- 1The Affiliated Hospital of Qingdao University, Qingdao University, Qingdao, China
- 2Department of Orthopedics, The Affiliated Hospital of Qingdao University, Qingdao, China
- 3Department of Nutrition, Peking University People’s Hospital, Qingdao, China
- 4Women and Children’s Hospital, Qingdao University, Qingdao, China
- 5Department of Spinal Surgery, Affiliated Hospital of Qingdao University, Qingdao, China
Adjuvant therapy is essential in cancer treatment to enhance primary treatment effectiveness, reduce adverse effects, and prevent recurrence. Small molecule inhibitors as adjuvants in cancer immunotherapy aim to harness their immunomodulatory properties to optimize treatment outcomes. By modulating the tumor microenvironment, enhancing immune cell function, and increasing tumor sensitivity to immunotherapy, small molecule inhibitors have the potential to improve patient responses. This review discusses the evolving use of small molecule inhibitors as adjuvants in cancer treatment, highlighting their role in enhancing the efficacy of immunotherapy and the opportunities for advancing cancer therapies in the future.
1 Introduction
Adjuvant therapy in cancer treatment refers to the use of additional treatments such as chemotherapy, radiation, or targeted therapies following primary treatments like surgery. The main objectives of adjuvant therapy are to enhance the effectiveness of the primary treatment, reduce adverse effects, and prevent disease recurrence (1–3). This approach targets residual cancer cells post-surgery, helping to reduce the risk of cancer returning and spreading, and thereby improving the overall success rates of cancer eradication (4, 5). In the context of cancer immunotherapy, small molecule inhibitors serve as immune adjuvants. These inhibitors aim to modulate the tumor microenvironment, enhance immune cell function, and increase tumor sensitivity to immunotherapy (6, 7). By leveraging their immunomodulatory properties, small molecule inhibitors can optimize treatment outcomes, improve patient responses, and provide new opportunities for advancing cancer therapies (8).
In cancer immunotherapy, the concept of using small molecule inhibitors as adjuvants involves leveraging the immunomodulatory effects of these drugs to enhance the effectiveness of immunotherapy. For example, small molecule inhibitors can modulate the tumor microenvironment, boost immune cell function, increase tumor sensitivity to immunotherapy, and achieve better treatment outcomes (9–11). Using small molecule inhibitors as adjuvants in cancer treatment is a rapidly evolving and expanding field. By researching how small molecule inhibitors interact with immunotherapy, optimizing treatment regimens, predicting patient responses to treatment, it can provide more opportunities and improvements for future cancer treatments. In this comprehensive review, we delve into the evolving role of small molecule inhibitors as adjuvants in cancer immunotherapy, exploring their mechanisms of action, clinical applications, and potential for improving treatment outcomes.
2 Mechanisms of action of small molecule inhibitors in cancer immunotherapy
Small molecule inhibitors play a significant role in cancer immunotherapy by targeting specific pathways and molecules involved in regulating the immune response to tumors. These inhibitors act through various mechanisms to modulate the tumor microenvironment and enhance the anti-tumor immune response. Some common mechanisms of action of small molecule inhibitors in cancer immunotherapy have been summarized.
2.1 Immune checkpoint blockade
Immune checkpoint blockade is a cutting-edge cancer immunotherapy that targets molecules like CTLA-4 and PD-1 to activate T cells and boost anti-tumor immunity. By blocking inhibitory signals, checkpoint inhibitors unleash the immune system to recognize and eliminate cancer cells (12, 13). Monoclonal antibodies targeting CTLA-4, such as ipilimumab, disrupt this inhibitory signal, enhancing T cell activation and anti-tumor immune responses (14). Small molecule inhibitors, on the other hand, are designed to interfere with intracellular signaling pathways, thus modulating immune responses indirectly. PD-1, expressed on T cells upon activation, interacts with PD-L1 to inhibit T cell function (15). Small molecule inhibitors targeting the PD-1/PD-L1 pathway can modulate intracellular signaling pathways, leading to T cell activation and immune-mediated tumor cell killing (16). By targeting these key immune checkpoint molecules with small molecule inhibitors, we can modulate immune responses to overcome tumor-induced immune suppression and expand the therapeutic landscape in cancer immunotherapy.
2.2 Signal transduction pathways
Signal transduction pathways play a critical role in regulating immune responses in cancer, including immune cell activation, proliferation, and effector functions. Small molecule inhibitors targeting key signaling molecules within these pathways have emerged as promising adjuvants in cancer immunotherapy (17) (18). For instance, inhibitors of the PI3K-Akt-mTOR pathway can modulate T cell activation and differentiation, enhancing anti-tumor immunity (19). Inhibitors of the MAPK pathway, such as MEK inhibitors, can regulate T cell function and cytokine production to optimize anti-tumor immune responses (20). Additionally, inhibitors of the NF-κB pathway can modulate inflammatory responses and immune cell activation. By selectively targeting specific nodes within these signaling pathways, small molecule inhibitors can fine-tune immune responses to promote effective anti-tumor immunity (21). Understanding the intricate interplay of signal transduction pathways and harnessing the therapeutic potential of small molecule inhibitors offer exciting avenues to expand the therapeutic landscape of cancer immunotherapy and improve patient outcomes.
2.3 Enhancing immune cell infiltration
Enhancing immune cell infiltration into tumors is a critical mechanism by which small molecule inhibitors act as adjuvants in cancer immunotherapy. Effective infiltration of immune cells into the tumor microenvironment is essential for mounting a robust anti-tumor immune response. Small molecule inhibitors target key pathways and mechanisms, such as angiogenesis, extracellular matrix remodeling, and immune cell infiltration, to enhance the immune response within the tumor microenvironment.
