- 1Department of Biology, Concordia University, Montréal, QC, Canada
- 2Department of Health, Kinesiology and Applied Physiology, Concordia University, Montréal QC, Canada
- 3Department of Electrical and Computer Engineering, Concordia University, Center of Applied Synthetic Biology, Montréal, QC, Canada
Introduction: Adrenergic receptors regulate metabolic, cardiovascular, and immunological functions in response to the sympathetic nervous system. The effect of β2-adrenergic receptor (AR) as a high expression receptor on different subpopulations of T cells is complex and varies depending on the type of ligand and context. While traditional β2-AR agonists generally suppress T cells, they potentially enhance IL-17A production by Th17 cells. The effects of pharmacological drugs that count as biased agonists of AR like nebivolol are not completely understood. We investigated the impact of nebivolol on human memory CD4+ T (Th1, Th2, Th17) cells and polarized naive Th17 cells, highlighting its potential for IL-17A suppression via a non-canonical β2-AR cell signaling pathway.
Methods: The effects of nebivolol were tested on healthy human peripheral blood mononuclear cells, purified memory Th cells, and polarized naive Th17 cells activated with anti-CD3/anti-CD28/anti-CD2 ImmunoCult reagent. IFN-γ, IL-4, and IL-17A, which are primarily derived from Th1, Th2, and Th17 cells, respectively, were quantified by ELISA and flow cytometry. IL-10 was measured by ELISA. Gene expression of RORC, ADRB1, ADRB2, and ADRB3 was evaluated by qPCR. The ADRB2 gene was knocked out in memory Th cells using CRISPR/Cas9. Protein expression of phosphorylated serine133-CREB and phosphorylated NF-κB p65 was assessed by Western blot. Proliferation was assessed by fluorescent dye loading and flow cytometry.
Results: Nebivolol treatment decreased IL-17A and IFN-γ secretion by activated memory Th cells and elevated IL-4 levels. Nebivolol reduced the proportion of IL-17A+ Th cells and downregulated RORC expression. Unlike the β2-AR agonist terbutaline, nebivolol inhibited the shift of naive CD4+ T cells toward the Th17 phenotype. IL-10 and the proliferation index remained unchanged. Nebivolol-treated β2-knockout memory Th cells showed significant inhibition of β2-AR-mediated signaling, evidenced by the absence of IL-17A suppression compared to controls. Phosphorylation of the NF-κB p65 subunit was inhibited by nebivolol, but CREB phosphorylation was not changed, suggesting a selective transcriptional control.
Conclusions: The findings demonstrate that nebivolol acts through a β2-AR-mediated signaling pathway, as a distinctive anti-inflammatory agent capable of selectively shifting Th17 cells and suppressing the phosphorylation of NF-κB. This highlights nebivolol’s potential for therapeutic interventions in chronic autoimmune conditions with elevated IL-17A levels.
1 Introduction
The sympathetic nervous system (SNS) is best known for its role in the fight or flight response where the catecholamines epinephrine and norepinephrine (NE) modulate metabolic, cardiovascular, and immune systems (1–3). Complex interactions regulate adaptive immune functions through neuroendocrine mechanisms (4). In the spleen and lymph nodes, sympathetic nerve fibers run alongside the location where T cells and dendritic cells (DCs) reside, indicating the potential neural modulation of T-cell functions (5–7). NE acts on T cells through adrenergic receptors (ARs). Several of the AR family members are classical G protein-coupled receptors (GPCRs). Upon stimulation, β2-AR initiates a canonical pathway involving Gs proteins, leading to adenylyl cyclase (AC) activation, an increase in cAMP production, and phosphorylation of cAMP response element-binding protein (CREB) on Ser133 by protein kinase A (PKA)(8, 9). These processes modulate T cell activation, proliferation, and production of certain cytokines including IL-2 and IFN-γ (10, 11). Receptor desensitization and signal termination occur through phosphorylation by regulatory kinases, such as GPCR kinases (GRKs), followed by β-arrestin 1 activation at Ser412 (8, 12). β2-ARs also engage in non-canonical, G protein-independent signaling via β-arrestin 2 which leads to sustained ERK1/2 activation (13). These pathways could regulate immune responses during chronic sympathetic tone by distinct downstream effects, including modulation of gene expression and inhibition of NF-κB activation (14). NF-κB is widely considered to be a proinflammatory transcription factor that drives the expression of IFN-γ and IL-17A in T cells, and its activity can be measured by assessing the phosphorylation of NF-κB p65 subunit at position Ser529 (15, 16).
Accumulating evidence supports a role for the β2-AR subtype in regulating T cells. Human CD4+ T (T helper) cells and CD8+ T (T cytotoxic) cells exhibit a predominant expression of β2-ARs (17). Memory T cells, crucial for faster and more effective immune responses upon antigen re-exposure, exhibit increased β2-AR expression compared to naive T cells, suggesting heightened responsiveness to immune and gene expression changes induced by the SNS (18, 19). T helper 1 (Th1) cells produce IFN-γ and express the transcription factor TBX21; Th17 cells produce IL-17A, among other cytokines, and express the transcription factor RORγ (RORC); and Th2 cells express IL-4, IL-5, and the transcription factor GATA3 (20). Activation of naive CD4+ T cells in the presence of NE and IL-12 in vivo induced more IFN-γ and polarized the response toward Th1 (21). β2-ARs are also expressed on dendritic cells, and NE treatment reduced IL-12 secretion, altering the IL-12/IL-23 balance. As a result, stimulated DCs promoted T helper cells to produce high IL-17A and lower IFN-γ (22). Consistent findings were observed by stimulating β2-AR with salbutamol in murine bone marrow-derived DC, which secreted IL-6 and IL-23, and upregulated the Th17 response (23). Similarly, salbutamol-treated DCs reduced the secretion of Th2 cytokine IL-4 (24). Our reports demonstrated that a specific β2-agonist drug (terbutaline) significantly increased IL-17A levels in a PKA-dependent manner in human memory Th cells (25, 26). Therefore, agonists of the β2-ARs may shift the balance of Th1, Th2, and Th17 cells by promoting a Th1 and/or Th17 bias in memory Th cells. Furthermore, they may alter the polarization of naive T cells as they shift to Th17 effector cells.
Emerging studies on β2 biased agonist drugs showed anti-inflammatory properties (27) by increasing the serum levels of IL-10, which is mainly produced by regulatory T cells (Tregs) (28), and inhibiting IL-2 production in activated human T cells (29). Biased agonists are ligands that preferentially activate one pathway over another, leading to distinct signaling outcomes compared to traditional agonists. Nebivolol is a third-generation β-blocker that is highly selective for β1-AR (30). Moreover, it can induce nitric oxide through the β3-AR (31). Nebivolol has a prominent pharmacological mechanism involving a β-arrestin biased agonist at the β2-AR (32), and it is FDA-approved to treat hypertension and heart failure (33, 34), but its impact on the immune system is not completely understood. We tested nebivolol on human memory Th1, Th2, Th17, and IL-10 and differentiated naive Th cells into Th17 cells, to determine if it shifted the immune response toward an anti-inflammatory response through the β2-AR cell signaling pathways.
2 Materials and methods
2.1 Isolation of human peripheral blood mononuclear cells
Blood was collected from healthy participants after an informed, signed consent had been provided. The Concordia University Human Research Ethics Committee, which follows the Declaration of Helsinki guidelines, approved the study (certificate 30009292). Health was determined by self-reporting during a semistructured interview. The exclusion criteria included those under 18 years of age, those having specific medical conditions, or those taking ineligible medications. If a participant took recreational drugs or was vaccinated in the past 2 weeks, their blood draw was rescheduled. PBS (1×) was used to dilute heparinized peripheral blood at a 1:1 v/v. In 50-ml conical tubes, 30 ml of diluted blood was layered over 15 ml of lymphocyte separation solution (Wisent Bioproducts, CA). The sample was centrifuged at 624×g for 30 min at room temperature (RT). In a separate 50-ml tube, mononuclear cells were collected and centrifuged at 433×g for 15 min with 25 ml of PBS. The supernatant was discarded, and the pellet was resuspended in 25 ml of PBS and centrifuged at 400×g for 12 min again. In order to isolate the T cells from the cell pellet, PBS containing 2% heat-inactivated fetal bovine serum (FBS) was used as a resuspension solution.
2.2 Isolation and purification check of CD4+ naive and memory T cells
Naive (CD3+CD4+CD45RA+CD45RO−) and memory (CD3+CD4+CD45RA−CD45RO+) T cells were isolated from peripheral blood mononuclear cells (PBMCs) in the recommended medium (PBS containing 2% FBS and 1 mM of EDTA) by using EasySep® Human Naive CD4+ T Cell Isolation Kit II and human memory CD4+ T-cell enrichment kit, respectively (StemCell Technologies, Vancouver, Canada), according to the manufacturer’s instructions. Purity was analyzed by flow cytometry (FACSVerse, BD Biosciences, CA) using anti-CD3-Peridinin-Chlorophyll-Cyanine5.5 (BD Biosciences, clone OKT3), anti-CD4-allophycocyanin (BD Biosciences, clone RPA-t4), anti-CD45RA-fluorescein isothiocyanate (BD Biosciences, clone HI100), and anti-CD45RO-phycoerythrin (BD Biosciences, clone UCHL1) mAbs. Purity was found to be >90% (Supplementary Figures).
2.3 Stimulation, culture, and drug treatment of memory Th cells
Purified memory Th cells were suspended in RPMI 1640 medium (Wisent Bioproducts) containing 10% heat-inactivated FBS, 1% penicillin and streptomycin, and 1% Glutaplus (Wisent Bioproducts) at the final concentration of 0.3 × 106 cells/ml and stimulated with ImmunoCult™ Human CD2/CD3/CD28 T-cell activator (StemCell Technologies). In the next step, nebivolol hydrochloride (Sigma-Aldrich, CA, Vancouver, Canada) dissolved in glycerol was added to the purified memory Th cells and incubated for 5 days in 96-well U-bottom plates at a final concentration of 10 μM. Since nebivolol was dissolved in glycerol, we included vehicle control groups with only glycerol added at an equivalent dilution. The activated memory Th cells were also treated with another β2-biased agonist carvedilol (10 μM, Sigma-Aldrich, CA) or combined with a selective β1-AR antagonist metoprolol tartrate (10 μM, Sigma-Aldrich, CA) or a non-selective β-AR antagonists bupranolol (10 μM, Cayman Chemical, CA) and H89 as a PKA inhibitor (2 μM, Sigma-Aldrich, CA) 30 min before adding the activator.
2.4 In vitro Th17 cell polarization and treatment of CD4+ naive T cells
Naive CD4+ T cells were cultured at 0.3 × 106 cells/well in X-VIVO™ 15 serum-free hematopoietic cell medium (Lonza, USA). Cells were expanded with ImmunoCult™ human CD2/CD3/CD28 T-cell activator (StemCell Technologies, Vancouver, Canada). Four types of cultures were established: 1) non-polarizing conditions as a control; 2) Th17-polarizing conditions in the presence of human recombinant TGF-β (1 ng/ml; Cedarlane, CA), human recombinant IL-21 (25 ng/ml; Cedarlane, CA), human recombinant IL-23 (25 ng/ml; Cedarlane, CA), anti-IL-4 (10 μg/ml; Abcepta, CA), and anti-IFN-γ (10 μg/ml; Abcepta, CA); 3) Th17-polarizing conditions as above plus 10 μM of nebivolol; and 4) Th17-polarizing conditions with 10 μM of terbutaline. After 7 days of culture, the following procedures were performed.
