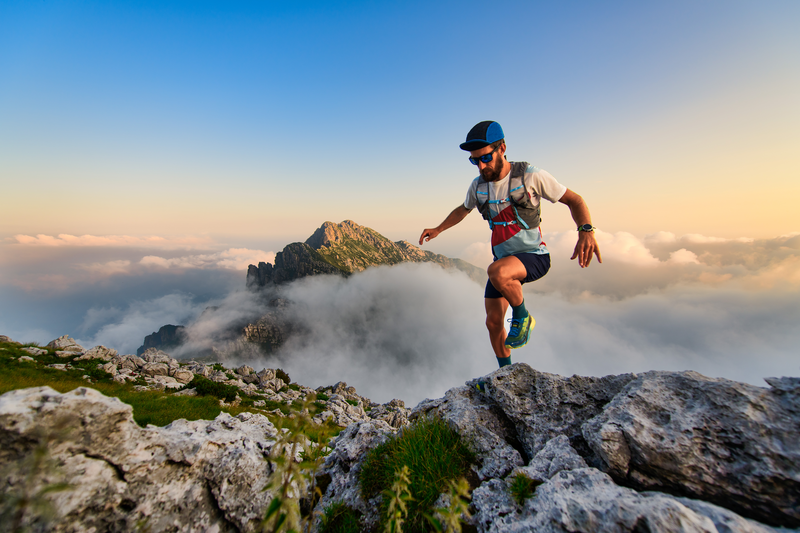
95% of researchers rate our articles as excellent or good
Learn more about the work of our research integrity team to safeguard the quality of each article we publish.
Find out more
REVIEW article
Front. Immunol. , 03 January 2025
Sec. Cytokines and Soluble Mediators in Immunity
Volume 15 - 2024 | https://doi.org/10.3389/fimmu.2024.1449411
This article is part of the Research Topic Modulation of Immune Response in Oral Health: Current Challenges and Future Directions View all 4 articles
Periodontal disease is a highly prevalent disease worldwide that seriously affects people’s oral health, including gingivitis and periodontitis. Although the current treatment of periodontal disease can achieve good control of inflammation, it is difficult to regenerate the periodontal supporting tissues to achieve a satisfactory therapeutic effect. In recent years, due to the good tissue regeneration ability, the research on Mesenchymal stromal/stem cells (MSCs) and MSC-derived exosomes has been gradually deepened, especially its ability to interact with the microenvironment of the body in the complex immunoregulatory network, which has led to many new perspectives on the therapeutic strategies for many diseases. This paper systematically reviews the immunomodulatory (including bone immunomodulation) properties of MSCs and their role in the periodontal inflammatory microenvironment, summarizes the pathways and mechanisms by which MSCs and MSC-EVs have promoted periodontal regeneration in recent years, lists potential areas for future research, and describes the issues that should be considered in future basic research and the direction of development of “cell-free therapies” for periodontal regeneration.
Periodontal tissue, a supportive structure comprised of various mineralized and non-mineralized tissues, including osteoid on the root surface of the teeth, alveolar bone, periodontal ligament, and gingiva (1). The concept of periodontal tissue regeneration refers to the regeneration of periodontal soft tissue, cementum, and alveolar bone. This process encompasses the emergence of new dentin, the reconstruction of functional periodontium, and the formation of fresh alveolar bone, among which the newborn and repair of alveolar bone is the basis of periodontal tissue regeneration. Periodontitis is a chronic inflammatory disease caused by oral microbial dysbiosis with plaque biofilm as the initiating factor and is one of the periodontal diseases with higher incidence and more severe symptoms. Its clinical features are destruction of periodontal supporting tissues, including gingival recession, attachment loss and alveolar bone resorption. In the clinical management of periodontal disease, periodontal tissue regeneration is usually used as the final treatment goal of periodontitis, under the premise of basic periodontal treatment and good control of inflammation, to realize tissue regeneration and functional reconstruction of periodontal lesions, or to accommodate subsequent restoration and implantation treatments.
Periodontitis is the leading cause of tooth loss in adult population and tooth loss, as a major symptom of advanced periodontitis, is inevitably associated with destruction and resorption of alveolar bone. The extensive loss of alveolar bone also poses great difficulties for subsequent prosthetic restoration or implant placement. Therefore, alveolar bone regeneration is clinically necessary to better restore patients’ masticatory function and oral and maxillofacial morphology (2). Research into the regeneration and reconstruction of periodontal supporting tissues has continued unabated over the past decades, ranging from the study of alveolar bone regeneration mechanisms to the exploration of the feasibility of new biological scaffolds to guide periodontal tissue regeneration, to the field of stem cells, which has attracted great attention in the past decade, the study of regenerative medicine has gradually deepened and become more popular.
Mesenchymal stromal/stem cells (MSCs), adult stem cells with multidirectional differentiation potential, are ubiquitously found in tissues such as bone marrow, adipose, muscle, peripheral blood, umbilical cord, and placenta (3). In recent years, MSCs have emerged as a focal point of research in the realm of tissue repair and regeneration, both in basic and clinical medicine, due to their exceptional accessibility, expansion capabilities, and multidirectional differentiation. Within dentistry, both odontogenic MSCs [e.g. Periodontal ligament stem cells (PDLSCs), Dental follicle stem cells (DFSCs), Dental-pulp-derived stem cells (DPSCs), Gingival mesenchymal stem cells (GMSCs), Stem cells from human exfoliated deciduous teeth (SHED)] and non-odontogenic MSCs [e.g. Bone marrow mesenchymal stem cells (BMSCs), Adipose-derived stem cells (ADSCs)] have been employed as seed cells for periodontal tissue engineering, demonstrating significant effects on periodontal tissue regeneration (Table 1). MSCs exhibit a beneficial immunomodulatory effect. This is primarily attributed to their low immunogenicity, characterized by negligible or minimal expression of MHC-II antigens (Table 2). Consequently, they cannot activate immune effectors such as lymphocytes, enabling them to be xenotransplanted for tissue repair (21). Furthermore, numerous studies have demonstrated that MSCs can suppress immune cell functions, including the proliferation and differentiation of T and B lymphocytes, as well as the secretion of inflammatory factors.
Table 2. Surface marker molecules of exosomes derived from human mesenchymal stem cells/human mesenchymal stem cells.
In the treatment of periodontal disease, the complexity of the periodontal tissue structure and its immune microenvironment is thought to be one of the reasons why periodontal tissue regeneration is difficult to achieve (22). Conventional treatments provide limited external control of inflammation and infection through mechanical scaling and do not regenerate lost alveolar bone and connective tissue. In contrast, after stem cell transplantation, a small number of stem cells can differentiate into tissue cells with specific functions in the area of periodontal lesions and directly participate in damage repair or even achieve cell replacement for tissue repair. At the same time, most of the stem cells secrete bioactive substances (e.g. growth factors, cytokines, chemokines) through exocytosis and other mechanisms to improve the regenerative environment of the periodontal lesion area from within the body, thus promoting periodontal tissue repair through the principle of cellular empowerment, and also providing the possibility of periodontal bone regeneration and complete regeneration of connective tissues. This paracrine effect varies according to different immune microenvironments, receptor cells and mechanisms. Extracellular vehicles (EVs) constitute a heterogeneous class of membranous vesicles that are incapable of replication. These vehicles exhibit diverse forms, perform a variety of functions, and mirror the physiological state of their cellular origin. They can/secret and produced by nearly all living cells. Depending on their cellular biogenesis, EVs can be broadly classified into three categories: exosomes, micro-vesicles, and apoptotic vesicles. The latest guidelines from the International Society for Extracellular Vesicles (ISEV) (2023) recommend the use of the generic term “EV” and its operational extensions (23). This is to avoid terms with inconsistent and sometimes misleading definitions, such as “exosome” or “micro-vesicle”, which are associated with difficult-to-establish biogenesis pathways. Therefore, it should be noted that in this paper, “MSCs-EV” refers specifically to exosomes of MSCs origin.
The periodontal immune microenvironment is a complex regulatory system composed of various host immune cells, extracellular matrix, and cytokines. These components regulate and influence each other, and any blockage or hyperfunction of any one of them may disrupt the overall regulation of the periodontal environment. Consequently, it is imperative to investigate the host immune regulation and the temporal dynamics of the periodontal immune microenvironment in the context of periodontal disease. Recent studies have elucidated that MSCs engage in intimate interactions with various immune and stromal cells at periodontal lesion sites. These interactions dictate the trajectory of disease progression and influence tissue regeneration. Concurrently, numerous researchers have investigated effective strategies and therapies that harness MSCs and their bioactive cargo to modulate host immune responses (24). This exploration is grounded in periodontal histopathology and immunology, offering a continuous stream of novel targets and insights for immunological studies and therapeutic approaches in periodontal diseases. In this review, we provide a comprehensive overview of MSC properties, advancements in research on immunoregulatory mechanisms during periodontal tissue regeneration and delineate the roles of MSCs in the interplay between the periodontal immune microenvironment, periodontal disease, and bone regeneration within the realms of periodontology and immunology/bone immunology. We also address current challenges faced by MSCs and MSC-derived extracellular vehicles (MSC-EV) in scientific research and discuss their implications for future directions in immunotherapy for periodontal diseases.
Localized plaque microorganisms primarily drive periodontal inflammation and bone destruction. These factors trigger an inflammatory response and bone resorption, which involve the release of endogenous cytokines from various immune cells and osteoclasts (OC). Some of these inflammatory factors act on different cells, mediating processes such as the inflammatory response and degradation of the bone matrix. They also contribute to secondary damage to periodontal tissues. Additionally, certain growth factors have a chemotactic effect on periodontal tissue regeneration. This is achieved by enhancing the ability of periodontal membrane cells to synthesize proteins, thereby contributing to the repair of periodontal tissues. In terms of periodontal bone regeneration, the recruitment of osteogenic precursor cells in the defect area and the induction of osteoblast (OB) formation are closely related to various regulatory factors and targeting pathways.
TGF-β, a pluripotent cytokine, exhibits direct cytostatic and anti-inflammatory effects. It exists in three isoforms: TGF-β1, TGF-β2, and TGF-β3. TGF-β3 facilitates the homing of endogenous MSCs and enhances tissue regeneration in situ by upregulating the secretion of monocyte chemoattractant protein-1 (MCP1) from vascular cells. This process primarily occurs through an indirect, smad3-dependent mechanism that accelerates MSC recruitment and initiates bone formation (24). In addition to its role in cartilage and odontoblast development, TGF-β primarily regulates bone repair in humans, upregulating genes associated with osteogenesis to maintain the balance between bone resorption and formation (25). Its role in inhibiting local inflammation during periodontal bone regeneration has also been increasingly recognized (26). A 2020 study found that varying levels of TGF-β1 had contrasting effects on osteogenic differentiation and bone healing. The mechanism may involve low-dose TGF-β1 activating smad3 to bind to the Bmp2 promoter, thereby upregulating Bmp2 expression in BMSCs. Conversely, high-dose TGF-β1 inhibited BMSC osteogenesis and diminished bone regeneration in vivo (27).
Matrix metalloproteinases (MMPs) are secreted by neutrophils, activated macrophages, and epithelial cells in periodontal inflammatory tissues. These include collagenases, elastases, and acid proteases, which play a crucial role in the destruction of periodontal tissue. The concentration of MMPs significantly increases during the active stage of periodontitis, making them an objective test indicator for determining whether periodontitis is active or inactive (28).
The primary isoforms of IL-1 encompass IL-1α, IL-1β, IL-1SS and IL-1 receptor antagonist (IL-1Ra), among others. IL-1 is a quintessential periodontal proinflammatory factor (29). In the context of periodontitis, IL-1 stimulates osteoclast activity, enhances the production of metalloproteinases, and intensifies collagen degradation within the periodontal matrix. Notably, IL-1β is deemed one of the principal destructive agents in periodontal tissues, making its concentration measurement a valuable diagnostic indicator for active periodontitis. IL-2 is predominantly secreted by Th1 cells, and recent research posits that genetic polymorphisms in IL-2 may be linked to the pathogenesis and prevention of periodontitis (30). IL-4 prompts the production of periodontal anti-inflammatory factors, such as CCL11 (31), and suppresses the transcription of proinflammatory factors, thereby impeding the progression of periodontitis. Furthermore, it has been postulated that IL-4 inhibits alveolar bone resorption by curtailing the formation of osteoclastogenic mediators via the IL-4/CCL22/CCR4 axis (32). IL-6 is a multifunctional cytokine that can be secreted by adipocytes and immune cells. The prevailing view is that IL-6 plays a pivotal role in exacerbating periodontal inflammation and facilitating osteoclastic bone resorption. However, recent studies have highlighted that IL-6 might exhibit an opposing effect in specific environments, such as inhibiting bone resorption and promoting the osteogenic differentiation of hPDLSC (33). IL-8 is a chemokine with pronounced effects on neutrophils, macrophages during inflammation, lymphocytes, and more.IL-10, an anti-inflammatory factor primarily derived from T cells and their subpopulations, plays a crucial role in the development of periodontitis. It inhibits this disease by acting on various immune cells. IL-13, a multifunctional helper T-cell 2 (Th2) cytokine, mitigates inflammatory responses. It can impede the progression of periodontitis by inducing the upregulation of TGF-β and downregulating MMP-1 production, both of which are involved in the regulation of collagen homeostasis in gingival fibroblasts (34). IL-17, another multifunctional cytokine mainly secreted by Th17 in periodontal tissues, contributes to the development of inflammation and bone destruction in periodontitis. In the mouse model of experimental periodontitis, it has been observed that the IL-17/Th17 response leads to the exacerbation of bone loss in early-stage periodontitis. However, IL-17 exerts some osteoprotective effects in advanced periodontitis by regulating the RANKL/OPG ratio (35). IL-23 also influences the development of periodontitis. Numerous studies have shown that elevated levels of IL-23 are directly correlated with the destruction of periodontal supporting tissues.
Tumor necrosis factor-α (TNF-α) is a pro-inflammatory cytokine that not only promotes the secretion of PGE2 but also stimulates the maturation of pro-osteoclasts and enhances bone resorption. Several studies have demonstrated that inhibiting TNF-α can effectively impede osteoclast formation. Interferon-γ (IFN-γ), another pro-inflammatory cytokine primarily secreted by Th cells, exhibits a dual function in tissue regeneration. On one hand, it encourages collagenase synthesis, while on the other, it restrains the proliferation of fibroblasts. These effects are contingent upon the specific injured tissues and the prevailing microenvironmental conditions. It has been observed that IFN-γ augments osteoclast genesis and periodontal destruction, leading to periodontal bone loss (36). Additionally, IFN-γ suppresses the activity of exogenous mesenchymal stem cells, which are crucial for bone regeneration. Conversely, a study by Gao Y et al. identified two opposing properties of IFN-γ: it directly inhibits osteoclast formation by targeting osteoclast precursors and indirectly promotes osteoclast genesis by stimulating antigen-dependent T-cell activation, causing these T-cells to secrete osteoclastogenic factors such as RANKL and TNF-α. However, during systemic inflammation, these opposing forces achieve a net balance that favors bone resorption (37).
Bone Morphogenetic Proteins (BMPs) are among the most potent growth factors contributing to bone formation. Except for BMP-1, all members of the BMP family belong to the TGF-β superfamily. These proteins play a crucial role in the development and reconstruction of maxillofacial and alveolar bone (38). They also promote the proliferation of human periodontal ligament cells (hPDLCs) and facilitate the mitotic division of undifferentiated mesenchymal stromal cells in periodontal tissues, leading to their differentiation into osteoblasts and odontogenic osteoblasts. hPDLCs possess properties like those of MSCs, making them suitable for xenografts. Furthermore, they contribute to the regulation of microenvironmental homeostasis and tissue regeneration in periodontal connective tissues.