2.3.1 Targeting angiogenic pathways
Tumor growth and progression are heavily dependent on the formation of new blood vessels, a process known as angiogenesis. Tumors secrete vascular endothelial growth factor (VEGF) to promote angiogenesis, which also creates an abnormal and disorganized vascular network that impedes immune cell infiltration. Small molecule inhibitors, such as those targeting VEGF receptors (VEGFR), can normalize the tumor vasculature. By inhibiting VEGF signaling, these inhibitors can reduce the formation of new blood vessels, disrupt existing abnormal vessels, and improve the overall vascular structure within the tumor. This normalization of the tumor vasculature facilitates better penetration and infiltration of immune cells, such as cytotoxic T lymphocytes (CTLs) and natural killer (NK) cells, into the tumor microenvironment (22).
2.3.2 Modulating the extracellular matrix
The extracellular matrix (ECM) within tumors often presents a physical barrier to immune cell infiltration. Small molecule inhibitors can modulate components of the ECM to enhance immune cell penetration. For instance, inhibitors targeting enzymes such as matrix metalloproteinases (MMPs) can degrade ECM components, thereby reducing the physical barriers that prevent immune cells from reaching the tumor core. By altering the ECM composition, these inhibitors create pathways for immune cells to infiltrate more effectively (23).
2.3.3 Reducing immunosuppressive cells
The tumor microenvironment often contains a high number of immunosuppressive cells, such as regulatory T cells (Tregs) and myeloid-derived suppressor cells (MDSCs), which inhibit the activity and infiltration of effector immune cells. Small molecule inhibitors can selectively target and reduce the population of these immunosuppressive cells. For example, inhibitors of the colony-stimulating factor-1 receptor (CSF-1R) can decrease the number of MDSCs, thereby reducing their suppressive effects on immune cell infiltration and function. This reduction in immunosuppressive cells enhances the ability of effector immune cells to infiltrate the tumor and exert their anti-tumor effects (24).
2.3.4 Enhancing chemokine signaling
Chemokines are signaling molecules that guide the migration of immune cells to sites of inflammation, including tumors. Small molecule inhibitors can enhance chemokine signaling pathways to promote the recruitment and infiltration of immune cells into tumors. For instance, inhibitors that upregulate the expression of chemokines such as CXCL9 and CXCL10 can attract more CTLs to the tumor site. By increasing the concentration of these chemokines in the tumor microenvironment, small molecule inhibitors enhance the directional migration of immune cells into the tumor, improving their infiltration and subsequent anti-tumor activity (25).
2.4 Immunomodulation
Small molecule inhibitors, by targeting immunomodulatory pathways, can shape the immune landscape within the tumor microenvironment to bolster immune-mediated tumor eradication (26). These inhibitors can modulate the activity of various immune cell populations, such as T cells, regulatory T cells, and myeloid-derived suppressor cells, to tip the balance in favor of anti-tumor immune responses. Furthermore, small molecule inhibitors can impact cytokine signaling networks, influencing the immune cell functions and interactions critical for mounting effective anti-tumor immune responses (27). By fine-tuning immune responses through targeted immunomodulation, small molecule inhibitors can overcome immune evasion mechanisms employed by tumors and enhance the efficacy of cancer immunotherapy. Leveraging the power of immunomodulation in conjunction with other therapeutic strategies, such as immune checkpoint blockade or targeted therapies, offers a multifaceted approach to expand the therapeutic landscape of cancer immunotherapy and improve patient outcomes.
By targeting these key pathways and mechanisms, small molecule inhibitors can synergize with immunotherapy approaches to improve treatment outcomes in cancer patients. Further research into the precise mechanisms of action of small molecule inhibitors in cancer immunotherapy holds promise for developing more effective and targeted cancer treatments.
3 Clinical applications of small molecule inhibitors as adjuvants in cancer immunotherapy
Small molecule inhibitors have demonstrated promising clinical applications in cancer immunotherapy across various types of cancer (9). These inhibitors play a crucial role as adjuvants by enhancing immune responses, overcoming resistance mechanisms, and improving overall treatment outcomes. Their ability to modulate the tumor microenvironment and improve immune cell infiltration makes them valuable assets in combination with existing immunotherapy approaches.
For instance, studies have shown that small molecule inhibitors targeting VEGFR can normalize tumor vasculature, facilitating better immune cell infiltration and enhancing the effectiveness of immune checkpoint inhibitors in clinical settings. In a phase II clinical trial, the combination of the VEGFR inhibitor axitinib with the PD-1 inhibitor pembrolizumab showed significant improvement in response rates and overall survival in patients with metastatic renal cell carcinoma (28). Similarly, the PI3K inhibitor idelalisib has been used successfully in combination with rituximab for the treatment of relapsed chronic lymphocytic leukemia, demonstrating the potential of small molecule inhibitors in enhancing the efficacy of immunotherapy (29).
3.1 Combination therapy
Combining small molecule inhibitors with other immunotherapeutic approaches, such as immune checkpoint inhibitors or adoptive T cell therapy, offers a synergistic approach to amplify immune responses and overcome resistance mechanisms (30). By targeting distinct signaling pathways or immune checkpoints simultaneously, combination therapy has the potential to broaden the spectrum of anti-tumor immune responses and improve treatment outcomes. Furthermore, combining small molecule inhibitors with traditional cancer treatments like chemotherapy or radiation therapy can create a multifaceted attack on tumor cells, leading to more comprehensive and durable responses (31). The rational design of combination regimens that leverage the strengths of different therapeutic modalities holds promise in expanding the therapeutic landscape of cancer immunotherapy and addressing the challenges of immune evasion and tumor heterogeneity.
3.2 Overcoming resistance
Resistance mechanisms, such as immune evasion and tumor heterogeneity, can limit the success of immunotherapeutic approaches. Small molecule inhibitors can help overcome resistance by targeting pathways involved in immune evasion and tumor immune escape (32). By disrupting these critical signaling pathways in the tumor microenvironment, small molecule inhibitors can enhance immune cell infiltration, reprogram immune responses, and restore immune recognition of tumor cells (33). Additionally, combination therapies that incorporate small molecule inhibitors alongside immunotherapies or other treatments present a comprehensive strategy to combat resistance and enhance treatment outcomes. Overall, by targeting resistance mechanisms, small molecule inhibitors play a vital role in expanding the therapeutic landscape of cancer immunotherapy and improving patient responses to treatment.