2.5 Cytokine analysis using ELISA
The culture supernatants of either PBMCs, memory Th cells, or polarized Th17 cells were collected after incubation by centrifugation of the 96-well culture plate at 100×g for 6 min to remove any debris. The supernatant was analyzed for IL-4, IFN-γ, IL-10 (BD Biosciences), and IL-17A (Thermo Fisher Scientific, Burlington, Canada) with ELISA kits following the manufacturer’s instructions. A standard curve was generated for each assay, with limits of detection of 500 pg/ml for IL-17A, IL-4, and IL-10 and 300 pg/ml for IFN-γ. The supernatants were diluted accordingly with assay diluent. Two technical ELISA replicates of the standard curve and each experimental group were done. Data were acquired on a spectrophotometer at 450 nm with a 570 nm correction factor (BioTek Agilent, Santa Clara, USA, Fisher Scientific).
2.6 Flow cytometry
Intracellular IL-17A and IFN-γ were measured using established intracellular cytokine staining (ICS) procedures (35). In brief, after the cell culture was complete, the cells were incubated with 0.5 μg/ml of ionomycin, 10 μg/ml of brefeldin A, and 0.05 μg/ml of phorbol 12-myristate 13-acetate (Sigma-Aldrich Millipore, Oakville, Canada) for four hours at 37°C with 5% CO2. The cells were fixed and permeabilized with a kit following the manufacturer’s instructions (BD Biosciences). Cells were incubated with saturating concentrations of fluorochrome-labeled Abs. The antibodies were CD4-allophycocyanin (BD Biosciences, clone RPA-t4), IL-17A-phycoerythrin (R&D Systems, Minneapolis, USA, clone 41802), INF-γ-Brilliant Violet 421 (BD Biosciences, clone B27), and IL-4-phycoerythrin-Cyanine7 (BD Biosciences, clone 8D4-8). Samples were incubated for 45 min on ice in the dark. Two washes (493×g, 5 min) with a staining buffer (1% FBS in 1× phosphate buffered saline) were done, and the samples were resuspended in a 500-μl staining buffer in microcentrifuge tubes. Each set of runs consisted of three technical replicates of each experimental group. At least 50,000 events were obtained on the flow cytometer (FACSVerse, BD Biosciences) and analyzed with FlowJo software (BD Biosciences).
2.7 Cell proliferation and viability assessment by using CFDASE and 7AAD staining
According to a published protocol (26), the tracking dye 5(6)-carboxyfluorescein diacetate N-succinimidyl ester (CFDASE) was used to measure proliferation. Memory Th cells were labeled with CFDASE, incubated in the specified activation conditions for 5 days, and then stained for a surface marker with CD4-APC. After 30 min, cells were washed and 5 μl of 7AAD-Peridinin-Chlorophyll-Protein reagent (BD Biosciences) was added to the cell suspension and incubated in the dark for 15 min at room temperature. In each group, at least 50,000 cells in three replicates were analyzed with a flow cytometer (BD Biosciences, CA, FACSVerse). The division index, proliferation index, and viability were determined in CD4-gated cells using the cell proliferation tool (BD Biosciences).
2.8 mRNA extraction and quantitative real-time PCR
The qPCR analysis was done according to published protocols (26). Briefly, memory Th cells or polarized naive Th17 cells were cultured with activating and drug conditions. At least 2 × 106 cells were collected from each experimental group, and total RNA was extracted using the PureLink™ RNA Mini Kit (BD Biosciences). The RNA concentration and purity were measured using a spectrophotometer (NanoDrop™ 2000c, Thermo Scientific). The RNA samples were then converted to cDNA using iScript™ Reverse Transcription Supermix for RT-qPCR (Bio-Rad Laboratories, USA). At least two technical replicates of each independent sample were used for real-time quantitative polymerase chain reaction (qPCR) using TaqMan™ gene probes from Thermo Fisher Scientific to measure ADRB1 (Hs02330048), ADRB2 (Hs00240532), ADRB3 (Hs_00609046_m1), RORC (Hs01076112), and the housekeeping gene PPIA (Hs99999904_m1). The housekeeping gene was normalized to the average of its expression and shown as a fold increase in relative expression 2−ΔΔCT. Fold change is calculated as the ratio of the normalized expression level of the target gene in the treated sample to that in the activated control group which is set to 1.
2.9 Knocking out the ADRB2 gene in memory Th cells using CRISPR/Cas9-based gene editing
Memory Th cells were activated at a concentration of 1 × 106 cells/ml using Dynabeads™ Human T-Activator CD3/CD28 (Fisher Scientific Ottawa, ON, #11131D) at a 1:1 cell to bead ratio in RPMI 1640 medium supplemented with 10% FBS, 100 U/ml of penicillin/streptomycin, and 100 IU/ml of recombinant human IL-2 (Fisher Scientific Ottawa, ON). Cells were cultured at 37°C with 5% CO2 for 2 days. After incubation, activator beads were removed by pipetting gently using a magnetic tube rack for 1–2 min to separate cells from the beads. The supernatant containing the cells was transferred to a fresh tube and maintained at 1 × 106 cells/ml with daily addition of complete culture media.
According to published protocols (36, 37), before electroporation briefly, 100 pmol of optimized multi single-guide RNA (sgRNA) (payload sequences attached in Supplementary Table 1) (Synthego, USA) and 50 pmol of Cas9 (Thermo Fisher, CA) per 1 million cells were mixed in 5 μl of Resuspension Buffer R and incubated at room temperature for 10 min to form the Cas9-ribonucleoprotein (RNP) complex. T cells (2 × 106) were washed with PBS, resuspended in Buffer R, and mixed with Cas9/gRNA complex. Electroporation was performed using the Neon™ 100-μl tip and program #24 (1,600 V, 10 ms, 3 pulses). Post-electroporation, the cells were incubated in a recovery buffer containing 10% FBS, 100 U/ml of penicillin/streptomycin, 400 IU/ml of recombinant human IL-2 at 37°C, and 5% CO2 for 72 h. Editing efficiency was assessed using ADRB2 mRNA expression levels via RT-qPCR in non-electroporated, non-targeting gRNA (nt-gRNA), T cell receptor-α constant (TRAC) multi-sgRNA (positive control), and ADRB2 multi-sgRNA electroporated conditions. Then, cells were treated with nebivolol at a final concentration of 10 μM for 5 days to assess the impact of β2-AR gene depletion on IL-17A production.
2.10 Western blot
A published protocol was followed to prepare whole-cell lysates after treatment of activated memory Th cells with nebivolol for 15 min (26). Protein concentrations were determined with a Bradford assay kit (Bio-Rad). For separation by electrophoresis, 15 μg of total protein in two technical replicates of each sample was loaded onto SDS-polyacrylamide gels according to standard protocols (SDS-PAGE) and then transferred to nitrocellulose. Membranes were blocked with 5% (wt/vol) non-fat milk (Anatol Spices, Montreal, Canada) in Tris-buffered saline with 0.1% Tween-20 (TBST) overnight at 4°C and then again incubated overnight at 4°C with recommended dilutions of primary antibodies. Antibodies were against human phospho-ser529 NF-κB p65 (1/500, clone A21012B, BioLegend, San Diego, USA), NF-κB p65 antibody (1/1,000, clone 14G10A21, BioLegend, San Diego, USA), phospho-Ser133 CREB1 (1/700, rabbit polyclonal, Cusabio, Cedarlane), phospho-Ser412-β-arrestin 1 (1/1,000, clone mAb #2416, Cell Signaling Technology, Danvers, USA), β2-AR (1/500, clone 4A6C9, Novusbio, Cedarlane), GAPDH (1/15,000, 10B4E3, MA000071M1m, Cusabio, Cedarlane), and α‐tubulin (1/1,000, Santa Cruz Biotechnology Inc., USA). Subsequently, membranes were repeatedly washed with TBST and incubated for 2 h with the appropriate HRP-conjugated secondary mouse antibody (1/1,500, Bio-Rad Laboratories) or secondary goat anti‐rabbit IgG HRP (1/5,000, Cusabio, Cedarlane) in 5% skim milk. Immunoreactivity was detected using the ECL prime reagent (GE Healthcare, Chicago, USA), and then the chemiluminescence signal was recorded in the Image Lab (Bio-Rad Laboratories). Data were analyzed with Image Lab software (Bio-Rad Laboratories). Total α-tubulin levels were used as a loading control.
2.11 Statistical analysis
Statistical analyses were conducted using ANOVA with Tukey’s multiple comparison tests to assess the effects of treatment and culturing conditions on the studied parameters. All data are presented as mean ± SEM. Statistical significance was determined using p-value thresholds: *p < 0.05, **p < 0.01, ***p < 0.001, and ****p < 0.0001. The number of independent biological experiments and technical replicates for each experimental condition is detailed in the legend of each figure. All analyses were performed using GraphPad Prism 9 software.
3 Results
3.1 Nebivolol promotes an anti-inflammatory shift in T helper cells, effectively suppressing Th1 and Th17 cells
To test the effects of nebivolol on Th1, Th2, and Th17 cytokines, activated human PBMCs were used in cell culture experiments. Nebivolol had a trend of decreasing IFN-γ, showed a trend of increasing IL-4, and significantly decreased IL-17A when compared to the control group without treatment and the vehicle control (Figures 1A–C). In contrast, our findings indicate that carvedilol, another β2 biased agonist, did not significantly alter cytokine production (Figures 1A–C). Measurements from PBMC experiments confirmed that nebivolol at the concentration of 10 μM did not cause cellular cytotoxicity (Figure 1D). In the next step, purified memory CD3+CD4+CD45RA−CD45RO+ T cells were extracted from PBMCs to a high degree of purity (Supplementary Figure). Memory Th cells were activated for 5 days with anti-CD3/anti-CD28/anti-CD2, with or without nebivolol treatment. Nebivolol-treated cells showed a significant decrease in IFN-γ and IL-17A production while increasing IL-4 levels compared to the activated control group without treatment. There was no significant change in IL-10 levels between the treated and untreated groups after activation (Figures 2A–D). To underscore nebivolol’s ability to shift cytokine secretion, intracellular cytokine staining was conducted on memory Th cells (Figures 3A–D). Nebivolol exhibited a decreasing trend in the proportion of IFN-γ+, an increasing trend in the proportion of IL-4+, and a significant decrease in the proportion of IL-17A+ memory Th cells (Figures 3E–G). Despite the changes in cytokines IFN-γ and IL-4, there were no changes in TBX21 and GATA3 as the main transcription factors of Th1 and Th2, respectively, in response to nebivolol (Figures 3H, I). However, the inhibition of IL-17A was concurrent with mRNA levels since nebivolol inhibited the expression of RORC, which is a main transcription factor of Th17 cells (Figure 3J). Together, the data indicate that nebivolol causes an anti-inflammatory shift, suggesting that it inhibits the Th17 development pathway.
