- 1Department of Medicine, Georgetown University, Washington, DC, United States
- 2National Centre for Neuroimmunology and Emerging Diseases, Griffith University, Gold Coast, QLD, Australia
Reduced natural killer (NK) cell cytotoxicity is the most consistent immune finding in myalgic encephalomyelitis/chronic fatigue syndrome (ME/CFS). Meta-analysis of the published literature determined the effect size of the decrement in ME/CFS. Databases were screened for papers comparing NK cell cytotoxicity in ME/CFS and healthy controls. A total of 28 papers and 55 effector:target cell ratio (E:T) data points were collected. Cytotoxicity in ME/CFS was significantly reduced to about half of healthy control levels, with an overall Hedges’ g of 0.96 (0.75–1.18). Heterogeneity was high but was explained by the range of E:T ratios, different methods, and potential outliers. The outcomes confirm reproducible NK cell dysfunction in ME/CFS and will guide studies using the NK cell model system for pathomechanistic investigations.
Systematic review registration: https://www.crd.york.ac.uk/prospero/, identifier CRD42024542140.
Introduction
Myalgic encephalomyelitis/chronic fatigue syndrome (ME/CFS) is a chronic disease with disability, fatigue, post-exertional malaise (PEM), cognitive lapses, non-refreshing sleep, interoceptive distress, pain, and orthostatic complaints (1–5). PEM is the key manifestation. Physical, cognitive, or emotional exertion at greater than usual levels leads to symptom relapse that may be of immediate onset or delayed by hours and that forces patients to remain in bed or house bound until recovery. Patients often recall a severe flu-like illness that never resolved. There have been epidemic outbreaks, but most cases are sporadic. The symptom profile mirrors long coronavirus disease 2019 (COVID), the persistent fatiguing illness that does not abate after acute severe acute respiratory syndrome coronavirus 2 (SARS-CoV-2) infection (6) and that was predicted to be a precursor to ME/CFS (7). Multiple lines of evidence suggest that ME/CFS and long COVID are parallel symptom complexes that share brain, metabolic, and immune pathologies (8). There are no diagnostic tests or approved therapies for either disorder.
Natural killer (NK) cell dysfunction has been observed in ME/CFS since 1987 (9) and contributes to the hypothesis of immune dysfunction in ME/CFS. In contrast, other measures such as aberrations in pro- and anti-inflammatory cytokines, lymphocyte populations, autoantibodies, metabolomics, and functional assays have not been shown to be reproducible (10). NK cell dysfunction is supported by extensive research into changes in NK cell phenotype profiles, surface regulatory receptors, reduced store-operated calcium levels, significantly reduced lytic protein production, and release. Cytotoxicity assesses all steps of NK cell activation from receptor-ligand binding, kinase pathway and calcium mobilization, microtubule and granule polarization, degranulation of dense and light granules with release of their contents, upregulation of markers of degranulation, and subsequent apoptosis with loss of membrane integrity of the target cells.
The K562 human erythroleukemia tumor cell line is the standard target cell to assess NK cell function in vitro. K562 cells do not express major histocompatibility complex class I (MHC-I) human leukocyte antigens (HLA)-A, HLA-B, or HLA-C that would normally be recognized by NK cell killer inhibitory receptors (KIR) and prevent cytotoxicity (11).
There are two major methods to measure NK cell cytotoxicity. The traditional “gold standard” 51Chromium (51Cr) release assay involves loading 51Cr into K562 cells, which are then incubated with whole blood, peripheral blood mononuclear cells (PBMCs), or isolated NK cells at effector to target cell (E:T) ratios of 50:1 to 6.25:1 for 4 h at 37°C in 5% carbon dioxide (12–15). NK cell cytotoxicity is quantitatively measured by the release of 51Cr into the supernatant and calculation of percentage (%) killing at each E:T ratio. A non-radioactive alternative method is fluorescent cytometry to detect NK-cell-induced apoptosis of K562 cells with expression of cell surface phosphatidylserine by binding fluorescently tagged annexin V (16). Unfortunately, both methods are labor intensive, require special safety measures, and are not approved as diagnostic clinical tests (17) for ME/CFS.
This meta-analysis was performed to quantify the effect size (Hedges’ g) for the difference in % cytotoxicity of NK cells between ME/CFS and healthy controls (HC). The literature was reviewed to improve understanding of the details of these tests. An innovation was to extract data and analyze the effects of individual E:T ratios and the extrapolated outcomes.
Results
Overview
The review process selected 28 manuscripts for the final meta-analysis (Figure 1 and Table 1). Title and abstract screening was completed for 579 records obtained from literature reviews by Strayer et al. (19), Eaton-Fitch et al. (10), and an additional review of the literature to include more recent publications by JNB. Strayer et al. cited referenced 48 references with 27 papers related to NK cell cytotoxicity. Eaton-Fitch et al. (10) identified a total of 523 papers from Medline (EBSCOhost) (n=111), Embase (n=159), PubMed (n=73), and Scopus (n=180) databases. Their review cited 64 manuscripts, but their analysis was limited to 17 NK cell function papers.
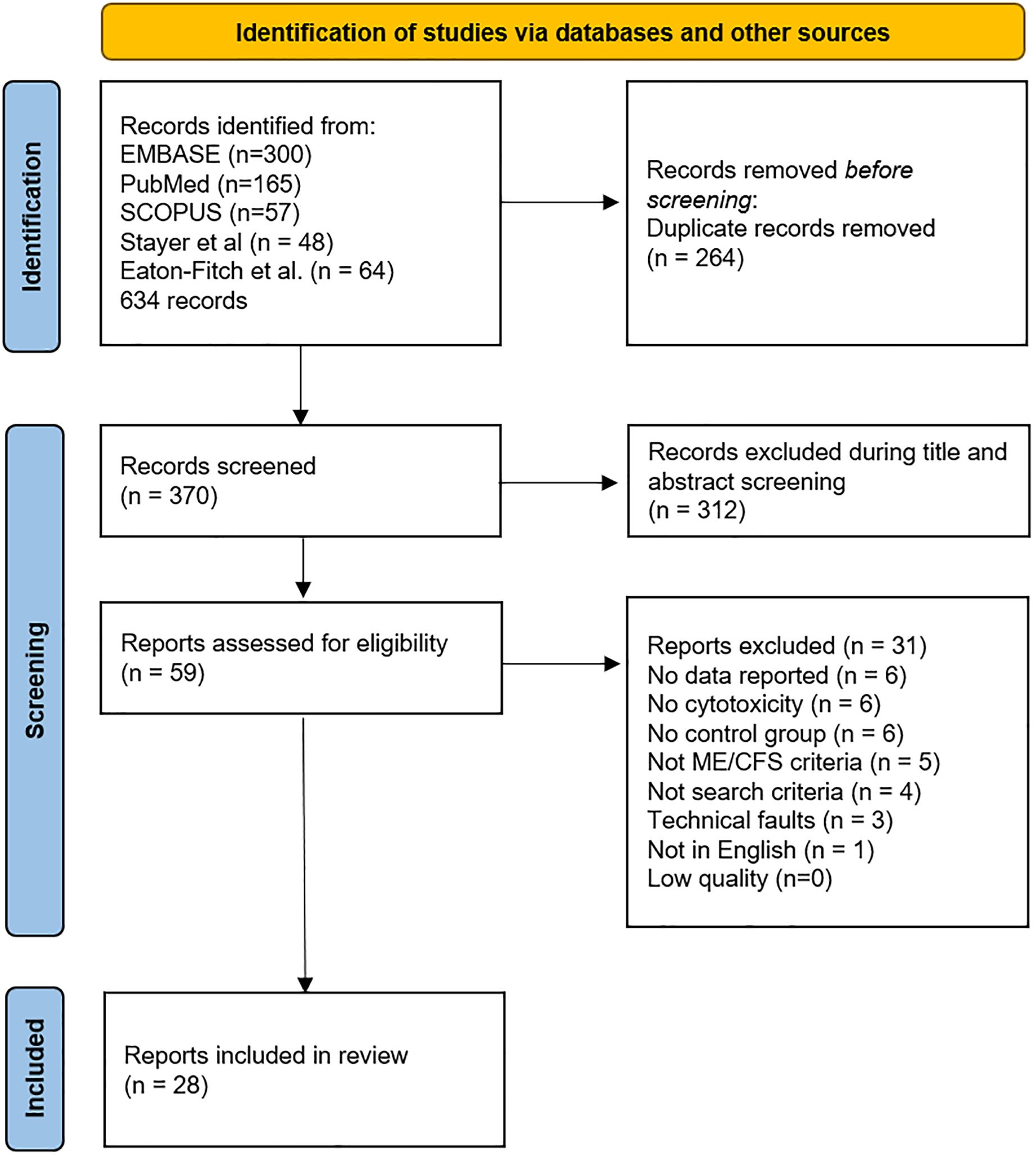
Figure 1. PRISMA flow diagram demonstrating searches of databases and other sources completed by JNB.
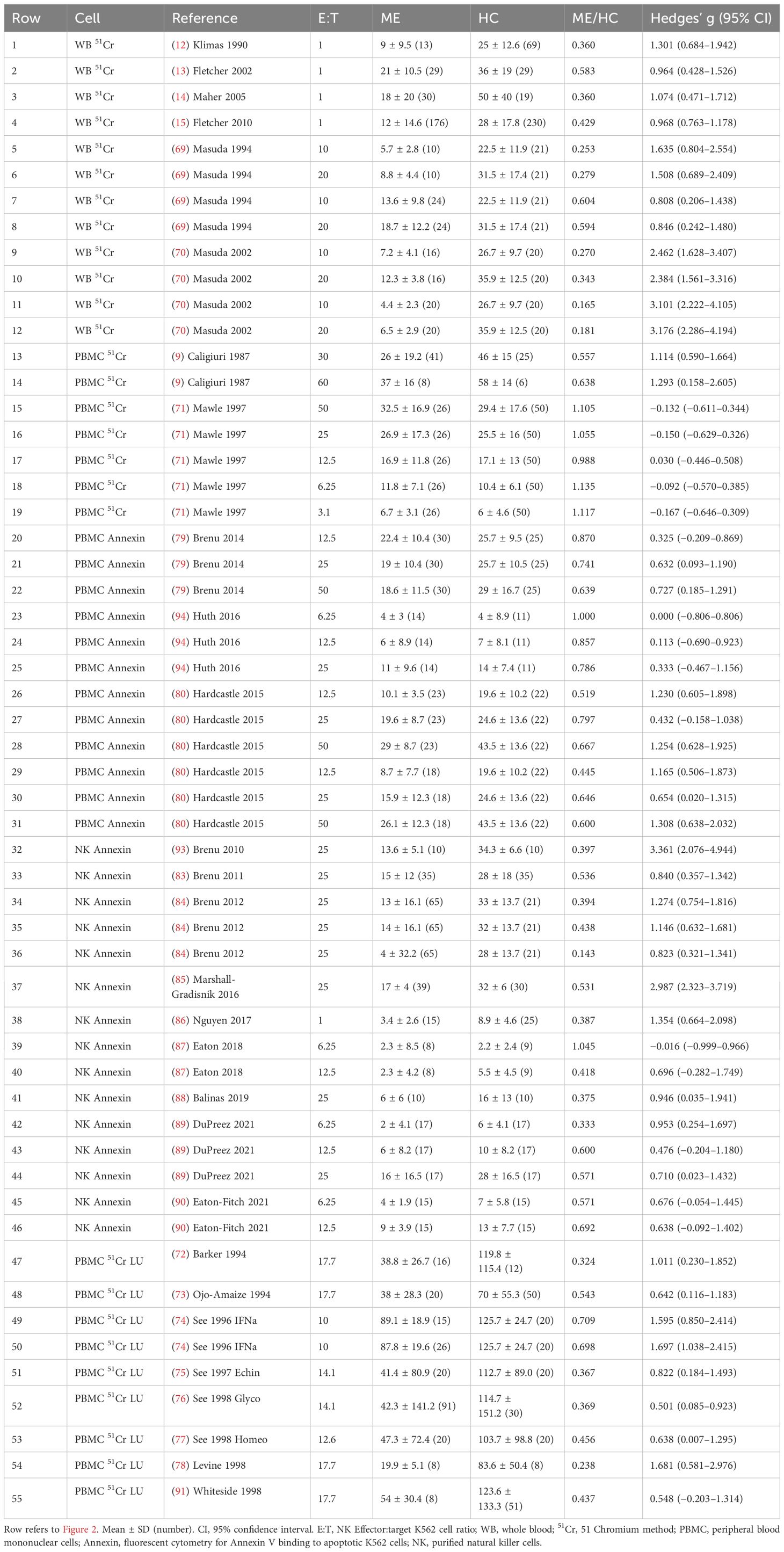
Table 1. Data for each E:T ratio. Hedges’ g between ME/CFS and HC was determined for each E:T ratio.