3.3 Personalized medicine and adjuvant therapy
By leveraging small molecule inhibitors in cancer immunotherapy, personalized medicine aims to identify specific molecular targets or pathways unique to each patient’s tumor (34). This precision medicine approach allows for the selection of the most effective small molecule inhibitors based on the molecular characteristics of the tumor, genetic profile of the patient, and immune response (35). By customizing treatment regimens to match the individual tumor biology and immune landscape, personalized medicine maximizes therapeutic efficacy while minimizing side effects.
Additionally, overcoming drug resistance is a critical aspect of personalized medicine. Certain genetic changes caused by drug resistance can be precisely regulated through targeted small molecule inhibitors. By identifying and targeting these specific genetic alterations, small molecule inhibitors can help to overcome resistance and restore sensitivity to treatment. The use of small molecule inhibitors as adjuvants in cancer immunotherapy represents a promising strategy to expand the therapeutic landscape, overcome treatment resistance, and improve patient responses to immunotherapy.
3.4 New targets and indications
Small molecule inhibitors offer the potential to target novel pathways and molecular targets that have not been previously exploited in immunotherapeutic approaches. By identifying and leveraging these new targets, researchers can broaden the scope of immunotherapy strategies, address the challenges of treatment resistance, and enhance therapeutic efficacy (36). Additionally, the discovery of new indications for small molecule inhibitors in cancer immunotherapy opens up opportunities to treat a wider range of cancer types and patient populations (37). The pursuit of novel targets and indications for small molecule inhibitors in cancer immunotherapy holds great promise for expanding the therapeutic landscape and improving outcomes for individuals with cancer.
The clinical applications of small molecule inhibitors in cancer immunotherapy represent a rapidly evolving field, with ongoing research focusing on optimizing treatment regimens, identifying biomarkers, and expanding the therapeutic potential of these inhibitors in various cancer types (38). As our understanding of the tumor microenvironment and immune response continues to advance, small molecule inhibitors are poised to play a pivotal role in shaping the future of cancer immunotherapy.
4 Examples of small molecule inhibitors with immunomodulatory effects in cancer immunotherapy
Small molecule inhibitors are increasingly recognized for their role in cancer immunotherapy due to their ability to modulate immune responses and enhance anti-tumor activity. These inhibitors target specific proteins and pathways involved in cancer cell proliferation and survival, as well as the tumor microenvironment, which can suppress the immune system (39). By inhibiting these targets, small molecule inhibitors can restore or enhance the immune system’s ability to recognize and destroy cancer cells.
Some well-known examples include Vemurafenib, which targets BRAF and modulates the tumor microenvironment to promote T cell infiltration in melanoma (40); Dasatinib, which targets multiple tyrosine kinases and enhances immune cell function in leukemia and solid tumors (41); and Ibrutinib, which inhibits BTK and modulates B-cell receptor signaling in B-cell malignancies (42). Other notable inhibitors, such as Sunitinib and Pazopanib, target multiple receptor tyrosine kinases and have shown efficacy in reducing regulatory T cells and myeloid-derived suppressor cells, thereby enhancing the overall immune response in various cancers (43, 44). These small molecule inhibitors, by targeting critical pathways involved in immune regulation and tumor growth, offer significant potential to improve the effectiveness of cancer immunotherapies and patient outcomes. In Table 1, selected examples of these small molecule inhibitors, which have been proven to exert excellent immunomodulatory effects, have been summarized with discussion of their action mechanisms.
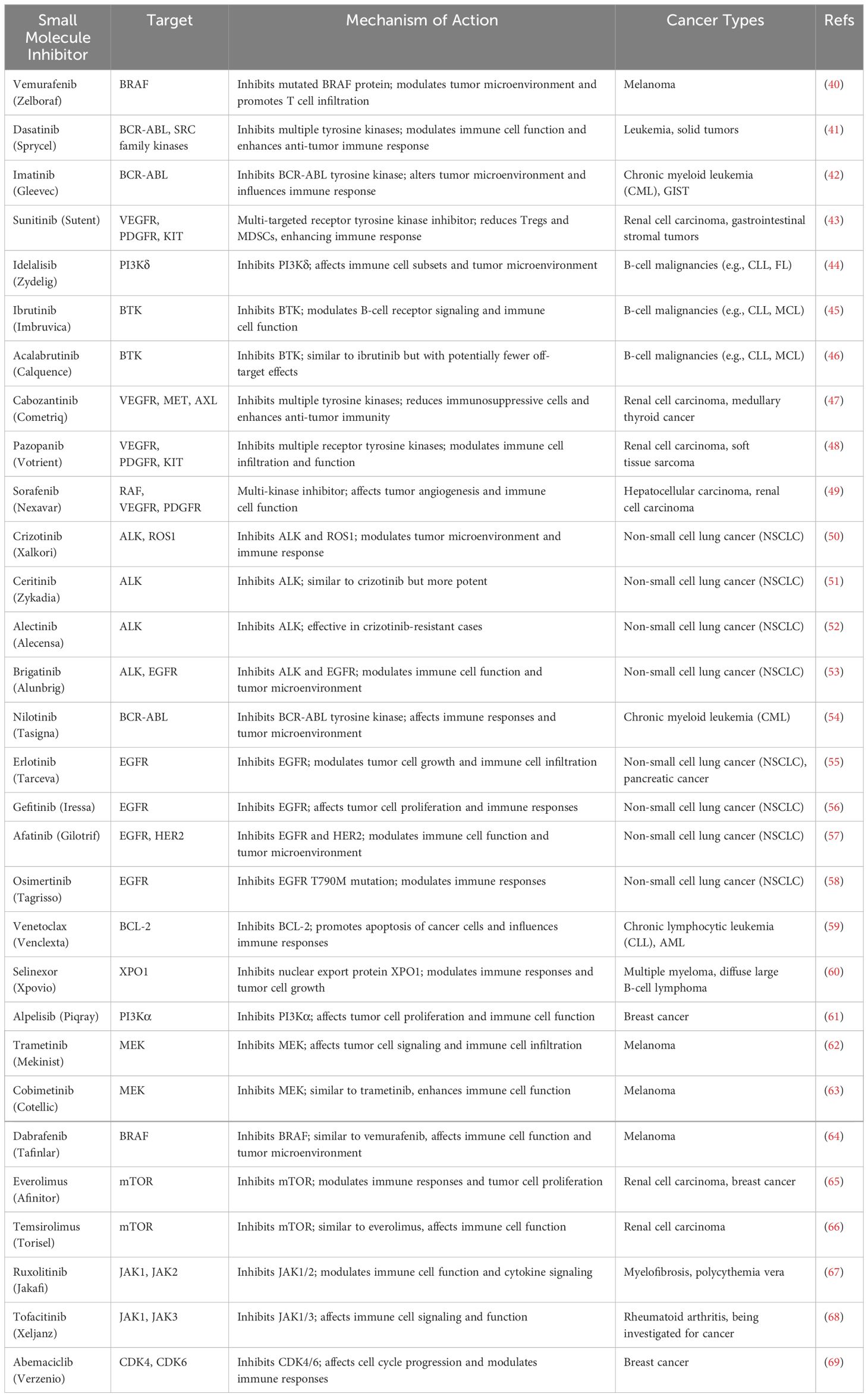
Table 1 Selected examples of small molecule inhibitors with immunomodulatory effects and their action mechanisms.
