- 1Department of Gastric Surgery, Liaoning Cancer Hospital and Institute, Cancer Hospital of China Medical University, Shenyang, China
- 2Department of Bone and Soft Tissue Tumor Surgery, Cancer Hospital of China Medical University, Liaoning Cancer Hospital & Institute, Shenyang, China
Mucosal melanoma (MM) poses a significant clinical challenge due to its aggressive nature and limited treatment options. In recent years, immunotherapy has emerged as a promising strategy for MM, with a particular focus on immune checkpoint inhibitors such as PD-1 and CTLA-4 inhibitors. These inhibitors have demonstrated substantial efficacy by harnessing the body’s immune response against tumors. Moreover, adoptive cell transfer (ACT), anti-angiogenic therapy, and combination therapies have garnered attention for their potential in MM treatment. ACT involves modifying T cells to target melanoma cells, showing promising antitumor activity. Anti-angiogenic therapy aims to impede tumor growth by inhibiting angiogenesis, while combination therapies, including immune checkpoint inhibitors and targeted therapies, offer a multifaceted approach to overcome treatment resistance. This comprehensive review explores the advancements in immunotherapy for MM, highlighting the role of diverse therapeutic modalities in enhancing treatment outcomes and addressing the challenges posed by this aggressive malignancy.
1 Introduction
Melanoma, a malignancy arising from the transformation of melanocytes, which are cells originating from the neuroectoderm and responsible for melanin synthesis, presents as a malignant tumor characterized by early metastasis, increased invasiveness, and an unfavorable prognosis (1, 2). Over recent years, there has been a consistent rise in the annual incidence and mortality rates of melanoma. Mucosal melanoma (MM), originating from mucosal tissues, typically presents as solitary lentil-shaped cells adhering to the surface of mucosal squamous epithelial cells (3, 4). Despite its representation in only 1-5% of all melanoma cases, MM poses considerable challenges due to its heightened malignancy. Classification of MM is predominantly based on the primary site, which may include melanomas originating from the head and neck (nasopharynx and oral cavity), gastrointestinal tract (upper and lower digestive tract), urinary system, respiratory system, and other anatomical regions (5, 6). Mucosal melanoma (MM) is a rare subset of melanoma characterized by its lower incidence rate compared to cutaneous melanoma. Despite its infrequent occurrence, MM presents significant challenges due to its heightened malignancy and treatment complexities. However, there are reports suggesting a more favorable prognosis for MM patients. Zhang et al. conducted an analysis and found that MM patients had improved prognoses compared to Chinese cutaneous melanoma patients. This study made key observations regarding disparities in progression-free survival (PFS) between mucosal and cutaneous melanoma patients, the impact of age on MM prognosis, as well as the crucial role of staging and treatment regimens in cutaneous melanoma prognosis (7). MM can arise in various mucosal sites, including the respiratory, gastrointestinal, and genitourinary tracts. In contrast to cutaneous melanoma, MM demonstrates notable differences in epidemiology, etiology, and pathogenesis. The incidence rate of MM remains relatively stable and tends to occur at a later age compared to cutaneous melanoma, typically between 50 and 80 years (8). Additionally, MM has a higher incidence rate in females, particularly in vulvar and vaginal manifestations (9). The exact etiology and pathogenesis of MM are still unknown, but genetic factors are believed to play a critical role in its development. Unlike cutaneous melanoma, MM is rarely associated with BRAF gene mutations, while mutations and amplifications of the KIT oncogene are more common (3, 10). These genetic differences underline the unique makeup of MM and contribute to its heightened aggressiveness and poorer prognoses (11).
In recent years, the development of immunotherapy has provided optimism for patients contending with drug-resistant, recurrent metastatic tumors, and those lacking actionable driver genes (12, 13). Immunotherapy functions by stimulating and enhancing the inherent immune response of the body to combat the growth and spread of tumor cells (14, 15). Key immunotherapeutic strategies involve the deployment of immune cells, antibodies, and tumor vaccines to provoke an immune response in the body (16). In contrast to traditional treatment modalities, immunotherapy offers clear advantages. It has the ability to target the diversity of tumor cells, thereby overcoming challenges related to drug resistance and adverse effects (17). Moreover, the impact of immunotherapy can be enduring, establishing immune memory. Once the immune system has developed memory against tumor cells, it continuously monitors any remaining or recurring tumor cells (18). In recent times, the rise of immunotherapy using Immune Checkpoint Inhibitors (ICIs) has emerged as a promising approach to enhance the prognosis of individuals suffering from MM. This article aims to synthesize the current landscape and potential directions of immunotherapeutic strategies for MM, elucidating the mechanisms and roles of ICIs in the context of MM. Our primary goal is to offer new insights and pathways for the therapeutic care of patients with MM.
2 Pathogenesis and clinical characteristics of MM
2.1 The etiopathogenesis of MM
The pathogenesis of melanoma is a multifaceted process that involves the intricate interplay of various pathways, including genetics, environment, inflammation, immunity, and cellular signaling (Figure 1). Further exploration is necessary to fully elucidate the specific mechanisms underlying the disease. Although melanoma is primarily associated with skin exposed to ultraviolet radiation, it can also originate in different extracutaneous tissues where melanocytes are present, such as mucous membranes, ocular structures, and certain internal organs (19, 20). Racial disparities have been observed in the presentation of different subtypes of melanoma. Importantly, immunotherapy has demonstrated a favorable safety profile, harnessing the body’s innate defense mechanisms and resulting in relatively few severe adverse reactions (21). One notable breakthrough in the field of immunotherapy is the development of immune checkpoint inhibitors (ICIs). Functionally, ICIs counteract the immune suppression imposed by tumor cells, thereby restoring the immune competence of the body to combat the tumor (22). These therapies primarily target immune checkpoint proteins located on the surface of tumor cells, such as Programmed Death-1 (PD-1) and Cytotoxic T lymphocyte-associated protein 4 (CTLA-4). By binding to their respective ligands, ICIs intercept T cell activation signals, allowing tumor cells to evade immune surveillance (23, 24). Current investigations highlight the significant efficacy of ICIs in melanoma, non-small cell lung cancer, and bladder cancer (25–30). However, in other types of tumors, their effectiveness may be relatively limited or require combination with adjunct treatment modalities to achieve optimal outcomes. The unique physiological characteristics of these sites contribute to the inconspicuous onset and rapid deep-tissue and lymphatic spread of MM (31). Although mutations in KIT Proto-Oncogene, Receptor Tyrosine Kinase (KIT), Neuroblastoma RAS Viral Oncogene Homolog (NRAS), B-Raf Proto-Oncogene, Serine/Threonine Kinase (BRAF), neurofibromatosis type-1 (NF1), and Splicing Factor 3b, Subunit 1 (SF3B1) have been associated with MM development, their exact mechanisms remain incompletely understood. The current management strategies for MM primarily involve surgical intervention, chemotherapy, and radiotherapy (32). However, outcomes for advanced MM are dismal, with a less than 25% 5-year survival rate. Hence, there is an urgent need to elucidate the underlying pathogenesis and explore novel therapeutic approaches for MM.
The major transcriptional regulators and signaling pathways associated with melanoma pathogenesis, including the RAS-RAF-MEK-ERK cascade and the PI3K-AKT pathway, which are commonly activated in this cancer type either through mutations or other genetic alterations. The growth factors triggering receptor tyrosine kinases (RTKs) and the c-KIT receptor initiate the activation of the MAPK/ERK signaling cascade with NRAS and BRAF mutants. In the center, the diagram showcases the AKT signaling pathway connected to cell survival and metabolism, often dysregulated in melanoma through mutations such as PTEN deletion. G protein-coupled receptor (GPCR) signaling pathway has been implicated in melanoma through mutations in GNAQ or GNA11. Cell-cycle control is another major aspect of melanoma regulation, the cyclin-dependent kinase inhibitor CDKN2A encodes P16 and P14ARF proteins, both of which can be mutated, deleted, or silenced in melanoma.
2.2 MM in different systems
2.2.1 Respiratory system
Respiratory mucosal melanoma (RTMM) is a rare neoplasm arising from melanocytes within the respiratory tract mucosa and can localize in various areas, including the nasal cavity, sinuses, oropharynx, larynx, trachea, and bronchi (33). Unlike cutaneous melanoma, RTMM displays distinctive etiological factors and clinical features (34). Genetic predisposition, chronic inflammatory responses, and prolonged viral infections have been suggested as potential etiological contributors to the development of RTMM (35). Furthermore, prolonged tobacco use and occupational exposures may increase an individual’s susceptibility to RTMM (35). Clinically, RTMM often presents insidiously, lacking early overt symptoms and demonstrating marked invasiveness. Its deep-seated location and inconspicuous clinical presentation commonly impede early detection, often leading to diagnosis at advanced stages. Patients may exhibit symptoms similar to other respiratory conditions, such as dyspnea, cough, hemoptysis, and hoarseness, thereby elevating the risk of misdiagnosis or delayed diagnosis (36). The diagnosis of RTMM primarily relies on histological examination and immunohistochemical profiling to ascertain tumor characteristics and origin. Given its invasive nature and proclivity for recurrence, conventional treatment approaches such as surgical resection, radiotherapy, and chemotherapy have shown limited efficacy (37). In recent years, immunotherapy has emerged as a promising therapeutic avenue. The development of immunotherapeutic agents, particularly immune checkpoint inhibitors (ICIs), has provided a degree of prognostic improvement for patients grappling with RTMM.
2.2.2 Gastrointestinal system
Gastrointestinal mucosal melanoma is a rare and highly invasive malignancy that develops in the mucosal linings of the digestive tract, including the esophagus, stomach, small intestine, colon, and rectum (37, 38). Unlike cutaneous melanoma, the pathogenesis of gastrointestinal MM is believed to be complex, potentially involving genetic predispositions, chronic inflammation, viral infections, and dietary factors (39, 40). The disease often progresses discreetly, with minimal symptoms in its early stages, leading to advanced-stage diagnoses in many patients. Clinical presentations commonly include dyspepsia, abdominal discomfort, nausea, vomiting, and melena (41, 42), which can mimic symptoms of other gastrointestinal disorders, leading to misdiagnosis and delayed treatment. The diagnosis of gastrointestinal MM typically involves a tissue biopsy and immunohistochemical profiling to characterize the tumor and determine its origin, guiding treatment strategies and prognostic evaluations. Currently, conventional treatment modalities, such as surgery, radiotherapy, and chemotherapy, have limited effectiveness in managing gastrointestinal MM (43), highlighting the need for innovative approaches. In recent years, immunotherapy, particularly the use of immune checkpoint inhibitors (ICIs), has emerged as a promising therapeutic paradigm, significantly improving the antitumor efficacy of the host’s immune system and enhancing patient survival rates and prognoses.