Osteoprotegerin (OPG) inhibits the formation of osteoclasts, and an increase in OPG secretion leads to increased bone mass. Conversely, osteoporosis is observed in mice deficient in OPG. The receptor activator of NF-κB (RANK) serves as the sole target signaling receptor on the surface of osteoclast precursor cells. It activates these cells by binding to RANKL, thereby initiating a signaling program. The ligand for the nuclear factor kB receptor activator of NF-κB (RANKL) is expressed on the surface of osteoblasts and stimulates the differentiation and maturation of osteoclasts. OPG can function as a decoy receptor for RANKL, competing with or antagonizing RANK (42). This prevents the activation of the RANKL/RANK pathway during the osteoclast process, thereby inhibiting bone resorption. Both OPG and RANKL are expressed in periodontal tissues. An increase in RANKL secretion leads to hyper resorption of periodontal bone. The OPG/RANK/RANKL triad is central to the bone immunity/bone regeneration regulatory pathway. Other periodontal cytokines and hormones lack corresponding receptors on the osteoclast surface, resulting in minimal modulation of bone regeneration by other pathways. The balance between MSCs (MSC-EV) and the OPG/RANK/RANKL signaling axis has been shown to be critical for the success of periodontal bone regeneration, orthodontic treatment and implant restoration (43, 44).
The WNT family comprises a group of secreted glycoproteins that influence neural crest-derived cells via various signaling pathways. These pathways regulate the development of teeth and maxillofacial bone, as well as participate in the regulation of bone homeostasis and density during maxillofacial bone remodeling. The signal transduction pathways can be broadly categorized into the Wnt classical pathway and the Wnt non-classical pathway, also known as the Wnt/β-Catenin pathway. This pathway is activated by Wnt1, Wnt3a, Wnt8, etc., and stimulates target genes by stabilizing β-Catenin in the nucleus. The non-classical Wnt pathway, activated by Wnt4, Wnt5a, etc., can function at both transcriptional and non-transcriptional levels. In mouse models, osteoblasts can highly express WNT proteins. After co-culturing with MSCs, the Wnt signaling pathway in osteoblasts is activated, leading to enhanced osteogenic properties. It has been demonstrated that Wnt5a regulates RANKL expression in periodontal ligament cells (PDLC) (45).
The Wnt signaling pathway has been a major focus in recent years in the study of oral and maxillofacial tissue regeneration and tissue engineering. Recent studies in the last two years have shown that hPDLSCs regulate periodontal tissue homeostasis and periodontal regeneration through different Wnt pathways, and that classical and non-classical Wnt pathways are triggered by different mechanisms (33). And activation of the Wnt/β-catenin pathway can regulate the phenotypic switch of macrophages in periodontal tissues (promoting polarization from M1 to M2 phenotype) (46), which plays a role in suppressing immune dysregulation and promoting periodontal regeneration.
The body’s immune system maintains physiological homeostasis and defends against pathogens by inducing immune responses, as is the case within the periodontal immune system. Immunomodulation is the role of the body’s immune system in controlling the type and intensity of the immune response and is primarily immunosuppressive in nature. The basic cells involved in immunomodulation are the immune cells, and these different types of regulatory cells interact in a variety of ways with some of the immunomodulatory molecules to keep the body’s immune response within homeostatic limits. Immune cells are broadly divided into lymphocytes and myeloid cells: lymphocytes include T cells, B cells and natural killer (NK) cells; myeloid cells are derived from myeloid precursor cells in the bone marrow, including neutrophils, monocytes/macrophages, mast cells, dendritic cells, etc. In the following, we state the relationship between the immunomodulation and action of MSCs on different types of immune cells.
The immunomodulatory effects of MSCs on T cells are particularly complex (47). It can be achieved through direct contact with immune cells and modulation of the secretion of cytokines, chemokines and growth factors (48). For example, the mechanism of immunosuppression by MSCs through direct contact with lymphocytes is to promote apoptosis through the classical Fas/FasL pathway, which is highly expressed on the surface of lymphocytes and can combine with FasL on the surface of MSCs to initiate the apoptotic program during direct contact (49); it has been found that MSCs inhibit the production of cytokines by T cells (IL-2, TNF-α, IFN-γ, IL-6, etc.) and increase the expression of IL-10 and TGF-β in T cells (50). In addition, MSCs inhibit the proliferation of cytotoxic T cells (CTL), thus inhibiting the production of cytotoxins and playing an indirect role in suppressing the immune response (51). The interleukin family, interferon and some other cytokines secreted by T lymphocytes and their subpopulations play a role in periodontal lesions through different mechanisms and pathways. The following figure briefly describes the network of their roles with cytokines in the development and regression of periodontitis and the involvement of MSCs in the regulation of the same, using T lymphocytes and their subpopulations as an example (Figure 1, Table 3).
Figure 1. This figure shows the mechanism of action of T lymphocytes and their subpopulations, cytokines in the periodontal microenvironment and the direction leading to disease regression, where green arrows indicate the direction of cell differentiation; black arrows indicate secretion, production of cytokines; red lines indicate promotion, up-regulation; green lines indicate inhibition, down-regulation; and grey arrows indicate the direction of bringing about a reversion in the nature of the microenvironment.
The CD4+/CD8+ phenotype is a co-receptor for T cells expressing the TCR complex, which is restricted by MHC class II/I molecules on the surface of antigen presenting cells and is therefore differentiated during antigen recognition. CD4+ T cells can differentiate into specialized effector subsets that can play a role both directly in protective immunity and in coordinating other immune molecules to play a role in adaptive immunity (56). CD8+ T cells express CD8 molecules. Their specialized subsets, CD8+ Treg (Except here is special labelling, all other “Treg” in the article refer to CD4+ Treg.) have been shown to influence the dynamic balance between osteogenesis and osteoblast genesis in the progression of periodontitis by inhibiting osteoclast genesis and modulating the immune response in the periodontal microenvironment (57). Many studies have found that the ratio of localized periodontal CD4+ to CD8+ T cells is significantly lower in periodontal inflammation compared to healthy individuals (58). One study found that human periodontal ligament stem cells (hPDLSC) inhibited the proliferation of CD4+ T lymphocytes, and their effect was enhanced by IFN-γ and IL-1β (59). In a study of periodontitis in patients with systemic lupus erythematosus (SLE), researchers found a negative correlation between the CD4/CD8 ratio and the periodontal index due to T lymphocyte activation in SLE, suggesting that the ratio may be a measure of the severity of periodontitis in SLE patients (60).
It is worth noting that in recent years, regulatory T cells (Treg) and helper T cells (Th cells) have received increased attention, such as the migration and recruitment of Treg cells in the microenvironment, the cooperation between Treg cells and other types of immune cells, and the distinct roles played by Treg cells in different disease processes, etc. (60–64). Both Treg and Th cells belong to the subpopulation of CD4+ T cells. Th cells include T helper 1 (Th1), T helper 2 (Th2), T helper 17 (Th17), T helper 9 (Th9), etc. These cells need different cytokines. These cells require different cytokines and transcription factors to be activated and are also capable of secreting different cytokines that have different effects in different immune microenvironments. For example, Th1 cells secrete IFN-γ and TNF-α to exert pro-inflammatory and pro-bone loss effects in the local microenvironment, whereas Th2 cells mainly secrete anti-inflammatory factors such as IL-10 and IL-4 to counterbalance Th1 function. MSCs induce Th1/Th2 in antagonistic relationships towards Th2 cells for anti-inflammatory immunomodulatory effects (54). The relationship between Treg and Th17 cells is highlighted below.
Treg are a naturally occurring subset of T cells in the normal human immune system with immunosuppressive effects, characterized by high expression of the transcription factor Foxp3. Upon antigenic stimulation, Treg cells can be differentiated from peripheral mature CD4+ T cells into inducible Treg (iTreg/adaptive Treg, aTreg), which regulate the adaptive immune response. Treg cells regulate the level of inflammation and maintain immune homeostasis by secreting IL-10, IL-12 and transforming growth factor-β. Th17 cells are differentiated from Th0 cells by antigen presentation or stimulation with pro-inflammatory cytokines (e.g. IL-1β, IL-6, IL-23). They have been shown to play a role both in host defense against pathogens and in the development of autoimmune diseases, as exemplified by their ability to induce tissue inflammation and secretion of a variety of cytokines (IL-17, IL-6, IL-21, TNF-α, etc.) and to enhance the antimicrobial capacity of neutrophils through the release of factors such as IL-1, IL-8, IL-23R and MMPs.
T lymphocytes in the periodontal microenvironment are similarly differentiated into different populations, including Treg cells and Th17 cells (65). Periodontitis has been shown to disrupt immune homeostasis by enhancing Th17 function and impairing Treg function (66). During the course of periodontitis, Treg and Th17 can also balance the ratio between the two, regulating each other and influencing periodontal bone loss by upregulating/downregulating the inflammatory response (67–69). When Th17 cells are increased and Treg cells are decreased, there is an increase in inflammatory cytokines and alveolar bone resorption, suggesting that Treg cells have a function in inhibiting periodontal bone loss while Th17 cells upregulate the inflammatory response and promote periodontal bone destruction (32). It has been found that local enrichment of Tregs restores local immune homeostasis, prevents bone loss and ameliorates local inflammation in a mouse model of periodontitis (70). Scholars have also found that Treg cells in mice with periodontitis exhibit characteristics of Th17 cells in the mid-stage of the disease, suggesting that Treg cells can transform into Th17 cells and suggesting a high degree of plasticity of Treg cells (71). In addition, many researchers have focused on the impact of the Treg/Th17 balance in innate and adaptive immunity in periodontal disease, for example, it has been proposed that in the presence of immunomodulatory factors such as NLRP3 (a protein complex involved in the regulation of innate immune responses), Foxp3 expression is suppressed, which disturbs the Treg/Th17 balance (i.e., Th17 cell/Treg ratio decreases) and increases the release of inflammatory cytokines, exacerbating periapical inflammation (72) and leading to periodontal damage. Therefore, one possible mechanism is that in early or progressive periodontitis lesions, Treg cells may be compensated to augment and attenuate the adaptive immune response. However, as periodontitis progresses, the inflammation becomes chronic and Treg in the inflammatory microenvironment gradually lose their immunosuppressive function and are gradually dominated by other pro-inflammatory factors and replaced by dominant cells such as Th17.
On the one hand, MSCs can promote the differentiation of Th0 cells towards Treg cells (53), promote the secretion of anti-inflammatory factors, and then inhibit the proliferation of CD4+ and CD8+ cells, indirectly inhibiting the function of CD4+ and CD8+ T cells; On the other hand, in the case of inflammatory diseases in the body, the balance of Th cell subsets is disturbed, and MSCs can be used as a regulator to restore or reverse the imbalanced cell populations, such as MSCs can prevent Treg to Th17 conversion and inhibit Th17 function, thus realizing the regulatory effect of Treg/Th17 in periodontal diseases. For example, Zheng Y et al. found that pro-inflammatory PDLSCs-EV could regulate the balance of Th17/Treg cell ratio in the microenvironment of chronic periodontitis and attenuate the inflammatory response (73); A study in 2023 investigated the effect of hBMSC-EVs on the dynamic homeostasis of T cells in periodontitis (66). hBMSC were isolated from the maxilla of healthy volunteers, and by using miRNA sequencing, the differentially expressed miRNAs and target genes in EVs generated from hBMSC stimulated with LPS were investigated, It was found that EV-miR-1246 efficiently reduced the Th17/Treg ratio in vitro (resulting in a 75% reduction in the number of Th17 cells measured experimentally), and EV-miR-1246 was shown to promote periodontal regeneration in a mouse model of experimental periodontitis in animal studies. This undoubtedly represents a promising therapeutic target for the targeted treatment of periodontitis.
The main function of B lymphocytes is to mediate humoral immunity. When stimulated by antigens, B cells activate, differentiate into plasma cells and produce antibodies. In addition, B cells and their subpopulations also play a role in immune regulation and tolerance. B cells have many subpopulations, such as B1 cells, B2 cells, B effector cells, and regulatory B cells (Breg).
B1 and B2 cells are classical B lymphocytes involved in intrinsic and humoral immune responses, respectively. While effector B cell subpopulations mainly play a secretory role in a specific immune microenvironment stimulated by specific cytokines, Breg cells, similar to Treg cells, are a type of B cell with a negative regulatory role that can produce large amounts of IL-10 and regulate the balance between Th1 and Th2, thereby suppressing harmful immune responses (74). Numerous studies have demonstrated the immunosuppressive effects of Breg, e.g. Breg can inhibit Th1 and Th17 responses and induce FoxP3+ Treg pools, which play a key role in maintaining peripheral tolerance (69, 75). These cells may become new targets for the treatment of inflammatory diseases and are a hot spot for future research.
A study in periodontal disease confirmed that Breg inhibits inflammation produced by immune cells such as T cells and reduces the expression of pro-inflammatory cytokines through the secretion of IL-10 (B10 cells), and that it inhibits RANKL-expressing neutrophils and blocks alveolar bone loss in experimental periodontitis in mice (76). Thus, Breg plays a role in inhibiting bone loss in periodontitis.
The vast majority of researchers believe that MSCs can inhibit the proliferation and differentiation of B cells through soluble cytokines (TGF-β, PGE2, etc.) and regulate the production and secretory function of Bregs through intercellular contacts, extracellular vesicles and other modalities, and exert an anti-inflammatory effect by promoting the increase of Bregs (69). In a minipig periodontitis model, MSCs block B cells in the G0/G1 phase of the cell cycle through intercellular contacts, inhibit B cell activation and proliferation, and induce Bregs production, thereby reducing humoral immunity, a process mediated mainly through programmed cell death protein 1 (PD-1) and PD-L1 (77). In contrast, MSCs-EV inhibit B cell proliferation and BCR-mediated Ca2+ mobilization and regulate the PI3K-AKT signaling pathway, which is an important link in the regulation of Breg cell development and an upstream pathway that regulates macrophage M1/M2 polarization (78). In addition, in contrast to reducing humoral immunity, a previously unrecognized function of PDLSCs in regulating humoral immunity has been proposed, i.e. when co-cultured with normal B cells, PDLSCs enhance B cell activity by upregulating IL-6 secretion (79) and promote B cell proliferation. Previous studies have shown that in active periodontitis pathology, IL-6 can be secreted by the body’s memory B cells, promoting inflammatory infiltration and facilitating periodontal tissue destruction (80). However, it has also been pointed out that IL-6 can also play a role in inhibiting bone resorption when the level of RANKL in the local microenvironment is altered (81), so we believe that in the disease process intervened by MSCs, their effect on the function of B cells at different stages of periodontitis may also vary depending on the developmental stage of the B cells and the changes in the microenvironment (the function of bioactive factors), and the mechanism needs to be further explored.
Monocytes are derived from hematopoietic stem cell precursors in the bone marrow and, when stimulated by inflammatory signals, can rapidly reach the site of infection, divide and differentiate into macrophages and dendritic cells. Macrophages can phagocytose pathogenic microorganisms, recognize antigens and relate signaling molecules, secrete inflammatory mediators and initiate intrinsic immune responses.
Macrophages are considered to be a highly heterogeneous population of cells, and the functions of individual populations can differ significantly in vivo (82). Among them, M1 (pro-inflammatory phenotype) and M2 (anti-inflammatory phenotype) are functionally distinct and easier to distinguish and are the two phenotypes that play important roles in the inflammatory microenvironment. The mechanism by which stem cells induce macrophage polarization towards the M1/M2 phenotype has been a hot research topic in recent years. For example, macrophage involvement in the development of periodontitis has been well documented (83, 84) and macrophage conversion from M1 to M2 promotes periodontal tissue regeneration and inhibits alveolar bone loss (85). For example, in the early stages of inflammation, such as the development of gingival inflammation and the appearance of periodontal pockets, M1 macrophages predominate and produce pro-inflammatory factors to enhance the inflammatory response. As the inflammation progresses to a certain level, M2 macrophages are gradually activated and play a role by releasing large amounts of IL-10, anti-inflammatory cytokines, to promote periodontal tissue regeneration.