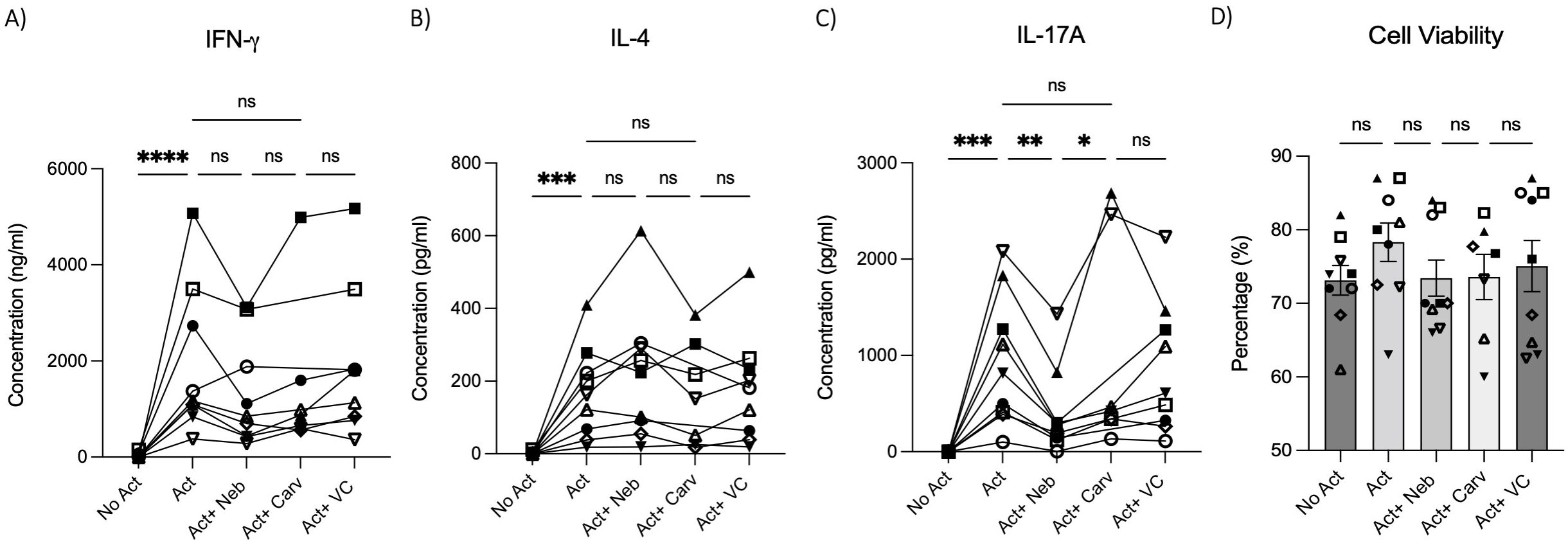
Figure 1. IFN-γ, IL-4, and IL-17A secretion by PBMCs treated with nebivolol or carvedilol. PBMC samples were not activated or activated with anti-CD3/anti-CD28/anti-CD2(Act) for 4 days with nebivolol (Neb) or carvedilol (Carv) in the concentration of 10 μM as compared to the equivalent dilution of vehicle control (VC). The (A) IFN- γ, (B) IL-4, and (C) IL-17A levels in cell culture supernatants were measured by ELISA. (D) The cell viability of the cells was measured by trypan blue. Pooled data are expressed as mean ± SEM of seven to nine independent biological experiments. One-way ANOVA followed by Tukey’s multiple comparison tests was performed. *<0.05, **<0.01, ***p<0.001, and ****p<0.0001. ns, non-significant.
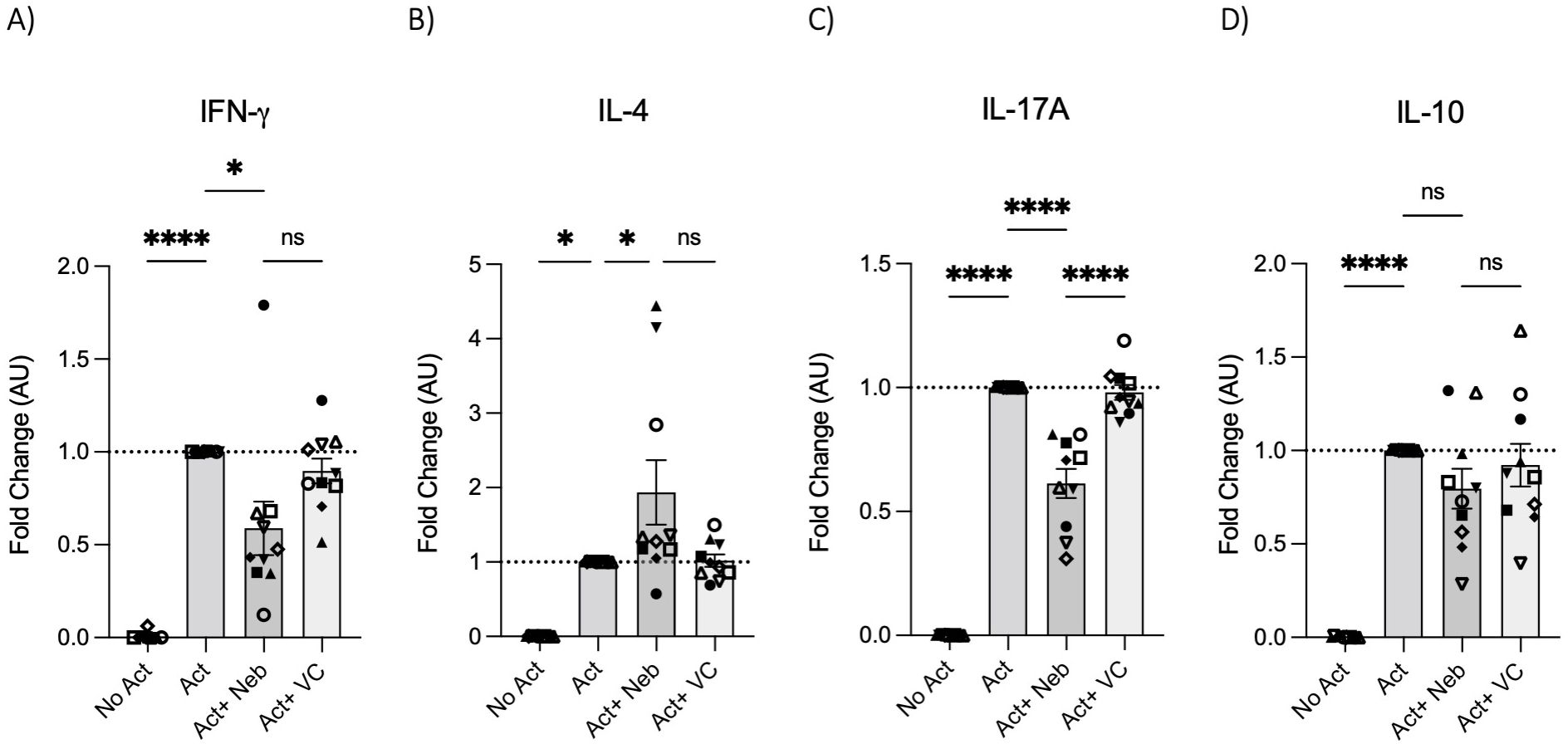
Figure 2. IFN-γ, IL-4, IL-17A, and IL-10 secretion by memory Th cells treated with nebivolol. Memory Th cells were not activated or activated with anti-CD3/anti-CD28/anti-CD2 for 5 days, and the (A) IFN-γ, (B) IL-4, (C) IL-17A, and (D) IL-10 levels in cell culture supernatants were measured by ELISA and expressed as fold change compared to the Act group, which was set to 1.0 (dotted line). Pooled data are expressed as mean ± SEM of 10 independent biological experiments. One-way ANOVA followed by Tukey’s multiple comparison tests. *<0.05 and ****p<0.0001. ns, non-significant.
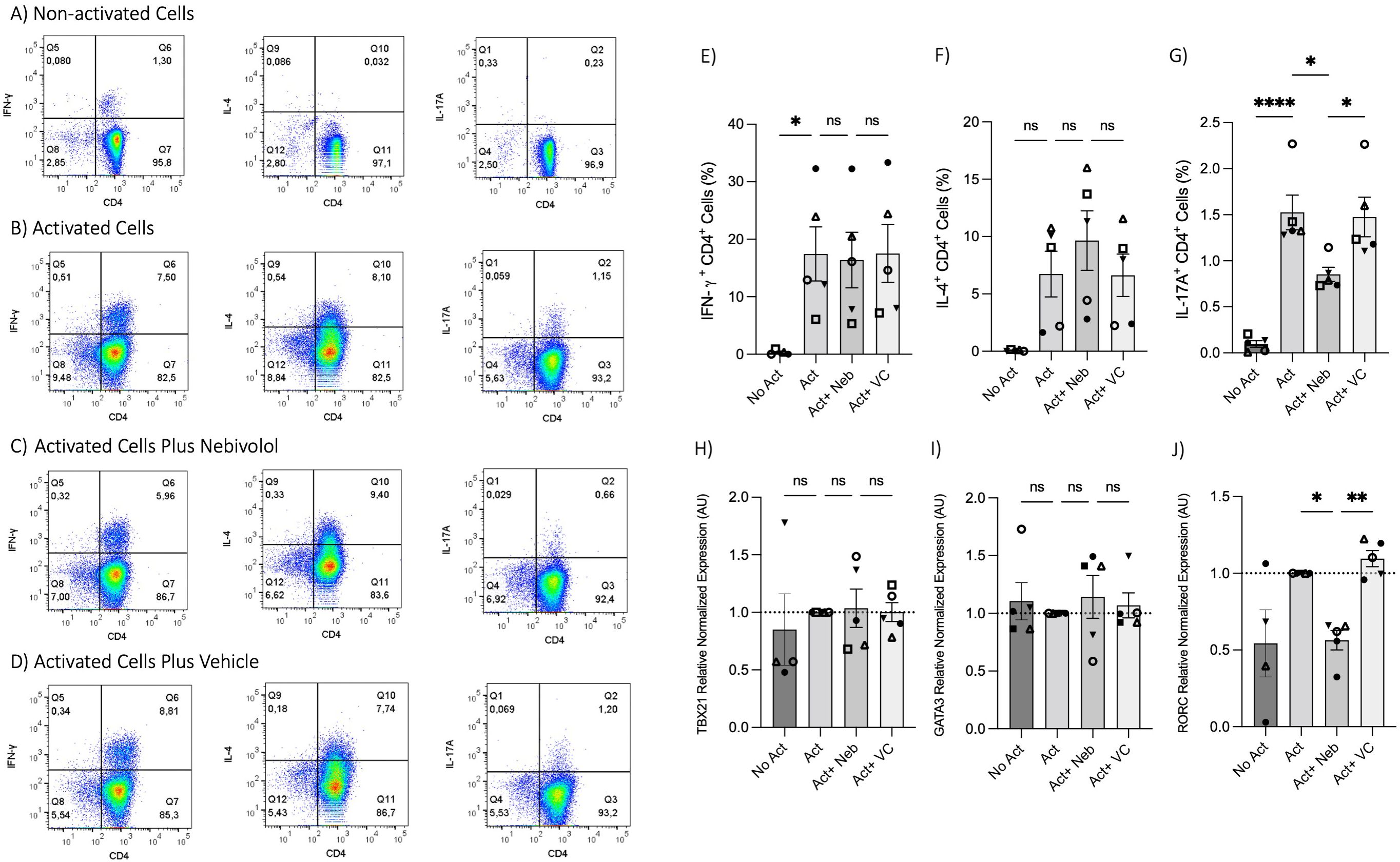
Figure 3. Intracellular cytokine staining and expression of transcription factor genes associated with Th1, Th2, and Th17 in memory Th cells treated with nebivolol. Memory Th cells were not activated or activated with anti-CD3/anti-CD28/anti-CD2 for 5 days, and the samples were stained for intracellular cytokines with or without nebivolol treatment and analyzed by flow cytometry. Representative dot plots are shown for CD4 and each of IFN-γ, IL-4, and IL-17A antibodies on memory Th lymphocytes. (A) Non-activated cells, (B) activated cells, (C) activated cells plus nebivolol, (D) vehicle control. (E) The proportion of memory Th cells expressing IFN-γ is shown as the percentage of IFN-γ+CD4+ T cells. (F) The proportion of CD4+ T cells expressing IL-4 is shown as the percentage of IL-4+CD4+ T cells. (G) The proportion of CD4+ T cells expressing IL-17A is shown as the percentage of IL-17A+CD4+ T cells. Expression of (H) TBX21, (I) GATA3, and (J) RORC in RNA extracted from memory Th cells, shown as the relative amounts normalized to housekeeping RNA and compared to the Act group, which was set to 1.0 (dotted line). Pooled data are expressed as mean ± SEM of five independent biological experiments. One-way ANOVA followed by Tukey’s multiple comparison tests. *<0.05, **<0.01, and ****p<0.0001. ns, non-significant.
