- 1Institute of Immunology/Molecular Pathogenesis, Center for Biotechnology and Biomedicine, Faculty of Veterinary Medicine, Leipzig University, Leipzig, Germany
- 2Department of Veterinary Pathology, Microbiology and Immunology, School of Veterinary Medicine, University of California, Davis, Davis, CA, United States
Conventional CD4pos regulatory T (Treg) cells characterized by expression of the key transcription factor forkhead box P3 (FoxP3) are crucial to control immune responses, thereby maintaining homeostasis and self-tolerance. Within the substantial population of non-conventional T cell receptor (TCR)αβpos CD4negCD8αneg double-negative (dn) T cells of dogs, a novel FoxP3pos Treg-like subset was described that, similar to conventional CD4pos Treg cells, is characterized by high expression of CD25. Noteworthy, human and murine TCRαβpos regulatory dn T cells lack FoxP3. Immunosuppressive capacity of canine dn T cells was hypothesized based on expression of inhibitory molecules (interleukin (IL)-10, cytotoxic T-lymphocyte associated protein 4, CTLA4). Here, to verify their regulatory function, the dnCD25pos (enriched for FoxP3pos Treg-like cells) and the dnCD25neg fraction, were isolated by fluorescence-activated cell sorting from peripheral blood mononuclear cells (PBMC) of Beagle dogs and analyzed in an in vitro suppression assay in comparison to conventional CD4posCD25pos Treg cells (positive control) and CD4posCD25neg T cells (negative control). Canine dnCD25pos T cells suppressed the Concanavalin A-driven proliferation of responder PBMC to a similar extent as conventional CD4posCD25pos Treg cells. Albeit to a lesser extent than FoxP3-enriched dn and CD4posCD25pos populations, even dnCD25neg T cells reduced the proliferation of responder cells. This is remarkable, as dnCD25neg T cells have a FoxP3neg phenotype comparable to non-suppressive CD4posCD25neg T cells. Both, CD25pos and CD25neg dn T cells, can mediate suppression independent of cell-cell contact and do not require additional signals from CD4posCD25neg T cells to secrete inhibitory factors in contrast to CD4posCD25pos T cells. Neutralization of IL-10 completely abrogated the suppression by dnCD25pos and CD4posCD25pos Treg cells in a Transwell™ system, while it only partially reduced suppression by dnCD25neg T cells. Taken together, unique canine non-conventional dnCD25pos FoxP3pos Treg-like cells are potent suppressor cells in vitro. Moreover, inhibition of proliferation of responder T cells by the dnCD25neg fraction indicates suppressive function of a subset of dn T cells even in the absence of FoxP3. The identification of unique immunoregulatory non-conventional dn T cell subpopulations of the dog in vitro is of high relevance, given the immunotherapeutic potential of manipulating regulatory T cell responses in vivo.
1 Introduction
The dog is not just a popular companion animal, but also an attractive model in immunological research. Autoimmune disorders (1), allergies (2, 3), and cancer (4, 5) regularly observed in dogs show similarities to these complex diseases in humans. Therefore, studies of pathogenesis mechanisms in dogs are not only valuable for the pet species itself but can also be useful in translational approaches to draw conclusions for human patients. In that regard, it is essential to intensify basic research on the canine immune system that is still poorly understood. Given the central role of T cells in immunity, a detailed knowledge of canine T cells can provide new insights into disease development and reveal new targets for therapies which could be eventually tested in this model species.
Across species, conventional T cells in the periphery express the T cell receptor (TCR)αβ and either CD8 or CD4 as a co-receptor. CD8 exists as CD8αα homodimer or as CD8αβ heterodimer, the latter being characteristic for conventional cytotoxic T cells (6). CD4 co-receptor expression is associated with T helper or regulatory T (Treg) cell function (7).
Besides conventional TCRαβ+ CD8 single-positive (sp) and CD4 sp T cells, a small population (1–3%) of non-conventional TCRαβ+ T cells that lack expression of both, CD4 and CD8, i.e. TCRαβpos CD4neg CD8neg double-negative (dn) T cells have been described in mice and humans (8–10). Of note, in dogs non-conventional TCRαβpos CD4negCD8αneg dn T cells constitute a large population of up to 15% of peripheral lymphocytes (11) raising the question regarding their function.
Conventional CD4pos Treg cells defined by expression of the master transcription factor forkhead box P3 (FoxP3) are key players for maintenance of immune homeostasis and peripheral tolerance by preventing aberrant activation of the immune system (12–14). Treg cell dysfunction is associated with several common autoimmune disorders including multiple sclerosis, type 1 diabetes, rheumatoid arthritis, and systemic lupus erythematosus in humans (14). Furthermore, Treg cells have been implicated in allergic diseases and play a pivotal role in the maintenance of allograft tolerance (15, 16). Conventional CD4pos FoxP3pos Treg cells have also been described and functionally characterized in the dog (17, 18).
Recently, we identified a novel non-conventional FoxP3pos Treg-like subset within the substantial population of canine CD4negCD8αneg dn T cells. Similar to conventional Treg cells, Treg-like dn T cells are characterized by high expression of the surface molecule CD25 (11). Potential immunosuppressive capacity of canine dn T cells was also indicated by their high stimulation-induced transcription of the co-inhibitory receptor cytotoxic T-lymphocyte associated protein 4 (CTLA4) and production of the inhibitory cytokine interleukin (IL)-10, as published by our group (19). The majority of IL-10-expressing dn T cells were CD25pos, corresponding to a T regulatory phenotype (19). Although a possible immunosuppressive function of FoxP3pos dn T cells in the dog has been discussed, it has not yet been analyzed (17). Of note, allergen desensitization of dogs with adverse food reactions leads to an increase of canine dn T cells, supporting a potential regulatory role of canine dn T cells in vivo (20). However, dn T cells were not further characterized e.g. by expression of FoxP3 in this study.
Despite lacking FoxP3 expression (9, 10, 21–23), the small population of human and murine TCRαβpos dn T cells has been demonstrated to play an important role in down-regulating immune responses both in vitro and in vivo (24–29). In this context dn regulatory T cells of mice were able to prevent allograft rejection (9, 30), auto-immune diabetes (22, 31–34), or allergic asthma (35). Furthermore, a protective role of both, murine and human TCRαβpos dn T cells was shown in graft-versus-host disease (36–39). Based on recent data, it was speculated that dogs may have non-conventional FoxP3pos and/or FoxP3neg dn T cells with immunosuppressive function (19). To verify the hypothesis of a regulatory function of canine dn T cells in the present study, sorted CD25pos and dnCD25neg populations were assessed in in vitro suppression assays. We demonstrate that FoxP3-enriched dnCD25pos T cells suppress proliferation of canine autologous responder peripheral blood mononuclear cells (PBMC) to a similar extent as conventional CD4pos Treg cells. Furthermore, dnCD25neg T cells but not conventional CD4posCD25neg T cells can suppress proliferation of PBMC responder populations. Non-conventional dnCD25pos and dnCD25neg T cells are able to mediate suppression via the secretion of soluble factors, with a central role of IL-10 in the former. In contrast to conventional CD4pos Treg cells, non-conventional dn T cells do not require signals from co-cultured CD4posCD25neg T cells to exert their regulatory function in a Transwell™ system in vitro.
Taken together, our data prove existence of unique and potent suppressive subpopulations of non-conventional dn T cells in dogs that might play a crucial role in immune regulation in vivo.
2 Materials and methods
2.1 Dogs, blood sample collection
Peripheral blood samples were taken from healthy adult Beagle dogs via the lateral saphenous vein into heparinized vacutainer tubes (BD Vacutainer, 10ml, Li-Heparin 17 IU/ml, Becton Dickinson, Heidelberg, Germany). All laboratory dogs (5 male, 4 female, aged 2 - 8 years) belonged to the Faculty of Veterinary Medicine, Leipzig University, Germany, at the time of blood sampling. The number of dogs used for individual analyses is indicated in the figure legends. Dogs were vaccinated against common pathogens and prophylactically treated against endo- and ectoparasites on a regular basis. The study was authorized by the Animal Care and Usage Committee of the Saxony State Office, Leipzig, Germany (approval number: DD24.1–5131/444/30).
2.2 Isolation of canine peripheral blood mononuclear cells
Peripheral blood mononuclear cells (PBMC) were isolated from fresh whole blood by density gradient centrifugation within one hour after blood sampling. Briefly, blood was diluted with phosphate buffered saline (PBS) (ratio 1:1), layered above Pancoll® Separating Solution (density 1.077 g/ml, PAN-Biotech, Aidenbach, Germany) and centrifuged (500g, 30 min at room temperature (RT) minimal acceleration and deceleration). Mononuclear cells from the interphase were collected and washed twice in PBS (500g, 15 min, RT). Cells were incubated for 5 min at RT in erythrocyte lysis buffer (150 mM NH4Cl, 8 mM KHCO3, 2 mM EDTA, pH 7.0). PBS with 3% fetal bovine serum (FBS, PAN-Biotech) was added to stop the reaction and remaining cells were washed again with PBS. The final cell number was determined using a Neubauer chamber (Laboroptik Lancing, UK), excluding dead cells by identification via trypan blue staining (Merck-Sigma, Darmstadt, Germany). PBMC were either used immediately for subsequent assays or were cryo-preserved in liquid nitrogen (in FBS + 10% DMSO) until further usage and used after thawing and washing in cell culture medium (IMDM with L-Glutamine, 25 mM HEPES and 3.024 g/L NaHCO3 (PAN-Biotech) supplemented with 100 U/ml penicillin, 100 μg/ml streptomycin (both purchased from PAA Laboratories, Cölbe, Germany), and 10% FBS).
