- 1Immunology and Molecular Oncology Unit, Veneto Institute of Oncology (IOV)-IRCCS, Padova, Italy
- 2Department of Surgery, Oncology and Gastroenterology, University of Padova, Padova, Italy
Triple-negative breast cancer (TNBC) represents a major therapeutic challenge due to its heterogeneous and aggressive phenotype, and limited target-specific treatment options. The trophoblast cell surface antigen (Trop-2), a transmembrane glycoprotein overexpressed in various cancers, has emerged as a promising target for TNBC. Sacituzumab govitecan (SG), an antibody-drug conjugate (ADC) that targets Trop-2, has recently entered treatment algorithms for advanced and metastatic TNBC, independently from Trop-2 expression status, with manageable toxicity. Despite the impressive results, questions remain unsolved regarding its efficacy, safety profile, and Trop-2 biological role in cancer. Currently, Trop-2 cannot be designated as a predictive biomarker in SG treatment, albeit its expression correlates with disease outcome, yet its levels are not uniform across all TNBCs. Additionally, data regarding Trop-2 expression variations in primary and metastatic sites, and its interplay with other biomarkers are still ambiguous but mandatory in light of future applications of SG in other indications and settings. This poses the questions of a careful evaluation of the efficacy and toxicity profile of SG in such early stages of disease, and in personalized and combinatorial strategies. Research and clinical data are mandatory to address SG drawbacks and minimize its benefits, to realize its full potential as therapeutic agent in different epithelial tumors.
Introduction
Breast cancer (BC) is one of the leading causes of female mortality (1), where triple-negative breast cancer (TNBC) subtype represents 15%-25% of all cases. This malignancy is characterized by high invasiveness, metastatic potential to distant sites, such as bone, lung, liver, and brain (2), leading to increased mortality rates, proneness to relapse (3), and short survival after the onset of metastatic disease (4). The aggressive tumor behavior and limited treatment options highlight the urgency to identify novel prognostic biomarkers and cancer-specific therapeutic targets to improve patient outcomes. Trophoblast cell surface antigen 2 (Trop-2) is a 35 kDa transmembrane calcium signal transducer glycoprotein, overexpressed in various epithelial tumors, representing a valuable target for managing malignancies with limited treatment options, such as TNBC (5). Antibody-drug conjugates (ADCs) are an emerging category of antineoplastic treatments that combine the selectivity of monoclonal antibodies (mAbs) with the potency of cytotoxic drugs (6). This approach has been successfully exploited, leading to the accelerated approval of sacituzumab govitecan (SG, IMMU-132) for the treatment of locally advanced or metastatic TNBC (mTNBC), suggesting a new era in TNBC treatment. However, questions about the efficacy and safety profile of SG are arising, as well as the still uncovered multiple biological interplays with different molecules and the predictive or prognostic value of its target Trop-2 in cancer. Here, in an attempt to shedding light on these unmet topics, we discuss recent data about TNBC treatment with SG from trials and clinical practice.
Triple-negative breast cancer: treatment options and new promising possibilities
The aggressive tumor behavior and the lack of expression of estrogen (ER) and progesterone (PR) receptors, along with the absence of human epidermal growth factor receptor 2 (HER2) overexpression (7), lead to the deficiency of targeted therapeutic solutions for TNBCs. Consequently, treatment options rely on surgical excision, radiation, and nonspecific chemotherapy, with limited efficacy and short progression-free survival (PFS), especially in patients who have previously received therapies in the metastatic setting (8). Despite these efforts, over 50% of TNBC patients experience relapse within 3-5 years, with a median overall survival (OS) of only 10.2 months (9). Moreover, all TNBC subtypes are directly associated with epithelial-to-mesenchymal transition (EMT), which plays a role in the development of drug resistance and further complicating treatment (10). In recent years, several promising therapeutic algorithms have emerged for TNBC. BRCA-targeted therapies such as olaparib (11) and talazoparib (12), exploit DNA repair deficiencies in BRCA-mutated TNBC. Immune checkpoint inhibitors (atezolizumab, pembrolizumab) have improved outcomes in programmed death-ligand 1 (PDL1)-positive mTNBC when combined with chemotherapy (13). Novel therapies targeting HER2-low expression (trastuzumab deruxtecan) show promise (14), while anti-androgen therapies (bicalutamide, enzalutamide) are explored in androgen receptor (AR)-positive TNBC (15). Inhibitors of the PI3K/AKT/mTOR pathway (alpelisib, everolimus) (16) and other targeted treatments like MEK inhibitors (trametinib) (17) are also alternative strategies still under investigations. However, such options are exploitable only for specific TNBC subgroups, and thus the identification of attractive targets in the therapeutic hunt for TNBCs is still an open challenge, together with the design and validation of effective ADCs. These included ladiratuzumab vedotin and patritumab deruxtecan that target LIV-1 and HER3, respectively. Despite the promising objective response rate (ORR), such treatments demonstrated suboptimal safety profiles (18).
Trop-2 as a therapeutic target: state of art
Among promising therapeutic target candidates, Trop-2 has recently emerged as one of the most promising. It was firstly identified as widely expressed in various healthy epithelial and mucosal tissues (19–21) where it plays an essential role in stem cell proliferation, embryonic development and placental tissue formation (22, 23). In pathological context, it recently arouses curiosity due to the anomalous overexpression of both protein and mRNA in various solid cancers (Figure 1A) (28–31), where it is associated with aggressive tumor characteristics, such as enhanced growth, invasion, and metastasis (32–36). Moreover, in occurrence of conventional treatments failure, enhanced Trop-2 expression levels have been associated with therapeutic success, paving the way to the exploitation of a novel class of therapeutics (37). Indeed, the correlation of Trop-2 expression with disease and/or clinical outcome in multiple tumor types, and the lower expression in normal adult tissues compared to several pathological counterpart (38–41), underscores the potential benefit of targeting Trop-2 to fill an unmet need in cancer treatment. Trop-2 is overexpressed across all BCs, especially in aggressive subtypes such as TNBC and HR+/HER2-, where it resulted a strong predictor of lymph node involvement, distant metastasis, and poor OS (42). Of note, its oncogenic potential was highlighted in TNBC, where it was linked to oncogenic metabolism elements, therapeutic resistance, poorer OS even at early stages (43, 44), leading to the approval of ADCs for metastatic malignancy even though only as third line treatment (45, 46). Moreover, its consistent high expression on circulating-tumor-cells, makes it a valuable potential marker for EMT and initiation of metastatic processes (47). Remarkably, as recently highlighted by the ASCENT trial results, TNBC patients belonging to the two highest Trop-2-expressing quartiles demonstrated an improvement in both PFS and OS, exhibiting better response rates to the treatment compared to those with low Trop-2 expression (48). In addition, data from clinical trials have reported response rates to SG not exceeding the 35%, augmenting the perplexity about how the absence or very low levels of Trop-2 expression may have contributed to therapy failures and enhanced side effects (49). In fact, it is crucial to consider the possibility of enhanced risk of side effects, due to its ubiquitous nature in widely represented normal tissues, as squamous epithelial and mucosa (50, 51).