The literature search completed by JNB for this meta-analysis on 1 January 2024 yielded 522 records from Embase (n=300), Scopus (n=57), and PubMed (n=165) databases. The search identified eight new references published after 2018 plus nine older studies not previously found for a total of 17 additional NK cell cytotoxicity records. One foreign article and two abstracts were found. References, related articles, and cited literature were examined for any additional pertinent information. The process yielded 58 relevant records.
The database search was repeated in PubMed and Google Scholar on 21 March 2024 and identified 498 records. Applying ME/CFS and cytotoxicity filters decreased the list to 244. Removing duplicates (n=67) and papers that did not report on NK cell cytotoxicity (n=121) or other criteria reduced this list to 25 publications. Papers published since 2019 and three older papers were found that had not been previously retrieved. Conversely, three studies found by the 1 January 2024 search were not found by the later database review. This emphasized the need to scrutinize references to find papers that do not share keywords or mention cytotoxicity in the title or abstract yet present data in Results or Supplementary Online Materials.
The survey results were compiled, duplicates removed, and the remaining 59 records read for quantitative NK cell cytotoxicity results from ME/CFS and healthy control subjects using fresh specimens. No relevant papers were excluded because they were behind paywalls or otherwise inaccessible. A total of 31 studies were excluded because of inadequate methods or missing data (19, 32, 40–68). The remaining 28 records (9, 12–15, 69–91) (Table 1 and Supplementary Table S1) were included in the meta-analysis.
Manuscript characteristics
The included studies were assessed for effects of cell source, method, anticoagulant, and diagnostic criteria (Supplementary Table S1). ME/CFS was diagnosed using 1988 Holmes (1) criteria (9, 12, 13, 69–78), 1994 Center for Disease Control “Fukuda” (2) criteria (14, 15, 79, 83–85, 87–93), and 2011 International Consensus Criteria (3, 86, 94). There was a paucity of quality of life and fatigue severity data, which limited investigation of relationships between these features of disease and NK cell cytotoxicity as a potential biomarker. Cells were collected as whole blood (12–15, 69, 70), PBMC (72–80, 91, 94), and NK cells (82–90, 93) purified by negative selection. Samples were collected via venipuncture in blood collection tubes containing the anticoagulants heparin or EDTA (20). Whole blood 51Cr studies were corrected for CD3-CD56+ NK cells (12–15). Isolated NK cells reported a high purity for CD3-CD56+ cells (>95%).
NK cell cytotoxicity methods were 51Cr release assay (9, 12–15, 45, 49, 69–78) and fluorescent cytometry of annexin V binding to cell surface phosphatidylserine as a marker of apoptosis (32, 59, 84, 87–90, 93, 94). E:T ratios ranged from 100:1 to 1:1 in the various studies with data reported for each E:T ratio, or extrapolated to theoretical ratios of 1:1 (12–15) or 50:1 (32). E:T ratios were inferred for studies reporting lytic units (LU). NK cell cytotoxicity data were presented for each E:T ratio, extrapolated E:T ratio, and LU (55 data points) (Table 1 and Supplementary Table S2). Six studies employed the whole blood 51Cr method of which four involved extrapolation of E:T dose responses to 1:1 (12–15). PBMCs and 51Cr quantification were used in 10 studies (9, 33, 71–78). PBMCs and fluorescent cytometry of annexin V on target cells was used in three studies with 12 E:T ratios (79, 80, 94). Purified NK cells with fluorescent cytometry of annexin V were assessed at 15 E:T ratios in nine studies (83–90, 93).
There were diverse reasons for study exclusion. Two studies found equivalent cytotoxicity in ME/CFS and HC but did not report the data (40, 41). Nine records reported significantly reduced cytotoxicity in ME/CFS, but six did not include control subjects (19, 43–45, 65, 68) and four did not provide any quantitative data (46–48, 66). Five cytotoxicity studies did not use accepted definitions of ME/CFS but instead defined subjects as low NK cell syndrome (51–54) and EBV infection (50). Seven studies did not report cytotoxicity (55–60, 67). Three papers were excluded for technical reasons including using cells that had been frozen or shipped overnight (32, 45, 49) and measurement of cytoplasmic LDH release into the supernatant (42). Four other papers did not meet the search criteria (61–63).
NK cell cytotoxicity in ME/CFS
Overall, Hedges’ g was 0.96 (0.75–1.18) (95% confidence interval) for the entire set of 28 studies, 2,982 subjects, and 55 E:T ratios using the random effects model (Supplementary Table S3) (35–37). The random forest plot showed the range of effect sizes for whole blood extrapolated to 1:1, LU, and individual E:T ratio data points (Figure 2). The data were explored to examine factors that may have influenced the effect size outcome (95). In general, effect sizes for methods were ranked whole blood 51Cr [1.60 (1.06–2.14)] > NK annexin [1.08 (0.63–1.53] > PBMC annexin [0.70 (0.41–0.98)] = PBMC 51Cr (Table 2 and Supplementary Table S3).
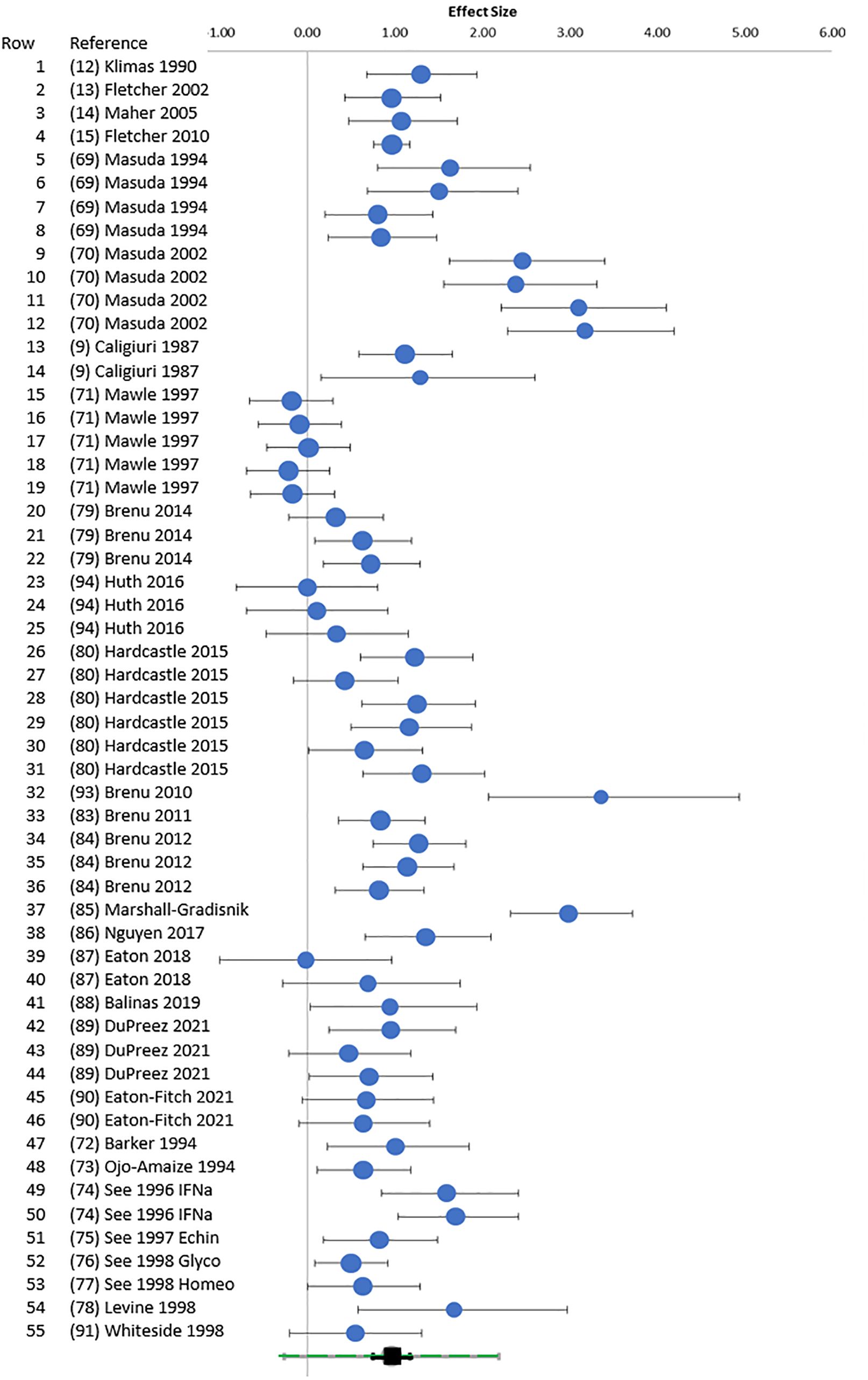
Figure 2. Random forest. Random forest plot. Hedges’ g (95% CI) was plotted for each E:T ratio for points cross-referenced to Table 1 (rows 1–55). The green square at the bottom was the overall Hedges’ g with 95% CI (black) and 95% prediction interval (green).
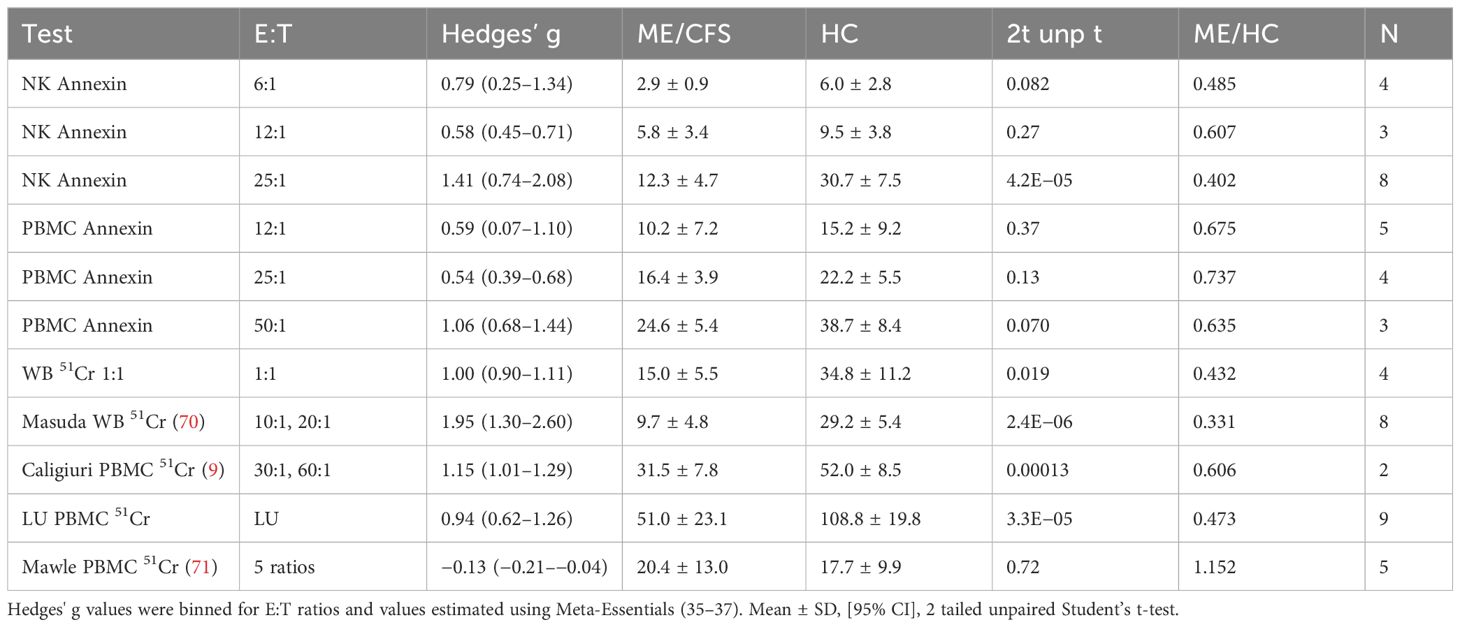
Table 2. Hedges’ g. Cytotoxicity levels were compared between ME/CFS and HC for bins of E:T ratios in annexin dose responses and 51Cr assays.
Heterogeneity was present based on the wide distribution of Hedges’ g values in the random forest plot (Figure 2), funnel plot (Figure 3), I2 of 80.03, and Q of 270.45. The high heterogeneity was likely due to low cytotoxicity at low E:T ratios, outliers with high g (70, 85, 93), and one apparently negative study that contributed five E:T ratios with Hedges’ g of 0.02 to −0.18 (71).