A part of the isolated PBMC from each individual dog was labeled with Cell Proliferation Dye eFluor™ 450 (Thermo Fisher Scientific, Carlsbad, USA) according to the manufacturer’s protocol. These proliferation dye-labeled PBMC were used as responder (Resp) cells in in vitro suppression assays (see below).
2.3 Sort of effector T cell subpopulations
Remaining PBMC (see 2.2) from each individual dog were used to isolate effector T cell populations (Teff) by fluorescence-activated cell sorting (FACS). All incubation steps described below were performed for 15–20 min on ice in the dark and separated by washing steps with PBS, 3% FBS (500g, 3 min, 4°C). For discrimination between viable and dead cells, a fixable viability dye (eFluor 506, Thermo Fisher Scientific) was applied following the manufacturer’s protocol. Detailed information on the primary antibodies used for flow cytometric staining is summarized in Table 1. To avoid binding by Fc receptors, cells were incubated with heat-inactivated serum derived from dog and rat (15% each in PBS). Then, cells were incubated with anti-canine TCRαβ (clone CA15.8G7) hybridoma supernatant. A PerCP/Cy5.5-conjugated goat-anti-mouse IgG secondary antibody (Biolegend, San Diego, USA) was used for detection. In a next step, cells were incubated with a mixture of the remaining cell surface antibodies, listed in Table 1. Stained cells were passed over a 30 µm filter (Sysmex Germany GmbH, Norderstedt, Germany) and immediately sorted using a BD FACSAria™ III Cell Sorter equipped with BD FACS Diva ™ software version 6.1.3 (Becton Dickinson).
2.4 Analysis of sorted T cell subpopulations
The portion of FoxP3pos cells within each sorted Teff population was determined using the FoxP3/Transcription Factor Staining Buffer Set (Thermo Fisher Scientific) according to the manufacturer’s protocol. After fixation and permeabilization, a mixture of heat-inactivated dog, rat and mouse normal serum (15% each in permeabilization buffer) was added to block binding by Fc receptors and cells were stained with the anti-canine FoxP3 antibody in permeabilzation buffer (Thermo Fisher Scientific) for 30 minutes at RT in the dark. Data were acquired with a BD LSR Fortessa™ Cell Analyzer equipped with FACS Diva™ software version 6.1.3 (Becton Dickinson) and assessed using the FlowJo 10™ software (Treestar Inc.).
2.5 In vitro suppression assay
Resp cells and sorted Teff cells were resuspended in cell culture medium and final cell numbers were determined using a Neubauer chamber, taking into account cell loss through FACS-sorting and staining procedures. 5x104 Resp cells were seeded into 96-well round bottom cell culture plates (Techno Plastic Products AG, Trasadingen, Switzerland) and stimulated with 0.5–1.0 µg/ml Concanavalin A (ConA) (Roth, Karlsruhe, Germany) to reach a proliferation rate of >25% that enables assessment of suppressive effects by Teff cells. For analysis of their suppressive capacity, the different autologous sorted Teff cells were added to proliferation dye-labelled Resp cells at a ratio of 1:1. Cells were incubated for 72 h at 37°C, 5% CO2 in a total volume of 200 µl culture medium. Resp cells alone incubated with medium or stimulated with ConA in parallel served as controls.
To allow for analysis of different T responder cell (Tresp) populations, the mixed cells were harvested after three days of culture, and re-stained for TCRαβ, CD4, and CD8α. Briefly, after washing, LIVE/DEAD™ Fixable Orange (602) Viability Kit (Thermo Fisher Scientific) was used following the manufacturer’s protocol. Staining for surface antibodies, data acquisition and analysis were performed as described above.
The suppressive effect of purified Teff cells on the proliferation of the different responder T cell populations was normalized to ConA-stimulated Tresp only and calculated as follows (18):
2.6 In vitro Transwell™ suppression assay
Transwell™ experiments were performed in 96-well plates (Corning Incorporated Costar, Tewksbury, USA) to determine if cell-cell contact is required to mediate suppressive effects. As described for co-culture suppression assays, proliferation dye-labeled Resp cells were seeded at a density of 5x104 cells in the bottom chamber of the Transwell™ system and stimulated with 0.4 µg/ml ConA. Cells assayed for their regulatory capacity (Teff) were cultured at an equal ratio in the top chamber, separated from Tresp via a 0.4 µm pore polycarbonate membrane. Teff cells were either cultured alone in the Transwell™ insert, or co-cultured with CD4posCD25neg cells at a ratio of 1:1 in order to compare suppressive effects with and without contact to CD4posCD25neg cells. To analyze whether IL-10 is involved in cell-cell contact independent suppression, a neutralizing anti-canine IL-10 antibody (clone 138128, R&D, Biotechne, Wiesbaden, Germany) was used at 10 µg/ml. A nonblocking mouse IgG1 antibody (clone 11711, R&D, Biotechne, Wiesbaden, Germany) was added as control to rule out unspecific effects of the neutralizing antibody. Similar to the standard co-culture suppression assay, cells were incubated for 72 h at 37°C, 5% CO2. Analysis of suppression was performed as described above.
2.7 Statistical analysis
The Graph Pad Prism10 software (GraphPad Software Inc., San Diego, USA) was used for statistical analysis and for graphical presentation of the data. The Shapiro-Wilk test was applied to test for normal distribution. Normally distributed data are presented with means, and were analyzed via One-way ANOVA with Bonferroni post hoc test. Non-parametric data are depicted with medians, and were compared by Kruskal-Wallis test with Dunn’s post test. (* p< 0.05; ** p< 0.01, *** p< 0.001). Significance of antibody-dependent effects (anti-IL-10 or isotype control) on suppression was calculated by direct comparison with the corresponding control without antibody addition using the Mann-Whitney test (two-tailed), (* p< 0.05).
3 Results
3.1 Canine non-conventional TCRαβpos CD4negCD8αneg double-negative (dn)CD25pos T cells are potent suppressors in vitro, and even dnCD25neg T cells act suppressive
To assess their suppressive function in vitro, TCRαβpos dnCD25pos and dnCD25neg subpopulations were isolated by fluorescence-activated cell sorting from peripheral blood of healthy Beagle dogs with high purity (>98.0%) (Figure 1A). Conventional CD4posCD25pos Treg and CD4posCD25neg cells were sorted as positive and negative control, respectively (17, 18) (Figure 1A). CD25pos and CD25neg T cell subpopulations were defined by use of a control staining including all antibodies except for CD25 which was replaced by its isotype control (Supplementary Figure 1). Consistent with our previous results, non-conventional TCRαβpos dn T cells contained an approximately three times higher proportion of CD25pos cells than their conventional CD4pos counterparts [Figure 1A (11)]. As expected, FoxP3pos cells were enriched in both, the conventional CD4posCD25pos fraction [median 30% FoxP3pos (17)] and the dnCD25pos fraction [median 18% FoxP3pos (11)], as shown by post-sort re-analysis (Figures 1B, C). In contrast, a comparable, mainly FoxP3neg phenotype was observed in sorted CD4posCD25neg and dnCD25neg populations (median<3% FoxP3pos). Interestingly, post-sort re-analysis revealed a significantly lower expression of TCRαβ in both non-conventional dn T cell subpopulations, compared to their conventional CD4pos counterparts (Supplementary Figure 2). The sorted T effector (Teff) cell populations’ regulatory capacities were assessed in an in vitro suppression assay. Autologous proliferation dye-labeled responder (Resp) PBMC were stimulated with ConA for three days in the presence or absence of sorted Teff cells (Figure 2A). Suppressive effects on all TCRαβpos T (Tresp) cells (Figure 2B) and individual Tresp cell subpopulations, i.e. CD4 single-positive (sp), CD8α sp and dn Tresp cells (Figure 2C) were analyzed (see Supplementary Figure 3 for detailed gating strategy). The proliferative response was similar when responder cells were gated for all viable lymphocytes or the dominating TCRαβpos population (Supplementary Figure 4). Noteworthy, while ConA induced strong proliferation of CD4 sp and CD8α sp Tresp cells, the proliferation rate of non-conventional dn Tresp cells was very low (Figure 2C) and did not allow for reliable quantification of suppressive effects of Teff cells in this subpopulation.
