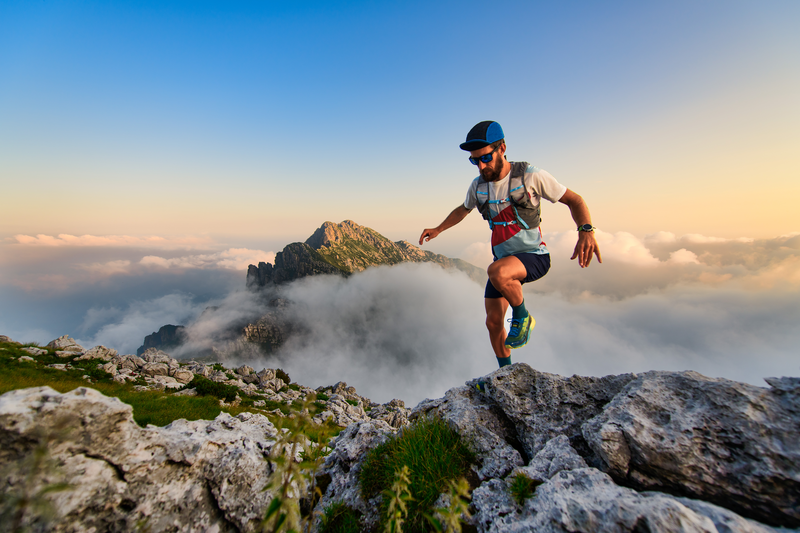
95% of researchers rate our articles as excellent or good
Learn more about the work of our research integrity team to safeguard the quality of each article we publish.
Find out more
REVIEW article
Front. Immunol. , 04 October 2024
Sec. Cancer Immunity and Immunotherapy
Volume 15 - 2024 | https://doi.org/10.3389/fimmu.2024.1441667
This article is part of the Research Topic Immune-Checkpoint Inhibitors and Immunometabolic Reprogramming in Cancer Immunotherapy View all 10 articles
Triple-negative breast cancer (TNBC) is a highly aggressive type of breast cancer that encompasses several distinct subtypes. Recent advances in immunotherapy offer a promising future for the treatment of these highly heterogeneous and readily metastatic tumors. Despite advancements, the efficacy of immunotherapy remains limited as shown by unimproved efficacy of PD-L1 biomarker and limited patient benefit. To enhance the effectiveness of TNBC immunotherapy, we conducted investigation on the microenvironment, and corresponding therapeutic interventions of TNBC and recommended further investigation into the identification of additional biomarkers that can facilitate the subtyping of TNBC for more targeted therapeutic approaches. TNBC is a highly aggressive subtype with dismal long-term survival due to the lack of opportunities for traditional endocrine and targeted therapies. Recent advances in immunotherapy have shown promise, but response rates can be limited due to the heterogeneous tumor microenvironments and developed therapy resistance, especially in metastatic cases. In this review, we will investigate the tumor microenvironment of TNBC and corresponding therapeutic interventions. We will summarize current subtyping strategies and available biomarkers for TNBC immunotherapy, with a particular emphasis on the need for further research to identify additional prognostic markers and refine tailored therapies for specific TNBC subtypes. These efforts aim to improve treatment sensitivity and ultimately enhance survival outcomes for advanced-stage TNBC patients.
Triple-negative breast cancer is a highly aggressive and heterogeneous type of breast cancer (BC) that lacks the expression of estrogen receptor (ER), progesterone receptor (PR), and human epidermal growth factor receptor 2 (HER2). These features make TNBC non-responsive to conventional hormonal and targeted therapies, resulting in poor clinical outcomes (1, 2).
Immunotherapy (IM) emerges as a promising therapeutic strategy for TNBC by leveraging immune system to identify and eradicate tumor cells. Several clinical trials have demonstrated the potential benefits of IM for TNBC patients, especially when combined with chemotherapy (3, 4, 5–8). In neoadjuvant setting, IMpassion031 showed that adding atezolizumab, an anti-PD-L1 antibody, to chemotherapy increased the pathological complete response (pCR) rate from 41.1% to 57.6% in patients with early-stage TNBC (9). In the metastatic setting, KEYNOTE-355 demonstrated that combining pembrolizumab, an anti-PD-1 antibody, with chemotherapy improved the overall survival (OS) from 16.1 months to 23.0 months in patients with PD-L1-positive TNBC (10). Based on these results, the FDA approved atezolizumab plus nab-paclitaxel and pembrolizumab plus chemotherapy for patients with early-stage and metastatic TNBC patients respectively with PD-L1 expression of 1% or more (11).
However, the effectiveness of immunotherapy for TNBC is not universally established, as evidenced by the IMpassion131 trial, which failed to show any benefit of adding atezolizumab to paclitaxel in patients with metastatic TNBC (12). The variation in chemotherapy choice between IMpassion131 and Impassion130 led to significant differences in trial results, indicating uncertainty in the effectiveness of immunotherapy for metastatic tumors. One of the major challenges in optimizing IM for metastatic tumors is the heterogeneity of the tumor microenvironment (TME). The TME in TNBC consists of various immune and stromal cells that can either promote or inhibit tumor growth and response to therapy. The complex interactions within the TME, including the presence of immune cells such as tumor-infiltrating lymphocytes (TILs) and the expression of immune checkpoint molecules like PD-1, LAG-3, and IDO, play crucial roles in determining the response to immunotherapy (11, 13).
The identification of reliable biomarkers for evaluating IM response and predicting resistance remains a significant challenge. Although PD-L1 expression is currently used as a biomarker for IM selection, it has several limitations, such as variable expression patterns, low specificity, and dynamic changes during treatment (14). Therefore, comprehensive and robust biomarkers are needed to capture the complexity and diversity of TNBC, thus providing a basis for patient identification and stratification treatments.
TNBC is not a single entity, but rather an umbrella that encompasses various subtypes with distinct genetic, transcriptional, histological, and clinical characteristics (15). These subtypes may have different immune phenotypes and responses to IM. Therefore, understanding the subtyping of TNBC based on TME features may help to improve patient stratification and tailor IM accordingly. In the present study, we review the current knowledge on the TME characteristics and subtyping of TNBC and discuss how they can be used to guide IM selection and overcome resistance.
The tumor immune microenvironment is featured by the neoplastic growth region along with the extracellular matrix and other anatomical constituents (16). The tumor microenvironment is a crucial factor in the progression of TNBC as well as its response to therapy, which exhibits significant inter-patient variability and is closely associated with treatment prognosis (Figure 1). Identifying spatial immune biomarkers can help to differentiate intrinsic prognostic immune features and inform therapeutic strategies for clinically actionable immune biomarkers in TNBC. At present, molecular components especially PD-1/PD-L1 expression are identified as first-line biomarkers for recognizing patients responsive to immune checkpoint blockade (ICB). However, the cellular components, namely stromal cells (fibroblasts, macrophages, and endothelial cells) and immune cells (T lymphocytes, etc.) also play a crucial role in the effect of TME on tumor progression and have gained increasing attention in the investigation of tumor immune landscape (17). The variation in cellular components significantly influences the therapeutic outcomes and underscores the importance of a comprehensive understanding of the TME. Furthermore, gene signatures corresponding with different features or cellular components can identify extracellular components such as growth factors, cytokines, hormones, extracellular matrix, and molecular component markers, thus having potential clinical effects (17, 126). This heterogeneity necessitates the integration of various biomarkers and gene signatures to develop more precise and personalized treatment strategies. Understanding the dynamic interactions within the TME is essential for the successful application of immunotherapies and improving patient outcomes in TNBC.
Figure 1. Tumor Microenvironment and Subtypes of TNBC. TNBC can be classified into four subtypes based on their genetic profiles and microenvironment, which provide diverse therapy choices. The tumor environment contains cellular interactions and molecular modulations. CNA, copy number alternations; CSCs, cancer stem cells; BLIS, basal-like immunosuppressed; MES, mesenchymal; LAR, luminal androgen receptor; IM, immunomodulatory.
Since 2014, The collection of TILs data has been conducted on a global scale, encompassing over 20,000 primary breast cancer samples (18, 19). The findings have reinforced a strong correlation between improved prognoses and the presence of TILs in both TNBC and HER2+ BC (20, 21). TILs therefore have been widely acknowledged as a well-known prognostic factor in early triple-negative breast cancer. The KEYNOTE-086 study found that patients with more TILs were inclined to get better results from ICB treatment (22, 23). A recent study from Nature also reinforces that TILs have an independent association with TNBC prognosis (16). A comprehensive evaluation of TME identified two subtypes correlated with TILs levels and immune-related pathways (24), among which the IM TNBC subtype was associated with better prognosis and response to chemotherapy and immune therapy in both the neoadjuvant and adjuvant cohorts (15, 25). To investigate the potential correlation between increased TILs and specific T cell subtypes, an immunophenotypic characterization has been conducted (26). This analysis revealed a consistent positive association between the overall number of TILs and all T cell subtypes, particularly emphasizing the density of CD8+ T cells, CD4+ lymphocytes, and FOXP3-expressing cells. High levels of TILs were found to be positively correlated with prolonged relapse-free survival and overall survival in TNBC patients (27, 28). Therefore, TILs could be identified as a subtyping biomarker for immune response TNBC. Neoplasms have demonstrated the ability to elude immune system defenses through a variety of mechanisms, including antigen recognition constraints, immune system suppression, and T cell exhaustion induction (29). By receiving personalized TILs immunotherapy, patients with refractory solid tumors, including TNBC, could have a better prognosis (30, 31). In 2018, Zacharakis reported a chemotherapy-resistant HR+ metastases BC patient who received TILs reactive against four proteins achieved a durable complete response after combination therapy with IL-2 and ICB (30). In 2020, preliminary efficacy for the combination of PD-1 inhibitor and TILs therapy in NSCLC treatment was reported (32). Despite promising results from clinical trials, there remain substantial challenges in broadening the application of TILs immunotherapy. The unknown characteristics of the high heterogeneity of TILs, immune evasion mechanisms, and limited patient response need to be addressed in future research agendas. In conclusion, TILs immunotherapy may provide a very promising treatment method for patients with drug-resistant TNBC.
