- 1The School of Pediatrics, Hengyang Medical School, University of South China (Hunan Children’s Hospital), Hengyang, China
- 2Pediatrics Research Institute of Hunan Province, Hunan Children’s Hospital, Changsha, China
- 3Department of Neurology, Hunan Children’s Hospital, Changsha, China
- 4Department of Emergency Center, Hunan Children’s Hospital, Changsha, China
Objective: The mechanisms driving the progression of infantile spasms are not well understood. We aimed to investigate the changes and correlations of the gut microbiota, the hypothalamus–pituitary–adrenal (HPA) axis hormones, and the inflammatory cytokines in children with infantile spasms before and after treatment in order to provide a reference for future pathogenesis research.
Methods: Children with infantile spasms who were admitted to our hospital were recruited into the case group. The case group was divided into the pre-treatment group (group A, n = 14), the 2 weeks after treatment group (group B), and the 1 month after treatment group (group C). On the other hand, healthy children with the same sex ratio as the case group were recruited into the control group (group D, n = 14). Three stool and blood samples were collected before treatment, 2 weeks after treatment, and 1 month after treatment. The serum samples were analyzed using cytometric bead array (CBA), enzyme-linked immunosorbent assay (ELISA), and chemiluminescent immunoassay (CLIA) to measure the levels of HPA axis hormones and inflammatory cytokines. The collected stool samples were sequenced using 16S rDNA.
Results: The pre-treatment group demonstrated elevated levels of corticotropin-releasing hormone (CRH), interleukin 2 (IL-2), IL-4, IL-6, and IL-17α, which decreased with treatment. The level of CRH was lower in the effective group than that in the ineffective group. Sutterellaceae was lower in the pre-treatment group than that in the control group. Lachnospiracea_incertae_sedis was positively associated with CRH concentration (p < 0.05). After treatment, Sutterellaceae was negatively associated with IL-2 and TNF-α (p < 0.05).
Conclusion: This study found that imbalance of the gut microbiota may be involved in the pathogenesis of infantile spasms and is related to the response to adrenocorticotropic hormone (ACTH). Lachnospiraceae and Lachnospiracea_incertae_sedis might be involved in the disease onset. Sutterellaceae might have a link to children’s improved health.
1 Introduction
Infantile spasms (IS), also known as West syndrome, is a refractory epilepsy syndrome characterized by clustered nodding, hugging spasm episodes, a hypsarrhythmic pattern in the electroencephalogram (EEG), and neurodevelopmental delay (1). Recently, IS has been reclassified into infantile epileptic spasms syndrome (IESS) to encompass those patients who do not fully meet the criteria for West syndrome (2). This condition primarily affects infants, with an incidence of approximately 2 to 5 per 10,000, predominantly in male infants, and peaks between the ages of 4 and 7 months (3). According to the most recent American Expert Consensus in 2010, adrenocorticotropic hormone (ACTH) and vigabatrin are the most commonly used first-line medications for this condition (4). Other therapeutic approaches, such as glucocorticoids, topiramate, pyridoxine, and a ketogenic diet, are often utilized as initial treatments in resistant or relapsed cases that do not respond to first-line medications (5), although these are generally less effective. It is generally acknowledged that the stress mechanism and the neuroinflammatory mechanism, caused by the disturbance of the hypothalamus–pituitary–adrenal (HPA) axis, are implicated in the pathogenesis of IS (6, 7).
In recent years, there have been increasing studies on the microbiota–gut–brain axis (MGBA) (8, 9), whose mechanism of action involves the gut microbiota being bidirectionally connected to the brain through the relevant pathways of the gut–brain axis (10) and may play a role in the pathogenesis of epilepsy. These pathways include the neuroendocrine (HPA axis) (11), the vagus nerve, the intestinal immune, the neurotransmitter, and the neuromodulator pathways (12, 13). This study focused on how the neuroendocrine and inflammatory response pathways of the gut microbiota affect IS.
The mechanisms driving the progression of IS are not well understood. Therefore, we aimed to investigate the changes and correlations of the gut microbiota, the HPA axis hormones, and the inflammatory cytokines in children with IS before and after treatment in order to provide a reference for future pathogenesis research.
2 Materials and methods
2.1 Subjects
Children with IS who were hospitalized in the Department of Neurology at our hospital and treated with ACTH from February 2021 to December 2021 were recruited into the case group. All children met the IS diagnostic criteria of the 2010 US Expert Consensus (4, 7). The inclusion criteria for the case group were as follows: 1) children diagnosed for the first time and not previously treated with antiepileptic drugs; 2) those who met the diagnostic criteria for IS established by the 2010 US Expert Consensus; and 3) those aged between 1 and 12 months. The exclusion criteria were: 1) severe malnutrition or excess nutrition; 2) history of digestive diseases and infections in the past 2 weeks with antibiotic treatment; 3) those immunocompromised and those who took hormones or immunosuppressants before diagnosis; 4) those with neurological disorders other than intracranial hemorrhage, stroke, and other epilepsy; and 5) patients with hematological malignancies (such as leukemia) that can cause impaired immunity or intracranial tumors that can disrupt hormone secretion or cause secondary epilepsy. The exclusion criteria after 2 weeks and 1 month of treatment were: 1) not receiving ACTH therapy during treatment; 2) with digestive diseases after enrollment; 3) using antibiotics and probiotics after enrollment; and 4) with neurological disorders other than intracranial hemorrhage, stroke, and other epilepsy. The pre-treatment group was defined as group A, the 2 weeks after treatment group was defined as group B, and the 1 month after treatment group was defined as group C. After 2 weeks of treatment, children with basic remission or with more than 50% decrease of daily seizure occurrence were classified as the effective group according to the clinical relief after using ACTH in the case group. Patients with less than a 50% reduction in daily seizure occurrence, or with little or no remission, were classified as the ineffective group (8).
Healthy children aged 1–12 months were recruited as a blank control group (group D) for the collection of single fecal and blood samples. The technology roadmap is shown in Supplementary Figure S1. This study has been reviewed by the ethics committee with number HCHLL-2020-53.
2.2 Methods
2.2.1 Sample and general information collection
Blood samples: The case group had a first blood collection before treatment, a second blood collection 2 weeks after ACTH treatment, followed by a third collection 1 month after the administration of ACTH or other medications. The samples were then centrifuged after collection, with the upper serum retained and frozen in a refrigerator at −80°C for testing.
Stool specimen: Three stool samples were collected before, 2 weeks after, and 1 month after treatment. All fecal samples were collected and immediately frozen in ice boxes before transportation to the laboratory within 30 min and then stored at −80°C.
Basic information: The following data were collected: name, gender, age, date of birth, weight, height, gestational age, birth weight, birth method, birth condition and feeding method, clinical data, type of diagnosis, pattern of seizure, frequency of seizures, duration of each seizure, time of the first onset, and EEG results.
Follow-up of treatment effect: Data on the current status of medication, the clinical efficacy (seizure frequency), and EEG changes were collected.
Drug use: After the diagnosis of IS, all children in the case group were treated with ACTH intravenous fluids and completed a 2-week course of treatment in the first and second weeks of treatment. During weeks 3–4 of treatment, three children continued ACTH therapy, while the remaining children received oral prednisone and other antiepileptic drugs.
2.2.2 Detection of HPA axis hormones and inflammatory cytokines
Cortisol hormone (COR) was measured using a chemiluminescent immunoassay (CLIA). The enzyme-linked immunosorbent assay (ELISA) method was used to measure ACTH and corticotropin-releasing hormone (CRH). The cytometric bead array (CBA) method was used to detect seven cytokines (i.e., IL-2, IL-4, IL-6, IL-10, IL-17α, TNF-α, and IFN-γ).
2.2.3 DNA extraction and high-throughput 16S rDNA gene sequencing
16S rDNA amplicon sequencing was performed by Genesky Biotechnologies Inc. (Shanghai, 201315, China). Briefly, total genomic DNA was extracted using the FastDNA® SPIN Kit for Soil (MP Biomedicals, Santa Ana, CA, USA) according to the manufacturer’s instructions. The integrity of genomic DNA was examined through agarose gel electrophoresis, while its concentration and purity were determined using the NanoDrop 2000 and Qubit 3.0 spectrophotometer. The V4–V5 hypervariable regions of the 16S rDNA gene were amplified with the primers 515F (5'-GTGCCAGCMGCCGCGG-3') and 907R (5'-CCGTCAATTCMTTTR AGTTT-3') and then sequenced using the Illumina MiSeq 6000 platform.
2.2.4 Gut microbial analysis
The raw read sequences were further filtered to remove adapter sequences, primers, and low-quality reads to improve the accuracy of the subsequent analysis.