Many studies have demonstrated the role of MSCs in stimulating monocytes/macrophages to convert to the M2 phenotype in the treatment of inflammatory diseases such as periodontitis. In the experiments of Liu J et al (85), the levels of IL-10 were increased in the rat group treated with PDLSCs, while the levels of TNF-α were decreased, suggesting that PDLSCs may promote the repair of periodontal function by influencing macrophage polarization, reducing periodontal tissue inflammation and promoting periodontal tissue regeneration. Gao X et al. (86) First, SHED and monocytes/macrophages were co-cultured in the Transwell system, and then a rat periodontitis model was used to observe the effect of SHED on periodontal regeneration. The result suggests that SHED may promote periodontal bone regeneration by promoting macrophage polarization to the CD206+ M2-like phenotype. Pang QM et al. demonstrated that peripheral blood mesenchymal stromal/stem cells (PBMSC) and their culture medium can mediate M2 macrophage polarization through activation of the IL10/STAT3 pathway, which promotes tissue repair and favors functional improvement in inflammatory diseases (87). In addition, a study in 2022 (88) showed that PDLSCs conditioned medium (PDLSCs-CM) significantly increased the expression of anti-inflammatory factors such as IL-10, TGF-β and CCL18, which in turn induced and enhanced IL-4 and IL-13-induced polarization of M2 macrophages.
Furthermore, MSCs can act synergistically with macrophages to enhance bone healing by modulating inflammation and promoting regeneration. For example, MSCs affect macrophage polarization, which in turn affects osteoclast differentiation and bone repair processes. Macrophages can activate endogenous MSCs and promote their differentiation into the osteogenic lineage, which is essential for effective bone tissue engineering and repair (89); macrophages can influence MSC differentiation by releasing extracellular vesicles, such as apoptotic vesicles enriched in miR155 (90). These vesicles regulate MSC fate mainly by modulating pathways involved in osteogenesis and adipogenesis. However, a number of scholars have similarly noted that under specific conditions, the function of MSCs is reversed by the influence of macrophages of different phenotypes, and this process is regulated by the concentration of inflammatory factors, e.g., when there is a lack of IL-6 in the local microenvironment, MSCs induce the differentiation of M0 into M1, which secretes TNF-α and IFN-γ and expresses the co-stimulatory molecule CD86, which contributes to T cell activation, which in turn produces a large number of inflammatory factors and stimulates MSCs to exert anti-inflammatory effects through a negative feedback mechanism. Therefore, the dynamic change of inflammatory factor concentration in the local microenvironment plays an important role in the pro-inflammatory or anti-inflammatory effects of MSCs and the transformation of macrophages of M1 and M2 phenotypes.
Recent studies have shown that macrophages exhibit significant plasticity, and dynamic changes in the local microenvironment can modulate their phenotype to produce changes beyond M1 and M2, as evidenced by different gene expression profiles associated with the M1 and M2 states, as well as intermediate and mixed states that do not fully conform to the M1/M2 dichotomy (91). Studies using advanced technologies such as single-cell RNA sequencing have identified new macrophage subpopulations in the tumor microenvironment, characterized by unique transcriptomic profiles distinct from traditional M1/M2 markers (92). Specific tumor macrophage (TAM) subpopulations have been identified as TREM2+, SPP1+, etc., each of which has a distinct role in cancer prognosis and treatment response.
Therefore, we believe that the M1 and M2 phenotypes are more inclined to be two idealized, borderline opposing forms of macrophages, and that the M1/M2 classification provides a practical framework for our understanding of macrophage function, but the complexity of macrophage biology suggests that macrophage activation states are continuous and dynamically regulated by the local microenvironment. Therefore, understanding the full spectrum of macrophage activation states can lead to more precise therapeutic strategies, and the interactions between MSCs and different phenotypes of macrophages in the microenvironment will be more precise if a more nuanced approach is adopted in research and therapy; therefore, identifying and targeting specific macrophage phenotypes in disease-targeted therapies is expected to improve the prognosis of a variety of diseases, and further probing into the interaction with the MSCs action of crosstalk will be of great benefit.
Dendritic cells (DCs) are antigen-presenting cells that capture, process and present antigens to lymphocytes to initiate and regulate adaptive immune responses and are therefore closely related to T lymphocytes with which they can form a unique immunoregulatory network. Dendritic cells direct the differentiation of CD4 T cells into T cell subpopulations such as Th1, Th2, Th17 and Treg cells and maintain their numbers (93). Prolonged exposure of periodontal tissues to oral bacteria stimulates an inflammatory response that leads to gingivitis or periodontitis. Dendritic cells play a destructive role in periodontal tissues by secreting inflammatory factors, mainly through activation of the acquired immune response (94).
The effects of MSCs on periodontal DCs can be direct or indirect. For example, MSCs can come into direct contact with DCs and inhibit their maturation, causing them to remain at a low differentiation stage and thus lose their ability to present antigens to down-regulate periodontal inflammation (95); Monica Reis, et al. confirmed that MSC-EV treatment inhibited DC maturation, reduced the secretion of pro-inflammatory cytokines (e. g., IL-6) in the microenvironment, and increased the secretion of anti-inflammatory cytokines (e. g., TGF- β) (55); MSCs can also stimulate the development of monocytes into DCs with immune-tolerant properties, resulting in an increase in the secretion of IL-10 and a decrease in the secretion of IFN-γ, TNF-α and IL-12, which impairs the antigen-presenting ability of DCs and thus inhibits T-cell activation and proliferation (96). Therefore, this process may play a role in preventing overactivation of the periodontal immune response.
Natural killer (NK) cells are important immune cells in the body that have an immunosurveillance role and can non-specifically kill a variety of target cells without antigenic stimulation and play an important role in regulating the balance of periodontal osteoblasts/osteoclasts (97). MSCs can inhibit the proliferation of NK cells and their secretion of inflammatory factors and reduce their non-specific killing effect on target cells. In recent years, several studies on natural killer T cells (NKT) have reported their immunomodulatory role in periodontal disease. Natural killer T cells are essentially a distinct subpopulation of T lymphocytes with some of the properties of NK cells that produce anti-inflammatory and pro-inflammatory factors involved in immunomodulation and play a predominantly pro-inflammatory role in the development of periodontitis. For example, a recent study showed that in the early stages of alveolar bone injury, NKT cells can interfere with the osteogenic differentiation of MSCs by upregulating inflammatory factors (e.g. CXCL2), thereby adversely affecting alveolar bone repair (98). However, whether MSCs can regulate the function of NKT cells in periodontal regeneration is still unknown and requires further investigation.
Neutrophils are intrinsic immune cells and important cells in the defense process of periodontitis, with a complex network of interactions with other types of immune cells. In addition to phagocytosis, secretion of antimicrobial molecules and enzymes, neutrophils can synthesize pro-inflammatory mediators to modulate adaptive immunity and activate osteoclasts to promote the development of periodontal inflammation. MSCs can play an immunosuppressive role by inhibiting NO secretion from neutrophils, reducing neutrophil infiltration and other mechanisms (99), and also indirectly modulate the number and activity of neutrophils in the microenvironment and by regulating Breg cells. It may also indirectly regulate the number and activity of neutrophils in the microenvironment by regulating Breg cells.
MSCs play an important immunomodulatory role in periodontal tissue regeneration at different stages of the periodontal disease process. In the early stage of periodontitis, endogenous MSCs can assist the host in activating the immune defense and mediate the expansion of the inflammatory process (100). Meanwhile, as the lesion progresses, MSCs can inhibit the inflammatory response through interactions with immune cells and paracrine mechanisms, causing the periodontal inflammation to pass through the high-level inflammatory stage as soon as possible and preventing the delay and entry into the chronic inflammatory state. For example, gingival tissues, as the most involved site in periodontal inflammation, have native GMSCs that play a pivotal role in regulating inflammation (101). Although GMSCs have been discovered relatively recently, they have been shown to be unique MSCs with inflammation resistance that can maintain stable stemness and multispectral differentiation potential in response to continuous stimulation by inflammatory factors (102). The immunomodulatory function of GMSCs has also been more studied, which is able to inhibit the function of immune cells by synthesizing PGE2 and secreting anti-inflammatory factors such as IDO and IL-10. In addition, under conditions of local inflammation, TNF-α secreted by M1 macrophages in the microenvironment can stimulate GMSCs to produce anti-inflammatory factors such as IL-6, and at the same time increase the number of secretion of GMSCs-EVs and increase the expression level of CD73, which induces the polarization of macrophages toward the M2 phenotype, thus rapidly controlling and reversing the inflammatory environment (45). Recent studies have shown that gingival mesenchymal stem cells derived from rheumatoid arthritis patients(RA-GMSC) can effectively inhibit T-cell proliferation, pro-inflammatory cytokine secretion, and osteoclast differentiation in vitro, as well as reduce Th1 and Th17 cell ratios and elevate Treg cell ratios, thus demonstrating significant efficacy in a model of collagen-induced arthritis (CIA) (103). And exogenous transplantation of MSCs may also lead to organic changes in the local microenvironment of tissues, freeing chronic inflammation from the dilemma of stasis and restarting tissue repair of chronic injuries (e.g. spinal cord injury (104) and chronic periodontitis), which may be beneficial for disease treatment., and these processes cannot be separated from the role of MSCs-EVs. And the above regulatory functions are microscopically controlled by the balance and coordination of many signaling pathways and environmental factors.
Numerous studies have shown that MSCs enter the organism and their live cell signals are no longer detectable after a relatively short period of time, yet they can have profound and lasting effects on disease treatment and tissue regeneration (105), which brings us to the paracrine role of MSCs.
MSC-EVs are membranous vesicles secreted by living cells from the late nuclear endosome, which is naturally present in body fluids including blood, saliva, urine and breast milk. MSCs can release a variety of biologically active factors through MSCs-EV, such as proteins, lipids, deoxyribonucleic acid (DNA), messenger RNA (mRNA), circular RNA (circRNA), microRNA (miRNA) and other non-coding RNAs (Table 4). They act as endogenous carriers of genetic material or chemical mediators, realize information transfer in intercellular communication, regulate the cellular biological activity of recipient cells and also participate in the immune regulation of the organism. For example, HUCSC-EVs have been shown to decrease pro-inflammatory cytokines such as tumor necrosis factor α, IFN-γ and IL-17A, while increasing anti-inflammatory cytokines such as TGF-β and IL-10 in periodontal tissues. This modulation contributes to the attenuation of inflammatory responses associated with periodontitis (120). MSCs pre-treated with TNF-α can produce EVs with enhanced immunomodulatory properties. These EVs inhibit pro-inflammatory markers and enhance repair markers in macrophages, contributing to reduced inflammation and improved bone regeneration in the tissue repair environment (121).
Consequently, their anti-inflammatory and immunosuppressive effects in different environments can be used as an effective tool in the treatment of periodontitis. Considering that MSCs-EV is an excellent bioactive carrier, there have been numerous studies and explorations of “cell-free therapy” strategies in recent years, and many scientists have attempted to use MSC and MSCs-EV in the treatment of periodontitis and have made preliminary progress in clinical trials (122, 123). However, due to the stability and retention of MSC-EVs in vivo after application (124), their main form of application in preclinical studies currently relies on the use of various types of hydrogels, collagen gel sponges, synthetic polymers and other biomaterials to encapsulate them for prolonged tissue retention and maintenance of bioactivity (125).
The pathology of periodontitis is essentially a tissue defense following bacterial infection, with plaque microorganisms as initiating factors triggering inflammatory responses of varying degrees in the periodontal tissues. The inflammatory microenvironment (126, 127) contains a variety of immune cells and related inflammatory factors that are highly aggregated locally in the inflamed tissues and can recruit and mobilize endogenous MSCs, as well as chemotactic homing of exogenous MSCs, activate the function of MSCs and drive the inflammatory microenvironment in a direction that favors regeneration through immune modulation. Similarly, once the internal and external environment conducive to tissue repair is disrupted, the function of MSCs is inhibited or apoptosis occurs and the local inflammatory cytokine imbalance is restored, which can plunge the tissue back into a prolonged inflammatory environment.
Under normal conditions, the body’s MSCs are controlled and quiescent and do not have the ability to immunomodulate (128). Instead, MSCs can be activated under the stimulation of periodontal inflammatory factors. In a low-inflammatory environment, MSCs can recruit immune cells or promote the formation of immune cells in the microenvironment to promote the development of inflammation, whereas when inflammatory cytokines exceed a certain threshold, MSCs can be converted to an anti-inflammatory phenotype (129), regulating immune cells by releasing anti-inflammatory factors (e.g. IL-4, IL-10) and reducing pro-inflammatory factors (TNF-α, IFN-γ). For example, in an inflammatory microenvironment dominated by IFN-γ and TNF-α, MSCs secrete a large number of T-cell chemokines and upregulate anti-inflammatory cytokines such as TGF-β and IL-10, which in combination exert an inhibitory effect on T-cell proliferation, thereby inducing immunosuppression; in oral soft tissue inflammation, local application of MSCs can induce local IFN-γ levels to some extent, inhibiting the immune response and increasing the efficiency of tissue repair and regeneration. However, whether it is pro-inflammatory or anti-inflammatory effects, we believe that MSCs can, within a certain range, act as an immune regulator, sense the changes in the immune microenvironment, support the normal function of all kinds of immune cells in the environment, and drive the immune microenvironment towards regeneration and repair.
Inflammatory factors in the microenvironment also influence the direction and intensity of the immunomodulatory effects of MSCs (130). It has been shown that in response to inflammatory stimuli, DFSCs can highly express periosteal proteins (a bioactive molecule closely related to periodontal homeostasis), which can improve the immune microenvironment in the inflammatory region by regulating macrophages (131). In addition, the inflammatory microenvironment dominated by pro-inflammatory factors influences the osteogenic and odontogenic differentiation of MSCs. In a mouse periodontal model, inflammatory PDLSCs-EV promoted macrophage polarization towards the M1 phenotype and upregulated the level of local inflammation through miR143-3p-mediated modulation of the PI3K/AKT/NF-κB pathway (108), whereas inflammatory DPSCs-EV exhibited pro-inflammatory properties like PDLSCs-EV. In a 2019 study, researchers used lipopolysaccharide (LPS) from Porphyromonas gingivalis to mimic the inflammatory microenvironment of chronic periodontitis. Porphyromonas gingivalis (P. gingivalis) is one of the three well-documented major periodontal pathogens that play a key role in the pathogenesis of periodontitis by disrupting host immune homeostasis (132) and in this model, exosomes were extracted from periodontal ligament stem cells (PDLSCs) in the chronic inflammatory milieu and compared with normal PDLSC exosomes, and again found that microRNA-155-5p in inflammatory PDLSCs-EV increased SIRT1 expression in CD4+ T cells, thereby attenuating inflammatory response in chronic periodontitis (73). In the periodontal ecological niche, high levels of inflammation in the microenvironment reduce the osteogenic properties of PDLSCs, and these impaired PDLSCs may disrupt the microenvironment by exacerbating the host immune response, promoting aberrant angiogenesis and promoting osteoclast activity (133).
In fact, inflammatory factors often act in combination or synergistically to affect MSC function, for example, it has been found that GMSCs-EV generated by combined stimulation of TNF-α and IFN-α can promote the polarization of M2 macrophages by increasing the expression of CD73 and CD5L, which in turn enhances the anti-inflammatory function of the stem cells (134); IFN-γ can exert an inhibitory effect on osteogenesis of exogenous MSCs by enhancing TNF-α signaling and inhibition of the Runx2 pathway to exert an inhibitory effect on osteogenesis of exogenous MSCs (135), while TNF-α levels, when upregulated to a certain threshold, would have a combined effect with IFN-γ to promote apoptosis of endogenous and exogenous MSCs, which would adversely affect the periodontal regenerative environment.
Firstly, MSCs in the organism have the function of osteogenic differentiation, which can be induced by BMP, TGF-β and other conditions to differentiate into osteoblasts and then produce bone-like structures or even form new bone. Second, endogenous and exogenous MSCs are able to secrete various osteogenic factors and bioactive substances to induce bone marrow stromal cells, connective tissue mesenchymal cells, etc., to transform into osteoblasts, and also to promote osteogenic differentiation of peripheral MSCs through paracrine effects. Table 5 lists some representative studies on MSCs and MSCs-EV in periodontal bone regeneration in the last several years.
Table 5. Representative studies of MSCs and MSCs-EV in periodontal bone regeneration in the last several years.