Moreover, a population of macrophages in TNBC microenvironment suggests a better prognosis for immunotherapy. Tumor-associated macrophages (TAMs) represent a diverse population characterized by pronounced plasticity and have been linked to tumor aggressiveness and unfavorable prognostic outcomes. M2 polarization of macrophages is one of the major reasons for immunosuppression (33), while macrophage-enriched subtype (MES) of TNBC displays responses to ICB (34, 35). However, macrophages expressing CD206 demarcate a subgroup of TNBCs that may have a better prognosis (36). Therefore, the relationship between macrophages and patient prognosis in TNBC is complex and may depend on the specific macrophage subtype. Challenges in targeting TAMs include overcoming their plasticity and immunosuppressive functions, as well as ensuring specificity to avoid adverse effects on normal macrophages. Macrophages-enriched subtype transit to neutrophils-enriched subtype (NES) when tumor develops resistance to ICIs, which contributes to immune suppression (35). Tumor-associated neutrophils (TANs) are recruited to the tumor site by tumor-derived factors and can also be further classified into N1 and N2 subtypes based on gene expression profiles despite the lack of markers to differentiate. TANs have pro-tumor activity by producing ARG1, IL-10, TGF-β, and VEGF, and inhibiting cytotoxic T cells by expressing higher levels of immunosuppressive molecules, such as PD-L1, ARG1, and IDO, and lower of anti-tumor molecules, such as ROS and TNF-α (37, 38). But the difficulty in distinguishing between N1 and N2 subtypes and mitigating the pro-tumor activities of TANs without affecting their essential immune functions presents challenges in targeting TANs. NES TNBC demonstrates both systemic and local accumulation of neutrophils that have immunosuppressive properties, resulting in resistance to ICB (35, 39). Neutrophils have been found to actuate the reprogramming of macrophage anti-inflammatory by suppressing NF-κB activation, which can balance cytokine generation at a fiery location and influence monocyte and macrophage fiery capacities amid the early stages of aggravation (40). Therefore, neutrophil-to-lymphocyte ratio (NLR) could be examined as a prognostic marker, with higher NLR values associated with worse outcomes (41). It’s mentioned that intratumoral genetic NLR-low TNBC was associated with a favorable tumor immune microenvironment (42). Consequently, exploiting neutrophils in monoclonal antibodies (mAbs) therapy can result in long-term antitumor immunity (43).
Dendritic cells contain a heterogeneous category that play fundamental roles in modulating both innate and adaptive immune responses by functioning as key initiators and regulators (44, 45). There exists considerable interest in the modulation of DC function as a means of enhancing tumor immunotherapy, and various strategies have been formulated to target DCs in tumors. Potential interventions for enhancing immune response involve the administration of immunomodulatory antigens and the advancement of dendritic cell-based vaccines. Numerous categories of vaccines directed towards dendritic cells have been employed in clinical investigations to enhance cancer therapy. The administration of antigens and adjuvants to DCs within the body is a critical approach toward the development of DC vaccines (46–48). Additionally, cytokine-induced killer (CIK/DC-CIK) cell immunotherapy is a therapeutic method that uses in vitro expansion and activation of CIK cells to eliminate tumor cells. CIK cells bind to the LFA-1 ligand on tumor cells through the surface adhesion molecule LFA-1, forming effector cell-target cell contacts. They induce tumor apoptosis through the Fas signaling pathway and can secrete a variety of cytokines to regulate the immune microenvironment and enhance anti-tumor immunity (49, 50). Recent clinical trials have been implemented to evaluate the efficacy of DC-CIK immunotherapy in solid tumors such as TNBC setting. The clinical result from SYSUCC demonstrates enhancement in the prognosis of patients with post-mastectomy breast cancer when utilizing adjuvant alternative CIK cell therapy in conjunction with natural killer cell immunotherapy (51). A multi-center national-wide phase II study investigating DC-CIK immunotherapy in 686 pretreated solid tumor patients is also under conduction in SYSUCC (NCT04476641). A personalized vaccine platform using autologous DCs, pulsed with tumor membrane vesicles made from tumor tissue, encapsulating antigens from individual tumors, could also provide the basis for personalized TNBC immunotherapy (46). Challenges such as the efficiency of CIK cell expansion, potential toxicity, and the complexities of personalizing vaccines need to be addressed to improve these therapeutic approaches.
Cancer-associated fibroblasts (CAFs) are a type of stromal cells that play a crucial role in TME of TNBC (52). CAFs promote proliferation, migration, and invasion of tumor cells through G protein-coupled estrogen receptor, and produce immune suppression in BC by inducing lipid-associated macrophages (53–55). TNBC can be classified into two CAF subtypes (CAF+ and CAF-) based on gene expression profiles, while the CAF- subtype was linked to longer overall survival and more immune cells than the CAF+ subtype (56). Understanding the origin and heterogeneity of CAFs is crucial to developing novel strategies targeting pro-tumor CAF subpopulations, which can improve treatment affectability and barrier tumor development. CAF among TNBC microenvironment can be identified into six subpopulations by single-cell RNA sequencing that are generally expressed in antigen-presenting cells, including myofibroblastic CAFs, inflammatory CAFs, and a CAF subpopulation expressing MHC II, which could be targeted as potential therapeutic approaches (52, 57). To date, major CAF-targeting strategies include decreasing CAFs in TME through CAR-T-cell therapy (58), a monoclonal antibody targeting fibroblast activation protein and tumor endothelial marker 8 (59), reducing immunosuppressive functions of CAFs to achieve greater T-cell accessibility to tumor cells and increased sensitivity to therapeutic approaches, decreasing the immunosuppressive characteristics of CAFs so that improving T cell accessibility to tumor cells and increasing sensitivity to therapeutic approaches. Targeting CAFs is challenging due to their heterogeneity and plasticity, which complicates therapy development and risks inadvertently affecting normal fibroblasts and tissue homeostasis. Despite these challenges, reducing CAFs’ immunosuppressive functions to enhance T-cell accessibility to tumor cells remains a promising goal.
Tumor cells also demonstrate significant heterogeneity in TNBC, characterized by varying gene copy numbers, mutations, and losses, with somatic copy number alterations (CNAs). Generally, the presence of a germline CNA is typically associated with genomic instability, chromosomal vulnerability, tumor immune suppression, and poorer prognosis (60–62). TNBC can be classified in accord with genomic characteristics, and specific immune subtypes exhibit a strong association with certain CNAs (15, 17, 63, 64). For example, the basal-like 1 subclass of TNBC demonstrates a significantly elevated level of CNAs compared to other TNBC subclasses (65, 66), including the amplifications and gains of MYC, PIK3CA, CDK6, AKT2, KRAS, FGFR1, IGF1R, CCNE1, and CDKN2A/B gene. Conversely, the subtype is also marked by deletions of the BRCA2, PTEN, MDM2, and RB1 genes. The luminal androgen subtype demonstrates a repetitive increase in EGFR and AKT1, while also frequently presenting deletions in CCND3, AKT2, ESR1, CDKN2A/B, SMAD4, NF1, NCOR1, and TP53. While mesenchymal subtype manifests frequent losses of PDGFRA, RB1, and MAP3K1, concomitant with recurrent gains or amplification of DNMT3A and TP53 (65).
Genetic mutations in either somatic or germ cells are indicators of immune response and microenvironment. TP53 mutations may enhance immunogenic activity in breast cancer, indicating TP53 mutation status as a potential biomarker for immunotherapy-responsive breast cancer patients (67, 68). Besides TP53, genetic mutations in BRCA1 and BRCA2 genes can affect the prognosis and treatment options for TNBC (69). Compared to BRCA1/2 wild-type tumors, BRCA1/2 mutation-associated cancers are more immunogenic. Some clinical trials have shown that patients with BRCA-mutant TNBC can achieve better survival and remission rates after receiving immune checkpoint inhibitors combined with chemotherapy or targeted drugs (70; 71). Olaparib combined with Durvalumab has good tolerability and response rate in patients with gBRCA-positive recurrent TNBC (8).
Epigenetic modifications and transcriptional reprogramming play an important role in drug therapy resistance and are considered critical in promoting tumor heterogeneity and TNBC progression (35, 39, 72–76). The characteristics of epigenetic changes mainly include DNA hypermethylation, dysfunction of covalent histone modifications, and chromatin deregulation, which result in TME regulation (77–82). The profiling of DNA methylation in TNBC tumors has revealed additional insights into the DNA methylation signatures that are associated with lymph node metastases and the identification of biomarkers in differentially methylated regions can foresee neoadjuvant therapy outcome (83). Dysregulation of histones covalent modifications is another prominent mechanism that has been demonstrated to be of utmost importance in the process of transcriptomic reprogramming, which plays a role in developed resistance to chemotherapy (84, 123 | Clinical Cancer Research | American Association for Cancer Research, n.d.; 82). Chromatin dysregulation drives TNBC biology via significant transcriptome changes (39, 81, 85). Epigenetic drugs modify cancer and immune cells, enhancing immunity, which could be promising if used combined with immune checkpoint inhibitors. Recent clinical trials investigating the combination of entinostat and atezolizumab (NCT02708680) have shown disappointing results in terms of achieving significant outcomes in PFS and secondary endpoints (86, 87). Despite the potential of epigenetic drugs to enhance immunity by modifying cancer and immune cells, the combination approach with immune checkpoint inhibitors requires further investigation to establish its efficacy in improving patient outcomes.