Alpha diversity was evaluated using abundance and diversity indices. Principal coordinates analysis (PCoA) of beta diversity (based on the Bray–Curtis distance) based on the operational taxonomic unit (OTU) abundance table was performed to evaluate the community composition and structure of the gut microbiota. Linear discriminant analysis effect size (LEfSe) was used to identify the species that are most likely to explain differences between groups with the linear discriminant analysis (LDA) histogram and the cladogram. Metastats analysis compared the samples between groups at different taxonomy levels, which determined the species with significant differences at each taxonomic level. A heat map for Spearman’s rank-sum correlation coefficient was used to evaluate the correlation between the gut microbiota of each group and the levels of inflammatory cytokines and HPA axis hormones.
2.2.5 Statistical analysis
The experimental data were analyzed using SPSS (Version 25) and R software (Version 4.2.3). The measurement data, when normally distributed, were expressed as the mean ± standard deviation (X ± S), with an independent-samples t-test and analysis of variance used to compare differences among groups; otherwise, data were expressed as the median and interquartile range [M (P25–P75)], with the Mann–Whitney test and the Kruskal–Wallis test used to compare differences among groups. Post-hoc multiple tests were used for pairwise comparisons between groups. Categorical data were expressed as percentages, and the comparison differences between two groups were evaluated with the chi-square test. The correlation between the gut microbiota and the levels of inflammatory cytokines and HPA axis hormones was evaluated using Spearman’s rank-sum correlation coefficient. A p-value <0.05 was considered as statistically significant.
3 Results
3.1 Data analysis between the pre-treatment group and the control group
3.1.1 Clinical characteristics of subjects
In this study, 19 children were recruited as cases based on the inclusion criteria, samples from whom were collected before treatment and 2 weeks and 1 month after treatment. During the collection process, two cases were not treated with ACTH, one case was excluded due to infection and antibiotic use during hospitalization, and two cases were lost during follow-up 1 month after treatment. Finally, 14 children with IS were included into the case group. There were 9 children out of the 14 in the case group who had more than three seizures per day, while four children had more than five seizures. Four children had each seizure lasting more than 1 min. There were five children with more than 10 times nodding and/or hugging spasms. There were no significant differences in the general data such as age, gestational age, birth weight, and mode of delivery between the pre-treatment group and the control group (p > 0.05), as shown in Table 1.
3.1.2 Comparison of the HPA axis hormone and inflammatory cytokine levels between the two groups
The analysis revealed that the levels of CRH, IL-2, IL-4, IL-6, and IL-17α in the pre-treatment group were significantly higher than those in the control group (p < 0.05) (Table 2).
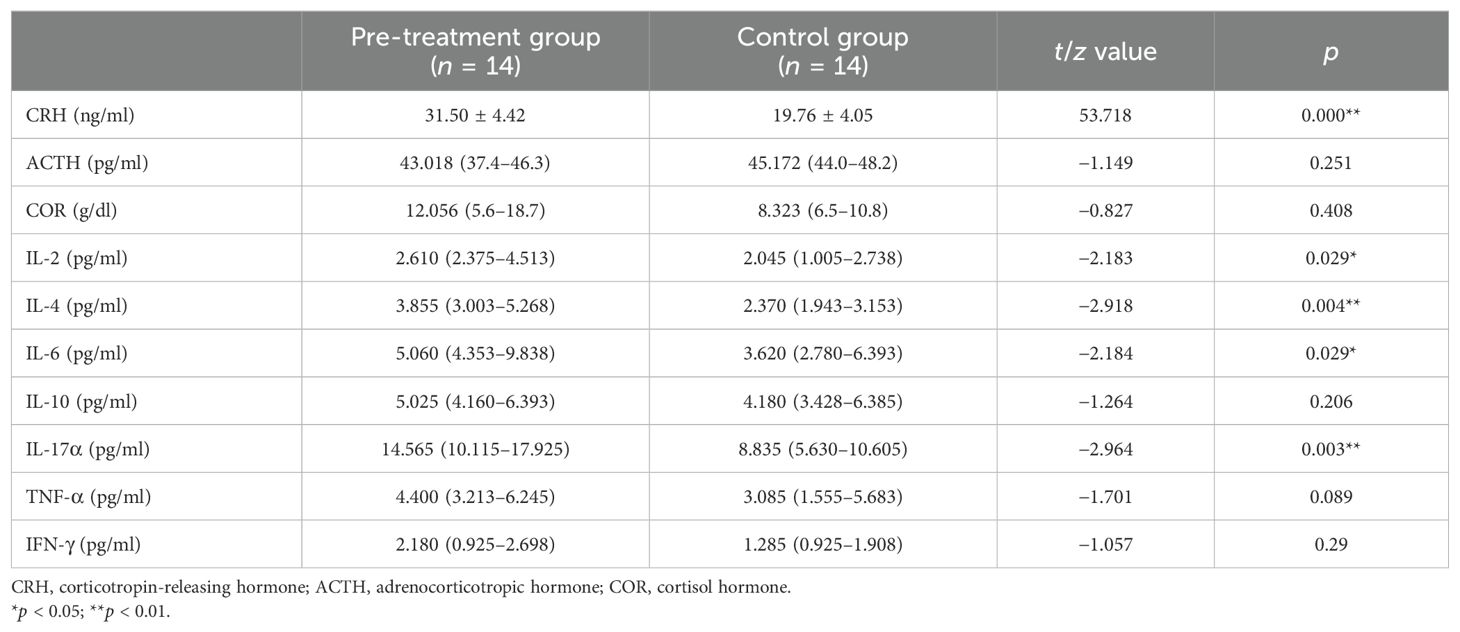
Table 2. Comparison of the levels of the hypothalamus–pituitary–adrenal (HPA) axis hormones and inflammatory cytokines between the pre-treatment and control groups.
3.1.3 Analysis of the gut microbiota differences between the two groups
There were no significant differences in the alpha diversity values between the pre-treatment group and the control group (p > 0.05). The PCoA found that the gut microbiota composition of the pre-treatment group was highly similar to that of the control group (Figures 1A, B).
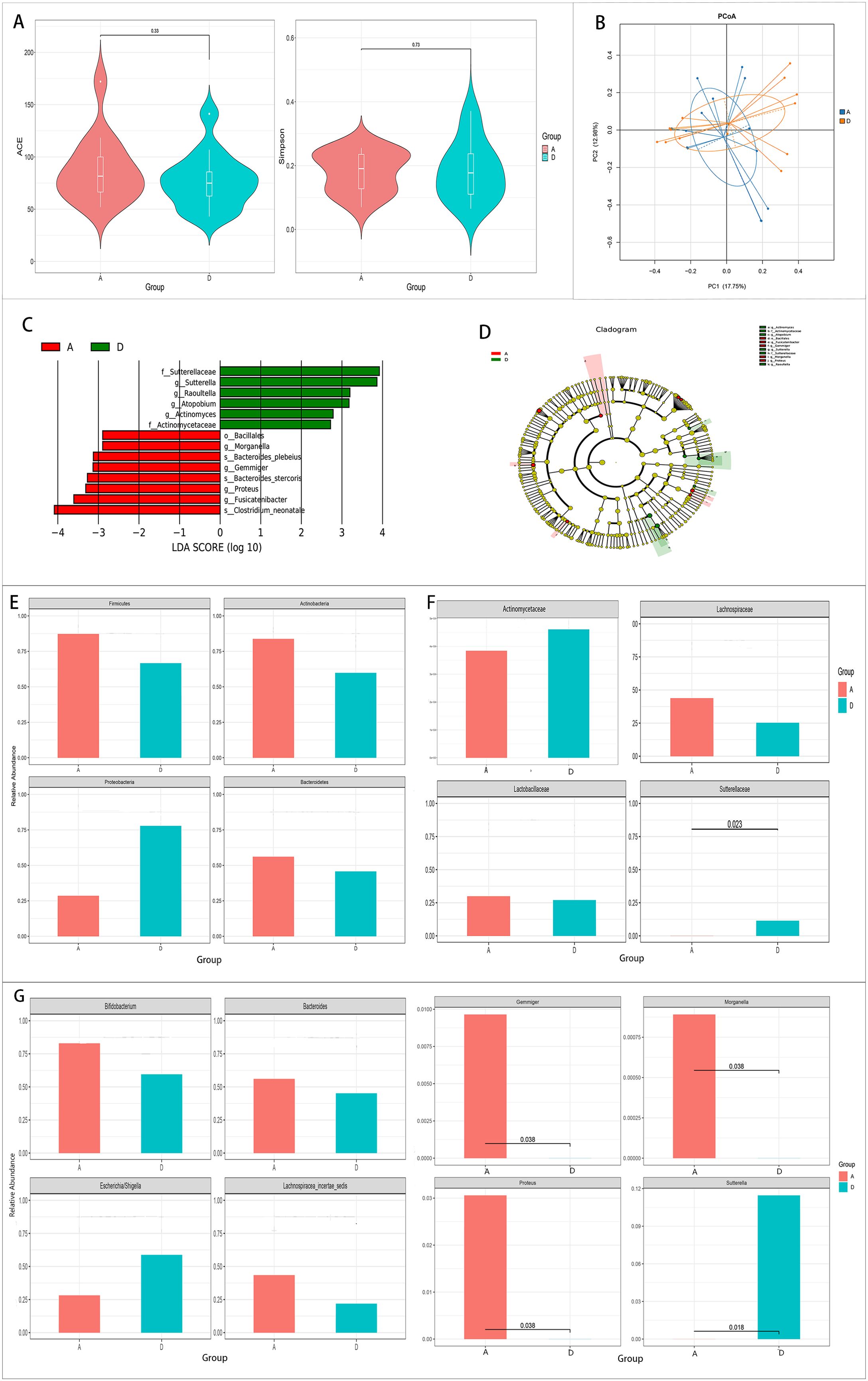
Figure 1. Comparison of the gut microbiota between the pre-treatment group (group A) and the control group (group D). (A, B) Comparison of the alpha diversity (A) and the beta diversity (B) between the two groups. (C, D) Linear discriminant analysis (LDA) value distribution and the cladogram of the linear discriminant analysis effect size (LEfSe) between the two groups. (E–G) Metastats analysis at the phylum (E), family (F), and genus (G) levels between the two groups.