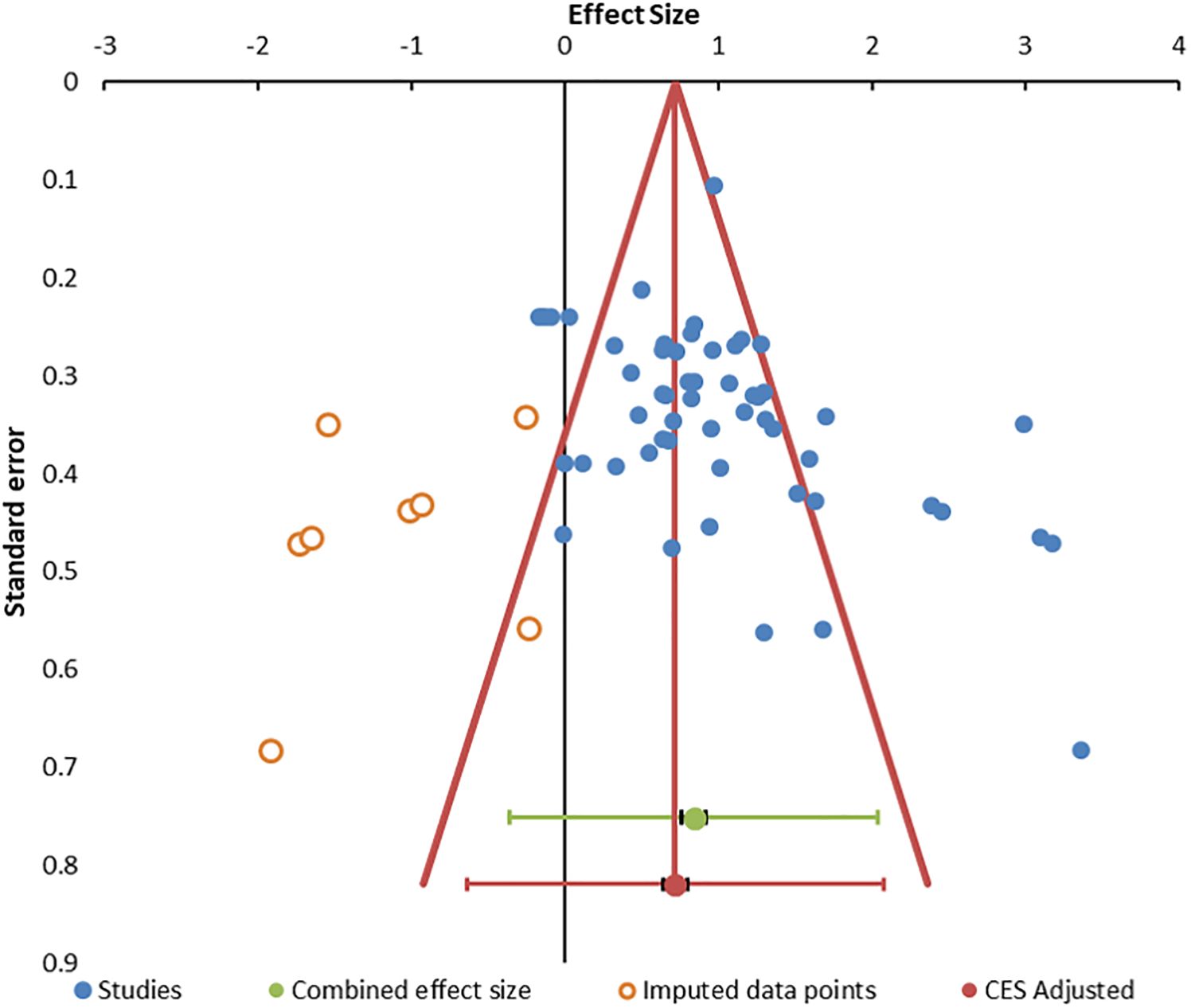
Figure 3. Funnel. Funnel plot for publication bias. The 55 data points are indicated by blue circles. Imputed data points are open red circles. The combined effect size (green circle), confidence interval, and prediction intervals are shown in green. The adjusted combined effect size is red.
The distribution of outliers was assessed by standardized residual histogram that was symmetrical with skew of 0.309, suggesting equal balance of high and low effect sizes around the mean but was squat (kurtosis = 0.606) with significant outliers at both ends of the curve (Supplementary Figure S1) (96, 97). The Galbraith plot reiterated the pattern of outliers. The quantile plot was linear (y = 2.258x + 0.242; R² = 0.969) suggesting a single population of studies (98). Hedges’ g was not related to sample size by regression analysis (R2 = 0.01), indicating that studies with small sample sizes did not bias the outcome (99). Data for these plots are in Supplementary Table S2.
Publication bias was suggested by the funnel plot (Figure 3) (96, 99) and significant Egger (p = 0.014) and Begg and Mazumdar (p = 0.001) tests (Supplementary Table S4). The trim and fill method predicted eight missing studies (Supplementary Table S5). The method of Gleser and Olkin (100) predicted that 53 unpublished studies (i.e., E:T ratios) would be needed to overcome the published literature (Supplementary Table S6). Rosenthal’s Failsafe N of 9,556 (z = 21.74) (101) and Fisher Failsafe-N of 3,571 (Fisher’s chi-squared p<0.0001) (102) suggested that large numbers of unpublished data would be required to negate the current meta-analysis. Reiteration to account for these influences led to an adjusted combined effect size of 0.75 (95% CI, 0.67–0.83).
Additional investigations found no differences based on number of subjects in each study, diagnostic criteria, anticoagulant, cell source, method, or E:T ratio by moderator analysis and univariate general linear modeling. Year of publication was not significant, which was in agreement with the consistency of the 51Cr and annexin methodologies over time. Estimated marginal means in univariate analysis with these variables found that effect sizes were larger in whole blood (WB) [1.53 (1.09–1.98), p = 0.00051] and NK [1.14 (0.79–1.50), p = 0.0081] than PBMC [0.44 (0.094–0.79)]. Variances were homogeneous between studies (Levene test p > 0.15). Effect sizes exhibited heteroskedasticity (p = 0.028 by the modified Breusch–Pagan test). There were insufficient data to infer effects of age, duration of disease, gender, fatigue severity, or disability.
The relationship between % cytotoxicity of ME/CFS and HC groups at each E:T ratio was examined by scatter plot. ME/CFS NK cell cytotoxicity was approximately half that of HC (Figure 4). Data from each combination of cell source and method were distributed along the regression lines. There was a strong correlation (R2 = 0.75) when LU data were plotted as the published % cytotoxicities (Supplementary Figure S2). Data from four whole blood 51Cr experiments that were extrapolated to 1:1 (open triangles) (12–15) were not used for the regressions because the 1:1 ratio was outside the range of ratios tested in the experiment and were not comparable to the other E:T data. NK cell cytotoxicity correlated with E:T ratios for HC (R2 = 0.57) and ME/CFS (R2 = 0.61). Data points for cytotoxicity <10% and E:T ≤ 12.5:1 were closely clustered and may not discriminate between HC and ME/CFS.
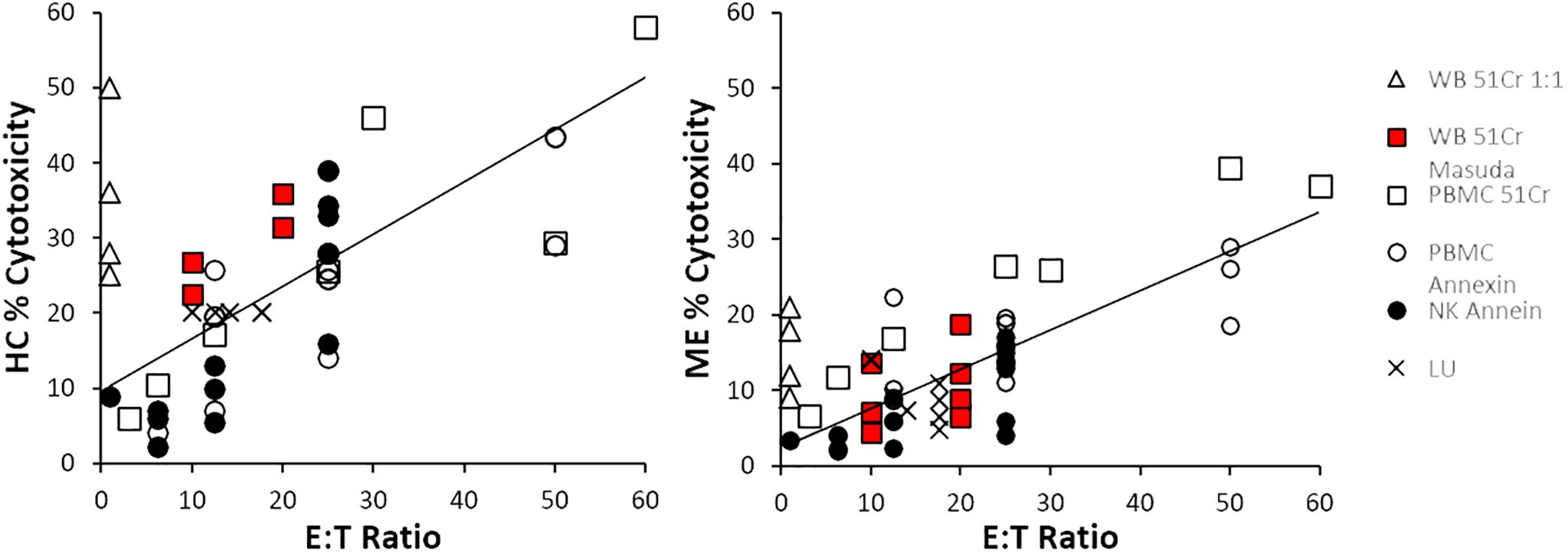
Figure 4. Cytotoxicity and E:T ratio. The correlations between cytotoxicity and E:T were determined for all data points with the exception of whole blood 51Cr extrapolated to 1:1, which were outside the experimental testing range of E:T ratios (open triangles). The slopes of the regression line from the other whole blood 51Cr tests (red squares), PMBC 51Cr (open squares), PBMC annexin (open circles), NK cells with annexin (black circles), and lytic units (LUs, crosses) were higher in HC than ME/CFS.
The linear relationship was studied further by plotting the ratio of ME/CFS to HC cytotoxicity against E:T (Supplementary Figure S3). In general, there was no correlation. The majority of the ME/CFS to HC values were between 0.15 and 0.8 (0.57 ± 0.26, mean ± SD, n=55). The mean plus on SD was 0.828, which may be serve as an upper limit of % cytotoxicity in ME/CFS.
There were no correlations between effect size and E:T ratio when all data were plotted (Supplementary Figure S4).
Receiver operating characteristics (ROC) investigated the optimal range for NK cell cytotoxicity % across assays. ROC were computed for % cytotoxicities of ME/CFS and HC for each cell source, method, LU, bins of E:T ratios, and values extrapolated to 1:1 to provide guidance for thresholds that would discern ME/CFS from HC. A general trend was the increase in threshold with increasing E:T ratios. The ratio of % cytotoxicity over E:T was taken as a proxy for killing per single NK cell and was 0.775 (0.317–0.777] and HC 1.45 (1.05–1.84) and had an ROC threshold of 0.84. ROC thresholds were calculated for individual methods (Supplementary Table S7). When all data were assessed, the overall threshold for % cytotoxicity was 17.1% with sensitivity and specificity of 0.764 and corresponded to an E:T ratio of approximately 25:1 (Table 3).
The average cytotoxicities for ME/CFS and HC at binned E:T ratios were presented to place the ROC (Table 3) and effect size (Table 2) data into perspective.
Discussion
NK cell cytotoxicity in ME/CFS groups was approximately half that of HC (Figure 4). The difference had a large effect size with Hedges’ g of 0.96 (0.75–1.18), indicating that the measurement is a reproducible biomarker despite differences in assay methods. The high heterogeneity was explained by low % cytotoxicity at low E:T and high and low outliers. The diversity of research methods complicates direct comparisons between studies and emphasizes the need for standardized protocols in future research.
There is no consensus in the literature for the range of normal for NK cell cytotoxicity and levels that indicate “significant” loss of cytotoxicity. Research practice has been to compare ME/CFS to HC cohorts. This strategy is difficult to convert to a viable clinical laboratory test because of the need for a parallel HC population and algorithms to deal with confounding conditions such as cancer, HIV, obesity, and congenital reductions in NK cell activity. One alternative has been to set the mean of the HC group minus one SD as the lower threshold of normal to account for 80% of the normal distribution (33, 78, 91). Conversely, the mean and one SD for the ME/CFS to HC ratios accounted for 83% of ME/CFS cytotoxicity (Supplementary Figure S3). The distribution for Hedges’ g ± one SD identified the high studies (70, 85, 93) and low outliers (71, 79, 87, 94) that generated the large study heterogeneity. ROC analysis calculated thresholds for cytotoxicity reached a consensus of 17.1% for E:T ratios of 25:1, 23.0 for WB 51Cr results extrapolated to 1:1 (12–15), and 18.6% for all other 51Cr results including LU (Table 3 and Supplementary Table S7).
The meta-analysis suggests that the 51Cr whole blood assay remains the gold standard (Figure 2). However, limitations remain due to the spontaneous 51Cr release from the target cells that will increase the background radioactivity and reduce the signal-to-background ratio over time. This sets a practical limit to the incubation time of 51Cr measurements (103). The method of cell lysis for the determination of total cellular 51Cr must be stated as detergent solubilization release more than hypotonic lysis. The potential health effects, specific skill set, and laboratory radiation safety requirements pose additional challenges. Fluorescent cytometry with NK cells at E:T of 25:1 and higher doses (Figure 3) is a viable non-radioactive method that correlates with 51Cr release (104, 105).