Currently, the primary recognized strategy for identifying appropriate TNBC patients for ICB are immune scores based on PD-L1 expression levels such as combined positive score (CPS) (88). Pembrolizumab is approved for use in the neoadjuvant setting for all patients with high-risk early-stage TNBC and those with a PD-L1 CPS >10 in the first-line metastatic setting. However, using different clones in immunohistochemistry (IHC) for PD-L1 detection, such as SP142 and 22C3, is important due to their poor consistency in TNBC patients, which can impact patient selection for immunotherapy (89). To address this issue, standardized approaches and further cross-validation between different assays are needed to ensure accurate patient stratification.
In the IMpassion130 trial, insignificant results were detected between the CPS>10 group and CPS>1 group, indicating that immunotherapy for TNBC requires additional selection criteria and biomarkers. The partial failure of CPS in metastases setting could be explained by the developed immune suppression in the heterogeneous microenvironment. This highlights a critical limitation of using PD-L1 as a sole biomarker, as it may not adequately capture the complexity of immune evasion mechanisms in TNBC. Therefore, adopting immune phenotypes and immune identifiers for precise patient selection could be a possible solution to improve the prognosis of immunotherapy. Furthermore, the ongoing combination therapy necessitates the utilization of biomarkers to correspond with individual patients with their optimal treatment alternative (90).
Numerous groups have employed various classifications in the past ten years to subtype TNBC, either by histochemistry, gene expression, mRNA and lncRNA expression, or metabolic pathways (Table 1). In 2011, Lehmann’s research took the process of profiling gene expression in tumor samples obtained from a cohort of 587 TNBC patients, which resulted in the delineation of basal like-1 (BL1), basal like-2 (BL2), luminal androgen receptor (LAR), immunomodulatory (IM), mesenchymal (M), mesenchymal stem-like (MSL) subtypes (66). Currently, research primarily focuses on the molecular characteristics of TNBC subtypes, whereas the specific microenvironment features remain unclear and require further investigation.
Generally speaking, the basal-like immune-associated (BLIA) subtype in Burstein’s subtyping overlaps with FUSCC IM subtype, which responds promisingly to immunotherapy (92). IM group exhibits immune response-related signatures and high expression levels of checkpoint inhibitory genes, including CTLA-4, PD-1, and PD-L1, which could be identified and suggest promising responses for ICIs (65). LAR subtype, also identified as androgen receptor (AR)-positive tumors and accounting for 10-15% of all TNBC, is characterized by a luminal-like gene expression profile, low proliferation rate, and resistance to chemotherapy. Progressing clinical trials suggest that effective androgen suppressors may improve anti-tumoral activity. The BLIS designates a subtype of TNBC that exhibits a particularly unfavorable prognosis and shows a dearth of immune activation by down-regulating B cell, T cell, and natural killer cell immune-regulating pathways, leading to the conjecture that the administration of ICB is unlikely to confer clinical benefits (91, 93). Several scientific literature has provided evidentiary support for a correlation between basal-like breast cancers and the manifestation of CK5/6, CK14, CK17, P-cadherin, p53, and EGFR. Mesenchymal or mesenchymal stem-like subtype was associated with higher angiogenic signature scores and characteristics of breast cancer stem cells (CSCs) (93, 94). The MES subtype exhibits distinct pathways, including cell cycle, mismatch repair, and DNA damage networks. Therefore, the application of beta-blockers, IGF inhibitors, or PDGFR inhibitors may prove to be promising therapeutic strategies for the management of MES tumors (95). The employment of EZH2-inhibitory agents also represents a promising strategy for reinstating MHC-1 expression in immune cold, PD-1 negative M-subtype tumors (47, 94). Despite their utility, current subtyping strategies and biomarkers have notable limitations, particularly in capturing the complex TNBC microenvironment. These strategies focus on molecular characteristics, often neglecting the tumor microenvironment. Future research should develop integrated approaches that consider both molecular and microenvironmental factors to improve patient stratification and treatment outcomes.
Recent advances in TNBC immunotherapy have brought new hope for improving outcomes in TNBC. A deeper exploration of existing and emerging therapeutic approaches is essential for developing effective treatment strategies. Current research highlights the promise of CAR-T cell therapy, immune checkpoint inhibitors, ICIs combination therapies, and inhibitors targeting specific pathways within the tumor microenvironment. As the field progresses, integrating these diverse therapeutic strategies can significantly improve patient outcomes in TNBC. However, the efficacy of these approaches remains inconsistent and often limited, necessitating a critical evaluation of existing and emerging strategies.
Immune checkpoint inhibitors especially PD-1 inhibitors have demonstrated efficacy by restoring immune function and enabling T cells to attack tumor cells. The sole accepted biomarker to identify TNBC patients benefit from ICIs is the expression of PD-L1, evaluated by the CPS and tumor proportion score (TPS) (13, 96, 97), yet the efficacy is not optimized. TNBC exhibits high levels of PD-L1, which promotes researchers to design multiple TNBC clinical trials using PD-L1 inhibitors thereby discovering the clinical benefits of adding ICB as first-line and second-line therapy for TNBC (98). Currently, the US FDA has approved four PD-L1 reagents and six ICIs, including 22C3 for Pembrolizumab, 28-8 for Nivolumab, SP142 for Atezolizumab, and SP263 for Durvalumab. Despite TNBC’s high PD-L1 expression levels, which have led to numerous clinical trials investigating PD-L1 inhibitors, the effectiveness of CPS as a sole biomarker has been questioned. A significant limitation of CPS as sole biomarker is its variable efficacy in different clinical settings, particularly in metastatic TNBC, where immune suppression within the tumor microenvironment can affect outcomes. Furthermore, the FDA has approved two ICIs, Avelumab and Cemiplimab, that do not show clinical association with PD-L1 expression status (97). This suggests that other biomarkers, such as tumor mutation burden (TMB), tumor-infiltrating lymphocytes, and gene expression profiles, might also play critical roles in predicting patient responses to immunotherapy (99, 100). In this context, the evaluation of co-inhibitory (CI) receptors has emerged as an important consideration to enhance the accuracy of patient selection for ICB therapy. The CI receptors, including CTLA4 and PD1, could have crucial but distinct roles in modulating immune responses, highlighting the complexity of the immune landscape in TNBC and the need for a multi-faceted approach to biomarker development (27). Other CI receptors such as immune cells (IC), TMB, LAG-3, TIM-3, and VISTA play critical roles in immune evasion and could provide additional predictive value for immunotherapy outcomes (101–106). Incorporating these CI evaluations alongside PD-L1 status could lead to more precise and personalized treatment strategies, ultimately improving the prognosis for TNBC patients.
Combining ICIs with novel agents such as antibody-drug conjugates (ADCs) and inhibitors targeting specific pathways within the tumor microenvironment (TME) can enhance the overall anti-tumor response. The integration of ICIs with novel antibody-drug conjugates (ADCs) represents a significant advancement in the treatment of TNBC, as suggested by recent clinical trials, such as MORPHEUS-pan BC and BEGONIA. These combination therapies leverage the immune-activating properties of ICIs with the targeted cytotoxic effects of ADCs, providing a dual approach that not only inhibits tumor growth but also stimulates the immune system to attack cancer cells more effectively (107, 124, 109). Specifically, the atezolizumab and sacituzumab govitecan-hziy (Trodelvy) combination has shown promising results in patients with locally advanced or metastatic TNBC (110.). Furthermore, results from Arm 6 and Arm 7 of the BEGONIA trial indicate that the combination of durvalumab and trastuzumab deruxtecan (T-DXd) or datopotamab deruxtecan (Dato-Dxd) holds significant potential for treating TNBC (111). Preliminary results from clinical trials have demonstrated that combining ICIs with novel ADCs can significantly improve overall response rates in TNBC patients. The promising outcomes highlight the potential of such combinations to address the challenges of TNBC treatment, with numerous clinical trials currently underway to further explore their efficacy (Table 2). The co-expression of the inhibitory immune checkpoint lymphocyte activation gene-3 (LAG-3) and PD-1 has been observed in exhausted T cells (112), where higher levels of LAG-3 and PD-L1 expression were detected in patients with TNBC (113). Preclinical research indicates that the inhibition of certain pathways within the immune system enhances the ability of CD8 T cells to fight against tumors. The simultaneous blockage of PD-1 and LAG-3 pathways yields a potent outcome. These findings suggest that targeting these immune checkpoints can improve anti-tumor responses. Moreover, the newly released findings of the I-SPY2 clinical trial, which assessed the efficacy of anti-LAG-3 and anti-PD1 treatment in patients with early-stage HER2-negative breast cancer, indicated a projected pathologic complete response rate of 60% for individuals with hormone receptor HR-negative, HER2-negative disease and 37% for those with HR-positive, HER2-negative disease (114). Therefore, a series of dual blockade approaches targeting LAG-3 and PD-1 is currently undergoing clinical evaluation as a potential treatment option for advanced breast cancer (NCT03742349 and NCT03005782), and double antibodies are under evaluation. Opdualag, combining nivolumab with relatlimab, was currently approved by the FDA for melanoma treatment, which may be a potential treatment for TNBC as these two malignancies share similarities in immune therapy (115) The combination of LAG-3 blockades and PD-1 blockade has been proven to be safe and promising in mTNBC, but the exact efficacy still needs large-scale clinical evaluation, and double antibody deserves investigation.