The results of LEfSe showed that six bacteria were enriched in the control group and eight bacteria were enriched in the pre-treatment group (Figures 1C, D).
Metastats analysis was conducted to determine the species with significant differences at the phylum, genus, and family levels. No significant differences were found in the major gut microbiota at the phylum level (Figure 1E). However, at the genus and family levels, Proteus, Gemmiger, and Morganella increased, while Sutterella and Sutterellaceae decreased significantly in the pre-treatment group (p < 0.05) (Figures 1F, G).
3.2 Data analysis of the case group before treatment and 2 weeks and 1 month after treatment
3.2.1 Comparison of the HPA axis hormone and inflammatory cytokine levels among the three groups
The levels of the HPA axis hormones and inflammatory cytokines in the case group decreased after drug therapy. Compared with the pre-treatment group, the levels of CRH and ACTH after 2 weeks of ACTH treatment decreased significantly (p < 0.05). After 1 month of treatment with ACTH and other drugs, the levels of CRH, ACTH, and COR decreased significantly compared with those in the pre-treatment group (p < 0.05). After 1 month of treatment with ACTH and other drugs, the levels of IL-2, IL-4, IL-6, and IL-17α decreased significantly compared with those before treatment (p < 0.05) (Table 3).
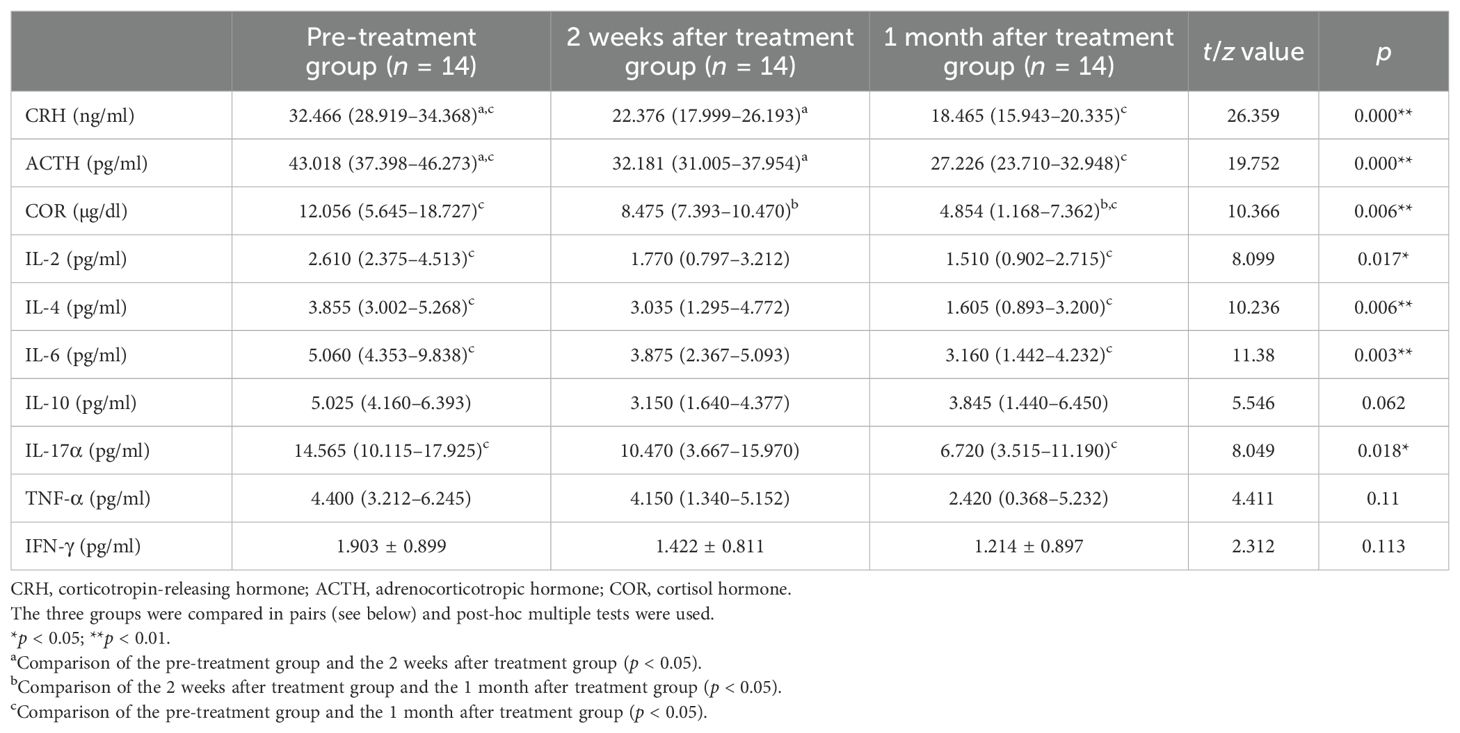
Table 3. Comparison of levels of the hypothalamus–pituitary–adrenal (HPA) axis hormones and inflammatory cytokines in the case group before treatment and 2 weeks and 1 month after treatment.
3.2.2 Analysis of the gut microbiota differences among the three groups
There were no differences in the alpha and beta diversity among the three groups (Figures 2A, B).