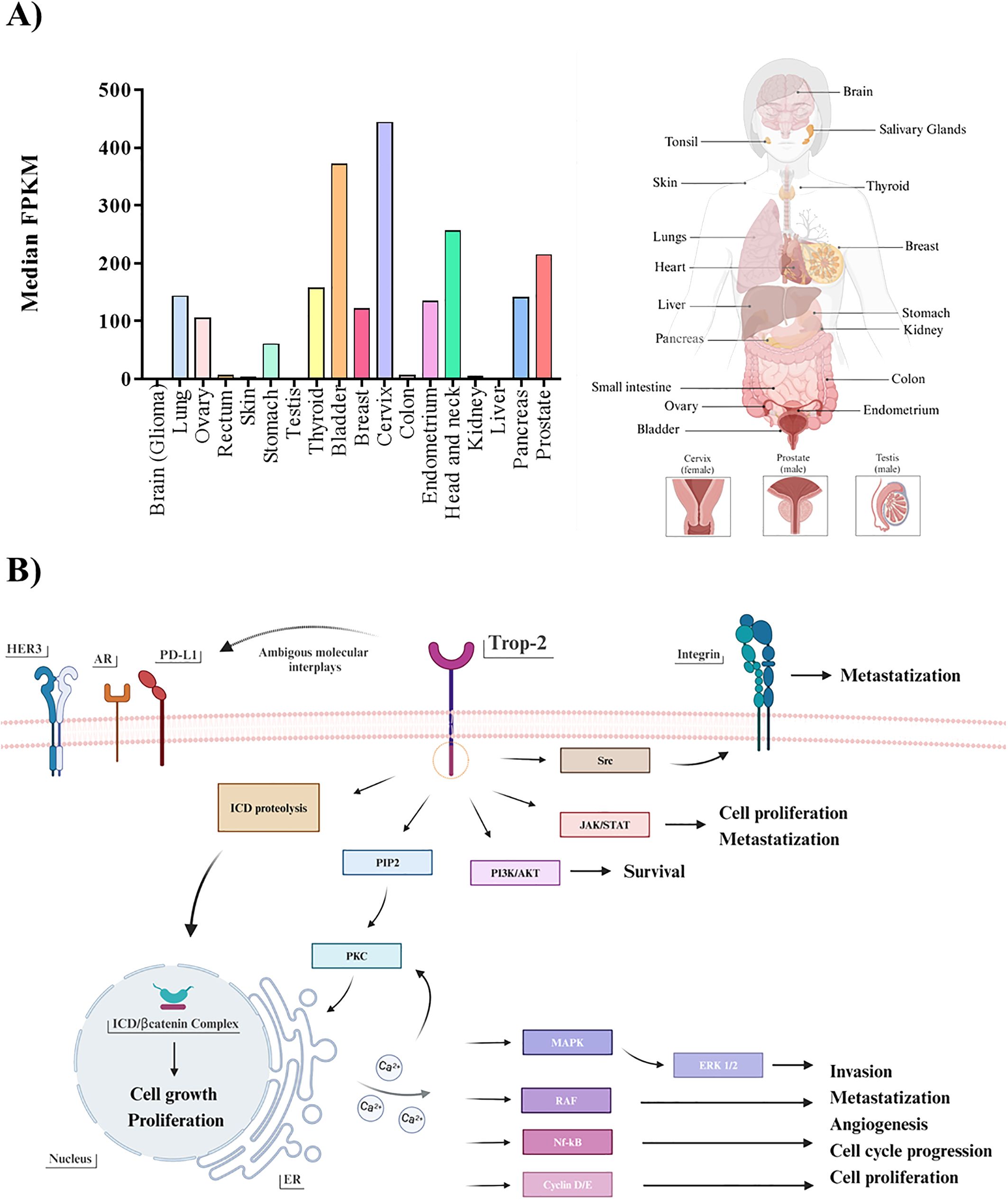
Figure 1. (A) Trop-2 mRNA profile. Boxplots summarizing the mRNA quantification of Trop-2 in pathological malignancies (left). Values represent the median number Fragments Per Kilobase of exon per Million reads (FPKM), generated by The Cancer Genome Atlas (TCGA). Values from brain, testis and liver ranging up to 10 median FPKM result not visible. All described tissues are indicated in the correspondent body district (right). (B) Trop-2 downstream pathways. Schematic resume of Trop-2 molecular downstream mechanisms triggered in cancerous setting: ERK1/2MAPK pathway emerges in all main tumorigenic, proliferative and metastatic processes, alongside with the other PIP2/PKC-induced calcium-regulated downstream pathways (RAF/Nf-kB/Cyclin D/E) (24). JAK/STAT and PI3K/AKT were described to be implicated in survival, proliferation and metastatic processes (25), as for the Src mediated intervention on the integrin-fibronectin axis (26). In addition, regulated proteolysis of Trop-2 was found to drive proliferative and self-renewal signaling via β-catenin (27). Possible ambiguous interplays occurring between Trop-2 and other plausible receptor already cited in the text are also illustrated.
On the other hand, it is pivotal to remark that the recruitment strategy of TNBC patients in ADC-based trials is most likely due to the limited therapeutic options available for those who have experienced progression after at least two prior therapies, rather than to a particularly high rate of Trop-2 expression. As matter of fact, as showed in a recent work by Dum et al., among a total subset of 18,563 different tissue tumor microarrays examined (n=2,139 for BC), TNBC does not rank among the top cancers with high Trop-2 expression, with only 54.4% of cases being strongly positive for the receptor (52). These findings may suggest that ranking tumor types by Trop-2 expression frequency and intensity may help identify a more precise approach to select patients for successful therapeutic outcome. Alongside, a further advancement in proper personalized treatment approaches and positive therapeutic rebound may be represented by following up Trop-2 level throughout the whole therapeutic regimen, starting from the early stages of the disease. Indeed, rigorous monitoring should be advocated not only concerning HR phenotype of metastases, but also focusing on Trop-2 expression fluctuations, related to metabolic and transcriptional plasticity induced by EMT (53). On this line, the establishment of a standardized detection methodology with diagnostic utility may aid in the comprehensive analysis of the receptor, essential for efficient tumor stratification and expression status check-up (54). Due to the complexity of Trop-2 induced downstream network (Figure 1B), attention was called to the differential role of Trop-2 in a tumor-type dependent manner, as recent studies indicated the promotion of tumorigenesis in concomitance with the loss of Trop-2, depending on the cell type and context (32, 55). Indeed, identifying reliable associated biomarkers is also essential to aid patient selection and prediction of therapeutic responses. In this regard, observations were reported in literature concerning in both prostate cancer and TNBC, where Trop-2 and AR showed interconnection in their expression (56, 57). Furthermore, in head-and-neck squamous cell carcinoma, the reduction of Trop-2 expression resulted in sensitivity to anti-HER3 antibodies (58) while in non-small cells lung cancer it was observed that Trop-2 overexpression was linked to the primary resistance to PD-L1 blockade, suggesting an interconnection existing between the two receptors, and thus recommending the administration of combinatorial therapy solely after a Trop-2-based selection (59). This emphasizes the pleiotropic nature of Trop-2 biology and interaction patterns, warranting further exploration of its tumorigenic downstream pathways and the consequential influence of these ambiguous molecular interplays in its potential biomarker/therapeutic target role, uncovering the reason behind suboptimal treatment outcomes and therapy failures.