In bone tissue, a dynamic balance between osteoclastic bone resorption and osteoblastic bone formation is essential to maintain normal alveolar bone volume. The mechanism of communication between the immune system and this dynamic balance is called osteoimmunology. Viewed through the lens of osteoimmunology from the perspective of homeostatic medicine (141), periodontitis/periodontal bone loss occurs because the host immune system triggers a disturbance in the osteogenic/osteoclastic balance through several pathways, such as upregulation of inflammation and RANKL. Several studies have shown that the inflammatory cytokines IFN-γ, TNF-α and IL-1b secreted by Th cells not only upregulate local inflammation but also inhibit osteogenic differentiation; approximately 50% of T cells and 90% of B cells in gingival tissue from patients with periodontitis express RANKL (142), which is an important source of RANKL in the imbalance of bone immune homeostasis; numerous studies have shown that dendritic cells and osteoclasts co-operate to mediate bone destruction and remodeling under inflammatory conditions (143), and dendritic cells has even been suggested to intervene in the inflammation-induced osteogenic/osteoclastic balance as an osteoclast precursor; NK cells, in addition to non-specific killing of target cells, express RANKL (144) under inflammatory conditions in a manner that promotes osteoclast apoptosis and are involved in bone immunomodulation. MSCs and MSC-EV may intervene in these perturbing factors to maintain the balance between periodontal bone immune homeostasis and host inflammation. For example, using a rat apical periapical inflammation model, Xiao L et al. found that the interaction of BMSC with macrophages could regulate inflammatory bone loss in the SPHK1-S1PR1-RANKL axis (145); BMSC-EV, which has typical immunomodulatory, tissue repair, anti-inflammatory and antioxidant roles, modulated osteoclast production and osteogenic differentiation in periodontal inflammation, shifting the local microenvironment in a direction favorable to regeneration.
MicroRNAs (miRNAs) are a class of endogenous non-coding small RNA molecules that are widely distributed in the body and regulate post-transcriptional target genes through complementary base pairing with mRNAs. miRNAs are considered to be an important component of stem cell biology, controlling the differentiation, immunomodulation and apoptosis of stem cells (odontogenic stem cells), as well as an important regulator of bone morphogenetic protein (BMP) (146). A 2022 study investigated whether SHED-derived exosomes could enhance the osteogenic capacity of hPDLCs (140). While the answer was positive, the researchers found a significant difference in miRNA content between SHED-derived exosomes and hPDLC-derived exosomes by analysis, suggesting that our miRNA has an important value in periodontal bone regeneration.
miRNAs can be carried by MSC-EVs and are being studied in many disciplines. For example, miR-1246 carried by hBMSC-EVs is a promising biomarker that has been used in many aspects of research, such as stem cell therapy, tumor immunity and elucidating the pathogenesis of certain diseases, and its use in tissue engineering is even considered as a potential key regulator of engineered DPSCs-derived exosomes (Ost-EVs) to promote periodontal tissue regeneration (147). In addition, in the body’s immune regulation, miRNAs may play a role in periodontal tissue regeneration by acting on various targets to regulate the signaling of key transcription factors (e.g. STATs) (148) and control the polarization and activity of cells such as macrophages. It has been shown that miR-1246 promotes M2 macrophage polarization and attenuates inflammation through the Wnt pathway, which in turn recruits MSCs to promote osteogenic differentiation.
ostoe-miRNAs refer to osteogenic differentiation-associated miRNAs, which are closely linked to the regulation of osteoblast differentiation and bone formation and accelerate bone healing through bone immunomodulation. For example, there is evidence that molecules such as miR-218 and miR374a can directly/indirectly promote osteogenesis of MSCs (ADSCs, PDLSCs) through the Wnt pathway (149, 150) and that down-regulation of their expression significantly inhibits the osteogenic differentiation of stem cells; several studies have shown that salivary expression of miR146a and miR155 is significantly increased in periodontitis patients (151, 152). They inhibit osteoclast genesis and stimulate activation of the NF-κB pathway. In addition, miR146a has been shown to be a predictive indicator of periodontitis in diabetic patients.
Studies on the involvement of miRNA-overexpressing MSC-EVs in immune regulation and bone metabolism have increased in recent years. Liu W et al. demonstrated that overexpressed EV-MiR-181b could promote hBMSC osteogenesis in vitro and bone healing in vivo by modulating the PRKCD/AKT pathway, promoting polarization of M2 phenotypic macrophages and inhibiting inflammatory responses (139). Nakao Y et al. found that miR-1260b overexpressed in GMSC-EV after inflammatory stimulation inhibited osteoclast activity by targeting the Wnt5a-mediated RANKL pathway (45) and promoted periodontal bone regeneration. Another study claimed that MiR-758-5p, which was upregulated in inflammatory DPSC-EVs, exerted a facilitating effect on the osteogenic and odontogenic differentiation of PDLSCs (153). Despite the promising therapeutic potential of MSCs-EV and its miRNA content, a number of challenges remain, e.g., the interaction of multiple miRNAs with their targets and the identification of the optimal miRNA therapeutic combinations are areas that require further research (154).
It is easy to see from the previous parts of the discussion that alveolar bone and periodontal tissue regeneration is a multifactorial synergistic process. It involves biomolecules and signaling pathways such as EV-miRNA, TGF-β/BMP, Wnt, PI3K/AKT, etc., which can act synergistically with each other and inevitably influence each other, resulting in a complex regulatory network (Figure 2). From controlling inflammation to maintaining the osteogenic/osteoblastic balance, the immunomodulatory effects of MSCs permeate the regulatory network of periodontal regeneration. As research continues to improve, more potential regulatory mechanisms remain to be discovered in the future. Below we will discuss a few research directions that have seen rapid growth in recent years.
Figure 2. This figure shows a partial of the currently known and unknown mechanisms by which MSCs promote periodontal regeneration. In addition to cell replacement, direct effects on immune cells and the immune microenvironment, they also include paracrine secretion and influence on OB/OC balance, etc. In addition, the inflammatory and regenerative microenvironments could have shifted to each other under certain conditions, and the regenerative effects of MSCs on the microenvironment as influenced by the microenvironment are not static, and more of these mechanisms need to be explored in the future.
Epigenetics refers to changes in gene expression during cell proliferation and division without altering the DNA sequence of the relevant genes, and its main molecular mechanisms include DNA methylation, histone modification, chromatin remodeling, non-coding RNAs, etc. In both heredity and disease development, the external environment can affect gene expression through epigenetics, resulting in biological phenotypic differences. A large body of evidence suggests that epigenetic mechanisms play an important role in the functional regulation of MSCs, which can influence the biological properties of MSCs and the regenerative potential of the participating bone-forming cells, and therefore are important for promoting periodontal bone regeneration and bone repair.
Histone acetylation is a post-translational histone modification that plays an important role in promoting osteogenic and dentinogenic differentiation of MSCs. In studies of periodontal tissue regeneration, histone deacetylase inhibitors (HDACi) have been shown to regulate osteogenesis and control inflammation by affecting epigenetics. It can promote MSC-mediated periodontal bone regeneration through mechanisms such as increasing the synthesis of osteogenesis-related proteins (e.g., osteoblasts, collagen-1α, and bone morphogenetic proteins) and inducing osteogenic differentiation of MSCs or osteogenic progenitor cells. In addition, both in vitro and animal studies have demonstrated the immunomodulatory ability of HDACi to inhibit T-cell activation and proliferation and to reduce the expression of pro-inflammatory factors. For example, it was shown that HDAC3 significantly reduced the expression of inflammatory mediators in gingival fibroblasts (GFs) from both healthy and diseased individuals in a model of periodontitis induced by TNF-α and Porphyromonas gingivalis (155). HDAC1 and HDAC3 can bind to NF-κB and promote the deacetylation process of NF-κB, thus inhibiting the inflammation mediated by NF-κB gene transcription and promoting periodontal tissue regeneration under inflammatory state.
Many studies have demonstrated that histone methylation plays an important role in regulating the dynamic balance of chromatin and the function of MSCs, and that histone methylation epigenetically regulates the differentiation of MSCs mainly by modifying gene transcripts. For example, in non-inflammatory environments, histone demethylases such as KDM6A, as well as certain miRNAs and lncRNAs, enhance the osteogenic capacity of PDLSC and promote osteogenesis. However, inflammation can inhibit osteogenic differentiation by upregulating DNA methylation of osteogenesis-related genes, modulating histone modifications, and causing alterations in noncoding RNAs (156); The histone demethylase KDM2A regulates the expression of the epidermal growth factor EREG and inhibits the osteogenic/odontogenic differentiation capacity of odontogenic MSCs and bone marrow-derived MSCs, thereby attenuating oral and maxillofacial bone regeneration.
Recent studies have shown that MSCs are also dependent on epigenetic modifications to differentiate into an osteoblastic lineage. Therefore, exploring the potential mechanisms of epigenetic regulation of MSC-driven differentiation may help us to enhance the activity and function of MSCs and provide new therapeutic targets for periodontal bone regeneration, which is a promising direction for future development (157). In addition, the important role of miRNAs in periodontal bone regeneration has already been mentioned in the previous section, and in fact, it was found that the expression levels of dozens of miRNAs changed significantly during the differentiation of MSCs into osteoblasts, including miR-30 family, let-7 family, miR-16, miR-21, miR-125, miR-155, etc. (158), which have strong or weak positive or negative regulatory effects on osteogenesis, and studies on these miRNAs in the past decade have been incessant, but at this stage, most of the studies on miRNAs are at the initial stage of cloning and screening specific miRNAs, and there is still a long way to go in our research on the functions, mechanisms, and applications of different miRNAs in different MSCs. There is still a long way to go for research on the function, mechanism and application of different miRNAs in different MSCs.
Periodontal lesions are closely associated with oral mucosal immunity (159). Periodontitis has certain peculiarities compared to other diseases of bone immunity or bone loss. Since the oral cavity is a bacterial environment, immune dysregulation in the microenvironment of oral inflammation occurs on the basis of a disruption of the immune balance between bacteria and the mucosa, and the immune homeostasis of the oral mucosa influences the acute and chronic episodes of the disease and its severity in the early stages of inflammation or at the forefront of the disruption and disintegration of the soft tissues of the oral cavity (e.g. gingival tissues).Human commensal flora and mucosal immunity have been a hot topic of research in recent years, providing many new ideas for the treatment of a variety of mucosal-associated diseases. Regulatory T cell subpopulations exist in the mucosal epithelium and lamina propria (e.g. Th1, Th2, Th17, etc.) and their respective functions are in a state of equilibrium in mucosal immune homeostasis. When this balance is disturbed by certain factors at the right time, certain T cell populations within the mucosa become abnormal, resulting in mucosal inflammation and tissue destruction.
Interestingly, in contrast to periodontitis, where Th17 cells upregulate inflammation and promote osteoblasts, Th17 cells have been shown to regulate flora microecology and play an important role in maintaining tissue homeostasis and immune defense of the mucosal barrier. They also play a role in the prevention of infectious mucosal diseases (e.g. oral candidiasis) when triggered and induced by specific microbiomes (160, 161). The immune mechanisms at the oral mucosal barrier are less well studied, but it has been suggested that gingival Th17 cells accumulate independently of the commensal microbiota in healthy environments, and the trigger for Th17 cell accumulation in the gingiva is the sustained injury that occurs during mastication (162), and this localized tissue injury triggers the epithelial cells to produce IL-6, whereas the homeostatic Th17 cell accumulation in the oral cavity associated with mucosal health is dependent on this fraction of IL-6 production. accumulation is dependent on this IL-6. Homeostatic Th17 cells secrete IL-17 and mediate IL-17-dependent protective barrier responses such as antimicrobial defense. When periodontal inflammation spreads to the gingival tissue, the local flora associated with periodontitis acts on Th17 cells to expand in the gingiva due to the combined effect of IL-6 and IL-23 (163). At this point, the steady-state Th17 in the microenvironment is transformed into pathogenic Th17 cells, and the pathogenic Th17 leads to periodontal bone loss through immunopathological mechanisms such as excessive neutrophil recruitment and suppression of Treg cell function. This suggests to us that IL-23 in oral mucosal epithelial cells may play a pivotal role in the initiation of mucosal immune dysregulation in periodontitis.
In 2024, a study by Niki M. Moutsopoulos’ group (164) at the NIH demonstrated that epithelial cell-derived IL-23 is the initiator of inflammation in periodontitis, promotes the oral mucosal immune response to microbial components of the bacterial flora (e.g. flagellin, etc.), and serves as a mediator of inflammation in the subsequent development and regression of periodontitis. Using a mouse model of periodontitis, the researchers first demonstrated that IL-23A knockout mice were protected from periodontal bone destruction compared to wild-type mice, and then developed a mouse bone marrow transplantation (BMT) assay to determine that non-blood (epithelial) sources of IL-23 mediate the development of periodontitis. For IL-23, which is derived from hematopoietic and immune cells, existing studies appear to be more mature and MSCs can downregulate its mediated inflammatory response through multiple pathways such as expression of specific proteins and inhibition of Th17 activation. However, at this stage further studies are needed on the regulation of epithelial-derived IL-23 by MSCs with oral mucosal immunity.
In recent years, the clinical concept of peri-implant tissue regeneration in implant restorations has subtly changed, with more and more senior clinicians recognizing the importance of soft tissue management (165), which is very different from the previous concept of focusing on bone augmentation, and believing that although bone augmentation is still important, soft tissue augmentation usually achieves the best results without the need for additional bone grafting. Therefore, it would have far-reaching clinical implications to combine the immunomodulatory effects of MSCs with oral mucosal immunity and to study their role in periodontitis as well as in periodontal soft tissue regeneration. In addition, oral mucosal stem cells (OMSCs) found in the oral mucosa have been shown to have a strong potential to differentiate into epithelial cells and maintain mucosal homeostasis through immunomodulation, are relatively easy to obtain and hold great promise for research (166, 167) (Figure 3).
Figure 3. This figure shows a simple mechanism of immune homeostasis in the oral mucosa. In healthy gingiva, oral flora and mucosal immunity are in a state of equilibrium, where gingival irritation and damage during mastication causes Th17 to accumulate and triggers epithelial production of IL-6, a process that does not lead to inflammation; however, in the presence of epithelial-derived IL-23, the Th17-associated mucosal immune homeostasis is dysfunctional, leading to inflammation. The role of OMSCs located in the oral mucosa in the regulation of epithelial-derived IL-23 needs to be further investigated.
Recent studies have found that combination therapy of MSCs with small molecules of natural and pharmaceutical origin significantly enhanced the efficiency and effectiveness of MSCs in bone regeneration, where polyphenols and flavonoids small molecules have shown preliminary promotive effects on periodontal bone regeneration. For example, Samiei et al. showed that the use of curcumin and calcitriol promoted the differentiation and mineralization of DPSCs (168). And curcumin stimulation increases ALP expression of mRNA in DPSC cells; flavonoids can protect periodontal tissues from oxidative stress damage by balancing free radicals and antioxidant properties (169).
Curcumin is a polyphenol known for its powerful anti-inflammatory, antioxidant and anticancer properties. As a small molecule compound of natural origin, polyphenols have been shown to exhibit potent antimicrobial effects against Porphyromonas gingivalis and Actinobacillus polymorpha, the two main bacteria of periodontitis (170), Polyphenols have also been shown to inhibit biofilm formation, e.g., curcumin and quercetin have been shown to disrupt biofilm structure and reduce bacterial viability (171), which is crucial in the treatment of periodontal disease. Polyphenols also modulate inflammatory pathways and reduce the expression of pro-inflammatory cytokines (e.g., tumor necrosis factor-α, IL-1β, and IL-6) (172). In addition, curcumin has been incorporated into topical drug delivery systems such as gels and nanoparticles in medical-industrial crossover experiments and tissue engineering to improve its stability and targeted delivery within periodontal pockets (173).
Flavonoids have been shown to promote periodontal tissue regeneration by enhancing osteogenic differentiation and increasing collagen secretion (174). In recent years, flavonoid-containing delivery systems have been widely explored in tissue engineering, such as thermosensitive hydrogels, to improve the retention and release of flavonoids at the site of periodontal lesions, thereby enhancing their therapeutic effects. Flavonoids can modulate inflammatory pathways, such as the Rank/NF-κB pathway, thereby decreasing the expression of inflammatory cytokines, such as IL-1β and TNF-α, in order to exert anti-inflammatory effects (175).
