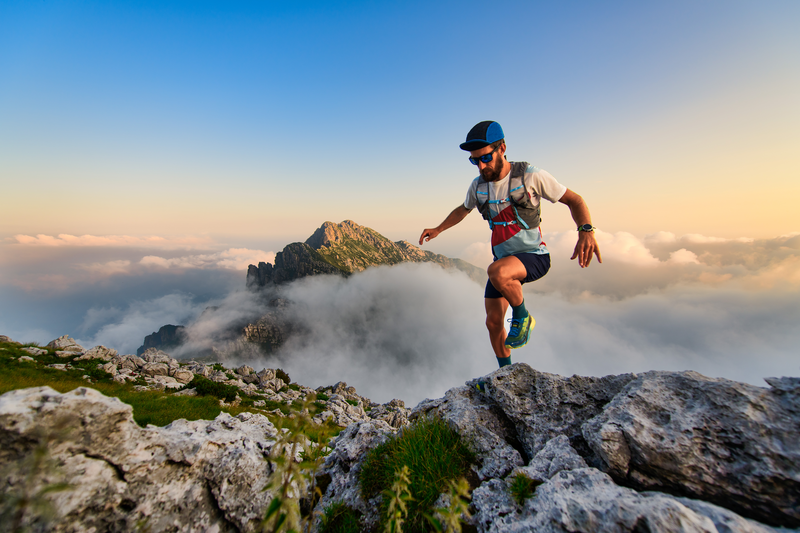
95% of researchers rate our articles as excellent or good
Learn more about the work of our research integrity team to safeguard the quality of each article we publish.
Find out more
ORIGINAL RESEARCH article
Front. Immunol. , 25 September 2024
Sec. Cancer Immunity and Immunotherapy
Volume 15 - 2024 | https://doi.org/10.3389/fimmu.2024.1437726
This article is part of the Research Topic Ubiquitination in Tumor Pathogenesis and Progression and its Therapeutic Potential View all articles
Background: Leukemia is a prevalent pediatric life-threatening hematologic malignancy with a poor prognosis. Targeting immune checkpoints (ICs) to reverse T cell exhaustion is a potentially effective treatment for leukemia. Tissue resident memory T (TRM) cells have been found to predict the efficacy of programmed death receptor-1 inhibitor (anti-PD-1) therapy in solid tumors. However, the IC characteristics of TRM cells in leukemia and their relationship with prognosis remain unclear.
Methods: We employed multi-color flow cytometry to evaluate the frequencies of CD103+CD4+ and CD103+CD8+ T cells in the peripheral blood (PB) of patients with acute myeloid leukemia and B-cell acute lymphoblastic leukemia compared to healthy individuals. We examined the expression patterns of PD-1 and T cell immunoreceptor with immunoglobulin and ITIM domain (TIGIT) within the circulating CD103+ T cell subsets affected by leukemia. To further elucidate the immunological landscape, we assessed the differentiation status of CD103+ T cells across various disease states in patients with leukemia.
Results: Our findings showed a significant increase in the frequency of CD103+CD8+ T cells in the PB of patients with leukemia who had achieved complete remission (CR) compared to those in the de novo (DN) and relapsed/refractory (RR) stages. This increase was accompanied by a notable decrease in the expression levels of PD-1 and TIGIT in CD103+CD8+ T cells in the CR stage. Additionally, our analysis revealed a higher proportion of CD103+CD8+ T cells in the central memory (TCM) and effector memory (TEM) subsets of the immune profile. Notably, the proportions of CD103+ naïve T cells, CD103+ TEM, and CD103+ terminally differentiated T cells within the CD8+ T cell population were significantly elevated in patients with CR compared to those in the DN/RR stages.
Conclusion: The data indicate that circulating higher frequency of CD103+CD8+ T cells with lower expression of PD-1 and TIGIT are associated with favorable outcomes in patients with leukemia. This suggests a potential role of TRM cells in leukemia prognosis and provides a foundation for developing targeted immunotherapies.
Immune checkpoint inhibitors (ICIs) have revolutionized tumor immunotherapy (1, 2). Programmed death receptor-1 (PD-1), the most well-known inhibitory immune checkpoint (IC), has been approved as a clinical target for cancer therapy (3). T cell immunoreceptor with immunoglobulin and ITIM domain (TIGIT) is an emerging checkpoint receptor that significantly influence the cancer microenvironment (4). Several clinical trials have shown that patients with acute myeloid leukemia (AML) can benefit from programed death receptor-1 inhibitor (anti-PD-1) therapy with minimal adverse reactions. However, some trials have reported less favorable outcomes (5). Several mechanisms contribute to resistance to anti-PD-1/PD-1 ligand 1 (PD-L1) therapy. These include the loss of tumor antigens, interaction with other ICs, activation of oncogenic pathways, epigenetic mutations in key tumor proteins, and changes in metabolism (6). The co-inhibition of TIGIT and PD-1/PD-L1 has shown effectiveness in both theoretical and clinical trials (7).