Purified NK cells provide a direct assay of cytotoxicity without other cellular or plasma interactions. Whole blood includes plasma, circulating cytokines, hormones, and other factors that help reflect the in vivo milieu, and platelets, erythrocytes, granulocytes, and lymphocytes that may interact or interfere with NK cell function. Transferable factors such as autoantibodies have been considered as pathological agents in ME/CFS that could be responsible for inhibiting NK cell function. However, removing plasma for PBMC and NK cell purification did not alleviate the deficit in ME/CFS. Studies of PBMCs using 51Cr and annexin had comparable effect sizes, suggesting that the two methods were equivalent.
Alternative methods may be introduced to decrease the number of NK cells required and simplify protocols for routine clinical laboratory use. Many variations have been proposed but not studied in ME/CFS including using 500 instead of 5,000 target cells per well in 51Cr assay (107), diverse labeling agents for target cells including calcein-acetoxymethyl diacetylester (calcein AM) (108) and europium (109, 110), combination of DNA staining SYTOX Green with Annexin V for apoptosis (111), and non-invasive, quantitative image-based cytometry (112) and newer instruments (113). Technological advances such as microfluidic devices may allow high throughput assays for highly reproducible robust endpoints. Individual cell analysis may reveal additional dysfunction such as cytolytic heterogeneity that may contribute to ME/CFS pathology. For example, only 20% of NK cells were reported to be highly efficient killers, suggesting another variable to take into consideration (114–116). The number of K562 cells killed per NK cell is lower in ME/CFS [0.11 (0.05–0.23) (95%CI)] than HC [0.64 (0.36–1.32) (95%CI)] (12), suggesting that the efficient killers may lyse several target cells during the 4-h incubation period while the majority may not contribute. A spectrum for killing efficiency may relate to differences in NK cell phenotypes between tissue-based cytokine-producing CD3-CD56+ that are approximately 10%–20% of peripheral blood NK cells and blood-borne CD3-CD56dimCD16+ and CD3-CD56dimCD16+C57+ cells with greater cytotoxic proficiency. Future studies should enumerate the phenotypes and estimate separate E:T ratios for each. Not adjusting for NK cell phenotype may explain the relatively poor cytotoxicity for PBMCs (Figure 4). Decreasing the yield of CD3-CD56dimCD16+ NK cells is another reason to avoid freezing (106).
Measuring NK cell degranulation is another alternative, as this function is an essential component of cytotoxicity. Measures of degranulation include upregulation of NK cell surface CD107 expression (58) and release of dense and light granule proteins such as perforin, granzyme, chemokines, and interferon gamma into supernatants (117). The frequency of upregulation of CD107a expression as a measure of degranulation was twice the rate of cytotoxicity (45). Degranulation assesses stages from membrane contact of NK cells and target cells to granule release and upregulation CD107a and other surface receptors but does not assess the killing function and induction of apoptosis in the target cell with upregulation of annexin V and entry of dyes that stain DNA (118). Studies of degranulation alone were excluded because they do not fully assess mechanisms of cell killing. Studies of antibody-dependent cellular cytotoxicity (ADCC) were also excluded (67) because the method depends on cellular targets coated with exogenous antibodies and activation through CD16. The mechanism of activation is different, although later stages may utilize many of the same pathways as natural cytotoxicity.
The sample condition is an important consideration in the experimental design. Freezing cells and overnight shipping of blood or PBMC leads to loss of cytotoxicity and, in particular, loss of the deficit in ME/CFS compared to control (45). Theorell et al. (49) was excluded because they used (a) frozen cells even though frozen NK cells do not preserve cytotoxicity after being thawed and (b) a non-standard method of quantification that did not report E:T ratios. Querec et al. (32) used shipped cells and measured cytotoxicity by intracellular DNA staining that does not provide a suitable measure of early apoptotic cells (111). It is well reported that NK surface marker expression and cytotoxicity are poorly preserved after delayed use and freezing (33, 106, 119–121). NK cell cytotoxicity is reduced after freezing overnight in 51Cr assays of whole blood and PBMC with 26.2% ± 21.6% cytotoxicity when fresh dropping to 5.0% ± 3.1% after freezing (45). The levels of cytotoxicity between fresh and frozen cells were correlated. The physiological and cellular cause(s) of the deficient NK cell cytotoxicity in ME/CFS and the loss of % cytotoxicity with storage, remain unknown. The use of specimens after prolonged shipping or freezing and thawing is discouraged until reliable cryopreservation methods are validated. Freshly isolated samples are advocated for higher sensitivity and accuracy of cytotoxicity measurements. New methods will be needed in the future to maintain NK cell function during shipping to central laboratories for approved clinical testing.
K562 cells were the targets for cytotoxicity testing. Studies with EBV-transformed and other tumor cell lines were excluded. Studies using non-specific PMA, PHA, and concavalin stimulation or other measures of immune dysregulation in CFS were excluded (43, 55, 80, 81, 89, 122–126). Culture with IL2, IL15, and IL18 can increase cytotoxicity by up to 10-fold (43, 89). However, cytokine-cultured cells were excluded because they develop “cytokine-induced cytotoxicity” (125, 126) and lymphokine-activated killer (LAK) cell phenotypes in vitro that do not reflect NK cell function in vivo. A complicating factor is that blood NK cells have a higher proportion of CD56+CD16+ NK cells with greater cytotoxic potential in contrast to tissues that contain more CD56Bright NK cells that may have different functions and roles in disease. This issue will remain to be addressed in the future.
Mechanisms for decreased cytotoxicity may involve increased expression of inhibitory KIR or checkpoint proteins, increased SHP1 expression that suppresses kinase pathways that are essential for degranulation and cytotoxicity, reduced intracellular dense or light granule proteins, decreased chemokine, TNFalpha or INFgamma production, suppression of mTOR and glycolysis, or other metabolic and energetic alterations. Investigation of degranulation issue is relevant to other degranulating cells such as CD8 T lymphocytes and presynaptic neurons that share many of the same pathways and proteins in shuttling granules for exocytosis. Therefore, studies in NK cells may shed light on important immune deficits of host defense and the cognitive dysfunction of ME/CFS. This line of reasoning highlights the case that NK cells may be a model system for studying the molecular pathogenesis of ME/CFS. Drug testing in the NK cell model in vitro is possible given past results with inosine (44), INFalpha (74), various supplements (75–77), glucagon-like peptide 1 (127), and naltrexone (90, 128, 129). The current outcomes support those innovations and provide effect sizes to help design future investigations.
The differential diagnosis of low NK cytotoxicity is of importance in considering the specificity for ME/CFS. Low NK syndrome with chronic fatigue was proposed by Aoki et al. (51, 52) and investigated in EBV (50) and families of ME/CFS patients (78). NK activity was reproducible over time in these studies (54, 130) and ME/CFS (84). NK cell cytotoxicity may decrease after 75 years of age (131). Genetically defined congenital immunodeficiency with <1% NK cells in blood has been associated with mutations of GATA2 and MCM4, while functional loss of antibody-dependent cellular cytotoxicity is associated with FCGR3A abnormalities (132). NK cell numbers and function are reduced in HIV infection (75). These diseases emphasize the need for history and physical and laboratory examination to rule out treatable and other diagnosed conditions in order to make the diagnosis of ME/CFS. Routine evaluations will prevent false positive attributions of reduced NK cell numbers or function due to major depression, alcohol abuse, neoplasia, HIV, and chronic infectious and autoimmune diseases. Hospitalization for acute severe depression was associated with reduced NK cell cytotoxicity (133, 134). The defects resolved after 6 months of successful treatment, suggesting that neuroendocrine alterations may be capable to induce NK cell dysfunction. Hospitalization per se and schizophrenia were not related to the immune deficit (135). Chronic alcohol abuse reduced cytotoxicity and had an additive effect with depression (136). The interaction of male gender and major depression may cause more significant deficits in NK cell function in contrast to women (134). These conditions are standard exclusions for ME/CFS diagnosis (137–140). Studies of interactions of these variables and ME/CFS could not be done using currently available data, but may be accessible at the individual level if common data elements for ME/CFS severity, quality of life patient-reported outcome measures, depression, and other relevant measures are included in future studies (32, 43, 79, 90) and used in multivariate analyses.
The study of Mawle et al. (71) contributed five E:T data points and appeared to be an outlier in the meta-analysis because NK cell cytotoxicity was equivalent between ME/CFS and HC. The authors reported no differences by subgroup analysis for sudden or gradual onset, duration of illness, or health status. Criticism of this study (71) noted that subjects with ME/CFS for longer than 10 years were excluded from recruitment (19), and frozen blood from the ME/CFS subjects did not show evidence of active or latent infection by herpes virus 6 (HHV-6) (141) in contrast to other studies (142–144). This study was considered a potential outlier for heterogeneity analysis.
Obesity reduces NK cell cytotoxicity (145–148). However, few studies reported BMI or excluded obese ME/CFS or control subjects (90), meaning that this variable cannot be addressed retrospectively. Hyperlipidemia, peroxisome proliferator-activated receptor (PPAR) alpha/delta agonist drugs, butyrate (149), and other metabolic changes in obesity reduce the function of mammalian target of rapamycin (mTOR) and NK cytotoxicity in vivo and in vitro (150). The molecular mechanisms may be relevant to ME/CFS pathophysiology.
Literature selection bias was a concern because the heterogeneity analysis suggested the possible underrepresentation of studies reporting equivalent NK cell cytotoxicity between ME/CFS and HC. The studies that were excluded were reviewed for additional insights into publication bias. Nine records stated that NK cell cytotoxicity in ME/CFS was significantly reduced relative to historical controls (19, 43–45, 65, 68) but did not provide quantitative data (46–48, 66). Five studies reported on “low NK syndrome” subjects with low cytotoxicity and symptoms attributable to post-EBV infection status and chronic fatigue that did not meet standard diagnostic criteria (50–54). These reports document a wide range of cytotoxicity in control cohorts and makes it necessary that parallel control groups be tested to define abnormal results. In contrast to these 14 potentially positive studies, only three studies reported equivalent NK cell cytotoxicity in ME/CFS and HC. However, they were excluded for not reporting the data (40, 41) or used the insensitive LDH release assay at E:T of 100:1 (40–42). If these publications are representative of missing “file drawer” studies (101), then any unpublished data would likely continue to support the deficit in NK cell cytotoxicity of ME/CFS.
Additional limitations included concerns that the surveyed manuscripts are not representative of the field. However, the current search extended the result of prior reviews of NK cell cytotoxicity in ME/CFS (10, 19) without finding additional foreign literature or obscure negative results.
Heterogeneity was high but likely due to small effect sizes at low E:T ratios for PBMC and NK studies, especially large effect sizes in three studies (70, 85, 93) and apparently negative outcomes from Mawle et al. (71).
It is not always possible to control for population-based confounding variables while synthesizing data for a meta-analysis further biasing data. Data were stratified in order to clarify effects of diagnostic criteria, anticoagulant, type of cells, method, and E:T ratios. Data have been published as the E:T dose response, extrapolated to 1:1 or 50:1, or as lytic units (LU), which presents a challenge for comparing study outcomes. This was circumvented by comparing results for each E:T ratio. Lytic units were discouraged because they were standardized to 20% cytotoxicity unlike all of the other studies. Expressing the data as E:T allowed investigation of dose responses that were not evident by extrapolation strategies. This exposed the stratification between PBMC 51Cr and annexin methods, NK annexin, and whole blood 51Cr methods (Tables 2, 3 and Figure 4). Variables such as criteria, cells, and methods were assessed as fixed factors to determine their impact. Unfortunately, there were insufficient data to investigate age, gender, fatigue, disability, and other clinical outcomes. Alternative modeling may be of value, particularly if NK cell cytotoxicity, quality of life, and other questionnaire data from individuals were used.
NK cell dysfunction has been reported in long COVID (151) and Gulf War Illness (122, 152) that have similar symptom profiles. It will be of interest to contrast molecular mechanisms underlying loss of NK cell cytotoxicity between these conditions.
Conclusion
NK cells from ME/CFS subjects have significantly lower cytotoxicity than control subjects. The reduction in K562 cell killing by fresh NK cells remains one of the most promising potential biomarkers for ME/CFS. Frozen and shipped cells do not retain sufficient cytotoxicity. Whole blood 51Cr assays have the largest effect size, but extrapolating without showing the raw data reduced the information that can be gained. Purified NK cells with E:T of 25:1 and detection by fluorescent cytometry using Annexin V for early and late apoptosis was a reasonable non-radioactive alternative. Hedges’ g and thresholds for ME/CFS and HC % cytotoxicity at various E:T values and different cell sources and methods provide guidelines to diagnose ME/CFS in future studies. Fresh specimens or new methods will be necessary for NK cell cytotoxicity to become a routine clinical laboratory test for diagnosis. Technical problems related to the assay methods are a limitation that may be overcome by innovative engineering. Future studies should report NK cell cytotoxicity with subjective common data elements to understand behavioral correlations and investigate interactions with dysfunction of metabolomics, mitochondria, and brain cell function using magnetic resonance imaging (153) in order to gain a better understanding of integrated disease pathophysiology and symptom generation. NK cells represent a model system to understand molecular mechanisms of disease in ME/CFS and for testing potential drugs in vitro (44, 68, 74–77, 90, 128, 129) for efficacy before human clinical trials. The effect sizes calculated here may allow improved design for future studies of deficient NK cell cytotoxicity in ME/CFS.