From the above, stem cell-based therapies have a bright future in combination with small molecule drugs, and not only that, but the combination of MSCs with therapies from other disciplines has shown great potential to improve the outcome of various diseases. These combinations both utilize and enhance the regenerative potential and immunomodulatory properties of MSCs and purposefully overcome the limitations of MSCs therapies. However, Amato et al. argue that it is important to consider the multifactorial etiology of periodontal disease in these processes, including systemic factors such as diabetes and oral microbiota imbalance. Addressing these underlying issues alongside regenerative treatments will improve the overall efficacy of periodontal inflammation control (176).
Tissue-resident macrophages (tRMs) are specific immune cells that are found in specific tissues (e.g. peritoneal macrophages in the peritoneal cavity, TIM4+ self-renewing resident macrophages (SRRMs) in skeletal muscle) and are an integral part of the innate immune system (177). They play a crucial role in disease through immunosurveillance, promotion of post-injury tissue remodeling, and resistance to inflammation (178). For example, in inflammatory bowel disease (IBD), tRMs regulate immune dynamic homeostasis and epithelial regeneration. Their differentiation into pro- or anti-inflammatory phenotypes affects disease progression, highlighting their potential as therapeutic targets (179). In addition, tissue-resident macrophage population changes appear to be important during cancer progression. For example, in breast cancer, tRMs in the breast microenvironment can promote disease progression and metastasis. They interact with other immune cells and cancer cells to influence immunosuppressive or cytotoxic pathways (180).
As the understanding of macrophage phenotypes and subpopulations has increased, the crosstalk between macrophages and stem cells has been extensively studied. Similar to other types of macrophages, tRM can produce cytokines and growth factors that regulate stem cell activity and activate stem cells by modulating the inflammatory milieu, and recent studies have shown that in the mammary gland, resident macrophages regulate stem cell activity via the TNF-α-PI3K-CDK1/Cyclin B1 axis. This interaction is critical for maintaining breast stem cell activity and dynamic homeostasis5. In various tissues of the human body, different macrophage populations play a direct role in the generation of tissue-resident stem cells, including macrophages guiding stem cells into permanent niches (181) and generating transient niches to support proliferation during regeneration (182).
However, in recent years there has been a greater understanding of the role of macrophages in direct cell-cell interactions with stem cells, which may suggest a greater regulatory role within stem cell ecological niches. For example, Kim et al. demonstrated that macrophages play a direct role in stem cell ecotopia generation within the gastrointestinal tract (GIT), including direct sampling of stem cells for screening to ensure cell quality (183). Manneken JD et al. argued that organelle-organelle transfer between resident histiocytes, or MSCs, and macrophages appeared to be a mechanism that directly affects macrophage phenotypes, which is likewise a cell- cell interaction response and a mechanism used for systemic metabolic homeostasis (184).
In summary, studying the role of tRM within periodontal soft tissues in the regulation of periodontal health as well as periodontal inflammation, and elucidating the interactions between periodontal tRM and periodontal stem cells are extremely promising developments. Recent studies have shown that the generation of long-lived CD206+ tissue-resident macrophages, which colonize mesenchymal niche cells (MNCs) during early gut development, also requires appropriate microbiota colonization (183). Since the oral microenvironment is then heavily colonized by microbiota, we note that studies based on the ecological niche of periodontal stem cells may have a role in the interaction between them and tRM and between them and the oral microbiota. In these crosstalk, there are a large number of variables to be discussed, analyzed and therefore specific conditions need to be qualified, thus making these studies challenging.
The development of MSC-based cell therapy has revolutionary clinical implications compared to conventional periodontal therapy (185). MSCs therapy offers a viable new approach to eradicate the intrinsic cause of periodontal disease and promote the reconstruction of periodontal supportive tissues while alleviating the pain suffered by patients during conventional instrumental and surgical treatments. In preclinical studies, periodontal MSCs have shown a higher proliferation rate, while bone marrow MSCs have an unprecedented differentiation potential (186). In addition, PDLSCs have shown superior results in periodontal regeneration, particularly in enhancing periodontal ligament and bone regeneration compared to other MSCs (187). However, in the inflammatory environment, DFSC outperform PDLSC, suggesting their potential in specific pathological conditions (188). However, MSCs from other sources, such as adipose-derived stem cells, have also demonstrated their unique strengths in their respective directions, and therefore MSCs from different sources have their own strengths and value depending on the needs in terms of differentiation ability, regenerative outcomes, and clinical applications.
From the above sections, it is clear that MSCs and MSC-EV can play a role in various aspects of periodontal tissue regeneration through immunomodulation (including bone immunomodulation), and this has been demonstrated in both in vivo and in vitro models of periodontitis treatment. However, current studies of MSCs have not been able to quantitatively assess the impact of their immunomodulatory properties on the body and local environment. Marwa et al. discussed for the first time the extent to which cell source and cell mass can alter the cellular profile of immune cytokines and the extent to which such changes can affect immune cells (189) using bone marrow and adipose tissue derived stem cells as examples, providing a new direction for the future development of stem cell therapy. development by providing new directions.
Cell-free therapies achieved by paracrine secretion of MSC-EVs are a promising approach to periodontal regeneration, and their advantages over cell-based therapies include reduced risk of immune rejection, greater stability, and fewer ethical concerns. This makes them a viable option for a wide range of clinical applications in the field of periodontal regeneration (190). However, most studies on this treatment method are still at the preclinical research stage, awaiting further mechanistic studies and the next step of clinical trials. In 2019, Peng Hu et al. (191) with a large number of experiments, as well as the understanding of the pathological microenvironment at different stages of the disease, put forward the idea and suggestion that the isolation and extraction of MSCs-EVs need to be combined with the specific pathological microenvironment of the disease to be treated, and pointed out the scientific problems in the current “cell-free therapy” research direction through the hypothesis of MSCs-mexos. They believe that how to better combine with the microenvironmental conditions of the disease or injury site to stimulate or induce MSCs to “accurately” secrete MSCs-EVs related to the therapeutic disease and significantly enhance their secretion will be a key point to overcome for MSCs-EV to replace MSCs into clinical application. Periodontitis is precisely a disease that can go through acute and chronic phases, with multiple phases of inflammation, and is difficult to cure completely. As introduced in the previous section, there are a number of studies investigating the interaction of the periodontal inflammatory microenvironment on the properties of MSCs, and the results are not the same. Therefore, for the regeneration of periodontal tissues or the precise treatment of periodontitis, the study of the inflammatory immune microenvironment needs to open new horizons, which is of great importance for the evaluation of the cellular therapeutic value of periodontal disease and periodontal bone regeneration.
Despite the promise of MSCs and MSCs-EVs therapy to promote periodontal regeneration, it inevitably suffers from some of the common problems faced by stem cell therapy, such as lack of cell sources, low implantation and proliferation efficiency, low survival rate, and insufficient ability to homing to target tissues (192). Therefore, more research is needed to fully understand its potential and limitations. In addition, the use of stem cell therapy in periodontitis faces challenges of standardization, long-term evaluation, and ethical considerations. Patient selection criteria and treatment protocols are critical for successful implementation. The current lack of comprehensive clinical evidence and the need for responsible regulatory compliance highlight the importance of continued research and development in this field (36). Overcoming the challenges of production, scalability and therapeutic efficacy in clinical trials will overcome the limitations of current MSC and MSC-EV therapies. Spherical culture of MSCs has been shown to improve the therapeutic efficacy of the cells and their exosomes. This three-dimensional culture method improves the production rate and therapeutic properties of MSC exosomes, which is essential for effective cell therapy applications (37). In MSC-EV therapy, therapeutic efficacy can be greatly improved by tailoring to specific clinical indications and using biomaterials for efficient delivery and controlled release (38). Research into a new production method that would enable the industrialization and large-scale production of EVs is also a pressing issue at present.
D-ZZ: Investigation, Software, Supervision, Validation, Writing – original draft, Writing – review & editing. R-LY: Investigation, Supervision, Writing – review & editing. H-XW: Writing – review & editing. KY: Supervision, Writing – review & editing. Y-BY: Writing – review & editing. N-XW: Investigation, Supervision, Writing – review & editing. QZ: Supervision, Writing – review & editing. FC: Investigation, Supervision, Writing – review & editing. TZ: Investigation, Supervision, Writing – review & editing.
The author(s) declare financial support was received for the research, authorship, and/or publication of this article. This work was supported by grants from the National Natural Science Foundation of China (No.82160599); Basic research projects of Guizhou Science’s Technology Department (No.Qiankehe Basic-ZK[2024] Key 070);and Joint Fund of Guizhou Science’s Technology Department(No. [2015]7508).
The authors declare that the research was conducted in the absence of any commercial or financial relationships that could be construed as a potential conflict of interest.
All claims expressed in this article are solely those of the authors and do not necessarily represent those of their affiliated organizations, or those of the publisher, the editors and the reviewers. Any product that may be evaluated in this article, or claim that may be made by its manufacturer, is not guaranteed or endorsed by the publisher.
1. Nagata M, English JD, Ono N, Ono W. Diverse stem cells for periodontal tissue formation and regeneration. Genesis. (2022) 60:e23495. doi: 10.1002/dvg.23495
2. Larsson L, Decker AM, Nibali L, Pilipchuk SP, Berglundh T, Giannobile WV. Regenerative medicine for periodontal and peri-implant diseases. J Dent Res. (2016) 95:255–66. doi: 10.1177/0022034515618887
3. Brown C, McKee C, Bakshi S, Walker K, Hakman E, Halassy S, et al. Mesenchymal stem cells: cell therapy and regeneration potential. J Tissue Eng Regener Med. (2019) 13:1738–55. doi: 10.1002/term.2914
4. Tomokiyo A, Wada N, Maeda H. Periodontal ligament stem cells: regenerative potency in periodontium. Stem Cells Dev. (2019) 28:974–85. doi: 10.1089/scd.2019.0031
5. Mohebichamkhorami F, Fattahi R, Niknam Z, Aliashrafi M, Khakpour Naeimi S, Gilanchi S, et al. Periodontal ligament stem cells as a promising therapeutic target for neural damage. Stem Cell Res Ther. (2022) 13:273. doi: 10.1186/s13287-022-02942-9
6. Lima RL, Holanda-Afonso RC, Moura-Neto V, Bolognese AM, DosSantos MF, Souza MM. Human dental follicle cells express embryonic, mesenchymal and neural stem cells markers. Arch Oral Biol. (2017) 73:121–8. doi: 10.1016/j.archoralbio.2016.10.003
7. Yamada Y, Nakamura-Yamada S, Konoki R, Baba S. Promising advances in clinical trials of dental tissue-derived cell-based regenerative medicine. Stem Cell Res Ther. (2020) 11:175. doi: 10.1186/s13287-020-01683-x
8. Zhou T, Pan J, Wu P, Huang R, Du W, Zhou Y, et al. Dental follicle cells: roles in development and beyond. Stem Cells Int. (2019) 2019:9159605. doi: 10.1155/2019/9159605
9. Tsutsui TW. Dental pulp stem cells: advances to applications. Stem Cells Cloning. (2020) 13:33–42. doi: 10.2147/sccaa.S166759
10. Tatullo M, Marrelli M, Shakesheff KM, White LJ. Dental pulp stem cells: function, isolation and applications in regenerative medicine. J Tissue Eng Regener Med. (2015) 9:1205–16. doi: 10.1002/term.1899
11. Fawzy El-Sayed KM, Rudert A, Geiken A, Tölle J, Mekhemar M, Dörfer CE. Toll-like receptor expression profile of stem/progenitor cells from human exfoliated deciduous teeth. Int J Paediatr Dent. (2023) 33:607–14. doi: 10.1111/ipd.13080
12. Wang W, Wang A, Hu G, Bian M, Chen L, Zhao Q, et al. Potential of an aligned porous hydrogel scaffold combined with periodontal ligament stem cells or gingival mesenchymal stem cells to promote tissue regeneration in rat periodontal defects. ACS Biomater Sci Eng. (2023) 9:1961–75. doi: 10.1021/acsbiomaterials.2c01440
13. Subba TA, Varma S, Thomas B, Rao S, Kumar M, Talwar A, et al. Comparison of cellular and differentiation characteristics of mesenchymal stem cells derived from human gingiva and periodontal ligament. J Int Soc Prev Community Dent. (2022) 12:235–44. doi: 10.4103/jispcd.JISPCD_259_21
14. Dave JR, Chandekar SS, Behera S, Desai KU, Salve PM, Sapkal NB, et al. Human gingival mesenchymal stem cells retain their growth and immunomodulatory characteristics independent of donor age. Sci Adv. (2022) 8:eabm6504. doi: 10.1126/sciadv.abm6504
15. Kim D, Lee AE, Xu Q, Zhang Q, Le AD. Gingiva-derived mesenchymal stem cells: potential application in tissue engineering and regenerative medicine - a comprehensive review. Front Immunol. (2021) 12:667221. doi: 10.3389/fimmu.2021.667221
16. Soltani A, Moradi M, Nejad AR, Moradi S, Javandoost E, Nazari H, et al. Adipose-derived stem cells: potentials, availability and market size in regenerative medicine. Curr Stem Cell Res Ther. (2023) 18:347–79. doi: 10.2174/1574888x17666220413092750
17. Liu L, Guo S, Shi W, Liu Q, Huo F, Wu Y, et al. Bone marrow mesenchymal stem cell-derived small extracellular vesicles promote periodontal regeneration. Tissue Eng Part A. (2021) 27:962–76. doi: 10.1089/ten.TEA.2020.0141
18. Ghaneialvar H, Soltani L, Rahmani HR, Lotfi AS, Soleimani M. Characterization and classification of mesenchymal stem cells in several species using surface markers for cell therapy purposes. Indian J Clin Biochem. (2018) 33:46–52. doi: 10.1007/s12291-017-0641-x
19. Dominici M, Le Blanc K, Mueller I, Slaper-Cortenbach I, Marini F, Krause D, et al. Minimal criteria for defining multipotent mesenchymal stromal cells. The international society for cellular therapy position statement. Cytotherapy. (2006) 8:315–7. doi: 10.1080/14653240600855905
20. Skovronova R, Grange C, Dimuccio V, Deregibus MC, Camussi G, Bussolati B. Surface marker expression in small and medium/large mesenchymal stromal cell-derived extracellular vesicles in naive or apoptotic condition using orthogonal techniques. Cells. (2021) 10:2948. doi: 10.3390/cells10112948
21. Javazon EH, Beggs KJ, Flake AW. Mesenchymal stem cells: paradoxes of passaging. Exp Hematol. (2004) 32:414–25. doi: 10.1016/j.exphem.2004.02.004
22. Liang C, Wang G, Liang C, Li M, Sun Y, Tian W, et al. Hierarchically patterned triple-layered gelatin-based electrospun membrane functionalized by cell-specific extracellular matrix for periodontal regeneration. Dent Mater. (2024) 40:90–101. doi: 10.1016/j.dental.2023.10.022
23. Welsh JA, Goberdhan DCI, O'Driscoll L, Buzas EI, Blenkiron C, Bussolati B, et al. Minimal information for studies of extracellular vesicles (Misev2023): from basic to advanced approaches. J Extracell Vesicles. (2024) 13:e12404. doi: 10.1002/jev2.12404
24. Ouchi T, Nakagawa T. Mesenchymal stem cell-based tissue regeneration therapies for periodontitis. Regener Ther. (2020) 14:72–8. doi: 10.1016/j.reth.2019.12.011
25. Liu D, Zhao Z, Jiang W, Zhu P, An X, Xie Y, et al. Panax notoginseng saponin promotes bone regeneration in distraction osteogenesis via the tgf-B1 signaling pathway. Evid Based Complement Alternat Med. (2021) 2021:2895659. doi: 10.1155/2021/2895659
26. Li C, Bi W, Zhang D, Sang Z, Li L, Yu Y. Transforming growth factor-beta1 promotes geistlich bio-oss(®) osteogenesis via inhibiting local inflammation response in vivo. Int J Clin Exp Pathol. (2017) 10:9310–7.
