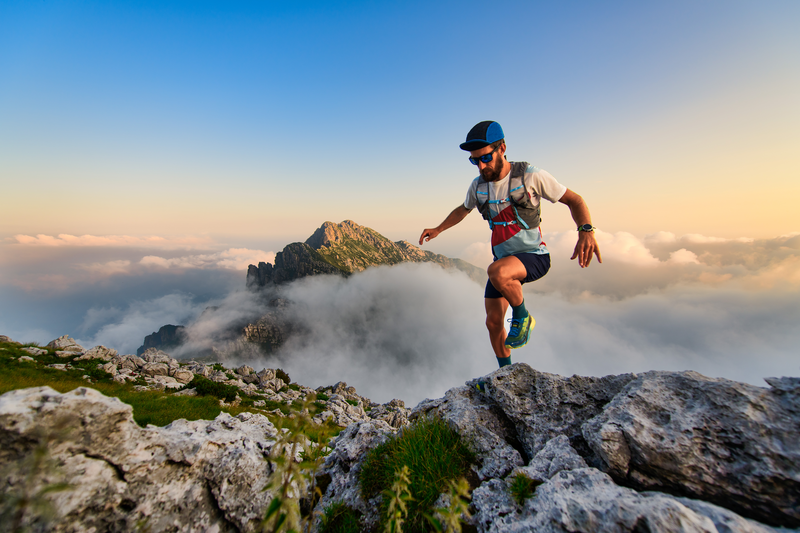
95% of researchers rate our articles as excellent or good
Learn more about the work of our research integrity team to safeguard the quality of each article we publish.
Find out more
OPINION article
Front. Immunol. , 12 August 2024
Sec. Cancer Immunity and Immunotherapy
Volume 15 - 2024 | https://doi.org/10.3389/fimmu.2024.1443726
A tumor antigen must be sufficiently immunogenic to induce antibody production, and immunogenicity is determined by the strength of presentation by major histocompatibility complex (MHC)-encoded histocompatibility antigens. Not each peptide has sufficiently strong affinity to each MHC antigen, and therefore antigen presentation and subsequent immune response is dependent on the histocompatibility antigen present in the host animal. By increasing the likelihood of effective presentation and resulting immune response, heterozygosity for histocompatibility antigens enhances the chances for protection from infectious diseases and cancer as originally shown in animal models (1) and also in humans (2). Here, we want to highlight these facts for the benefit of scientists who want to improve the efficiency of their monoclonal antibody development programs using immunization of animals followed by hybridoma technology.
Immunization of laboratory animals, usually mice, with known or yet unknown immunogens, like cancer antigens, is the most common source of monoclonal antibodies to use for diagnostic or therapeutic purposes (3, 4). Once the animals produce monoclonal antibodies, the production at scale is most commonly achieved by the hybridoma technology (5). This is not a fail-proof method and a lot of modifications have been described, resulting in different immunization protocols that aim to stimulate the immune response in animals (6). The focus has been to increase the immunogenicity of antigens (7) and with success (8).
The production of antibodies, however, could also be enhanced at the source by careful choice of the animals used. It has been a convention to use BALB/c mice because of its unique superiority in mounting immune response to immunological challenges (9), mainly due to being a Th2-type strain (10), which is particularly attractive if the aim is to induce humoral immune response. However strong the capacity of producing antibodies may be, a major determinant of antibody response is antigen presentation, which is governed by the MHC loci (H-2 in mice and HLA in humans) (11, 12). What remains unappreciated is that being an inbred mouse strain, BALB/c mice are homozygous at the entire H-2 haplotype (H-2d haplotype), i.e, in each and every H-2 locus. This means that BALB/c mice, even though equipped with a strong humoral immune (antibody) response capacity, are restricted only to respond to antigens that can be presented by the H-2 loci of the H-2d haplotype. A review of the development of all FDA approved therapeutic monoclonal antibodies has revealed that the majority are mouse antibodies developed using hybridoma technology which predominantly immunizes BALB/c mice to generate antibodies (13–16). A selection of such antibodies developed with hybridoma technology are presented in Table 1. The approach to immunize mice from different mouse strains to enhance the diversity of the resulting antibody repertoire has not been universally adopted although there are anecdotal examples in response to pathogens and vaccine development (9, 19, 20). This means we have only recorded success for maybe a small fraction of potential antibodies which could have been generated if multiple strains of mice with different H-2 haplotypes were used or even better, a set of H-2-congenic BALB/c mice were used, which are readily available (21). Another approach would be the use of obligatory heterozygote progeny of inbred mice (like the F1 generation of BALB/c and C57BL matings (21) or even progeny of H-2-congenic BALB/c mice to benefit from Th-2-dominant immune response in BALB/c, and the fact that many of the myeloma cells used for fusion to develop hybridomas have the same BALB/c origin (14).
Table 1. A selection of FDA / EMA-approved Monoclonal Antibodies Identified Using BALB/c Mice Immunisation and Hybridoma Technologya.