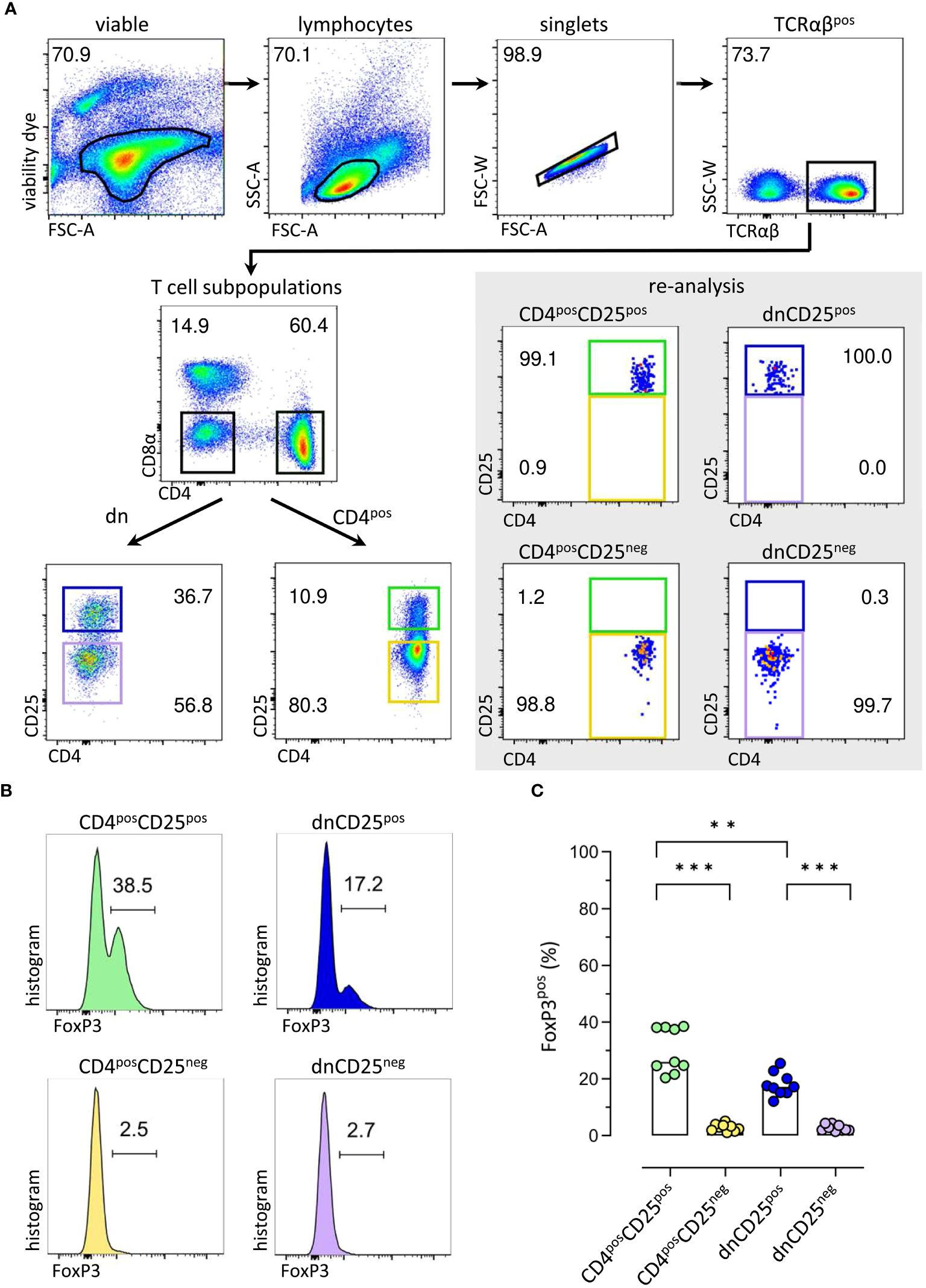
Figure 1 Sorting of canine T effector (Teff) cells for comparative analysis of conventional and non-conventional populations in an in vitro suppression assay results in enrichment of FoxP3pos cells in CD4posCD25pos and CD4negCD8αneg double-negative (dn)CD25pos TCRαβpos T cell fractions. (A) The strategy for isolation of different Teff cell populations from canine peripheral blood mononuclear cells (PBMC) by fluorescence-activated cell sorting is shown. After gating on single viable TCRαβpos lymphocytes, conventional CD4pos single-positive and non-conventional CD4negCD8αneg double-negative (dn) T cells were further divided according to CD25 expression to obtain four Teff populations: CD4posCD25pos, CD4posCD25neg, dnCD25pos, dnCD25neg. High purity (>98%) of the isolated populations was confirmed by re-analysis. Representative data of one dog are depicted. Gated populations’ percentages are presented as numbers in the plots. (B, C) FoxP3 expression in sorted Teff populations demonstrates that FoxP3pos cells are enriched in the CD4posCD25pos and dnCD25pos Teff populations. (B) Representative flow cytometric plots show the expression of FoxP3 (%) in the sorted Teff populations indicated. Gating was performed using CD8αpos T cells as internal control. (C) Quantification of the frequencies of FoxP3pos cells in the different sorted Teff populations. Each dot represents one individual dog analysed in independent experiments. Bars represent medians. Statistical analysis was done by Kruskal-Wallis test with Dunn’s post-test (** p< 0.01, *** p<0.001).
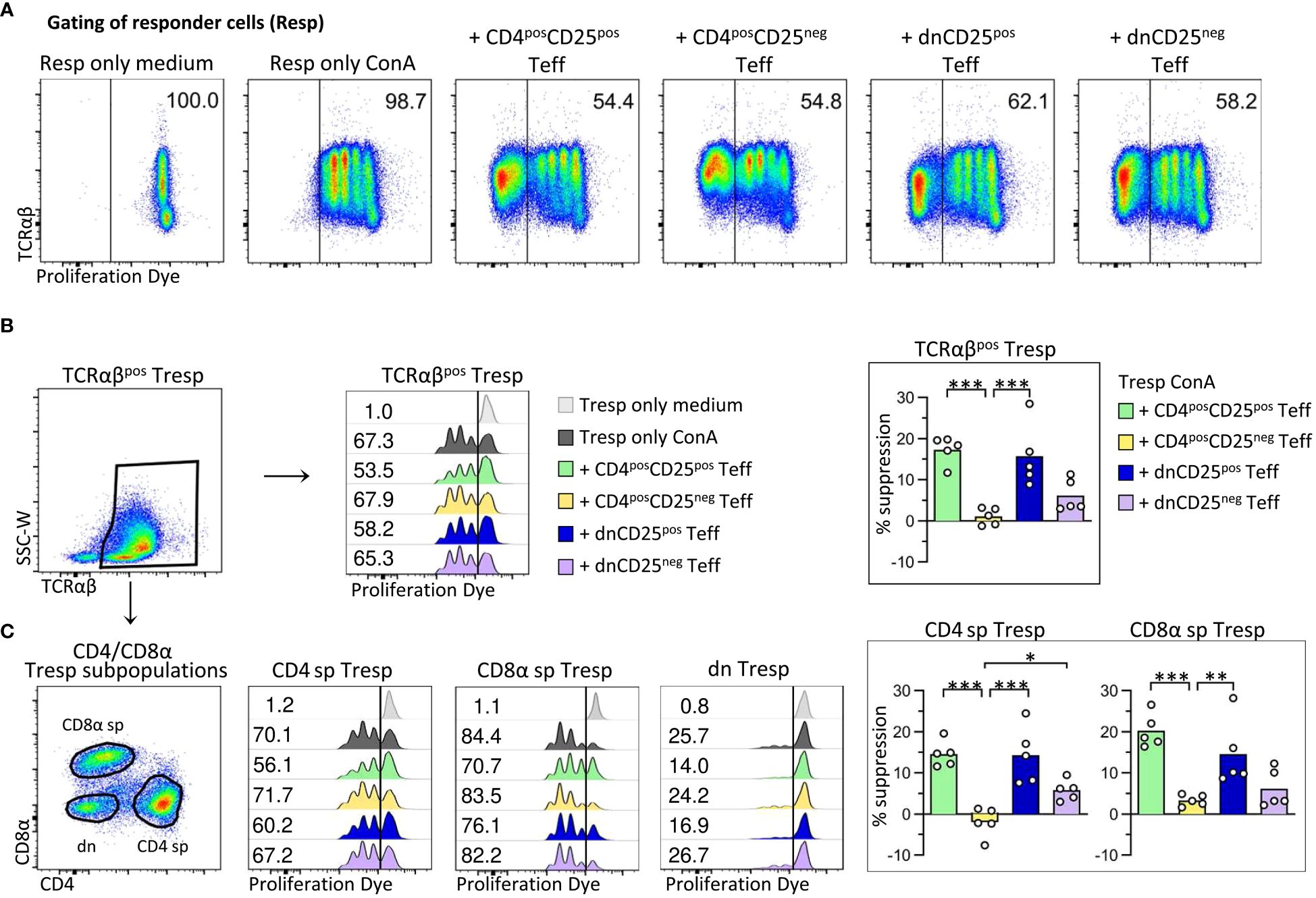
Figure 2 Canine non-conventional FoxP3-enriched CD4negCD8aneg double-negative (dn)CD25pos T cells, and, to a lesser extent, dnCD25neg T cells suppress the proliferation of autologous responder PBMC in vitro. Proliferation dye-labelled responder PBMC (Resp) were co-cultured with indicated sorted effector T cell (Teff) populations (CD4posCD25pos, CD4posCD25neg, dnCD25pos, dnCD25neg) at an effector: responder ratio of 1:1 and stimulated with Concanavalin A (ConA). Responder cells cultured alone under medium or ConA conditions served as controls. After three days of culture, the proliferation of different responder T cell populations was evaluated by flow cytometry and the suppressive effect of Teff cells was calculated. (A) From viable single lymphocytes gated as shown in Figure 1A, proliferation dye-negative Teff cells were excluded and responder cells (Resp) selected for analysis of proliferation. The gate was set based on ConA-stimulated responder cells cultured alone (Resp only ConA). Representative data of one dog are shown with the frequency of responder cells under all conditions. (B, C) Whole PBMC as responder cells enabled analysis of suppressive effects of Teff cells on the proliferation of different responder cell populations, i.e. (B) all TCRαβpos responder cells (Tresp), and (C) TCRαβpos CD4pos (CD4 sp) and CD8αpos (CD8α sp) single-positive as well as CD4negCD8αneg double-negative (dn) Tresp subpopulations. (detailed gating strategy in Supplementary Figure 3 for all co-culture conditions) (B, C) Flow cytometric histograms of one representative dog show the proliferation frequencies of indicated Tresp populations, co-cultured with indicated Teff cell population (see color code). The gate was set according to the unstimulated negative control (Resp only medium). Note that the low proliferation rate of dn Tresp upon ConA stimulation did not allow reliable quantification of suppressive effects on these. But suppression was quantified for TCRαβpos Tresp (B) as well as for CD4 sp and CD8α sp Tresp (C). Summary bar graphs (B, C) show the percent suppression of proliferation of the indicated Tresp population, mediated by the different Teff cell population (see color code). The percentage of suppression was normalized to ConA-stimulated Tresp only and calculated as follows: (% of proliferated “Tresp only ConA” − % of proliferated “Tresp ConA co-cultured with indicated Teff”)/(% of proliferated “Tresp only ConA”) × 100. Each dot represents one individual dog analysed in independent experiments. Data are presented with the mean and statistical analysis was performed using One-way ANOVA with Bonferroni’s post-test (* p< 0.05; ** p< 0.01, *** p< 0.001). (Supplementary Figure 4 shows suppressive effects mediated by different Teff cell populations on TCRαβpos Tresp, compared to all viable lymphocytes as responder cells (Resp).).