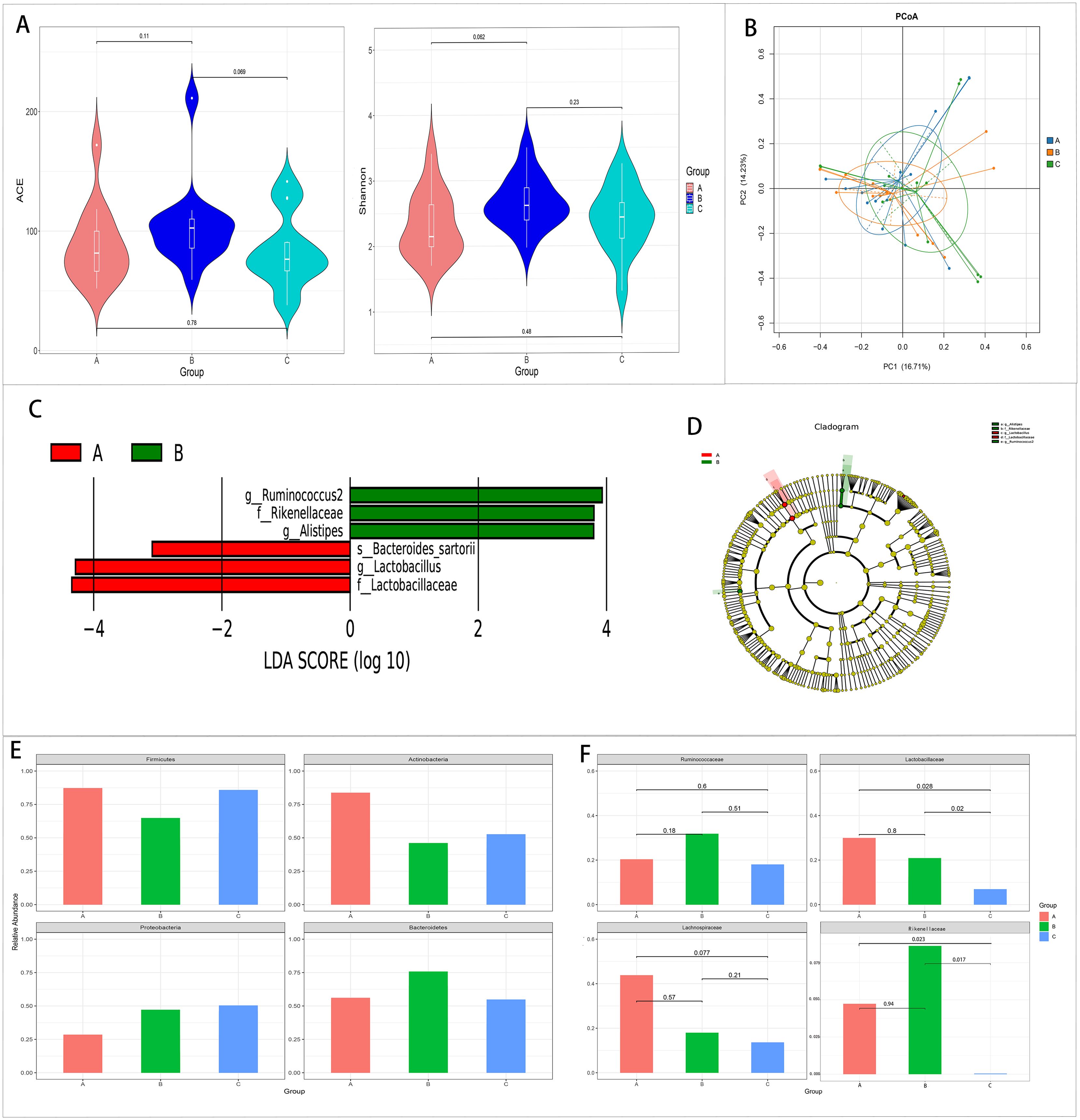
Figure 2. Comparison of the gut microbiota among the pre-treatment (group A), 2 weeks after treatment (group B), and 1 month after treatment (group C) groups in the case group. (A, B) Comparison of the alpha diversity (A) and the beta diversity (B) among the three groups. (C, D) Linear discriminant analysis (LDA) value distribution and the cladogram of the linear discriminant analysis effect size (LEfSe) among the three groups. (E, F) Metastats analysis at the phylum (E) and family (F) levels among the three groups.
The results of LEfSe showed that three bacteria were enriched in the pre-treatment group and three bacteria were enriched in the 2 weeks after treatment group. There was no abundant gut microbiota in the 1 month after treatment group (Figures 2C, D).
Metastats analysis showed that Lactobacillus and Lactobacillaceae decreased with the extension of the treatment time, and the relative abundance of these two bacteria in the 1 month after treatment group was significantly lower than that in the other two groups (p < 0.05). At the same time, Lachnospiraceae decreased with the extension of the treatment time. However, no significant difference was found (p < 0.05) (Figures 2E, F, 3).
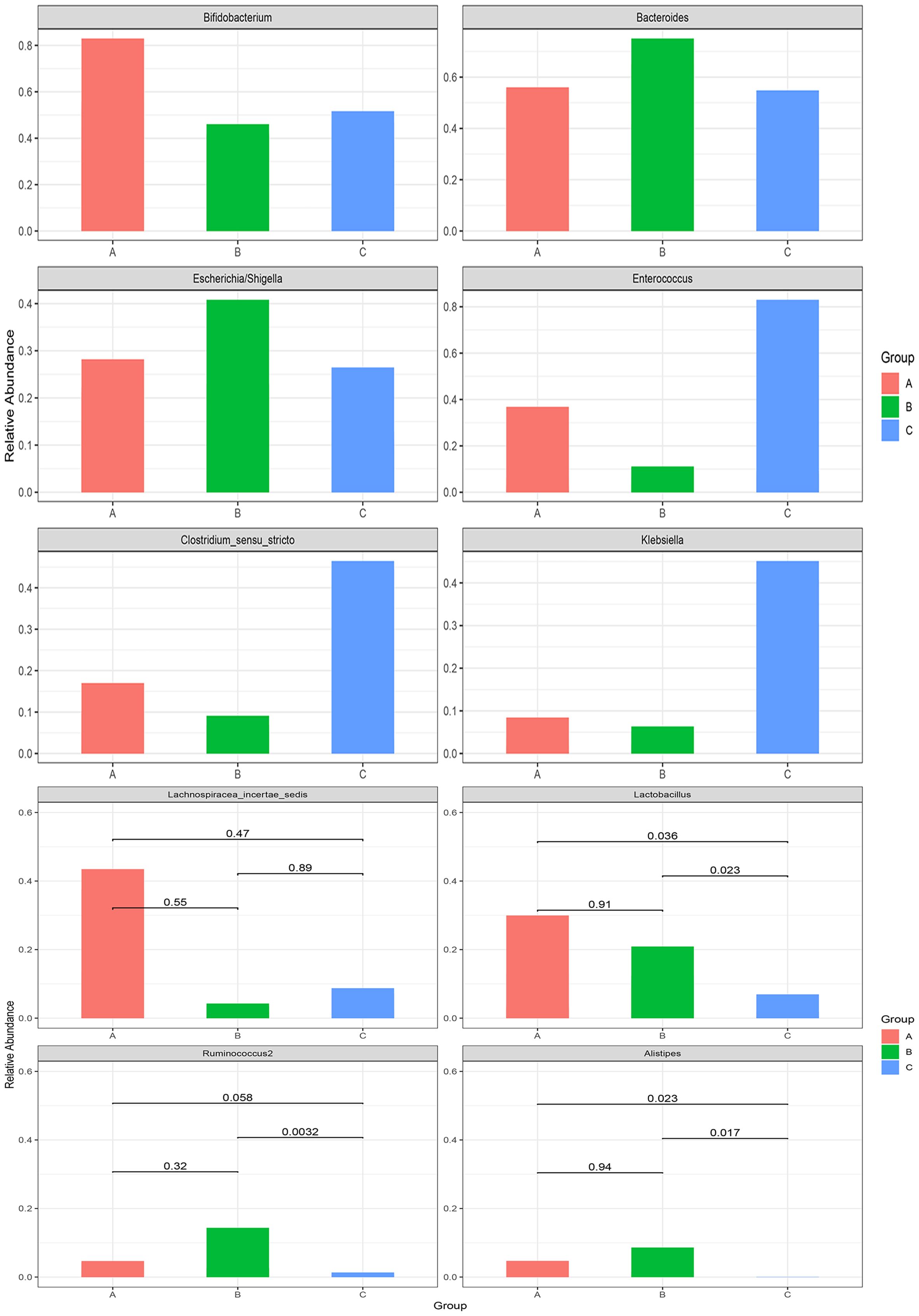
Figure 3. Metastats analysis at the genus level among the pre-treatment group (group A), the 2 weeks after treatment group (group B), and the 1 month after treatment group (group C) in the case group.
3.3 Data analysis between the effective and ineffective groups in the 2 weeks after treatment group
3.3.1 Comparison of the HPA axis hormone and inflammatory cytokine levels between the two groups
The level of IFN-γ was significantly higher and that of CRH was significantly lower in the effective group compared with the ineffective group (Table 4).