These examples highlight the diverse mechanisms of action and clinical applications of small molecule inhibitors with immunomodulatory effects in cancer immunotherapy. By targeting specific pathways involved in immune regulation and tumor growth, these inhibitors have the potential to enhance the efficacy of immunotherapy and improve outcomes for cancer patients.
5 Challenges and future directions in small molecule inhibitors as adjuvants in cancer immunotherapy
5.1 Resistance mechanisms
One of the challenges in using small molecule inhibitors as adjuvants in cancer immunotherapy is the development of resistance mechanisms by tumors (32). Tumors can acquire mutations or activate alternative signaling pathways to bypass the effects of these inhibitors. For example, resistance to BTK inhibitors like ibrutinib in B-cell malignancies often involves mutations in the BTK binding site or activation of PLCγ2 signaling (70). Future research should focus on understanding these resistance mechanisms and developing strategies to overcome them, such as combination therapies with other targeted agents or immunotherapies.
5.2 Specificity and off-target effects
Small molecule inhibitors may have off-target effects on normal cells, leading to toxicities and adverse effects (71). For instance, the multi-kinase inhibitor sunitinib has been associated with cardiotoxicity and hypertension due to its off-target effects on other kinases (72). Improving the specificity of these inhibitors to target tumor cells while sparing healthy tissues is crucial for minimizing toxicity and improving the safety profile of combination therapies.
5.3 Biomarker identification
Biomarkers that predict response to small molecule inhibitors as adjuvants in cancer immunotherapy are still evolving (73). Identifying reliable biomarkers to predict response to small molecule inhibitors as adjuvants in cancer immunotherapy is another unique challenge. For example, PD-L1 expression is a known biomarker for response to checkpoint inhibitors, but similar biomarkers for small molecule inhibitors are still being explored (74). Research efforts should prioritize the discovery and validation of biomarkers that can guide treatment selection and monitor response to therapy.
5.4 Optimal dosing and scheduling
Determining the optimal dosing and scheduling of small molecule inhibitors in combination with immunotherapy is essential for maximizing therapeutic efficacy while minimizing toxicity. This is particularly important for inhibitors that may have cumulative toxicities when used in combination regimens. Studies have shown that staggered dosing schedules can reduce toxicity and improve outcomes in combination therapies involving kinase inhibitors and immunotherapies (75).
5.5 Combination therapy strategies
Developing rational combination therapy strategies with small molecule inhibitors and immunotherapy agents is a complex and evolving field (30). For instance, combining VEGFR inhibitors with checkpoint inhibitors has shown promise in preclinical models, but optimal combinations and sequences need to be established through clinical trials (76). Future directions should explore novel combinations, target multiple pathways simultaneously, and leverage advances in tumor immunology to enhance the anti-tumor immune response and overcome treatment resistance.
5.6 Translational research and clinical trials
Translating preclinical findings into clinical practice and conducting well-designed clinical trials are essential for evaluating the safety and efficacy of small molecule inhibitors as adjuvants in cancer immunotherapy (77). Future research directions should prioritize rigorous clinical testing and validation of promising combination therapies.
Overall, addressing these challenges and advancing research efforts in biomarker identification, treatment optimization, combination therapy strategies, and clinical trial design will be critical for harnessing the full potential of small molecule inhibitors as adjuvants in cancer immunotherapy and improving outcomes for cancer patients. Collaborative efforts between researchers, clinicians, and industry stakeholders will be essential for driving progress in this rapidly evolving field.
6 Conclusion
In conclusion, small molecule inhibitors have emerged as promising adjuvants in cancer immunotherapy, offering the potential to enhance the anti-tumor immune response and improve treatment outcomes for cancer patients. By targeting specific signaling pathways involved in tumor growth and immune evasion, these inhibitors can modulate the tumor microenvironment, sensitize tumors to immune-mediated destruction, and potentiate the effects of immunotherapy agents.
Despite the significant progress in the development and clinical use of small molecule inhibitors in cancer treatment, several challenges remain to be addressed. Resistance mechanisms, off-target effects, biomarker identification, optimal dosing and scheduling, as well as rational combination therapy strategies are important considerations that need to be carefully addressed in future research and clinical practice. Moving forward, future directions in small molecule inhibitors as adjuvants in cancer immunotherapy should focus on overcoming resistance mechanisms, improving specificity and safety profiles, identifying predictive biomarkers, optimizing treatment regimens, developing innovative combination therapies, and conducting robust translational research and clinical trials.