As expected (17, 18), canine conventional CD4posCD25pos Treg cells suppressed proliferation of autologous CD4 sp Tresp cells (mean suppression 15%) (Figures 2B, C). Furthermore, proliferation of CD8αpos Tresp cells was suppressed by 20% in the presence of CD4posCD25pos Teff cells, expanding the knowledge on target cells of canine conventional CD4pos Treg cells.
Importantly, canine non-conventional TCRαβpos dnCD25pos Teff cells suppressed the ConA-driven proliferation of Tresp cells to a similar extent as conventional CD4posCD25pos Treg cells (mean suppression 15%) (Figures 2B, C). DnCD25pos-mediated suppression of proliferation was observed for TCRαβpos CD4pos Tresp and TCRαβpos CD8αpos Tresp cells (Figure 2C).
Remarkably, despite having a comparable FoxP3neg phenotype as non-suppressive CD4posCD25neg T cells (Figures 1B, C), dnCD25neg Teff cells also reduced proliferation of Tresp cells (mean suppression 6%), albeit to a lesser extent than the FoxP3-enriched dnCD25pos and CD4posCD25pos populations (Figures 2B, C).
Taken together, these findings clearly indicate that, besides conventional CD4posCD25pos Treg cells, the canine immune system contains circulating non-conventional immunoregulatory TCRαβpos dn T cell subpopulations, i.e. FoxP3-enriched dnCD25pos T cells, and, less suppressive, dnCD25neg T cells.
3.2 Canine non-conventional TCRαβpos CD4negCD8αneg double-negative (dn) T cells secrete inhibitory molecules in a cell-cell contact independent manner
Next, we were interested in the mechanisms by which TCRαβpos dn T cells suppress Resp cells’ proliferation. We first asked whether the observed suppression depended on cell-cell contact and/or secretion of soluble factors. Transwell™ suppression assays, in which Teff cells are separated from Resp cells by a semi-permeable membrane that prevents cell-cell contact, while diffusion of soluble factors is maintained (Figure 3A, upper panel), are a suitable method to address this question (44–46).
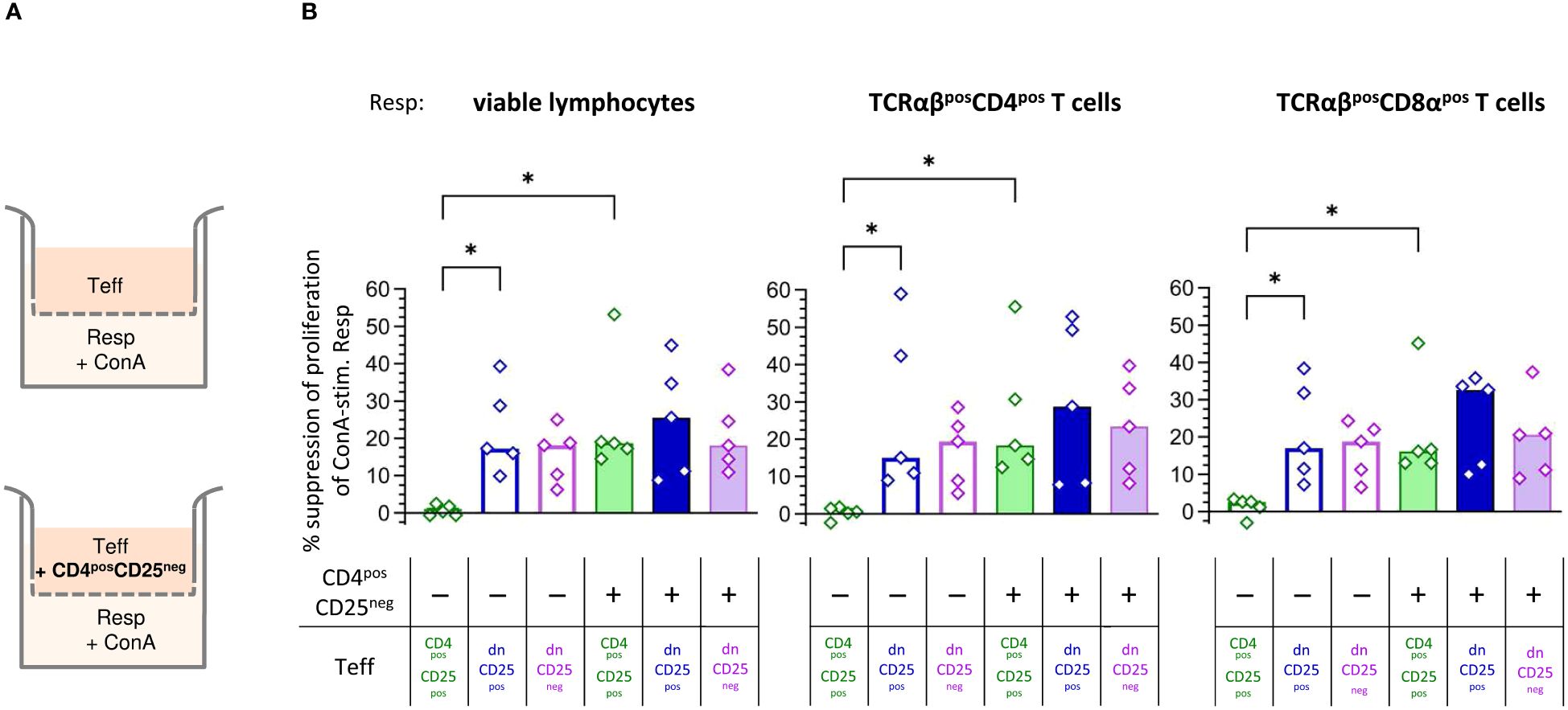
Figure 3 Canine non-conventional CD4negCD8aneg double-negative (dn)CD25pos and dnCD25neg T cells suppress responder cells independent of cell-cell contact in vitro. (A) Experimental setup of the suppression assay in the Transwell™ system (schematic): indicated effector T (Teff) cell populations, assayed for their suppressive capacity, were cultured in the top well of a 96-well Transwell™ plate and separated from ConA-stimulated proliferation dye-labelled responder cells (Resp) in the bottom chamber by a 0.4 µm semi-permeable membrane that only allows soluble factors to pass through. Teff cells in the top chamber were cultured as separate Teff, or co-cultured with the same number of CD4posCD25neg cells (indicated ‘+’) to test for the requirement of cell-cell contact to induce production of suppressive soluble factors in the different Teff cell populations (CD4posCD25pos, dnCD25pos, dnCD25neg). After three days of incubation, Resp cells were harvested from the bottom chamber and analysed by flow cytometry. (B) The suppression of proliferation of Resp cells, mediated by CD4posCD25pos, dnCD25pos and dnCD25neg Teff cells (see color code) with/without contact to CD4posCD25neg T cells was calculated by normalization to the respective negative control (i.e. stimulated Resp, that were cultured under separation from non-suppressive CD4posCD25neg Teff cells by the Transwell™ membrane). The suppressive effects on viable lymphocytes, TCRαβpos CD4pos and TCRαβpos CD8α populations are shown. Each dot represents cells from one individual dog analysed in five independent experiments, bars represent medians. Statistical analysis was performed using Kruskal-Wallis test with Dunn’s post test. (* p< 0.05).
Human, murine and porcine conventional CD4posCD25pos Treg cells do not suppress proliferation of Resp cells when physically separated (45–52). Similarly, when cultured alone in the Transwell™ insert, suppressive capacity of canine CD4posCD25pos Teff cells was completely abolished (Figure 3B). In striking contrast, both dnCD25pos and dnCD25neg Teff populations mediated suppression independent of cell-cell contact in the Transwell™ system (Figure 3B). Secretion of suppressive molecules by murine conventional CD4posCD25pos Treg cells is dependent on co-culture with other cells, such as CD4posCD25neg cells (44). To investigate the impact of signals from other cells on the suppressive capacity of the Teff cells by secretion of inhibitory factors, they were cultured in the upper chamber in presence of non-suppressive CD4posCD25neg T cells (Figure 3A, lower panel). Indeed, the addition of CD4posCD25neg T cells (+ columns in Figure 3B) significantly restored suppression by CD4posCD25pos Teff cells in the Transwell™ system (Figure 3B). Thus, secretion of suppressive soluble factors by canine conventional CD4posCD25pos Treg cells depends on activation signals from other cells in close proximity. In contrast, contact to CD4posCD25neg cells in the Transwell™ system did not significantly increase suppressive effects of dnCD25pos and dnCD25neg Teff cells showing intrinsic capacity to secrete inhibitory molecules (Figure 3B).