Sacituzumab govitecan: a new era for immunotherapy of TNBC (with future challenges)
SG is a third-generation ADC that exploits the humanized anti-Trop-2 mAb hRS7 (IgG1κ) to deliver SN-38, the active metabolite of the topoisomerase I inhibitor irinotecan. Both the hydrophobicity and high potency of SN-38 preclude its direct use in the clinic, with patients experiencing grade 3/4 diarrhea (60). Conversely, SG delivers higher levels of SN-38 with an improved safety and tolerability profile (61–63). This is the result of SG unique design, in which SN38 is linked to the mAb through the hydrolizable pH-sensitive linker CL2A, allowing the delivery of up to 8 moieties per single molecule (Figure 2A) (61). Overall, SG represents a breakthrough in TNBC treatment, as a paradigm shift and challenge in ADC design, since the majority of ADCs delivers ultra-toxic payloads (IC50 values of pM ranges and often narrow therapeutic index). The lower potency of SN38 (IC50 of nM) enables a high drug to antibody ratio (DAR), and the administration of the highest clinical dosing regimen of all Food and Drug Administration (FDA)-approved ADCs: 10 mg/kg administered on days 1 and 8 of a 21-day cycle (69), avoiding side effects usually associated with other ADCs, such as interstitial lung disease or pneumonitis (70). The putative mechanism of action of SG is summarized in Figure 2B. IMMU-132-01 was the first phase I/II multicenter, single-arm, basket study to evaluate the single-agent activity, safety and tolerability of SG. The study enrolled patients with different advanced epithelial cancers, refractory to/relapsed after at least one standard line of chemotherapy in the metastatic setting. Subjects with active brain metastasis or under systemic corticosteroids for more than 2 weeks before enrolment were not eligible. Notably, the 69 patients with mTNBC were heavily pretreated, with a median of 5 (range 1-12) prior lines of therapies. Twenty-one subjects (30%) achieved an ORR, and the median duration of response (DoR) at the time was 8.9 months (95% CI, 6.1-11.3). Both BRCA germline status and Trop-2 expression were evaluated (60% primary tumors and 40% miscellaneous metastases), with 7/43 patients being BRCA1 mutated and 42/48 subjects having moderate-high Trop-2 staining. Consistent with preclinical findings showing greater antitumor effects of SG in mice bearing tumors with high Trop-2 expression (62, 67, 68), a positive trend was observed in PFS for patients with 2+ or 3+ Trop-2. Conversely, subjects bearing weak or negative Trop-2 levels obtained only stable disease as best response (71). In 2019, efficacy results were furtherly confirmed in a larger cohort of mTNBC, with an ORR 33.3% (95% CI, 24.6-43.1), a median time to response (TTR) of 2.0 months (1.6-13.5 range), and a median DoR of 7.7 months (95% CI, 4.9-10.8). A clinical benefit was observed in 49 patients (45.4%). For survival endpoints, the PFS was 5.5 months (95% CI, 4.1-6.3) and OS reached 13 months (95% CI, 11.2-13.7), with a manageable toxicity profile (49). Efficacy and safety in mTNBC were further evaluated in the phase III confirmatory ASCENT trial, in comparison to the treatment of physician’s choice (TPC). The primary endpoint was PFS in patients without brain metastases, while secondary endpoints included PFS in the intention-to-treat population, OS in both populations, ORR, DoR, quality of life (QoL), and safety. SG outperformed TPC in terms of PFS (5.6 months versus 1.7 months; HR 0.41, 95% CI 0.32–0.52) and OS (12.1 months vs 6.7 months; HR 0.48, 95% CI 0.38–0.59) in the population without brain metastases, with an ORR of 31% versus 4% in the full population (72). The trial was halted early in March 2020 due to compelling evidence of efficacy, granting accelerated approval by the FDA and the European Medicine Agency (EMA) for patients with unresectable locally advanced or mTNBC who received two or more prior lines of therapy, including at least one for metastatic disease. Parallelly, SG was also approved for advanced HR-positive, HER2-negative BCs that had already received endocrine-based therapy and at least two additional systemic therapies in the metastatic setting (TROPiCS-02) (73). In April 2021, FDA granted accelerated approval for the treatment of advanced urothelial cancer after platinum and PD-1/PD-L1-directed checkpoint inhibitors (TROPHY-U-01) (74). Updated results from the ASCENT trial confirmed the previous data, with SG improving outcomes over TPC despite Trop-2 expression level. However, a trend in Trop-2 subgroups is noticeable: in the lower quartiles of Trop-2, the improvement of clinical outcomes appeared to be less pronounced, with PFS of 2.7 months (HR 0.58, 95% CI 1.4-5.7) and OS of 8.7 (HR 0.74, 95% CI 6.9-12.9). These data, even if explorative, suggest that also Trop-2-low mTNBCs may benefit from SG, as occurred for trastuzumab deruxtecan in HER2-low BCs (44). Adverse events (AEs) observed were consistent with those reported in previous trials (46). The most common registered toxicities of any grade included neutropenia (the prevalent reason for treatment interruption), nausea, diarrhea, fatigue, anemia, and vomiting. The most frequent grade ≥ 3 AEs for SG were neutropenia (52% vs 34%), febrile neutropenia (6% vs 3%), diarrhea (12% vs 1%), leukopenia (11% vs 6%), anemia (9% vs 6%), nausea and abdominal pain (both 3% vs ~1%), primarily associated with SN-38 and manageable with supportive care. Unlike other ADCs, SG did not show an increased risk of interstitial lung disease or cardiovascular toxicity, with patients reporting a meaningful improvement in QoL (75). Interestingly, no correlation between AEs ad Trop-2 status has emerged (48). Overall, these data supported the potential of SG to mark a substantial advancement toward pretreated mTNBC. Nevertheless, many questions are still unresolved. Since SG, as other ADCs, is gaining increasing interest as first-line agent or in the adjuvant setting (Table 1), its long-term efficacy, safety and reversibility of side effects are becoming of paramount importance, as the response rates may drastically vary based on indications and settings. The geriatric population (age ≥ 65 years) showed a tendency to experience higher rates of SG discontinuation due to AEs. However, such difference in the ASCENT study was not statistically significant, probably because this population is a minority (SG and TCP together, 19%) (48, 72). Trials concerning other tumor histotypes in which geriatrics were much more represented (74, 76) reported increased discontinuation rates for SG, highlighting the necessity to further investigate such subpopulation in TNBCs. Real-world studies and pharmacovigilance safety databases will contribute to a clear comprehension of AEs in elderly and other subgroups (i.e., ethnical minorities, patients with ECOG-PS ≥ 2, and/or with active brain metastases, etc.). Indeed, the few available studies, while confirming the efficacy of SG, also pointed out the complexity of such populations and the necessity of a thorough evaluation and understanding of some AEs such as alopecia and pneumonitis (77–79). As discussed above, Trop-2 expression evolution in cancer is still unclear, and samples analyzed in clinical trials were a miscellaneous of primary and various metastatic sites. Its overexpression may be detected at very early-stages of disease, even during tumor formation, opening new possibility to a prompt therapeutic intervention for TNBC, and posing questions about a universal method for Trop-2 detection. Despite being well-established and validated, the immunohistochemical analysis reported in SG clinical trials differed in terms of antibody selection for Trop-2, ranging from polyclonal (80) to murine mAb (81), mining interpretation and comparison of such data. Additionally, some chemotherapies, i.e. irinotecan itself, may alter Trop-2 expression (82), which seems also to be directly involved in promoting drug resistance (59, 82, 83). In this connection, the relapse of cancer patients responding to SG raises the question of resistance against the cytotoxic payload and/or the mAb. The mechanisms underlying resistance to ADCs are likely to be complex. While data concerning resistance to the unconjugated mAb counterparts are available for other ADCs (84–86), knowledge regarding SG is more than preliminary. On one hand, topoisomerase inhibitors are known to induce multidrug resistance (87), while on the other, it was recently demonstrated that distinct acquired resistance mutations involving both Trop-2 and topoisomerase I emerged within different metastatic lesions of a SG-treated patient (88). The management of SG-derived AEs poses the question whether these events may depend on SN-38 itself or Trop-2-bound ADC in off-target tissues. ADCs may bind and then be internalized in healthy tissues which express low levels of the target antigen, even though the majority of AEs is likely attributable to off-target events or release of the cytotoxic payload in the bloodstream. Moreover, toxicity in normal cells may be related to ADC uptake mediated by Fcγ receptors, with cells expressing high levels of mannose receptors such as in myeloid, endothelial and hepatic cells tissues, displaying higher abilities to interact with a relatively high proportion of galactosylated glycans on the Fc domain of the mAb, favoring off-target toxicities (89, 90).