It is essential that collaborative efforts and multidisciplinary approaches be employed to advance the field of small molecule inhibitors in cancer immunotherapy. By addressing these challenges and pursuing innovative research strategies, we can harness the full potential of small molecule inhibitors to improve patient outcomes, enhance treatment response rates, and ultimately pave the way for more effective and personalized cancer therapies in the future.
Author contributions
HJ: Writing – original draft, Writing – review & editing. XW: Writing – original draft, Writing – review & editing. NF: Data curation, Writing – review & editing. CW: Data curation, Writing – review & editing. ZG: Writing – original draft, Writing – review & editing.
Funding
The author(s) declare financial support was received for the research, authorship, and/or publication of this article. This study was supported by Natural Science Foundation of Shandong Province (ZR2022MH218; ZR2022QC165); Youth Research Fund of Affiliated Hospital of Qingdao University in 2021(QDFYQN202101012); The Affiliated Hospital of Qingdao University Clinical Medicine+X Research Project (QDFY+X2023141; QDFY+X202101054).
Conflict of interest
The authors declare that the research was conducted in the absence of any commercial or financial relationships that could be construed as a potential conflict of interest.
Publisher’s note
All claims expressed in this article are solely those of the authors and do not necessarily represent those of their affiliated organizations, or those of the publisher, the editors and the reviewers. Any product that may be evaluated in this article, or claim that may be made by its manufacturer, is not guaranteed or endorsed by the publisher.
References
1. Yang L, Yang J, Kleppe A, Danielsen HE, Kerr DJ. Personalizing adjuvant therapy for patients with colorectal cancer. Nat Rev Clin Oncol. (2024) 21:67–79. doi: 10.1038/s41571-023-00834-2
2. Fitzgerald KN, Motzer RJ, Lee C-H. Adjuvant therapy options in renal cell carcinoma — targeting the metastatic cascade. Nat Rev Urol. (2023) 20:179–93. doi: 10.1038/s41585-022-00666-2
3. Leow JJ, Ray S, Dason S, Singer EA, Chang SL. The promise of neoadjuvant and adjuvant therapies for renal cancer. Urologic Clinics North America. (2023) 50:285–303. doi: 10.1016/j.ucl.2023.01.011
4. Kuo Y-H, Lin Y-T, Ho C-H, Chou C-L, Cheng L-C, Tsai C-J, et al. Adjuvant chemotherapy and survival outcomes in rectal cancer patients with good response (ypT0-2N0) after neoadjuvant chemoradiotherapy and surgery: A retrospective nationwide analysis. Front Oncol. (2022) 12:1087778. doi: 10.3389/fonc.2022.1087778
5. Zha S, Li T, Zheng Q, Li L. Whether patients with stage II/III Colorectal cancer benefit from adjuvant chemotherapy: A modeling analysis of literature aggregate data. Front Pharmacol. (2022) 13:826785. doi: 10.3389/fphar.2022.826785
6. Smith W, Purvis I, Bomstad C, Labak C, Velpula K, Tsung A, et al. Therapeutic targeting of immune checkpoints with small molecule inhibitors. Am J Trans Res. (2019) 11:529–41.
7. Shuyu J, Zhuo S-H, Takemoto Y, Li Y-M, Uesugi M. Self-assembling small-molecule adjuvants as antigen nano-carriers. Chem Commun. (2022) 58:12228–31. doi: 10.1039/D2CC05016A
8. Moser B, Escalante-Buendia Y, Steinhardt R, Rosenberger M, Cassaidy B, Naorem N, et al. Small molecule NF-κB inhibitors as immune potentiators for enhancement of vaccine adjuvants. Front Immunol. (2020) 11:511513. doi: 10.3389/fimmu.2020.511513
9. Schlicher L, Green L, Romagnani A, Renner F. Small molecule inhibitors for cancer immunotherapy and associated biomarkers – the current status. Front Immunol. (2023) 14:1297175. doi: 10.3389/fimmu.2023.1297175
10. Zhang J, Yu J, Liu M, Xie Z, Lei X, Yang X, et al. Small-molecule modulators of tumor immune microenvironment. Bioorganic Chem. (2024) 145:107251. doi: 10.1016/j.bioorg.2024.107251
11. Tsukidate T, Hespen CW, Hang HC. Small molecule modulators of immune pattern recognition receptors††Electronic supplementary information (ESI) available. RSC Chem Biol. (2023) 4:1014–36. doi: 10.1039/D3CB00096F
12. Yu L, Sun M, Zhang Q, Zhou Q, Wang Y. Harnessing the immune system by targeting immune checkpoints: Providing new hope for Oncotherapy. Front Immunol. (2022) 13:982026. doi: 10.3389/fimmu.2022.982026
13. Kubli SP, Berger T, Araujo DV, Siu LL, Mak TW. Beyond immune checkpoint blockade: emerging immunological strategies. Nat Rev Drug Discovery. (2021) 20:899–919. doi: 10.1038/s41573-021-00155-y
14. Graziani G, Tentori L, Navarra P. Ipilimumab: A novel immunostimulatory monoclonal antibody for the treatment of cancer. Pharmacol Res. (2012) 65:9–22. doi: 10.1016/j.phrs.2011.09.002