2.2.3 Urogenital system
Urogenital tract mucosal melanoma is evident within the mucosal tissues of various anatomical sites, including the urethra, bladder, cervix, vagina, testes, and prostate (6). In contrast to cutaneous melanoma, urogenital tract MM is closely associated with chronic inflammatory processes, genetic predispositions, exposure to carcinogens, and hormonal influences (44). Clinically, urogenital tract MM displays similarities with other melanoma subtypes, characterized by subtle progression, limited early symptoms, and marked invasiveness. Symptoms typically arise only in advanced stages of the disease, such as hematuria, urinary frequency, dysuria, and vaginal bleeding (45). These symptoms resemble those observed in various urogenital disorders, resulting in diagnostic challenges and delays in treatment. The diagnosis of urogenital tract MM primarily relies on histopathological evaluation and immunohistochemical profiling to determine tumor characteristics and origin (46, 47). Traditional treatment modalities, including surgical resection, radiotherapy, and chemotherapy, demonstrate limited effectiveness in managing urogenital tract MM (36). However, in recent years, immunotherapy has emerged as a promising therapeutic approach in this area. The introduction of immune checkpoint inhibitors (ICIs) and other immunomodulatory agents offers the potential to improve patient outcomes and survival rates to a certain extent.
2.3 The genetic landscape of MM
One of the prominent mutational drivers in melanoma is the BRAF gene, specifically the BRAF V600 mutation. This mutation is prevalent in melanoma, accounting for nearly half of all cases (48). The BRAF V600 mutation leads to sustained activation of BRAF kinase activity, resulting in abnormal stimulation of the Rapidly Accelerated Fibrosarcoma (RAF)/Mitogen-Activated Protein Kinase/Extracellular Signal-Regulated Kinase Kinase (MEK)/Extracellular Signal-Regulated Kinase (ERK) signaling cascade (49). Another significant mutational driver is NRAS, which is notably found in melanoma cases without BRAF mutations (48, 50). NRAS mutations activate proteins, initiating the Mitogen-Activated Protein Kinase (MAPK) signaling pathway and promoting cellular proliferation and survival (51). In mucosal malignant melanoma (MMM), Riobello et al. identified inactivation mutations and intragenic deletions of the NF1 gene, as well as activating mutations in the NRAS and KRAS genes. Cases with NF1 gene alterations showed reduced overall survival (OS) rates, indicating the potential prognostic and therapeutic relevance of these genetic alterations (52). Additionally, mutations in other genes such as Tumor Protein p53 (TP53), Cyclin-Dependent Kinase Inhibitor 2A (CDKN2A), KIT, and Phosphatase and Tensin Homolog (PTEN) are implicated in the etiology and progression of MM (3, 53–55). MM is characterized by reduced levels of T-cell infiltration and increased PD-L1 expression, indicating immune regulation dysfunction (56, 57). Genetic alterations in MM, including protein inactivation, mutagenesis, and proliferation-related mutations, play complex roles throughout the pathogenesis and evolution of the disease (58, 59). Given the intricacies of these genetic alterations, further comprehensive research is necessary to unravel their nuanced roles and therapeutic implications in MM.
2.4 Surgical and systemic treatment of MM
Due to its unique biological characteristics and clinical presentation, the management of Mucosal melanoma (MM) has consistently presented a significant challenge. Among the available treatment options, surgical resection is a widely used approach, particularly in cases of early-stage MM or individuals with a solitary resectable lesion (60). In localized MM cases, surgical intervention aims to remove the tumor along with the surrounding healthy tissue. This can be achieved through mucosal resection, mucosal resection with frozen section margin assessment, or margin clearance surgery, commonly performed in regions such as the oral and nasal cavities, genitalia, and digestive tract (61). For cases requiring more extensive excisions, surgical resection ensures the eradication of microscopic lesions around the tumor by removing the tumor along with a specified margin of adjacent healthy tissue (62). Heppt et al. conducted a study on MM in German patients, identifying the head and neck, female genital tract, and anorectal region as the primary sites, with anorectal MM having the worst prognosis (5). Additionally, NRAS, KIT, and BRAF gene mutations were found to be uniformly distributed. Male gender, advanced tumor stage, lymph node involvement, and incomplete resection were identified as risk factors for disease progression, with anorectal MM having the bleakest prognosis in European populations.
In cases where adjuvant radiotherapy is administered prior to surgical resection, surgery is usually performed to control local lesions or as a supplement to subsequent therapeutic interventions. Radiotherapy is commonly used as an adjuvant treatment in MM management, especially for postoperative lesion control or when surgery is not feasible. Although radiotherapy effectively targets cancer cells, it can cause damage to surrounding normal tissues. Chemotherapy is a systemic treatment option for advanced or metastatic MM, aimed at relieving symptoms and slowing tumor progression. In cases where surgery is not feasible, chemotherapy may be the primary therapeutic approach. Adjuvant chemotherapy can be administered before or after surgery to reduce tumor size, control lesions, decrease the risk of recurrence, or improve surgical success rates (63). Dacarbazine and vinblastine are commonly used chemotherapeutic agents in MM, acting by disrupting DNA synthesis or the division process of cancer cells. Combination chemotherapy, involving the simultaneous administration of multiple drugs like dacarbazine with vinblastine or oxaliplatin, is also utilized. Genetic profiling can identify individuals who may benefit from targeted therapy, which interferes with specific signaling pathways involved in cancer cell growth. Targeted therapy options include inhibitors of BRAF and MEK (64). Nevertheless, the high propensity of MM for recurrence and metastasis emphasizes the growing importance of systemic treatment approaches. Immunotherapy and targeted therapy have emerged as focal points of research efforts for individuals with locally advanced or metastatic MM. Immunotherapy uses drugs to enhance the patient’s immune response against MM (65). Flukes et al. conducted an analysis of patients with sinonasal MM (SNMM), highlighting surgical resection as the primary treatment modality, with a notable shift towards the adoption of endoscopic surgery over time. Radiotherapy was frequently utilized, and some patients showed benefit from immune checkpoint inhibitors (ICI) with partial or complete responses. The study emphasized the challenges and poor prognosis associated with SNMM, underscoring the importance of local disease control and immunotherapeutic interventions (66).
3 The tumor immune microenvironment of melanoma
The immune microenvironment of melanoma is a complex system that includes a variety of immune cells crucial for both tumor immune surveillance and suppression (Figure 2) (67, 68) (Figure 2). Among these cells, CD8+ T cells play a central role as effector cells responsible for directly eliminating melanoma cells (69). In contrast, CD4+ T cells exert regulatory control over immune responses (68), while natural killer (NK) cells function as vigilant sentinels, identifying and removing melanoma cells (70). Additionally, dendritic cells (DCs) act as pivotal coordinators, stimulating immune cell responses (71). Despite the collaborative actions of these immune effectors, melanoma employs immunosuppressive strategies mediated by tumor-associated macrophages (TAMs) that inhibit the function of other immune cells, demonstrating an immunosuppressive aspect within the tumor microenvironment (72). Moreover, regulatory T cells (Tregs), polymorphonuclear neutrophils (PMNs), and B cells play nuanced roles in the context of melanoma (25, 73). The interactions among these diverse immune cell populations are of utmost importance in the field of melanoma immunotherapy. Melanoma cells utilize sophisticated evasion mechanisms to evade immune surveillance by releasing immunosuppressive factors and diminishing antigen presentation capabilities. This evasion tactic hampers immune cell function, reducing their ability to recognize and eliminate tumor antigens, thereby facilitating tumor immune evasion.
Various cell types regulate and interact with the tumor microenvironment, including cytotoxic T lymphocytes (CTLs), dendritic cells (DCs), M2 type macrophages (M2), regulatory T cells (Tregs), exhausted T cells (Tex), myeloid-derived suppressor cells (MDSC), TH2 type helper T cells (TH2), T follicular helper cells (Tfh), and B cells. Each cell type is associated with different immune functions and interactions. For example, MDSCs interact with Tregs via the PD-L1 and PD-1 checkpoint pathway. Key molecules such as prostaglandin E2 (PGE2), vascular endothelial growth factor (VEGF), interleukin-10 (IL-10), and galectin-9 (GAL9) are indicated, showing their involvement in immune modulation. The tumor-associated tertiary lymphoid structures include positive immune regulators (CTLs and TH1 cells) and negative immune regulators (Tregs, MDSCs, and M2 macrophages).
ICIs have emerged as cornerstone therapeutics in the management of melanoma (74, 75). Among these, the most prominent are PD-1 and CTLA-4 inhibitors. PD-1 inhibitors function by impeding the interaction between the immune checkpoint molecule PD-1 and its ligand PD-L1, thereby thwarting the mechanism through which melanoma cells elude immune surveillance (76). Conversely, CTLA-4 inhibitors obstruct the CTLA-4 molecule on the surface of inhibitory tumor immune cells, bolstering the activity of immune effectors (77). The application of ICIs in melanoma therapy has yielded remarkable clinical efficacy. Comparative studies evince that, vis-à-vis traditional chemotherapy, ICIs not only prolong the survival duration of melanoma patients but also confer enduring therapeutic benefits whilst mitigating treatment-related adverse events. Nevertheless, ICIs prove efficacious in only a subset of patients, with some developing immune resistance. Consequently, ongoing research endeavors are earnestly pursuing novel ICIs or exploring combinatorial immunotherapeutic approaches to further augment treatment outcomes for melanoma patients.
4 Immunotherapy in MM
In recent years, substantial strides have been made in elucidating the immune mechanisms underpinning MM, catalyzing the development of innovative immunotherapeutic strategies. ICIs have emerged as promising agents capable of disrupting immune checkpoint interactions, thereby potently activating the host’s immune system and precipitating tumor cell destruction. In tandem with ICIs, modalities such as ACT, vascular endothelial growth factor (VEGF) inhibitors, and combination therapies have also demonstrated robust anti-tumor activities. The clinical trials investigating the efficacy of ICIs in MM are comprehensively outlined in Table 1. While The clinical trials investigating the efficacy of VEGF inhibitors in MM are comprehensively outlined in Table 2. These noteworthy advancements in immunotherapeutic approaches signify the advent of a new epoch in MM treatment.