Molecular subtype-based optimized treatment strategies offer a promising outlook for improving therapeutic outcome in TNBC. Utilizing PD-L1 and PD-1 inhibitors has proven efficient in TNBC immunomodulatory subtype, both early-stage and metastases setting. Based on current TNBC subtypes, individuals diagnosed with TNBC are suggested to undergo preliminary screening to evaluate the expression of PD1 or PD-L1 before contemplating the administration of immunotherapy as a treatment modality. ICIs are advisable if the value of CPS surpasses a threshold of 10 in metastatic TNBC and in all patients in the neoadjuvant setting regardless of CPS score, while additional biomarkers such as LAG-3, TILs may also provide additional therapeutic perspective. As an alternative, it is suggested to perform patient testing to assess the existence of androgen receptors and in the event of positive outcomes, it is supported to pursue pharmacological intervention through anti-androgen receptor therapy. Otherwise, it should be noted that the patient may display indications that are congruous with BLIS or MES categories, and the utilization of DNA profiling may have the ability to differentiate among the subcategories and enable the recognition of ideal pharmaceutical treatments. By categorizing TNBC into molecular subtypes such as PD-L1, LAR, BLIS, and MES, treatment strategies could be tailored more effectively, thereby enhancing therapeutic outcomes. The FUTURE-SUPER trial underscores the clinical advantages of employing molecular subtype-based treatment optimization for patients with TNBC, indicating a direction for further clinical research (116). A series of clinical trials have been conducted based on the subtyping of triple-negative breast cancer (Table 3).
In recent years, CAR-T cell therapy has made significant progress in the treatment of solid tumors, including TNBC. Historically, the immunosuppressive signals within the TME of solid tumors have limited the efficacy of CAR-T cells. In TNBC, research is ongoing to enhance the delivery of tumors and improve the persistence of CAR-T cells. In preclinical studies and early clinical trials, several antigens have been established as viable targets for CAR-T cell therapy in TNBC. NKG2D ligands, expressed on various tumor types and immunosuppressive cells within the tumor microenvironment, present a promising target for cancer therapy (117). In mouse studies, CAR-T cells engineered with derivatives of HLA-A2/NY-ESO-1 have been used in cancer immunotherapy, showing extended overall survival in TNBC and primary melanoma models (125). However, the selection of optimal targets remains a challenge in CAR-T cell therapy to minimize off-target effects and enhance specificity (118). Early results from clinical studies show that CAR-T therapy in TNBC has not led to significant on-target, off-tumor toxicities related to specific targets like ROR1 (119). Clinical and preclinical models have identified numerous antigens suitable for CAR-T cell therapy in TNBC. TROP2, GD2, ROR1, MUC1, and EpCAM have been identified as the most promising targets, and CARs developed against these targets have shown the ability to penetrate and migrate through TNBC cultures, eliciting significant antitumor responses (108, 118, 120–122). To summarize, the successful application of CAR-T cell therapy in TNBC requires overcoming barriers related to the tumor microenvironment and antigen heterogeneity.
In summary, while immunotherapy offers significant promise for TNBC treatment, its current application is hampered by variability in patient response, the need for better biomarkers, and the challenges associated with advanced therapeutic strategies. Future research should focus on optimizing these approaches, improving patient selection criteria, and developing more effective and less toxic combination therapies (99, 100).
Triple-negative breast cancer is a heterogeneous group containing several distinct subtypes. Subtyping TNBC is deemed necessary to properly identify suitable patients for immunotherapy as well as facilitate the identification of optimal alternate treatment protocols for non-responsive patients. TNBC can be classified into different molecular subtypes based on gene expression profiles. Different profiles within each category demonstrate the distinct characteristics of the immune response, metabolism processes, and supporting tissue. Nonetheless, most previous clinical studies have not focused on distinct subpopulations to identify efficacy indicators. Recent research has identified biomarkers for characterizing TNBC subtypes and assessing therapeutic effects of drugs, filling a critical research gap.
TNBC contains a diverse TME that includes TILs, macrophages, neutrophils, DCs, and CAFs. These components can impact tumor growth and the immune response, and each of them holds prognostic value and potential for targeted therapy. TNBC exhibits high immunogenicity through TILs, which are associated with clinical outcomes. TILs can serve as a subtyping biomarker for immune response in TNBC, and personalized TILs immunotherapy shows promise for patients with drug-resistant TNBC. Targeting the macrophage-enriched subtype and reprogramming macrophages from an immunosuppressive M2 phenotype to a pro-inflammatory state can enhance the effectiveness of ICIs. TANs, particularly the immunosuppressive N2 subtype, contribute to ICB resistance; modulating TANs and using the NLR as a prognostic marker can help tailor therapeutic approaches and improve outcomes. Dendritic cell-based therapies, such as vaccines and CIK cell immunotherapy, are being explored to enhance anti-tumor immune responses, including the administration of immunomodulatory antigens and the use of autologous DCs pulsed with tumor antigens for personalized immunotherapy. Targeting specific CAF subpopulations and reducing their immunosuppressive functions can increase T cell accessibility to tumor cells and improve sensitivity to therapies; strategies include CAR-T-cell therapy and monoclonal antibodies targeting CAF-associated markers. To improve the response to immunotherapy, it is crucial to classify TNBC based on TME characteristics and consider combining TME-targeted therapies. Regular monitoring of TME changes using biomarkers can help adjust treatment plans and serve as prognostic indicators. For instance, adopting immune phenotypes and immune identifiers for precise patient selection could improve immunotherapy prognosis. Combining TME-targeted treatments with immunotherapy could address the issue of immunosuppression in the tumor environment, potentially leading to better outcomes.
Ethical considerations are crucial in the development and application of TNBC immunotherapy. The potential for off-target effects, where therapies inadvertently impact non-cancerous tissues, poses significant risks to patients. For instance, immune checkpoint inhibitors can trigger severe immune-related adverse events affecting organs such as the liver, lungs, and endocrine glands. Ensuring thorough preclinical testing and vigilant monitoring during clinical trials can mitigate these risks. Furthermore, the need for informed consent is paramount, as patients must be fully aware of the potential benefits and risks associated with new treatments. Transparent communication about the experimental nature of some therapies and the possibility of adverse effects is essential for ethical clinical practice. Furthermore, equitable access to these novel treatments and considering the socioeconomic factors that may influence patient participation in clinical trials are critical ethical issues.
The management of TNBC is undergoing substantial changes, as the identification and characterization of the distinct molecular profile of the tumors, including the evaluation of PD-L1 and the androgen receptor, are broadening the spectrum of therapeutic interventions available in clinical practice. It’s promising for future research agendas to focus on the identification of additional targetable and innovative biomarkers, which have the potential to define therapeutic targets or prognostic indicators more comprehensively. The treatment of TNBC has evolved beyond a uniform application for all individuals, and subgroup therapeutic regimens are anticipated. Additional clinical trials are being conducted to demonstrate the efficacy of new medications and assess the potential benefits of identifying novel biomarkers. These advancements pave the way for more precise and personalized treatment strategies, ultimately aiming to improve long-term outcomes for patients with TNBC. To further improve the efficacy of TNBC immunotherapy, it is crucial to delve deeper into several key areas. Identifying and validating additional biomarkers for patient stratification and predicting response to immunotherapy is essential. In-depth studies on molecular immune subtyping of TNBC subtypes and clinical research exploring prognosis can enhance treatment strategies. Exploring combination therapies that target multiple components of the TME may provide a more comprehensive approach to overcoming tumor resistance. Personalized immunotherapies based on the unique genetic and immune profiles of individual tumors will allow for more effective treatments. Investigating the mechanisms of resistance to immunotherapy and strategies to overcome them is critical for improving long-term outcomes for patients with TNBC.
To take part in the Resource Identification Initiative, please use the corresponding catalog number and RRID in your current manuscript. For more information about the project and for steps on how to search for an RRID, please click here.
Life Science Identifiers (LSIDs) for ZOOBANK registered names or nomenclatural acts should be listed in the manuscript before the keywords with the following format:
urn:lsid:<Authority>:<Namespace>:<ObjectID>[:<Version>]
YY: Conceptualization, Methodology, Project administration, Visualization, Writing – original draft. HL: Writing – review & editing, Conceptualization, Methodology. YS: Conceptualization, Methodology, Writing – review & editing. WY: Conceptualization, Methodology, Writing – review & editing.
The author(s) declare financial support was received for the research, authorship, and/or publication of this article. This work was supported by the National Key Research and Development Program of China (2021YFE0206300) and the Innovation and Entrepreneurship Training Program of Guangdong Province (S202410558147).
We extend our sincere gratitude to YS for her invaluable guidance and support throughout the preparation of this review paper.
The authors declare that the research was conducted in the absence of any commercial or financial relationships that could be construed as a potential conflict of interest.
All claims expressed in this article are solely those of the authors and do not necessarily represent those of their affiliated organizations, or those of the publisher, the editors and the reviewers. Any product that may be evaluated in this article, or claim that may be made by its manufacturer, is not guaranteed or endorsed by the publisher.