B-acute lymphoblastic leukemia (B-ALL) and AML are life-threatening pediatric hematologic malignancies. B-ALL is defined as an uncontrolled proliferation of immature B lymphoid cells in the bone marrow, peripheral blood (PB), and other organs (8). High-risk patients may undergo intensive chemotherapy regimens, including corticosteroids, vincristine, and l-asparaginase, with allo-HSCT considered for those in first complete remission (CR) (9). While the event-free survival (EFS) rate for patients with B-ALL has significantly improved to 85% (10), the advent of novel immunotherapies, such as blinatumomab and chimeric antigen receptor T cells have expanded treatment options for patients in the relapsed/refractory (RR) stage. However, it is important to note that these new immunotherapeutic approaches have also been linked to the emergence of new types of relapsed diseases (11, 12). Conversely, acute myeloid leukemia (AML) is relatively uncommon in pediatric populations and is characterized by a diverse range of primary neoplasms originating from myeloid precursor cells, resulting in the accumulation of blasts in the PB, bone marrow, and other tissues (13). The standard therapy for AML involves aggressive induction regimens with anthracyclines and cytarabine and follow-up allo-HSCT for eligible high-risk patients to achieve curative outcomes (13, 14). AML continues to have a poor prognosis, with a 5-year EFS rate of only 50% (15). Furthermore, 10–15% of patients newly diagnosed with AML fail to achieve CR even with intensive chemotherapy, and up to 50% of those who do achieve CR eventually experience relapse (15, 16). Advancements in ALL treatments have outpaced those in AML therapy (17). Patients with RR status typically experience poor outcomes, highlighting the need for novel therapeutic approaches. Recent studies have identified PD-1 and TIGIT expression in exhausted T cells of patients with AML and B-ALL (18, 19). Therefore, investigating PD-1 and TIGIT expression in T cells may offer a new theoretical foundation for the combined use of ICIs.
Tissue resident memory T (TRM) cells are a subset of memory T cells that develop and persist predominantly in peripheral nonlymphoid organs, such as the intestines and skin (20–22). They are characterized by the expression of CD103 and CD69. Hobit, Blimp1, Id3, and Runx3 transcription factors are integral to the formation and maintenance of the tissue-resident properties of TRM cells (23, 24). These cells are known for their role in defending organs and tissues against infections (25, 26). Numerous studies have shown that TRM cells exhibit antitumor capabilities in various cancers and are associated with better patient outcomes (27–29). CD8+ TRM cells are targeted by ICI (for example, anti-PD-1 and so on) therapy (30, 31). TRM cells within tumor tissues express multiple inhibitory receptors, with elevated PD-1 and TIGIT expression levels on CD8+CD103+ T cells impairing antitumor function (32, 33). A dual antibody treatment strategy has shown efficacy in ameliorating this depletion activity (34). Our previous study identified a circulating CD103+CD8+ T cell subset characterized by a high expression of exhaustion-related genes, which was elevated in patients with B-ALL (35). A recent study suggested that CD103+CD8+ T cells in the bloodstream may be a biomarker for responding to anti-PD-1 regimens in gastric cancer (36). These findings indicate that exhausted circulating TRM cells may be novel therapeutic targets for ICI treatment.
The objective of this study was to investigate the correlation between circulating TRM cells and patient prognosis. This was accomplished by quantifying the proportion of CD103+CD8+ T cells and assessing the expression levels of PD-1 and TIGIT in the peripheral blood of AML and B-ALL patients with diverse outcomes, utilizing multi-color flow cytometry.
PB samples were collected from patients with AML or B-ALL and healthy individuals (HIs). We obtained 13 samples from patients with leukemia in DN/RR states, 9 samples from patients in the CR state, and 20 samples from HIs. Peripheral blood mononuclear cells (PBMCs) were isolated from the blood samples and analyzed using a FACSLyric cytometer (BD Biosciences, San Jose, CA, USA).
Fluorescence-labeled antibodies used in the study were as follows: CD3-BV786 (clone OKT3, BD), CD4-APC-H7 (clone RPA-T4, BD), CD8-Percp-cy5.5 (clone SK1, BD), CD103 (clone Ber-ACT8, BD), CD45RA-BV510 (clone HI100, BD), CCR7-BV605 (clone G043H7, BioLegend), PD-1-PE-CY7 (clone EH12.1, BD), and TIGIT (clone TgMab-2, BD). Fluorescence-Minus-One Control (FMO) was control background staining and accurate gating, given the low proportion of CD103+ T cells in the peripheral blood. PBMCs were first stained with human FC Receptor Binding Inhibitor (Thermo Fisher Scientific) for 15 minutes in PBS, then incubated with antibodies mentioned above at 4°C for 30 mins. Flow cytometry analysis was conducted on washed cells using a FACSLyric cytometer (BD Biosciences), and the data were analyzed using Flowjo 10.6 software.
We analyzed differences between groups using the Mann–Whitney U test and differences within groups using the nonparametric Wilcoxon matched-pair signed-rank test. Computations were conducted using GraphPad Prism version 8.02 software. A significance level of p < 0.05 was considered significant, with levels of significance indicated as *p < 0.05, **p < 0.01, and ***p < 0.001.