15. Liu N, Zhang R, Shi Q, Jiang H, Zhou Q. Intelligent delivery system targeting PD-1/PD-L1 pathway for cancer immunotherapy. Bioorganic Chem. (2023) 136:106550. doi: 10.1016/j.bioorg.2023.106550
16. Awadasseid A, Wu Y, Zhang W. Advance investigation on synthetic small-molecule inhibitors targeting PD-1/PD-L1 signaling pathway. Life Sci. (2021) 282:119813. doi: 10.1016/j.lfs.2021.119813
17. Chen T, Gao C. Innate immune signal transduction pathways to fungal infection: Components and regulation. Cell Insight. (2024) 3:100154. doi: 10.1016/j.cellin.2024.100154
18. Zhu M, Sun Y, Bai H, Wang Y, Yang B, Wang Q, et al. Effects of saponins from Chinese herbal medicines on signal transduction pathways in cancer: A review. Front Pharmacol. (2023) 14:1159985. doi: 10.3389/fphar.2023.1159985
19. Wylaź M, Kaczmarska A, Pajor D, Hryniewicki M, Gil D, Dulińska-Litewka J. Exploring the role of PI3K/AKT/mTOR inhibitors in hormone-related cancers: A focus on breast and prostate cancer. Biomedicine Pharmacotherapy. (2023) 168:115676. doi: 10.1016/j.biopha.2023.115676
20. Itamura H, Muranushi H, Shindo T, Kitaura K, Okada S, Shin-I T, et al. The MEK inhibitor trametinib enhances diverse T cell reconstitution with suppressing xenogeneic graft-versus-host-disease. Blood. (2019) 134:1929. doi: 10.1182/blood-2019-128913
21. Oikawa D, Gi M, Kosako H, Shimizu K, Takahashi H, Shiota M, et al. OTUD1 deubiquitinase regulates NF-κB- and KEAP1-mediated inflammatory responses and reactive oxygen species-associated cell death pathways. Cell Death Dis. (2022) 13:694. doi: 10.1038/s41419-022-05145-5
22. Yang M, Mu Y, Yu X, Gao D, Zhang W, Li Y, et al. Survival strategies: How tumor hypoxia microenvironment orchestrates angiogenesis. Biomedicine Pharmacotherapy. (2024) 176:116783. doi: 10.1016/j.biopha.2024.116783
23. Joyce JA, Pollard JW. Microenvironmental regulation of metastasis. Nat Rev Cancer. (2009) 9:239–52. doi: 10.1038/nrc2618
24. Jain RK. Normalizing tumor microenvironment to treat cancer: bench to bedside to biomarkers. J Clin Oncol. (2013) 31:2205–18. doi: 10.1200/JCO.2012.46.3653
25. Griffioen AW, Molema G. Angiogenesis: potentials for pharmacologic intervention in the treatment of cancer, cardiovascular diseases, and chronic inflammation. Pharmacol Rev. (2000) 52:237–68.
26. Zhang S, Xu Q, Sun W, Zhou J, Zhou J. Immunomodulatory effects of CDK4/6 inhibitors. Biochim Biophys Acta (BBA) - Rev Cancer. (2023) 1878:188912. doi: 10.1016/j.bbcan.2023.188912
27. Gupta M, Chandan K, Sarwat M. Natural products and their derivatives as immune check point inhibitors: Targeting cytokine/chemokine signalling in cancer. Semin Cancer Biol. (2022) 86:214–32. doi: 10.1016/j.semcancer.2022.06.009
28. Atkins M, Plimack E, Puzanov I, Fishman M, McDermott D, Cho D, et al. Axitinib in combination with pembrolizumab in patients with advanced renal cell cancer: a non-randomised, open-label, dose-finding, and dose-expansion phase 1b trial. Lancet Oncol. (2018) 19:405–15. doi: 10.1016/S1470-2045(18)30081-0
29. Furman R, Sharman J, Coutre S, Cheson B, Pagel J, Hillmen P, et al. Idelalisib and rituximab in relapsed chronic lymphocytic leukemia. New Engl J Med. (2014) 370:997–1007. doi: 10.1056/NEJMoa1315226
30. Wang M, Liu Y, Cheng Y, Wei Y, Wei X. Immune checkpoint blockade and its combination therapy with small-molecule inhibitors for cancer treatment. Biochim Biophys Acta (BBA) - Rev Cancer. (2019) 1871:199–224. doi: 10.1016/j.bbcan.2018.12.002
31. Guo R, Li J, Hu J, Fu Q, Yan Y, Xu S, et al. Combination of epidrugs with immune checkpoint inhibitors in cancer immunotherapy: From theory to therapy. Int Immunopharmacol. (2023) 120:110417. doi: 10.1016/j.intimp.2023.110417
32. Zhao M, Yan C-Y, Wei Y-N, Zhao X-H. Breaking the mold: Overcoming resistance to immune checkpoint inhibitors. Antiviral Res. (2023) 219:105720. doi: 10.1016/j.antiviral.2023.105720
33. Yang J, Yu Y-C, Wang Z-X, Li Q-Q, Ding N, Leng X-J, et al. Research strategies of small molecules as chemotherapeutics to overcome multiple myeloma resistance. Eur J Medicinal Chem. (2024) 271:116435. doi: 10.1016/j.ejmech.2024.116435
34. Choi D, Kang TG, Kim T, Moon C-W, Choi M, Kim D-H, et al. Immortalized nanodendritic cells decorated with immune-checkpoint inhibitors for personalized cancer immunotherapy. Nano Today. (2024) 56:102237. doi: 10.1016/j.nantod.2024.102237
35. Chen Z, John J, Wang J. Why responses to immune checkpoint inhibitors are heterogeneous in head and neck cancers: Contributions from tumor-intrinsic and host-intrinsic factors. Front Oncol. (2022) 12:995434. doi: 10.3389/fonc.2022.995434
36. Wei S, Duffy C, Allison J. Fundamental mechanisms of immune checkpoint blockade therapy. Cancer Discovery. (2018) 8:1069–86. doi: 10.1158/2159-8290.CD-18-0367
37. Sharma P, Allison J. Immune checkpoint targeting in cancer therapy: toward combination strategies with curative potential. Cell. (2015) 161:205–14. doi: 10.1016/j.cell.2015.03.030