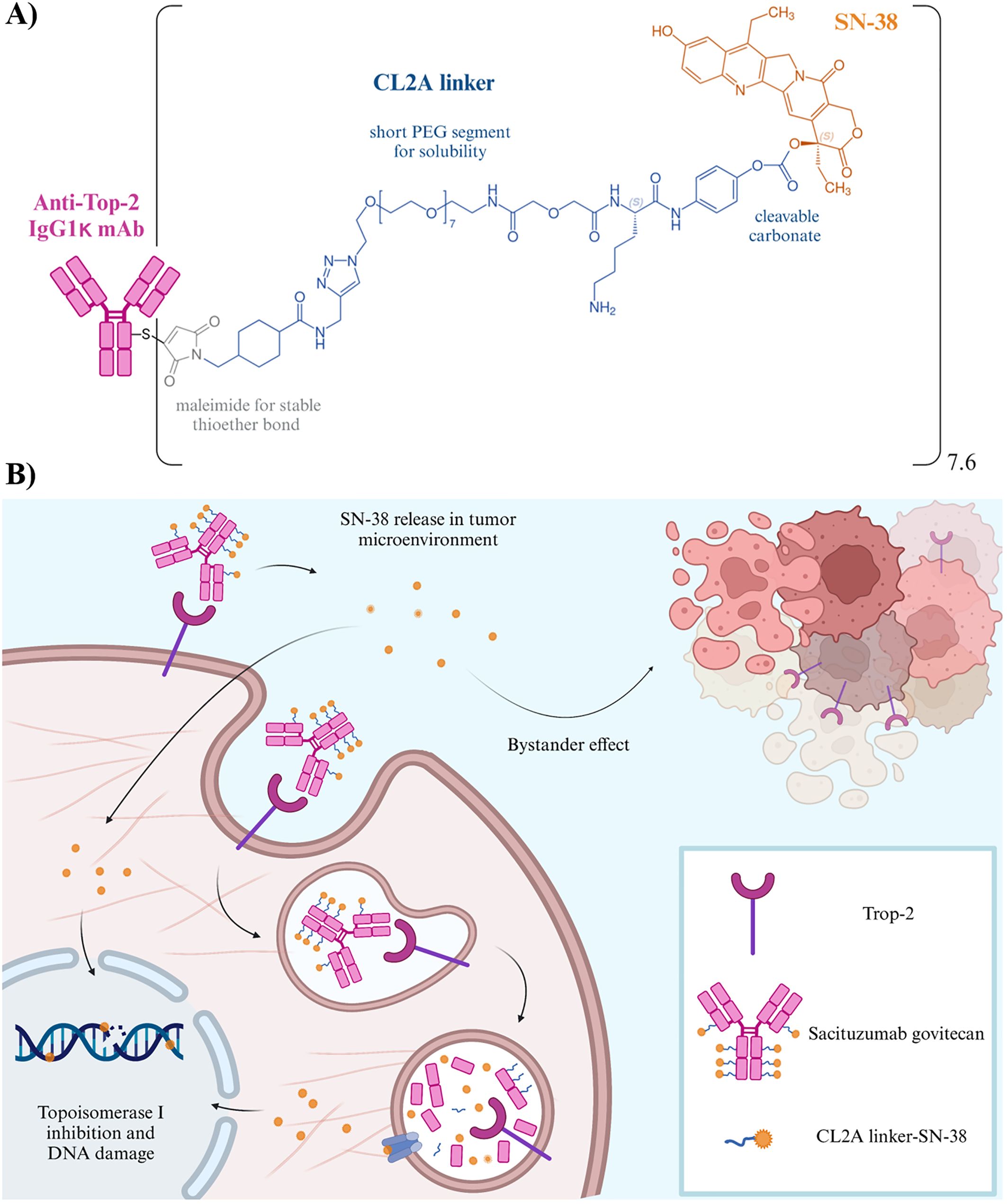
Figure 2. (A) Structure of SG. Schematic representation of the chemical structure of the linkage of SN-38 (shown in orange) to the hRS7 antibody (pink) via the CL2A-linker (blue). Specifically, the CL2A linker binds to the 20th position of SN-38, stabilizing the lactone ring and forming a pH-sensitive carbonate bond. The presence of a short polyethylene glycol (PEG) segment enhances the water solubility of such SN-38 conjugate. Moreover, the maleimide inserted at the end of the linker enables a stable thioether bond with sulfhydryl moieties formed after mild reduction of the hRS7 mAb. The average DAR is 7.6 (61). (B) Mechanism of action of SG. i) The ADC recognizes and binds to Trop-2 on the tumor cell, being then be internalized; ii) the payload undergoes intracellular trafficking and enters the lysosomes following antibody catabolism and hydrolysis of the linker, the payload is released and induces apoptotic cell death. Neighboring cancer cells are affected by the bystander effect (64, 65), due to the release of SN-38 from the target cell or within the extracellular space, thus contributing to an amplified anti-tumor effect (61, 66). The unconjugated hRS7 mAb showed some effector function in vitro (i.e. antibody-dependent cellular cytoxicity), which resulted mitigated in the ADC due to mAb reduction for conjugation purposes (67, 68).
Conclusions and outlook
SG has emerged as a promising option in TNBC due to its high efficacy and safety profile and unique mechanism of action. On the other hand, it is crucial to highlight that the road to success is paved with challenges, especially for entering new treatment algorithms in different contexts and settings. Indeed, “old” challenges are still present, which are basically related to patient selection and biomarker assessment. As research and clinical experience with SG continue to grow, a precise analysis leading back to bench aimed at addressing its limitations and enhancing its efficacy profile, will pave solid bases for exploiting its full potential in the management not only of TNBC and other malignancies, but also to promote the improvement of other Trop-2 targeting strategies.