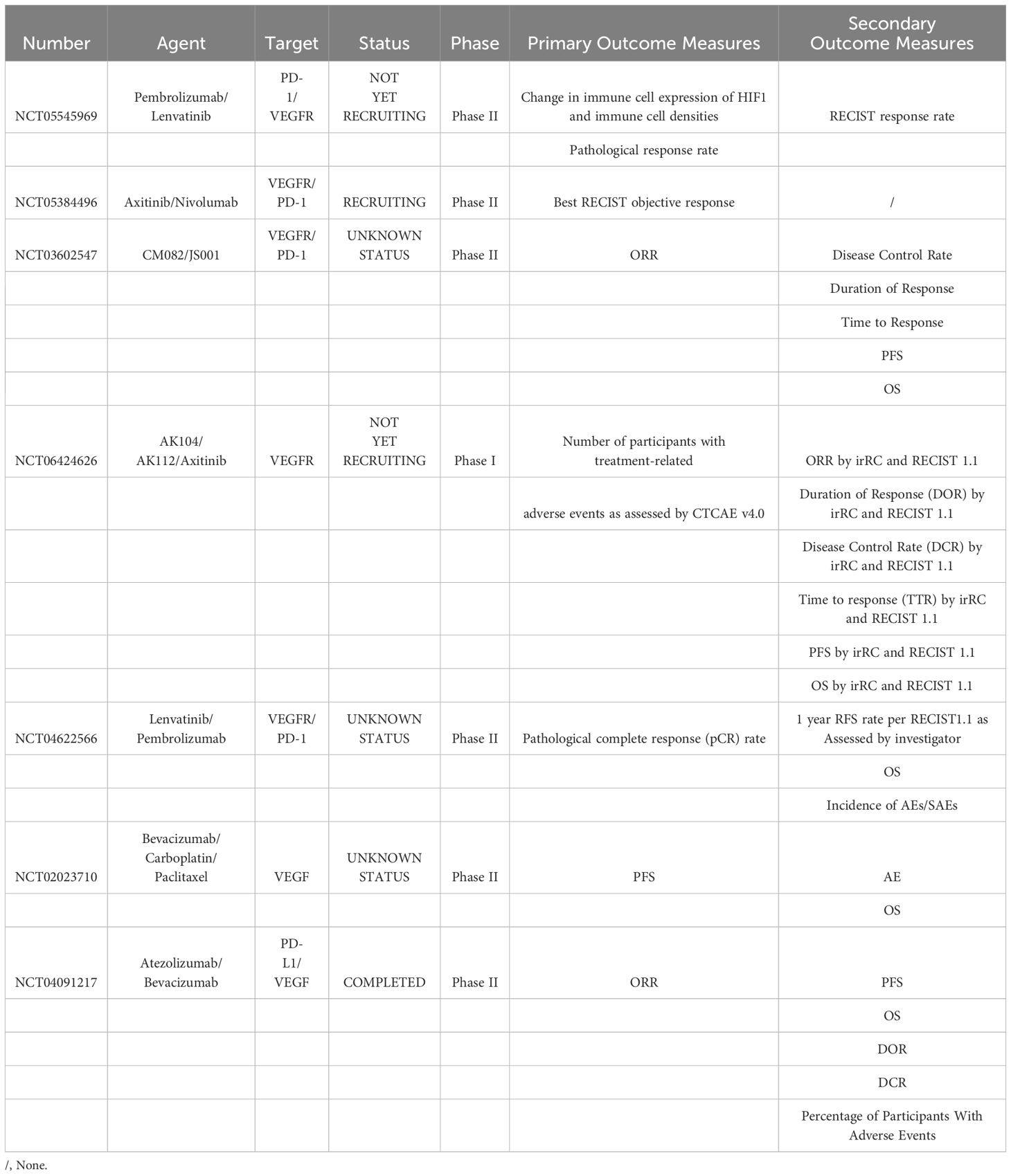
Table 2. Summary of immunotherapy clinical trials targeting VEGF/VEGFR of patients with mucosal melanoma.
4.1 ICIs
4.1.1 PD-1 inhibitors
PD-1 inhibitors comprise a pharmacological subclass of therapeutic agents designed to specifically target the immune checkpoint protein PD-1, thereby inhibiting the interaction between PD-1 and its ligands (Figure 3). This inhibition reinstates and amplifies the immune response of activated T cells against tumor cells (78, 79). Importantly, PD-1 inhibitors have exhibited considerable efficacy in patients with MM, as evidenced by a series of clinical trials validating their notable performance in MM therapy. These trials underscore the favorable and lasting responses achieved with PD-1 inhibitor monotherapy, providing survival benefits for individuals with advanced and metastatic melanoma. Promising outcomes include instances of complete remission or sustained periods free of disease progression. Thierauf et al. conducted an investigation into the effectiveness of anti-PD-1 therapy in patients with head and neck MM, evaluating the expression of Programmed Death-Ligand 1 (PD-L1) and PD-1 in tumor specimens. Despite the observed low levels of PD-L1 expression in these specimens, the study revealed that anti-PD-1 treatment did not elicit clinical responses in patients, highlighting the challenges and limitations associated with checkpoint inhibitors in the treatment of advanced MM (80). Similarly, Buchbinder et al. noted that MM displayed a relatively lower response rate (RR) to immune checkpoint blockade (ICB) compared to cutaneous melanoma. Targeted sequencing identified mutations in genes such as SF3B1, KIT, and NF1 in MM, but these genetic abnormalities did not significantly correlate with the response to ICB treatment (81). In a separate study, Ascierto et al. discerned that changes in lactate dehydrogenase (LDH) levels could serve as predictive markers of treatment response, with nivolumab demonstrating complete remission in certain patients. Despite the occurrence of manageable mild adverse events, the study emphasized the importance of identifying patients who are most likely to benefit from nivolumab monotherapy (82). Furthermore, Otsuka et al. revealed that patients experiencing immune-related adverse events (irAEs) during nivolumab therapy generally exhibited prolonged progression-free survival (PFS) and overall survival (OS), with the disease control rate (DCR) being significantly associated with the occurrence of irAEs. These findings suggest that irAEs could potentially serve as an effective biomarker for predicting treatment outcomes (83).
Indeed, while programmed cell death protein 1 (PD-1) inhibitors represent a promising therapeutic approach for the treatment of melanoma, not all patients experience optimal responses to these agents. Some individuals may demonstrate intrinsic resistance, while others may encounter adverse immune reactions. A case study by Sohail et al. focused on a female patient diagnosed with primary gastric mucosal melanoma (MMM) who exhibited an inadequate response to nivolumab, leading to a transition to palliative care. This case emphasized the infrequency and aggressive nature of primary gastric melanoma, highlighting the consideration of clinical trials for treatment-naive melanoma patients (84). Additionally, Zumelzu et al. reported a case of an 83-year-old patient who developed gingival mucosal erosions and blisters after discontinuing a 10-month pembrolizumab regimen for metastatic melanoma. Subsequent diagnosis revealed mild mucosal pemphigoid (MMP), which promptly achieved complete remission with minimal intervention (doxycycline). Noteworthy is the absence of melanoma recurrence 14 months post-pembrolizumab cessation, suggesting the possibility of unusual immune-related complications following PD-1 inhibitor therapy discontinuation. Furthermore, Nomura et al. conducted a study evaluating the efficacy and safety of nivolumab monotherapy for unresectable or metastatic melanoma, reporting an overall response rate (RR) of 23.5% (85). One patient achieved complete remission, three attained partial remission, and five experienced stable disease. The median progression-free survival (PFS) was 1.4 months, the median overall survival (OS) was 12.0 months, and the one-year OS rate was 50.0%. Notably, treatment-related grade 3 or 4 adverse events occurred in 15% of patients, managed effectively with corticosteroid therapy, highlighting the therapeutic potential of nivolumab despite associated adverse events (86).
4.1.2 CTLA-4 inhibitors
CTLA-4 inhibitors exert their effects primarily through targeted inhibition of the CTLA-4 protein present on the cell surface, an essential modulator of T cell function (87, 88) (Figure 3). These inhibitors function by preventing the interaction between CTLA-4 and its ligands (B7-1 and B7-2), thereby eliminating the inhibitory signals on T cells, leading to enhanced anti-tumor immune responses (77, 89, 90). Initial clinical investigations have consistently shown notable therapeutic efficacy of CTLA-4 inhibitors in patients with MM. Saijo et al. performed a comprehensive analysis involving patients with advanced MM who had previously failed nivolumab therapy and subsequently received ipilimumab treatment. While complete or partial responses were not observed in any patients, disease stabilization was evident, with some individuals experiencing a reduction in tumor size. These findings highlight the potential of ipilimumab as an adjunct therapy that can provide additional benefits in terms of progression-free survival for patients with advanced MM refractory to nivolumab (91).
Immune checkpoints are critical molecules in the regulation of immune response cells, and tumor cells exploit these molecules to evade immune detection. The PD-1/PD-L1 and CTLA-4 pathways are significant immune checkpoint pathways with distinct mechanisms in cancer therapy. PD-1 primarily regulates immune responses by inhibiting T cell activity, whereas CTLA-4 predominantly regulates the initial activation of T cells in lymphoid tissue. CTLA-4 inhibits T cell activation and proliferation by competitively binding to B7 molecules, thereby reducing the binding of CD28 to B7. PD-1 inhibits T cell activation and function by binding to its ligand PD-L1, thus preventing excessive immune responses. Targeted therapies such as PD-1 inhibitors and CTLA-4 inhibitors can block these checkpoints, restore the anti-tumor activity of T cells, and enhance the immune system’s capacity to recognize and attack tumors.
Despite the demonstrated efficacy of CTLA-4 inhibitors in the treatment of melanoma, there are notable limitations and associated side effects to consider. Immune activation-related toxicities, such as immune-mediated side effects, can lead to severe adverse reactions in specific patient populations. Postow et al. conducted a retrospective analysis on patients with metastatic melanoma treated with ipilimumab, revealing immune-related responses that included complete response, partial response, stable disease, and progressive disease, albeit with an overall low response rate (RR) (92). Vecchio et al. assessed the efficacy of ipilimumab in metastatic melanoma patients, highlighting some response efficacy in addition to the ability to manage immune-related adverse events. Notably, treatment with ipilimumab resulted in improvements in progression-free survival (PFS) and overall survival (OS), though it was accompanied by a high incidence of treatment-related severe adverse events (93). Alexander et al. observed that melanoma patients treated with ipilimumab exhibited modest improvements in OS and PFS, with favorable one- and two-year survival rates. Factors such as melanoma subtype, lymphocyte count, and BRAF mutation status were identified as key influencers of treatment efficacy. However, despite comparable efficacy to clinical trials, the incidence of severe adverse events remained elevated (94). Additionally, Bello et al. reported that metastatic uveal melanoma patients undergoing ipilimumab treatment experienced symptoms resembling gastritis, initially mistaken for immune-related adverse events but indicative of an effective antitumor immune response against micro-metastases in the stomach. The study underscored the significance of a multidisciplinary approach in the management of patients receiving immunotherapy, particularly in cases involving atypical symptoms or rare etiologies (95).