38. Ribas A, Wolchok JD. Cancer immunotherapy using checkpoint blockade. Science. (2018) 359:1350–5. doi: 10.1126/science.aar4060
39. Colley A, Brauns T, Sluder AE, Poznansky MC, Gemechu Y. Immunomodulatory drugs: a promising clinical ally for cancer immunotherapy. Trends Mol Med. (2024). doi: 10.1016/j.molmed.2024.05.001
40. Kim A, Cohen M. The discovery of vemurafenib for the treatment of BRAF-mutated metastatic melanoma. Expert Opin Drug Discovery. (2016) 11:907–16. doi: 10.1080/17460441.2016.1201057
41. Hořínková J, Šíma M, Slanar O. Pharmacokinetics of dasatinib. Prague Med Rep. (2019) 120:52–63. doi: 10.14712/23362936.2019.10
42. Li L, Manzhi Z, Kiernan C, Eiro M, Meurs M, Brouwers-Haspels I, et al. Ibrutinib directly reduces CD8+T cell exhaustion independent of BTK. Front Immunol. (2023) 14:1201415. doi: 10.3389/fimmu.2023.1201415
43. AboulMagd AM, Abdelwahab NS. Analysis of sunitinib malate, a multi-targeted tyrosine kinase inhibitor: A critical review. Microchemical J. (2021) 163:105926. doi: 10.1016/j.microc.2021.105926
44. Ranieri G, Mammì M, Donato Di Paola E, Russo E, Gallelli L, Citraro R, et al. Pazopanib a tyrosine kinase inhibitor with strong anti-angiogenetic activity: A new treatment for metastatic soft tissue sarcoma. Crit Rev Oncology/Hematology. (2014) 89:322–9. doi: 10.1016/j.critrevonc.2013.08.012
45. Ran F, Liu Y, Wang C, Xu Z, Zhang Y, Liu Y, et al. Review of the development of BTK inhibitors in overcoming the clinical limitations of ibrutinib. Eur J Medicinal Chem. (2022) 229:114009. doi: 10.1016/j.ejmech.2021.114009
46. Wu J, Zhang M, Liu D. Acalabrutinib (ACP-196): A selective second-generation BTK inhibitor. J Hematol Oncol. (2016) 9:21. doi: 10.1186/s13045-016-0250-9
47. Maroto P, Porta C, Capdevila J, Apolo A, Viteri S, Rodriguez-Antona C, et al. Cabozantinib for the treatment of solid tumors: a systematic review. Ther Adv Med Oncol. (2022) 14:175883592211071. doi: 10.1177/17588359221107112
48. Frampton J. Pazopanib: a review in advanced renal cell carcinoma. Targeted Oncol. (2017) 12:543–54. doi: 10.1007/s11523-017-0511-8
49. Zhang K, Zhang Q, Jia R, Xiang S, Xu L. A comprehensive review of the relationship between autophagy and sorafenib-resistance in hepatocellular carcinoma: ferroptosis is noteworthy. Front Cell Dev Biol. (2023) 11:1156383. doi: 10.3389/fcell.2023.1156383
50. Ye M, Zhang X, Li N, Zhang Y, Jing P, Chang N, et al. ALK and ROS1 as targeted therapy paradigms and clinical implications to overcome crizotinib resistance. Oncotarget. (2016) 7:12289–304. doi: 10.18632/oncotarget.v7i11
51. Li X, Wang Z, Chen C, Yang F, Liu P, Fang S, et al. A small-molecule degrader selectively inhibits the growth of ALK-rearranged lung cancer with ceritinib resistance. iScience. (2024) 27:109015. doi: 10.1016/j.isci.2024.109015
52. Cheung JM, Kang J, Yeap BY, Peterson JL, Do A, Gainor JF, et al. Efficacy and safety of dose-escalated alectinib in patients with metastatic ALK-positive NSCLC and central nervous system relapse on standard-dose alectinib. JTO Clin Res Rep. (2024) 5:100645. doi: 10.1016/j.jtocrr.2024.100645
53. Mezquita L, Planchard D. The role of brigatinib in crizotinib-resistant non-small cell lung cancer. Cancer Manage Res. (2018) 10:123–30. doi: 10.2147/CMAR
54. Dörfel D, Lechner C, Joas S, Funk T, Gutknecht M, Salih J, et al. The BCR-ABL inhibitor nilotinib influences phenotype and function of monocyte-derived human dendritic cells. Cancer Immunology Immunotherapy. (2018) 67:775–83. doi: 10.1007/s00262-018-2129-9
55. Liu M, Xiao K, Yang L. EGFR inhibitor erlotinib plus monoclonal antibody versus erlotinib alone for first-line treatment of advanced non-small cell lung carcinoma: A systematic review and meta-analysis. Int Immunopharmacol. (2023) 119:110001. doi: 10.1016/j.intimp.2023.110001
56. Singh S, Sadhukhan S, Sonawane A. 20 years since the approval of first EGFR-TKI, gefitinib: Insight and foresight. Biochim Biophys Acta (BBA) - Rev Cancer. (2023) 1878:188967. doi: 10.1016/j.bbcan.2023.188967
57. Masood A, Kancha RK, Subramanian J. Epidermal growth factor receptor (EGFR) tyrosine kinase inhibitors in non-small cell lung cancer harboring uncommon EGFR mutations: Focus on afatinib. Semin Oncol. (2019) 46:271–83. doi: 10.1053/j.seminoncol.2019.08.004
58. Nakagawa N, Miyake N, Ochi N, Yamane H, Takeyama M, Nagasaki Y, et al. Targeting ROR1 in combination with osimertinib in EGFR mutant lung cancer cells. Exp Cell Res. (2021) 409:112940. doi: 10.1016/j.yexcr.2021.112940
59. Zhou V, Moat L, Yu M, Parashar D, Shour A, Zhang L, et al. Inhibiting FOXM1 sensitizes myeloma cells to BCL2 inhibitor venetoclax by repressing MYC pathway. Blood. (2023) 142:1950. doi: 10.1182/blood-2023-180268