Author contributions
VR: Visualization, Writing – original draft, Writing – review & editing. AT: Writing – original draft, Writing – review & editing. AR: Funding acquisition, Writing – review & editing. DC: Conceptualization, Funding acquisition, Writing – original draft, Writing – review & editing.
Funding
The author(s) declare financial support was received for the research, authorship, and/or publication of this article. The authors are grateful to the 5 per mille reference year 2019 - BRIDGE for the financial support of the project FREEDA to DC. They also thank the funding of the Associazione Italiana per la Ricerca sul Cancro AIRC-MFAG 2023 ID 28866 to DC; AIRC-IG 2018 ID 21354 to AR.
Conflict of interest
The authors declare that the research was conducted in the absence of any commercial or financial relationships that could be construed as a potential conflict of interest.
Publisher’s note
All claims expressed in this article are solely those of the authors and do not necessarily represent those of their affiliated organizations, or those of the publisher, the editors and the reviewers. Any product that may be evaluated in this article, or claim that may be made by its manufacturer, is not guaranteed or endorsed by the publisher.
References
1. Siegel RL, Miller KD, Wagle NS, Jemal A. Cancer statistics, 2023. CA Cancer J Clin. (2023) 73:17–48. doi: 10.3322/caac.21763
2. Meng X, Cai Y, Chang X, Guo Y. A novel conditional survival nomogram for monitoring real-time prognosis of non-metastatic triple-negative breast cancer. Front Endocrinol (Lausanne). (2023) 14. doi: 10.3389/fendo.2023.1119105
3. Boyle P. Triple-negative breast cancer: Epidemiological considerations and recommendations. Ann Oncol. (2012) 23(Suppl 6):vi7–12. doi: 10.1093/annonc/mds187
4. Bou Zerdan M, Ghorayeb T, Saliba F, Allam S, Bou Zerdan M, Yaghi M, et al. Triple negative breast cancer: updates on classification and treatment in 2021. Cancers. (2022) 14(5):1253. doi: 10.3390/cancers14051253
5. Qiu S, Zhang J, Wang Z, Lan H, Hou J, Zhang N, et al. Targeting Trop-2 in cancer: Recent research progress and clinical application. Biochim Biophys Acta Rev Cancer. (2023) 1878:188902. doi: 10.1016/j.bbcan.2023.188902
6. Fu Z, Li S, Han S, Shi C, Zhang Y. Antibody drug conjugate: the “biological missile” for targeted cancer therapy. Signal Transduction Targeted Ther. (2022) 7(1):93. doi: 10.1038/s41392-022-00947-7
7. Almansour NM. Triple-negative breast cancer: A brief review about epidemiology, risk factors, signaling pathways, treatment and role of artificial intelligence. Front Mol Biosci. (2022) 9:836417/full. doi: 10.3389/fmolb.2022.836417/full
8. Catalano A, Iacopetta D, Ceramella J, Mariconda A, Rosano C, Scumaci D, et al. New achievements for the treatment of triple-negative breast cancer. Appl Sci (Switzerland). (2022) 12(11):5554. doi: 10.3390/app12115554
9. Newton EE, Mueller LE, Treadwell SM, Morris CA, MaChado HL. Molecular targets of triple-negative breast cancer: where do we stand? Cancers. (2022) 14(3):482. doi: 10.3390/cancers14030482
10. De Las Rivas J, Brozovic A, Izraely S, Casas-Pais A, Witz IP, Figueroa A. Cancer drug resistance induced by EMT: novel therapeutic strategies. Arch Toxicol. (2021) 95:2279–97. doi: 10.1007/s00204-021-03063-7
11. Eikesdal HP, Yndestad S, Elzawahry A, Llop-Guevara A, Gilje B, Blix ES, et al. Olaparib monotherapy as primary treatment in unselected triple negative breast cancer. Ann Oncol. (2021) 32:240–9. doi: 10.1016/j.annonc.2020.11.009
12. Savill KMZ, Ivanova J, Asgarisabet P, Falkenstein A, Balanean A, Niyazov A, et al. Characteristics, treatment, and outcomes of real-world talazoparib-treated patients with germline BRCA-mutated advanced HER2-negative breast cancer. Oncologist. (2023) 28:414–24. doi: 10.1093/oncolo/oyad021
13. Nozaki Y, Yamamuro M, Tanaka N, Kamo N, Konishi J. Successful retreatment of metastatic triple-negative breast cancer with immune checkpoint inhibitors and chemotherapy. Cancer Diagnosis Prognosis. (2023) 3:387–91. doi: 10.21873/cdp.
14. Tsurutani J, Iwata H, Krop I, Jänne PA, Doi T, Takahashi S, et al. Targeting her2 with trastuzumab deruxtecan: A dose-expansion, phase i study in multiple advanced solid tumors. Cancer Discovery. (2020) 10:688–701. doi: 10.1158/2159-8290.CD-19-1014
15. Khadela A, Chavda VP, Soni S, Megha K, Pandya AJ, Vora L. Anti-androgenic therapies targeting the luminal androgen receptor of a typical triple-negative breast cancer. Cancers. (2023) 15(1):233. doi: 10.3390/cancers15010233
16. Cerma K, Piacentini F, Moscetti L, Barbolini M, Canino F, Tornincasa A, et al. Targeting PI3K/AKT/mTOR pathway in breast cancer: from biology to clinical challenges. Biomedicines. (2023) 11(1):109. doi: 10.3390/biomedicines11010109
17. Lim B, Peterson CB, Davis A, Cho E, Pearson T, Liu H, et al. ONC201 and an MEK inhibitor trametinib synergistically inhibit the growth of triple-negative breast cancer cells. Biomedicines. (2021) 9(10):1410. doi: 10.3390/biomedicines9101410
18. Corti C, Giugliano F, Nicolò E, Ascione L, Curigliano G. Antibody–drug conjugates for the treatment of breast cancer. Cancers. (2021) 13(12):2898. doi: 10.3390/cancers13122898
19. Shvartsur A, Bonavida B. Trop2 and its overexpression in cancers: regulation and clinical/therapeutic implications. Genes Cancer (2015) 6:84–105. doi: 10.18632/genesandcancer.v6i3-4
20. Liu X, Li J, Deng J, Zhao J, Zhao G, Zhang T, et al. Targeting Trop2 in solid tumors: a look into structures and novel epitopes. Front Immunol. (2023) 14:1–8. doi: 10.3389/fimmu.2023.1332489
21. Stepan LP, Trueblood ES, Hale K, Babcook J, Borges L, Sutherland CL. Expression of Trop2 cell surface glycoprotein in normal and tumor tissues: Potential implications as a cancer therapeutic target. J Histochem Cytochemistry. (2011) 59:701–10. doi: 10.1369/0022155411410430
22. Sakach E, Sacks R, Kalinsky K. Trop-2 as a therapeutic target in breast cancer. Cancers (Basel). (2022) 14(23):5936. doi: 10.3390/cancers14235936