Taken together, canine dnCD25pos and dnCD25neg can mediate suppression independent of cell-cell contact via secretion of inhibitory factors. Induction of suppression mediated by both non-conventional dn Teff cell populations clearly differs from that of conventional CD4posCD25pos Tregs, as, in contrast to the latter, they do not require interaction with co-cultured CD4posCD25neg T cells to secrete suppressive soluble factors in the Transwell™ system.
3.3 IL-10 is an important suppressive mediator of canine non-conventional CD4negCD8αneg double-negative (dn)CD25pos and conventional CD4posCD25pos T cells in the Transwell™ system
Non-conventional TCRαβpos dn T cells of the dog are potent IL-10 producers in vitro, similar to their CD4pos counterparts (19). To determine whether IL-10 plays a role for cell-contact independent suppression of proliferation mediated by the non-conventional dn T cell subpopulations as well as conventional CD4posCD25pos T cells, a neutralizing anti-IL-10 antibody was added to the upper chamber of the Transwell™ system. A non-neutralizing isotype control antibody was used for control. As CD4posCD25pos T cells need contact to CD4posCD25neg T cells to secrete inhibitory factors (Figure 3B), we decided to use the experimental set-up including these together with the Teff cells (compare lower panel of Figure 3A). Of note, the addition of the neutralizing anti-IL-10 antibody completely abrogated suppression by dnCD25pos Teff cells in the Transwell™ system, while their suppressive activity was unaltered in the presence of the isotype control antibody (Figure 4). Similar results were obtained for CD4posCD25pos Teff cells. The addition of the neutralizing anti-IL-10 antibody specifically abolished suppression mediated across the semi-permeable membrane (Figure 4). Taken together, these data clearly show that IL-10 is necessary to mediate suppression by canine dnCD25pos and CD4posCD25pos Teff cells in the Transwell™ system. Although a tendency towards reduced suppressive effects of dnCD25neg Teff cells on TCRαβpos CD8αpos Tresp cells was observed, neutralization of IL-10 did not significantly reduce suppression by dnCD25neg Teff cells (Figure 4), indicating that other soluble suppressive mediators may be secreted by this non-conventional subpopulation.
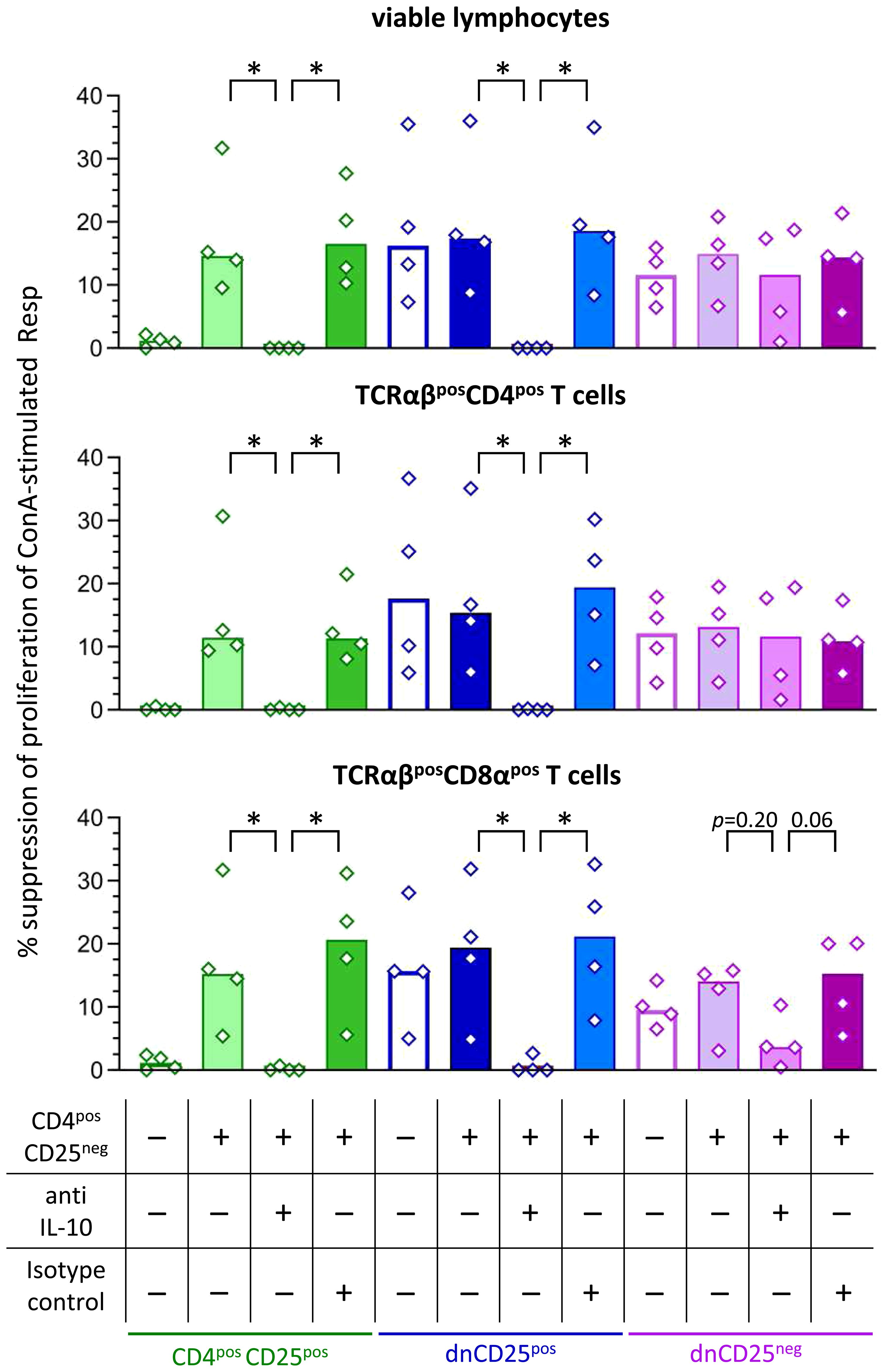
Figure 4 Secretion of IL-10 by canine dnCD25pos and CD4posCD25pos Teff cells is necessary to mediate suppression in the Transwell™ system. Suppression assays were performed in the Transwell™ system as described in Figure 3A. Proliferation dye-labeled ConA-stimulated responder (Resp) cells were cultured in the bottom well, separated by a semipermeable membrane from indicated Teff cell populations in the top well. Where indicated, CD4posCD25neg cells were added at a ratio of 1:1 to Teff cells (top well). The IL-10-dependency of suppression was assessed by addition of a neutralizing anti-IL-10 antibody in comparison to a non-blocking isotype control antibody (see table). Resp cells were harvested after 72 h and analysed by flow cytometry. Summary bar graphs show the median suppression of proliferation of viable lymphocytes, TCRαβpos CD4pos and TCRαβpos CD8αpos Tresp mediated by the different Teff cell population under each condition (see table). The percentage of suppression was normalized to the negative control (ConA stimulated Resp + CD4posCD25neg Teff) of each condition (+/- CD4posCD25neg, +/- anti IL-10 antibody, +/- isotype control). In case addition of neutralizing IL-10 antibody led to negative values for suppression (i.e. ablation of suppression), values are depicted as 0% suppression. Notably, neutralization of IL-10 completely abrogates the suppression by dnCD25pos and CD4posCD25pos Teff cells in the Transwell™ system, while it only partially reduces the suppression by dnCD25neg Teff cells. The results of three independent experiments are shown. Each dot represents one individual dog, bars represent medians. Significance of antibody-dependent effects (anti-IL-10) on suppression was calculated by direct comparison with the corresponding controls (i.e. control without antibody addition and isotype control) using the Mann-Whitney test (two-tailed). (* p < 0.05).
4 Discussion
The role of conventional CD4posFoxP3pos Treg cells is well recognized as guardians of the immune system, to maintain tolerance, and to prevent autoimmunity (53–55). Furthermore, human and murine non-conventional TCRαβpos CD4negCD8αneg dn T cells have been shown to be suppressive and play an important role in down-regulation of immune responses both in vitro and in vivo (9, 21, 24–29).
The relatively rare TCRαβpos dn T cells of men and mice are universally FoxP3neg (9, 10, 21–23), but dogs’ PBMC contain a substantial population of TCRαβpos dn T cells including a subset expressing FoxP3 (11). Immunosuppressive capacity of canine dn T cells was hypothesized based on high stimulation-induced upregulation of inhibitory molecules, including IL-10 (19).
Using an in vitro suppression assay here, we proved the existence of unique immunoregulatory TCRαβpos dn T cell subpopulations of the dog, which clearly differ from their murine and human counterparts (Table 2). We demonstrated that canine FoxP3-enriched dnCD25pos T cells suppress the ConA-driven proliferation of responder cells to a similar extent as conventional CD4pos Treg cells in vitro. Albeit to a lesser extent, dnCD25neg T cells are also suppressive despite a FoxP3neg phenotype comparable to non-suppressive CD4posCD25neg T cells. Irrespective of this similarity to murine and human FoxP3neg dn T cells, canine dnCD25neg T cells were found to be distinct in terms of activation requirements and suppressive mechanisms. Table 2 summarizes functional similarities and differences between human, murine and canine non-conventional TCRαβpos dn T cells, as well as conventional CD4pos Treg cells.