3.2 Nebivolol inhibits naive CD4+ T-cell shift toward the Th17 phenotype when compared to terbutaline
To further illustrate the impact of the β2-AR biased agonist on the polarization shift of naive CD4+ T cells in the context of Th17 cell development, we initially examined the expression levels of ADRB1, ADRB2, and ADRB3 in RNA extracted from naive Th cells. Naive T cells expressed ADRB1 and ADRB2; however, ADRB3 was notably absent (Figure 4A). Subsequently, nebivolol or terbutaline was administered to naive CD4+ T cells that were activated with Th17-polarizing cytokines and blocking antibodies. Nebivolol demonstrates a significant reduction in IL-17A levels within cell culture supernatants, accompanied by a suppression of RORC expression in Th17-polarized naive Th cells (Figures 4B, C). Moreover, it effectively decreases the percentage of CD4+IL-17A+ cells (Figures 4D, E). Conversely, terbutaline exhibits an opposing effect, elevating IL-17A levels, enhancing RORC expression, and increasing the proportion of CD4+IL-17A+ cells (Figures 4B–E). These findings confirm that β2-AR activation by a biased agonist hinders the shift of human naive Th cells toward a Th17 phenotype, whereas β2-AR agonist promotes such a shift.
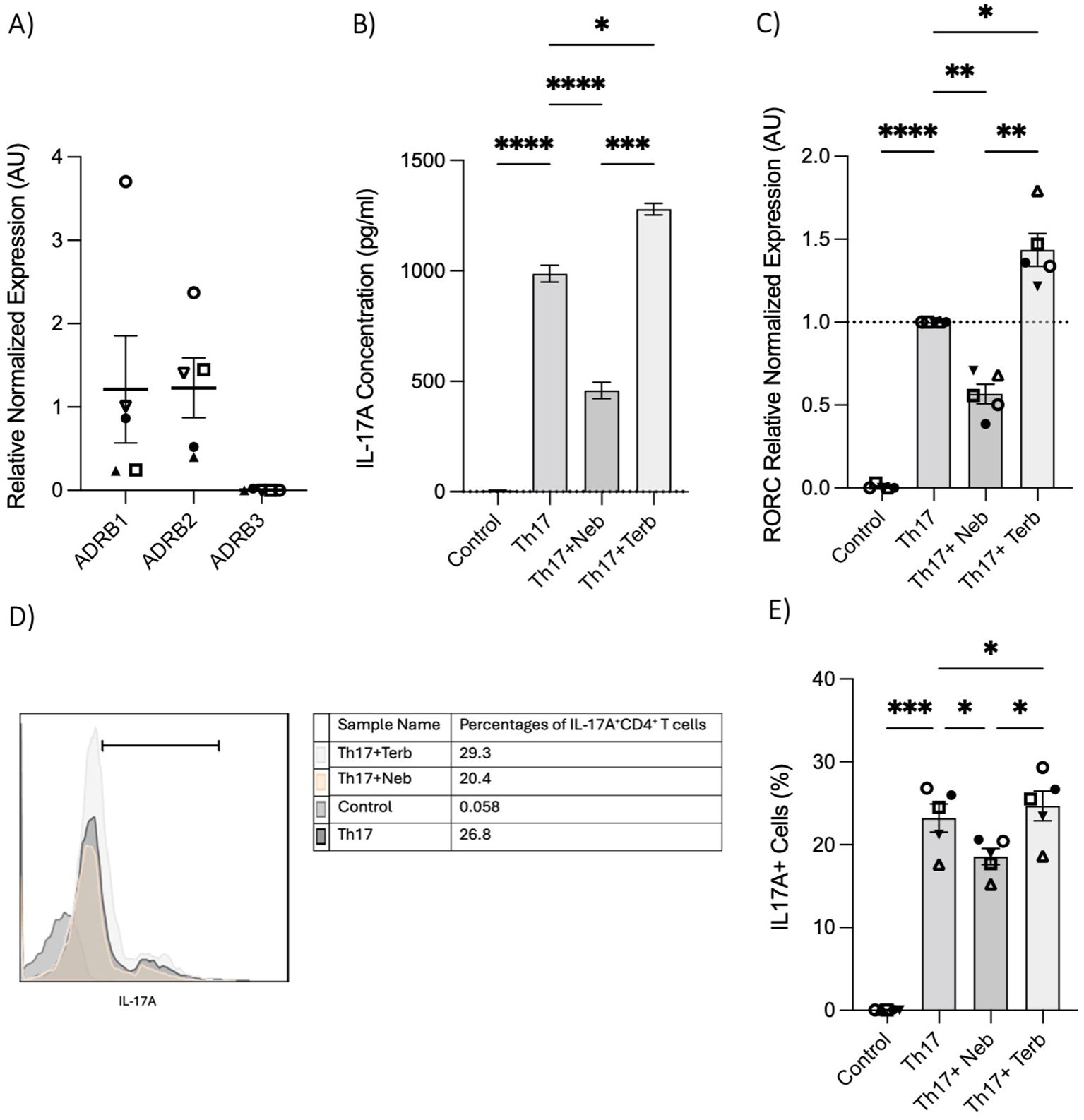
Figure 4. Nebivolol inhibits naive Th cell shift toward the Th17 phenotype, in contrast to terbutaline which augments the shift. (A) Expression of ADRB1–3 in RNA extracted from naive CD4+ T cells shown as the relative amounts normalized to housekeeping RNA. (B) Naive Th cells were activated with polarizing cytokines and blocking antibodies (Th17) with treatment with nebivolol (Neb) or terbutaline (Terb) for 7 days, and a representative of IL-17A levels in cell culture supernatants was shown. (C) RNA expression of the Th17 cell-specific transcriptional factor RORC differentiated Th17 cells shown as the relative amounts normalized to housekeeping RNA and compared to shifted Th17 cells, which was set to 1.0 (dotted line). (D) A representative of overlapping histogram and (E) pooled data of intracellular cytokine staining shown for CD4+IL-17A+ cell percentage in polarized Th17 cells after treatment with nebivolol or terbutaline. Pooled data are expressed as mean ± SEM of five independent biological experiments. One-way ANOVA followed by Tukey’s multiple comparison tests. *<0.05, **<0.01, ***p<0.001, and ****p<0.0001.
3.3 No significant proliferation and viability effects under nebivolol treatment in memory Th cells
The changes in cytokines and percentages suggested that nebivolol could influence proliferation. However, nebivolol did not change the proliferation index and division index of memory Th cells significantly after treatment (Figures 5A–E). Nebivolol did not change cell viability when compared to activation conditions, as measured by the percentage of necrotic or late apoptotic memory CD4+ T cells with 7AAD after 5 days of cell culture (Figure 5F).
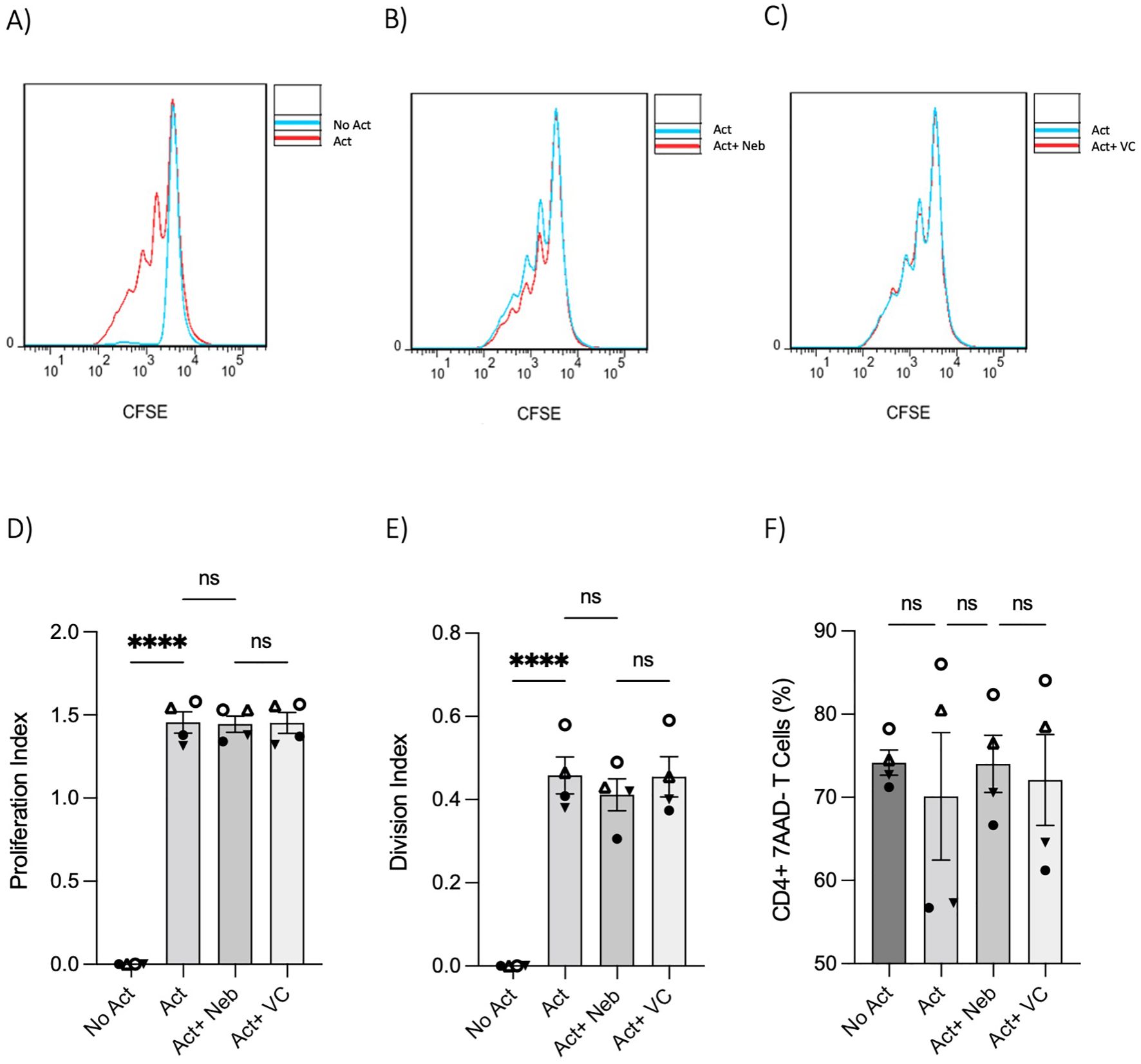
Figure 5. T-cell proliferation and viability were measured by flow cytometry assay. Memory Th cells were not activated or activated with anti-CD3/anti-CD28/anti-CD2 for 5 days. Representative histograms of the proliferation of memory Th cells in cultures with different groups including (A) activated cells overlayed with non-activated cells, (B) activated cells plus nebivolol overlayed with activation-only condition, and (C) activated vehicle control overlayed with activation-only cells. (D) The proliferation index of memory Th cells in culture with activation and nebivolol. (E) The division index of pooled data. (F) The percentage of alive memory CD4+, 7AAD− T cells with or without treatment for 5 days gated on all cells in a dot plot by staining with 7AAD in cultures with different groups. Pooled data are expressed as mean ± SEM of four independent biological experiments. One-way ANOVA followed by Tukey’s multiple comparison tests. ****p<0.0001. ns, non-significant.