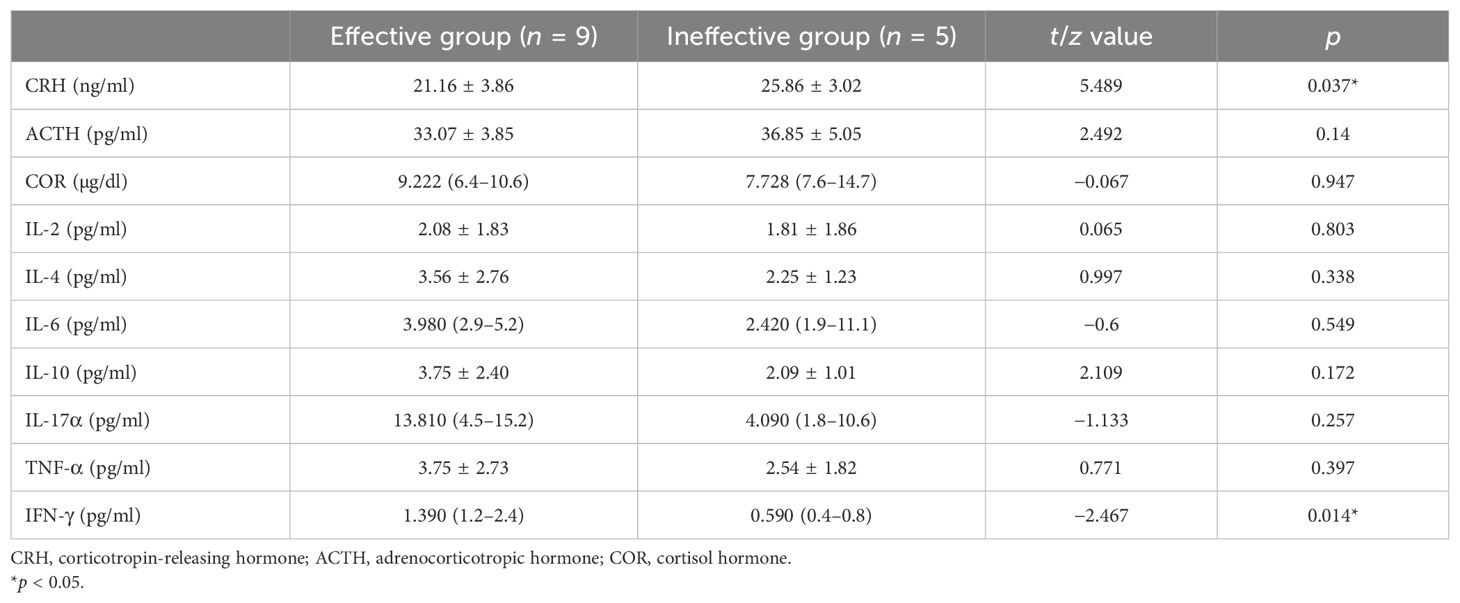
Table 4. Comparison of the levels of the hypothalamus–pituitary–adrenal (HPA) axis hormones and inflammatory cytokines between the effective and ineffective groups.
3.3.2 Analysis of the gut microbiota differences between the two groups
There were no differences in the alpha and beta diversity between the two groups.
The results of LEfSe revealed three bacteria enriched in the effective group and 10 bacteria enriched in the ineffective group.
Metastats analysis showed that Alistipes and Rikenellaceae were significantly higher and that Megamonas, Faecalibacterium, and Ruminococcus were significantly lower in the effective group than those in the ineffective group (p < 0.05) (Figure 4).
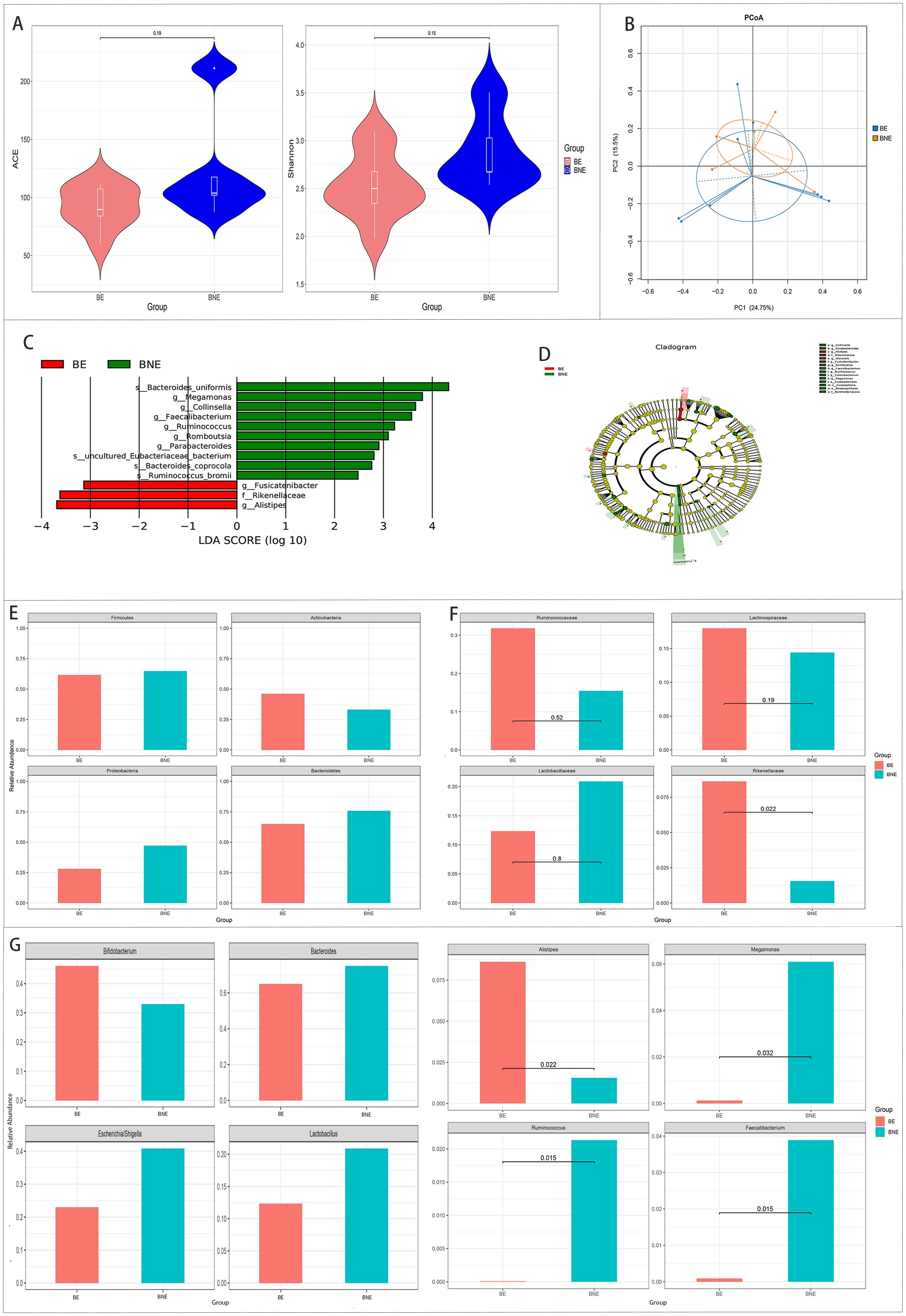
Figure 4. Comparison of the gut microbiota between the effective (BE) and ineffective (BNE) groups in the 2 weeks after treatment group. (A, B) Comparison of the alpha diversity (A) and the beta diversity (B) between the two groups. (C, D) Linear discriminant analysis (LDA) value distribution and the cladogram of the linear discriminant analysis effect size (LEfSe) between the two groups. (E–G) Metastats analysis at the phylum (E), family (F), and genus (G) levels between the two groups.
3.4 Correlation analysis between the gut microbiota and the HPA axis hormone and inflammatory cytokine levels
We used the heat map of the Spearman’s rank-sum correlation coefficients to determine the correlation between the gut microbiota and the HPA axis hormone and inflammatory cytokine levels. In the pre-treatment group, Lachnospiracea_incertae_sedis was positively associated with CRH. Lactobacillaceae and Lactobacillus were positively associated with IL-2 and IL-4. Alistipes and Rikenellaceae were negatively correlated with IL-17a and IFN-γ in the 2 weeks after treatment group. In the 1 month after treatment group, Sutterellaceae was positively associated with CRH and negatively associated with IL-2 and TNF-α. Alistipes and Rikenellaceae were negatively associated with IL-6 and IFN-α (Figure 5A).