1. Foulkes WD, Smith IE, Reis-Filho JS. Triple-Negative breast cancer. New Engl J Med. (2010) 363:1938–485. doi: 10.1056/NEJMra1001389
2. Brenton JD, Carey LA, Ahmed AA, Caldas C. Molecular classification and molecular forecasting of breast cancer: ready for clinical application? J Clin Oncol. (2005) 23:7350–605. doi: 10.1200/JCO.2005.03.3845
3. Ignatiadis M, McArthur HL, Bailey A, Martinez J, de Azambuja E, Metzger O, et al. (2019). 289TiP - ALEXANDRA/IMpassion030: A phase III study of standard adjuvant chemotherapy with or without atezolizumab in early stage triple negative breast cancer. In: Annals of Oncology. Barcelona, Spain: European Society for Medical Oncology (ESMO), v97. doi: 10.1093/annonc/mdz240.112
4. Ademuyiwa FO, Gao F, Street CR, Chen I, Northfelt DW, Wesolowski R, et al. A randomized phase 2 study of neoadjuvant carboplatin and paclitaxel with or without atezolizumab in triple negative breast cancer (TNBC) - NCI 10013. NPJ Breast Cancer. (2022) 8:1–11. doi: 10.1038/s41523-022-00500-3
5. Mittendorf EA, Zhang H, Barrios CH, Saji S, Jung KH, Hegg R, et al. Neoadjuvant atezolizumab in combination with sequential nab-paclitaxel and anthracycline-based chemotherapy versus placebo and chemotherapy in patients with early-stage triple-negative breast cancer (IMpassion031): A randomised, double-blind, phase 3 trial. Lancet (London England). (2020) 396:1090–100. doi: 10.1016/S0140-6736(20)31953-X
6. Loibl S, Schneeweiss A, Huober J, Braun M, Rey J, Blohmer JU, et al. Neoadjuvant durvalumab improves survival in early triple-negative breast cancer independent of pathological complete response. Ann Oncol. (2022) 33:1149–58. doi: 10.1016/j.annonc.2022.07.1940
7. Gianni L, Huang CS, Egle D, Bermejo B, Zamagni C, Thill M, et al. Pathologic complete response (pCR) to neoadjuvant treatment with or without atezolizumab in triple-negative, early high-risk and locally advanced breast cancer: neoTRIP michelangelo randomized study. Ann Oncology: Off J Eur Soc Med Oncol. (2022) 33:534–43. doi: 10.1016/j.annonc.2022.02.004
8. Pusztai L, Yau C, Wolf DM, Han HS, Du L, Wallace AM, et al. Durvalumab with olaparib and paclitaxel for high-risk HER2-negative stage II/III breast cancer: results from the adaptively randomized I-SPY2 trial. Cancer Cell. (2021) 39:989–998.e5. doi: 10.1016/j.ccell.2021.05.009
9. Schmid P, Rugo HS, Adams S, Schneeweiss A, Barrios CH, Iwata H, et al. Atezolizumab plus nab-paclitaxel as first-line treatment for unresectable, locally advanced or metastatic triple-negative breast cancer (IMpassion130): updated efficacy results from a randomised, double-blind, placebo-controlled, phase 3 trial. Lancet Oncol. (2020) 21:44–59. doi: 10.1016/S1470-2045(19)30689-8
10. Cortes J, Cescon DW, Rugo HS, Nowecki Z, Im S-A, Yusof MMd, et al. Pembrolizumab plus Chemotherapy versus Placebo plus Chemotherapy for Previously Untreated Locally Recurrent Inoperable or Metastatic Triple-Negative Breast Cancer (KEYNOTE-355): A Randomised, Placebo-Controlled, Double-Blind, Phase 3 Clinical Trial. Lancet. (2020) 396:1817–28. doi: 10.1016/S0140-6736(20)32531-9
11. Schmid P, Cortes J, Pusztai L, McArthur H, Kümmel S, Bergh J, et al. Pembrolizumab for early triple-negative breast cancer. New Engl J Med. (2020) 382:810–21. doi: 10.1056/NEJMoa1910549
12. Miles D, Gligorov J, André F, Cameron D, Schneeweiss A, Barrios C, et al. Primary results from IMpassion131, a double-blind, placebo-controlled, randomised phase III trial of first-line paclitaxel with or without atezolizumab for unresectable locally advanced/metastatic triple-negative breast cancer. Ann Oncol. (2021) 32:994–1004. doi: 10.1016/j.annonc.2021.05.801
13. Badve SS, Penault-Llorca Frédérique, Reis-Filho JS, Deurloo R, Siziopikou KP, D’Arrigo C, et al. Determining PD-L1 status in patients with triple-Negative breast cancer: lessons learned from IMpassion130. JNCI: J Natl Cancer Institute. (2022) 114:664–755. doi: 10.1093/jnci/djab121
14. Tarantino P, Corti C, Schmid P, Cortes J, Mittendorf EA, Rugo H, et al. Immunotherapy for early triple negative breast cancer: research agenda for the next decade. NPJ Breast Cancer. (2022) 8:1–75. doi: 10.1038/s41523-022-00386-1
15. Derakhshan F, Reis-Filho JS. Pathogenesis of triple-Negative breast cancer. Annu Rev Pathology: Mech Dis. (2022) 17:181–2045. doi: 10.1146/annurev-pathol-042420-093238
16. Carter JM, Chumsri S, Hinerfeld DA, Ma Y, Wang X, Zahrieh D, et al. Distinct spatial immune microlandscapes are independently associated with outcomes in triple-egative breast cancer. Nat Commun. (2023) 14:1–2. doi: 10.1038/s41467-023-37806-0
17. Bareche Y, Venet D, Ignatiadis M, Aftimos P, Piccart M, Rothe F, et al. Unravelling triple-negative breast cancer molecular heterogeneity using an integrative multiomic analysis. Ann Oncol. (2018) 29:895–902. doi: 10.1093/annonc/mdy024
18. Liu J, Wang Y, Mu C, Li M, Li K, Li S, et al. Pancreatic tumor eradication via selective pin1 inhibition in cancer-associated fibroblasts and T lymphocytes engagement. Nat Commun. (2022) 13:4308. doi: 10.1038/s41467-022-31928-7
19. Losurdo A, Sanctis RDe, Fernandes B, Errico V, Sagona A, Masci G, et al. A monocentric retrospective analysis of TILs and AR as hints for prognosis definition in TNBC. J Clin Oncol. (2018) 36:e12580–0. doi: 10.1200/JCO.2018.36.15_suppl.e12580
20. Blackley EF, Loi S. Targeting immune pathways in breast cancer: review of the prognostic utility of TILs in early stage triple negative breast cancer (TNBC) 1. Breast. (2019) 48:S44–48. doi: 10.1016/S0960-9776(19)31122-1
21. Loi S, Michiels S, Adams S, Loibl S, Budczies J, Denkert C, et al. The journey of tumor-infiltrating lymphocytes as a biomarker in breast cancer: clinical utility in an era of checkpoint inhibition. Ann Oncol. (2021) 32:1236–44. doi: 10.1016/j.annonc.2021.07.007
22. Voorwerk L, Slagter M, Horlings HM, Sikorska K, Vijver KKVDe, Maaker MDe, et al. Immune induction strategies in metastatic triple-negative breast cancer to enhance the sensitivity to PD-1 blockade: the TONIC trial. Nat Med. (2019) 25:920–28. doi: 10.1038/s41591-019-0432-4
23. Hu W, Zi Z, Jin Y, Li G, Shao K, Cai Q, et al. CRISPR/cas9-mediated PD-1 disruption enhances human mesothelin-targeted CAR T cell effector functions. Cancer Immunology Immunotherapy. (2019) 68:365–775. doi: 10.1007/s00262-018-2281-2
24. Gou Q, Liu Z, Xie Y, Deng Y, Ma Ji, Li J, et al. Systematic evaluation of tumor microenvironment and construction of a machine learning model to predict prognosis and immunotherapy efficacy in triple-negative breast cancer based on data mining and sequencing validation. Front Pharmacol. (2022) 13:995555. doi: 10.3389/fphar.2022.995555
25. Jiang Y-Z, Liu Y, Xiao Yi, Hu X, Jiang L, Zuo W-J, et al. Molecular subtyping and genomic profiling expand precision medicine in refractory metastatic triple-negative breast cancer: the FUTURE trial. Cell Res. (2021) 31:178–86. doi: 10.1038/s41422-020-0375-9
26. Watson RA, Tong O, Cooper R, Taylor CA, Sharma PK, Aires AVdel, et al. Immune checkpoint blockade sensitivity and progression-free survival associates with baseline CD8+ T cell clone size and cytotoxicity. Sci Immunol. (2021) 6:eabj8825. doi: 10.1126/sciimmunol.abj8825
27. Deepak KGK, Vempati R, Nagaraju GP, Dasari VR, Nagini S, Rao DN, et al. Tumor microenvironment: challenges and opportunities in targeting metastasis of triple negative breast cancer. Pharmacol Res. (2020) 153:104683. doi: 10.1016/j.phrs.2020.104683
28. Bottai G, Raschioni C, Losurdo A, Tommaso LDi, Tinterri C, Torrisi R, et al. An immune stratification reveals a subset of PD-1/LAG-3 double-positive triple-negative breast cancers. Breast Cancer Research : BCR. (2016) 18:121. doi: 10.1186/s13058-016-0783-4
29. Kim SK, Cho SW. The evasion mechanisms of cancer immunity and drug intervention in the tumor microenvironment. Front Pharmacol. (2022) 13:868695. doi: 10.3389/fphar.2022.868695
30. Zacharakis N, Chinnasamy H, Black M, Xu H, Lu Y-C, Zheng Z, et al. Immune recognition of somatic mutations leading to complete durable regression in metastatic breast cancer. Nat Med. (2018) 24:724–30. doi: 10.1038/s41591-018-0040-8
