy and the influence on immune function and inflammatory factors. Exp Ther Med. (2016) 12:983–6. doi: 10.3892/etm.2016.3404
129. Aoyama T, Yoshikawa T, Hayashi T, Hasegawa S, Tsuchida K, Yamada T, et al. Randomized comparison of surgical stress and the nutritional status between laparoscopy-assisted and open distal gastrectomy for gastric cancer. Ann Surg Oncol. (2014) 21:1983–90. doi: 10.1245/s10434-014-3509-9
130. Fujii K, Sonoda K, Izumi K, Shiraishi N, Adachi Y, Kitano S. T lymphocyte subsets and Th1/Th2 balance after laparoscopy-assisted distal gastrectomy. Surg Endosc. (2003) 17:1440–4. doi: 10.1007/s00464-002-9149-3
131. Shu ZB, Cao HP, Li YC, Sun LB. Influences of laparoscopic-assisted gastrectomy and open gastrectomy on serum interleukin-6 levels in patients with gastric cancer among Asian populations: a systematic review. BMC Gastroenterol. (2015) 15:52. doi: 10.1186/s12876-015-0276-4
132. Lv AQ, Huang LC, Lao WL, Song QL, Zhou QF, Jiang ZM, et al. Effects of different depth of anesthesia on perioperative inflammatory reaction and hospital outcomes in elderly patients undergoing laparoscopic radical gastrectomy. BMC Anesthesiol. (2022) 22:328. doi: 10.1186/s12871-022-01854-8
133. Liu W, Wu L, Zhang M, Zhao L. Effects of general anesthesia with combined epidural anesthesia on inflammatory response in patients with early-stage gastric cancer undergoing tumor resection. Exp Ther Med. (2019) 17:35–40. doi: 10.3892/etm.2018.6898
134. Wang L, Liang S, Chen H, Xu Y, Wang Y. The effects of epidural anaesthesia and analgesia on T lymphocytes differentiation markers and cytokines in patients after gastric cancer resection. BMC Anesthesiol. (2019) 19:102. doi: 10.1186/s12871-019-0778-7
135. Kun L, Tang L, Wang J, Yang H, Ren J. Effect of combined general/epidural anesthesia on postoperative NK cell activity and cytokine response in gastric cancer patients undergoing radical resection. Hepatogastroenterology. (2014) 61:1142–7.
136. Konstantis G, Tsaousi G, Kitsikidou E, Zacharoulis D, Pourzitaki C. The immunomodulatory effect of various anaesthetic practices in patients undergoing gastric or colon cancer surgery: A systematic review and meta-analysis of randomized clinical trials. J Clin Med. (2023) 12:6027. doi: 10.3390/jcm12186027
137. Liu R, Qin H, Wang M, Li K, Zhao G. Transversus abdominis plane block with general anesthesia blunts the perioperative stress response in patients undergoing radical gastrectomy. BMC Anesthesiol. (2019) 19:205. doi: 10.1186/s12871-019-0861-0
138. Moon J, Chun DH, Kong HJ, Lee HS, Jeon S, Park J, et al. The intraoperative administration of dexmedetomidine alleviates postoperative inflammatory response in patients undergoing laparoscopy-assisted gastrectomy: A double-blind randomized controlled trial. Biomedicines. (2023) 11:3253. doi: 10.3390/biomedicines11123253
139. Zhu M, Qi Y, He H, Zhang S, Mei Y. Effect of quadratus lumborum block on postoperative cognitive function in elderly patients undergoing laparoscopic radical gastrectomy: a randomized controlled trial. BMC Geriatr. (2021) 21:238. doi: 10.1186/s12877-021-02179-w
140. Lao WL, Song QL, Jiang ZM, Chen WD, Zheng XH, Chen ZH. The effect of oxycodone on post-operative pain and inflammatory cytokine release in elderly patients undergoing laparoscopic gastrectomy. Front Med (Lausanne). (2021) 8:700025. doi: 10.3389/fmed.2021.700025
141. Kim Y, Hwang W, Cho ML, Her YM, Ahn S, Lee J. The effects of intraoperative esmolol administration on perioperative inflammatory responses in patients undergoing laparoscopic gastrectomy: a dose-response study. Surg Innov. (2015) 22:177–82. doi: 10.1177/1553350614532534
142. Zang YF, Li FZ, Ji ZP, Ding YL. Application value of enhanced recovery after surgery for total laparoscopic uncut Roux-en-Y gastrojejunostomy after distal gastrectomy. World J Gastroenterol. (2018) 24:504–10. doi: 10.3748/wjg.v24.i4.504
143. Tang A, Zhou S. Analysis on the application value of goal-directed fluid therapy in patients undergoing laparoscopy-assisted radical gastrectomy with fast-track anesthesia. Am J Transl Res. (2021) 13:5174–82.