4.2 ACT
Adoptive cell therapy (ACT) has emerged as a promising therapeutic approach in the field of melanoma treatment, attracting considerable attention (96). This innovative strategy involves the utilization of recombinant T cell receptors (TCR) or chimeric antigen receptors (CAR) to genetically modify a patient’s own T cells, equipping them with the capability to recognize and eliminate melanoma cells (97). Noteworthy is the personalized nature of this therapeutic modality, allowing for customization to individual patient profiles, thus enhancing treatment precision and effectiveness. Within the realm of melanoma, ACT has shown significant promise and considerable antitumor activity. Clinical trials utilizing engineered T cells targeting specific antigens, such as tumor-associated antigens (TAA) or tumor-specific antigens (TSA), have produced remarkable therapeutic results. Particularly in cases of metastatic or treatment-resistant melanoma, ACT has demonstrated encouraging long-term remissions and even complete clinical responses (98). Zhang et al. conducted an evaluation of the effectiveness and success rate of cytokine injection, cryosurgery, and adoptive cell transfer in managing patients with advanced oral MM. Their findings revealed objective clinical efficacy in all patients, including seven instances of sustained complete remission and three cases of partial remission, with five patients still alive. Notably, combined treatment led to an increase in the proportion of CD3+ lymphocytes and interferon-γ secretion, coupled with a decrease in interleukin-10 levels. Moreover, improved cytokine-induced killer cell assays showcased a decline in CD4+CD25+ Tregs alongside an upregulation of NK cells. Additionally, the proliferation rate of modified cytokine-induced killer cells cultured in vitro exhibited enhancement post-treatment (99).
4.3 Anti-angiogenic therapy
Anti-angiogenic therapy plays a crucial role in pharmacological intervention to impede the growth and metastasis of tumors by inhibiting angiogenesis. Within the context of melanoma, this therapeutic approach has shown promise and effectiveness. Research highlights the enhanced angiogenic capacity of MM compared to other types of melanoma, providing a basis for exploiting anti-angiogenic therapy (100). This therapeutic modality targets key signaling pathways that are essential for tumor angiogenesis, specifically the vascular endothelial growth factor-vascular endothelial growth factor receptor (VEGF-VEGFR) pathway, in order to disrupt the formation of tumor blood vessels. Consequently, anti-angiogenic therapy reduces tumor growth and blood supply, thus inhibiting tumor proliferation and spread (101, 102). Clinical trials have investigated the application of anti-angiogenic therapy in MM, assessing the efficacy of anti-angiogenic drugs as standalone interventions and in combination with other modalities such as immunotherapy (103). While ongoing research is still in the early stages, evidence suggests the effectiveness of anti-angiogenic therapy in MM. However, this therapeutic approach is accompanied by challenges and side effects, including hypertension, bleeding risk, and potential development of resistance. Moreover, the significant heterogeneity of MM may result in diverse responses to anti-angiogenic therapy among different cases. Therefore, further comprehensive research and clinical trials are necessary to fully understand the potential of anti-angiogenic therapy in MM and determine the optimal treatment strategy.
4.4 Combination therapies
Emerging evidence indicates that the use of PD-1 monotherapy in patients with MM frequently confronts a substantial obstacle characterized by primary resistance. In order to tackle this dilemma, emphasis has shifted towards combination therapies that employ PD-1 inhibitors as a foundational element. By integrating supplementary agents that target distinct pathways, such as other checkpoint inhibitors and targeted therapies, there exists the potential to surmount primary resistance and enhance treatment outcomes.
4.4.1 Anti−PD−1 combined with other ICIs
Shustef et al. observed a regression of pigmented lesions in the gastric mucosa following ICB therapy, indicating the potential of ICIs such as ipilimumab and pembrolizumab in the treatment of advanced melanoma. It is worth noting that primary gastric melanoma is a rare condition with generally poor prognosis. Nevertheless, these treatments have been shown to enhance anti-tumor activity (104). In a study conducted by Plana et al. (105), the response of MM patients to anti-CTLA4 and anti-PD1 immunotherapy was evaluated. The findings revealed that the objective response rate (RR) and progression-free survival (PFS) were comparable to those observed in cutaneous melanoma patients. Notably, pembrolizumab demonstrated a more favorable risk-benefit ratio. Umeda et al. (106) treated multiple patients with advanced MM using anti-PD-1 monotherapy or a combination of anti-PD-1 and anti-CTLA-4 therapy. The addition of radiotherapy did not significantly impact the objective RR, PFS, or overall survival (OS). It was concluded that while radiotherapy can improve local tumor control and alleviate symptoms, it does not extend the survival of patients with advanced MM. A case study by Sezen et al. (107) reported a patient with metastatic vaginal MM who achieved complete remission through dual checkpoint inhibitor immunotherapy combined with high-dose and low-dose radiotherapy. The treatment regimen included ipilimumab and nivolumab. The pathological features showed characteristics of mucosal melanoma (MM), and staging and genetic alterations were taken into consideration. Lymphocyte-activation gene 3 (LAG-3) is an immune checkpoint protein that inhibits T cell activity and is upregulated in various tumor types, including melanoma. Preclinical models have demonstrated synergistic effects when combining anti-LAG3 and anti-PD-1 treatments (108). In the recently presented RELATIVITY-047 phase II/III study (109), the combination of the LAG-3 inhibitor relatlimab with nivolumab exhibited promising efficacy, particularly in patients with cutaneous melanoma. Other potential combinations that show promise include targeting T cell immunoglobulin and mucin-domain-containing-3 (TIM-3) and glucocorticoid-induced tumor necrosis factor receptor (GITR).
4.4.2 Anti−PD−1/PD−L1 combined with targeted therapy agents
A phase Ib study examining the combination of toripalimab and axitinib in patients with metastatic melanoma demonstrated notable responses (110). Among a cohort of 29 treatment-naïve individuals, the overall response rate (ORR) was 48.3%, and the disease control rate (DCR) was 86.2%. The median duration of response (DOR) was 13.7 months, with a median progression-free survival (PFS) of 7.5 months and overall survival (OS) of 20.7 months. Of interest, there was no significant association between programmed death-ligand 1 (PD-L1) expression or tumor mutational burden (TMB) and treatment response. However, a strong correlation was observed between gene expression programming (GEP) scores of certain immune-related and angiogenesis-related genes and treatment response. Building on these findings, a randomized three-arm phase II trial has been initiated to compare the toripalimab/axitinib combination with toripalimab or axitinib monotherapy. In another phase II study, the combination of vorolanib with toripalimab yielded an ORR of 22.2%, a DCR of 55.5%, and a median PFS of 5.7 months (111). Additionally, encouraging results were observed with the combination of lenvatinib and pembrolizumab in melanoma, renal cell carcinoma, and endometrial cancer. These findings have prompted the initiation of an ongoing international multicenter phase III trial for the treatment of metastatic melanoma (112). Moreover, the combination of atezolizumab, an anti-PD-L1 inhibitor, with the vascular endothelial growth factor receptor (VEGFR) monoclonal antibody bevacizumab demonstrated a favorable ORR of 36.4% and a tolerable safety profile in patients with advanced melanoma (113). Heppt et al. presented a case report of a male patient with nivolumab-refractory unresectable melanoma who received intralesional injections of interleukin-2 (IL-2) (114). After six months, a notable reduction in tumor size was observed. This study underscores the potential efficacy of intralesional IL-2 therapy and highlights the underutilized opportunities for intratumoral treatment modalities.
4.4.3 Anti−PD−1/PD−L1 combined with other candidates
Adileh et al. analyzed the effects of ICI therapy in patients undergoing surgical resection of anorectal melanoma. The results indicated that ICI therapy did not significantly enhance patient survival, and no clinical or pathological features were identified that correlated with ICI treatment response or survival outcomes. The study suggests that ICI therapy alone may not improve survival rates in anorectal melanoma patients, highlighting the need for further research into combination therapies (115). KIMURA et al. found that for female patients treated with nivolumab and undergoing debulking surgery, the combination of debulking surgery with ICI could offer potential benefits. Specifically, for patients with large tumors at the start of treatment or those whose tumors grew after ICI therapy, this combined approach could enhance response and prolong the duration of the response. Additionally, debulking surgery might enhance the efficacy of ICI by reducing tumor burden and inducing abscopal effects (116). Beyond the aforementioned combinations, other agents are being investigated for their potential to augment the efficacy of anti-PD-1 antibodies in melanoma. These include epigenetic regulators such as EZH2, DNA methyltransferase (DNMT), and histone deacetylase (HDAC), as well as oncolytic virotherapies and toll-like receptor 9 (TLR9) agonists. Additionally, engineered cytokines capable of modulating the tumor microenvironment (TME) have shown promising antitumor activity both as monotherapies and in combination with pembrolizumab. These innovative approaches hold significant potential for improving treatment outcomes in melanoma patients (117). Tang et al. found that the combination of axitinib and anti-PD-1 antibody therapy was effective for treating MM. The efficacy in patients with liver metastases treated with transcatheter arterial chemoembolization (TACE) varied and was associated with LDH levels and Eastern Cooperative Oncology Group (ECOG) status. The study results suggest that combination therapy is suitable for advanced MM, especially as a first-line treatment, and that TACE might improve treatment outcomes (118).
5 Discussion and prospects
Melanoma represents a rare and highly aggressive tumor, presenting significant challenges in both therapeutic approaches and research endeavors. Nevertheless, the continuous progress in scientific and technological realms, accompanied by a deepened comprehension of this condition, are facilitating advancements towards enhanced MM management and treatment strategies. The combination of surgical interventions alongside systemic therapies has demonstrated efficacy in facilitating the control of disease progression and metastatic dissemination. Neoadjuvant therapy, which entails the administration of therapeutic agents prior to the primary tumor-directed treatment, offers various benefits. Principally, this approach can lead to tumor size reduction, enabling less extensive surgical resections and the potential preservation of vital anatomical structures. Moreover, this preoperative maneuver permits early evaluation of tumor responsiveness to the therapeutic regimen, thereby informing subsequent treatment modalities. Additionally, neoadjuvant therapy holds the potential to eradicate micrometastatic foci, thereby enhancing overall survival outcomes. Nonetheless, noteworthy drawbacks exist. The deferment of definitive surgery could prove deleterious if the tumor fails to exhibit favorable responses to the prescribed therapy, consequently allowing for disease progression. Furthermore, adverse effects stemming from the therapeutic agents may compromise the patient’s general well-being, consequently complicating subsequent surgical procedures. Moreover, the optimal timing and combination of neoadjuvant therapies remain subjects of ongoing investigation across various cancer types, necessitating a delicate balance between prospective benefits and associated risks. These considerations underscore the imperative for individualized treatment strategies and the necessity for continued research efforts. The benefits and drawbacks of neoadjuvant therapy are illustrated in Figure 4.