Method
This meta-analysis was undertaken according to Cochrane review guidelines and aimed to identify observational studies of NK cell cytotoxic activity in ME/CFS and HC research participants for quantitative analysis.
Database and literature search
The current analysis was built on previous systematic literature searches into NK cell function in ME/CFS carried out by Natelson in 2002 (18); Strayer, Scott, and Carter in 2015 (19); and Eaton-Fitch et al. in 2019 (10). Strayer, Scott, and Carter searched for publications in English in 2015 on PubMed and in “Google” using key words “Natural Killer Cell Activity” (NK Cell Activity), “chronic fatigue syndrome” (CFS), “Flow Cytometry,” and “Chromium 51” (19). Eaton-Fitch et al. (10) screened publications in PubMed, Medline (EBSCOhost), Embase, and Scopus for full-text terms “chronic fatigue syndrome” OR “myalgic encephalomyelitis” OR “ME/CFS” AND “natural killer cell” and medical subject headings (MeSH) for “chronic fatigue syndrome/myalgic encephalomyelitis” [including systematic exertion intolerance disease (SEID)] and “natural killer cells” (10). Papers from Natelson were cited in the more recent review.
The protocol was compared with published listings on the PROSPERO website (National Institute for Health Research) (https://www.crd.york.ac.uk/prospero/) for duplication and prospectively registered on the database (ID: CRD42024542140). An updated search of PubMed, Embase, and Scopus databases followed Preferred Reporting Items for Systematic Reviews and Meta-analyses (PRISMA) guidelines and was completed 1 January 2024 by JNB. Full-text search terms included “chronic fatigue syndrome” OR “myalgic encephalomyelitis” OR “ME/CFS” AND “natural killer cell cytotoxicity.”. Medical subject headings (MeSH) terms were used for chronic fatigue syndrome/myalgic encephalomyelitis (including systematic exertion intolerance disease), natural killer cells, natural killer cell function, and cytotoxicity. Proximity operators were not used during the literature search. Reference lists were checked, and citations were searched for additional publications. Unpublished literature was not searched. No additional papers were identified through alternative search databases such as Griffith University institute library or Google Scholar. The search strategy used in this meta-analysis was independently validated on 21 March 2024 by NE-F.
Selection criteria
The collected articles were initially screened at the level of titles and abstracts to include studies that reported on NK cell cytotoxicity in ME/CFS patient groups. Candidate papers were read in depth to find figures, tables, and other data reporting NK cell cytotoxicity in Results and Supplementary Online Materials sections and additional leads to other relevant literature. This comprehensive analysis ensured that the manuscripts fulfilled inclusion criteria for the meta-analysis:
i. free full text publication available through institutional access;
ii. original research without duplicate publication;
iii. comparison of ME/CFS versus healthy control (HC) subjects;
iv. diagnosis of ME/CFS according to criteria including 1988 Holmes (1), 1994 Center for Disease Control (“Fukuda”) (2), Canadian Consensus Criteria (CCC) for ME/CFS (5), International Consensus Criteria (ICC) for ME/CFS (3), Institute of Medicine criteria for Systemic Exertion Intolerance Disease SEID (4), or other established criteria;
v. research participants were human adults age 18 years and older;
vi. manuscripts detailed core information including numbers of subjects in each group;
vii. sample collection method with anticoagulation by heparin or EDTA (20);
viii. sources of cells in whole blood (WB), PBMC, or purified NK cells;
ix. the condition of the samples as either fresh versus frozen or stored cells;
x. method of NK cell cytotoxicity analysis; and
xi. E:T cell ratios or lytic units (LUs).
Records were excluded from the primary analysis if the ME/CFS cohort was not compared to healthy controls. This excluded treatment studies; comparisons to other patient groups such as fibromyalgia, multiple sclerosis, chronic fatigue not meeting ME/CFS definitions; Epstein–Barr virus or HIV infection; comparisons to historical control groups; binary stratification into normal and low cytotoxicity based on local laboratory standards; cells incubated with cytokines to enhance or maintain their viability and functions; and use of frozen cells or specimens with prolonged overnight shipping.
Quality assessment
Studies were evaluated for quality and bias using the Joanna Briggs Institute Checklist for case control studies (21). Additionally, the Downs and Black checklist was followed to assess the clarity of descriptions of outcomes and findings, reported probability outcomes, recruitment details and participant representation of populations (22, 23), and described previously (10).
Data extraction
Figures, tables, text, and Supplementary Materials were assessed for individual data points, sample size (N), mean, median, standard error of the mean (SEM), standard deviation (SD), 95% confidence intervals (CI), interquartile 25% and 75% ranges (IQR), and range of % cytotoxicity. Results were converted to mean, SD, and N (24–31) for each E:T ratio or extrapolated E:T values such as 1:1 (12–15), 1:50 (32), and LU (33).
Studies extrapolating to LU represented a special case. They used dose responses with E:T from 50:1 to 6:1 but did not report cytotoxicity at each ratio. Instead, data were interpolated to 20% cytotoxicity for each subject, and the number of PBMC (effectors) extrapolated per 10e7 cells using the formula of Pross et al. (34). One LU was defined as the number of effector cells needed to lyse 20% of the K562 target cells (1,000 out of 5,000 targets). The numbers of effector and K562 cells at 20% cytotoxicity for HC were interpolated and converted to E:T ratios and the corresponding % cytotoxicity for ME/CFS calculated from the published LU values.
Meta-analysis and other statistics
Effect sizes for the difference between ME/CFS and HC in each study were calculated as Hedges’ g using Meta-Essentials (35–37). In addition to the overall weighted Hedges’ g from all studies, effect sizes were calculated for individual E:T ratios, cell sources, and NK cell cytotoxicity methods. Study heterogeneity was tested by Q and I2 (38, 39). Publication bias was examined by funnel plot and Failsafe N test as per the Meta-Essentials manual.
Results were displayed as % cytotoxicity for ME/CFS and HC groups, ME/HC ratio of % cytotoxicity, Hedges g,and E:T ratios. Hedges’ g for subgroups were amalgamated using Meta-Essentials software (35–37). Differences between subgroups were determined by analysis of variance (ANOVA) followed by Tukey’s honest significant difference for correction of multiple comparisons. Univariate regression of ME/HC, ME/CFS, and HC data was used to study interactions of diagnostic criteria (Holmes, Fukuda, and International) (1–3), cell sources (whole blood, PBMC, and purified NK cells), anticoagulant (heparin and EDTA), methods (51Cr, annexin), number of participants in each study, and year of publication as a measure of evolving trends in methodologies. There was insufficient data to evaluate age, gender, duration of ME/CFS, quality of life, or fatigue severity.
Receiver operating characteristics (ROC) were applied to ME/CFS and HC data for each method and E:T ratio to infer significant threshold values that may be used to optimize the assays and distinguish ME/CFS from HC.
Data availability statement
The original contributions presented in the study are included in the article/Supplementary Material. Further inquiries can be directed to the corresponding author.
Author contributions
JB: Conceptualization, Data curation, Formal analysis, Investigation, Methodology, Project administration, Resources, Supervision, Validation, Visualization, Writing – original draft, Writing – review & editing. NE-F: Conceptualization, Data curation, Investigation, Methodology, Validation, Writing – original draft, Writing – review & editing. SM-G: Conceptualization, Funding acquisition, Project administration, Supervision, Writing – review & editing.
Funding
The author(s) declare financial support was received for the research, authorship, and/or publication of this article. The Stafford Fox Medical Research Foundation (489798).
Acknowledgments
We are thankful to Ms. Tania Manning, Ms. Kay Schwarz, and Mrs. Vivienne Baraniuk for the oversight and recruitment of participants for this study.
Conflict of interest
The authors declare that the research was conducted in the absence of any commercial or financial relationships that could be construed as a potential conflict of interest.
Publisher’s note
All claims expressed in this article are solely those of the authors and do not necessarily represent those of their affiliated organizations, or those of the publisher, the editors and the reviewers. Any product that may be evaluated in this article, or claim that may be made by its manufacturer, is not guaranteed or endorsed by the publisher.
Supplementary material
The Supplementary Material for this article can be found online at: https://www.frontiersin.org/articles/10.3389/fimmu.2024.1440643/full#supplementary-material
References
1. Holmes GP, Kaplan JE, Gantz NM, Komaroff AL, Schonberger LB, Straus SE, et al. Chronic fatigue syndrome: a working case definition. Ann Intern Med. (1988) 108:387–9. doi: 10.7326/0003-4819-108-3-387
2. Fukuda K, Straus SE, Hickie I, Sharpe MC, Dobbins JG, Komaroff A. The chronic fatigue syndrome: a comprehensive approach to its definition and study. International Chronic Fatigue Syndrome Study Group. Ann Intern Med. (1994) 121:953–9. doi: 10.7326/0003-4819-121-12-199412150-00009
3. Carruthers BM, van de Sande MI, De Meirleir KL, Klimas NG, Broderick G, Mitchell T, et al. Myalgic encephalomyelitis: international consensus criteria. J Intern Med. (2011) 270:327–38. doi: 10.1111/j.1365-2796.2011.02428.x
4. Committee on the Diagnostic Criteria for Myalgic Encephalomyelitis/Chronic Fatigue Syndrome, Board on the Health of Select Populations, Institute of Medicine. Beyond Myalgic Encephalomyelitis/Chronic Fatigue Syndrome Redefining an Illness. Washington (DC): National Academies Press (US) (2015). doi: 10.17226/19012
5. Carruthers BM. Definitions and aetiology of myalgic encephalomyelitis: how the Canadian consensus clinical definition of myalgic encephalomyelitis works. J Clin Pathol. (2007) 60:117–9. doi: 10.1136/jcp.2006.042754
6. Thaweethai T, Jolley SE, Karlson EW, Levitan EB, Levy B, McComsey GA, et al. Development of a definition of postacute sequelae of SARS-coV-2 infection. JAMA. (2023) 329:1934. doi: 10.1001/jama.2023.8823
7. Komaroff AL, Lipkin WI. Insights from myalgic encephalomyelitis/chronic fatigue syndrome may help unravel the pathogenesis of postacute COVID-19 syndrome. Trends Mol Med. (2021) 27:895–906. doi: 10.1016/j.molmed.2021.06.002
8. Komaroff AL, Lipkin WI. ME/CFS and Long COVID share similar symptoms and biological abnormalities: road map to the literature. Front Med (Lausanne). (2023) 10:1187163. doi: 10.3389/fmed.2023.1187163
9. Caligiuri M, Murray C, Buchwald D, Levine H, Cheney P, Peterson D, et al. Phenotypic and functional deficiency of natural killer cells in patients with chronic fatigue syndrome. J Immunol. (1987) 139:3306–13. doi: 10.4049/jimmunol.139.10.3306
10. Eaton-Fitch N, du Preez S, Cabanas H, Staines D, Marshall-Gradisnik S. A systematic review of natural killer cells profile and cytotoxic function in myalgic encephalomyelitis/chronic fatigue syndrome. Syst Rev. (2019) 8:279. doi: 10.1186/s13643-019-1202-6
11. Klein E, Ben-Bassat H, Neumann H, Ralph P, Zeuthen J, Polliack A, et al. Properties of the K562 cell line, derived from a patient with chronic myeloid leukemia. Int J Cancer. (1976) 18:421–31. doi: 10.1002/ijc.2910180405
12. Klimas NG, Salvato FR, Morgan R, Fletcher MA. Immunologic abnormalities in chronic fatigue syndrome. J Clin Microbiol. (1990) 28:1403–10. doi: 10.1128/jcm.28.6.1403-1410.1990
13. Fletcher MA, Maher KJ, Klimas NG. Natural killer cell function in chronic fatigue syndrome. Clin Appl Immunol Rev. (2002) 2:129–39. doi: 10.1016/S1529-1049(01)00047-2
14. Maher KJ, Klimas NG, Fletcher MA. Chronic fatigue syndrome is associated with diminished intracellular perforin. Clin Exp Immunol. (2005) 142:505–11. doi: 10.1111/j.1365-2249.2005.02935.x
15. Fletcher MA, Zeng XR, Maher K, Levis S, Hurwitz B, Antoni M, et al. Biomarkers in chronic fatigue syndrome: evaluation of natural killer cell function and dipeptidyl peptidase IV/CD26. PloS One. (2010) 5:e10817. doi: 10.1371/journal.pone.0010817
16. Aubry JP, Blaecke A, Lecoanet-Henchoz S, Jeannin P, Herbault N, Caron G, et al. Annexin V used for measuring apoptosis in the early events of cellular cytotoxicity. Cytometry. (1999) 37:197–204. doi: 10.1002/(ISSN)1097-0320
17. Motzer SA, Tsuji J, Hertig V, Johnston SK, Scanlan J. Natural killer cell cytotoxicity: a methods analysis of 51chromium release versus flow cytometry. Biol Res Nurs. (2003) 5:142–52. doi: 10.1177/1099800403257196
18. Natelson BH, Haghighi MH, Ponzio NM. Evidence for the presence of immune dysfunction in chronic fatigue syndrome. Clin Diagn Lab Immunol. (2002) 9:747–52. doi: 10.1128/CDLI.9.4.747-752.2002
19. Strayer D, Scott V, Carter W. Low NK cell activity in chronic fatigue syndrome (CFS) and relationship to symptom severity. J Clin Cell Immunol. (2015) 6:348. doi: 10.4172/2155-9899.1000348
20. Son BK, Roberts RL, Ank BJ, Stiehm ER. Effects of anticoagulant, serum, and temperature on the natural killer activity of human peripheral blood mononuclear cells stored overnight. Clin Diagn Lab Immunol. (1996) 3:260–4. doi: 10.1128/cdli.3.3.260-264.1996
21. Munn Z, Barker TH, Moola S, Tufanaru C, Stern C, McArthur A, et al. Methodological quality of case series studies: an introduction to the JBI critical appraisal tool. JBI Evid Synth. (2020) 18(10):2127–33. doi: 10.11124/JBISRIR-D-19-00099
22. Downs SH, Black N. The feasibility of creating a checklist for the assessment of the methodological quality both of randomised and non-randomised studies of health care interventions. J Epidemiol Community Health. (1978) 52:377–84. doi: 10.1136/jech.52.6.377
23. Hooper P, Jutai JW, Strong G, Russell-Minda E. Age-related macular degeneration and low-vision rehabilitation: a systematic review. Can J Ophthalmol. (2008) 43:180–7. doi: 10.3129/i08-001
24. Higgins JPT, Thompson SG, Deeks JJ, Altman DG. Measuring inconsistency in meta-analyses. BMJ. (2003) 327:557–60. doi: 10.1136/bmj.327.7414.557
25. Hozo SP, Djulbegovic B, Hozo I. Estimating the mean and variance from the median, range, and the size of a sample. BMC Med Res Methodol. (2005) 5:13. doi: 10.1186/1471-2288-5-13
26. Shi J, Luo D, Weng H, Zeng XT, Lin L, Chu H, et al. Optimally estimating the sample standard deviation from the five-number summary. Res Synth Methods. (2020) 11:641–54. doi: 10.1002/jrsm.1429
27. Shi J, Luo D, Wan X, Liu Y, Liu J, Bian Z, et al. Detecting the skewness of data from the five-number summary and its application in meta-analysis. Stat Methods Med Res. (2023) 32:1338–60. doi: 10.1177/09622802231172043
28. Luo D, Wan X, Liu J, Tong T. Optimally estimating the sample mean from the sample size, median, mid-range, and/or mid-quartile range. Stat Methods Med Res. (2018) 27:1785–805. doi: 10.1177/0962280216669183
29. Estimating the sample mean and standard deviation (SD) from the five-number summary and its application in meta-analysis (2024). Available online at: https://www.math.hkbu.edu.hk/~tongt/papers/median2mean.html. Accessed December 2, 2023.