The argument above may be extended to genetically engineered mouse models (GEMMs), which use knock-out/transgene method to replace the genes for immunoglobulins with human counterparts to produce humanized monoclonal antibodies upon immunization (22, 23). GEMMs are transgenic mice with humanized humoral immune systems (24). They have been used for production of immunomodulatory monoclonal antibodies as those used in cancer immunotherapy or autoimmune disease treatments (17), and anti-viral antibody production (25). Seven human mAbs developed using GEMMs are FDA approved (17). Even though, GEMMs are not frequently used for novel antigen discovery, most of these genetically humanized mouse models are derived from the C57BL/6 strain due to its well-characterized genome, robust immune response, and suitability for genetic engineering. However, some platforms may use BALB/c or a combination of strains to leverage specific characteristics beneficial for producing human antibodies. Both these strains are inbred mice and homozygous for H-2 haplotypes. This means the same arguments regarding the likelihood of presentation of novel antigens by H-2 also apply to GEMMs if these mice are used to develop monoclonal antibodies against novel cancer antigens.
It appears that there is a missed opportunity to increase the chances of obtaining many more monoclonal antibodies with potential to be used in diagnostic and therapeutic fields not only in cancer but also in vaccine development. By using congenic strains of BALB/c with different H-2 haplotypes (H-2d, H-2b, H-2k), an expanded antigen presentation capacity would naturally result in a large number of new monoclonal antibodies to be identified for clinical use. Most of the currently FDA-approved monoclonal antibodies have been produced by hybridoma technology (17), and mainly for cancer treatment (4) using H-2 haplotype homozygous mice. It is quite possible that many novel cancer antigens may have been missed due to lack of their presentation by the H-2 antigens of the inbred mice used. The bottom line is that techniques that used a single strain of inbred mice homozygous for an H-2 haplotype have a limited potential to discover novel antigens and many more antibodies could have been discovered to be tested for diagnostic and therapeutic potential.
The untapped potential highlighted in this paper may be exploited to identify many more cancer-specific monoclonal antibodies that may have been missed before by taking different approaches. These may be repeating the same experiments with immunologically more diverse mice or set of mice, or trying some of the more modern approaches that do not depend on hybridoma technology (17).
EC: Conceptualization, Writing – original draft, Writing – review & editing. MD: Supervision, Writing – original draft, Writing – review & editing, Conceptualization.
The author(s) declare that no financial support was received for the research, authorship, and/or publication of this article.
The authors declare that the research was conducted in the absence of any commercial or financial relationships that could be construed as a potential conflict of interest.
All claims expressed in this article are solely those of the authors and do not necessarily represent those of their affiliated organizations, or those of the publisher, the editors and the reviewers. Any product that may be evaluated in this article, or claim that may be made by its manufacturer, is not guaranteed or endorsed by the publisher.
1. Doherty PC, Zinkernagel RM. Enhanced immunological surveillance in mice heterozygous at the H-2 gene complex. Nature. (1975) 256:50–2. doi: 10.1038/256050a0
2. Wang QL, Wang TM, Deng CM, Zhang WL, He YQ, Xue WQ, et al. Association of HLA diversity with the risk of 25 cancers in the UK Biobank. EBioMedicine. (2023) 92:104588. doi: 10.1016/j.ebiom.2023.104588
3. Muhsin A, Rangel R, Vien L, Bover L. Monoclonal antibodies generation: updates and protocols on hybridoma technology. Methods Mol Biol. (2022) 2435:73–93. doi: 10.1007/978-1-0716-2014-4_6
4. Delgado M, Garcia-Sanz JA. Therapeutic monoclonal antibodies against cancer: present and future. Cells. (2023) 12(24):2837. doi: 10.3390/cells12242837
5. Moraes JZ, Hamaguchi B, Braggion C, Speciale ER, Cesar FBV, Soares G, et al. Hybridoma technology: is it still useful? Curr Res Immunol. (2021) 2:32–40. doi: 10.1016/j.crimmu.2021.03.002
6. Asensio MA, Lim YW, Wayham N, Stadtmiller K, Edgar RC, Leong J, et al. Antibody repertoire analysis of mouse immunization protocols using microfluidics and molecular genomics. MAbs. (2019) 11:870–83. doi: 10.1080/19420862.2019.1583995
7. de Almeida R, Nakamura CN, de Lima Fontes M, Deffune E, Felisbino SL, Kaneno R, et al. Enhanced immunization techniques to obtain highly specific monoclonal antibodies. MAbs. (2018) 10:46–54. doi: 10.1080/19420862.2017.1331804
8. Phakham T, Boonkrai C, Wongtangprasert T, Audomsun T, Attakitbancha C, Saelao P, et al. Highly efficient hybridoma generation and screening strategy for anti-PD-1 monoclonal antibody development. Sci Rep. (2022) 12:17792. doi: 10.1038/s41598-022-20560-6