27. Xu J, Liu J, Gan Y, Dai K, Zhao J, Huang M, et al. High-dose tgf-B1 impairs mesenchymal stem cell-mediated bone regeneration via bmp2 inhibition. J Bone Miner Res. (2020) 35:167–80. doi: 10.1002/jbmr.3871
28. Kim HN. Changes in Salivary Matrix Metalloproteinase-3, -8, and -9 Concentrations after 6 weeks of Non-Surgical Periodontal Therapy. BMC Oral Health. (2022) 22:175. doi: 10.1186/s12903-022-02185-3
29. Wang RP, Huang J, Chan KWY, Leung WK, Goto T, Ho YS, et al. Il-1β and tnf-A Play an important role in modulating the risk of periodontitis and alzheimer's disease. J Neuroinflamm. (2023) 20:71. doi: 10.1186/s12974-023-02747-4
30. Liu X, Li H. A systematic review and meta-analysis on multiple cytokine gene polymorphisms in the pathogenesis of periodontitis. Front Immunol. (2021) 12:713198. doi: 10.3389/fimmu.2021.713198
31. Hosokawa Y, Hosokawa I, Shindo S, Ozaki K, Matsuo T. Il-4 modulates ccl11 and ccl20 productions from il-1β-stimulated human periodontal ligament cells. Cell Physiol Biochem. (2016) 38:153–9. doi: 10.1159/000438617
32. Araujo-Pires AC, Vieira AE, Francisconi CF, Biguetti CC, Glowacki A, Yoshizawa S, et al. Il-4/ccl22/ccr4 axis controls regulatory T-cell migration that suppresses inflammatory bone loss in murine experimental periodontitis. J Bone Miner Res. (2015) 30:412–22. doi: 10.1002/jbmr.2376
33. Purwaningrum M, Giachelli CM, Osathanon T, Rattanapuchpong S, Sawangmake C. Dissecting specific wnt components governing osteogenic differentiation potential by human periodontal ligament stem cells through interleukin-6. Sci Rep. (2023) 13:9055. doi: 10.1038/s41598-023-35569-8
34. Beklen A. Effects of il-13 on tgf-B and mmp-1 in periodontitis. Biotech Histochem. (2017) 92:374–80. doi: 10.1080/10520295.2017.1312526
35. Wilharm A, Binz C, Sandrock I, Rampoldi F, Lienenklaus S, Blank E, et al. Interleukin-17 is disease promoting in early stages and protective in late stages of experimental periodontitis. PloS One. (2022) 17:e0265486. doi: 10.1371/journal.pone.0265486
36. Bharuka T, Reche A. Advancements in periodontal regeneration: A comprehensive review of stem cell therapy. Cureus. (2024) 16:e54115. doi: 10.7759/cureus.54115
37. Hazrati A, Malekpour K, Soudi S, Hashemi SM. Mesenchymal stromal/stem cells spheroid culture effect on the therapeutic efficacy of these cells and their exosomes: A new strategy to overcome cell therapy limitations. BioMed Pharmacother. (2022) 152:113211. doi: 10.1016/j.biopha.2022.113211
38. Zhu Y, Liao ZF, Mo MH, Xiong XD. Mesenchymal stromal cell-derived extracellular vesicles for vasculopathies and angiogenesis: therapeutic applications and optimization. Biomolecules. (2023) 13:1109. doi: 10.3390/biom13071109
39. Tan J, Dai A, Pan L, Zhang L, Wang Z, Ke T, et al. Inflamm-aging-related cytokines of il-17 and ifn-Γ Accelerate osteoclastogenesis and periodontal destruction. J Immunol Res. (2021) 2021:9919024. doi: 10.1155/2021/9919024
40. Gao Y, Grassi F, Ryan MR, Terauchi M, Page K, Yang X, et al. Ifn-gamma stimulates osteoclast formation and bone loss in vivo via antigen-driven T cell activation. J Clin Invest. (2007) 117:122–32. doi: 10.1172/jci30074
41. Liu L, Chen Y, Song D, Huang D. Bmp9 is a potential therapeutic agent for use in oral and maxillofacial bone tissue engineering. Biochem Soc Trans. (2020) 48:1269–85. doi: 10.1042/bst20200376
42. Mota RF, Cavalcanti de Araújo PH, Cezine MER, Matsuo FS, Metzner RJM, Oliveira de Biagi Junior CA, et al. Rankl impairs the tlr4 pathway by increasing traf6 and rank interaction in macrophages. BioMed Res Int. (2022) 2022:7740079. doi: 10.1155/2022/7740079
43. Hooshiar SH, Tobeiha M, Jafarnejad S. Soy isoflavones and bone health: focus on the rankl/rank/opg pathway. BioMed Res Int. (2022) 2022:8862278. doi: 10.1155/2022/8862278
44. Kapasa ER, Giannoudis PV, Jia X, Hatton PV, Yang XB. The effect of rankl/opg balance on reducing implant complications. J Funct Biomater. (2017) 8:42. doi: 10.3390/jfb8040042
45. Nakao Y, Fukuda T, Zhang Q, Sanui T, Shinjo T, Kou X, et al. Exosomes from tnf-A-treated human gingiva-derived mscs enhance M2 macrophage polarization and inhibit periodontal bone loss. Acta Biomater. (2021) 122:306–24. doi: 10.1016/j.actbio.2020.12.046
46. Zheng X, Wang S, Xiao L, Han P, Xie K, Ivanovski S, et al. Licl-induced immunomodulatory periodontal regeneration via the activation of the wnt/B-catenin signaling pathway. J Periodontal Res. (2022) 57:835–48. doi: 10.1111/jre.13022
47. Müller L, Tunger A, Wobus M, von Bonin M, Towers R, Bornhäuser M, et al. Immunomodulatory properties of mesenchymal stromal cells: an update. Front Cell Dev Biol. (2021) 9:637725. doi: 10.3389/fcell.2021.637725
48. Zhao X, Jiao J, Li X, Hou R, Li J, Niu X, et al. Immunomodulatory effect of psoriasis-derived dermal mesenchymal stem cells on th1/th17 cells. Eur J Dermatol. (2021) 31:318–25. doi: 10.1684/ejd.2021.4050
49. Akiyama K, Chen C, Wang D, Xu X, Qu C, Yamaza T, et al. Mesenchymal-stem-cell-induced immunoregulation involves fas-ligand-/fas-mediated T cell apoptosis. Cell Stem Cell. (2012) 10:544–55. doi: 10.1016/j.stem.2012.03.007
50. Pedrosa M, Gomes J, Laranjeira P, Duarte C, Pedreiro S, Antunes B, et al. Immunomodulatory effect of human bone marrow-derived mesenchymal stromal/stem cells on peripheral blood T cells from rheumatoid arthritis patients. J Tissue Eng Regener Med. (2020) 14:16–28. doi: 10.1002/term.2958
51. Terraza-Aguirre C, Campos-Mora M, Elizondo-Vega R, Contreras-López RA, Luz-Crawford P, Jorgensen C, et al. Mechanisms behind the immunoregulatory dialogue between mesenchymal stem cells and th17 cells. Cells. (2020) 9:1660. doi: 10.3390/cells9071660
52. Zhang J, Ma X, Cao L, He X, Li S, Yang M, et al. Enhancing and stabilization of cord blood regulatory T-cell suppressive function by human mesenchymal stem cell (Msc)-derived exosomes. Clin Exp Immunol. (2022) 208:255–67. doi: 10.1093/cei/uxac035
53. Luo Y, Guo J, Zhang P, Cheuk YC, Jiang Y, Wang J, et al. Mesenchymal stem cell protects injured renal tubular epithelial cells by regulating mtor-mediated th17/treg axis. Front Immunol. (2021) 12:684197. doi: 10.3389/fimmu.2021.684197
54. Salek Farrokhi A, Zarnani AH, Moazzeni SM. Mesenchymal stem cells therapy protects fetuses from resorption and induces th2 type cytokines profile in abortion prone mouse model. Transpl Immunol. (2018) 47:26–31. doi: 10.1016/j.trim.2018.01.002
55. Reis M, Mavin E, Nicholson L, Green K, Dickinson AM, Wang XN. Mesenchymal stromal cell-derived extracellular vesicles attenuate dendritic cell maturation and function. Front Immunol. (2018) 9:2538. doi: 10.3389/fimmu.2018.02538
56. Ashfaq H, Soliman H, Saleh M, El-Matbouli M. Cd4: A vital player in the teleost fish immune system. Vet Res. (2019) 50:1. doi: 10.1186/s13567-018-0620-0
57. Han YK, Jin Y, Miao YB, Shi T, Lin XP. Cd8(+) foxp3(+) T cells affect alveolar bone homeostasis via modulating tregs/th17 during induced periodontitis: an adoptive transfer experiment. Inflammation. (2018) 41:1791–803. doi: 10.1007/s10753-018-0822-7
58. Cole KL, Seymour GJ, Powell RN. Phenotypic and functional analysis of T cells extracted from chronically inflamed human periodontal tissues. J Periodontol. (1987) 58:569–73. doi: 10.1902/jop.1987.58.8.569
59. Behm C, Blufstein A, Gahn J, Nemec M, Moritz A, Rausch-Fan X, et al. Cytokines differently define the immunomodulation of mesenchymal stem cells from the periodontal ligament. Cells. (2020) 9:1222. doi: 10.3390/cells9051222
60. Handono K, Putra Gofur NR, Nurdiana N, Kalim H, Wahono CS, Poeranto S, et al. Role of lymphocytes cd4/cd8 ratio and immunoglobulin G cytomegalovirus as potential markers for systemic lupus erythematosus patients with periodontal disease. Eur J Dent. (2020) 14:544–50. doi: 10.1055/s-0040-1715788
61. Sommer MEL, Dalia RA, Nogueira AVB, Cirelli JA, Vinolo MAR, Fachi JL, et al. Immune response mediated by th1 / il-17 / caspase-9 promotes evolution of periodontal disease. Arch Oral Biol. (2019) 97:77–84. doi: 10.1016/j.archoralbio.2018.09.009
62. Slots J. Focal infection of periodontal origin. Periodontol 2000. (2019) 79:233–5. doi: 10.1111/prd.12258
63. Souto GR, Queiroz-Junior CM, de Abreu MH, Costa FO, Mesquita RA. Pro-inflammatory, th1, th2, th17 cytokines and dendritic cells: A cross-sectional study in chronic periodontitis. PloS One. (2014) 9:e91636. doi: 10.1371/journal.pone.0091636
64. Dituri F, Mancarella S, Serino G, Chaoul N, Lupo LG, Villa E, et al. Direct and indirect effect of tgfβ on treg transendothelial recruitment in hcc tissue microenvironment. Int J Mol Sci. (2021) 22:11765. doi: 10.3390/ijms222111765
65. Balaji S, Cholan PK, Victor DJ. An emphasis of T-cell subsets as regulators of periodontal health and disease. J Clin Transl Res. (2021) 7:648–56.
66. Xia Y, Cheng T, Zhang C, Zhou M, Hu Z, Kang F, et al. Human bone marrow mesenchymal stem cell-derived extracellular vesicles restore th17/treg homeostasis in periodontitis via mir-1246. FASEB J. (2023) 37:e23226. doi: 10.1096/fj.202300674RR
67. Gao L, Zhao Y, Wang P, Zhang L, Zhang C, Chen Q, et al. Detection of th17/treg cells and related factors in gingival tissues and peripheral blood of rats with experimental periodontitis. Iran J Basic Med Sci. (2017) 20:294–300. doi: 10.22038/ijbms.2017.8359
68. Dutzan N, Abusleme L. T helper 17 cells as pathogenic drivers of periodontitis. Adv Exp Med Biol. (2019) 1197:107–17. doi: 10.1007/978-3-030-28524-1_9
69. Liu J, Liu Q, Chen X. The immunomodulatory effects of mesenchymal stem cells on regulatory B cells. Front Immunol. (2020) 11:1843. doi: 10.3389/fimmu.2020.01843
70. Greene AC, Shehabeldin M, Gao J, Balmert SC, Ratay M, Sfeir C, et al. Local induction of regulatory T cells prevents inflammatory bone loss in ligature-induced experimental periodontitis in mice. Sci Rep. (2022) 12:5032. doi: 10.1038/s41598-022-09150-8
71. Kou Y, Jiang Y, Liu S, Yang P, Lu Y, Liu H, et al. Regulatory T cells showed characteristics of T helper-17(Th17) cells in mice periodontitis model. Oral Dis. (2023) 29:1149–62. doi: 10.1111/odi.14072
72. Leng S, Xu W, Wu L, Liu L, Du J, Yang F, et al. Nlrp3 disturbs treg/th17 cell balance to aggravate apical periodontitis. J Dent Res. (2023) 102:656–66. doi: 10.1177/00220345231151692
73. Zheng Y, Dong C, Yang J, Jin Y, Zheng W, Zhou Q, et al. Exosomal microrna-155-5p from pdlscs regulated th17/treg balance by targeting sirtuin-1 in chronic periodontitis. J Cell Physiol. (2019) 234:20662–74. doi: 10.1002/jcp.28671
74. Veh J, Ludwig C, Schrezenmeier H, Jahrsdörfer B. Regulatory B cells-immunopathological and prognostic potential in humans. Cells. (2024) 13:357. doi: 10.3390/cells13040357
75. Zhang M, Zheng X, Zhang J, Zhu Y, Zhu X, Liu H, et al. Cd19(+)Cd1d(+)Cd5(+) B cell frequencies are increased in patients with tuberculosis and suppress th17 responses. Cell Immunol. (2012) 274:89–97. doi: 10.1016/j.cellimm.2012.01.007
76. Cao G, Zhang X, Song Y, Sun Y, Ling H, Han X, et al. Local promotion of B10 function alleviates experimental periodontitis bone loss through antagonizing rankl-expressing neutrophils. J Periodontol. (2021) 92:907–20. doi: 10.1002/jper.20-0074
77. Liu O, Xu J, Ding G, Liu D, Fan Z, Zhang C, et al. Periodontal ligament stem cells regulate B lymphocyte function via programmed cell death protein 1. Stem Cells. (2013) 31:1371–82. doi: 10.1002/stem.1387
78. Vergadi E, Ieronymaki E, Lyroni K, Vaporidi K, Tsatsanis C. Akt signaling pathway in macrophage activation and M1/M2 polarization. J Immunol. (2017) 198:1006–14. doi: 10.4049/jimmunol.1601515
79. Bailly C. The implication of the pd-1/pd-L1 checkpoint in chronic periodontitis suggests novel therapeutic opportunities with natural products. Jpn Dent Sci Rev. (2020) 56:90–6. doi: 10.1016/j.jdsr.2020.04.002
80. Mahanonda R, Champaiboon C, Subbalekha K, Sa-Ard-Iam N, Rattanathammatada W, Thawanaphong S, et al. Human memory B cells in healthy gingiva, gingivitis, and periodontitis. J Immunol. (2016) 197:715–25. doi: 10.4049/jimmunol.1600540
81. Feng W, Liu H, Luo T, Liu D, Du J, Sun J, et al. Combination of il-6 and sil-6r differentially regulate varying levels of rankl-induced osteoclastogenesis through nf-Kb, erk and jnk signaling pathways. Sci Rep. (2017) 7:41411. doi: 10.1038/srep41411
82. Karlinsey K, Qu L, Matz AJ, Zhou B. A novel strategy to dissect multifaceted macrophage function in human diseases. J Leukoc Biol. (2022) 112:1535–42. doi: 10.1002/jlb.6mr0522-685r
83. Zhang B, Yang Y, Yi J, Zhao Z, Ye R. Hyperglycemia modulates M1/M2 macrophage polarization via reactive oxygen species overproduction in ligature-induced periodontitis. J Periodontal Res. (2021) 56:991–1005. doi: 10.1111/jre.12912
84. Cui D, Lyu J, Li H, Lei L, Bian T, Li L, et al. Human B-defensin 3 inhibits periodontitis development by suppressing inflammatory responses in macrophages. Mol Immunol. (2017) 91:65–74. doi: 10.1016/j.molimm.2017.08.012
85. Liu J, Chen B, Bao J, Zhang Y, Lei L, Yan F. Macrophage polarization in periodontal ligament stem cells enhanced periodontal regeneration. Stem Cell Res Ther. (2019) 10:320. doi: 10.1186/s13287-019-1409-4
86. Gao X, Shen Z, Guan M, Huang Q, Chen L, Qin W, et al. Immunomodulatory role of stem cells from human exfoliated deciduous teeth on periodontal regeneration. Tissue Eng Part A. (2018) 24:1341–53. doi: 10.1089/ten.TEA.2018.0016
87. Pang QM, Yang R, Zhang M, Zou WH, Qian NN, Xu QJ, et al. Peripheral blood-derived mesenchymal stem cells modulate macrophage plasticity through the il-10/stat3 pathway. Stem Cells Int. (2022) 2022:5181241. doi: 10.1155/2022/5181241
88. Liu J, Wang H, Zhang L, Li X, Ding X, Ding G, et al. Periodontal ligament stem cells promote polarization of M2 macrophages. J Leukoc Biol. (2022) 111:1185–97. doi: 10.1002/jlb.1ma1220-853rr
89. Zhang FF, Hao Y, Zhang KX, Yang JJ, Zhao ZQ, Liu HJ, et al. Interplay between mesenchymal stem cells and macrophages: promoting bone tissue repair. World J Stem Cells. (2024) 16:375–88. doi: 10.4252/wjsc.v16.i4.375
90. Zhu Y, Zhang X, Yang K, Shao Y, Gu R, Liu X, et al. Macrophage-derived apoptotic vesicles regulate fate commitment of mesenchymal stem cells via mir155. Stem Cell Res Ther. (2022) 13:323. doi: 10.1186/s13287-022-03004-w
91. Jiang L, Li X, Zhang Y, Zhang M, Tang Z, Lv K. Microarray and bioinformatics analyses of gene expression profiles in balb/C murine macrophage polarization. Mol Med Rep. (2017) 16:7382–90. doi: 10.3892/mmr.2017.7511
92. Rakina M, Larionova I, Kzhyshkowska J. Macrophage diversity in human cancers: new insight provided by single-cell resolution and spatial context. Heliyon. (2024) 10:e28332. doi: 10.1016/j.heliyon.2024.e28332
93. Lim Y, Kim HY, An SJ, Choi BK. Activation of bone marrow-derived dendritic cells and cd4(+) T cell differentiation by outer membrane vesicles of periodontal pathogens. J Oral Microbiol. (2022) 14:2123550. doi: 10.1080/20002297.2022.2123550
94. El-Awady AR, Elashiry M, Morandini AC, Meghil MM, Cutler CW. Dendritic cells a critical link to alveolar bone loss and systemic disease risk in periodontitis: immunotherapeutic implications. Periodontol 2000. (2022) 89:41–50. doi: 10.1111/prd.12428
95. Racz GZ, Kadar K, Foldes A, Kallo K, Perczel-Kovach K, Keremi B, et al. Immunomodulatory and potential therapeutic role of mesenchymal stem cells in periodontitis. J Physiol Pharmacol. (2014) 65:327–39.