The potential impacts of neoadjuvant therapy include the following: It may elicit a more extensive and potent immune response due to a broader array of tumor antigens. Increased intratumoral immune infiltration is associated with a pathological response and extended event-free survival (EFS), as immune cells migrate to potential micrometastases elsewhere in the body. Reducing tumor size may facilitate easier surgical removal. Additionally, assessing the pathological response post-treatment provides a rapid indication of the treatment’s effectiveness. However, surgery might be complicated by immune checkpoint blockade (ICB) side effects or delayed due to side effects or their management. Furthermore, disease progression can complicate surgery or render the tumor inoperable.
In recent years, significant progress has been made in the field of immunotherapy for the treatment of melanoma (119, 120). Immune checkpoint inhibitors (ICIs), such as PD-1 inhibitors and CTLA-4 inhibitors, have emerged as promising therapeutic agents by enhancing the host’s immune response against MM (121, 122). Clinical studies have demonstrated positive responses and favorable disease control with the administration of PD-1 and CTLA-4 inhibitors, leading to prolonged survival in MM patients. Additionally, cellular immunotherapy, involving the modification of T cells to specifically target and eliminate melanoma cells, has shown potential as an immunotherapeutic strategy. Although these approaches are currently at experimental stages, initial results suggest encouraging therapeutic possibilities. Furthermore, the combination of immunotherapy with conventional drug therapies, such as targeted therapy, has been investigated. The co-administration of immunotherapeutic drugs like ICIs with BRAF inhibitors or MEK inhibitors has shown substantial improvement in treatment response rates and overall survival of MM patients (123). In conclusion, significant advancements in the field of immunotherapy have been witnessed in the management of Mucosal melanoma (MM). Though the current stage of research represents the early phases, the accruing results provide a beacon of hope for patients, thus emphasizing the imperative nature of further exploration and refinement of treatment modalities for enhanced efficacy. Notwithstanding the strides made in MM treatment and investigative endeavors, a spectrum of challenges and complexities persists. These obstacles encompass the issue of resistance to immunotherapeutic interventions and the pressing necessity to ameliorate the prognosis and overall survival rates among individuals diagnosed with MM. While immunotherapy exhibits a degree of efficacy, its universal applicability is not absolute, with instances of patients developing resistance or tolerance to Immune Checkpoint Inhibitors (ICIs) and other immune-based pharmaceuticals. Hence, the pivotal direction for research lies in the realms of predicting patient responsiveness to immunotherapy, coupled with a profound comprehension of the underlying biochemical pathways involved. Furthermore, the combination of immunotherapeutic agents, cellular therapies, and radiotherapy presents a promising avenue for future investigation to bolster patient response to treatment. Additionally, the enhancement of survival outcomes and prognostic indicators for MM patients stands as a formidable challenge. Despite notable successes in immunotherapeutic advancements, a sizeable cohort of MM patients grapple with the looming risks of disease recurrence and metastasis, hence resulting in unfavorable prognoses. Thus, delving into the intricate molecular frameworks and the disease progression dynamics of MM assumes critical importance. This knowledge reservoir will be instrumental in sculpting tailor-made and precise therapeutic approaches, thereby fostering improved clinical outcomes for patients.
In conclusion, the treatment and research of MM still face significant challenges. However, with the advancement of science and technology, as well as deeper research, the understanding of MM and the available treatment methods will continue to improve. Our collective efforts aim to achieve more accurate early diagnosis, develop more effective treatment strategies, and improve prognoses.
Author contributions
ZS: Writing – original draft. FL: Writing – original draft, Writing – review & editing.
Funding
The author(s) declare that no financial support was received for the research, authorship, and/or publication of this article.
Acknowledgments
We thank the generous support by Liaoning Cancer Hospital & Institute (Shenyang) and Dalian University of Technology (Dalian).
Conflict of interest
The authors declare that the research was conducted in the absence of any commercial or financial relationships that could be construed as a potential conflict of interest.
Publisher’s note
All claims expressed in this article are solely those of the authors and do not necessarily represent those of their affiliated organizations, or those of the publisher, the editors and the reviewers. Any product that may be evaluated in this article, or claim that may be made by its manufacturer, is not guaranteed or endorsed by the publisher.
Glossary
MM: Mucosal melanoma
ICIs: Immune checkpoint inhibitors
MMM: mucosal malignant melanoma
SNMM: sinonasal MM
NK: natural killer
DCs: Dendritic cells
TAMs: tumor-associated macrophages
Tregs: regulatory T cells
PMNs: Polymorphonuclear Neutrophils
PD-L1: Programmed Death-Ligand 1
ICB: immune checkpoint blockade
LDH: lactate dehydrogenase
irAEs: immune-related adverse events
MMP: mild mucosal pemphigoid
RR: response rate
PFS: progression-free survival
ACT: Adoptive cell therapy
TCR: T cell receptors
CAR: chimeric antigen receptors
TAA: tumor-associated antigens
TSA: tumor-specific antigens
CTLA-4: Cytotoxic T lymphocyte-associated protein 4
LAG-3: Lymphocyte-activation gene 3
TIM-3: mucin-domain-containing-3
GITR: glucocorticoid-induced tumor necrosis factor receptor
ORR: overall response rate
DCR: disease control rate
DOR: duration of response
OS: overall survival
TMB: tumor mutational burden
GEP: gene expression programming
IL-2: interleukin-2
DNMT: DNA methyltransferase
HDAC: histone deacetylase
TLR9: toll-like receptor 9
TME: tumor microenvironment
TACE: transcatheter arterial chemoembolization
ECOG: Eastern Cooperative Oncology Group
PD-1: Programmed Death-1
BRAF: B-Raf Proto-Oncogene, Serine/Threonine Kinase
KIT: KIT Proto-Oncogene, Receptor Tyrosine Kinase
NRAS: Neuroblastoma RAS Viral Oncogene Homolog
NF1: neurofibromatosis type-1
SF3B1: Splicing Factor 3b, Subunit 1
RAF: Rapidly Accelerated Fibrosarcoma
MEK: Mitogen-Activated Protein Kinase/Extracellular Signal-Regulated Kinase Kinase
ERK: Extracellular Signal-Regulated Kinase
MAPK: Mitogen-Activated Protein Kinase
TP53: Tumor Protein p53
CDKN2A: Cyclin-Dependent Kinase Inhibitor 2A
PTEN: Phosphatase and Tensin Homolog
VEGF: vascular endothelial growth factor
VEGFR: vascular endothelial growth factor receptor
References
1. Long GV, Swetter SM, Menzies AM, Gershenwald JE, Scolyer RA. Cutaneous melanoma. Lancet. (2023) 402:485–502. doi: 10.1016/S0140-6736(23)00821-8
2. Xu M, Li S. Nano-drug delivery system targeting tumor microenvironment: A prospective strategy for melanoma treatment. Cancer Lett. (2023) 574:216397. doi: 10.1016/j.canlet.2023.216397
3. Nassar KW, Tan AC. The mutational landscape of mucosal melanoma. Semin Cancer Biol. (2020) 61:139–48. doi: 10.1016/j.semcancer.2019.09.013
4. Li S, Kang Y, Zeng Y. Targeting tumor and bone microenvironment: Novel therapeutic opportunities for castration-resistant prostate cancer patients with bone metastasis. Biochim Biophys Acta Rev Cancer. (2024) 1879:189033. doi: 10.1016/j.bbcan.2023.189033
5. Ascierto PA, Accorona R, Botti G, Farina D, Fossati P, Gatta G, et al. Mucosal melanoma of the head and neck. Crit Rev Oncol Hematol. (2017) 112:136–52. doi: 10.1016/j.critrevonc.2017.01.019
6. Olapeju O, Shakesprere J, Boykin C, Bacaj P, Salkini M, Kolodney J. Primary Malignant melanoma of the genitourinary tract: case series of a rare form of primary mucosal melanoma. Melanoma Manag. (2024) 10:Mmt67. doi: 10.2217/mmt-2023-0009
7. Zhang Y, Fu X, Qi Y, Gao Q. A study of the clinical characteristics and prognosis of advanced mucosal and cutaneous melanoma in a Chinese population. Immunotherapy. (2019) 11:91–9. doi: 10.2217/imt-2018-0030
8. Li C, Wang Y, Ren X, Li Y. Chitinase-3 like-protein-1 (YKL-40) Promotes Anorectal Mucosal Melanoma Progression via the PI3K-AKT Signaling Pathway. Altern Ther Health Med. (2023).