We initially evaluated CD103 expression levels among CD3+, CD4+, and CD8+ T cells in PB (Figure 1A). There were no significant differences in the proportions of CD103+CD3+ T cells, CD103+CD4+ T cells, and CD103+CD8+ T cells between patients with DN-AML and those with DN-B-ALL (Figure 1B). Therefore, subsequent analyses pooled patients with AML and B-ALL. The frequencies of CD103+CD3+, CD103+CD4+, and CD103+CD8+ T cells were significantly higher in patients with leukemia in CR than in HIs and DN/RR. The percentage of CD103+CD8+ T cells in patients with leukemia in the DN/RR states was lower than in HIs. However, this was not statistically significant (p = 0.072, Figure 1C). Furthermore, in HIs and patients with leukemia in DN/RR states, CD103+CD8+ T cells were more prevalent than CD103+CD4+ T cells within the same peripheral blood sample. In patients with leukemia in the CR state, no significant difference was observed between CD103+CD4+ andCD103+CD8+ T cells from the same samples (Figure 1D). The t-stochastic neighbor embedding analysis of CD3+ T cells from HIs and patients with leukemia in CR, DN/RR states is shown in Figure 2.
Figure 1. Increased levels of CD103+CD3+ T cells, CD103+CD4+ T cells and CD103+CD8+ T cells in CR patients compared to HI and DN/RR patients and the frequencies of CD103+CD8+ T cells was higher than CD103+CD4+ T cells of the same PB sample. (A). The gating strategies of CD103+CD3+ T cells, CD103+CD4+ T cells and CD103+CD8+ T cells are shown. (B) The ratio of CD103+CD3+ T cells, CD103+CD4+ T cells and CD103+CD8+ T cells in de novo AML and de novo B-ALL patients. Values are indicated as medians. The significance of differences was calculated using the nonparametric Mann–Whitney U test. (C). The frequencies of CD103+CD3+ T cells, CD103+CD4+ T cells and CD103+CD8+ T cells in CR, HIs and DN/RR patients. (CR n = 9, HI n = 20, DN/RR n = 13). Values are indicated as medians. The significance of differences was calculated using the nonparametric Mann–Whitney U test. (D) Frequencies of CD103+CD4+ T cells and CD103+CD8+ T cells of the same peripheral blood sample. The significance of differences was calculated using the nonparametric Wilcoxon matched-pairs signed-rank test. (*p < 0.05, **p < 0.01, ***p < 0.001, ****p < 0.0001) CR, complete remission; HIs, healthy individuals; DN/RR, de novo and relapsed/refractory. P values < 0.05 were considered statistically significant.
Figure 2. T-distributed random neighbor embedded (tSNE) analysis of the distribution of CD103+ T cells in CD3+, CD4+, and CD8+ T cells in CR, HIs and DN/RR patients.
To characterize the exhaustion characteristics of the CD103+ T cell subset, we analyzed the expression levels of PD-1 and TIGIT in CD103+CD4+ and CD103+CD8+ T cells (Figure 3A). Patients in DN/RR states exhibited significantly higher proportions of PD-1+CD103+CD4+ and PD-1+CD103+CD8+ T cells than HIs. There were no statistically significant differences in the proportions of PD-1+CD103+CD4+ T cells and PD-1+CD103+CD8+ T cells between CR patients and HIs or between CR and DN/RR patients. Furthermore, patients with DN/RR displayed higher frequencies of TIGIT+CD103+CD4+ T cells and TIGIT+CD103+CD8+ T cells than those with HIs. A trend toward a higher level of TIGIT+CD103+CD4+ T cells was observed in CR patients than in HIs, although this did not reach statistical significance (p = 0.0617, Figures 3B, C). In summary, elevated PD-1 and TIGIT expression levels in CD103+ T cells were observed in patients with leukemia in the DN and RR states.
Figure 3. Higher frequencies of PD-1+CD103+CD4+ T cells, PD-1+CD103+CD8+ T cells, TIGIT+CD103+CD4+ T cells and TIGIT+CD103+CD8+ T cells in DN/RR patients compared with HI. (A) Flow cytometry analysis of the ration of the frequency of PD-1 and TIGIT in CD103+ T cells. (B) The percentages of PD-1+CD103+CD4+ T cells, PD-1+CD103+CD8+ T cells in CR, HIs and DN/RR patients. (CR n = 8, due to one of the CR patients using nivolumab treatment regimen, the PD-1 expression frequency cannot be detected.) (C) The percentages of TIGIT+CD103+CD4+ T cells, TIGIT+CD103+CD8+ T cells in CR, HIs and DN/RR patients. Values are indicated as medians. The significance of differences was calculated using the nonparametric Mann–Whitney U test. (*p < 0.05, **p < 0.01).
We analyzed the differentiation status of T cells using the naïve T cell marker CD45RA and the chemokine receptor CCR7. This analysis categorized T cells as naïve (CD45RA+CCR7+), central memory (TCM, CD45RA−CCR7+), effector memory (TEM, CD45RA−CCR7−), and terminally differentiated (TEMRA, CD45RA+CCR7−) subsets. We then examined the distribution of CD103+ T cells across these differentiation subsets (Figure 4A). CD103+CD4+ T cells level was higher in the TEM and TEMRA subsets, with a lower level found in the naïve subset than in the other differentiation subsets. This pattern was not statistically significant among patients with leukemia in the CR state (Figure 4B). However, for CD103+CD8+ T cells, the highest level was observed in the TCM subset, followed by the TEM subset. The naïve and TEMRA subsets exhibited the lowest level of CD103+CD8+ T cells in the CR, HIs, DN/RR groups (Figure 4C). To avoid variations in the distribution of naïve, TCM, TEM, and TEMRA subsets among individuals, we analyzed the levels of CD103+ naïve, TCM, TEM, and TEMRA T cells within the total CD8+ T cells. The levels of CD103+ naïve, TEM, and TEMRA T cells were higher in the CR than in the DN/RR groups. Furthermore, the CR groups exhibited elevated levels of CD103+ TEMRA T cells within CD8+ T cells compared to HIs (Figure 4D). No significant differences were found in the levels of CD103+ TCM T cells within CD8+ T cells among the CR, HI, DN/RR groups. The elevated level of CD103+CD8+ T cells in the TCM and TEM subsets indicates that TRM cells in PB may promptly respond to secondary antigen exposure and exert antitumor functions similar to TCM and TEM cells. Moreover, CD103+ naïve, TEM, and TEMRA T cells within CD8+ T cells may contribute to CR outcomes in patients with AML and B-ALL.