3.4 Nebivolol’s effect on IL-17A is β2-AR-dependent
To investigate receptor involvement, we measured the expression of ADRB1, ADRB2, and ADRB3 in memory Th cells. Similar to observations in naive Th cells, the freshly isolated resting memory Th cells expressed ADRB1 and ADRB2, but not ADRB3 (Figure 6A). Next, the expression of ADRB2 in memory Th cells was evaluated after a 5-day activation with or without drug treatment. ADRB2 levels were higher in the non-activated control condition as compared to the activation or activation with nebivolol conditions (Figure 6B). The expression level of the β2AR protein had a trend to be diminished at the 5-day timepoint which resembles the 5-day mRNA expression data (Figures 6C, D).
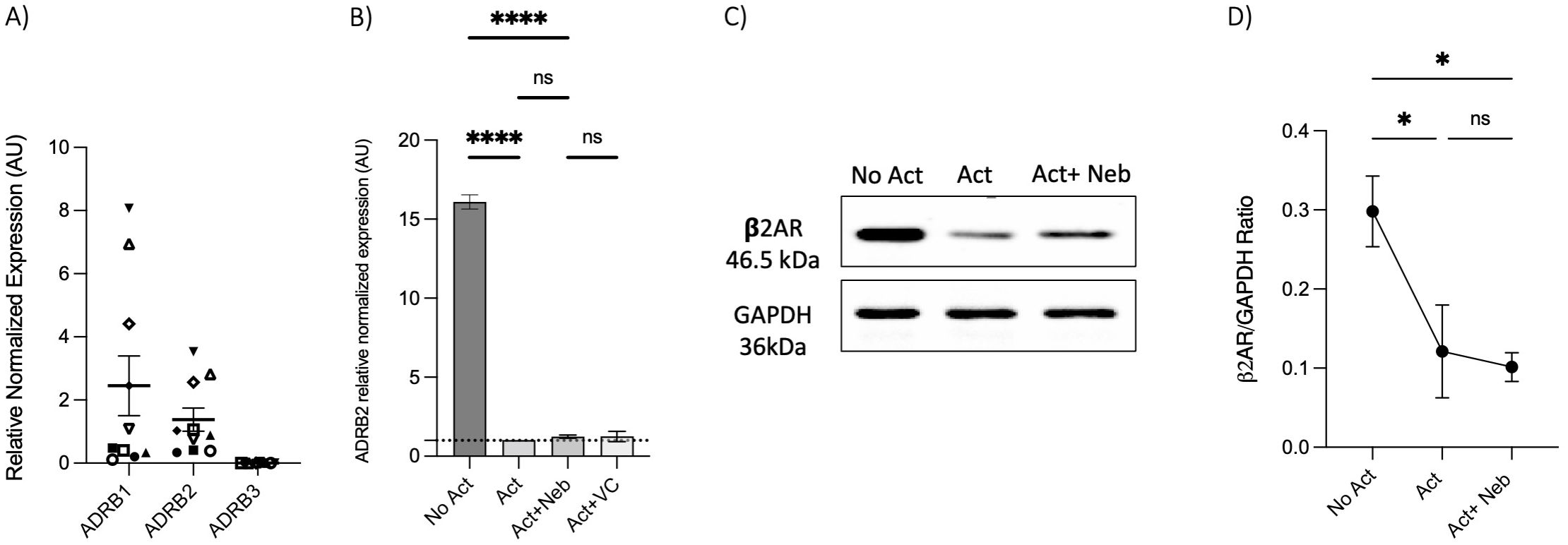
Figure 6. Expression of ADRB1–3 in RNA extracted from enrichment memory Th cells after 5 days with or without treatment. Memory Th cells were not activated or activated with anti-CD3/anti-CD28/anti-CD2 for 5 days. (A) Expression of ADRB1–3 in RNA extracted from enrichment memory Th cells shown as the relative amounts normalized to housekeeping RNA. Pooled data are expressed as mean ± SEM of 10 independent biological experiments. (B) Expression of ADRB2 at mRNA levels from memory Th cells with or without treatment after 5 days of culture, shown as the relative amounts normalized to housekeeping RNA and compared to the Act group. The data of this figure are representative of three experiments. (C) Representative Western blot data of equal amounts of protein from the cell lysates were shown for β2-AR and GAPDH as a loading control. (D) A representative band intensity of five biological experiments was quantified and shown corrected to the loading control as a ratio. The bars show the mean ± SEM. ANOVA followed by Tukey’s multiple comparison tests. *<0.05 and ****p<0.0001. ns, non-significant.
Next, we used a pharmacological approach to block receptors in cell culture experiments. The β1-AR antagonist (metoprolol) and the pan-β1,2,3-AR antagonist (bupranolol) were applied to cell cultures of activated memory Th cells. Metoprolol did not prevent nebivolol from inhibiting IL-17A; in contrast, bupranolol prevented nebivolol from inhibiting IL-17A (Figures 7A, B). To confirm the specific receptor involved in nebivolol’s function, we used electroporation with β2-AR-specific multi-sgRNA which significantly reduced β2-AR mRNA levels compared to the non-targeting and non-electroporated cells (control). The viability of cells before and after electroporation was maintained, and the CRISPR/Cas9-mediated knockout of TRAC as a positive control demonstrated over 90% efficiency in mRNA level reduction (Supplementary Figures). Quantitative RT-PCR analysis revealed a knockout efficiency of approximately 80%, confirming the successful targeting of the β2-AR gene (Figure 7C). Subsequent nebivolol treatment significantly inhibited the β2-AR-mediated signaling pathways in β2-AR knockout memory Th cells, as indicated by the lack of suppression of IL-17A production compared to the control (Figure 7D). These findings suggest that nebivolol acts in a β2-AR-dependent manner, with ADRB3 not being expressed in the cells, ruling it out as a potential ligand for nebivolol.
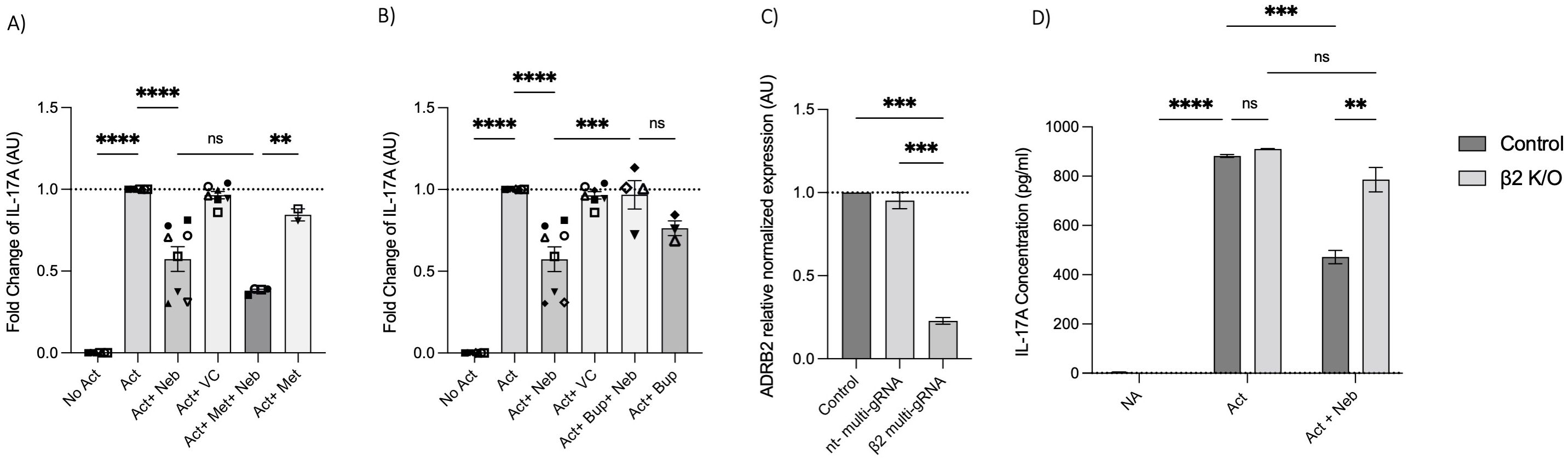
Figure 7. Effect of β-AR antagonists and CRISPR/Cas9-mediated knockout on IL-17A production. Effect of (A) β1-AR antagonist (metoprolol) and (B) non-selective β-AR antagonist (bupranolol) on the production of IL-17A on memory Th cells. Activated memory Th cells were blocked for 30 min with metoprolol or bupranolol before treatment with nebivolol and incubated for 5 days. IL-17A was measured in cell culture supernatants. Pooled data are expressed as the mean ± SEM of four independent biological experiments. Effect of CRISPR/Cas9-mediated knockout of β2AR on nebivolol treatment in activated memory Th cells. Stimulated memory Th cells were electroporated with a CRISPR/Cas9 system targeted by multi-sgRNA against β2-AR or non-targeting genes. (C) The expression of ADRB2 at mRNA levels as verification in non-electroporated, non-targeting and β2 multi-sgRNA conditions has shown as the relative amounts normalized to housekeeping RNA and compared to a control set to 1.0 (dotted line). The data of this figure are representative of three independent biological experiments. Three days after electroporation, memory Th cells were cultured with or without nebivolol for another 5 days and (D) A representative of IL-17A levels in cell culture supernatants in both control and knock-out β2-AR conditions was shown. ANOVA was followed by Tukey’s multiple comparison tests. *<0.05, **<0.01, ***p<0.001, and****p<0.0001. ns, non-significant.
3.5 Under non-canonical signaling, nebivolol does not change CREB phosphorylation but inhibits the phosphorylation of NF-κB p65
As previously mentioned, our findings indicate that the β2-AR agonist terbutaline enhances IL-17A production through a mechanism dependent on PKA and CREB pathways (26). Hypothesizing that nebivolol, acting as a biased agonist, might diverge from this pathway, we investigated its effects on CREB phosphorylation. Memory Th cells were activated and treated with nebivolol or terbutaline. Nebivolol did not change the phosphorylation levels of CREB after 15 min although terbutaline showed a significant increase as was expected (Figures 8A, B). When memory Th cells were co-treated with H89 (a PKA inhibitor) and nebivolol, it did not abrogate the effects of nebivolol on IL-17A (Figure 8C). Thus, the canonical G-protein cAMP–PKA–CREB axis does not appear to be part of nebivolol’s signaling pathway. Given the potential involvement of the NF-κB signaling pathway, we further examined the phosphorylation of NF-κB p65 in activated memory Th cells. Nebivolol inhibited the phosphorylation of the NF-κB p65 subunit at position Ser529 (Figures 9A, B). Moreover, there was no significant change in phosphorylation of the β-arrestin 1-mediated desensitization after 15 min of treatment (Supplementary Figures). Thus, nebivolol exerts its anti-inflammatory shift by suppressing NF-κB activity possibly through an alternative pathway in memory Th cells.