9. Zhang J, Tian H, Mao L, Si L. Treatment of acral and mucosal melanoma: Current and emerging targeted therapies. Crit Rev Oncol Hematol. (2024) 193:104221. doi: 10.1016/j.critrevonc.2023.104221
10. Corti C, Conforti F, Pala L, Catania C, Cocorocchio E, Ferrucci PF, et al. Differential activity of avapritinib in patients with metastases from mucosal melanoma and thymic carcinoma harbouring KIT exon 17 mutations: Initial experience from a Compassionate Use Program in Italy. Eur J Cancer. (2022) 172:332–9. doi: 10.1016/j.ejca.2022.06.015
11. Clavero-Rovira L, Gómez-Tomás Á, Bassas-Freixas P, Bodet D, Ferrer B, Hernández-Losa J, et al. Mucosal melanoma clinical management and prognostic implications: A retrospective cohort study. Cancers (Basel). (2024) 16(1):227. doi: 10.3390/cancers16010227
12. Yang K, Halima A, Chan TA. Antigen presentation in cancer - mechanisms and clinical implications for immunotherapy. Nat Rev Clin Oncol. (2023) 20:604–23. doi: 10.1038/s41571-023-00789-4
13. Chen Z, Yue K Yang Z, Shen. Z Cheng C, Zhou X, Li S. Four ounces can move a thousand pounds: the enormous value of nanomaterials in tumor immunotherapy. Adv Healthc Mater. (2023) 12:e2300882. doi: 10.1002/adhm.202300882
14. Cao LL, Kagan JC. Targeting innate immune pathways for cancer immunotherapy. Immunity. (2023) 56:2206–17. doi: 10.1016/j.immuni.2023.07.018
15. Liu Y, Hu Y, Xue J, Li J, Yi J, Bu J, et al. Advances in immunotherapy for triple-negative breast cancer. Mol Cancer. (2023) 22:145. doi: 10.1186/s12943-023-01850-7
16. Yi M, Li T, Niu M, Mei Q, Zhao B, Chu Q, et al. Exploiting innate immunity for cancer immunotherapy. Mol Cancer. (2023) 22:187. doi: 10.1186/s12943-023-01885-w
17. Peri A, Salomon N, Wolf Y, Kreiter S, Diken M, Samuels Y. The landscape of T cell antigens for cancer immunotherapy. Nat Cancer. (2023) 4:937–54. doi: 10.1038/s43018-023-00588-x
18. Kang JH, Zappasodi R. Modulating Treg stability to improve cancer immunotherapy. Trends Cancer. (2023) 9:911–27. doi: 10.1016/j.trecan.2023.07.015
19. Loda A, Semeraro F, Parolini S, Ronca R, Rezzola S. Cancer stem-like cells in uveal melanoma: novel insights and therapeutic implications. Biochim Biophys Acta Rev Cancer. (2024) 1879:189104. doi: 10.1016/j.bbcan.2024.189104
20. Viegas J, Sarmento B. Bridging the gap between testing and clinics exploring alternative pre-clinical models in melanoma research. Adv Drug Delivery Rev. (2024) 208:115295. doi: 10.1016/j.addr.2024.115295
21. Fenis A, Demaria O, Gauthier L, Vivier E, Narni-Mancinelli E. New immune cell engagers for cancer immunotherapy. Nat Rev Immunol. (2024) 24(7):471–86. doi: 10.1038/s41577-023-00982-7
22. Topalian SL, Forde PM, Emens LA, Yarchoan M, Smith KN, Pardoll DM. Neoadjuvant immune checkpoint blockade: A window of opportunity to advance cancer immunotherapy. Cancer Cell. (2023) 41:1551–66. doi: 10.1016/j.ccell.2023.07.011
23. Rimassa L, Finn RS, Sangro B. Combination immunotherapy for hepatocellular carcinoma. J Hepatol. (2023) 79:506–15. doi: 10.1016/j.jhep.2023.03.003
24. Van Wagoner CM, Rivera-Escalera F, Jaimes-Delgadillo NC, Chu CC, Zent CS, Elliott MR. Antibody-mediated phagocytosis in cancer immunotherapy. Immunol Rev. (2023) 319:128–41. doi: 10.1111/imr.13265
25. Ozbay Kurt FG, Lasser S, Arkhypov I, Utikal J, Umansky V. Enhancing immunotherapy response in melanoma: myeloid-derived suppressor cells as a therapeutic target. J Clin Invest. (2023) 133(13):e170762. doi: 10.1172/JCI170762
26. Splendiani E, Besharat ZM, Covre A, Maio M, Di Giacomo AM, Ferretti E. Immunotherapy in melanoma: Can we predict response to treatment with circulating biomarkers? Pharmacol Ther. (2024) 256:108613. doi: 10.1016/j.pharmthera.2024.108613
27. John AO, Ramnath N. Neoadjuvant versus adjuvant systemic therapy for early-stage non-small cell lung cancer: the changing landscape due to immunotherapy. Oncologist. (2023) 28:752–64. doi: 10.1093/oncolo/oyad125
28. Stanley R, Flanagan S, Reilly DO, Kearney E, Naidoo J, Dowling CM. Immunotherapy through the lens of non-small cell lung cancer. Cancers (Basel). (2023) 15(11):2996. doi: 10.3390/cancers15112996
29. Singh A, Osbourne AS, Koshkin VS. Perioperative immunotherapy in muscle-invasive bladder cancer. Curr Treat Options Oncol. (2023) 24:1213–30. doi: 10.1007/s11864-023-01113-z
30. Larroquette M, Lefort F, Domblides C, Héraudet L, Robert G, Ravaud A, et al. How immunotherapy has redefined the treatment paradigm of metastatic or locally advanced muscle-invasive urothelial bladder carcinoma. Cancers (Basel). (2024) 16(9):1780. doi: 10.3390/cancers16091780
31. Ichimura N, Urata Y, Kobayashi T, Hibi H. Mutational landscape of oral mucosal melanoma based on comprehensive cancer genomic profiling tests in a Japanese cohort. Oral Oncol. (2024) 152:106807. doi: 10.1016/j.oraloncology.2024.106807
32. Mao L, Qi Z, Zhang L, Guo J, Si L. Immunotherapy in acral and mucosal melanoma: current status and future directions. Front Immunol. (2021) 12:680407. doi: 10.3389/fimmu.2021.680407
33. Uematsu M, Nakajima H, Hosono A, Kiyohara H, Hirota A, Takahashi N, et al. Safety of immune checkpoint inhibitors after proton beam therapy in head and neck mucosal melanoma: a case series. Melanoma Res. (2023) 33:547–52. doi: 10.1097/CMR.0000000000000924
34. Boer FL, Ho VKY, Louwman MWJ, Schrader AMR, Zuur CL, Blank CU, et al. Trends in incidence and survival of 1496 patients with mucosal melanoma in the Netherlands (1990-2019). Cancers (Basel). (2023) 15(5):1541. doi: 10.3390/cancers15051541
35. Jarrom D, Paleri V, Kerawala C, Roques T, Bhide S, Newman L, et al. Mucosal melanoma of the upper airways tract mucosal melanoma: A systematic review with meta-analyses of treatment. Head Neck. (2017) 39:819–25. doi: 10.1002/hed.v39.4
36. Kirchoff DD, Deutsch GB, Foshag LJ, Lee JH, Sim MS, Faries MB. Evolving therapeutic strategies in mucosal melanoma have not improved survival over five decades. Am Surg. (2016) 82:1–5. doi: 10.1177/000313481608200102
37. Mattei J, Trindade EN, Chedid MF. Mucosal melanoma: from molecular landscape to current treatment strategies. Melanoma Res. (2023) 33:447–53. doi: 10.1097/CMR.0000000000000916
38. Abu-Heija U, Darweesh M, Kusmic D, Young M. Primary gastric mucosal melanoma: A rare etiology of iron deficiency anemia. Cureus. (2023) 15:e38668. doi: 10.7759/cureus.38668
39. Farooq A, Rahman H, Bani Fawwaz BA, Hurairah A. Wandering mucosal melanoma presenting as occult gastrointestinal blood loss anemia. Cureus. (2022) 14:e25614. doi: 10.7759/cureus.25614
40. Zhu X, Li S. Nanomaterials in tumor immunotherapy: new strategies and challenges. Mol Cancer. (2023)22:94. doi: 10.1186/s12943-023-01797-9
41. Zhang X, Jin F, Jiang S, Cao J, Meng Y, Xu Y, et al. Rh-endostatin combined with chemotherapy in patients with advanced or recurrent mucosal melanoma: retrospective analysis of real-world data. Invest New Drugs. (2022) 40:453–60. doi: 10.1007/s10637-021-01172-9
42. Guo X, Gao C, Yang DH, Li S. Exosomal circular RNAs: A chief culprit in cancer chemotherapy resistance. Drug Resist Updates. (2023) 67:100937. doi: 10.1016/j.drup.2023.100937
43. Lian B, Li Z, Wu N, Li M, Chen X, Zheng H, et al. Phase II clinical trial of neoadjuvant anti-PD-1 (toripalimab) combined with axitinib in resectable mucosal melanoma. Ann Oncol. (2024) 35:211–20. doi: 10.1016/j.annonc.2023.10.793
44. Smith HG, Bagwan I, Board RE, Capper S, Coupland SE, Glen J, et al. Ano-uro-genital mucosal melanoma UK national guidelines. Eur J Cancer. (2020) 135:22–30. doi: 10.1016/j.ejca.2020.04.030
45. Szabó B, Szűcs M, Horváth A, Székely E, Pánczél G, Liszkay G, et al. [Mucosal melanoma primary and metastatic cases with urogenital localization in our department]. Orv Hetil. (2019) 160:378–85. doi: 10.1556/650.2019.31303
46. Ng YYR, Tan GHC, Quek RHH, Farid M, Soo KC, Teo MCC. Clinical patterns and management of primary mucosal melanoma: a single centre experience. ANZ J Surg. (2018) 88:1145–50. doi: 10.1111/ans.14373
47. Tacastacas JD, Bray J, Cohen YK, Arbesman J, Kim J, Koon HB, et al. Update on primary mucosal melanoma. J Am Acad Dermatol. (2014) 71:366–75. doi: 10.1016/j.jaad.2014.03.031
48. Dumaz N, Jouenne F, Delyon J, Mourah S, Bensussan A, Lebbé C, et al. Atypical BRAF and NRAS Mutations in Mucosal Melanoma. Cancers (Basel). (2019) 11(8):1133. doi: 10.3390/cancers11081133
49. Bai X, Mao LL, Chi ZH, Sheng XN, Cui CL, Kong Y, et al. BRAF inhibitors: efficacious and tolerable in BRAF-mutant acral and mucosal melanoma. Neoplasma. (2017) 64:626–32. doi: 10.4149/neo_2017_419
50. Zebary A, Jangard M, Omholt K, Ragnarsson-Olding B, Hansson J, KIT NRAS. and BRAF mutations in sinonasal mucosal melanoma: a study of 56 cases. Br J Cancer. (2013) 109:559–64. doi: 10.1038/bjc.2013.373
51. Thierauf JC, Kaluziak ST, Codd E, Dybel SN, Jobbagy S, Purohit R, et al. Prognostic biomarkers for survival in mucosal melanoma. Pigment Cell Melanoma Res. (2023) 36:378–87. doi: 10.1111/pcmr.13104
52. Riobello C, Casanueva Muruais R, Suarez-Fernandez L, Garcia-Marin R, Cabal VN, Blanco-Lorenzo V, et al. Intragenic NF1 deletions in sinonasal mucosal Malignant melanoma. Pigment Cell Melanoma Res. (2022) 35:88–96. doi: 10.1111/pcmr.13015