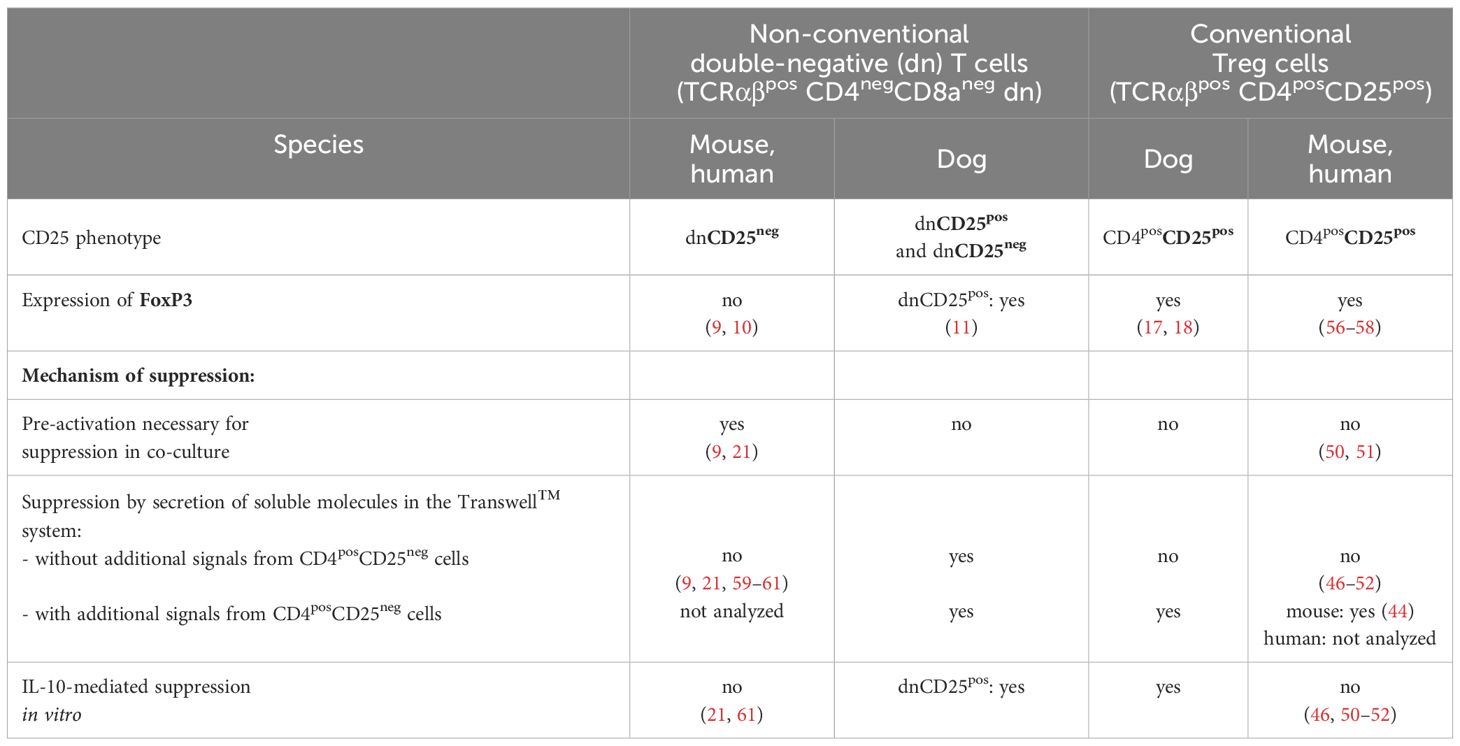
Table 2 Unique features of immunosuppressive double-negative (dn) T cells and conventional regulatory T cells of the dog in vitro in comparison to their murine and human counterparts.
While freshly isolated human and murine dn T cells are unable to suppress responder cells under resting conditions in vitro (9, 21), canine dnCD25pos and dnCD25neg T cells do not need pre-activation before co-culture to exert their suppressive function. Using the Transwell™ system, we could show that both, canine non-conventional dnCD25pos and dnCD25neg T cells are able to mediate suppression cell-cell contact independently via secretion of inhibitory molecules. In striking contrast, despite a diverse spectrum of inhibitory mechanisms (12, 13, 49, 53, 54, 62), suppression by human and murine dn T cells requires cell–cell contact and is not solely mediated by soluble factors (9, 21, 59–61). The secretion of soluble immunosuppressive factors, such as IL-10, TGF-β (63, 64), and IL-35 (65) is an important mechanism of suppression by conventional CD4pos Treg cells in vivo (53, 54). Noteworthy, we could show that, similar to murine Treg cells (44), canine conventional CD4pos Treg cells need signals from other cells (CD4posCD25neg) to secrete suppressive molecules in vitro. In contrast, non-conventional dnCD25pos and dnCD25neg T cells of the dog seem to have intrinsic capacity to secrete inhibitory factors as indicated by our Transwell™ experiments. Neutralization of IL-10 in the Transwell™ system completely abrogated suppression mediated by canine dnCD25pos T cells, suggesting that IL-10 is a highly relevant cytokine for cell-cell contact independent suppression of proliferation by this subpopulation in vitro. In contrast, IL-10 does not play a role for suppression by murine (9, 26) and human non-conventional dn T cells in vitro. Although human dn T cells secrete some IL-10 (10, 21, 66, 67), addition of a neutralizing anti-IL-10 antibody was not able to abrogate their suppressive activity in co-culture experiments (21). Similarly, neutralization of IL-10 did not alter suppressive effects of murine and human conventional CD4pos Treg cells in vitro (46, 50–52). Noteworthy, we could show that canine conventional CD4posCD25pos Treg cells differ from their murine and human counterparts and use IL-10 secretion as a potent suppression mechanism in vitro. In contrast, the suppressive effects of dnCD25neg T cells did not rely on IL-10 secretion. Whether other inhibitory soluble factors or synergistic effects between different cytokines play a role in canine cells needs further investigation. Synergism has been shown for TGF-β and IL-10 in mice (68, 69).
In conclusion, our data demonstrate that canine CD25pos and CD25neg TCRαβpos CD4negCD8αneg dn T cells are potent suppressor cells in vitro with unique features compared to dn T cells of mice and humans as well as conventional CD4pos Treg cells (summarized in Table 2). This provides the basis for future in vivo studies addressing the role of canine non-conventional immunoregulatory dn T cell subpopulations in health and disease, which may ultimately inform new immunotherapeutic strategies.
Data availability statement
The original contributions presented in the study are included in the article/Supplementary Material. Further inquiries can be directed to the corresponding author.
Ethics statement
The animal study was approved by Saxony State Office (Landesdirektion Sachsen) in Leipzig, Germany (approval number: DD24.1-5131/444/30). The study was conducted in accordance with the local legislation and institutional requirements.
Author contributions
LK: Data curation, Formal analysis, Investigation, Methodology, Visualization, Writing – original draft, Writing – review & editing. PM: Resources, Writing – original draft, Writing – review & editing. GA: Conceptualization, Resources, Supervision, Writing – original draft, Writing – review & editing. ME: Conceptualization, Data curation, Formal analysis, Funding acquisition, Investigation, Methodology, Project administration, Supervision, Visualization, Writing – original draft, Writing – review & editing.
Funding
The author(s) declare financial support was received for the research, authorship, and/or publication of this article. This study was funded by grant ES 645/1–1 (to ME) from the German Research Foundation (Deutsche Forschungsgemeinschaft, DFG). The authors further acknowledge support from the German Research Foundation (DFG) and Universität Leipzig within the program of OpenAccess Publishing.
Acknowledgments
We thank Aileen Wingenfeld and Anett Grohs for their technical support and commitment. We also thank Ina Hochheim and Caroline Schöller from the Institute of Pharmacology, Pharmacy and Toxicology, Faculty of Veterinary Medicine, Leipzig University for providing blood samples and their extensive care of the Beagle dogs. Dr. Christiane Schnabel, Dr. Uwe Müller, and Dr. Mathias Büttner are thanked for critical reading of the manuscript. Flow cytometry was performed at the Core Unit Flow Cytometry (CUDZ) of the Faculty of Veterinary Medicine, Leipzig University, maintained by Dr. Uwe Müller.
Conflict of interest
The authors declare that the research was conducted in the absence of any commercial or financial relationships that could be construed as a potential conflict of interest.
Publisher’s note
All claims expressed in this article are solely those of the authors and do not necessarily represent those of their affiliated organizations, or those of the publisher, the editors and the reviewers. Any product that may be evaluated in this article, or claim that may be made by its manufacturer, is not guaranteed or endorsed by the publisher.