Figure 4. The frequency of CD103+CD8+ T cells was higher in TCM and TEM subsets and the ratio of CD103+ naïve T cells, CD103+ TEM T cells, and CD103+ TEMRA T cells in CD8+ T cells were higher in CR patients than in DN/RR patients. (A) Flow cytometry analysis of the CD103+ T cells in different differentiation subsets. (B, C) The percentages of CD103+CD4+ T cells, CD103+ CD8+ T cells in each differentiation subset. Values are indicated as medians. The significance of differences was calculated using the nonparametric Wilcoxon matched-pairs signed-rank test. (D) The proportion of CD103+ naïve T cells, CD103+ TEM T cells, and CD103+ TEMRA T cells in CD8+ T cells of CR, HIs and DN/RR patients. Values are indicated as medians. The significance of differences was calculated using the nonparametric Mann–Whitney U test. (*p < 0.05, **p < 0.01, ***p < 0.001, ****p < 0.0001).
We analyzed the distribution and exhaustion of CD103+ T cells in the PB of patients with AML and B-ALL respectively. In patients with AML, the frequencies of CD103+CD4+ and CD103+CD8+ T cells were higher in the CR group than in the HIs, DN/RR groups. No significant differences were observed between the HIs, DN/RR groups. In patients with B-ALL, a similar increase in CD103+CD4+ T cells was observed in the CR group compared to the HIs, DN/RR groups. Furthermore, the level of CD103+CD8+ T cells tended to decrease in the DN/RR groups compared to that in the CR and HIs groups, although the difference was not statistically significant (DN/RR vs. CR, p = 0.0850; DN/RR vs. HIs, p = 0.0750). No significant differences were observed between patients with AML and B-ALL (Figures 5A, D). The proportion of PD-1+CD103+CD4+ T cells, indicative of an exhausted phenotype, was higher in CR, DN/RR patients with B-ALL than in HIs. Similarly, the expression level of TIGIT+CD103+CD8+ T cells, also defined as exhausted phenotype, increased compared to the HIs. No further significant differences were identified among these groups (Figures 5B, C, E, F).
Figure 5. The proportion of CD103+CD4+ T cells and CD103+CD8+ T cells in CR patients compared to HI and DN/RR patients and the frequencies of exhausted phenotype CD103+ cells was higher in DN/RR patients with AML and B-ALL respectively and comparison between AML and B-ALL patients. (A, D) The frequencies of CD103+CD4+ T cells and CD103+CD8+ T cells in CR, HIs and DN/RR patients with AML and B-ALL respectively and comparison between AML and B-ALL patients. (AML CR n = 6, DN/RR n = 5; B-ALL CR n = 3, DN/RR n = 8). (B, E) The percentages of PD-1+CD103+CD4+ T cells, PD-1+CD103+CD8+ T cells in CR, HIs and DN/RR patients with AML and B-ALL respectively and comparison between AML and B-ALL patients. (B-ALL CR n = 2, due to one of the CR patients using nivolumab treatment regimen, the PD-1 expression frequency cannot be detected.) (C, F) The percentages of TIGIT+CD103+CD4+ T cells, TIGIT+CD103+CD8+ T cells in CR, HIs and DN/RR patients with AML and B-ALL respectively and comparison between AML and B-ALL patients. Values are indicated as medians. The significance of differences was calculated using the nonparametric Mann–Whitney U test. (*p < 0.05, **p < 0.01).
While PD-1/PD-L1 inhibitors have proven effective in treating solid tumors, their activity in AML and B-ALL has been less significant (37). Identifying novel and specific targets may be crucial to addressing this disparity. In our previous study, we identified circulating TRM-like cells with a relatively high level of exhaustion genes in patients with B-ALL using single-cell sequencing (35), indicating that TRM cells could be a potential new target for ICIs. However, in this study, we investigated the differences in CD103+CD8+ T cells between patients with leukemia in the CR state and those with DN/RR states.
First, a study reported that a subset of TRM cells, characterized by their recognition of ovarian cancer antigens, effectively inhibited tumor growth compared to CD103-CD8+ T cells (38). This finding is corroborated by RNA sequencing data indicating that CD103+CD8+ T cells possess an enhanced cytotoxic profile and exhibit a gene signature associated with interferon-γ (IFN-γ) production, distinguishing them from other CD8+ T cells (39). Given their dual ability to recognize tumor cells, inhibit growth, secrete IFN-γ, and perform cytotoxic functions, we suggest that these cells may be tumor-specific and potentially associated with favorable clinical outcomes. Our findings align with previous studies on head and neck cancer, demonstrating that patients who achieved remission following anti-PD-1 monoclonal antibody therapy exhibited a significant increase in CD103+CD8+ T cells in their pretreatment biopsy tissues compared to those who did not achieve remission (30). The higher expression level of CD103+CD8+ T cells in PB from patients in CR, as opposed to those in DN/RR, supports the antitumor function of circulating TRM cells in patients with acute leukemia.