60. Binder A, Walker C, Mark T, Baljevic M. Impacting T-cell fitness in multiple myeloma: potential roles for selinexor and XPO1 inhibitors. Front Immunol. (2023) 14:1275329. doi: 10.3389/fimmu.2023.1275329
61. Savas P, Lo L, Luen S, Blackley E, Callahan J, Moodie K, et al. Alpelisib monotherapy for PI3K-altered, pretreated advanced breast cancer: A phase II study. Cancer Discovery. (2022) 12:2058–73. doi: 10.1158/2159-8290.CD-21-1696
62. Khan Z, Real A, Marsiglia W, Chow A, Duffy M, Yerabolu JR, et al. Structural basis for the action of the drug trametinib at KSR-bound MEK. Nature. (2020) 588:509–14. doi: 10.1038/s41586-020-2760-4
63. Indini A, Tondini C, Mandala M. Cobimetinib in Malignant melanoma: How to MEK an impact on long-term survival. Future Oncol. (2019) 15:967–77. doi: 10.2217/fon-2018-0659
64. Long G, Hauschild A, Santinami M, Atkinson V, Mandala M, Chiarion-Sileni V, et al. Adjuvant dabrafenib plus trametinib in stage III BRAF -mutated melanoma. New Engl J Med. (2017) 377:1813–23. doi: 10.1056/NEJMoa1708539
65. Nath A, Cosgrove P, Chang J, Bild A. Predicting clinical response to everolimus in ER+ breast cancers using machine-learning. Front Mol Biosci. (2022) 9:981962. doi: 10.3389/fmolb.2022.981962
66. Huang S, Du K, Liu Z, Li J. Inhibition of mTOR by temsirolimus overcomes radio-resistance in nasopharyngeal carcinoma. Clin Exp Pharmacol Physiol. (2022) 49:703–9. doi: 10.1111/1440-1681.13649
67. Keenan C, Nichols K, Albeituni S. Use of the JAK inhibitor ruxolitinib in the treatment of hemophagocytic lymphohistiocytosis. Front Immunol. (2021) 12:614704. doi: 10.3389/fimmu.2021.614704
68. Liu E, Aslam N, Nigam G, Limdi J. Tofacitinib and newer JAK inhibitors in inflammatory bowel disease—where we are and where we are going. Drugs Context. (2022) 11:1–17. doi: 10.7573/17404398
69. El Hachem G, Gombos A. Abemaciclib, a third CDK 4/6 inhibitor for the treatment of hormone receptor-positive, human epidermal growth factor receptor 2-negative advanced or metastatic breast cancer. Expert Rev Anticancer Ther. (2020) 21:81–92. doi: 10.1080/14737140.2020.1834385
70. Woyach J, Furman R, Liu T-M, Ozer H, Zapatka M, Ruppert A, et al. Resistance mechanisms for the bruton’s tyrosine kinase inhibitor ibrutinib. New Engl J Med. (2014) 370:2286–94. doi: 10.1056/NEJMoa1400029
71. Lin A, Giuliano C, Palladino A, John K, Abramowicz C, Yuan M, et al. Off-target toxicity is a common mechanism of action of cancer drugs undergoing clinical trials. Sci Trans Med. (2019) 11:eaaw8412. doi: 10.1126/scitranslmed.aaw8412
72. Chu T, Rupnick M, Kerkela R, Dallabrida S, Zurakowski D, Nguyen L, et al. Cardiotoxicity associated with tyrosine kinase inhibitor sunitinib. Lancet. (2007) 370:2011–9. doi: 10.1016/S0140-6736(07)61865-0
73. Mino-Kenudson M, Schalper K, Cooper W, Dacic S, Hirsch F, Jain D, et al. Predictive biomarkers for immunotherapy in lung cancer: perspective from the IASLC pathology committee. J Thorac Oncol. (2022) 17:1335–54. doi: 10.1016/j.jtho.2022.09.109
74. Topalian S, Taube J, Anders R, Pardoll D. Mechanism-driven biomarkers to guide immune checkpoint blockade in cancer therapy. Nat Rev Cancer. (2016) 16:275–87. doi: 10.1038/nrc.2016.36
75. Ramalingam SS, Yang JCH, Lee CK, Kurata T, Kim D-W, John T, et al. Osimertinib as first-line treatment of EGFR mutation–positive advanced non–small-cell lung cancer. J Clin Oncol. (2017) 36:841–9.
76. Apolo AB, Infante JR, Balmanoukian A, Patel MR, Wang D, Kelly K, et al. Avelumab, an anti–programmed death-ligand 1 antibody, in patients with refractory metastatic urothelial carcinoma: results from a multicenter, phase ib study. J Clin Oncol. (2017) 35:2117–24. doi: 10.1200/JCO.2016.71.6795
Keywords: adjuvant, adjuvant therapy, neoadjuvant therapy, anti-tumor drug, cancer immunotherapy, small molecule inhibitor
Citation: Wu X, Feng N, Wang C, Jiang H and Guo Z (2024) Small molecule inhibitors as adjuvants in cancer immunotherapy: enhancing efficacy and overcoming resistance. Front. Immunol. 15:1444452. doi: 10.3389/fimmu.2024.1444452
Received: 14 June 2024; Accepted: 22 July 2024;
Published: 05 August 2024.
Edited by:
Zong Sheng Guo, University at Buffalo, United StatesCopyright © 2024 Wu, Feng, Wang, Jiang and Guo. This is an open-access article distributed under the terms of the Creative Commons Attribution License (CC BY). The use, distribution or reproduction in other forums is permitted, provided the original author(s) and the copyright owner(s) are credited and that the original publication in this journal is cited, in accordance with accepted academic practice. No use, distribution or reproduction is permitted which does not comply with these terms.
*Correspondence: Hongfei Jiang, amlhbmdob25nZmVpQHFkdS5lZHUuY24=; Zhu Guo, Z3Vvemh1Z3pAMTI2LmNvbQ==