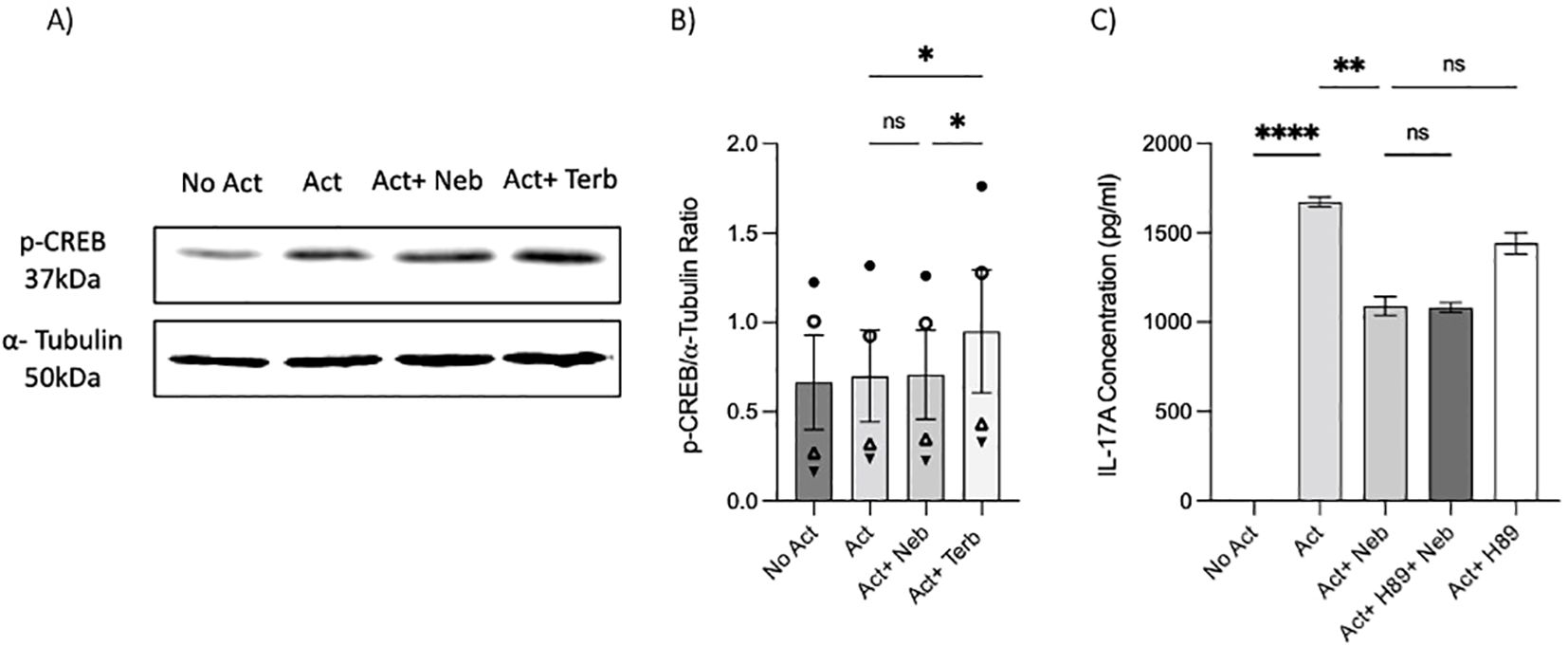
Figure 8. Nebivolol did not stimulate phospho-Ser133-CREB (p-CREB) in memory Th cells. Blasted memory Th cells activated with anti-CD3/anti-CD28/anti-CD2 for 15 min in conditions of non-activated cells, activated cells, activated cells plus nebivolol, and activated cells plus terbutaline. (A) Representative Western blot data of equal amounts of protein from the cell lysates were shown for p-CREB and α-tubulin as a loading control. (B) Band intensity was quantified and shown corrected to the loading control as a ratio, with data pooled from three independent biological experiments. (C) Memory Th cells were activated for 5 days, with nebivolol plus or minus H89. Representative IL-17A ELISA data for the effect of PKA in nebivolol’s signaling pathway by using H89 as a PKA inhibitor. ANOVA was followed by Tukey’s multiple comparison tests. *<0.05, **<0.01, and ****p<0.0001. ns, non-significant.
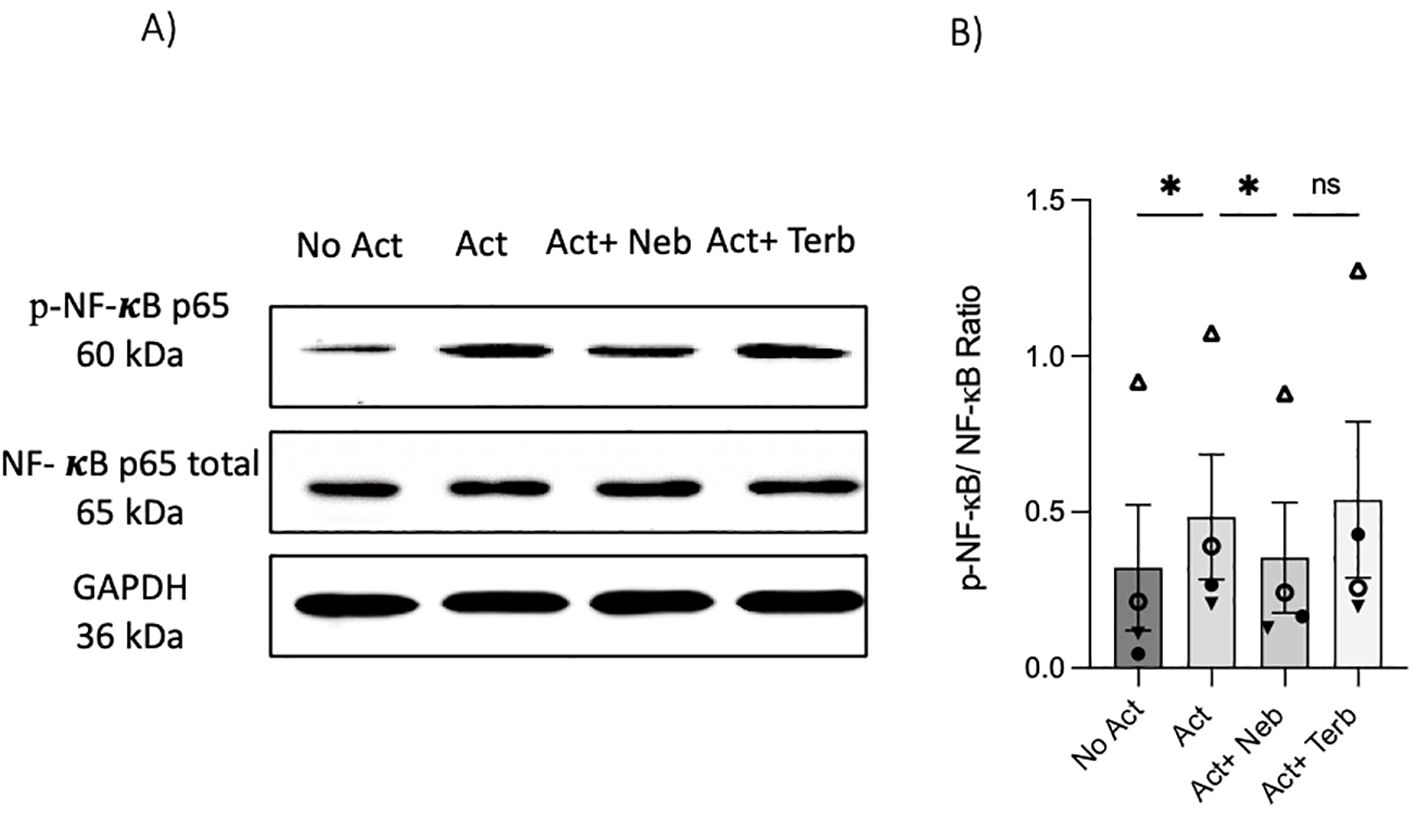
Figure 9. Nebivolol inhibited the phosphorylation of NF-κB p65 in memory Th cells. Blasted memory Th cells were activated with anti-CD3/anti-CD28/anti-CD2 for 15 min in conditions of non-activated cells, activated cells, and activated cells plus nebivolol. (A) Representative Western blot data of equal amounts of protein from the cell lysates were shown for p-NF-κB p65 and total NF-κB p65 as a loading control. (B) Band intensity was quantified and shown corrected to the loading control as a ratio, with data pooled from four independent biological experiments. ANOVA was followed by Tukey’s multiple comparison tests. *<0.05. ns, non-significant.
4 Discussion
T cells express AR and respond to adrenergic ligands, but how this impacts pro- or anti-inflammatory cytokine expression is not completely understood. This study demonstrated that nebivolol exerts a notable inhibitory effect on activated naive and memory Th17 cells, impacting IL-17A secretion levels and the expression of the transcription factor (RORC). The data indicate the involvement of a non-canonical NF-κB signaling pathway.
Our initial experiments on PBMCs revealed that nebivolol exhibits anti-inflammatory effects by reducing IFN-γ and IL-17A levels while inducing IL-4 production. In contrast, carvedilol did not demonstrate these effects, highlighting the biased and drug-specific nature of nebivolol’s immunomodulatory properties. Since PBMCs are a mixed population, we directed further studies on purified memory and naive Th cells. Consistent with an anti-inflammatory profile, nebivolol inhibited IFN-γ and augmented IL-4 in memory Th cells although the proportions of Th1 and Th2 and the respective transcription factors TBX21 and GATA3 did not show significant alterations. This discrepancy between cytokine levels and mRNA expression of transcription factors suggests that potential post-transcriptional or post-translational regulatory mechanisms are at play (38). The drug treatment may influence protein stability, translation efficiency, or cytokine secretion pathways, rather than directly impacting mRNA expression of TBX21 and GATA3 which needs further investigation. When examining the levels of IL-10, there was no significant effect of nebivolol. IL-10 is primarily produced by Tregs (28) and has a general anti-inflammatory profile. This lack of effect may stem from a selective impact on certain T-cell subsets or signaling pathways. Moreover, we did not assess the proportion of Tregs present in memory Th cell preparations. Our findings demonstrated that nebivolol mitigated a shift of naive Th cells toward the Th17 phenotype, whereas terbutaline, acting as a β2-AR agonist, promoted the shift toward Th17 cells. These results imply that nebivolol inhibits Th17 differentiation and function through a distinct mechanism of action, activating β2-AR on CD4+ T cells in a manner different from that of a traditional agonist. Overall, nebivolol has the profile of being anti-inflammatory by lowering Th1 and Th17 while augmenting Th2 cell responses.
The study further probed the expression patterns of ADRB1–3 in isolated naive and memory human Th cells, shedding light on the receptors’ differential presence in these subsets. Naive and memory Th cells were devoid of ADRB3 but expressed ADRB1 and ADRB2. These findings are novel since previous research reported that immune cells express β2-AR predominantly; however, memory Th cells were not assessed previously (39). Given nebivolol’s higher affinity for β1-AR as a blocker (30), we introduced the AR antagonists into the experimental setup to determine which receptor was mediating the effect. The distinct responses observed with the β1-AR antagonist and non-selective β-AR antagonist emphasize the unique anti-inflammatory effects of nebivolol, consistent with previous findings (40). Using a CRISPR/cas9 method of genome targeting ADRB2 which enables rapid and flexible experimental manipulation (41), we achieved an 80% knockout efficiency in human memory Th cells. This demonstrated that nebivolol’s inhibitory function on IL-17A production is mediated through the β2-AR pathway.