23. Zeng P, Chen MB, Zhou LN, Tang M, Liu CY, Lu PH. Impact of TROP2 expression on prognosis in solid tumors: A Systematic Review and Meta-analysis. Sci Rep. (2016) 6:33658. doi: 10.1038/srep33658
24. Strop P, Tran TT, Dorywalska M, Delaria K, Dushin R, Wong OK, et al. RN927C, a site-specific trop-2 antibody-drug conjugate (ADC) with enhanced stability, is highly efficacious in preclinical solid tumor models. Mol Cancer Ther. (2016) 15:2698–708. doi: 10.1158/1535-7163.MCT-16-0431
25. Lenárt S, Lenárt P, Šmarda J, Remšík J, Souček K, Beneš P. Trop2: Jack of all trades, master of none. Cancers. (2020) 12:1–28. doi: 10.3390/cancers12113328
26. Guerra E, Trerotola M, Alberti S. Targeting trop-2 as a cancer driver. J Clin Oncol. (2023) 41:4688–92. doi: 10.1200/JCO.23.01207
27. Stoyanova T, Goldstein AS, Cai H, Drake JM, Huang J, Witte ON. Regulated proteolysis of Trop2 drives epithelial hyperplasia and stem cell self-renewal via β-catenin signaling. Genes Dev. (2012) 26:2271–85. doi: 10.1101/gad.196451.112
28. Trerotola M, Cantanelli P, Guerra E, Tripaldi R, Aloisi AL, Bonasera V, et al. Upregulation of Trop-2 quantitatively stimulates human cancer growth. Oncogene. (2013) 32:222–33. doi: 10.1038/onc.2012.36
29. Mas L, Cros JF, Svrcek M, Luc J, Van Laethem B, François J, et al. Trop-2 is a ubiquitous and promising target in pancreatic adenocarcinoma. Clin Res Hepatol Gastroenterol. (2023) 2023:10. doi: 10.1016/j.clinre.2023.102108
30. Tang G, Tang Q, Jia L, Chen Y, Lin L, Kuai X, et al. TROP2 increases growth and metastasis of human oral squamous cell carcinoma through activation of the PI3K/Akt signaling pathway. Int J Mol Med. (2019) 44:2161–70. doi: 10.3892/ijmm
31. Chou J, Trepka K, Sjöström M, Egusa EA, Chu CE, Zhu J, et al. TROP2 expression across molecular subtypes of urothelial carcinoma and enfortumab vedotin-resistant cells. Eur Urol Oncol. (2022) 5:714–8. doi: 10.1016/j.euo.2021.11.005
32. Liu X, Deng J, Yuan Y, Chen W, Sun W, Wang Y, et al. Advances in Trop2-targeted therapy: Novel agents and opportunities beyond breast cancer. Pharmacol Ther. (2022) 239:108296. doi: 10.1016/j.pharmthera.2022.108296
33. Hoppe S, Meder L, Gebauer F, Ullrich RT, Zander T, Hillmer AM, et al. Trophoblast cell surface antigen 2 (TROP2) as a predictive bio-marker for the therapeutic efficacy of sacituzumab govitecan in adenocarcinoma of the esophagus. Cancers (Basel). (2022) 14(19):4789. doi: 10.3390/cancers14194789
34. Liao S, Wang B, Zeng R, Bao H, Chen X, Dixit R, et al. Recent advances in trophoblast cell-surface antigen 2 targeted therapy for solid tumors. Drug Dev Res. (2021) 82:1096–110. doi: 10.1002/ddr.21870
35. Lombardi P, Filetti M, Falcone R, Altamura V, Paroni Sterbini F, Bria E, et al. Overview of trop-2 in cancer: from pre-clinical studies to future directions in clinical settings. Cancers. (2023) 15(6):1744. doi: 10.3390/cancers15061744
36. Shen M, Liu S, Stoyanova T. The role of Trop2 in prostate cancer: an oncogene, biomarker, and therapeutic target. Am J Clin Exp Urol. (2021) 9(1):73–8.
37. Yao L, Chen J, Ma W. Decoding TROP2 in breast cancer: significance, clinical implications, and therapeutic advancements. Front Oncol. (2023) 13:1–9. doi: 10.3389/fonc.2023.1292211
38. Erber R, Spoerl S, Mamilos A, Krupar R, Hartmann A, Ruebner M, et al. Impact of spatially heterogeneous trop-2 expression on prognosis in oral squamous cell carcinoma. Int J Mol Sci. (2022) 23(1):87. doi: 10.3390/ijms23010087
39. Yeung V, Zaemes J, Yeh J, Giancarlo C, Ahn J, Reuss JE, et al. High levels of expression of Trop-2 in thymic epithelial tumors. Lung Cancer. (2023) 184:107324. doi: 10.1016/j.lungcan.2023.107324
40. Bignotti E, Todeschini P, Calza S, Falchetti M, Ravanini M, Tassi RA, et al. Trop-2 overexpression as an independent marker for poor overall survival in ovarian carcinoma patients. Eur J Cancer. (2010) 46:944–53. doi: 10.1016/j.ejca.2009.12.019
41. Omori S, Muramatsu K, Kawata T, Miyawaki E, Miyawaki T, Mamesaya N, et al. Trophoblast cell-surface antigen 2 expression in lung cancer patients and the effects of anti-cancer treatments. J Cancer Res Clin Oncol. (2022) 148:2455–63. doi: 10.1007/s00432-021-03784-3
42. Lin H, Huang JF, Qiu JR, Zhang HL, Tang XJ, Li H, et al. Significantly upregulated TACSTD2 and Cyclin D1 correlate with poor prognosis of invasive ductal breast cancer. Exp Mol Pathol. (2013) 94:73–8. doi: 10.1016/j.yexmp.2012.08.004
43. Jeon Y, Jo U, Hong J, Gong G, Lee HJ. Trophoblast cell-surface antigen 2 (TROP2) expression in triple-negative breast cancer. BMC Cancer. (2022) 22:1–9. doi: 10.1186/s12885-022-10076-7
44. Carvalho FM. Triple-negative breast cancer: from none to multiple therapeutic targets in two decades. Front Oncol. (2023) 13. doi: 10.3389/fonc.2023.1244781
45. Okajima D, Yasuda S, Maejima T, Karibe T, Sakurai K, Aida T, et al. Datopotamab deruxtecan, a novel TROP2-directed antibody-drug conjugate, demonstrates potent antitumor activity by efficient drug delivery to tumor cells. Mol Cancer Ther. (2021) 20:2329–40. doi: 10.1158/1535-7163.MCT-21-0206
46. Bardia A, Messersmith WA, Kio EA, Berlin JD, Vahdat L, Masters GA, et al. Sacituzumab govitecan, a Trop-2-directed antibody-drug conjugate, for patients with epithelial cancer: final safety and efficacy results from the phase I/II IMMU-132-01 basket trial. Ann Oncol. (2021) 32:746–56. doi: 10.1016/j.annonc.2021.03.005
47. Liao Q, Zhang R, Ou Z, Ye Y, Zeng Q, Wang Y, et al. TROP2 is highly expressed in triple-negative breast cancer CTCs and is a potential marker for epithelial mesenchymal CTCs. Mol Therapy: Oncol. (2024) 32:200762. doi: 10.1016/j.omton.2024.200762
48. Bardia A, Rugo HS, Tolaney SM, Loirat D, Punie K, Oliveira M, et al. Final results from the randomized phase III ASCENT clinical trial in metastatic triple-negative breast cancer and association of outcomes by human epidermal growth factor receptor 2 and trophoblast cell surface antigen 2 expression. J Clin Oncol. (2024) 42:1738–44. doi: 10.1200/JCO.23.01409