Supplementary material
The Supplementary Material for this article can be found online at: https://www.frontiersin.org/articles/10.3389/fimmu.2024.1439213/full#supplementary-material
References
1. Gershwin LJ. Autoimmune diseases in small animals. Vet Clin North Am Small Anim Pract. (2010) 40:439–57. doi: 10.1016/j.cvsm.2010.02.003
2. Lehtimäki J, Sinkko H, Hielm-Björkman A, Laatikainen T, Ruokolainen L, Lohi H. Simultaneous allergic traits in dogs and their owners are associated with living environment, lifestyle and microbial exposures. Sci Rep. (2020) 10:21954. doi: 10.1038/s41598-020-79055-x
3. Mueller RS, Jensen-Jarolim E, Roth-Walter F, Marti E, Janda J, Seida AA, et al. Allergen immunotherapy in people, dogs, cats and horses - differences, similarities and research needs. Allergy. (2018) 73:1989–99. doi: 10.1111/all.13464
4. Oh JH, Cho J-Y. Comparative oncology: overcoming human cancer through companion animal studies. Exp Mol Med. (2023) 55:725–34. doi: 10.1038/s12276-023-00977-3
5. Overgaard NH, Fan TM, Schachtschneider KM, Principe DR, Schook LB, Jungersen G. Of mice, dogs, pigs, and men: choosing the appropriate model for immuno-oncology research. ILAR J. (2018) 59:247–62. doi: 10.1093/ilar/ily014
6. Chang H-C, Tan K, Ouyang J, Parisini E, Liu J, Le Y, et al. Structural and mutational analyses of a CD8alphabeta heterodimer and comparison with the CD8alphaalpha homodimer. Immunity. (2005) 23:661–71. doi: 10.1016/j.immuni.2005.11.002
7. Zhu J, Yamane H, Paul WE. Differentiation of effector CD4 T cell populations (*). Annu Rev Immunol. (2010) 28:445–89. doi: 10.1146/annurev-immunol-030409-101212
8. Hillhouse EE, Lesage S. A comprehensive review of the phenotype and function of antigen-specific immunoregulatory double negative T cells. J Autoimmun. (2013) 40:58–65. doi: 10.1016/j.jaut.2012.07.010
9. Zhang ZX, Yang L, Young KJ, DuTemple B, Zhang L. Identification of a previously unknown antigen-specific regulatory T cell and its mechanism of suppression. Nat Med. (2000) 6:782–9. doi: 10.1038/77513
10. Fischer K, Voelkl S, Heymann J, Przybylski GK, Mondal K, Laumer M, et al. Isolation and characterization of human antigen-specific TCR alpha beta+ CD4(-)CD8- double-negative regulatory T cells. Blood. (2005) 105:2828–35. doi: 10.1182/blood-2004-07-2583
11. Rabiger FV, Rothe K, von Buttlar H, Bismarck D, Büttner M, Moore PF, et al. Distinct features of canine non-conventional CD4-CD8α- double-negative TCRαβ+ vs. TCRγδ+ T cells. Front Immunol. (2019) 10:2748. doi: 10.3389/fimmu.2019.02748
12. Shevyrev D, Tereshchenko V. Treg heterogeneity, function, and homeostasis. Front Immunol. (2019) 10:3100. doi: 10.3389/fimmu.2019.03100
13. Sakaguchi S, Yamaguchi T, Nomura T, Ono M. Regulatory T cells and immune tolerance. Cell. (2008) 133:775–87. doi: 10.1016/j.cell.2008.05.009
14. Sumida TS, Cheru NT, Hafler DA. The regulation and differentiation of regulatory T cells and their dysfunction in autoimmune diseases. Nat Rev Immunol. (2024) 24:503–517. doi: 10.1038/s41577-024-00994-x
15. Noval Rivas M, Chatila TA. Regulatory T cells in allergic diseases. J Allergy Clin Immunol. (2016) 138:639–52. doi: 10.1016/j.jaci.2016.06.003
16. Wood KJ. Regulatory T cells in transplantation. Transplant Proc. (2011) 43:2135–6. doi: 10.1016/j.transproceed.2011.06.050
17. Pinheiro D, Singh Y, Grant CR, Appleton RC, Sacchini F, Walker KR, et al. Phenotypic and functional characterization of a CD4(+) CD25(high) FOXP3(high) regulatory T-cell population in the dog. Immunology. (2011) 132:111–22. doi: 10.1111/j.1365-2567.2010.03346.x
18. Wu Y, Chang Y-M, Stell AJ, Priestnall SL, Sharma E, Goulart MR, et al. Phenotypic characterisation of regulatory T cells in dogs reveals signature transcripts conserved in humans and mice. Sci Rep. (2019) 9:13478. doi: 10.1038/s41598-019-50065-8
19. Protschka M, Di Placido D, Moore PF, Büttner M, Alber G, Eschke M. Canine peripheral non-conventional TCRαβ+ CD4-CD8α- double-negative T cells show T helper 2-like and regulatory properties. Front Immunol. (2024) 15:1400550. doi: 10.3389/fimmu.2024.1400550
20. Maina E, Devriendt B, Cox E. Food allergen-specific sublingual immunotherapy modulates peripheral T cell responses of dogs with adverse food reactions. Vet Immunol Immunopathol. (2019) 212:38–42. doi: 10.1016/j.vetimm.2019.05.003
21. Voelkl S, Gary R, Mackensen A. Characterization of the immunoregulatory function of human TCR-ab+ CD4-CD8- double-negative T cells. Eur J Immunol. (2011) 2011:739–48. doi: 10.1002/eji.201040982
22. Hillhouse EE, Beauchamp C, Chabot-Roy G, Dugas V, Lesage S. Interleukin-10 limits the expansion of immunoregulatory CD4-CD8- T cells in autoimmune-prone non-obese diabetic mice. Immunol Cell Biol. (2010) 88:771–80. doi: 10.1038/icb.2010.84
23. Allgäuer A, Schreiner E, Ferrazzi F, Ekici AB, Gerbitz A, Mackensen A, et al. IL-7 abrogates the immunosuppressive function of human double-negative T cells by activating akt/mTOR signaling. J Immunol. (2015) 195:3139–48. doi: 10.4049/jimmunol.1501389
24. Wu Z, Zheng Y, Sheng J, Han Y, Yang Y, Pan H, et al. CD3+CD4-CD8- (Double-negative) T cells in inflammation, immune disorders and cancer. Front Immunol. (2022) 13:816005. doi: 10.3389/fimmu.2022.816005
25. Velikkakam T, Gollob KJ, Dutra WO. Double-negative T cells: Setting the stage for disease control or progression. Immunology. (2022) 165:371–85. doi: 10.1111/imm.13441
26. Bafor EE, Valencia JC, Young HA. Double negative T regulatory cells: an emerging paradigm shift in reproductive immune tolerance? Front Immunol 13:886645. doi: 10.3389/fimmu.2022.886645
27. Brandt D, Hedrich CM. TCRαβ+CD3+CD4-CD8- (double negative) T cells in autoimmunity. Autoimmun Rev. (2018) 17:422–30. doi: 10.1016/j.autrev.2018.02.001
28. D’Acquisto F, Crompton T. CD3+CD4-CD8- (double negative) T cells: saviours or villains of the immune response? Biochem Pharmacol. (2011) 82:333–40. doi: 10.1016/j.bcp.2011.05.019
29. Thomson CW, Lee BP-L, Zhang L. Double-negative regulatory T cells: non-conventional regulators. Immunol Res. (2006) 35:163–78. doi: 10.1385/IR:35:1:163
30. Chen W, Ford MS, Young KJ, Zhang L. Infusion of in vitro-generated DN T regulatory cells induces permanent cardiac allograft survival in mice. Transplant Proc. (2003) 35:2479–80. doi: 10.1016/j.transproceed.2003.08.030
31. Dugas V, Beauchamp C, Chabot-Roy G, Hillhouse EE, Lesage S. Implication of the CD47 pathway in autoimmune diabetes. J Autoimmun. (2010) 35:23–32. doi: 10.1016/j.jaut.2010.01.002
32. Duncan B, Nazarov-Stoica C, Surls J, Kehl M, Bona C, Casares S, et al. Double negative (CD3 + 4- 8-) TCR alphabeta splenic cells from young NOD mice provide long-lasting protection against type 1 diabetes. PloS One. (2010) 5:e11427. doi: 10.1371/journal.pone.0011427
33. Ford MS, Chen W, Wong S, Li C, Vanama R, Elford AR, et al. Peptide-activated double-negative T cells can prevent autoimmune type-1 diabetes development. Eur J Immunol. (2007) 37:2234–41. doi: 10.1002/eji.200636991
34. Zhang D, Zhang W, Ng TW, Wang Y, Liu Q, Gorantla V, et al. Adoptive cell therapy using antigen-specific CD4-CD8-T regulatory cells to prevent autoimmune diabetes and promote islet allograft survival in NOD mice. Diabetologia. (2011) 54:2082–92. doi: 10.1007/s00125-011-2179-4
35. Tian D, Yang L, Wang S, Zhu Y, Shi W, Zhang C, et al. Double negative T cells mediate Lag3-dependent antigen-specific protection in allergic asthma. Nat Commun. (2019) 10:4246. doi: 10.1038/s41467-019-12243-0
36. He KM, Ma Y, Wang S, Min W-P, Zhong R, Jevnikar A, et al. Donor double-negative Treg promote allogeneic mixed chimerism and tolerance. Eur J Immunol. (2007) 37:3455–66. doi: 10.1002/eji.200737408
37. McIver Z, Serio B, Dunbar A, O’Keefe CL, Powers J, Wlodarski M, et al. Double-negative regulatory T cells induce allotolerance when expanded after allogeneic haematopoietic stem cell transplantation. Br J Haematol. (2008) 141:170–8. doi: 10.1111/j.1365-2141.2008.07021.x