144. Miyachi T, Tsuchiya T, Oyama A, Tsuchiya T, Abe N, Sato A, et al. Perioperative oral administration of cystine and theanine enhances recovery after distal gastrectomy: a prospective randomized trial. JPEN J Parenter Enteral Nutr. (2013) 37:384–91. doi: 10.1177/0148607112458798
145. Cao W, Zheng C, Xu X, Jin R, Huang F, Shi M, et al. Clostridium butyricum potentially improves inflammation and immunity through alteration of the microbiota and metabolism of gastric cancer patients after gastrectomy. Front Immunol. (2022) 13:1076245. doi: 10.3389/fimmu.2022.1076245
146. Fu H, Li B, Liang Z. Effect of enteral immunonutrition compared with enteral nutrition on surgical wound infection, immune and inflammatory factors, serum proteins, and cellular immunity in subjects with gastric cancer undergoing a total gastrectomy: A meta-analysis. Int Wound J. (2022) 19:1625–36. doi: 10.1111/iwj.13763
147. Cheng Y, Zhang J, Zhang L, Wu J, Zhan Z. Enteral immunonutrition versus enteral nutrition for gastric cancer patients undergoing a total gastrectomy: a systematic review and meta-analysis. BMC Gastroenterol. (2018) 18:11. doi: 10.1186/s12876-018-0741-y
148. Sedman PC, Ramsden CW, Brennan TG, Giles GR, Guillou PJ. Effects of low dose perioperative interferon on the surgically induced suppression of antitumour immune responses. Br J Surg. (1988) 75:976–81. doi: 10.1002/bjs.1800751012
149. Romano F, Cesana G, Berselli M, Gaia Piacentini M, Caprotti R, Bovo G, et al. Biological, histological, and clinical impact of preoperative IL-2 administration in radically operable gastric cancer patients. J Surg Oncol. (2004) 88:240–7. doi: 10.1002/jso.20155
150. Rajala P, Kaasinen E, Raitanen M, Liukkonen T, Rintala E. Perioperative single dose instillation of epirubicin or interferon-alpha after transurethral resection for the prophylaxis of primary superficial bladder cancer recurrence: a prospective randomized multicenter study–FinnBladder III long-term results. J Urol. (2002) 168:981–5. doi: 10.1016/S0022-5347(05)64556-9
151. Schneider C, von Aulock S, Zedler S, Schinkel C, Hartung T, Faist E. Perioperative recombinant human granulocyte colony-stimulating factor (Filgrastim) treatment prevents immunoinflammatory dysfunction associated with major surgery. Ann Surg. (2004) 239:75–81. doi: 10.1097/01.sla.0000103062.21049.82
152. Wang X, Tang S, Cui X, Yang J, Geng C, Chen C, et al. Cytokine-induced killer cell/dendritic cell-cytokine-induced killer cell immunotherapy for the postoperative treatment of gastric cancer: A systematic review and meta-analysis. Med (Baltimore). (2018) 97:e12230. doi: 10.1097/MD.0000000000012230
153. Mu Y, Zhou CH, Chen SF, Ding J, Zhang YX, Yang YP, et al. Effectiveness and safety of chemotherapy combined with cytokine-induced killer cell /dendritic cell-cytokine-induced killer cell therapy for treatment of gastric cancer in China: A systematic review and meta-analysis. Cytotherapy. (2016) 18:1162–77. doi: 10.1016/j.jcyt.2016.05.015
154. Faghfuri E, Shadbad MA, Faghfouri AH, Soozangar N. Cellular immunotherapy in gastric cancer: adoptive cell therapy and dendritic cell-based vaccination. Immunotherapy. (2022) 14:475–88. doi: 10.2217/imt-2021-0285
155. Entezam M, Sanaei MJ, Mirzaei Y, Mer AH, Abdollahpour-Alitappeh M, Azadegan-Dehkordi F, et al. Current progress and challenges of immunotherapy in gastric cancer: A focus on CAR-T cells therapeutic approach. Life Sci. (2023) 318:121459. doi: 10.1016/j.lfs.2023.121459
156. Long B, Qin L, Zhang B, Li Q, Wang L, Jiang X, et al. CAR T−cell therapy for gastric cancer: Potential and perspective (Review). Int J Oncol. (2020) 56:889–99. doi: 10.3892/ijo.2020.4982
157. Wu X, Huang S. HER2-specific chimeric antigen receptor-engineered natural killer cells combined with apatinib for the treatment of gastric cancer. Bull Cancer. (2019) 106:946–58. doi: 10.1016/j.bulcan.2019.03.012
158. Yost KE, Satpathy AT, Wells DK, Qi Y, Wang C, Kageyama R, et al. Clonal replacement of tumor-specific T cells following PD-1 blockade. Nat Med. (2019) 25:1251–9. doi: 10.1038/s41591-019-0522-3
159. Wu TD, Madireddi S, de Almeida PE, Banchereau R, Chen YJ, Chitre AS, et al. Peripheral T cell expansion predicts tumour infiltration and clinical response. Nature. (2020) 579:274–8. doi: 10.1038/s41586-020-2056-8
160. Valpione S, Galvani E, Tweedy J, Mundra PA, Banyard A, Middlehurst P, et al. Immune-awakening revealed by peripheral T cell dynamics after one cycle of immunotherapy. Nat Cancer. (2020) 1:210–21. doi: 10.1038/s43018-019-0022-x
Keywords: gastric cancer, systemic immunity, detection, prognosis, cancer therapy
Citation: Zhang Y, Li J, Li J and Wang J (2024) Dysregulation of systemic immunity and its clinical application in gastric cancer. Front. Immunol. 15:1450128. doi: 10.3389/fimmu.2024.1450128
Received: 18 June 2024; Accepted: 22 August 2024;
Published: 05 September 2024.
Edited by:
Alessandro Mangogna, University of Udine, ItalyReviewed by:
Kyo Young Song, Catholic University of Korea, Republic of KoreaYuQin Sun, Zhangzhou Affiliated Hospital of Fujian Medical University, China
Copyright © 2024 Zhang, Li, Li and Wang. This is an open-access article distributed under the terms of the Creative Commons Attribution License (CC BY). The use, distribution or reproduction in other forums is permitted, provided the original author(s) and the copyright owner(s) are credited and that the original publication in this journal is cited, in accordance with accepted academic practice. No use, distribution or reproduction is permitted which does not comply with these terms.
*Correspondence: Jisheng Wang, MjQ0NzU0MzRAcXEuY29t; Jian Li, NjU0NzQ3OTczQHFxLmNvbQ==
†ORCID: Jian Li, orcid.org/0000-0002-5807-2360