49. Bardia A, Mayer IA, Vahdat LT, Tolaney SM, Isakoff SJ, Diamond JR, et al. Sacituzumab govitecan-hziy in refractory metastatic triple-negative breast cancer. New Engl J Med. (2019) 380:741–51. doi: 10.1056/NEJMoa1814213
50. Cubas R, Li M, Chen C, Yao Q. Trop2: A possible therapeutic target for late stage epithelial carcinomas. Biochim Biophys Acta - Rev Cancer. (2009) 1796:309–14. doi: 10.1016/j.bbcan.2009.08.001
51. D’Arienzo A, Verrazzo A, Pagliuca M, Napolitano F, Parola S, Viggiani M, et al. Toxicity profile of antibody-drug conjugates in breast cancer: practical considerations. eClinicalMedicine. (2023) 62:102113. doi: 10.1016/j.eclinm.2023.102113
52. Dum D, Taherpour N, Menz A, Höflmayer D, Völkel C, Hinsch A, et al. Trophoblast cell surface antigen 2 expression in human tumors: A tissue microarray study on 18,563 tumors. Pathobiology. (2022) 89:245–58. doi: 10.1159/000522206
53. Kong D, Hughes CJ, Ford HL. Cellular plasticity in breast cancer progression and therapy. Front Mol Biosci. (2020) 7. doi: 10.3389/fmolb.2020.00072
54. Liu H, Shi J, Lin F. The potential diagnostic utility of TROP-2 in thyroid neoplasms. Appl Immunohistochem Mol Morphol. (2017) 25(8):525–33. (2016). doi: 10.1097/PAI.0000000000000332
55. Lin JC, Wu YY, Wu JY, Lin TC, Wu CT, Chang YL, et al. TROP2 is epigenetically inactivated and modulates IGF-1R signaling in lung adenocarcinoma. EMBO Mol Med. (2012) 4:472–85. doi: 10.1002/emmm.201200222
56. Hsu EC, Rice MA, Bermudez A, Jose F, Marques G, Aslan M, et al. Trop2 is a driver of metastatic prostate cancer with neuroendocrine phenotype via PARP1. Proc Natl Acad Sci USA. (2020) 117(4):2032–42. doi: 10.1073/pnas.1905384117
57. Izci H, Punie K, Waumans L, Laenen A, Wildiers H, Verdoodt F, et al. Correlation of TROP-2 expression with clinical–pathological characteristics and outcome in triple-negative breast cancer. Sci Rep. (2022) 12(1):22498. doi: 10.1038/s41598-022-27093-y
58. Redlich N, Robinson AM, Nickel KP, Stein AP, Wheeler DL, Adkins DR, et al. Anti-Trop2 blockade enhances the therapeutic efficacy of ErbB3 inhibition in head and neck squamous cell carcinoma. Cell Death Dis. (2018) 9(1):5. doi: 10.1038/s41419-017-0029-0
59. Bessede A, Peyraud F, Besse B, Cousin S, Cabart M, Chomy F, et al. TROP2 is associated with primary resistance to immune checkpoint inhibition in patients with advanced non-small cell lung cancer. Clin Cancer Res. (2024) 30:779–85. doi: 10.1158/1078-0432.CCR-23-2566
60. de Man FM, Goey AKL, van Schaik RHN, Mathijssen RHJ, Bins S. Individualization of irinotecan treatment: A review of pharmacokinetics, pharmacodynamics, and pharmacogenetics. Clin Pharmacokinet. (2018) 57:1229–54. doi: 10.1007/s40262-018-0644-7
61. Cardillo TM, Govindan SV, Sharkey RM, Trisal P, Goldenberg DM. Humanized anti-trop-2 IgG-SN-38 conjugate for effective treatment of diverse epithelial cancers: Preclinical studies in human cancer xenograft models and monkeys. Clin Cancer Res. (2011) 17:3157–69. doi: 10.1158/1078-0432.CCR-10-2939
62. Cardillo TM, Govindan SV, Sharkey RM, Trisal P, Arrojo R, Liu D, et al. Sacituzumab govitecan (IMMU-132), an Anti-Trop-2/SN-38 antibody-drug conjugate: Characterization and efficacy in pancreatic, gastric, and other cancers. Bioconjug Chem. (2015) 26:919–31. doi: 10.1021/acs.bioconjchem.5b00223
63. Sharkey RM, McBride WJ, Cardillo TM, Govindan SV, Wang Y, Rossi EA, et al. Enhanced delivery of SN-38 to human tumor xenografts with an anti-Trop-2-SN-38 antibody conjugate (sacituzumab govitecan). Clin Cancer Res. (2015) 21:5131–8. doi: 10.1158/1078-0432.CCR-15-0670
64. Wu M, Huang W, Yang N, Liu Y. Learn from antibody–drug conjugates: consideration in the future construction of peptide-drug conjugates for cancer therapy. Exp Hematol Oncol. (2022) 11:1–18. doi: 10.1186/s40164-022-00347-1
65. Staudacher AH, Brown MP. Antibody drug conjugates and bystander killing: is antigen-dependent internalization required. Br J Cancer. (2017) 117:1736–42. doi: 10.1038/bjc.2017.367
66. Kopp A, Hofsess S, Cardillo TM, Govindan SV, Donnell J, Thurber GM. Antibody-drug conjugate sacituzumab govitecan drives efficient tissue penetration and rapid intracellular drug release. Mol Cancer Ther. (2023) 22:102–11. doi: 10.1158/1535-7163.MCT-22-0375
67. Zeybek B, Manzano A, Bianchi A, Bonazzoli E, Bellone S, Buza N, et al. Cervical carcinomas that overexpress human trophoblast cell-surface marker (Trop-2) are highly sensitive to the antibody-drug conjugate sacituzumab govitecan. Sci Rep. (2020) 10:2–11. doi: 10.1038/s41598-020-58009-3
68. Perrone E, Lopez S, Zeybek B, Bellone S, Bonazzoli E, Pelligra S, et al. Preclinical activity of sacituzumab govitecan, an antibody-drug conjugate targeting trophoblast cell-surface antigen 2 (Trop-2) linked to the active metabolite of irinotecan (SN-38), in ovarian cancer. Front Oncol. (2020) 10:1–11. doi: 10.3389/fonc.2020.00118
69. Goldenberg DM, Stein R, Sharkey RM. The emergence of trophoblast cell-surface antigen 2 (TROP-2) as a novel cancer target. Oncotarget. (2018) 9:28989–9006. doi: 10.18632/oncotarget.v9i48
70. Zhu Z, Shen G, Li J, Qiu T, Fang Q, Zheng Y, et al. Incidence of antibody–drug conjugates-related pneumonitis in patients with solid tumors: A systematic review and meta-analysis. Crit Rev Oncol Hematol. (2023) 184:103960. doi: 10.1016/j.critrevonc.2023.103960
71. Bardia A, Mayer IA, Diamond JR, Moroose RL, Isakoff SJ, Starodub AN, et al. Efficacy and safety of anti-trop-2 antibody drug conjugate sacituzumab govitecan (IMMU-132) in heavily pretreated patients with metastatic triple-negative breast cancer. J Clin Oncol. (2017) 2017:2141–8. doi: 10.1200/JCO.2016.70.8297.2017.2.test
72. Bardia A, Hurvitz SA, Tolaney SM, Loirat D, Punie K, Oliveira M, et al. Sacituzumab govitecan in metastatic triple-negative breast cancer. New Engl J Med. (2021) 384:1529–41. doi: 10.1056/NEJMoa2028485