Second, patients in the DN/RR states showed significantly higher levels of exhausted phenotype CD103+CD8+ T cells than HIs. A recent study systematically outlined the dynamics of TRM cells in response to pembrolizumab therapy. It was observed that patients who achieved remission following treatment predominantly exhibited an increase in cytotoxic TRM cells, characterized by the expression of granzyme H, granzyme K, SLAM family member 7, and eomesodermin, alongside reduced exhaustion markers. Conversely, tissues from patients who did not achieve remission exhibited amplified TRM cells with increased exhaustion profiles, characterized by the expression of PDCD1, T-cell immunoglobulin and mucin-domain containing-2, TIGIT, cytotoxic T-lymphocyte-associated protein 4, and thymocyte selection-associated high mobility group box protein (30). This indicates that besides the decrease in CD103+CD8+ T cells, diminished antileukemia function also contributes to the occurrence and progression of AML and B-ALL. TIGIT, an emerging IC molecule, is gaining attention in hematological malignancies and is seen as a potential therapeutic target (40, 41). Studies have demonstrated that TIGIT inhibits CD8+ T cells function in the immune response against tumors and viruses (42). In our previous research, we found that the TIGIT expression of CD8+ T cells in the bone marrow and peripheral blood of AML patients was higher than that in HIs (43), suggesting that TIGIT may play an important role in T cell dysfunction in leukemia patients. Dual blockade of the PD-1 and TIGIT enhance the anti-tumor ability of CD8+ T cells (44). Consistent with a recent study on endometrial cancer (34), high PD-1 and TIGIT expression levels indicate that CD103+CD8+ T cells are promising targets for ICIs. Additionally, another study identified the expression of immunosuppressive receptors on the surface of TRM cells, where PD-1 and TIM-3 double-positive cells show functional impairment (45, 46).
Third, among the naïve, TCM, TEM, and TEMRA subsets, the TCM and TEM subsets exhibited higher expression levels of CD103+CD8+ T cells. This finding is corroborated by another study on circulating TRM cells (36) suggesting that upon re-exposure to tumor antigens, CD103+CD8+ T cells differentiate into effector cells, such as TCM and TEM cells, thereby exerting an antitumor function. Reports indicate that CD8+ memory T cells are the predominant phenotype expanded during anti-PD-1 blockade therapy (47), potentially explaining why the CD103+CD8+ T cell subset is a biomarker for ICI efficacy. Due to variations in proportions of naïve/TCM/TEM/TEMRA subgroups among individuals, we conducted a detailed analysis of the frequencies of CD103+ naïve, TCM, TEM, and TEMRA T cells within CD8+ T cells across patients in CR, DN/RR states and HIs. The expression levels of CD103+ naïve, TEM, and TEMRA within CD8+ T cells were higher in patients in the CR state than in HIs and patients in those in DN/RR states, suggesting that increased expression levels of CD103+ T cells in these subsets may facilitate CR. Studies have shown that CCR7, known for mediating T cell entry into secondary lymphoid organs (48), is crucial in forming CD103+CD8+ T cells and its tumor-clearing capabilities (49). We also suggest that the increase in CD103+ naïve T cells in patients in the CR state is a significant factor in achieving CR.
Finally, the differences in CD103+ T cells in the PB of patients with AML and B-ALL were examined. We also found higher expression levels of CD103+CD4+ and CD103+CD8+ T cells in patients with AML or B-ALL in the CR state than in the DN/RR states and HIs. Similarly, a higher expression level of the exhausted phenotype was observed in CD103+ T cells in patients in DN/RR states than in HIs. Nevertheless, this study has some limitations. The low incidence of hematologic diseases in pediatric populations has constrained our ability to identify significant differences in the expression levels of CD103+CD3+ T cells, CD103+CD4+ T cells, and CD103+CD8+ T cells between patients in DN/RR states and HIs, primarily due to small sample sizes. This limitation also affected the data analysis for patients with AML and B-ALL. Therefore, further research with larger cohort sizes is needed to thoroughly analyze the distribution patterns and exhaustion phenotypes of circulating TRM cells.
In summary, these findings indicate that a higher frequency of CD103+CD8+ T cells in PB and lower expression levels of PD-1+CD103+CD8+ T cells and TIGIT+CD103+CD8+ T cells are associated with a higher likelihood of achieving CR outcomes in AML and B-ALL. A higher level of CD103+CD8+ T cells was observed within the TCM and TEM subsets. Moreover, the levels of CD103+ naïve, TEM, and TEMRA T cells within the CD8+ T cells of patients in the CR state were higher than those in the DN and RR states. Identifying CD103+CD8+ T cells as a potential predictive factor for favorable prognosis requires further validation, which may necessitate larger sample sizes. In addition, selecting biomarkers suitable for immune checkpoint drugs in AML and B-ALL patients, and even predicting the efficacy of adoptive T cell therapy, including chimeric antigen receptor T (CAR-T) cell therapy. Further study is necessary to validate CD103+CD8+ T cells as a promising novel target for enhancing current cancer immunotherapies in pediatric patients.