9. Zhang Q, Zhu W, Zou Z, Yu W, Gao P, Wang Y, et al. A preliminary study in immune response of BALB/c and C57BL/6 mice with a locally allergic rhinitis model. Am J Rhinol Allergy. (2023) 37:410–8. doi: 10.1177/19458924231157619
10. Watanabe H, Numata K, Ito T, Takagi K, Matsukawa A. Innate immune response in Th1- and Th2-dominant mouse strains. Shock. (2004) 22:460–6. doi: 10.1097/01.shk.0000142249.08135.e9
11. Benacerraf B, Katz DH. The histocompatibility-linked immune response genes. Adv Cancer Res. (1975) 21:121–73. doi: 10.1016/S0065-230X(08)60972-0
12. Pishesha N, Harmand TJ, Ploegh HL. A guide to antigen processing and presentation. Nat Rev Immunol. (2022) 22:751–64. doi: 10.1038/s41577-022-00707-2
13. Kohler G, Milstein C. Continuous cultures of fused cells secreting antibody of predefined specificity. Nature. (1975) 256:495–7. doi: 10.1038/256495a0
14. Leenaars M, Hendriksen CF. Critical steps in the production of polyclonal and monoclonal antibodies: evaluation and recommendations. ILAR J. (2005) 46:269–79. doi: 10.1093/ilar.46.3.269
15. Holzlohner P, Hanack K. Generation of murine monoclonal antibodies by hybridoma technology. J Vis Exp. (2017) 2(19):54832. doi: 10.3791/54832
16. Parray HA, Shukla S, Samal S, Shrivastava T, Ahmed S, Sharma C, et al. Hybridoma technology a versatile method for isolation of monoclonal antibodies, its applicability across species, limitations, advancement and future perspectives. Int Immunopharmacol. (2020) 85:106639. doi: 10.1016/j.intimp.2020.106639
17. Singh R, Chandley P, Rohatgi S. Recent advances in the development of monoclonal antibodies and next-generation antibodies. Immunohorizons. (2023) 7:886–97. doi: 10.4049/immunohorizons.2300102
18. Lu RM, Hwang YC, Liu IJ, Lee CC, Tsai HZ, Li HJ, et al. Development of therapeutic antibodies for the treatment of diseases. J BioMed Sci. (2020) 27:1. doi: 10.1186/s12929-019-0592-z
19. Banic DM, Delplace P, Mazingue C, Camus D. H-2b restriction of the immune response to the p126 Plasmodium falciparum antigen. Clin Exp Immunol. (1994) 95:472–8. doi: 10.1111/j.1365-2249.1994.tb07021.x
20. Linke S, Neubauer K, Dorner MB, Dorner BG, Pauli G, Schweiger B. Generation and characterisation of monoclonal antibodies against influenza virus A, subtype H5N1. J Virol Methods. (2011) 175:85–94. doi: 10.1016/j.jviromet.2011.04.025
21. Asherson GL, Dieli F, Gautam Y, Siew LK, Zembala M. Major histocompatibility complex regulation of the class of the immune response: the H-2d haplotype determines poor interferon-gamma response to several antigens. Eur J Immunol. (1990) 20:1305–10. doi: 10.1002/eji.1830200616
22. Scheer N, Snaith M, Wolf CR, Seibler J. Generation and utility of genetically humanized mouse models. Drug Discov Today. (2013) 18:1200–11. doi: 10.1016/j.drudis.2013.07.007
23. Macdonald LE, Karow M, Stevens S, Auerbach W, Poueymirou WT, Yasenchak J, et al. Precise and in situ genetic humanization of 6 Mb of mouse immunoglobulin genes. Proc Natl Acad Sci U S A. (2014) 111:5147–52. doi: 10.1073/pnas.1323896111
24. Bruggemann M, Osborn MJ, Ma B, Hayre J, Avis S, Lundstrom B, et al. Human antibody production in transgenic animals. Arch Immunol Ther Exp (Warsz). (2015) 63:101–8. doi: 10.1007/s00005-014-0322-x
Keywords: monoclonal antibody development, mice, BALB/c, hybridoma, histocompatibility, MHC restriction, antigen presentation, Th2 immune response
Citation: Chakravarty E and Dorak MT (2024) Missed opportunities to increase efficiency of monoclonal antibody development using hybridoma technology and mice as the source animal. Front. Immunol. 15:1443726. doi: 10.3389/fimmu.2024.1443726
Received: 04 June 2024; Accepted: 25 July 2024;
Published: 12 August 2024.
Edited by:
Jose A. Garcia-Sanz, Spanish National Research Council (CSIC), SpainReviewed by:
Andrew Buchanan, AstraZeneca, United KingdomCopyright © 2024 Chakravarty and Dorak. This is an open-access article distributed under the terms of the Creative Commons Attribution License (CC BY). The use, distribution or reproduction in other forums is permitted, provided the original author(s) and the copyright owner(s) are credited and that the original publication in this journal is cited, in accordance with accepted academic practice. No use, distribution or reproduction is permitted which does not comply with these terms.
*Correspondence: Esha Chakravarty, azEzMzc0NzdAa2luZ3N0b24uYWMudWs=
Disclaimer: All claims expressed in this article are solely those of the authors and do not necessarily represent those of their affiliated organizations, or those of the publisher, the editors and the reviewers. Any product that may be evaluated in this article or claim that may be made by its manufacturer is not guaranteed or endorsed by the publisher.
Research integrity at Frontiers
Learn more about the work of our research integrity team to safeguard the quality of each article we publish.