73. Rugo HS, Bardia A, Tolaney SM, Arteaga C, Cortes J, Sohn J, et al. TROPiCS-02: A Phase III study investigating sacituzumab govitecan in the treatment of HR+/HER2-metastatic breast cancer. Future Oncol. (2020) 16:705–12. doi: 10.2217/fon-2020-0163
74. Loriot Y, Petrylak DP, Rezazadeh Kalebasty A, Fléchon A, Jain RK, Gupta S, et al. TROPHY-U-01, a phase II open-label study of sacituzumab govitecan in patients with metastatic urothelial carcinoma progressing after platinum-based chemotherapy and checkpoint inhibitors: updated safety and efficacy outcomes. Ann Oncol. (2024) 35:392–401. doi: 10.1016/j.annonc.2024.01.002
75. Loibl S, Loirat D, Tolaney SM, Punie K, Oliveira M, Rugo HS, et al. Health-related quality of life in the phase III ASCENT trial of sacituzumab govitecan versus standard chemotherapy in metastatic triple-negative breast cancer. Eur J Cancer. (2023) 178:23–33. doi: 10.1016/j.ejca.2022.10.003
76. Rugo HS, Bardia A, Marmé F, Cortés J, Schmid P, Loirat D, et al. Overall survival with sacituzumab govitecan in hormone receptor-positive and human epidermal growth factor receptor 2-negative metastatic breast cancer (TROPiCS-02): a randomized, open-label, multicenter, phase 3 trial. Lancet. (2023) 402:1423–33. doi: 10.1016/S0140-6736(23)01245-X
77. Reinisch M, Bruzas S, Spoenlein J, Shenoy S, Traut A, Harrach H, et al. Safety and effectiveness of sacituzumab govitecan in patients with metastatic triple-negative breast cancer in real-world settings: first observations from an interdisciplinary breast cancer center in Germany. Ther Adv Med Oncol. (2023) 15:259–61. doi: 10.1177/17588359231200454
78. Hanna D, Merrick S, Ghose A, Devlin MJ, Yang DD, Phillips E, et al. Real world study of sacituzumab govitecan in metastatic triple-negative breast cancer in the United Kingdom. Br J Cancer. (2024) 130(12):1916–20. doi: 10.1038/s41416-024-02685-9
79. Gui X, Zhao J, Ding L, Chai J, Lai H, Cai Y, et al. Assessing real-world safety concerns of Sacituzumab govitecan: a disproportionality analysis using spontaneous reports in the FDA adverse event reporting system. Front Oncol. (2023) 13:1–14. doi: 10.3389/fonc.2023.1276976
80. Spring LM, Tolaney SM, Fell G, Bossuyt V, Abelman RO, Wu B, et al. Response-guided neoadjuvant sacituzumab govitecan for localized triple-negative breast cancer: results from the NeoSTAR trial. Ann Oncol. (2024) 35:293–301. doi: 10.1016/j.annonc.2023.11.018
81. Bardia A, Tolaney SM, Punie K, Loirat D, Oliveira M, Kalinsky K, et al. Biomarker analyses in the phase III ASCENT study of sacituzumab govitecan versus chemotherapy in patients with metastatic triple-negative breast cancer. Ann Oncol. (2021) 32:1148–56. doi: 10.1016/j.annonc.2021.06.002
82. Zhu J, Wu W, Togashi Y, Taira Nihira N, Johmura Y, Zhu D, et al. Alteration of Trop-2 expression in breast cancer cells by clinically used therapeutic agents and acquired tamoxifen resistance. Breast Cancer. (2022) 29:1076–87. doi: 10.1007/s12282-022-01389-3
83. Koltai T, Fliegel L. The relationship between trop-2, chemotherapeutic drugs, and chemoresistance. Int J Mol Sci. (2024) 25(1):87. doi: 10.3390/ijms25010087
84. Ibragimova KIE, Geurts SME, Laczkó D, Meegdes M, Erdkamp F, Heijns JB, et al. Trastuzumab resistance in patients with HER2-positive advanced breast cancer: results from the SONABRE registry. Clin Breast Cancer. (2024) 24:103–11. doi: 10.1016/j.clbc.2023.10.009
85. Hunter FW, Barker HR, Lipert B, Rothé F, Gebhart G, Piccart-Gebhart MJ, et al. Mechanisms of resistance to trastuzumab emtansine (T-DM1) in HER2-positive breast cancer. Br J Cancer. (2020) 122:603–12. doi: 10.1038/s41416-019-0635-y
86. Wang ZH, Zheng ZQ, Jia S, Liu SN, Xiao XF, Chen GY, et al. Trastuzumab resistance in HER2-positive breast cancer: Mechanisms, emerging biomarkers and targeting agents. Front Oncol. (2022) 12:1–9. doi: 10.3389/fonc.2022.1006429
87. Xu Y, Villalona-Calero MA. Irinotecan: Mechanisms of tumor resistance and novel strategies for modulating its activity. Ann Oncol. (2002) 13:1841–51. doi: 10.1093/annonc/mdf337
88. Coates JT, Sun S, Leshchiner I, Thimmiah N, Martin EE, McLoughlin D, et al. Parallel genomic alterations of antigen and payload targets mediate polyclonal acquired clinical resistance to sacituzumab govitecan in triple-negative breast cancer. Cancer Discovery. (2021) 11:2436–45. doi: 10.1158/2159-8290.CD-21-0702
89. Dumontet C, Reichert JM, Senter PD, Lambert JM, Beck A. Antibody–drug conjugates come of age in oncology. Nat Rev Drug Discovery. (2023) 22:641–61. doi: 10.1038/s41573-023-00709-2
Keywords: triple negative breast cancer (TNBC), sacituzumab govitecan (IMMU-132), antibody-drug conjugate (ADC), Trop-2, immunotherapy, target therapeutics, metastatic TNBC
Citation: Rossi V, Turati A, Rosato A and Carpanese D (2024) Sacituzumab govitecan in triple-negative breast cancer: from bench to bedside, and back. Front. Immunol. 15:1447280. doi: 10.3389/fimmu.2024.1447280
Received: 11 June 2024; Accepted: 26 July 2024;
Published: 15 August 2024.
Edited by:
Lisheng Wang, University of Ottawa, CanadaReviewed by:
Saverio Alberti, University of Messina, ItalyCopyright © 2024 Rossi, Turati, Rosato and Carpanese. This is an open-access article distributed under the terms of the Creative Commons Attribution License (CC BY). The use, distribution or reproduction in other forums is permitted, provided the original author(s) and the copyright owner(s) are credited and that the original publication in this journal is cited, in accordance with accepted academic practice. No use, distribution or reproduction is permitted which does not comply with these terms.
*Correspondence: Debora Carpanese, ZGVib3JhLmNhcnBhbmVzZUBpb3YudmVuZXRvLml0
†These authors have contributed equally to this work and share first authorship
‡These authors have contributed equally to this work and share last authorship