96. Shahir M, Mahmoud Hashemi S, Asadirad A, Varahram M, Kazempour-Dizaji M, Folkerts G, et al. Effect of mesenchymal stem cell-derived exosomes on the induction of mouse tolerogenic dendritic cells. J Cell Physiol. (2020) 235:7043–55. doi: 10.1002/jcp.29601
97. Seidel A, Seidel CL, Weider M, Junker R, Gölz L, Schmetzer H. Influence of natural killer cells and natural killer T cells on periodontal disease: A systematic review of the current literature. Int J Mol Sci. (2020) 21:9766. doi: 10.3390/ijms21249766
98. Lin W, Li Q, Liu L, Wang Q, Zhang D, Wang F, et al. Early infiltrating nkt lymphocytes attenuate bone regeneration through secretion of cxcl2. Sci Adv. (2024) 10:eadl6343. doi: 10.1126/sciadv.adl6343
99. Joel MDM, Yuan J, Wang J, Yan Y, Qian H, Zhang X, et al. Msc: immunoregulatory effects, roles on neutrophils and evolving clinical potentials. Am J Transl Res. (2019) 11:3890–904.
100. Iliopoulos JM, Layrolle P, Apatzidou DA. Microbial-stem cell interactions in periodontal disease. J Med Microbiol. (2022) 71. doi: 10.1099/jmm.0.001503
101. Zhang F, Si M, Wang H, Mekhemar MK, Dörfer CE, Fawzy El-Sayed KM. Il-1/tnf-A Inflammatory and anti-inflammatory synchronization affects gingival stem/progenitor cells' Regenerative attributes. Stem Cells Int. (2017) 2017:1349481. doi: 10.1155/2017/1349481
102. Zhou L, Dörfer CE, Chen L, Fawzy El-Sayed KM. Porphyromonas Gingivalis Lipopolysaccharides Affect Gingival Stem/Progenitor Cells Attributes through Nf-Kb, but Not Wnt/B-Catenin, Pathway. J Clin Periodontol. (2017) 44:1112–22. doi: 10.1111/jcpe.12777
103. Hou. Y, Zeng. D, Zhang. X, Yang. L, Dang. J, Wu. W, et al. Gingival mesenchymal stem cells derived from patients with rheumatoid arthritis treats experimental arthritis. (2023). doi: 10.2139/ssrn.4604836
104. Xia Y, Zhu J, Yang R, Wang H, Li Y, Fu C. Mesenchymal stem cells in the treatment of spinal cord injury: mechanisms, current advances and future challenges. Front Immunol. (2023) 14:1141601. doi: 10.3389/fimmu.2023.1141601
105. Aguiar Koga BA, Fernandes LA, Fratini P, Sogayar MC, Carreira ACO. Role of msc-derived small extracellular vesicles in tissue repair and regeneration. Front Cell Dev Biol. (2022) 10:1047094. doi: 10.3389/fcell.2022.1047094
106. Liu M, Chen R, Xu Y, Zheng J, Wang M, Wang P. Exosomal mir-141-3p from pdlscs alleviates high glucose-induced senescence of pdlscs by activating the keap1-nrf2 signaling pathway. Stem Cells Int. (2023) 2023:7136819. doi: 10.1155/2023/7136819
107. Chiricosta L, Silvestro S, Gugliandolo A, Marconi GD, Pizzicannella J, Bramanti P, et al. Extracellular vesicles of human periodontal ligament stem cells contain micrornas associated to proto-oncogenes: implications in cytokinesis. Front Genet. (2020) 11:582. doi: 10.3389/fgene.2020.00582
108. Wang Y, Zhang X, Wang J, Zhang Y, Ye Q, Wang Y, et al. Inflammatory periodontal ligament stem cells drive M1 macrophage polarization via exosomal mir-143-3p-mediated regulation of pi3k/akt/nf-Kb signaling. Stem Cells. (2023) 41:184–99. doi: 10.1093/stmcls/sxac087
109. Zhao R, Wang L, Wang T, Xian P, Wang H, Long Q. Inhalation of msc-evs is a noninvasive strategy for ameliorating acute lung injury. J Control Release. (2022) 345:214–30. doi: 10.1016/j.jconrel.2022.03.025
110. Zhang Z, Shuai Y, Zhou F, Yin J, Hu J, Guo S, et al. Erratum: pdlscs regulate angiogenesis of periodontal ligaments via vegf transferred by exosomes in periodontitis: erratum. Int J Med Sci. (2022) 19:833. doi: 10.7150/ijms.74583
111. Sun D, Mou S, Chen L, Yang J, Wang R, Zhong A, et al. High yield engineered nanovesicles from adsc with enriched mir-21-5p promote angiogenesis in adipose tissue regeneration. Biomater Res. (2022) 26:83. doi: 10.1186/s40824-022-00325-y
112. Lai TC, Lee TL, Chang YC, Chen YC, Lin SR, Lin SW, et al. Microrna-221/222 mediates adsc-exosome-induced cardioprotection against ischemia/reperfusion by targeting puma and ets-1. Front Cell Dev Biol. (2020) 8:569150. doi: 10.3389/fcell.2020.569150
113. Symonds EKC, Black B, Brown A, Meredith I, Currie MJ, Hally KE, et al. Adipose derived stem cell extracellular vesicles modulate primary human macrophages to an anti-inflammatory phenotype in vitro. J Extracell Biol. (2023) 2:e104. doi: 10.1002/jex2.104
114. Chen J, Shi X, Deng Y, Dang J, Liu Y, Zhao J, et al. Mirna-148a-containing gmsc-derived evs modulate treg/th17 balance via ikkb/nf-Kb pathway and treat a rheumatoid arthritis model. JCI Insight. (2024) 9:e177841. doi: 10.1172/jci.insight.177841
115. Silvestro S, Chiricosta L, Gugliandolo A, Pizzicannella J, Diomede F, Bramanti P, et al. Extracellular vesicles derived from human gingival mesenchymal stem cells: A transcriptomic analysis. Genes (Basel). (2020) 11:118. doi: 10.3390/genes11020118
116. Srivastava J, Kundal K, Rai B, Saxena P, Katiyar S, Tripathy N, et al. Global microrna profiling of bone marrow-msc derived extracellular vesicles identifies mirnas associated with hematopoietic dysfunction in aplastic anemia. Sci Rep. (2024) 14:19654. doi: 10.1038/s41598-024-70369-8
117. Li Y, Wang J, Ma Y, Du W, Feng H, Feng K, et al. Retraction note: microrna-15b shuttled by bone marrow mesenchymal stem cell-derived extracellular vesicles binds to wwp1 and promotes osteogenic differentiation. Arthritis Res Ther. (2022) 24:250. doi: 10.1186/s13075-022-02950-3
118. Jin J, Ou Q, Wang Z, Tian H, Xu JY, Gao F, et al. Bmsc-derived extracellular vesicles intervened the pathogenic changes of scleroderma in mice through mirnas. Stem Cell Res Ther. (2021) 12:327. doi: 10.1186/s13287-021-02400-y
119. Li Y, Sun M, Wang X, Cao X, Li N, Pei D, et al. Dental stem cell-derived extracellular vesicles transfer mir-330-5p to treat traumatic brain injury by regulating microglia polarization. Int J Oral Sci. (2022) 14:44. doi: 10.1038/s41368-022-00191-3
120. Wang X. Human umbilical cord mesenchymal stem cells and their extracellular vesicles modulate pro- and anti-inflammatory cytokines in ligature-induced periodontitis. Iran J Immunol. (2023) 20:446–55. doi: 10.22034/iji.2023.100211.2683
121. Kang M, Huang CC, Gajendrareddy P, Lu Y, Shirazi S, Ravindran S, et al. Extracellular vesicles from tnfα Preconditioned mscs: effects on immunomodulation and bone regeneration. Front Immunol. (2022) 13:878194. doi: 10.3389/fimmu.2022.878194
122. Sánchez N, Fierravanti L, Núñez J, Vignoletti F, González-Zamora M, Santamaría S, et al. Periodontal regeneration using a xenogeneic bone substitute seeded with autologous periodontal ligament-derived mesenchymal stem cells: A 12-month quasi-randomized controlled pilot clinical trial. J Clin Periodontol. (2020) 47:1391–402. doi: 10.1111/jcpe.13368
123. Song WP, Jin LY, Zhu MD, Wang H, Xia DS. Clinical trials using dental stem cells: 2022 update. World J Stem Cells. (2023) 15:31–51. doi: 10.4252/wjsc.v15.i3.31
124. Kodama J, Wilkinson KJ, Otsuru S. Msc-ev therapy for bone/cartilage diseases. Bone Rep. (2022) 17:101636. doi: 10.1016/j.bonr.2022.101636
125. Zhong W, Liu Z, Ma L, Wan X, Chen S, Ma K, et al. Hydrogels loaded with MSC-derived small extracellular vesicles: A novel cell-free tissue engineering system for diabetic wound management. View. (2024). doi: 10.1002/viw.20230110
126. Wang R, Ji Q, Meng C, Liu H, Fan C, Lipkind S, et al. Role of gingival mesenchymal stem cell exosomes in macrophage polarization under inflammatory conditions. Int Immunopharmacol. (2020) 81:106030. doi: 10.1016/j.intimp.2019.106030
127. Cassano JM, Schnabel LV, Goodale MB, Fortier LA. Inflammatory licensed equine mscs are chondroprotective and exhibit enhanced immunomodulation in an inflammatory environment. Stem Cell Res Ther. (2018) 9:82. doi: 10.1186/s13287-018-0840-2
128. Weiss ARR, Dahlke MH. Immunomodulation by mesenchymal stem cells (Mscs): mechanisms of action of living, apoptotic, and dead mscs. Front Immunol. (2019) 10:1191. doi: 10.3389/fimmu.2019.01191
129. Wedzinska A, Figiel-Dabrowska A, Kozlowska H, Sarnowska A. The effect of proinflammatory cytokines on the proliferation, migration and secretory activity of mesenchymal stem/stromal cells (Wj-mscs) under 5% O(2) and 21% O(2) culture conditions. J Clin Med. (2021) 10:1813. doi: 10.3390/jcm10091813
130. Suzdaltseva Y, Goryunov K, Silina E, Manturova N, Stupin V, Kiselev SL. Equilibrium among inflammatory factors determines human msc-mediated immunosuppressive effect. Cells. (2022) 11:1210. doi: 10.3390/cells11071210
131. Wei X, Guo S, Liu Q, Liu L, Huo F, Wu Y, et al. Dental follicle stem cells promote periodontal regeneration through periostin-mediated macrophage infiltration and reprogramming in an inflammatory microenvironment. Int J Mol Sci. (2023) 24:6353. doi: 10.3390/ijms24076353
132. Xu W, Zhou W, Wang H, Liang S. Roles of porphyromonas gingivalis and its virulence factors in periodontitis. Adv Protein Chem Struct Biol. (2020) 120:45–84. doi: 10.1016/bs.apcsb.2019.12.001
133. Zhang Z, Deng M, Hao M, Tang J. Periodontal ligament stem cells in the periodontitis niche: inseparable interactions and mechanisms. J Leukoc Biol. (2021) 110:565–76. doi: 10.1002/jlb.4mr0421-750r
134. Watanabe Y, Fukuda T, Hayashi C, Nakao Y, Toyoda M, Kawakami K, et al. Extracellular vesicles derived from gmscs stimulated with tnf-A and ifn-A Promote M2 macrophage polarization via enhanced cd73 and cd5l expression. Sci Rep. (2022) 12:13344. doi: 10.1038/s41598-022-17692-0
135. Liu Y, Wang L, Kikuiri T, Akiyama K, Chen C, Xu X, et al. Mesenchymal stem cell-based tissue regeneration is governed by recipient T lymphocytes via ifn-Γ and tnf-A. Nat Med. (2011) 17:1594–601. doi: 10.1038/nm.2542
136. Xie L, Ren X, Yang Z, Zhou T, Zhang M, An W, et al. Exosomal circ_0000722 derived from periodontal ligament stem cells undergoing osteogenic differentiation promotes osteoclastogenesis. Int Immunopharmacol. (2024) 128:111520. doi: 10.1016/j.intimp.2024.111520
137. Qiao X, Tang J, Dou L, Yang S, Sun Y, Mao H, et al. Dental pulp stem cell-derived exosomes regulate anti-inflammatory and osteogenesis in periodontal ligament stem cells and promote the repair of experimental periodontitis in rats. Int J Nanomedicine. (2023) 18:4683–703. doi: 10.2147/ijn.S420967
138. Sun J, Wang Z, Liu P, Hu Y, Li T, Yang J, et al. Exosomes derived from human gingival mesenchymal stem cells attenuate the inflammatory response in periodontal ligament stem cells. Front Chem. (2022) 10:863364. doi: 10.3389/fchem.2022.863364
139. Liu W, Yu M, Chen F, Wang L, Ye C, Chen Q, et al. A novel delivery nanobiotechnology: engineered mir-181b exosomes improved osteointegration by regulating macrophage polarization. J Nanobiotechnology. (2021) 19:269. doi: 10.1186/s12951-021-01015-y
140. Wang M, Li J, Ye Y, Chen D, Song J. Shed-derived exosomes improve the repair capacity and osteogenesis potential of hpdlcs. Oral Dis. (2023) 29:1692–705. doi: 10.1111/odi.14153
141. Wang S, Qin L. Homeostatic medicine: A strategy for exploring health and disease. Curr Med (Cham). (2022) 1:16. doi: 10.1007/s44194-022-00016-9
142. Settem RP, Honma K, Chinthamani S, Kawai T, Sharma A. B-cell rankl contributes to pathogen-induced alveolar bone loss in an experimental periodontitis mouse model. Front Physiol. (2021) 12:722859. doi: 10.3389/fphys.2021.722859
143. Wang B, Dong Y, Tian Z, Chen Y, Dong S. The role of dendritic cells derived osteoclasts in bone destruction diseases. Genes Dis. (2021) 8:401–11. doi: 10.1016/j.gendis.2020.03.009
144. Söderström K, Stein E, Colmenero P, Purath U, Müller-Ladner U, de Matos CT, et al. Natural killer cells trigger osteoclastogenesis and bone destruction in arthritis. Proc Natl Acad Sci U.S.A. (2010) 107:13028–33. doi: 10.1073/pnas.1000546107
145. Xiao L, Zhou Y, Zhu L, Yang S, Huang R, Shi W, et al. Sphk1-S1pr1-rankl axis regulates the interactions between macrophages and bmscs in inflammatory bone loss. J Bone Miner Res. (2018) 33:1090–104. doi: 10.1002/jbmr.3396
146. Nasiri K, Jahri M, Kolahdouz S, Soleimani M, Makiya A, Saini RS, et al. Micrornas function in dental stem cells as a promising biomarker and therapeutic target for dental diseases. Mol Diagn Ther. (2023) 27:703–22. doi: 10.1007/s40291-023-00675-w
147. Wang L, Wei X, He X, Xiao S, Shi Q, Chen P, et al. Osteoinductive dental pulp stem cell-derived extracellular vesicle-loaded multifunctional hydrogel for bone regeneration. ACS Nano. (2024) 18:8777–97. doi: 10.1021/acsnano.3c11542
148. Li H, Jiang T, Li MQ, Zheng XL, Zhao GJ. Transcriptional regulation of macrophages polarization by micrornas. Front Immunol. (2018) 9:1175. doi: 10.3389/fimmu.2018.01175
149. Karimi Z, Seyedjafari E, Khojasteh A, Hashemi SM, Kazemi B, Mohammadi-Yeganeh S. Microrna-218 competes with differentiation media in the induction of osteogenic differentiation of mesenchymal stem cell by regulating B-catenin inhibitors. Mol Biol Rep. (2020) 47:8451–63. doi: 10.1007/s11033-020-05885-7
150. Sun F, Ma Y, Cai Z, Yang Z. Mir-218 promotes osteogenic differentiation of periodontal ligament stem cell through activation of wnt signaling by targeting sfrp2. Int J OF Clin AND Exp Pathol. (2016) 9:10188–96.