Our investigation into the expression of β2-AR following activation and treatment over 5 days at both the mRNA and protein levels revealed a notable reduction in receptor expression in memory Th cells treated with or without nebivolol compared to the non-activation group. This decrease in β2-AR expression may be attributed to receptor desensitization induced by stimulation of the T-cell receptor (TCR) and β2-AR signaling pathways. This feedback loop involves several steps leading to a decrease in receptor quantity on both the cell surface and intracellular compartments. These steps include the degradation of internalized receptors and the promotion of mRNA decay (42, 43), indicating the involvement of transcriptional control mechanisms (44).
The significant inhibition of NF-κB p65 activation by nebivolol, while CREB phosphorylation remained unaffected, suggests a divergence from the canonical β2-AR signaling pathway. In canonical signaling, activation of PKA typically facilitates CREB phosphorylation, competing with NF-κB activation and impeding its transcriptional activity (45). However, PKA activation of cytoplasmic kinases, such as p38 mitogen-activated protein kinase (MAPK), enhances IκBα degradation, thereby activating NF-κB-driven transcription (46). Notably, our results indicate that the β2-AR agonist drug terbutaline, unlike nebivolol, facilitated CREB phosphorylation along with NF-κB activation, consistent with prior research on other types of T cells (47, 48). These nuanced differences underscore the complexity of β2-AR-mediated pathways and suggest that nebivolol may act through a non-canonical pathway.
While we measured β-arrestin 1, our study did not measure β-arrestin 2 activation or its potential involvement in nebivolol’s effects. β-Arrestin 2 is known to inhibit NF-κB activation and the expression of its target genes by binding to and inhibiting the degradation of IκBα (14). Our findings provide insight into nebivolol’s mechanism of action, suggesting a β-arrestin biased agonism that selectively modulates downstream signaling molecules. This may explain nebivolol’s suppressive impact on IL-17A, independent of alterations in T-cell proliferation or viability. Thus, nebivolol appears to modulate cell signaling pathways relevant to cytokine expression without affecting the cell cycle or causing cellular cytotoxicity.
The observed outcomes align with established knowledge about adrenergic signaling in immune cells, emphasizing the role of AR in regulating T-cell function. β2-ARs, expressed widely throughout the body, including immune and non-immune cells, play a bystander role in immune responses, making them an optimal focal point for studying immune control. While β2-AR agonists have been recognized as modulators of inflammatory responses, β2-AR biased agonists (49), like nebivolol, selectively stimulate specific pathways, offering a more targeted approach to modulating immune cell function and treating disease(50). Nebivolol, identified as a notable β2 biased agonist in this study, emerges as a promising therapeutic target for chronic inflammation. Recent findings indicate its efficacy in reducing inflammatory signs of psoriatic lesions, inhibiting serum inflammatory biomarkers like IL-17A and TNF-α which were independent of β1-blockade activity (51, 52). The significance of nebivolol lies in its antioxidant and anti-inflammatory potential, providing a promising avenue for addressing persistent health concerns through the modulation of immune responses and cytokine production.
The immunomodulatory properties of nebivolol, particularly its ability to inhibit proinflammatory Th17 cell responses and promote anti-inflammatory Th2 responses, suggest promising therapeutic applications in autoimmune and inflammatory diseases such as multiple sclerosis, rheumatoid arthritis, and psoriasis characterized by elevated Th17 response (53, 54). Moreover, memory Th cells hold significant relevance in autoimmune diseases due to their enduring nature and ability to orchestrate recurrent autoimmune reactions (18). Memory Th17 and Th1 cells in the blood of MS patients correlate with disease severity (55). The established safety profile of nebivolol as a cardiovascular drug enhances its potential for repurposing, offering a well-tolerated option that could complement existing therapies. Future research should focus on elucidating the mechanisms underlying nebivolol’s biased agonism and assessing its long-term efficacy and safety in chronic inflammation, which could pave the way for innovative treatment strategies in immune modulation.
Our study acknowledges several limitations. The small sample size may limit the generalizability of our findings, necessitating larger cohorts in future research. Assessing specific molecules within the β-arrestin signaling pathway could confirm nebivolol’s biased agonist properties. Additionally, evaluating nebivolol’s effects in vivo in inflammatory situations would provide valuable insights into its therapeutic potential. The lack of transcriptomic analyses such as RNA sequencing is a limitation that could be addressed in future studies to explore the broader molecular effects of nebivolol. Addressing these limitations will build on our findings and deepen our understanding of nebivolol’s role in immune modulation.
In conclusion, this study advances our understanding of nebivolol’s immunomodulatory potential by specifically elucidating its impact on IL-17A secretion in Th17 cells and the underlying mechanisms. Nebivolol emerges as a promising candidate for further exploration in immunopharmacology, leveraging its established safety profile through drug repurposing. These findings offer avenues for targeted therapeutic interventions in chronic inflammation management, potentially reshaping treatment approaches with a familiar and accessible tool.
Data availability statement
The original contributions presented in the study are included in the article/Supplementary Material. Further inquiries can be directed to the corresponding author.
Ethics statement
The studies involving humans were approved by the Concordia University Human Research Ethics Committee, which follows the Declaration of Helsinki guidelines (certificate 30009292). The studies were conducted in accordance with the local legislation and institutional requirements. The participants provided their written informed consent to participate in this study.
Author contributions
MH: Conceptualization, Data curation, Formal analysis, Funding acquisition, Investigation, Methodology, Project administration, Software, Supervision, Validation, Visualization, Writing – original draft, Writing – review & editing. FG: Conceptualization, Methodology, Investigation, Software, Writing – review & editing. EH: Methodology, Visualization, Writing – review & editing. SL: Methodology, Writing – review & editing. NR: Methodology, Writing – review & editing. IA: Methodology, Writing – review & editing. CL: Methodology, Writing – review & editing. KT: Methodology, Writing – review & editing. SS: Project administration, Validation, Conceptualization, Supervision, Resources, Writing – review & editing. PD: Conceptualization, Funding acquisition, Investigation, Project administration, Resources, Supervision, Validation, Writing – review & editing.
Funding
The author(s) declare financial support was received for the research, authorship, and/or publication of this article. This work was funded by NSERC Discovery grant RGPIN‐2019‐06980 and FRNQT doctoral fellowship 2023-2024 - B2X – 334802.
Conflict of interest
The authors declare that the research was conducted in the absence of any commercial or financial relationships that could be construed as a potential conflict of interest.
Publisher’s note
All claims expressed in this article are solely those of the authors and do not necessarily represent those of their affiliated organizations, or those of the publisher, the editors and the reviewers. Any product that may be evaluated in this article, or claim that may be made by its manufacturer, is not guaranteed or endorsed by the publisher.
Supplementary material
The Supplementary Material for this article can be found online at: https://www.frontiersin.org/articles/10.3389/fimmu.2024.1446424/full#supplementary-material
References
1. Borchard U. The role of the sympathetic nervous system in cardiovascular disease. J Clin Basic Cardiol. (2001) 4:175–7.
2. Karemaker JM. An introduction into autonomic nervous function. Physiol measurement. (2017) 38:R89. doi: 10.1088/1361-6579/aa6782
3. Madden KS. Catecholamines, sympathetic innervation, and immunity. Brain behavior immunity. (2003) 17:5–10. doi: 10.1016/S0889-1591(02)00059-4
4. Del Rey A, Besedovsky H. Sympathetic-immune interactions during different types of immune challenge. Neuroimmunomodulation. (2023) 31:1–11. doi: 10.1159/000535467.
5. Elenkov IJ, Wilder RL, Chrousos GP, Vizi ES. The sympathetic nerve—an integrative interface between two supersystems: the brain and the immune system. Pharmacol Rev. (2000) 52:595–638.
6. Bellinger DL, Lorton D, Felten SY, Felten DL. Innervation of lymphoid organs and implications in development, aging, and autoimmunity. Int J immunopharmacology. (1992) 14:329–44. doi: 10.1016/0192-0561(92)90162-E
7. Nance DM, Sanders VM. Autonomic innervation and regulation of the immune system (1987–2007). Brain behavior immunity. (2007) 21:736–45. doi: 10.1016/j.bbi.2007.03.008
8. Lorton D, Bellinger DL. Molecular mechanisms underlying β-adrenergic receptor-mediated cross-talk between sympathetic neurons and immune cells. Int J Mol Sci. (2015) 16:5635–65. doi: 10.3390/ijms16035635
9. Naqvi S, Martin KJ, Arthur JSC. CREB phosphorylation at Ser133 regulates transcription via distinct mechanisms downstream of cAMP and MAPK signalling. Biochem J. (2014) 458:469–79. doi: 10.1042/BJ20131115
10. Guereschi MG, Araujo LP, Maricato JT, Takenaka MC, Nascimento VM, Vivanco BC, et al. Beta2-adrenergic receptor signaling in CD 4+ F oxp3+ regulatory T cells enhances their suppressive function in a PKA-dependent manner. Eur J Immunol. (2013) 43:1001–12. doi: 10.1002/eji.201243005
11. Sanders VM, Baker RA, Ramer-Quinn DS, Kasprowicz DJ, Fuchs BA, Street NE. Differential expression of the beta2-adrenergic receptor by Th1 and Th2 clones: implications for cytokine production and B cell help. J Immunol. (1997) 158:4200–10. doi: 10.4049/jimmunol.158.9.4200
12. Sharma D, Parameswaran N. Multifaceted role of β-arrestins in inflammation and disease. Genes Immunity. (2015) 16:499–513. doi: 10.1038/gene.2015.37
13. Shenoy SK, Drake MT, Nelson CD, Houtz DA, Xiao K, Madabushi S, et al. [amp]]beta;-Arrestin-dependent, G protein-independent ERK1/2 activation by the β2 adrenergic receptor. J Biol Chem. (2006) 281:1261–73. doi: 10.1074/jbc.M506576200
14. Gao H, Sun Y, Wu Y, Luan B, Wang Y, Qu B, et al. Identification of β-arrestin2 as a G protein-coupled receptor-stimulated regulator of NF-κB pathways. Mol Cell. (2004) 14:303–17. doi: 10.1016/S1097-2765(04)00216-3
15. Balasubramani A, Shibata Y, Crawford GE, Baldwin AS, Hatton RD, Weaver CT. Modular utilization of distal cis-regulatory elements controls Ifng gene expression in T cells activated by distinct stimuli. Immunity. (2010) 33:35–47. doi: 10.1016/j.immuni.2010.07.004
16. Ruan Q, Kameswaran V, Zhang Y, Zheng S, Sun J, Wang J, et al. The Th17 immune response is controlled by the Rel–RORγ–RORγT transcriptional axis. J Exp Med. (2011) 208:2321–33. doi: 10.1084/jem.20110462
17. Sanders VM, Kasprowicz DJ, Kohm AP, Swanson MA. Neurotransmitter receptors on lymphocytes and other lymphoid cells. Psychoneuroimmunology. (2001) 1:161–96.
18. Raphael I, Joern RR, Forsthuber TG. Memory CD4+ T cells in immunity and autoimmune diseases. Cells. (2020) 9:531. doi: 10.3390/cells9030531
19. Slota C, Shi A, Chen G, Bevans M, Weng N-p. Norepinephrine preferentially modulates memory CD8 T cell function inducing inflammatory cytokine production and reducing proliferation in response to activation. Brain behavior immunity. (2015) 46:168–79. doi: 10.1016/j.bbi.2015.01.015
20. Pawlak M, Ho AW, Kuchroo VK. Cytokines and transcription factors in the differentiation of CD4+ T helper cell subsets and induction of tissue inflammation and autoimmunity. Curr Opin Immunol. (2020) 67:57–67. doi: 10.1016/j.coi.2020.09.001
21. Swanson MA, Lee WT, Sanders VM. IFN-γ production by Th1 cells generated from naive CD4+ T cells exposed to norepinephrine. J Immunol. (2001) 166(1):232–40.