30. Lee YH. An overview of meta-analysis for clinicians. Korean J Intern Med. (2018) 33:277–83. doi: 10.3904/kjim.2016.195
31. Wan X, Wang W, Liu J, Tong T. Estimating the sample mean and standard deviation from the sample size, median, range and/or interquartile range. BMC Med Res Methodol. (2014) 14:135. doi: 10.1186/1471-2288-14-135
32. Querec TD, Lin JMS, Chen Y, Helton B, Kogelnik AM, Klimas NG, et al. Natural killer cytotoxicity in myalgic encephalomyelitis/chronic fatigue syndrome (ME/CFS): a multi-site clinical assessment of ME/CFS (MCAM) sub-study. J Transl Med. (2023) 21:242. doi: 10.1186/s12967-023-03958-2
33. Whiteside TL, Bryant J, Day R, Herberman RB. Natural killer cytotoxicity in the diagnosis of immune dysfunction: criteria for a reproducible assay. J Clin Lab Anal. (1990) 4:102–14. doi: 10.1002/jcla.1860040207
34. Pross HF, Baines MG, Rubin P, Shragge P, Patterson MS. Spontaneous human lymphocyte-mediated cytotoxicity against tumor target cells. IX. The quantitation of natural killer cell activity. J Clin Immunol. (1981) 1:51–63. doi: 10.1007/BF00915477
35. Suurmond R, van Rhee H, Hak T. Introduction, comparison and validation of MetaEssentials: A free and simple tool for meta-analysis. Res Synth Methods. (2017) 8(4):537–53. doi: 10.1002/jrsm.1260
36. Meta-Essentials. Available online at: http://systematicreviewtools.com/tool.php?ref=Meta-Essentials. Accessed January 17, 2022.
37. Van Rhee HJ, Suurmond R, Hak T. User manual for Meta-Essentials: Workbooks for meta-analysis (version 1.4). Rotterdam, The Netherlands: Erasmus Research Institute of Management (2015). Retrieved from www.erim.eur.nl/research-support/meta-essentials. Accessed January 17, 2022.
38. Deeks J, Higgins J, Altman D. Chapter 10 Analysing Data and Undertaking meta-Analyses. In: Cochrane Handbook for Systematic Reviews of Interventions version 6.3 (2022). Available at: https://training.cochrane.org/handbook/current/chapter-10. Accessed January 17, 2022.
39. Cochran WG. The combination of estimates from different experiments. Biometrics. (1954) 10:101. doi: 10.2307/3001666
40. Rasmussen AK, Nielsen H, Andersen V, Barington T, Bendtzen K, Hansen MB, et al. Chronic fatigue syndrome–a controlled cross sectional study. J Rheumatol. (1994) 21:1527–31.
41. Nishikai M. Chronic fatigue syndrome–study of 51 cases treated at the Second Tokyo National Hospital. Nihon Rinsho. (1992) 50:2641–7.
42. Ogawa M, Nishiura T, Yoshimura M, Horikawa Y, Yoshida H, Okajima Y, et al. Decreased nitric oxide-mediated natural killer cell activation in chronic fatigue syndrome. Eur J Clin Invest. (1998) 28:937–43. doi: 10.1046/j.1365-2362.1998.00373.x
43. Siegel SD, Antoni MH, Fletcher MA, Maher K, Segota MC, Klimas N. Impaired natural immunity, cognitive dysfunction, and physical symptoms in patients with chronic fatigue syndrome: preliminary evidence for a subgroup? J Psychosom Res. (2006) 60:559–66. doi: 10.1016/j.jpsychores.2006.03.001
44. Diaz-Mitoma F, Turgonyi E, Kumar A, Lim W, Larocque L, Hyde BM. Clinical improvement in chronic fatigue syndrome is associated with enhanced natural killer cell-mediated cytotoxicity: the results of a pilot study with isoprinosine®. J Chronic Fatigue Syndr. (2003) 11:71–95. doi: 10.1300/J092v11n02_06
45. Querec TD, Abrams J, Stewart JJ, Barnes Z, Balbin E, Klimas N, et al. Evaluation of natural killer cell assay performance on shipped blood specimens. J Immunol Methods. (2021) 495:113049. doi: 10.1016/j.jim.2021.113049
46. Eby NL, Grufferman S, Huang M, Whiteside T, Sumaya C. Natural killer cell activity in the chronic fatigue–immune dysfunction syndrome. In: Ades EW, Lopez C (eds.) Natural Killer Cells and Host Defense, Karger, Basel. (1989). doi: 10.1159/isbn.978-3-318-04961-9
47. Wemm KM, Trestman RL. The effects of a laboratory stressor on natural killer cell function in chronic fatigue syndrome patients. Psychosomatics. (1991) 32:470–1. doi: 10.1016/S0033-3182(91)72060-4
48. Tirelli V, Pinto A, Marotta G, Crovato M, Quaia M, De Paoli P, et al. Clinical and immunologic study of 205 patients with chronic fatigue syndrome: a case series from Italy. Arch Intern Med. (1993) 153:116–7. doi: 10.1001/archinte.1993.00410010136014
49. Theorell J, Bileviciute-Ljungar I, Tesi B, Schlums H, Johnsgaard MS, Asadi-Azarbaijani B, et al. Unperturbed cytotoxic lymphocyte phenotype and function in myalgic encephalomyelitis/chronic fatigue syndrome patients. Front Immunol. (2017) 8:723. doi: 10.3389/fimmu.2017.00723
50. Gold D, Bowden R, Sixbey J, Riggs R, Katon WJ, Ashley R, et al. Chronic fatigue. A prospective clinical and virologic study. JAMA. (1990) 264:48–53. doi: 10.1001/jama.1990.03450010052029
51. Aoki T, Miyakoshi H, Usuda Y, Herberman RB. Low NK syndrome and its relationship to chronic fatigue syndrome. Clin Immunol Immunopathol. (1993) 69:253–65. doi: 10.1006/clin.1993.1178
52. Aoki T, Usuda Y, Miyakoshi H, Tamura K, Herberman RB. Low natural killer syndrome: clinical and immunologic features. Nat Immun Cell Growth Regul. (1987) 6:116–28.
53. Levy S, Herberman R, Whiteside T, Simons A. Stress, Coping, and Infectius Illness: Persistently Low Natural Killer Cell Activity as a Host Risk Factor. Annual Report. Defense Technical Information Center (1988). Available online at: https://apps.dtic.mil/sti/pdfs/ADA192826 and https://apps.dtic.mil/sti/tr/pdf/ADA202830.pdf. Accessed November 19, 2023.
54. Levy SM, Herberman RB, Simons A, Whiteside T, Lee J, McDonald R, et al. Persistently low natural killer cell activity in normal adults: immunological, hormonal and mood correlates. Nat Immun Cell Growth Regul. (1989) 8:173–86.
55. Curriu M, Carrillo J, Massanella M, Rigau J, Alegre J, Puig J, et al. B- and T-cell phenotype and function in patients suffering from Chronic Fatigue Syndrome. J Transl Med. (2013) 11:68. doi: 10.1186/1479-5876-11-68
56. Rivas JL, Palencia T, Fernández G, García M. Association of T and NK cell phenotype with the diagnosis of myalgic encephalomyelitis/chronic fatigue syndrome (ME/CFS). Front Immunol. (2018) 9:1028. doi: 10.3389/fimmu.2018.01028
57. Brenu EW, Hardcastle SL, Atkinson GM, van Driel ML, Kreijkamp-Kaspers S, Ashton KJ, et al. Natural killer cells in patients with severe chronic fatigue syndrome. Auto Immun Highlights. (2013) 4:69–80. doi: 10.1007/s13317-013-0051-x
58. Cliff JM, King EC, Lee JS, Sepúlveda N, Wolf AS, Kingdon C, et al. Cellular immune function in myalgic encephalomyelitis/chronic fatigue syndrome (ME/CFS). Front Immunol. (2019) 10:796. doi: 10.3389/fimmu.2019.00796
59. Nguyen T, Staines D, Nilius B, Smith P, Marshall-Gradisnik S. Novel identification and characterisation of Transient receptor potential melastatin 3 ion channels on Natural Killer cells and B lymphocytes: effects on cell signalling in Chronic fatigue syndrome/Myalgic encephalomyelitis patients. Biol Res. (2016) 49:27. doi: 10.1186/s40659-016-0087-2
60. Chu DT, Lin JR. The effect of F3 on natural killer cell activity in the patients with chronic fatigue syndrome. Proc Am Assoc Cancer Res. (1994) 35:516.
61. Prieto J, Subirá ML, Castilla A, Serrano M. Naloxone-reversible monocyte dysfunction in patients with chronic fatigue syndrome. Scand J Immunol. (1989) 30:13–20. doi: 10.1111/j.1365-3083.1989.tb01183.x
62. Van Rinsum J, Smets LA, Van Rooy H, Van den Eijnden DH. Specific inhibition of human natural killer cell-mediated cytotoxicity by sialic acid and sialo-oligosaccharides. Int J Cancer. (1986) 38:915–22. doi: 10.1002/ijc.2910380620
63. Feistritzer C, Clausen J, Sturn DH, Djanani A, Gunsilius E, Wiedermann CJ, et al. Natural killer cell functions mediated by the neuropeptide substance P. Regul Pept. (2003) 116:119–26. doi: 10.1016/S0167-0115(03)00193-9
64. Maya J. Surveying the metabolic and dysfunctional profiles of T cells and NK cells in myalgic encephalomyelitis/chronic fatigue syndrome. Int J Mol Sci. (2023) 24(15):11937. doi: 10.3390/ijms241511937
65. Porter N, Lerch A, Jason LA, Sorenson M, Fletcher MA, Herrington J. A comparison of immune functionality in viral versus non-viral CFS subtypes. J Behav Neurosci Res. (2010) 8:1–8.