The original contributions presented in the study are included in the article/supplementary material. Further inquiries can be directed to the corresponding authors.
The studies involving humans were approved by Ethics Committee of the Fifth Affiliated Hospital of Guangzhou Medical University. The studies were conducted in accordance with the local legislation and institutional requirements. Written informed consent for participation in this study was provided by the participants’ legal guardians/next of kin.
LL: Writing – review & editing, Writing – original draft, Formal analysis. WL: Writing – original draft, Investigation, Formal analysis. XZ: Writing – review & editing, Writing – original draft, Investigation. SC: Writing – original draft, Investigation. XL: Writing – original draft, Supervision. HT: Writing – review & editing, Supervision.
The author(s) declare that no financial support was received for the research, authorship, and/or publication of this article.
The authors would like to thank Yangqiu Li of the School of Medicine of Jinan University for helpful discussions on topics related to this work.
The authors declare that the research was conducted in the absence of any commercial or financial relationships that could be construed as a potential conflict of interest.
The reviewer ZL declared a shared affiliation with the authors to the handling editor at the time of review.
All claims expressed in this article are solely those of the authors and do not necessarily represent those of their affiliated organizations, or those of the publisher, the editors and the reviewers. Any product that may be evaluated in this article, or claim that may be made by its manufacturer, is not guaranteed or endorsed by the publisher.
ICs, Immune Checkpoints; TRM, Tissue Resident Memory T; PB, Peripheral Blood; AML, Acute Myeloid Leukemia; B-ALL, B-cell Acute Lymphoblastic Leukemia; HIs, Healthy Individuals; PD-1, Programmed Death Receptor-1; TIGIT, T Cell Immunoreceptor With Immunoglobulin And ITIM Domain; CR, Complete Remission; DN, De Novo; RR, Relapsed/Refractory; TCM, Central Memory T cells; TEM, Effector Memory T cells; TEMRA, Terminally Differentiated Effector Memory T cells; PDCD1, Programmed cell death protein 1.
1. Vaddepally RK, Kharel P, Pandey R, Garje R, Chandra AB. Review of indications of FDA-approved immune checkpoint inhibitors per NCCN guidelines with the level of evidence. Cancers. (2020) 12:738. doi: 10.3390/cancers12030738
2. Tan J, Tan H, Li Y. Targeting TIM-3 for hematological Malignancy: Latest updates from the 2022 ASH annual meeting. Exp Hematol Oncol. (2023) 12:62. doi: 10.1186/s40164-023-00421-2
3. Tang Q, Chen Y, Li X, Long S, Shi Y, Yu Y, et al. The role of PD-1/PD-L1 and application of immune-checkpoint inhibitors in human cancers. Front Immunol. (2022) 13:964442. doi: 10.3389/fimmu.2022.964442
4. Chauvin JM, Zarour HM. TIGIT in cancer immunotherapy. J Immunother Cancer. (2020) 8:e000957. doi: 10.1136/jitc-2020-000957
5. Abaza Y, Zeidan AM. Immune checkpoint inhibition in acute myeloid leukemia and myelodysplastic syndromes. Cells. (2022) 11. doi: 10.3390/cells11142249
6. Wang Z, Wu X, Wu X. Study and analysis of antitumor resistance mechanism of PD1/PD-L1 immune checkpoint blocker. Cancer Med. (2020) 9:8086–121. doi: 10.1002/cam4.3410
7. Chu X, Tian W, Wang Z, Zhang J, Zhou R. Co-inhibition of TIGIT and PD-1/PD-L1 in cancer immunotherapy: Mechanisms and clinical trials. Mol Cancer. (2023) 22:93. doi: 10.1186/s12943-023-01800-3
8. Paul S, Kantarjian H, Jabbour EJ. Adult acute lymphoblastic leukemia. Mayo Clin Proc. (2016) 91:1645–66. doi: 10.1016/j.mayocp.2016.09.010
9. Brown PA, Shah B, Advani A, Aoun P, Boyer MW, Burke PW, et al. Acute Lymphoblastic Leukemia. version 2.2021, NCCN clinical practice guidelines in oncology. J Natl Compr Canc Netw. (2021) 19:1079–109. doi: 10.6004/jnccn.2021.0042
10. Brivio E, Baruchel A, Beishuizen A, Bourquin JP, Brown PA, Cooper T, et al. Targeted inhibitors and antibody immunotherapies: Novel therapies for paediatric leukaemia and lymphoma. Eur J Cancer. (2022) 164:1–17. doi: 10.1016/j.ejca.2021.12.029
11. Brown PA, Ji L, Xu X, Devidas M, Hogan LE, Borowitz MJ, et al. Effect of postreinduction therapy consolidation with Blinatumomab vs chemotherapy on disease-free survival in children, adolescents, and young adults with first relapse of B-cell acute lymphoblastic leukemia: A randomized clinical trial. J Am Med Assoc. (2021) 325:833–42. doi: 10.1001/jama.2021.0669