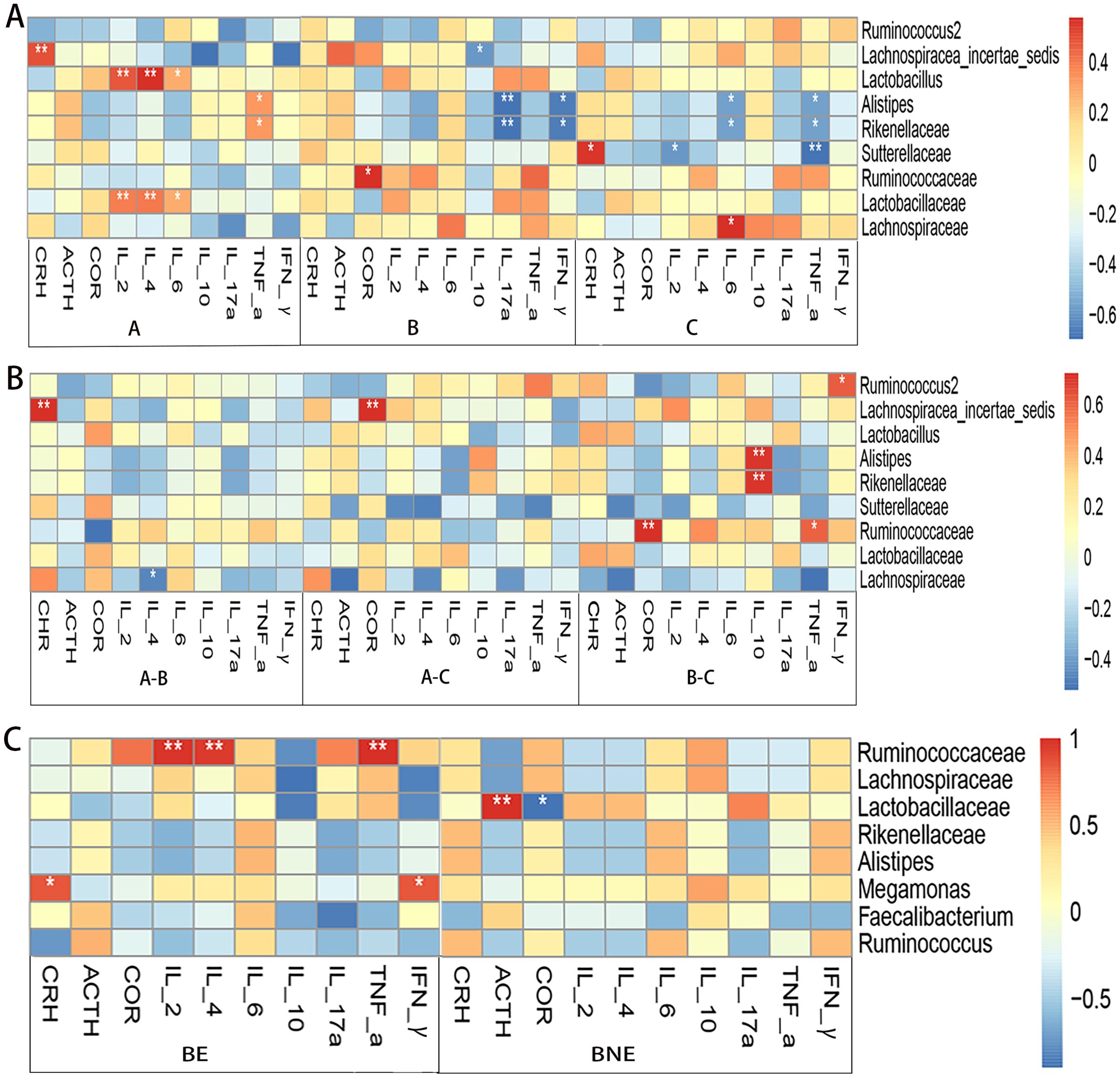
Figure 5. Correlation analysis between the gut microbiota and the levels of hypothalamus–pituitary–adrenal (HPA) axis hormones and inflammatory cytokines. (A) Correlation analysis of the pre-treatment (group A), 2 weeks after treatment (group B), and 1 month after treatment (group C) groups in the case group. (B) Correlation analysis between the differences in the gut microbiota with differences in the levels of HPA axis hormones and inflammatory cytokines in the case group. (C) Correlation analysis of the effective (BE) and ineffective (BNE) groups *p < 0.05; **p < 0.01.
Subsequently, we compared the correlation between the differences in the gut microbiota and the differences in the levels of HPA axis hormones and inflammatory cytokines. It was found that Lachnospiracea_incertae_sedis was positively associated with CRH and COR. Alistipes and Rikenellaceae were negatively associated with IL-10 (the relative abundance of Alistipes and Rikenellaceae in group B was higher than that in group C, while the level of IL-10 in group B was lower than that in group C) (Figure 5B).
In the effective group, Ruminococcaceae was positively associated with IL-2, IL-4, and IFN-γ. Lactobacillaceae was positively associated with ACTH (Figure 5C).
4 Discussion
In this study, it was found that the levels of IL-2, IL-4, IL-6, and IL-17α in the pre-treatment group were considerably greater than those in the control group, indicating that the level of inflammatory cytokines in infants with IS increased. After disruption of the central nervous system in infants with spasm, damage of the endothelial cells in the brain tissue causes the release of a variety of inflammatory factors. Based on pathological research, the inflammatory regulators and receptors have been found to be greatly enhanced in brain tissue specimens from patients with epilepsy, viral encephalitis, and other neurological injuries, as well as in the cerebral cortex and limbic tissues of rats with epilepsy. For instance, inflammatory factors such as IL-2 and IL-6 in serum showed a substantial increase (14, 15). IL-2, a γc family cytokine, is primarily secreted by T cells, promoting lymphocyte proliferation, inducing natural killer (NK) cells, and participating in immune regulation (16). In the central nervous system, IL-6 possesses a range of biological actions, which can regulate the functions of nerve cells (17). Studies have indicated that IL-6 is overexpressed in the brain tissue of patients with epilepsy and is released into circulation through cerebral blood vessels. The level of IL-6 was found to be much higher in patients with recurrent generalized tonic–clonic seizures compared with those with single seizures (18). IL-17α is an important inflammatory factor that can stimulate macrophages, endothelial cells, fibroblasts, and epithelial cells to produce a variety of inflammatory factors, promoting the incidence and development of neurological diseases (19). Related studies have reported that the level of IL-17α was higher in the cerebrospinal fluid of children with acute seizures, which is consistent with our findings (20).
This study discovered that the CRH level in the pre-treatment group was much greater than that in the control group, and the levels of CRH, ACTH, and COR on the HPA axis decreased following ACTH therapy. Previous studies have shown that the concentrations of CRH can increase in response to certain conditions such as external stress and can trigger IS by interacting with brain-specific cortical targets (21). ACTH, an anti-epileptic drug, is currently derived from corticotropin extracted from exogenous bovine, horse, and other animals. Its mechanism may involve negative feedback to inhibit CRH secretion in the HPA axis, thereby temporarily suppressing the secretion of endogenous ACTH, resulting in a decreased ACTH concentration after treatment (22). COR is a part of the HPA axis, regulated by superiors. Most of the children in this study received prednisone oral therapy following ACTH administration at the end of 2 weeks of treatment. Prednisone, acting as COR, could regulate the upstream CRH and ACTH levels with negative feedback, leading to a decrease in the COR concentration after 1 month of treatment. According to previous studies, patients with epilepsy had abnormal immune function, which affected the endocrine system through the “neuroendocrine–immune network” and promoted cortisol release (23).
Interestingly, the levels of IL-2, IL-4, IL-6, and IL-17α decreased significantly after treatment, which further explained the effect of cranial nerve injury and inflammatory response on the pathological changes of IS. Previous studies have demonstrated that ACTH exerts a regulatory effect on the immune-mediated inflammatory responses (24). This mechanism might account for the reduction in the levels of inflammatory cytokines in the case group following treatment. Given the incomplete understanding of the specific pathogenesis of IS, additional research is needed to elucidate the precise mechanism of ACTH in the treatment of IS.
There were no significant differences in the gut microbiota diversity across all groups in this study, suggesting that the gut microbiota of patients were similar to those of normal infants. Moreover, the treatment and efficacy had little effect on the diversity of the gut microbiota, which is consistent with the findings of a study on infantile spasmodic gut microbiota (9).
Compared with the control group, Sutterellaceae and Sutterella decreased significantly in the pre-treatment group. Sutterella belongs to Sutterellaceae, and animal model studies have shown that Sutterellaceae are typical intestinal dominant bacteria whose variations in abundance are related to the function of the intestinal mucosal barrier (25). These findings showed that a reduction in Sutterellaceae is associated with disease severity.
When comparing the conditions before and after treatment in the case group, Lachnospiraceae was found to decrease with the extension of the treatment time. After 2 weeks of treatment, Lachnospiracea_incertae_sedis decreased compared with that before treatment. However, there was no significant difference. Lachnospiracea_incertae_sedis, which belongs to Lachnospiraceae, are anaerobic bacteria known to promote glucose fermentation and to produce lactic and formic acids, impacting intestinal permeability and stimulating intestinal chromaffin cell synthesis and the release of serotonin (5-HT) (26). 5-HT, a neurotransmitter, influences the excitability/inhibitory balance of the cerebral cortex and subcortical regions and is involved in various physiological and pathological processes in the brain. Studies have shown that 5-HT could affect the onset and progression of epilepsy by influencing the response to ACTH therapy in infants with IS (27). Furthermore, other research found that the relative abundance of Lachnospiracea_incertae_sedis in patients with Parkinson’s disease increased, while it decreased significantly in patients who responded to treatment (28). This supports the notion that, as therapy continued and the condition improved, the dominant bacteria of the gut microbiota in children with IS gradually shifted toward those of a normal human gut microbiota.