38. Young KJ, Yang L, Phillips MJ, Zhang L. Donor-lymphocyte infusion induces transplantation tolerance by activating systemic and graft-infiltrating double-negative regulatory T cells. Blood. (2002) 100:3408–14. doi: 10.1182/blood-2002-01-0235
39. Young KJ, DuTemple B, Phillips MJ, Zhang L. Inhibition of graft-versus-host disease by double-negative regulatory T cells. J Immunol. (2003) 171:134–41. doi: 10.4049/jimmunol.171.1.134
40. Biller BJ, Elmslie RE, Burnett RC, Avery AC, Dow SW. Use of FoxP3 expression to identify regulatory T cells in healthy dogs and dogs with cancer. Vet Immunol Immunopathol. (2007) 116:69–78. doi: 10.1016/j.vetimm.2006.12.002
41. Mizuno T, Suzuki R, Umeki S, Okuda M. Crossreactivity of antibodies to canine CD25 and Foxp3 and identification of canine CD4+CD25 +Foxp3+ cells in canine peripheral blood. J Vet Med Sci. (2009) 71:1561–8. doi: 10.1292/jvms.001561
42. Rabiger FV, Bismarck D, Protschka M, Köhler G, Moore PF, Büttner M, et al. Canine tissue-associated CD4+CD8α+ double-positive T cells are an activated T cell subpopulation with heterogeneous functional potential. PloS One. (2019) 14:e0213597. doi: 10.1371/journal.pone.0213597
43. Volkmann M, Hepworth MR, Ebner F, Rausch S, Kohn B, Hartmann S. Frequencies of regulatory T cells in the peripheral blood of dogs with primary immune-mediated thrombocytopenia and chronic enteropathy: a pilot study. Vet J (London Engl 1997). (2014) 202:630–3. doi: 10.1016/j.tvjl.2014.10.012
44. Collison LW, Pillai MR, Chaturvedi V, Vignali DA. Regulatory T cell suppression is potentiated by target T cells in a cell contact, IL-35- and IL-10-dependent manner. J Immunol. (2009) 182:6121–8. doi: 10.4049/jimmunol.0803646
45. Käser T, Gerner W, Saalmüller A. Porcine regulatory T cells: mechanisms and T-cell targets of suppression. Dev Comp Immunol. (2011) 35:1166–72. doi: 10.1016/j.dci.2011.04.006
46. Dieckmann D, Plottner H, Berchtold S, Berger T, Schuler G. Ex vivo isolation and characterization of CD4(+)CD25(+) T cells with regulatory properties from human blood. J Exp Med. (2001) 193:1303–10. doi: 10.1084/jem.193.11.1303
47. Cederbom L, Hall H, Ivars F. CD4+CD25+ regulatory T cells down-regulate co-stimulatory molecules on antigen-presenting cells. Eur J Immunol. (2000) 30:1538–43. doi: 10.1002/1521-4141(200006)30:6<1538:AID-IMMU1538>3.0.CO;2-X
48. Shevach EM, McHugh RS, Piccirillo CA, Thornton AM. Control of T-cell activation by CD4+ CD25+ suppressor T cells. Immunol Rev. (2001) 182:58–67. doi: 10.1034/j.1600-065X.2001.1820104.x
49. Workman CJ, Szymczak-Workman AL, Collison LW, Pillai MR, Vignali DA. The development and function of regulatory T cells. Cell Mol Life Sci CMLS. (2009) 66:2603–22. doi: 10.1007/s00018-009-0026-2
50. Takahashi T, Kuniyasu Y, Toda M, Sakaguchi N, Itoh M, Iwata M, et al. Immunologic self-tolerance maintained by CD25+CD4+ naturally anergic and suppressive T cells: induction of autoimmune disease by breaking their anergic/suppressive state. Int Immunol. (1998) 10:1969–80. doi: 10.1093/intimm/10.12.1969
51. Thornton AM, Shevach EM. CD4+CD25+ immunoregulatory T cells suppress polyclonal T cell activation in vitro by inhibiting interleukin 2 production. J Exp Med. (1998) 188:287–96. doi: 10.1084/jem.188.2.287
52. Jonuleit H, Schmitt E, Stassen M, Tuettenberg A, Knop J, Enk AH. Identification and functional characterization of human CD4(+)CD25(+) T cells with regulatory properties isolated from peripheral blood. J Exp Med. (2001) 193:1285–94. doi: 10.1084/jem.193.11.1285
53. Sojka DK, Huang Y-H, Fowell DJ. Mechanisms of regulatory T-cell suppression - a diverse arsenal for a moving target. Immunology. (2008) 124:13–22. doi: 10.1111/j.1365-2567.2008.02813.x
54. Vignali DA, Collison LW, Workman CJ. How regulatory T cells work. Nat Rev Immunol. (2008) 8:523–32. doi: 10.1038/nri2343
55. Cheru N, Hafler DA, Sumida TS. Regulatory T cells in peripheral tissue tolerance and diseases. Front Immunol. (2023) 14:1154575. doi: 10.3389/fimmu.2023.1154575
56. Fontenot JD, Gavin MA, Rudensky AY. Foxp3 programs the development and function of CD4+CD25+ regulatory T cells. Nat Immunol. (2003) 4:330–6. doi: 10.1038/ni904
57. Hori S, Nomura T, Sakaguchi S. Control of regulatory T cell development by the transcription factor Foxp3. Science. (2003) 299:1057–61. doi: 10.1126/science.1079490
58. Ziegler SF. FOXP3: of mice and men. Annu Rev Immunol. (2006) 24:209–26. doi: 10.1146/annurev.immunol.24.021605.090547
59. Young KJ, Zhang L. The nature and mechanisms of DN regulatory T-cell mediated suppression. Hum Immunol. (2002) 63:926–34. doi: 10.1016/S0198-8859(02)00446-9
60. Chen W, Ford MS, Young KJ, Zhang L. The role and mechanisms of double negative regulatory T cells in the suppression of immune responses. Cell Mol Immunol. (2004) 1:328–35.>
61. Achita P, Dervovic D, Ly D, Lee JB, Haug T, Joe B, et al. Infusion of ex-vivo expanded human TCR-αβ+ double-negative regulatory T cells delays onset of xenogeneic graft-versus-host disease. Clin Exp Immunol. (2018) 193:386–99. doi: 10.1111/cei.13145
62. Shevach EM. Regulatory T cells in autoimmmunity*. Annu Rev Immunol. (2000) 18:423–49. doi: 10.1146/annurev.immunol.18.1.423
63. Joetham A, Takeda K, Taube C, Miyahara N, Matsubara S, Koya T, et al. Naturally occurring lung CD4(+)CD25(+) T cell regulation of airway allergic responses depends on IL-10 induction of TGF-beta. J Immunol. (2007) 178:1433–42. doi: 10.4049/jimmunol.178.3.1433
64. Asseman C, Mauze S, Leach MW, Coffman RL, Powrie F. An essential role for interleukin 10 in the function of regulatory T cells that inhibit intestinal inflammation. J Exp Med. (1999) 190:995–1004. doi: 10.1084/jem.190.7.995
65. Collison LW, Workman CJ, Kuo TT, Boyd K, Wang Y, Vignali KM, et al. The inhibitory cytokine IL-35 contributes to regulatory T-cell function. Nature. (2007) 450:566–9. doi: 10.1038/nature06306
66. Crispín JC, Tsokos GC. Human TCR-alpha beta+ CD4- CD8- T cells can derive from CD8+ T cells and display an inflammatory effector phenotype. J Immunol. (2009) 183:4675–81. doi: 10.4049/jimmunol.0901533
67. Martina MN, Noel S, Saxena A, Bandapalle S, Majithia R, Jie C, et al. Double-negative αβ T cells are early responders to AKI and are found in human kidney. J Am Soc Nephrol JASN. (2016) 27:1113–23. doi: 10.1681/ASN.2014121214
68. Strauss L, Bergmann C, Szczepanski M, Gooding W, Johnson JT, Whiteside TL. A unique subset of CD4+CD25highFoxp3+ T cells secreting interleukin-10 and transforming growth factor-beta1 mediates suppression in the tumor microenvironment. Clin Cancer Res an Off J Am Assoc Cancer Res. (2007) 13:4345–54. doi: 10.1158/1078-0432.CCR-07-0472
Keywords: regulatory T cells, canine non-conventional Treg cells, TCRαβpos CD4neg CD8neg double-negative T cells, FoxP3, interleukin-10, peripheral blood
Citation: Karwig L, Moore PF, Alber G and Eschke M (2024) Distinct characteristics of unique immunoregulatory canine non-conventional TCRαβpos CD4negCD8αneg double-negative T cell subpopulations. Front. Immunol. 15:1439213. doi: 10.3389/fimmu.2024.1439213
Received: 27 May 2024; Accepted: 12 July 2024;
Published: 09 August 2024.
Edited by:
Robert David Miller, University of New Mexico, United StatesReviewed by:
Guan-Jun Yang, Ningbo University, ChinaVikash Kumar, Central Inland Fisheries Research Institute (ICAR), India
Amanda Guth, Colorado State University, United States
Copyright © 2024 Karwig, Moore, Alber and Eschke. This is an open-access article distributed under the terms of the Creative Commons Attribution License (CC BY). The use, distribution or reproduction in other forums is permitted, provided the original author(s) and the copyright owner(s) are credited and that the original publication in this journal is cited, in accordance with accepted academic practice. No use, distribution or reproduction is permitted which does not comply with these terms.
*Correspondence: Maria Eschke, maria.eschke@bbz.uni-leipzig.de