31. Kazemi MH, Sadri M, Najafi A, Rahimi A, Baghernejadan Z, Khorramdelazad H, et al. Tumor-infiltrating lymphocytes for treatment of solid tumors: it takes two to tango. Front Immunol. (2022) 13:1018962. doi: 10.3389/fimmu.2022.1018962
32. Creelan BC, Wang C, Teer JK, Toloza EM, Yao J, Kim S, et al. Tumor-infiltrating lymphocyte treatment for anti-PD-1 resistant metastatic lung cancer: A phase I trial. Nat Med. (2021) 27:1410–18. doi: 10.1038/s41591-021-01462-y
33. Pe KCS, Saetung R, Yodsurang V, Chaotham C, Suppipat K, Chanvorachote P, et al. Triple-Negative breast cancer influences a mixed M1/M2 macrophage phenotype associated with tumor aggressiveness. PloS One. (2022) 17:e02730445. doi: 10.1371/journal.pone.0273044
34. Santoni M, Romagnoli E, Saladino T, Foghini L, Guarino S, Capponi M, et al. Triple negative breast cancer: key role of tumor-associated macrophages in regulating the activity of anti-PD-1/PD-L1 agents. Biochim Biophys Acta (BBA) - Rev Cancer. (2018) 1):78–84. doi: 10.1016/j.bbcan.2017.10.007
35. Kim C, Gao R, Sei E, Brandt R, Hartman J, Hatschek T, et al. Chemoresistance evolution in triple-Negative breast cancer delineated by single cell sequencing. Cell. (2018) 173:879–8935.e13. doi: 10.1016/j.cell.2018.03.041
36. Bobrie Angélique, Massol Océane, Ramos J, Mollevi C, Lopez-Crapez E, Bonnefoy N, et al. Association of CD206 protein expression with immune infiltration and prognosis in patients with triple-Negative breast cancer. Cancers. (2022) 14:48295. doi: 10.3390/cancers14194829
37. SenGupta S, Hein LE, Xu Y, Zhang J, Konwerski J, Li Ye, et al. Triple-negative breast cancer cells recruit neutrophils by secreting tgf-β And cxcr2 ligands. bioRxiv. (2021) 12:10–14. doi: 10.1101/2021.01.28.428630
38. McKenna E, Mhaonaigh AUi, Wubben R, Dwivedi A, Hurley T, Kelly LA, et al. Neutrophils: need for standardized nomenclature. Front Immunol. (2021) 12:602963. doi: 10.3389/fimmu.2021.602963
39. So JY, Ohm J, Lipkowitz S, Yang. Li. Triple negative breast cancer (TNBC): non-genetic tumor heterogeneity and immune microenvironment: emerging treatment options. Pharmacol Ther. (2022) 237:108253. doi: 10.1016/j.pharmthera.2022.108253
40. Marwick JA, Mills R, Kay O, Michail K, Stephen J, Rossi AG, et al. Neutrophils induce macrophage anti-Inflammatory reprogramming by suppressing NF-κB activation. Cell Death Dis. (2018) 9:6655. doi: 10.1038/s41419-018-0710-y
41. Pearson A, Justine P, Diakos CI, Dewar R, Chan D, Guminski AD, et al. Prognostic utility of tumour infiltrating lymphocytes (TILs) and neutrophil-to-lymphocyte ratio (NLR) in early-stage triple negative breast cancer (TNBC). J Clin Oncol. (2016) 34:1075–5. doi: 10.1200/JCO.2016.34.15_suppl.1075
42. Tokumaru Y, Oshi M, Murthy V, Tian W, Yan Li, Angarita FA, et al. Low intratumoral genetic neutrophil-to-lymphocyte ratio (NLR) is associated with favorable tumor immune microenvironment and with survival in triple negative breast cancer (TNBC). Am J Cancer Res. (2021) 11:5743–55.
43. Heemskerk N, Egmond Mv. Monoclonal antibody-mediated killing of tumour cells by neutrophils. Eur J Clin Invest. (2018) 48:e12962. doi: 10.1111/eci.12962
44. Binnewies M, Mujal AM, Pollack JL, Combes AJ, Hardison EA, Barry KC, et al. Unleashing type-2 dendritic cells to drive protective antitumor CD4+ T cell immunity. Cell. (2019) 177:556–571.e16. doi: 10.1016/j.cell.2019.02.005
45. Wculek SK, Cueto FJ, Mujal AM, Melero I, Krummel MF, David S. Dendritic cells in cancer immunology and immunotherapy. Nat Rev Immunol. (2020) 20:7–245. doi: 10.1038/s41577-019-0210-z
46. Munoz LE, Monterroza L, Bommireddy R, Shafizadeh Y, Pack CD, Ramachandiran S, et al. Dendritic cells pulsed with cytokine-Adjuvanted tumor membrane vesicles inhibit tumor growth in HER2-Positive and triple negative breast cancer models. Int J Mol Sci. (2021) 22:83775. doi: 10.3390/ijms22168377
47. Huang L, Rong Y, Tang X, Yi K, Qi P, Hou J, et al. Engineered exosomes as an in situ DC-primed vaccine to boost antitumor immunity in breast cancer. Mol Cancer. (2022) 21:45. doi: 10.1186/s12943-022-01515-x
48. Huo W, Yang X, Wang B, Cao L, Fang Z, Li Z, et al. Biomineralized hydrogel DC vaccine for cancer immunotherapy: A boosting strategy via improving immunogenicity and reversing immune-inhibitory microenvironment. Biomaterials. (2022) 288:121722. doi: 10.1016/j.biomaterials.2022.121722
49. Wang L, Dai Y, Zhu F, Qiu Z, Wang Y, Yinghui Hu Efficacy of DC-CIK-Based immunotherapy combined with chemotherapy in the treatment of intermediate to advanced non-Small cell lung cancer. Am J Trans Res. (2021) 13:13076–835.
50. Guo Y, Weidong H. Cytokine-Induced killer (CIK) cells: from basic research to clinical translation. Chin J Cancer. (2015) 34:65. doi: 10.1186/s40880-015-0002-1
51. Yang X, Weng D, Pan Q, Xiang T, Yang C, Wu Z, et al. Adjuvant alternative cytokine-induced killer cell combined with natural killer cell immunotherapy improves the prognosis of post-mastectomy breast cancer. Front Immunol. (2022) 13:974487. doi: 10.3389/fimmu.2022.974487
52. Sarkar M, Nguyen T, Gundre E, Ogunlusi O, El-Sobky M, Giri B, et al. Cancer-associated fibroblasts: the chief architect in the tumor microenvironment. Front Cell Dev Biol. (2023) 11:1089068. doi: 10.3389/fcell.2023.1089068
53. Yang K, Yufeng Y. Mechanism of GPER promoting proliferation, migration and invasion of triple-Negative breast cancer cells through CAF. Am J Trans Res. (2019) 11:5858–685.
54. Costa A, Kieffer Y, Scholer-Dahirel A, Pelon F, Bourachot B, Cardon M, et al. Fibroblast heterogeneity and immunosuppressive environment in human breast cancer. Cancer Cell. (2018) 33:463–479.e10. doi: 10.1016/j.ccell.2018.01.011
55. Timperi E, Gueguen P, Molgora M, Magagna I, Kieffer Y, Lopez-Lastra S, et al. Lipid-associated macrophages are induced by cancer-associated fibroblasts and mediate immune suppression in breast cancer. Cancer Res. (2022) 82:3291–306. doi: 10.1158/0008-5472.CAN-22-1427
56. Zheng S, Zou Y, Tang Y, Yang A, Liang J-Y, Wu L, et al. Landscape of cancer-associated fibroblasts identifies the secreted biglycan as a protumor and immunosuppressive factor in triple-negative breast cancer. Oncoimmunology. (2022) 11:20209845. doi: 10.1080/2162402X.2021.2020984
57. Sebastian A, Hum NR, Martin KA, Gilmore SF, Peran I, Byers SW, et al. Single-Cell transcriptomic analysis of tumor-Derived fibroblasts and normal tissue-Resident fibroblasts reveals fibroblast heterogeneity in breast cancer. Cancers. (2020) 12:13075. doi: 10.3390/cancers12051307
58. Sakemura R, Hefazi M, Siegler EL, Cox MJ, Larson DP, Hansen MJ, et al. Targeting cancer-associated fibroblasts in the bone marrow prevents resistance to CART-cell therapy in multiple myeloma. Blood. (2022) 139:3708–21. doi: 10.1182/blood.2021012811
59. Hanley CJ, Thomas GJ. T-cell tumour exclusion and immunotherapy resistance: A role for CAF targeting. Br J Cancer. (2020) 123:1353–555. doi: 10.1038/s41416-020-1020-6
60. Wang D, Gao S, Qian H, Yuan P, Zhang B. Prognostic value of copy number alteration burden in early-Stage breast cancer and the construction of an 11-Gene copy number alteration model. Cancers. (2022) 14:41455. doi: 10.3390/cancers14174145
61. Li Z, Zhang X, Hou C, Zhou Y, Chen J, Cai H, et al. Comprehensive identification and characterization of somatic copy number alterations in triple-Negative breast cancer. Int J Oncol. (2019) 56:522–305. doi: 10.3892/ijo.2019.4950
62. Salehi S, Kabeer F, Ceglia N, Andronescu M, Williams MJ, Campbell KR, et al. Clonal fitness inferred from time-series modelling of single-cell cancer genomes. Nature. (2021) 595:585–90. doi: 10.1038/s41586-021-03648-3
63. Bareche Y, Buisseret L, Gruosso T, Girard E, Venet D, Dupont F, et al. Unraveling triple-negative breast cancer tumor microenvironment heterogeneity: towards an optimized treatment approach. JNCI: J Natl Cancer Institute. (2020) 112:708–19. doi: 10.1093/jnci/djz208