53. Kang Y, Li S. Nanomaterials: Breaking through the bottleneck of tumor immunotherapy. Int J Biol Macromol. (2023) 230:123159. doi: 10.1016/j.ijbiomac.2023.123159
54. Liu F, Li S. Non-coding RNAs in skin cancers:Biological roles and molecular mechanisms. Front Pharmacol. (2022) 13:934396. doi: 10.3389/fphar.2022.934396
55. Wang HY, Liu Y, Deng L, Jiang K, Yang XH, Wu XY, et al. Clinical significance of genetic profiling based on different anatomic sites in patients with mucosal melanoma who received or did not receive immune checkpoint inhibitors. Cancer Cell Int. (2023) 23:187. doi: 10.1186/s12935-023-03032-3
56. Forschner A, Hilke FJ, Bonzheim I, Gschwind A, Demidov G, Amaral T, et al. MDM2, MDM4 and EGFR amplifications and hyperprogression in metastatic acral and mucosal melanoma. Cancers (Basel). (2020) 12(3):540. doi: 10.3390/cancers12030540
57. Shui IM, Liu XQ, Zhao Q, Kim ST, Sun Y, Yearley JH, et al. Baseline and post-treatment biomarkers of resistance to anti-PD-1 therapy in acral and mucosal melanoma: an observational study. J Immunother Cancer. (2022) 10(7):e004879. doi: 10.1136/jitc-2022-004879
58. Du M, Wang Y, Gu D, Guo L. Identification of vital genes and pathways associated with mucosal melanoma in Chinese. Ann Diagn Pathol. (2021) 50:151648. doi: 10.1016/j.anndiagpath.2020.151648
59. Broit N, Johansson PA, Rodgers CB, Walpole ST, Newell F, Hayward NK, et al. Meta-analysis and systematic review of the genomics of mucosal melanoma. Mol Cancer Res. (2021) 19:991–1004. doi: 10.1158/1541-7786.MCR-20-0839
60. Vaccari S, Barisani A, Dika E, Fanti PA, DE Iaco P, Gurioli C, et al. Management and treatment of mucosal melanoma of the genital tract. G Ital Dermatol Venereol. (2017) 152:270–3. doi: 10.23736/S0392-0488.17.05573-0
61. Amit M, Na'ara S, Hanna EY. Contemporary treatment approaches to sinonasal mucosal melanoma. Curr Oncol Rep. (2018) 20:10. doi: 10.1007/s11912-018-0660-7
62. Heppt MV, Roesch A, Weide B, Gutzmer R, Meier F, Loquai C, et al. Prognostic factors and treatment outcomes in 444 patients with mucosal melanoma. Eur J Cancer. (2017) 81:36–44. doi: 10.1016/j.ejca.2017.05.014
63. Abiri A, Yasaka TM, Lehrich BM, Goshtasbi K, Papagiannopoulos P, Tajudeen BA, et al. Adjuvant therapy and prognosticators of survival in head and neck mucosal melanoma. Laryngoscope. (2022) 132:584–92. doi: 10.1002/lary.29807
64. Ma Y, Xia R, Ma X, Judson-Torres RL, Zeng H. Mucosal melanoma: pathological evolution, pathway dependency and targeted therapy. Front Oncol. (2021) 11:702287. doi: 10.3389/fonc.2021.702287
65. Ohshima S, Ueki Y, Yokoyama Y, Takahashi T, Shodo R, Yamazaki K, et al. Treatment outcomes of mucosal melanoma of head and neck: Efficacy of immune checkpoint inhibitors for advanced disease. Front Surg. (2022) 9:1032626. doi: 10.3389/fsurg.2022.1032626
66. Flukes S, Lohia S, Barker CA, Cracchiolo JR, Ganly I, Patel SG, et al. Are our patients doing better? A single institution experience of an evolving management paradigm for sinonasal mucosal melanoma. Oral Oncol. (2021) 112:105006. doi: 10.1016/j.oraloncology.2020.105006
67. Carvalho LAD, Aguiar FC, Smalley KSM, Possik PA. Acral melanoma: new insights into the immune and genomic landscape. Neoplasia. (2023) 46:100947. doi: 10.1016/j.neo.2023.100947
68. Shirley CA, Chhabra G, Amiri D, Chang H, Ahmad N. Immune escape and metastasis mechanisms in melanoma: breaking down the dichotomy. Front Immunol. (2024) 15:1336023. doi: 10.3389/fimmu.2024.1336023
69. Klobuch S, Seijkens TTP, Schumacher TN, Haanen J. Tumour-infiltrating lymphocyte therapy for patients with advanced-stage melanoma. Nat Rev Clin Oncol. (2024) 21:173–84. doi: 10.1038/s41571-023-00848-w
70. Rahimi A, Malakoutikhah Z, Rahimmanesh I, Ferns GA, Nedaeinia R, Ishaghi SMM, et al. The nexus of natural killer cells and melanoma tumor microenvironment: crosstalk, chemotherapeutic potential, and innovative NK cell-based therapeutic strategies. Cancer Cell Int. (2023) 23:312. doi: 10.1186/s12935-023-03134-y
71. Gerlini G, Susini P, Sestini S, Brandani P, Giannotti V, Borgognoni L. Langerhans cells in sentinel lymph nodes from melanoma patients. Cancers (Basel). (2024) 16(10):1890. doi: 10.3390/cancers16101890
72. Kharouf N, Flanagan TW, Hassan SY, Shalaby H, Khabaz M, Hassan SL, et al. Tumor microenvironment as a therapeutic target in melanoma treatment. Cancers (Basel). (2023) 15(12):3147. doi: 10.3390/cancers15123147
73. Los C, Klobuch S, Haanen J. Tumor-infiltrating lymphocyte and other cell therapies for metastatic melanoma. Cancer J. (2024) 30:113–9. doi: 10.1097/PPO.0000000000000705
74. Haist M, Stege H, Kuske M, Bauer J, Klumpp A, Grabbe S, et al. Combination of immune-checkpoint inhibitors and targeted therapies for melanoma therapy: The more, the better? Cancer Metastasis Rev. (2023) 42:481–505. doi: 10.1007/s10555-023-10097-z
75. Tang W, Chen J, Ji T, Cong X. TIGIT, a novel immune checkpoint therapy for melanoma. Cell Death Dis. (2023) 14:466. doi: 10.1038/s41419-023-05961-3
76. Hassel JC, Zimmer L, Sickmann T, Eigentler TK, Meier F, Mohr P, et al. Medical needs and therapeutic options for melanoma patients resistant to anti-PD-1-directed immune checkpoint inhibition. Cancers (Basel). (2023) 15(13):3448. doi: 10.3390/cancers15133448
77. Kreidieh FY, Tawbi HA. The introduction of LAG-3 checkpoint blockade in melanoma: immunotherapy landscape beyond PD-1 and CTLA-4 inhibition. Ther Adv Med Oncol. (2023) 15:17588359231186027. doi: 10.1177/17588359231186027
78. Parvez A, Choudhary F, Mudgal P, Khan R, Qureshi KA, Farooqi H, et al. PD-1 and PD-L1: architects of immune symphony and immunotherapy breakthroughs in cancer treatment. Front Immunol. (2023) 14:1296341. doi: 10.3389/fimmu.2023.1296341
79. Gao M, Shi J, Xiao X, Yao Y, Chen X, Wang B, et al. PD-1 regulation in immune homeostasis and immunotherapy. Cancer Lett. (2024) 588:216726. doi: 10.1016/j.canlet.2024.216726
80. Thierauf J, Veit JA, Hess J, Treiber N, Lisson C, Weissinger SE, et al. Checkpoint inhibition for advanced mucosal melanoma. Eur J Dermatol. (2017) 27:160–5. doi: 10.1684/ejd.2016.2949
81. Buchbinder EI, Weirather JL, Manos M, Quattrochi BJ, Sholl LM, Brennick RC, et al. Characterization of genetics in patients with mucosal melanoma treated with immune checkpoint blockade. Cancer Med. (2021) 10:2627–35. doi: 10.1002/cam4.3789
82. Ascierto PA, Vanella V, Grimaldi AM, Lucia F, Palla M, Simeone E, et al. Complete response to nivolumab monotherapy in a treatment-naive, BRAF wild-type patient with advanced mucosal melanoma and elevated lactate dehydrogenase: a case report from a phase III trial. Cancer Immunol Immunother. (2016) 65:1395–400. doi: 10.1007/s00262-016-1898-2
83. Otsuka M, Sugihara S, Mori S, Hamada K, Sasaki Y, Yoshikawa S, et al. Immune-related adverse events correlate with improved survival in patients with advanced mucosal melanoma treated with nivolumab: A single-center retrospective study in Japan. J Dermatol. (2020) 47:356–62. doi: 10.1111/1346-8138.15246
84. Sohail Z, Samar MR, Qureshi NJ, Arshad S, Zaki A. A curious case of primary gastric mucosal melanoma. Korean J Gastroenterol. (2024) 83:33–6. doi: 10.4166/kjg.2023.136
85. Zumelzu C, Alexandre M, Le Roux C, Weber P, Guyot A, Levy A, et al. Mucous membrane pemphigoid, bullous pemphigoid, and anti-programmed death-1/ programmed death-ligand 1: A case report of an elderly woman with mucous membrane pemphigoid developing after pembrolizumab therapy for metastatic melanoma and review of the literature. Front Med (Lausanne). (2018) 5:268. doi: 10.3389/fmed.2018.00268
86. Nomura M, Oze I, Masuishi T, Yokota T, Satake H, Iwasawa S, et al. Multicenter prospective phase II trial of nivolumab in patients with unresectable or metastatic mucosal melanoma. Int J Clin Oncol. (2020) 25:972–7. doi: 10.1007/s10147-020-01618-9
87. Zhang H, Mi J, Xin Q, Cao W, Song C, Zhang N, et al. Recent research and clinical progress of CTLA-4-based immunotherapy for breast cancer. Front Oncol. (2023) 13:1256360. doi: 10.3389/fonc.2023.1256360
88. Farhangnia P, Ghomi SM, Akbarpour M, Delbandi AA. Bispecific antibodies targeting CTLA-4: game-changer troopers in cancer immunotherapy. Front Immunol. (2023) 14:1155778. doi: 10.3389/fimmu.2023.1155778
89. Babamohamadi M, Mohammadi N, Faryadi E, Haddadi M, Merati A, Ghobadinezhad F, et al. Anti-CTLA-4 nanobody as a promising approach in cancer immunotherapy. Cell Death Dis. (2024) 15:17. doi: 10.1038/s41419-023-06391-x
90. Liu Z, Xu X, Liu H, Zhao X, Yang C, Fu R. Immune checkpoint inhibitors for multiple myeloma immunotherapy. Exp Hematol Oncol. (2023) 12:99. doi: 10.1186/s40164-023-00456-5
91. Saijo K, Imai H, Ouchi K, Okada Y, Sato Y, Komine K, et al. Therapeutic benefits of ipilimumab among Japanese patients with nivolumab-refractory mucosal melanoma: A case series study. Tohoku J Exp Med. (2019) 248:37–43. doi: 10.1620/tjem.248.37