22. Takenaka MC, Araujo LP, Maricato JT, Nascimento VM, Guereschi MG, Rezende RM, et al. Norepinephrine controls effector T cell differentiation through β2-adrenergic receptor–mediated inhibition of NF-κB and AP-1 in dendritic cells. J Immunol. (2016) 196:637–44. doi: 10.4049/jimmunol.1501206
23. Manni M, Granstein RD, Maestroni G. [amp]]beta;2-Adrenergic agonists bias TLR-2 and NOD2 activated dendritic cells towards inducing an IL-17 immune response. Cytokine. (2011) 55:380–6. doi: 10.1016/j.cyto.2011.05.013
24. Kima JWYB, Junga JBS, Hana JPM, Baeka S-K, Kima TH. Regulation of T helper cell type 2 immune response by controlling beta-2 adrenergic receptor in dendritic cells of patients with allergic rhinitis. Int Arch Allergy Immunol. (2023) 184:1173–83. doi: 10.1159/000531956
25. Carvajal Gonczi CM, Tabatabaei Shafiei M, East A, Martire E, Maurice-Ventouris MH, Darlington PJ. Reciprocal modulation of helper Th1 and Th17 cells by the β2-adrenergic receptor agonist drug terbutaline. FEBS J. (2017) 284:3018–28. doi: 10.1111/febs.2017.284.issue-18
26. Gonczi CMC, Hajiaghayi M, Gholizadeh F, Soares MAX, Touma F, Naranjo CL, et al. The β2-adrenergic receptor agonist terbutaline upregulates T helper-17 cells in a protein kinase A-dependent manner. Hum Immunol. (2023) 84:515–24. doi: 10.1016/j.humimm.2023.06.007
27. Gonczi CMC, Touma F, Daigneault T, Pozzebon C, Burchell-Reyes K, Darlington PJ. Modulation of IL-17A and IFNγ by β2-adrenergic agonist terbutaline and inverse-agonist nebivolol, influence of ADRB2 polymorphisms. AIMS Allergy Immunol. (2021) 5:222–39. doi: 10.3934/Allergy.2021017
28. Diego LM, Jazmin FM, Diana RH, German-Isauro GF, Salvador FC, Maria-Elena HC. Modulation of TNF-α, interleukin-6, and interleukin-10 by nebivolol–valsartan and nebivolol–lisinopril polytherapy in SHR rats. Pharmacol Res Perspectives. (2024) 12:e1189. doi: 10.1002/prp2.v12.2
29. Yang S-P, Ho L-J, Lin Y-L, Cheng S-M, Tsao T-P, Chang D-M, et al. Carvedilol, a new antioxidative β-blocker, blocks in vitro human peripheral blood T cell activation by downregulating NF-κB activity. Cardiovasc Res. (2003) 59:776–87. doi: 10.1016/S0008-6363(03)00459-0
30. Veverka A, Salinas J L. Nebivolol in the treatment of chronic heart failure. Vasc Health Risk management. (2007) 3:647–54.
31. Zhang Z, Ding L, Jin Z, Gao G, Li H, Zhang L, et al. Nebivolol protects against myocardial infarction injury via stimulation of beta 3-adrenergic receptors and nitric oxide signaling. PloS One. (2014) 9:e98179. doi: 10.1371/journal.pone.0098179
32. Erickson CE, Gul R, Blessing CP, Nguyen J, Liu T, Pulakat L, et al. The β-blocker nebivolol is a GRK/β-arrestin biased agonist. PloS One. (2013) 8:e71980. doi: 10.1371/journal.pone.0071980
33. Van de Water A, Janssens W, Van Neuten J, Xhonneux R, De Cree J, Verhaegen H, et al. Pharmacological and hemodynamic profile of nebivolol, a chemically novel, potent, and selective beta 1-adrenergic antagonist. J Cardiovasc Pharmacol. (1988) 11:552–63. doi: 10.1097/00005344-198805000-00007
34. Brixius K, Bundkirchen A, Bölck B, Mehlhorn U, Schwinger RH. Nebivolol, bucindolol, metoprolol and carvedilol are devoid of intrinsic sympathomimetic activity in human myocardium. Br J Pharmacol. (2001) 133:1330–8. doi: 10.1038/sj.bjp.0704188
35. Darlington PJ, Touil T, Doucet JS, Gaucher D, Zeidan J, Gauchat D, et al. Diminished Th17 (not Th1) responses underlie multiple sclerosis disease abrogation after hematopoietic stem cell transplantation. Ann neurology. (2013) 73:341–54. doi: 10.1002/ana.23784
36. Little SR, Leung Z, Quach AB, Hirukawa A, Gholizadeh F, Hajiaghayi M, et al. A tri-droplet liquid structure for highly efficient intracellular delivery in primary mammalian cells using digital microfluidics. Advanced Materials Technologies. (2023) 8:2300719. doi: 10.1002/admt.202300719
37. Schumann K, Lin S, Boyer E, Simeonov DR, Subramaniam M, Gate RE, et al. Generation of knock-in primary human T cells using Cas9 ribonucleoproteins. Proc Natl Acad Sci. (2015) 112:10437–42. doi: 10.1073/pnas.1512503112
38. Phillips T. Regulation of transcription and gene expression in eukaryotes. Nat Education. (2008) 1:199.
39. Sanders VM. The beta2-adrenergic receptor on T and B lymphocytes: do we understand it yet? Brain behavior Immun. (2012) 26:195–200. doi: 10.1016/j.bbi.2011.08.001
40. Mason RP, Kubant R, Jacob RF, Walter MF, Boychuk B, Malinski T. Effect of nebivolol on endothelial nitric oxide and peroxynitrite release in hypertensive animals: role of antioxidant activity. J Cardiovasc Pharmacol. (2006) 48:862–9. doi: 10.1097/01.fjc.0000238593.67191.e2
41. Roth TL, Puig-Saus C, Yu R, Shifrut E, Carnevale J, Li PJ, et al. Reprogramming human T cell function and specificity with non-viral genome targeting. Nature. (2018) 559:405–9. doi: 10.1038/s41586-018-0326-5
42. Danner S, Frank M, Lohse MJ. Agonist regulation of human β2-adrenergic receptor mRNA stability occurs via a specific AU-rich element. J Biol Chem. (1998) 273:3223–9. doi: 10.1074/jbc.273.6.3223
43. Mayati A, Podechard N, Rineau M, Sparfel L, Lagadic-Gossmann D, Fardel O, et al. Benzo (a) pyrene triggers desensitization of β2-adrenergic pathway. Sci Rep. (2017) 7:3262. doi: 10.1038/s41598-017-03646-4
44. Penela P, Ribas C, Mayor F. Mechanisms of regulation of the expression and function of G protein-coupled receptor kinases. Cell signalling. (2003) 15:973–81. doi: 10.1016/S0898-6568(03)00099-8
45. Wen AY, Sakamoto KM, Miller LS. The role of the transcription factor CREB in immune function. J Immunol. (2010) 185:6413–9. doi: 10.4049/jimmunol.1001829
46. Kong Y, Ruan L, Qian L, Liu X, Le Y. Norepinephrine promotes microglia to uptake and degrade amyloid β peptide through upregulation of mouse formyl peptide receptor 2 and induction of insulin-degrading enzyme. J Neurosci. (2010) 30:11848–57. doi: 10.1523/JNEUROSCI.2985-10.2010
47. Kohm AP, Mozaffarian A, Sanders VM. B cell receptor-and β2-adrenergic receptor-induced regulation of B7-2 (CD86) expression in B cells. J Immunol. (2002) 168:6314–22. doi: 10.4049/jimmunol.168.12.6314
48. Singh A, Ranjan A. Adrenergic receptor signaling regulates the CD40-receptor mediated anti-tumor immunity. Front Immunol. (2023) 14:1141712. doi: 10.3389/fimmu.2023.1141712
49. Azzi M, Charest PG, Angers S, Rousseau G, Kohout T, Bouvier M, et al. [amp]]beta;-Arrestin-mediated activation of MAPK by inverse agonists reveals distinct active conformations for G protein-coupled receptors. Proc Natl Acad Sci. (2003) 100:11406–11. doi: 10.1073/pnas.1936664100
50. Michel MC, Charlton SJ. Biased agonism in drug discovery—is it too soon to choose a path? Mol Pharmacol. (2018) 93:259–65. doi: 10.1124/mol.117.110890
51. Sanaee F, Jamali F. Action and disposition of the β3-agonist nebivolol in the presence of inflammation; an alternative to conventional β1-blockers. Curr Pharm Design. (2014) 20:1311–7. doi: 10.2174/13816128113199990550
52. Mahdi ZA, Hussain AM, Alblesh HA. Histopathological and immunological effects of nebivolol 5% Topical cream in mice model of imiquimod-induced psoriasis. Al-Rafidain J Med Sci (ISSN 2789-3219). (2024) 6:133–41. doi: 10.54133/ajms.v6i1.527
53. McGeachy MJ, Cua DJ, Gaffen SL. The IL-17 family of cytokines in health and disease. Immunity. (2019) 50:892–906. doi: 10.1016/j.immuni.2019.03.021
54. Raphael I, Nalawade S, Eagar TN, Forsthuber TG. T cell subsets and their signature cytokines in autoimmune and inflammatory diseases. Cytokine. (2015) 74:5–17. doi: 10.1016/j.cyto.2014.09.011
55. Paroni M, Maltese V, De Simone M, Ranzani V, Larghi P, Fenoglio C, et al. Recognition of viral and self-antigens by TH1 and TH1/TH17 central memory cells in patients with multiple sclerosis reveals distinct roles in immune surveillance and relapses. J Allergy Clin Immunol. (2017) 140:797–808. doi: 10.1016/j.jaci.2016.11.045
Keywords: nebivolol, Th17 cells, IL-17A, biased agonist, beta-adrenergic receptor, anti-inflammatory response, NF-κB activation
Citation: Hajiaghayi M, Gholizadeh F, Han E, Little SR, Rahbari N, Ardila I, Lopez Naranjo C, Tehranimeh K, Shih SCC and Darlington PJ (2024) The β2-adrenergic biased agonist nebivolol inhibits the development of Th17 and the response of memory Th17 cells in an NF-κB-dependent manner. Front. Immunol. 15:1446424. doi: 10.3389/fimmu.2024.1446424
Received: 09 June 2024; Accepted: 11 September 2024;
Published: 09 October 2024.
Edited by:
Ahmed Nadeem, King Saud University, Saudi ArabiaReviewed by:
Qiang Bai, INSERM U1046 Physiologie et Médecine Expérimentale du Coeur et des Muscles, FranceNeetu Singh, University of Texas MD Anderson Cancer Center, United States
Copyright © 2024 Hajiaghayi, Gholizadeh, Han, Little, Rahbari, Ardila, Lopez Naranjo, Tehranimeh, Shih and Darlington. This is an open-access article distributed under the terms of the Creative Commons Attribution License (CC BY). The use, distribution or reproduction in other forums is permitted, provided the original author(s) and the copyright owner(s) are credited and that the original publication in this journal is cited, in accordance with accepted academic practice. No use, distribution or reproduction is permitted which does not comply with these terms.
*Correspondence: Peter J. Darlington, peter.darlington@concordia.ca
†Present addresses: Eric Han, School of Medicine, McGill University, Montréal, QC, Canada Carolina Lopez Naranjo, Department of Experimental Medicine, McGill University, Montréal, QC, Canada