After 2 weeks of treatment in the case group, Alistipes and Rikenellaceae increased in the effective group, while Megamonas, Faecalibacterium, Ruminococcus, and Romboutsia increased in the ineffective group. Ruminococcus belongs to Firmicutes, and its abundance increased in the gut microbiota of drug-resistant epilepsy patients (29). Ruminococcus and other uncommon bacteria could participate in the occurrence of epilepsy by regulating the adenosine triphosphate binding cassette transporters (29). Ruminococcus has been shown to be positively correlated with glutamate and to be negatively correlated with 5-HT in animal studies (30). In addition, Ruminococcus is associated with lower levels of N-acetylaspartic acid in patients with epilepsy, which is a neuronal health marker (31). Therefore, Ruminococcus is thought to influence drug susceptibility to epilepsy by regulating certain neurotransmitters.
This study found that Lachnospiracea_incertae_disedis was positively associated with CRH. Lachnospiracea_incertae_disedis is significantly higher in children with autism spectrum disorder, and studies have shown that autism is associated with disorders of the HPA axis caused by maternal stress and trauma during pregnancy (32). These might suggest that Lachnospiracea_incertae_disedis could influence the occurrence of neurological diseases through the HPA pathway. Ruminococcaceae was positively associated with IL-2, IL-4, and IFN-γ. This is consistent with the increase in the relative abundance of Ruminococcus in the ineffective group.
It was also discovered that, after treatment, Sutterellaceae was positively associated with CRH and negatively associated with IL-2 and TNF-α. Sutterella has been shown to be negatively associated with inflammatory cytokines (IL-12, IL-13, and IFN-γ) in a clinical cohort study (33), which is consistent with this study. The CRH levels increased and Sutterellaceae decreased, which did not match the expected results. This could be related to factors such as the experimental design, the subjects’ age, and the sample acquisition time, among others, which made it difficult to cross-compare some experiments.
In summary, the MGBA plays an important role in IS. In a study on the association between IS and the gut microbiota, researchers found that Lactobacillus, Roseburia, and Lachnospira were lower in the IS group than those in the healthy group. Compared with that in the ACTH-NR (no response) group, Bifidobacterium was higher in the ACTH-response group (34). Studies have shown that probiotic supplementation could significantly reduce the frequency and the severity of seizures (35, 36). In addition, research found that the ratio of Bacteroidota-to-Firmicutes increased in epileptic (Epi) rats compared with non-epileptic (No-Epi) and sham control rats (37). These are consistent with the findings of this study, where the gut microbiota was involved in the development of IS. Specific gut microbiota could be used as a potential therapeutic target for IS, and disease control might be achieved by restoring the gut microbiota.
This study has certain limitations. Firstly, the sample size was small, which should be expanded in future studies to improve the accuracy of the results. Secondly, in this study, 16S rDNA sequencing was used for the gut microbiota, which could only ensure the accuracy of the microflora at the genus level and above. Therefore, future research needs to use metagenomic sequencing technology to improve the breadth and accuracy of sequencing. Thirdly, the follow-up time of the study was short, and follow-up should be up to 3 and 6 months and 1 year after treatment. Data on the improvement of the children, the serological samples at the time of review, and the stool results should be collected in order to track the changes in the relationship of the HPA hormones, inflammatory cytokines, and gut microbiota. Finally, we studied the relationship of the HPA axis hormones, inflammatory cytokines, and gut microbiota; however, the various ways how the gut microbiota affects the development of IS, as well as the in-depth mechanism of this effect, have not been studied. Therefore, future studies need to conduct animal and cell experiments to explore how the gut microbiota affects the pathogenesis of IS.
5 Conclusion
The gut microbiota of children with IS differed from that of healthy children. Lachnospiraceae and Lachnospiracea_incertae_disedis might be associated with the disease onset. Sutterellaceae might be linked to children’s improved health. In addition, certain gut microbiota might affect the levels of some HPA axis hormones or inflammatory cytokines in IS.
Data availability statement
The datasets presented in this study can be found in online repositories. The names of the repository/repositories and accession number(s) can be found https://www.ncbi.nlm.nih.gov/sra/PRJNA1145298, PRJNA1145298.
Ethics statement
The studies involving humans were approved by the Ethics Committee of Hunan Children’s Hospital (Changsha, China. No. HCHLL-2020-53). The studies were conducted in accordance with the local legislation and institutional requirements. Written informed consent for participation in this study was provided by the participants’ legal guardians/next of kin.
Author contributions
JJY: Writing – review & editing, Writing – original draft, Visualization, Validation, Software, Methodology, Investigation, Formal Analysis, Data curation, Conceptualization. LL: Writing – original draft, Visualization, Software, Methodology, Data curation, Conceptualization. XFP: Writing – review & editing, Software, Methodology. LWW: Writing – review & editing, Resources, Project administration. LHT: Writing – review & editing, Supervision, Resources, Project administration. CCZ: Writing – review & editing, Software, Data curation. SWF: Writing – review & editing, Software. ZHX: Writing – review & editing, Supervision, Resources, Project administration, Funding acquisition. JQ: Writing – review & editing, Validation, Supervision, Software, Resources, Project administration, Conceptualization.
Funding
The author(s) declare financial support was received for the research, authorship, and/or publication of this article. This research was funded by: Scientific Research Project of Hunan Provincial Health Commission, China, Grant Number: 202206034019. The Natural Science Foundation of Hunan Province, China, Grant Number: 2023JJ30319. Science and Technology Department of Hunan Province, China, Grant Number: 2020SK1014-3.
Acknowledgments
The authors thank all the participant children and their parents in the study and appreciate all of the support of the data collectors. All authors had access to the study data and reviewed and approved the final manuscript.
Conflict of interest
The authors declare that the research was conducted in the absence of any commercial or financial relationships that could be construed as a potential conflict of interest.
Publisher’s note
All claims expressed in this article are solely those of the authors and do not necessarily represent those of their affiliated organizations, or those of the publisher, the editors and the reviewers. Any product that may be evaluated in this article, or claim that may be made by its manufacturer, is not guaranteed or endorsed by the publisher.
Supplementary material
The Supplementary Material for this article can be found online at: https://www.frontiersin.org/articles/10.3389/fimmu.2024.1442677/full#supplementary-material
References
1. Harini C, Nagarajan E, Bergin AM, Pearl P, Loddenkemper T, Takeoka M, et al. Mortality in infantile spasms: A hospital-based study. Epilepsia. (2020) 61:702–13. doi: 10.1111/epi.16468
2. Smith MS, Matthews R, Rajnik M, Mukherji P. Infantile epileptic spasms syndrome (West syndrome). In: StatPearls. StatPearls Publishing LLC, Treasure Island (FL (2024). Disclosure: Rebecca Matthews declares no relevant financial relationships with ineligible companies. Disclosure: Michael Rajnik declares no relevant financial relationships with ineligible companies. Disclosure: Pinaki Mukherji declares no relevant financial relationships with ineligible companies.: StatPearls Publishing Copyright © 2024.