92. Postow MA, Luke JJ, Bluth MJ, Ramaiya N, Panageas KS, Lawrence DP, et al. Ipilimumab for patients with advanced mucosal melanoma. Oncologist. (2013) 18:726–32. doi: 10.1634/theoncologist.2012-0464
93. Del Vecchio M, Di Guardo L, Ascierto PA, Grimaldi AM, Sileni VC, Pigozzo J, et al. Efficacy and safety of ipilimumab 3mg/kg in patients with pretreated, metastatic, mucosal melanoma. Eur J Cancer. (2014) 50:121–7. doi: 10.1016/j.ejca.2013.09.007
94. Alexander M, Mellor JD, McArthur G, Kee D. Ipilimumab in pretreated patients with unresectable or metastatic cutaneous, uveal and mucosal melanoma. Med J Aust. (2014) 201:49–53. doi: 10.5694/mja13.10448
95. Bello E, Cohen JV, Mino-Kenudson M, Dougan M. Antitumor response to microscopic melanoma in the gastric mucosa mimicking ipilimumab-induced gastritis. J Immunother Cancer. (2019) 7:41. doi: 10.1186/s40425-019-0524-1
96. Barras D, Ghisoni E, Chiffelle J, Orcurto A, Dagher J, Fahr N, et al. Response to tumor-infiltrating lymphocyte adoptive therapy is associated with preexisting CD8(+) T-myeloid cell networks in melanoma. Sci Immunol. (2024) 9:eadg7995. doi: 10.1126/sciimmunol.adg7995
97. Yarza R, Bover M, Herrera-Juarez M, Rey-Cardenas M, Paz-Ares L, Lopez-Martin JA, et al. Efficacy of T-cell receptor-based adoptive cell therapy in cutaneous melanoma: A meta-analysis. Oncologist. (2023) 28:e406–15. doi: 10.1093/oncolo/oyad078
98. Hall MS, Teer JK, Yu X, Branthoover H, Snedal S, Rodriguez-Valentin M, et al. Neoantigen-specific CD4(+) tumor-infiltrating lymphocytes are potent effectors identified within adoptive cell therapy products for metastatic melanoma patients. J Immunother Cancer. (2023) 11(10):e007288. doi: 10.1136/jitc-2023-007288
99. Zhang J, Yu M, Li X, Huang X, Wang H. Combination therapy improves immune response and prognosis in patients with advanced oral mucosal melanoma: A clinical treatment success. Oral Surg Oral Med Oral Pathol Oral Radiol. (2018) 126:307–16. doi: 10.1016/j.oooo.2018.05.004
100. Li Z, Zhang Q, Zhang X, Jin Q, Yue Q, Li N, et al. Dihydroartemisinin inhibits melanoma migration and metastasis by affecting angiogenesis. Phytother Res. (2023). doi: 10.1002/ptr.8065
101. Dong Y, Wei J, Yang F, Qu Y, Huang J, Shi D. Nutrient-based approaches for melanoma: prevention and therapeutic insights. Nutrients. (2023) 15(20):4483. doi: 10.3390/nu15204483
102. In GK, Ribeiro JR, Yin J, Xiu J, Bustos MA, Ito F, et al. Multi-omic profiling reveals discrepant immunogenic properties and a unique tumor microenvironment among melanoma brain metastases. NPJ Precis Oncol. (2023) 7:120. doi: 10.1038/s41698-023-00471-z
103. Cui C, Mao L, Chi Z, Si L, Sheng X, Kong Y, et al. randomized, double-blind, placebo-controlled multicenter trial of Endostar in patients with metastatic melanoma. Mol Ther. (2013) 21:1456–63. doi: 10.1038/mt.2013.79
104. Shustef E, Torres-Cabala CA, Curry JL, Tetzlaff MT, Nagarajan P, Ivan D, et al. Intraepithelial melanoma in the stomach after treatment with immune checkpoint blockade therapy. Am J Dermatopathol. (2017) 39:e116–8. doi: 10.1097/DAD.0000000000000859
105. Moya-Plana A, Herrera Gómez RG, Rossoni C, Dercle L, Ammari S, Girault I, et al. Evaluation of the efficacy of immunotherapy for non-resectable mucosal melanoma. Cancer Immunol Immunother. (2019) 68:1171–8. doi: 10.1007/s00262-019-02351-7
106. Umeda Y, Yoshikawa S, Kiniwa Y, Maekawa T, Yamasaki O, Isei T, et al. Real-world efficacy of anti-PD-1 antibody or combined anti-PD-1 plus anti-CTLA-4 antibodies, with or without radiotherapy, in advanced mucosal melanoma patients: A retrospective, multicenter study. Eur J Cancer. (2021) 157:361–72. doi: 10.1016/j.ejca.2021.08.034
107. Sezen D, Patel RR, Tang C, Onstad M, Nagarajan P, Patel SP, et al. Immunotherapy combined with high- and low-dose radiation to all sites leads to complete clearance of disease in a patient with metastatic vaginal melanoma. Gynecol Oncol. (2021) 161:645–52. doi: 10.1016/j.ygyno.2021.03.017
108. Gestermann N, Saugy D, Martignier C, Tille L, Fuertes Marraco SA, Zettl M, et al. LAG-3 and PD-1+LAG-3 inhibition promote anti-tumor immune responses in human autologous melanoma/T cell co-cultures. Oncoimmunology. (2020) 9:1736792. doi: 10.1080/2162402X.2020.1736792
109. SChadendorf D, Tawbi H, Lipson EJ, Stephen Hodi F, Rutkowski P, Gogas H, et al. Health-related quality of life with nivolumab plus relatlimab versus nivolumab monotherapy in patients with previously untreated unresectable or metastatic melanoma: RELATIVITY-047 trial. Eur J Cancer. (2023) 187:164–73. doi: 10.1016/j.ejca.2023.03.014
110. Sheng X, Yan X, Chi Z, Si L, Cui C, Tang B, et al. Axitinib in combination with toripalimab, a humanized immunoglobulin G(4) monoclonal antibody against programmed cell death-1, in patients with metastatic mucosal melanoma: an open-label phase IB trial. J Clin Oncol. (2019) 37:2987–99. doi: 10.1200/JCO.19.00210
111. Yan X, Sheng X, Chi Z, Si L, Cui C, Kong Y, et al. Randomized phase II study of bevacizumab in combination with carboplatin plus paclitaxel in patients with previously untreated advanced mucosal melanoma. J Clin Oncol. (2021) 39:881–9. doi: 10.1200/JCO.20.00902
112. Taylor MH, Lee CH, Makker V, Rasco D, Dutcus CE, Wu J, et al. Phase IB/II trial of lenvatinib plus pembrolizumab in patients with advanced renal cell carcinoma, endometrial cancer, and other selected advanced solid tumors. J Clin Oncol. (2020) 38:1154–63. doi: 10.1200/JCO.19.01598
113. Mao L, Fang M, Chen Y, Wei X, Cao J, Lin J, et al. Atezolizumab plus bevacizumab in patients with unresectable or metastatic mucosal melanoma: A multicenter, open-label, single-arm phase II study. Clin Cancer Res. (2022) 28:4642–8. doi: 10.1158/1078-0432.CCR-22-1528
114. Heppt MV, Goldscheider I, Tietze JK, Berking C. Intralesional interleukin-2 for unresectable mucosal melanoma refractory to nivolumab. Cancer Immunol Immunother. (2017) 66:1377–8. doi: 10.1007/s00262-017-2012-0
115. Adileh M, Yuval JB, Huang S, Shoushtari AN, Quezada-Diaz F, Pappou EP, et al. Anorectal mucosal melanoma in the era of immune checkpoint inhibition: should we change our surgical management paradigm? Dis Colon Rectum. (2021) 64:555–62. doi: 10.1097/DCR.0000000000001872
116. Kimura T, Takahama T, Wakasa T, Adachi S, Akashi Y, Tamura T, et al. Role of debulking surgery in combination with immune therapy: A successfully treated case of locally advanced mucosal melanoma. Mol Clin Oncol. (2022) 16:2. doi: 10.3892/mco
117. Diab A, Tannir NM, Bentebibel SE, Hwu P, Papadimitrakopoulou V, Haymaker C, et al. Bempegaldesleukin (NKTR-214) plus nivolumab in patients with advanced solid tumors: phase I dose-escalation study of safety, efficacy, and immune activation (PIVOT-02). Cancer Discovery. (2020) 10:1158–73. doi: 10.1158/2159-8290.CD-19-1510
118. Tang B, Mo J, Yan X, Duan R, Chi Z, Cui C, et al. Real-world efficacy and safety of axitinib in combination with anti-programmed cell death-1 antibody for advanced mucosal melanoma. Eur J Cancer. (2021) 156:83–92. doi: 10.1016/j.ejca.2021.07.018
119. Pozniak J, Pedri D, Landeloos E, Van Herck Y, Antoranz A, Vanwynsberghe L, et al. A TCF4-dependent gene regulatory network confers resistance to immunotherapy in melanoma. Cell. (2024) 187:166–183.e25. doi: 10.1016/j.cell.2023.11.037
120. Redondo-Muñoz M, Rodriguez-Baena FJ, Aldaz P, Caballé-Mestres A, Moncho-Amor V, Otaegi-Ugartemendia M, et al. Metabolic rewiring induced by ranolazine improves melanoma responses to targeted therapy and immunotherapy. Nat Metab. (2023) 5:1544–62. doi: 10.1038/s42255-023-00861-4
121. Liu N, Yan M, Tao Q, Wu J, Chen J, Chen X, et al. Inhibition of TCA cycle improves the anti-PD-1 immunotherapy efficacy in melanoma cells via ATF3-mediated PD-L1 expression and glycolysis. J Immunother Cancer. (2023) 11(9):e007146. doi: 10.1136/jitc-2023-007146
122. Garbe C, Dummer R, Amaral T, Amaria RN, Ascierto PA, Burton EM, et al. Neoadjuvant immunotherapy for melanoma is now ready for clinical practice. Nat Med. (2023) 29:1310–2. doi: 10.1038/s41591-023-02336-1
Keywords: mucosal melanoma, tumor microenvironment, immunotherapy, immune checkpoint inhibitors, cancer
Citation: Shan Z and Liu F (2024) Advances in immunotherapy for mucosal melanoma: harnessing immune checkpoint inhibitors for improved treatment outcomes. Front. Immunol. 15:1441410. doi: 10.3389/fimmu.2024.1441410
Received: 31 May 2024; Accepted: 01 July 2024;
Published: 30 July 2024.
Edited by:
Weiliang Hou, Tongji University, ChinaReviewed by:
Jiaji Yue, Shenzhen Second People’s Hospital, ChinaJialu Song, Jinhua Central Hospital, China
Copyright © 2024 Shan and Liu. This is an open-access article distributed under the terms of the Creative Commons Attribution License (CC BY). The use, distribution or reproduction in other forums is permitted, provided the original author(s) and the copyright owner(s) are credited and that the original publication in this journal is cited, in accordance with accepted academic practice. No use, distribution or reproduction is permitted which does not comply with these terms.
*Correspondence: Fei Liu, bGl1ZmVpMDFAY2FuY2VyaG9zcC1sbi1jbXUuY29t