64. Liu Y-R, Jiang Y-Z, Xu X-E, Yu K-D, Jin Xi, Hu X, et al. Comprehensive transcriptome analysis identifies novel molecular subtypes and subtype-specific RNAs of triple-negative breast cancer. Breast Cancer Res. (2016) 18:33. doi: 10.1186/s13058-016-0690-8
65. Lehmann BD, Jovanović B, Chen Xi, Estrada MV, Johnson KN, Shyr Yu, et al. Refinement of triple-Negative breast cancer molecular subtypes: implications for neoadjuvant chemotherapy selection. PloS One. (2016) 11:e01573685. doi: 10.1371/journal.pone.0157368
66. Lehmann BD, Bauer JA, Chen Xi, Sanders ME, Chakravarthy AB, Shyr Yu, et al. Identification of human triple-Negative breast cancer subtypes and preclinical models for selection of targeted therapies. J Clin Invest. (2011) 121:2750–675. doi: 10.1172/JCI45014
67. Sasaki K, Takahashi S, Ouchi K, Otsuki Y, Wakayama S, Ishioka C. Different impacts of TP53 mutations on cell cycle-related gene expression among cancer types. Sci Rep. (2023) 13:4868. doi: 10.1038/s41598-023-32092-8
68. Yi D, Xu L, Luo J, You X, Huang T, Zi Yi, et al. Germline TP53 and MSH6 mutations implicated in sporadic triple-negative breast cancer (TNBC): A preliminary study. Hum Genomics. (2019) 13:4. doi: 10.1186/s40246-018-0186-y
69. Loizides S, Constantinidou A. Triple negative breast cancer: immunogenicity, tumor microenvironment, and immunotherapy. Front Genet. (2023) 13:1095839. doi: 10.3389/fgene.2022.1095839
70. Fu X, Tan W, Song Q, Pei H, Li J. BRCA1 and breast cancer: molecular mechanisms and therapeutic strategies. Front Cell Dev Biol. (2022) 10. doi: 10.3389/fcell.2022.813457
71. Zhao J, Xie X. Prediction of prognosis and immunotherapy response in breast cancer based on neutrophil extracellular traps-related classification. Front Mol Biosci. (2023) 10. doi: 10.3389/fmolb.2023.1165776
72. Davis RT, Blake K, Ma D, Mari B, Gabra I, Hernandez GA, et al. Transcriptional diversity and bioenergetic shift in human breast cancer metastasis revealed by single-cell RNA sequencing. Nat Cell Biol. (2020) 22:310–20. doi: 10.1038/s41556-020-0477-0
73. Hinohara K, Kornelia P. Intratumoral heterogeneity: more than just mutations. Trends Cell Biol. (2019) 29:569–795. doi: 10.1016/j.tcb.2019.03.003
74. Sharma A, Merritt E, Hu X, Cruz A, Jiang C, Sarkodie H, et al. Non-Genetic intra-Tumor heterogeneity is a major predictor of phenotypic heterogeneity and ongoing evolutionary dynamics in lung tumors. Cell Rep. (2019) 29:2164–21745.e5. doi: 10.1016/j.celrep.2019.10.045
75. Sheffield NC, Pierron G, Klughammer J, Datlinger P, Schönegger A, Schuster M, et al. DNA methylation heterogeneity defines a disease spectrum in ewing sarcoma. Nat Med. (2017) 23:386–95. doi: 10.1038/nm.4273
76. Silwal-Pandit L, Nord S, Gythfeldt HVDL, Møller EK, Fleischer T, Rødland E, et al. The longitudinal transcriptional response to neoadjuvant chemotherapy with and without bevacizumab in breast cancer. Clin Cancer Res. (2017) 23:4662–70. doi: 10.1158/1078-0432.CCR-17-0160
77. Thorsson Vésteinn, Gibbs DL, Brown SD, Wolf D, Bortone DS, Yang T-HOu, et al. The immune landscape of cancer. Immunity. (2018) 48:812–830.e14. doi: 10.1016/j.immuni.2018.03.023
78. Baylin SB, Jones PA. A decade of exploring the cancer epigenome — Biological and translational implications. Nat Rev Cancer. (2011) 11:726–345. doi: 10.1038/nrc3130
79. Rinaldi G, Rossi M, Fendt S-M. Metabolic interactions in cancer: cellular metabolism at the interface between the microenvironment, the cancer cell phenotype and the epigenetic landscape. WIREs Syst Biol Med. (2018) 10:9–12. doi: 10.1002/wsbm.1397
80. Chi P, Allis CD, Gang Greg W. Covalent histone modifications — Miswritten, misinterpreted and mis-erased in human cancers. Nat Rev Cancer. (2010) 10:457–695. doi: 10.1038/nrc2876
81. Sarthy JF, Henikoff S, Kami A. Chromatin bottlenecks in cancer. Trends Cancer. (2019) 5:183–945. doi: 10.1016/j.trecan.2019.01.003
82. Zhao S, Allis CD, Wang GG. The language of chromatin modification in human cancers. Nat Rev Cancer. (2021) 21:413–305. doi: 10.1038/s41568-021-00357-x
83. Meyer B, Clifton S, Locke W, Luu P-L, Du Q, Lam D, et al. Identification of DNA methylation biomarkers with potential to predict response to neoadjuvant chemotherapy in triple-negative breast cancer. Clin Epigenet. (2021) 13:226. doi: 10.1186/s13148-021-01210-6
84. Deblois Geneviève, Tonekaboni SAM, Grillo G, Martinez C, Kao YI, Tai F, et al. Epigenetic switch-induced viral mimicry evasion in chemotherapy-resistant breast cancer. Cancer Discovery. (2020) 10:1312–29. doi: 10.1158/2159-8290.CD-19-1493
85. Wu SZ, Al-Eryani G, Roden DL, Junankar S, Harvey K, Andersson A, et al. A single-cell and spatially resolved atlas of human breast cancers. Nat Genet. (2021) 53:1334–47. doi: 10.1038/s41588-021-00911-1
86. O’Shaughnessy J, Moroose RL, Babu S, Baramidze K, Chan D, Leitner SP, et al. Results of ENCORE 602 (TRIO025), a phase II, randomized, placebo-controlled, double-blinded, multicenter study of atezolizumab with or without entinostat in patients with advanced triple-negative breast cancer (aTNBC). J Clin Oncol. (2020) 38:1014–4. doi: 10.1200/JCO.2020.38.15_suppl.1014
87. Llinàs-Arias P, Íñiguez-Muñoz S, McCann K, Voorwerk L, Orozco JIJ, Ensenyat-Mendez M, et al. Epigenetic regulation of immunotherapy response in triple-Negative breast cancer. Cancers. (2021) 13:41395. doi: 10.3390/cancers13164139
88. Gruosso T, Gigoux M, Manem VSK, Bertos N, Zuo D, Perlitch I, et al. Spatially distinct tumor immune microenvironments stratify triple-negative breast cancers. J Clin Invest. (2019) 129:1785–800. doi: 10.1172/JCI96313
89. Sigurjonsdottir Gudbjörg, Marchi TDe, Ehinger A, Hartman J, Bosch A, Staaf J, et al. Comparison of SP142 and 22C3 PD-L1 assays in a population-Based cohort of triple-Negative breast cancer patients in the context of their clinically established scoring algorithms. Breast Cancer Res. (2023) 25:1235. doi: 10.1186/s13058-023-01724-2
90. Garrido-Castro AC, Lin NU, Polyak K. Insights into molecular classifications of triple-Negative breast cancer: improving patient selection for treatment. Cancer Discovery. (2019) 9:176–985. doi: 10.1158/2159-8290.CD-18-1177
91. Burstein MD, Tsimelzon A, Poage GM, Covington KR, Contreras A, Fuqua SAW, et al. Comprehensive genomic analysis identifies novel subtypes and targets of triple-negative breast cancer. Clin Cancer Res. (2015) 21:1688–98. doi: 10.1158/1078-0432.CCR-14-0432
92. Marra A, Trapani D, Viale G, Criscitiello C, Giuseppe C. Practical classification of triple-Negative breast cancer: intratumoral heterogeneity, mechanisms of drug resistance, and novel therapies. NPJ Breast Cancer. (2020) 6:1–165. doi: 10.1038/s41523-020-00197-2
93. Jiang Y-Z, Ma D, Suo C, Shi J, Xue M, Hu X, et al. Genomic and transcriptomic landscape of triple-negative breast cancers: subtypes and treatment strategies. Cancer Cell. (2019) 35:428–440.e5. doi: 10.1016/j.ccell.2019.02.001
94. Lehmann BD, Colaprico A, Silva TC, Chen J, An H, Ban Y, et al. Multi-omics analysis identifies therapeutic vulnerabilities in triple-negative breast cancer subtypes. Nat Commun. (2021) 12:2–7. doi: 10.1038/s41467-021-26502-6
95. Rigiracciolo DC, Nohata N, Lappano R, Cirillo F, Talia M, Scordamaglia D, et al. IGF-1/IGF-1R/FAK/YAP transduction signaling prompts growth effects in triple-negative breast cancer (TNBC) cells. Cells. (2020) 9:10105. doi: 10.3390/cells9041010
96. Noske A, Wagner D-C, Schwamborn K, Foersch S, Steiger K, Kiechle M, et al. Interassay and interobserver comparability study of four programmed death-ligand 1 (PD-L1) immunohistochemistry assays in triple-negative breast cancer. Breast (Edinburgh Scotland). (2021) 60:238–44. doi: 10.1016/j.breast.2021.11.003
97. Doroshow DB, Bhalla S, Beasley MB, Sholl LM, Kerr KM, Gnjatic S, et al. PD-L1 as a biomarker of response to immune-checkpoint inhibitors. Nat Rev Clin Oncol. (2021) 18:345–625. doi: 10.1038/s41571-021-00473-5
98. Keilson JM, Knochelmann HM, Paulos CM, KudChadkar RR, Lowe MC. The evolving landscape of immunotherapy in solid tumors. J Surg Oncol. (2021) 123:798–8065. doi: 10.1002/jso.26416