66. Sabath DE, Barcy S, Koelle DM, Zeh J, Ashton S, Buchwald D. Cellular immunity in monozygotic twins discordant for chronic fatigue syndrome. J Infect Dis. (2002) 185:828–32. doi: 10.1086/339194
67. Sung AP, Tang JJJ, Guglielmo MJ, Smith-Gagen J, Bateman L, Navarrete-Galvan L, et al. Antibody-dependent cell-mediated cytotoxicity (ADCC) in familial myalgic encephalomyelitis/chronic fatigue syndrome (ME/CFS). Fatigue. (2020) 8:226–44. doi: 10.1080/21641846.2021.1876613
68. McDermott C, Richards SCM, Thomas PW, Montgomery J, Lewith G. A placebo-controlled, double-blind, randomized controlled trial of a natural killer cell stimulant (BioBran MGN-3) in chronic fatigue syndrome. QJM. (2006) 99:461–8. doi: 10.1093/qjmed/hcl063
69. Masuda A, Nozoe SI, Matsuyama T, Tanaka H. Psychobehavioral and immunological characteristics of adult people with chronic fatigue and patients with chronic fatigue syndrome. Psychosom Med. (1994) 56:512–8. doi: 10.1097/00006842-199411000-00006
70. Masuda A, Munemoto T, Yamanaka T, Takei M, Tei C. Psychosocial characteristics and immunological functions in patients with postinfectious chronic fatigue syndrome and noninfectious chronic fatigue syndrome. J Behav Med. (2002) 25:477–85. doi: 10.1023/A:1020423124675
71. Mawle AC, Nisenbaum R, Dobbins JG, Gary HE, Stewart JA, Reyes M, et al. Immune responses associated with chronic fatigue syndrome: a case-control study. J Infect Dis. (1997) 175:136–41. doi: 10.1093/infdis/175.1.136
72. Barker E, Fujimura SF, Fadem MB, Landay AL, Levy JA. Immunologic abnormalities associated with chronic fatigue syndrome. Clin Infect Dis. (1994) 18 Suppl 1:S136–41. doi: 10.1093/clinids/18.Supplement_1.S136
73. Ojo-Amaize EA, Conley EJ, Peter JB. Decreased natural killer cell activity is associated with severity of chronic fatigue immune dysfunction syndrome. Clin Infect Dis. (1994) 18 Suppl 1:S157–9. doi: 10.1093/clinids/18.Supplement_1.S157
74. See DM, Tilles JG. alpha-Interferon treatment of patients with chronic fatigue syndrome. Immunol Invest. (1996) 25:153–64. doi: 10.3109/08820139609059298
75. See DM, Broumand N, Sahl L, Tilles JG. In vitro effects of eChinacea and ginseng on natural killer and antibody-dependent cell cytotoxicity in healthy subjects and chronic fatigue syndrome or acquired immunodeficiency syndrome patients. Immunopharmacology. (1997) 35:229–35. doi: 10.1016/S0162-3109(96)00125-7
76. See DM, Cimoch P, Chou S, Chang J, Tilles J. The in vitro immunomodulatory effects of glyconutrients on peripheral blood mononuclear cells of patients with chronic fatigue syndrome. Integr Physiol Behav Sci. (1998) 33:280–7. doi: 10.1007/BF02688668
78. Levine PH, Whiteside TL, Friberg D, Bryant J, Colclough G, Herberman RB. Dysfunction of natural killer activity in a family with chronic fatigue syndrome. Clin Immunol Immunopathol. (1998) 88:96–104. doi: 10.1006/clin.1998.4554
79. Brenu EW, Huth TK, Hardcastle SL, Fuller K, Kaur M, Johnston S, et al. Role of adaptive and innate immune cells in chronic fatigue syndrome/myalgic encephalomyelitis. Int Immunol. (2014) 26:233–42. doi: 10.1093/intimm/dxt068
80. Hardcastle SL, Brenu EW, Johnston S, Nguyen T, Huth T, Wong N, et al. Characterisation of cell functions and receptors in Chronic Fatigue Syndrome/Myalgic Encephalomyelitis (CFS/ME). BMC Immunol. (2015) 16:35. doi: 10.1186/s12865-015-0101-4
81. Huth TK, Brenu EW, Ramos S, Nguyen T, Broadley S, Staines D, et al. Pilot study of natural killer cells in chronic fatigue syndrome/myalgic encephalomyelitis and multiple sclerosis. Scand J Immunol. (2016) 83:44–51. doi: 10.1111/sji.12388
82. Brenu EW, Ashton KJ, van Driel M, Staines DR, Peterson D, Atkinson GM, et al. Cytotoxic lymphocyte microRNAs as prospective biomarkers for Chronic Fatigue Syndrome/Myalgic Encephalomyelitis. J Affect Disord. (2012) 141:261–9. doi: 10.1016/j.jad.2012.03.037
83. Brenu EW, van Driel ML, Staines DR, Ashton KJ, Ramos SB, Keane J, et al. Immunological abnormalities as potential biomarkers in Chronic Fatigue Syndrome/Myalgic Encephalomyelitis. J Transl Med. (2011) 9:81. doi: 10.1186/1479-5876-9-81
84. Brenu EW, van Driel ML, Staines DR, Ashton KJ, Hardcastle SL, Keane J, et al. Longitudinal investigation of natural killer cells and cytokines in chronic fatigue syndrome/myalgic encephalomyelitis. J Transl Med. (2012) 10:88. doi: 10.1186/1479-5876-10-88
85. Marshall-Gradisnik S, Huth T, Chacko A, Johnston S, Smith P, Staines D. Natural killer cells and single nucleotide polymorphisms of specific ion channels and receptor genes in myalgic encephalomyelitis/chronic fatigue syndrome. Appl Clin Genet. (2016) 9:39–47. doi: 10.2147/TACG
86. Nguyen T, Johnston S, Clarke L, Smith P, Staines D, Marshall-Gradisnik S. Impaired calcium mobilization in natural killer cells from chronic fatigue syndrome/myalgic encephalomyelitis patients is associated with transient receptor potential melastatin 3 ion channels. Clin Exp Immunol. (2017) 187:284–93. doi: 10.1111/cei.12882
87. Eaton N, Cabanas H, Balinas C, Klein A, Staines D, Marshall-Gradisnik S. Rituximab impedes natural killer cell function in Chronic Fatigue Syndrome/Myalgic Encephalomyelitis patients: A pilot in vitro investigation. BMC Pharmacol Toxicol. (2018) 19:12. doi: 10.1186/s40360-018-0203-8
88. Balinas C, Cabanas H, Staines D, Marshall-Gradisnik S. Transient receptor potential melastatin 2 channels are overexpressed in myalgic encephalomyelitis/chronic fatigue syndrome patients. J Transl Med. (2019) 17:401. doi: 10.1186/s12967-019-02155-4
89. Du Preez S, Eaton-Fitch N, Cabanas H, Staines D, Marshall-Gradisnik S. Characterization of IL-2 stimulation and TRPM7 pharmacomodulation in NK cell cytotoxicity and channel co-localization with PIP2 in myalgic encephalomyelitis/chronic fatigue syndrome patients. Int J Environ Res Public Health. (2021) 18(22):11879. doi: 10.3390/ijerph182211879
90. Eaton-Fitch N, Cabanas H, du Preez S, Staines D, Marshall-Gradisnik S. The effect of IL-2 stimulation and treatment of TRPM3 on channel co-localisation with PIP2 and NK cell function in myalgic encephalomyelitis/chronic fatigue syndrome patients. J Transl Med. (2021) 19:306. doi: 10.1186/s12967-021-02974-4
91. Whiteside TL, Friberg D. Natural killer cells and natural killer cell activity in chronic fatigue syndrome. Am J Med. (1998) 105:27S–34S. doi: 10.1016/S0002-9343(98)00155-7
92. Hardcastle SL, Brenu EW, Johnston S, Nguyen T, Huth T, Ramos S, et al. Serum immune proteins in moderate and severe chronic fatigue syndrome/myalgic encephalomyelitis patients. Int J Med Sci. (2015) 12:764–72. doi: 10.7150/ijms.12399
93. Brenu EW, Staines DR, Baskurt OK, Ashton KJ, Ramos SB, Christy RM, et al. Immune and hemorheological changes in chronic fatigue syndrome. J Transl Med. (2010) 8:1. doi: 10.1186/1479-5876-8-1
94. Huth TK, Staines D, Marshall-Gradisnik S. ERK1/2, MEK1/2 and p38 downstream signalling molecules impaired in CD56 dim CD16+ and CD56 bright CD16 dim/- natural killer cells in Chronic Fatigue Syndrome/Myalgic Encephalomyelitis patients. J Transl Med. (2016) 14:97. doi: 10.1186/s12967-016-0859-z
96. Sutton A, Abrams K, Jones D, Sheldon T, Song F. Methods for Meta-analysis in Medical Research Vol. 22. Sutton AJ, Abrams KR, Jones DR, Sheldon TA, Song F, editors. Chichester, U.K.: Wiley (2000) p. 3112–4.
97. Lin L, Chu H. Quantifying publication bias in meta-analysis. Biometrics. (2018) 74:785–94. doi: 10.1111/biom.12817
98. Wang MC, Bushman BJ. Using the normal quantile plot to explore meta-analytic data sets. Psychol Methods. (1998) 3:46–54. doi: 10.1037/1082-989X.3.1.46
99. Sterne JA, Egger M. Funnel plots for detecting bias in meta-analysis: guidelines on choice of axis. J Clin Epidemiol. (2001) 54:1046–55. doi: 10.1016/S0895-4356(01)00377-8
100. Gleser LJ, Olkin I. Models for estimating the number of unpublished studies. Stat Med. (1996) 15:2493–507. doi: 10.1002/(ISSN)1097-0258
101. Rosenthal R. The file drawer problem and tolerance for null results. Psychol Bull. (1979) 86:638–41. doi: 10.1037/0033-2909.86.3.638
102. Fisher RA. Statistical Methods for Research Workers. Oliver and Boyd, Edinburgh, Scotland. (1925).
103. Peper JK, Schuster H, Löffler MW, Schmid-Horch B, Rammensee HG, Stevanović S. An impedance-based cytotoxicity assay for real-time and label-free assessment of T-cell-mediated killing of adherent cells. J Immunol Methods. (2014) 405:192–8. doi: 10.1016/j.jim.2014.01.012
104. Ozdemir O, Ravindranath Y, Savaşan S. Cell-mediated cytotoxicity evaluation using monoclonal antibody staining for target or effector cells with annexinV/propidium iodide colabeling by fluorosphere-adjusted counts on three-color flow cytometry. Cytom A. (2003) 56:53–60. doi: 10.1002/cyto.a.10081
105. Lotzerich H, Hirt W. Flow cytometric non-radioactive method for the determination of the cytotoxic activity of natural killer cells: Comparison with the classical 51Cr-release assay. Laboratoriums Medizin. (1997) 21:13–20.
106. Mata MM, Mahmood F, Sowell RT, Baum LL. Effects of cryopreservation on effector cells for antibody dependent cell-mediated cytotoxicity (ADCC) and natural killer (NK) cell activity in (51)Cr-release and CD107a assays. J Immunol Methods. (2014) 406:1–9. doi: 10.1016/j.jim.2014.01.017
107. Mariani E, Monaco MC, Sgobbi S, de Zwart JF, Mariani AR, Facchini A. Standardization of a micro-cytotoxicity assay for human natural killer cell lytic activity. J Immunol Methods. (1994) 172:173–8. doi: 10.1016/0022-1759(94)90104-X
108. Jang YY, Cho D, Kim SK, Shin DJ, Park MH, Lee JJ, et al. An improved flow cytometry-based natural killer cytotoxicity assay involving calcein AM staining of effector cells. Ann Clin Lab Sci. (2012) 42:42–9.