151. Wu P, Feng J, Wang W. Expression of mir-155 and mir-146a in the saliva of patients with periodontitis and its clinical value. Am J Transl Res. (2021) 13:6670–7.
152. Öngöz Dede F, Gökmenoğlu C, Türkmen E, Bozkurt Doğan Ş, Ayhan BS, Yildirim K. Six mirna expressions in the saliva of smokers and non-smokers with periodontal disease. J Periodontal Res. (2023) 58:195–203. doi: 10.1111/jre.13081
153. Yan C, Li N, Xiao T, Ye X, Fu L, Ye Y, et al. Extracellular vesicles from the inflammatory microenvironment regulate the osteogenic and odontogenic differentiation of periodontal ligament stem cells by mir-758-5p/lmbr1/bmp2/4 axis. J Transl Med. (2022) 20:208. doi: 10.1186/s12967-022-03412-9
154. Dos Santos CC, Lopes-Pacheco M, English K, Rolandsson Enes S, Krasnodembskaya A, Rocco PRM. The msc-ev-micrornaome: A perspective on therapeutic mechanisms of action in sepsis and ards. Cells. (2024) 13:122. doi: 10.3390/cells13020122
155. Lagosz KB, Bysiek A, Macina JM, Bereta GP, Kantorowicz M, Lipska W, et al. Hdac3 regulates gingival fibroblast inflammatory responses in periodontitis. J Dent Res. (2020) 99:98–106. doi: 10.1177/0022034519885088
156. Cao J, Zhang Q, Yang Q, Yu Y, Meng M, Zou J. Epigenetic regulation of osteogenic differentiation of periodontal ligament stem cells in periodontitis. Oral Dis. (2023) 29:2529–37. doi: 10.1111/odi.14491
157. Wang Z, Wen S, Zhong M, Yang Z, Xiong W, Zhang K, et al. Epigenetics: novel crucial approach for osteogenesis of mesenchymal stem cells. J Tissue Eng. (2023) 14:20417314231175364. doi: 10.1177/20417314231175364
158. Mazziotta C, Lanzillotti C, Iaquinta MR, Taraballi F, Torreggiani E, Rotondo JC, et al. Micrornas modulate signaling pathways in osteogenic differentiation of mesenchymal stem cells. Int J Mol Sci. (2021) 22:2362. doi: 10.3390/ijms22052362
159. Williams DW, Greenwell-Wild T, Brenchley L, Dutzan N, Overmiller A, Sawaya AP, et al. Human oral mucosa cell atlas reveals a stromal-neutrophil axis regulating tissue immunity. Cell. (2021) 184:4090–104.e15. doi: 10.1016/j.cell.2021.05.013
160. Aggor FEY, Zhou C, Abbott D, Musgrove J, Bruno V, Hand TW, et al. Th17-driven immunity to oral candidiasis is dependent on the microbiome and can be triggered by mono-colonization with segmented filamentous bacteria. J OF Immunol. (2022) 208. doi: 10.4049/jimmunol.208.Supp.115.18
161. Wang Y, Yin Y, Chen X, Zhao Y, Wu Y, Li Y, et al. Induction of intestinal th17 cells by flagellins from segmented filamentous bacteria. Front Immunol. (2019) 10:2750. doi: 10.3389/fimmu.2019.02750
162. Gaffen SL, Moutsopoulos NM. Regulation of host-microbe interactions at oral mucosal barriers by type 17 immunity. Sci Immunol. (2020) 5:eaau4594. doi: 10.1126/sciimmunol.aau4594
163. Dutzan N, Kajikawa T, Abusleme L, Greenwell-Wild T, Zuazo CE, Ikeuchi T, et al. A dysbiotic microbiome triggers T(H)17 cells to mediate oral mucosal immunopathology in mice and humans. Sci Transl Med. (2018) 10:eaat0797. doi: 10.1126/scitranslmed.aat0797
164. Kim TS, Ikeuchi T, Theofilou VI, Williams DW, Greenwell-Wild T, June A, et al. Epithelial-derived interleukin-23 promotes oral mucosal immunopathology. Immunity. (2024) 57:859–75.e11. doi: 10.1016/j.immuni.2024.02.020
165. Roccuzzo M, Roccuzzo A, Marruganti C, Fickl S. The importance of soft tissue condition in bone regenerative procedures to ensure long-term peri-implant health. Periodontol 2000. (2023) 93:129–38. doi: 10.1111/prd.12496
166. López S, Hoz L, Tenorio EP, Buentello B, Magaña FS, Wintergerst A, et al. Can human oral mucosa stem cells differentiate to corneal epithelia? Int J Mol Sci. (2021) 22:5976. doi: 10.3390/ijms22115976
167. Radavi-Asgar M, Sabet N, Khaksari M, Jafari E, Soltani Z, Dehghanian F. The prescription of oral mucosal mesenchymal stem cells post-traumatic brain injury improved the kidney and heart inflammation and oxidative stress. BioMed Res Int. (2022) 2022:8235961. doi: 10.1155/2022/8235961
168. Samiei M, Abedi A, Sharifi S, Maleki Dizaj S. Early osteogenic differentiation stimulation of dental pulp stem cells by calcitriol and curcumin. Stem Cells Int. (2021) 2021:9980137. doi: 10.1155/2021/9980137
169. Carvalho JS, Ramadan D, de Paiva Gonçalves V, Maquera-Huacho PM, Assis RP, Lima TFO, et al. Impact of citrus flavonoid supplementation on inflammation in lipopolysaccharide-induced periodontal disease in mice. Food Funct. (2021) 12:5007–17. doi: 10.1039/d0fo03338c
170. Song P, Hao Y, Lin D, Jin Y, Lin J. Evaluation of the antibacterial effect of epigallocatechin gallate on the major pathogens of canine periodontal disease and therapeutic effects on periodontal disease mice. Front Microbiol. (2023) 14:1329772. doi: 10.3389/fmicb.2023.1329772
171. Slobodníková L, Fialová S, Rendeková K, Kováč J, Mučaji P. Antibiofilm activity of plant polyphenols. Molecules. (2016) 21:1717. doi: 10.3390/molecules21121717
172. Chin YT, Hsieh MT, Lin CY, Kuo PJ, Yang YC, Shih YJ, et al. 2,3,5,4'-tetrahydroxystilbene-2-O-B-glucoside isolated from polygoni multiflori ameliorates the development of periodontitis. Mediators Inflammation. (2016) 2016:6953459. doi: 10.1155/2016/6953459
173. Jayusman PA, Nasruddin NS, Mahamad Apandi NI, Ibrahim N, Budin SB. Therapeutic potential of polyphenol and nanoparticles mediated delivery in periodontal inflammation: A review of current trends and future perspectives. Front Pharmacol. (2022) 13:847702. doi: 10.3389/fphar.2022.847702
174. Tang M, Li J, Wang G, Wang Y, Peng C, Chang X, et al. Cubic liquid crystals containing propolis flavonoids as in situ thermo-sensitive hydrogel depots for periodontitis treatment: preparation, pharmacodynamics and therapeutic mechanisms. Eur J Pharm Sci. (2024) 196:106762. doi: 10.1016/j.ejps.2024.106762
175. Tang M, Wang G, Li J, Wang Y, Peng C, Chang X, et al. Flavonoid extract from propolis alleviates periodontitis by boosting periodontium regeneration and inflammation resolution via regulating tlr4/myd88/nf-Kb and rank/nf-Kb pathway. J Ethnopharmacol. (2024) 319:117324. doi: 10.1016/j.jep.2023.117324
176. Amato M, Santonocito S, Viglianisi G, Tatullo M, Isola G. Impact of oral mesenchymal stem cells applications as a promising therapeutic target in the therapy of periodontal disease. Int J Mol Sci. (2022) 23:13419. doi: 10.3390/ijms232113419
177. Gao Y, Tian X, Zhang X, Milebe Nkoua GD, Chen F, Liu Y, et al. The roles of tissue-resident macrophages in sepsis-associated organ dysfunction. Heliyon. (2023) 9:e21391. doi: 10.1016/j.heliyon.2023.e21391
178. Cao M, Wang Z, Lan W, Xiang B, Liao W, Zhou J, et al. The roles of tissue resident macrophages in health and cancer. Exp Hematol Oncol. (2024) 13:3. doi: 10.1186/s40164-023-00469-0
179. Ma S, Zhang J, Liu H, Li S, Wang Q. The role of tissue-resident macrophages in the development and treatment of inflammatory bowel disease. Front Cell Dev Biol. (2022) 10:896591. doi: 10.3389/fcell.2022.896591
180. Biswas M. Understanding tissue-resident macrophages unlocks the potential for novel combinatorial strategies in breast cancer. Front Immunol. (2024) 15:1375528. doi: 10.3389/fimmu.2024.1375528
181. Li D, Xue W, Li M, Dong M, Wang J, Wang X, et al. Vcam-1(+) macrophages guide the homing of hspcs to a vascular niche. Nature. (2018) 564:119–24. doi: 10.1038/s41586-018-0709-7
182. Ratnayake D, Nguyen PD, Rossello FJ, Wimmer VC, Tan JL, Galvis LA, et al. Macrophages provide a transient muscle stem cell niche via nampt secretion. Nature. (2021) 591:281–7. doi: 10.1038/s41586-021-03199-7
183. Kim JE, Li B, Fei L, Horne R, Lee D, Loe AK, et al. Gut microbiota promotes stem cell differentiation through macrophage and mesenchymal niches in early postnatal development. Immunity. (2022) 55:2300–17.e6. doi: 10.1016/j.immuni.2022.11.003
184. Manneken JD, Currie PD. Macrophage-stem cell crosstalk: regulation of the stem cell niche. Development. (2023) 150:dev201510. doi: 10.1242/dev.201510
185. Anvari Y, Afrashteh A, Pourkaveh S, Salek SB, Al-Numan L, Khademnezhad S. Emerging role of mesenchymal stem cell-derived extracellular vesicles in periodontal regeneration. J Taibah Univ Med Sci. (2024) 19:390–402. doi: 10.1016/j.jtumed.2024.01.006
186. Costela-Ruiz VJ, Melguizo-Rodríguez L, Bellotti C, Illescas-Montes R, Stanco D, Arciola CR, et al. Different sources of mesenchymal stem cells for tissue regeneration: A guide to identifying the most favorable one in orthopedics and dentistry applications. Int J Mol Sci. (2022) 23:6356. doi: 10.3390/ijms23116356
187. Peng Y, Iwasaki K, Taguchi Y, Ishikawa I, Umeda M. Mesenchymal stem cell-derived protein extract induces periodontal regeneration. Cytotherapy. (2024). doi: 10.1016/j.jcyt.2024.10.003
188. Mendoza AH, Balzarini D, Alves T, Rovai ES, Holzhausen M. Potential of mesenchymal stem cell sheets on periodontal regeneration: A systematic review of pre-clinical studies. Curr Stem Cell Res Ther. (2023) 18:958–78. doi: 10.2174/1574888x17666220706092520
189. El-Sayed M, El-Feky MA, El-Amir MI, Hasan AS, Tag-Adeen M, Urata Y, et al. Immunomodulatory effect of mesenchymal stem cells: cell origin and cell quality variations. Mol Biol Rep. (2019) 46:1157–65. doi: 10.1007/s11033-018-04582-w
190. Chen L, Zhu S, Guo S, Tian W. Mechanisms and clinical application potential of mesenchymal stem cells-derived extracellular vesicles in periodontal regeneration. Stem Cell Res Ther. (2023) 14:26. doi: 10.1186/s13287-023-03242-6
191. Hu P, Yang Q, Wang Q, Shi C, Wang D, Armato U, et al. Mesenchymal stromal cells-exosomes: A promising cell-free therapeutic tool for wound healing and cutaneous regeneration. Burns Trauma. (2019) 7:38. doi: 10.1186/s41038-019-0178-8
Keywords: periodontal tissue regeneration, mesenchymal stem cells, periodontitis, alveolar bone loss, immunomodulation, inflammatory microenvironment
Citation: Zhao D-Z, Yang R-L, Wei H-X, Yang K, Yang Y-B, Wang N-X, Zhang Q, Chen F and Zhang T (2025) Advances in the research of immunomodulatory mechanism of mesenchymal stromal/stem cells on periodontal tissue regeneration. Front. Immunol. 15:1449411. doi: 10.3389/fimmu.2024.1449411
Received: 15 June 2024; Accepted: 13 December 2024;
Published: 03 January 2025.
Edited by:
Zahi Badran, University of Sharjah, United Arab EmiratesCopyright © 2025 Zhao, Yang, Wei, Yang, Yang, Wang, Zhang, Chen and Zhang. This is an open-access article distributed under the terms of the Creative Commons Attribution License (CC BY). The use, distribution or reproduction in other forums is permitted, provided the original author(s) and the copyright owner(s) are credited and that the original publication in this journal is cited, in accordance with accepted academic practice. No use, distribution or reproduction is permitted which does not comply with these terms.
*Correspondence: Tao Zhang, b2NlYW56dEAxNjMuY29t; Fang Chen, Mzg5Mzk0MjNAcXEuY29t
Disclaimer: All claims expressed in this article are solely those of the authors and do not necessarily represent those of their affiliated organizations, or those of the publisher, the editors and the reviewers. Any product that may be evaluated in this article or claim that may be made by its manufacturer is not guaranteed or endorsed by the publisher.
Research integrity at Frontiers
Learn more about the work of our research integrity team to safeguard the quality of each article we publish.