99. Powles T, Sridhar SS, Loriot Y, Bellmunt J, Mu XJ, Ching KA, et al. Avelumab maintenance in advanced urothelial carcinoma: biomarker analysis of the phase 3 JAVELIN bladder 100 trial. Nat Med. (2021) 27:2200–11. doi: 10.1038/s41591-021-01579-0
100. Choucair K, Morand S, Stanbery L, Edelman G, Dworkin L, Nemunaitis. J. TMB: A promising immune-response biomarker, and potential spearhead in advancing targeted therapy trials. Cancer Gene Ther. (2020) 27:841–535. doi: 10.1038/s41417-020-0174-y
101. Murga-Zamalloa CA, Brown NA, Wilcox RA. Expression of the checkpoint receptors LAG-3, TIM-3 and VISTA in peripheral T cell lymphomas. J Clin Pathol. (2020) 73:197–2035. doi: 10.1136/jclinpath-2019-206117
102. Cai L, Li Y, Tan J, Xu L, Li Y. Targeting LAG-3, TIM-3, and TIGIT for cancer immunotherapy. J Hematol Oncol. (2023) 16:1015. doi: 10.1186/s13045-023-01499-1
103. Rugo HS, Loi S, Adams S, Schmid P, Schneeweiss A, Barrios CH, et al. PD-L1 immunohistochemistry assay comparison in atezolizumab plus nab-paclitaxel-treated advanced triple-negative breast cancer. J Natl Cancer Institute. (2021) 113:1733–43. doi: 10.1093/jnci/djab108
104. Emens LA, Molinero L, Loi S, Rugo HS, Schneeweiss A, Diéras Véronique, et al. Atezolizumab and nab-paclitaxel in advanced triple-negative breast cancer: biomarker evaluation of the IMpassion130 study. J Natl Cancer Institute. (2021) 113:1005–16. doi: 10.1093/jnci/djab004
105. O’Meara TA, Tolaney SM. Tumor mutational burden as a predictor of immunotherapy response in breast cancer. Oncotarget. (2021) 12:394–4005. doi: 10.18632/oncotarget.27877
106. Narayan P, Wahby S, Gao JJ, Amiri-Kordestani L, Ibrahim A, Bloomquist E, et al. FDA approval summary: atezolizumab plus paclitaxel protein-bound for the treatment of patients with advanced or metastatic TNBC whose tumors express PD-L1. Clin Cancer Research: Off J Am Assoc Cancer Res. (2020) 26:2284–89. doi: 10.1158/1078-0432.CCR-19-3545
107. Lu L, Niu Z, Chao Z, Fu C, Chen K, Shi Y. Exploring the therapeutic potential of ADC combination for triple-Negative breast cancer. Cell Mol Life Sci. (2023) 80:3505. doi: 10.1007/s00018-023-04946-x
108. Li D, Guo X, Yang K, Yang Y, Zhou W, Huang Y, et al. EpCAM-targeting CAR-T cell immunotherapy is safe and efficacious for epithelial tumors. Sci Adv. (2023) 9:eadg9721. doi: 10.1126/sciadv.adg9721
109. Nucera S, Conti C, Martorana F, Wilson B, Genta S. Antibody-Drug conjugates to promote immune surveillance: lessons learned from breast cancer. Biomedicines. (2024) 12:14915. doi: 10.3390/biomedicines12071491
110. Oh D-Y, Al-Batran S-E, Chung HC, Hollebecque A, Iqbal S, Kim K-P, et al. MORPHEUS: A phase ib/II trial platform evaluating the safety and efficacy of multiple cancer immunotherapy combinations in patients with gastric or pancreatic cancer. (2018) J Clin Oncol. 36:1.
111. Schmid P, Im S-A, Armstrong A, Park YH, Chung W-P, Nowecki Z, et al. BEGONIA: phase 1b/2 study of durvalumab (D) combinations in locally advanced/metastatic triple-negative breast cancer (TNBC)—Initial results from arm 1, D+paclitaxel (P), and arm 6, D+trastuzumab deruxtecan (T-DXd). J Clin Oncol. (2021) 39:1023–3. doi: 10.1200/JCO.2021.39.15_suppl.1023
112. Anderson AC, Joller N, Kuchroo. VK. Lag-3, tim-3, and TIGIT: co-Inhibitory receptors with specialized functions in immune regulation. Immunity. (2016) 44:989–10045. doi: 10.1016/j.immuni.2016.05.001
113. Tahtaci Gözde, Günel N, Sadi̇Oğlu A, Akyürek N, Boz Oğulcan, Üner Aytuğ. LAG-3 expression in tumor microenvironment of triple-negative breast cancer. Turkish J Med Sci. (2023) 53:142–485. doi: 10.55730/1300-0144.5567
114. Isaacs C, Nanda R, Chien Jo, Trivedi MS, Stringer-Reasor E, Vaklavas C, et al. Abstract GS5-03: evaluation of anti-PD-1 cemiplimab plus anti-LAG-3 REGN3767 in early-stage, high-risk HER2-negative breast cancer: results from the neoadjuvant I-SPY 2 TRIAL. Cancer Res. (2023) 83:GS5–03-GS5-03. doi: 10.1158/1538-7445.SABCS22-GS5-03
115. Kaplon Hélène, Crescioli S, Chenoweth A, Visweswaraiah J, Reichert JM. Antibodies to watch in 2023. mAbs. (2023) 15:21534105. doi: 10.1080/19420862.2022.2153410
116. Fan L, Wang Z-H, Ma L-X, Wu S-Y, Wu J, Yu K-D, et al. Optimising first-line subtyping-based therapy in triple-negative breast cancer (FUTURE-SUPER): A multi-cohort, randomised, phase 2 trial. Lancet Oncology. (2024) 25:184–97. doi: 10.1016/S1470-2045(23)00579-X
117. Han Y, Xie W, Song D-G, Powell DJ. Control of triple-negative breast cancer using ex vivo self-enriched, costimulated NKG2D CAR T cells. J Hematol Oncol. (2018) 11:92. doi: 10.1186/s13045-018-0635-z
118. Corti C, Venetis K, Sajjadi E, Zattoni L, Curigliano G, Nicola F. CAR-T cell therapy for triple-negative breast cancer and other solid tumors: preclinical and clinical progress. Expert Opin Investigational Drugs. (2022) 31:593–6055. doi: 10.1080/13543784.2022.2054326
119. Xie Y, Hu Yi, Zhou N, Yao C, Wu L, Liu L, et al. CAR T-cell therapy for triple-negative breast cancer: where we are. Cancer Lett. (2020) 491:121–31. doi: 10.1016/j.canlet.2020.07.044
120. Wallstabe L, Göttlich C, Nelke LC, Kühnemundt J, Schwarz T, Nerreter T, et al. ROR1-CAR T cells are effective against lung and breast cancer in advanced microphysiologic 3D tumor models (Accessed July 26, 2024).
121. Seitz CM, Schroeder S, Knopf P, Krahl A-C, Hau J, Schleicher S, et al. GD2-targeted chimeric antigen receptor T cells prevent metastasis formation by elimination of breast cancer stem-like cells. OncoImmunology January. (2020) 9: 4–10. doi: 10.1080/2162402X.2019.1683345
122. Gutierrez R, Shah P, Hamid O, Garfall A, Posey A, Bishop M, et al. Phase I experience with first in class tnMUC1 targeted chimeric antigen receptor T-cells in patients with advanced tnMUC1 positive solid tumors. J Clin Oncol. (2021) 39:e14513–3. doi: 10.1200/JCO.2021.39.15_suppl.e14513
123. Connolly RM, Li H, Jankowitz RC, Zhang Z, Rudek MA, Jeter SC, et al. Combination epigenetic therapy in advanced breast cancer with 5-azacitidine and entinostat: a phase II national cancer institute/stand up to cancer study. Clin Cancer Res : An Off J of the Am Assoc for Cancer Res. (2017) 23(11):2691–701. doi: 10.1158/1078-0432.CCR-16-1729
124. Li L, Zhang F, Liu Z, Fan Z. Immunotherapy for triple-negative breast cancer: combination strategies to improve outcome. Cancers. (2023) 15:3215. doi: 10.3390/cancers15010321
125. Liu X, Xu Y, Xiong W, Yin B, Huang Y, Chu J, et al. Development of a TCR-like antibody and chimeric antigen receptor against NY-ESO-1/HLA-A2 for cancer immunotherapy. J Immunotherapy Cancer. (2022) 10:e004035. doi: 10.1136/jitc-2021-004035
Keywords: immunotherapy, breast cancer, microenvironment, TNBC, immune subtype, therapeutic target, biomarker
Citation: Yang Y, Li H, Yang W and Shi Y (2024) Improving efficacy of TNBC immunotherapy: based on analysis and subtyping of immune microenvironment. Front. Immunol. 15:1441667. doi: 10.3389/fimmu.2024.1441667
Received: 31 May 2024; Accepted: 10 September 2024;
Published: 04 October 2024.
Edited by:
Vijay Kumar, Morehouse School of Medicine, United StatesReviewed by:
Luis De La Cruz-Merino, Virgen Macarena University Hospital, SpainCopyright © 2024 Yang, Li, Yang and Shi. This is an open-access article distributed under the terms of the Creative Commons Attribution License (CC BY). The use, distribution or reproduction in other forums is permitted, provided the original author(s) and the copyright owner(s) are credited and that the original publication in this journal is cited, in accordance with accepted academic practice. No use, distribution or reproduction is permitted which does not comply with these terms.
*Correspondence: Yanxia Shi, c2hpeXhAc3lzdWNjLm9yZy5jbg==
Disclaimer: All claims expressed in this article are solely those of the authors and do not necessarily represent those of their affiliated organizations, or those of the publisher, the editors and the reviewers. Any product that may be evaluated in this article or claim that may be made by its manufacturer is not guaranteed or endorsed by the publisher.
Research integrity at Frontiers
Learn more about the work of our research integrity team to safeguard the quality of each article we publish.