12. Cordoba S, Onuoha S, Thomas S, Pignataro DS, Hough R, Ghorashian S, et al. CAR T cells with dual targeting of CD19 and CD22 in pediatric and young adult patients with relapsed or refractory B cell acute lymphoblastic leukemia: A phase 1 trial. Nat Med. (2021) 27:1797–805. doi: 10.1038/s41591-021-01497-1
13. Tallman MS, Wang ES, Altman JK, Appelbaum FR, Bhatt VR, Bixby D, et al. Acute Myeloid Leukemia. version 3.2019, NCCN clinical practice guidelines in oncology. J Natl Compr Canc Netw. (2019) 17:721–49. doi: 10.6004/jnccn.2019.0028
14. Döhner H, Wei AH, Appelbaum FR, Craddock C, DiNardo CD, Dombret H, et al. Diagnosis and management of AML in adults: 2022 recommendations from an international expert panel on behalf of the ELN. Blood. (2022) 140:1345–77. doi: 10.1182/blood.2022016867
15. Rasche M, Zimmermann M, Borschel L, Bourquin JP, Dworzak M, Klingebiel T, et al. Successes and challenges in the treatment of pediatric acute myeloid leukemia: A retrospective analysis of the AML-BFM trials from 1987 to 2012. Leukemia. (2018) 32:2167–77. doi: 10.1038/s41375-018-0071-7
16. Zwaan CM, Kolb EA, Reinhardt D, Abrahamsson J, Adachi S, Aplenc R, et al. Collaborative efforts driving progress in pediatric acute myeloid leukemia. J Clin Oncol. (2015) 33:2949–62. doi: 10.1200/JCO.2015.62.8289
17. Rubnitz JE, Kaspers GJL. How I treat pediatric acute myeloid leukemia. Blood. (2021) 138:1009–18. doi: 10.1182/blood.2021011694
18. Wang M, Bu J, Zhou M, Sido J, Lin Y, Liu G, et al. CD8+ T cells expressing both PD-1 and TIGIT but not CD226 are dysfunctional in acute myeloid leukemia (AML) patients. Clin Immunol. (2018) 190:64–73. doi: 10.1016/j.clim.2017.08.021
19. Zhang X, Zhang H, Chen L, Feng Z, Gao L, Li Q. TIGIT expression is upregulated in T cells and causes T cell dysfunction independent of PD-1 and Tim-3 in adult B lineage acute lymphoblastic leukemia. Cell Immunol. (2019) 344:103958. doi: 10.1016/j.cellimm.2019.103958
20. Gebhardt T, Wakim LM, Eidsmo L, Reading PC, Heath WR, Carbone FR. Memory T cells in nonlymphoid tissue that provide enhanced local immunity during infection with herpes simplex virus. Nat Immunol. (2009) 10:524–30. doi: 10.1038/ni.1718
21. Masopust D, Choo D, Vezys V, Wherry EJ, Duraiswamy J, Akondy R, et al. Dynamic T cell migration program provides resident memory within intestinal epithelium. J Exp Med. (2010) 207:553–64. doi: 10.1084/jem.20090858
22. MacKay LK, Rahimpour A, Ma JZ, Collins N, Stock AT, Hafon ML, et al. The developmental pathway for CD103+ CD8+ tissue-resident memory T cells of skin. Nat Immunol. (2013) 14:1294–301. doi: 10.1038/ni.2744
23. Milner JJ, Toma C, He Z, Kurd NS, Nguyen QP, McDonald B, et al. Heterogenous populations of tissue-resident CD8+ T cells are generated in response to infection and Malignancy. Immunity. (2020) 52:808–824.e7. doi: 10.1016/j.immuni.2020.04.007
24. Gebhardt T, Whitney PG, Zaid A, Mackay LK, Brooks AG, Heath WR, et al. Different patterns of peripheral migration by memory CD4+ and CD8+ T cells. Nature. (2011) 477:216–9. doi: 10.1038/nature10339
25. Teijaro JR, Turner D, Pham Q, Wherry EJ, Lefrançois L, Farber DL. Cutting edge: Tissue-retentive lung memory CD4 T cells mediate optimal protection to respiratory virus infection. J Immunol. (2011) 187:5510–4. doi: 10.4049/jimmunol.1102243
26. von Hoesslin M, Kuhlmann M, de Almeida GP, Kanev K, Wurmser C, Gerullis AK, et al. Secondary infections rejuvenate the intestinal CD103+ tissue-resident memory T cell pool. Sci Immunol. (2022) 7:eabp9553. doi: 10.1126/sciimmunol.abp9553
27. Mami-Chouaib F, Blanc C, Corgnac S, Hans S, Malenica I, Granier C, et al. Resident memory T cells, critical components in tumor immunology. J Immunother Cancer. (2018) 6:87. doi: 10.1186/s40425-018-0399-6
28. Luoma AM, Suo S, Wang Y, Gunasti L, Porter CBM, Nabilsi N, et al. Tissue-resident memory and circulating T cells are early responders to pre-surgical cancer immunotherapy. Cell. (2022) 185:2918–2935.e29. doi: 10.1016/j.cell.2022.06.018
29. Hu W, Sun R, Chen L, Zheng X, Jiang J. Prognostic significance of resident CD103+CD8+T cells in human colorectal cancer tissues. Acta Histochem. (2019) 121:657–63. doi: 10.1016/j.acthis.2019.05.009