3. Pavone P, Polizzi A, Marino SD, Corsello G, Falsaperla R, Marino S, et al. West syndrome: a comprehensive review. Neurol Sci. (2020) 41:3547–62. doi: 10.1007/s10072-020-04600-5
4. Pellock JM, Hrachovy R, Shinnar S, Baram TZ, Bettis D, Dlugos DJ, et al. Infantile spasms: a U.S. consensus report. Epilepsia. (2010) 51:2175–89. doi: 10.1111/j.1528-1167.2010.02657.x
5. Riikonen R. Combination therapy for treatment of infantile spasms. Lancet Neurol. (2017) 16:19–20. doi: 10.1016/s1474-4422(16)30276-9
6. Philbin M, Niewoehner J, Wan GJ. Clinical and economic evaluation of repository corticotropin injection: A narrative literature review of treatment efficacy and healthcare resource utilization for seven key indications. Adv Ther. (2017) 34:1775–90. doi: 10.1007/s12325-017-0569-9
7. Osborne JP, Edwards SW, Dietrich Alber F, Hancock E, Johnson AL, Kennedy CR, et al. The underlying etiology of infantile spasms (West syndrome): Information from the International Collaborative Infantile Spasms Study (ICISS). Epilepsia. (2019) 60:1861–9. doi: 10.1111/epi.16305
8. Wan L, Yang G, Zhang S, Sun Y, Li Z, Wang J, et al. Investigation of the association between imbalance of the intestinal flora and infantile spasms: a pilot case-control study. Transl Pediatr. (2021) 10:819–33. doi: 10.21037/tp-20-384
9. Xu L, Chen D, Zhao C, Jiang L, Mao S, Song C, et al. Decreased abundance of Akkermansia after adrenocorticotropic hormone therapy in patients with West syndrome. BMC Microbiol. (2021) 21:126. doi: 10.1186/s12866-021-02189-z
10. Jiaojiao W, Ruiguang Z, Xiaorong D. Recent advance in interaction between intestinal flora and brain function. Chin J Neuromed. (2018) 17:1281–6. doi: 10.3760/cma.j.issn.1671-8925.2018.12.000
11. O’Mahony SM, Marchesi JR, Scully P, Codling C, Ceolho AM, Quigley EM, et al. Early life stress alters behavior, immunity, and microbiota in rats: implications for irritable bowel syndrome and psychiatric illnesses. Biol Psychiatry. (2009) 65:263–7. doi: 10.1016/j.biopsych.2008.06.026
12. Maroso M, Balosso S, Ravizza T, Liu J, Aronica E, Iyer AM, et al. Toll-like receptor 4 and high-mobility group box-1 are involved in ictogenesis and can be targeted to reduce seizures. Nat Med. (2010) 16:413–9. doi: 10.1038/nm.2127
13. De Caro C, Leo A, Nesci V, Ghelardini C, di Cesare Mannelli L, Striano P, et al. Intestinal inflammation increases convulsant activity and reduces antiepileptic drug efficacy in a mouse model of epilepsy. Sci Rep. (2019) 9:13983. doi: 10.1038/s41598-019-50542-0
14. Michael BD, Solomon T. Seizures and encephalitis: clinical features, management, and potential pathophysiologic mechanisms. Epilepsia. (2012) 53 Suppl 4:63–71. doi: 10.1111/j.1528-1167.2012.03615.x
15. Kennedy PGE, Quan PL, Lipkin WI. Viral encephalitis of unknown cause: current perspective and recent advances. Viruses. (2017) 9:138. doi: 10.3390/v9060138
16. Guo Q. Increased levels of HMGB1, IL-2, IL-6 in the serum of epileptic children and its significance. Zhengzhou University (2015).
17. Chao L, Qi G. Changes of serum IL-1β, IL-2, IL-6, IL-8 and TNF-α Levels in patients with epilepsy and clinical significance. J Prev Med Chin People’s Liberation Army. (2018) 36:375–377,385. doi: 10.13704/j.cnki.jyyx.2018.03.023
18. Dede F, Karadenizli S, Ozsoy OD, Eraldemir FC, Sahin D, Ates N. Antagonism of adenosinergic system decrease SWD occurrence via an increment in thalamic NFkB and IL-6 in absence epilepsy. J Neuroimmunol. (2019) 326:1–8. doi: 10.1016/j.jneuroim.2018.11.004
19. Rahman MT, Ghosh C, Hossain M, Linfield D, Rezaee F, Janigro D, et al. IFN-γ, IL-17A, or zonulin rapidly increase the permeability of the blood-brain and small intestinal epithelial barriers: Relevance for neuro-inflammatory diseases. Biochem Biophys Res Commun. (2018) 507:274–9. doi: 10.1016/j.bbrc.2018.11.021
20. Qianqian Z, Linyan M, Jing W, Yang C, Jing D, Xi W. Changes of interleukin-17A and related inflammatory factors levels in the cerebrospinal fluid during the acute phase of seizures. Chin J Clin Neurosci. (2021) 29:393–8.
21. Xiaolin Y, Xiaoqing Z, Bing C, Wei L. Expression of corticotropin releasing hormone and its receptors in infantile spasm. J Epilepsy. (2017) 3:3–14. doi: 10.7507/2096-0247.20170001
22. Ross DL. Suppressed pituitary ACTH response after ACTH treatment of infantile spasms. J Child Neurol. (1986) 1:34–7. doi: 10.1177/088307388600100105
23. Pei L, Li T, Na L, Fenxiang W. Relationship between seizure cluster of temporal lobe epilepsy with hippocampal sclerosis and cortisol rhythm change. Chin J Neurol. (2021) 54:1273–81. doi: 10.3760/cma.j.cn113694-20210824-00582
24. Doodnauth AV, Klar M, Mulatu YS, Malik ZR, Patel KH, McFarlane SI. Pembrolizumab-induced hypophysitis with isolated adrenocorticotropic hormone (ACTH) deficiency: A rare immune-mediated adverse event. Cureus. (2021) 13:e15465. doi: 10.7759/cureus.15465
25. Dongmei L, Fang P, Yunhao Y, Qingqian X. Electroacupuncture improves cognitive ability of APP/PS1 mice based on mechanism of affecting gut microbiota. Chin J Pathophysiol. (2021) 37:1774–83. doi: 10.3969/j.issn.1000-4718.2021.10.006
26. Yano JM, Yu K, Donaldson GP, Shastri GG, Ann P, Ma L, et al. Indigenous bacteria from the gut microbiota regulate host serotonin biosynthesis. Cell. (2015) 161:264–76. doi: 10.1016/j.cell.2015.02.047
27. Shi XY, Zou LP, Yang G, Ding YX, He B, Sun YH, et al. Association of serotonin transporter polymorphisms with responsiveness to adrenocorticotropic hormone in infantile spasm. World J Pediatr. (2013) 9:251–5. doi: 10.1007/s12519-013-0420-6
28. Cheng C, Huiyan Y, Wei L, Jing S. Structural changes of gut microbiota in patients with Parkinson’s disease. Chin J Neurol. (2018) 51:498–503. doi: 10.3760/cma.j.issn.1006-7876.2018.07.004
29. Peng A, Qiu X, Lai W, Li W, Zhang L, Zhu X, et al. Altered composition of the gut microbiome in patients with drug-resistant epilepsy. Epilepsy Res. (2018) 147:102–7. doi: 10.1016/j.eplepsyres.2018.09.013
30. Deng X, Xie Y, Chen Y. Effect of neuroinflammation on ABC transporters: possible contribution to refractory epilepsy. CNS Neurol Disord Drug Targets. (2018) 17:728–35. doi: 10.2174/1871527317666180828121820
31. Strandwitz P, Kim KH, Terekhova D, Liu JK, Sharma A, Levering J, et al. GABA-modulating bacteria of the human gut microbiota. Nat Microbiol. (2019) 4:396–403. doi: 10.1038/s41564-018-0307-3
32. Jingjing L, Jianjun O, Chen C. Maternal stress and autism spectrum disorder: mediating the inflammatory response. J Int Psychiatry. (2019) 46:590–3. doi: 10.13479/j.cnki.jip.2019.04.005
33. Kaakoush NO. Sutterella species, igA-degrading bacteria in ulcerative colitis. Trends Microbiol. (2020) 28:519–22. doi: 10.1016/j.tim.2020.02.018
34. Sasaki M, Takenouchi T, Sakaguchi Y, Takahashi T. Decisive evidence of direct effect of ACTH treatment in West syndrome: A case report. Seizure. (2021) 91:49–51. doi: 10.1016/j.seizure.2021.05.016
35. Gómez-Eguílaz M, Ramón-Trapero JL, Pérez-Martínez L, Blanco JR. The beneficial effect of probiotics as a supplementary treatment in drug-resistant epilepsy: a pilot study. Benef Microbes. (2018) 9:875–81. doi: 10.3920/bm2018.0018
36. Bagheri S, Heydari A, Alinaghipour A, Salami M. Effect of probiotic supplementation on seizure activity and cognitive performance in PTZ-induced chemical kindling. Epilepsy Behav. (2019) 95:43–50. doi: 10.1016/j.yebeh.2019.03.038
Keywords: gut microbiota, infantile spasm, inflammatory cytokines, HPA axis hormones, correlations
Citation: You J, Liu L, Pan X, Wu L, Tan L, Zhou C, Fang S, Xiao Z and Qiu J (2024) Study on the gut microbiota, HPA, and cytokine levels in infantile spasms. Front. Immunol. 15:1442677. doi: 10.3389/fimmu.2024.1442677
Received: 02 June 2024; Accepted: 03 September 2024;
Published: 10 October 2024.
Edited by:
Omar Ramos-Lopez, Universidad Autónoma de Baja California, MexicoReviewed by:
Silvia Vincentiis, University of São Paulo, BrazilPasquale Striano, Giannina Gaslini Institute (IRCCS), Italy
Copyright © 2024 You, Liu, Pan, Wu, Tan, Zhou, Fang, Xiao and Qiu. This is an open-access article distributed under the terms of the Creative Commons Attribution License (CC BY). The use, distribution or reproduction in other forums is permitted, provided the original author(s) and the copyright owner(s) are credited and that the original publication in this journal is cited, in accordance with accepted academic practice. No use, distribution or reproduction is permitted which does not comply with these terms.
*Correspondence: Jun Qiu, cWl1anVudHJldm9yQDE2My5jb20=
†These authors have contributed equally to this work and share first authorship