109. von Zons P, Crowley-Nowick P, Friberg D, Bell M, Koldovsky U, Whiteside TL. Comparison of europium and chromium release assays: cytotoxicity in healthy individuals and patients with cervical carcinoma. Clin Diagn Lab Immunol. (1997) 4:202–7. doi: 10.1128/cdli.4.2.202-207.1997
110. Buller CW, Mathew SO. NK cell isolation and cytotoxicity by radioactive chromium release assay and DELFIA-euTDA cytotoxicity assay. Methods Mol Biol. (2022) 2463:221–33. doi: 10.1007/978-1-0716-2160-8_16
111. Zhang D, Teng R, Lv N, Lei L, Wang Y, Williamson RA, et al. A novel CD2 staining-based flow cytometric assay for assessment of natural killer cell cytotoxicity. J Clin Lab Anal. (2020) 34:e23519. doi: 10.1002/jcla.23519
112. Patel RS, Lucas J, Timmins LM, Mukundan S, Teryek M, Bhatt R, et al. Non-invasive image-based cytometry for high throughput NK cell cytolysis analysis. J Immunol Methods. (2021) 491:112992. doi: 10.1016/j.jim.2021.112992
113. Somanchi SS, McCulley KJ, Somanchi A, Chan LL, Lee DA. A novel method for assessment of natural killer cell cytotoxicity using image cytometry. PloS One. (2015) 10:e0141074. doi: 10.1371/journal.pone.0141074
114. Vanherberghen B, Olofsson PE, Forslund E, Sternberg-Simon M, Khorshidi MA, Pacouret S, et al. Classification of human natural killer cells based on migration behavior and cytotoxic response. Blood. (2013) 121:1326–34. doi: 10.1182/blood-2012-06-439851
115. Guldevall K, Brandt L, Forslund E, Olofsson K, Frisk TW, Olofsson PE, et al. Microchip screening platform for single cell assessment of NK cell cytotoxicity. Front Immunol. (2016) 7. doi: 10.3389/fimmu.2016.00119
116. Subedi N, Van Eyndhoven LC, Hokke AM, Houben L, Van Turnhout MC, Bouten CVC, et al. An automated real-time microfluidic platform to probe single NK cell heterogeneity and cytotoxicity on-chip. Sci Rep. (2021) 11:17084. doi: 10.1038/s41598-021-96609-9
117. Broadbent S, Coutts R. Intermittent and graded exercise effects on NK cell degranulation markers LAMP-1/LAMP-2 and CD8+CD38+ in chronic fatigue syndrome/myalgic encephalomyelitis. Physiol Rep. (2017) 5(5):e13091. doi: 10.14814/phy2.13091
118. Kasatori N, Ishikawa F, Ueyama M, Urayama T. A differential assay of NK-cell-mediated cytotoxicity in K562 cells revealing three sequential membrane impairment steps using three-color flow-cytometry. J Immunol Methods. (2005) 307:41–53. doi: 10.1016/j.jim.2005.09.005
119. Gómez-Mora E, Carrillo J, Urrea V, Rigau J, Alegre J, Cabrera C, et al. Impact of long-term cryopreservation on blood immune cell markers in myalgic encephalomyelitis/chronic fatigue syndrome: implications for biomarker discovery. Front Immunol. (2020) 11:582330. doi: 10.3389/fimmu.2020.582330
120. Martí F, Miralles A, Peiró M, Amill B, de Dalmases C, Piñol G, et al. Differential effect of cryopreservation on natural killer cell and lymphokine-activated killer cell activities. Transfusion (Paris). (1993) 33:651–5. doi: 10.1046/j.1537-2995.1993.33893342746.x
121. Yao X, Matosevic S. Cryopreservation of NK and T cells without DMSO for adoptive cell-based immunotherapy. BioDrugs. (2021) 35:529–45. doi: 10.1007/s40259-021-00494-7
122. Zhang Q, Zhou XD, Denny T, Ottenweller JE, Lange G, LaManca JJ, et al. Changes in immune parameters seen in Gulf War veterans but not in civilians with chronic fatigue syndrome. Clin Diagn Lab Immunol. (1999) 6:6–13. doi: 10.1128/CDLI.6.1.6-13.1999
123. Stewart CC, Cookfair DL, Hovey KM, Wende KE, Bell DS, Warner CL. Predictive immunophenotypes: disease-related profile in chronic fatigue syndrome. Cytom B Clin Cytom. (2003) 53:26–33. doi: 10.1002/cyto.b.10034
124. Robertson MJ, Schacterle RS, Mackin GA, Wilson SN, Bloomingdale KL, Ritz J, et al. Lymphocyte subset differences in patients with chronic fatigue syndrome, multiple sclerosis and major depression. Clin Exp Immunol. (2005) 141:326–32. doi: 10.1111/j.1365-2249.2005.02833.x
125. Smith SL, Kennedy PR, Stacey KB, Worboys JD, Yarwood A, Seo S, et al. Diversity of peripheral blood human NK cells identified by single-cell RNA sequencing. Blood Adv. (2020) 4:1388–406. doi: 10.1182/bloodadvances.2019000699
126. Yang C, Siebert JR, Burns R, Gerbec ZJ, Bonacci B, Rymaszewski A, et al. Heterogeneity of human bone marrow and blood natural killer cells defined by single-cell transcriptome. Nat Commun. (2019) 10:3931. doi: 10.1038/s41467-019-11947-7
127. De Barra C, Khalil M, Mat A, O’Donnell C, Shaamile F, Brennan K, et al. Glucagon-like peptide-1 therapy in people with obesity restores natural killer cell metabolism and effector function. Obes (Silver Spring). (2023) 31(7):1787–97. doi: 10.1002/oby.23772
128. Cabanas H, Muraki K, Eaton-Fitch N, Staines DR, Marshall-Gradisnik S. Potential therapeutic benefit of low dose naltrexone in myalgic encephalomyelitis/chronic fatigue syndrome: role of transient receptor potential melastatin 3 ion channels in pathophysiology and treatment. Front Immunol. (2021) 12:687806. doi: 10.3389/fimmu.2021.687806
129. Cabanas H, Muraki K, Staines D, Marshall-Gradisnik S. Naltrexone restores impaired transient receptor potential melastatin 3 ion channel function in natural killer cells from myalgic encephalomyelitis/chronic fatigue syndrome patients. Front Immunol. (2019) 10:2545. doi: 10.3389/fimmu.2019.02545
130. Levy SM, Herberman RB, Lee J, Whiteside T, Beadle M, Heiden L, et al. Persistently low natural killer cell activity, age, and environmental stress as predictors of infectious morbidity. Nat Immun Cell Growth Regul. (1991) 10:289–307.
131. Kutza J, Murasko DM. Effects of aging on natural killer cell activity and activation by interleukin-2 and IFN-alpha. Cell Immunol. (1994) 155:195–204. doi: 10.1006/cimm.1994.1112
132. Orange JS. Natural killer cell deficiency. J Allergy Clin Immunol. (2013) 132:515–25. doi: 10.1016/j.jaci.2013.07.020
133. Irwin M, Lacher U, Caldwell C. Depression and reduced natural killer cytotoxicity: a longitudinal study of depressed patients and control subjects. Psychol Med. (1992) 22:1045–50. doi: 10.1017/S0033291700038617
134. Evans DL, Folds JD, Petitto JM, Golden RN, Pedersen CA, Corrigan M, et al. Circulating natural killer cell phenotypes in men and women with major depression. Relation to cytotoxic activity and severity of depression. Arch Gen Psychiatry. (1992) 49:388–95. doi: 10.1001/archpsyc.1992.01820050052009
135. Caldwell CL, Irwin M, Lohr J. Reduced natural killer cell cytotoxicity in depression but not in schizophrenia. Biol Psychiatry. (1991) 30:1131–8. doi: 10.1016/0006-3223(91)90183-M
136. Irwin M, Caldwell C, Smith TL, Brown S, Schuckit MA, Gillin JC. Major depressive disorder, alcoholism, and reduced natural killer cell cytotoxicity. Role of severity of depressive symptoms and alcohol consumption. Arch Gen Psychiatry. (1990) 47:713–9. doi: 10.1001/archpsyc.1990.01810200021003
137. Brown AA, Jason LA, Evans MA, Flores S. Contrasting Case Definitions: The ME International Consensus Criteria vs. the Fukuda et al. CFS Criteria. N Am J Psychol. (2013) 15:103–20.
138. Conroy KE, Islam MF, Jason LA. Evaluating case diagnostic criteria for myalgic encephalomyelitis/chronic fatigue syndrome (ME/CFS): toward an empirical case definition. Disabil Rehabil. (2023) 45:840–7. doi: 10.1080/09638288.2022.2043462
139. Reeves WC, Lloyd A, Vernon SD, Klimas N, Jason LA, Bleijenberg G, et al. Identification of ambiguities in the 1994 chronic fatigue syndrome research case definition and recommendations for resolution. BMC Health Serv Res. (2003) 3:25. doi: 10.1186/1472-6963-3-25
140. Jones JF, Lin JMS, Maloney EM, Boneva RS, Nater UM, Unger ER, et al. An evaluation of exclusionary medical/psychiatric conditions in the definition of chronic fatigue syndrome. BMC Med. (2009) 7:57. doi: 10.1186/1741-7015-7-57
141. Reeves WC, Stamey FR, Black JB, Mawle AC, Stewart JA, Pellett PE. Human herpesviruses 6 and 7 in chronic fatigue syndrome: a case-control study. Clin Infect Dis. (2000) 31:48–52. doi: 10.1086/313908
142. Buchwald D, Cheney PR, Peterson DL, Henry B, Wormsley SB, Geiger A, et al. A chronic illness characterized by fatigue, neurologic and immunologic disorders, and active human herpesvirus type 6 infection. Ann Intern Med. (1992) 116:103–13. doi: 10.7326/0003-4819-116-2-103
143. Ablashi DV, Eastman HB, Owen CB, Roman MM, Friedman J, Zabriskie JB, et al. Frequent HHV-6 reactivation in multiple sclerosis (MS) and chronic fatigue syndrome (CFS) patients. J Clin Virol. (2000) 16:179–91. doi: 10.1016/S1386-6532(99)00079-7
144. Chapenko S, Krumina A, Kozireva S, Nora Z, Sultanova A, Viksna L, et al. Activation of human herpesviruses 6 and 7 in patients with chronic fatigue syndrome. J Clin Virol. (2006) 37 Suppl 1:S47–51. doi: 10.1016/S1386-6532(06)70011-7
145. Lynch LA, O’Connell JM, Kwasnik AK, Cawood TJ, O’Farrelly C, O’Shea DB. Are natural killer cells protecting the metabolically healthy obese patient? Obes (Silver Spring). (2009) 17:601–5. doi: 10.1038/oby.2008.565
146. O’Shea D, Hogan AE. Dysregulation of natural killer cells in obesity. Cancers (Basel). (2019) 11(4):573. doi: 10.3390/cancers11040573
147. Viel S, Besson L, Charrier E, Marçais A, Disse E, Bienvenu J, et al. Alteration of Natural Killer cell phenotype and function in obese individuals. Clin Immunol. (2017) 177:12–7. doi: 10.1016/j.clim.2016.01.007
148. Naujoks W, Quandt D, Hauffe A, Kielstein H, Bähr I, Spielmann J. Characterization of surface receptor expression and cytotoxicity of human NK cells and NK cell subsets in overweight and obese humans. Front Immunol. (2020) 11:573200. doi: 10.3389/fimmu.2020.573200
149. Zaiatz-Bittencourt V, Jones F, Tosetto M, Scaife C, Cagney G, Jones E, et al. Butyrate limits human natural killer cell effector function. Sci Rep. (2023) 13:2715. doi: 10.1038/s41598-023-29731-5
150. Michelet X, Dyck L, Hogan A, Loftus RM, Duquette D, Wei K, et al. Metabolic reprogramming of natural killer cells in obesity limits antitumor responses. Nat Immunol. (2018) 19:1330–40. doi: 10.1038/s41590-018-0251-7
151. Sasso EM, Muraki K, Eaton-Fitch N, Smith P, Lesslar OL, Deed G, et al. Transient receptor potential melastatin 3 dysfunction in post COVID-19 condition and myalgic encephalomyelitis/chronic fatigue syndrome patients. Mol Med. (2022) 28:98. doi: 10.1186/s10020-022-00528-y
152. Whistler T, Fletcher MA, Lonergan W, Zeng XR, Lin JM, Laperriere A, et al. Impaired immune function in Gulf War Illness. BMC Med Genomics. (2009) 2:12. doi: 10.1186/1755-8794-2-12
Keywords: meta-analysis, natural killer cells, cytotoxicity, myalgic encephalomyelitis/chronic fatigue syndrome, ME/CFS
Citation: Baraniuk JN, Eaton-Fitch N and Marshall-Gradisnik S (2024) Meta-analysis of natural killer cell cytotoxicity in myalgic encephalomyelitis/chronic fatigue syndrome. Front. Immunol. 15:1440643. doi: 10.3389/fimmu.2024.1440643
Received: 29 May 2024; Accepted: 09 September 2024;
Published: 17 October 2024.
Edited by:
Vincent C. Lombardi, University of Nevada, United StatesReviewed by:
Pawel Zalewski, Nicolaus Copernicus University in Toruń, PolandMaureen R. Hanson, Cornell University, United States
Copyright © 2024 Baraniuk, Eaton-Fitch and Marshall-Gradisnik. This is an open-access article distributed under the terms of the Creative Commons Attribution License (CC BY). The use, distribution or reproduction in other forums is permitted, provided the original author(s) and the copyright owner(s) are credited and that the original publication in this journal is cited, in accordance with accepted academic practice. No use, distribution or reproduction is permitted which does not comply with these terms.
*Correspondence: James N. Baraniuk, baraniuj@georgetown.edu