30. Oliveira G, Egloff AM, Afeyan AB, Wolff JO, Zeng Z, Chernock RD, et al. Preexisting tumor-resident T cells with cytotoxic potential associate with response to neoadjuvant anti-PD-1 in head and neck cancer. Sci Immunol. (2023) 8:eadf4968. doi: 10.1126/sciimmunol.adf4968
31. Corgnac S, Malenica I, Mezquita L, Auclin E, Voilin E, Kacher J, et al. CD103+CD8+ TRM cells accumulate in tumors of anti-PD-1-Responder lung cancer patients and are tumor-reactive lymphocytes enriched with Tc17. Cell Rep Med. (2020) 1:100127. doi: 10.1016/j.xcrm.2020.100127
32. Laumont CM, Wouters MCA, Smazynski J, Gierc NS, Chavez EA, Chong LC, et al. Single-cell profiles and prognostic impact of tumor-infiltrating lymphocytes coexpressing CD39, CD103, and PD-1 in ovarian cancer. Clin Cancer Res. (2021) 27:4089–100. doi: 10.1158/1078-0432.CCR-20-4394
33. Pearce H, Croft W, Nicol SM, Margielewska-Davies S, Powell R, Cornall R, et al. Tissue-resident memory T cells in pancreatic ductal adenocarcinoma Coexpress PD-1 and TIGIT and functional inhibition is reversible by dual antibody blockade. Cancer Immunol Res. (2023) 11:435–49. doi: 10.1158/2326-6066.CIR-22-0121
34. Jiang F, Mao M, Jiang S, Jiao Y, Cao D, Xiang Y. PD-1 and TIGIT coexpressing CD8 + CD103 + tissue-resident memory cells in endometrial cancer as potential targets for immunotherapy. Int Immunopharmacol. (2024) 127:111381. doi: 10.1016/j.intimp.2023.111381
35. Lai W, Wang X, Liu L, Xu L, Mao L, Tan J, et al. Single-cell profiling of T cells uncovers a tissue-resident memory-like T-cell subset associated with bidirectional prognosis for B-cell acute lymphoblastic leukemia. Front Immunol. (2022) 13:957436. doi: 10.3389/fimmu.2022.957436
36. Nose Y, Saito T, Yamamoto K, Yamashita K, Tanaka K, Yamamoto K, et al. The tissue-resident marker CD103 on peripheral blood T cells predicts responses to anti-PD-1 therapy in gastric cancer. Cancer Immunol Immunother. (2023) 72:169–81. doi: 10.1007/s00262-022-03240-2
37. Daver N, Alotaibi AS, Bücklein V, Subklewe M. T-cell-based immunotherapy of acute myeloid leukemia: Current concepts and future developments. Leukemia. (2021) 35:1843–63. doi: 10.1038/s41375-021-01253-x
38. Anadon CM, Yu X, Hänggi K, Biswas S, Chaurio RA, Martin A, et al. Ovarian cancer immunogenicity is governed by a narrow subset of progenitor tissue-resident memory T cells. Cancer Cell. (2022) 40:545–557.e13. doi: 10.1016/j.ccell.2022.03.008
39. Jin K, Yu Y, Zeng H, Liu Z, You R, Zhang H, et al. CD103+CD8+ tissue-resident memory T cell infiltration predicts clinical outcome and adjuvant therapeutic benefit in muscle-invasive bladder cancer. Br J Cancer. (2022) 126:1581–8. doi: 10.1038/s41416-022-01725-6
40. Liao Z, Lv X, Liu S, He Z, Chen S, Wang L, et al. Different aberrant expression pattern of immune checkpoint receptors in patients with PTCL and NK/T-CL. Asia Pac J Clin Oncol. (2018) 14:e252–8. doi: 10.1111/ajco.12850
41. Mahnke K, Enk AH. TIGIT-CD155 interactions in melanoma: A novel co-inhibitory pathway with potential for clinical intervention. J Invest Dermatol. (2016) 136:9–11. doi: 10.1016/j.jid.2015.10.048
42. Johnston RJ, Comps-Agrar L, Hackney J, Yu X, Huseni M, Yang Y, et al. The immunoreceptor TIGIT regulates antitumor and antiviral CD8(+) T cell effector function. Cancer Cell. (2014) 26:923–37. doi: 10.1016/j.ccell.2014.10.018
43. Xu L, Liu L, Yao D, Zeng X, Zhang Y, Lia J, et al. PD-1 and TIGIT are highly co-expressed on CD8+ T cells in AML patient bone marrow. Front Oncol. (2021) 11:686156. doi: 10.3389/fonc.2021.686156
44. Banta KL, Xu X, Chitre AS, Au-Yeung A, Takahashi C, O’Gorman WE, et al. Mechanistic convergence of the TIGIT and PD-1 inhibitory pathways necessitates co-blockade to optimize anti-tumor CD8+ T cell responses. Immunity. (2022) 55:512–526.e9. doi: 10.1016/j.immuni.2022.02.005
45. Huang S, Liang C, Zhao Y, Deng T, Tan J, Zha X, et al. Increased TOX expression concurrent with PD-1, Tim-3, and CD244 expression in T cells from patients with acute myeloid leukemia. Cytom B-Clin Cytom. (2022) 102:143–52. doi: 10.1002/cyto.b.22049
46. Yang G, Cai S, Hu M, Li C, Yang L, Zhang W, et al. Spatial features of specific CD103+CD8+ tissue-resident memory T cell subsets define the prognosis in patients with non-small cell lung cancer. J Transl Med. (2024) 22:27. doi: 10.1186/s12967-023-04839-4
47. Ribas A, Shin DS, Zaretsky J, Frederiksen J, Cornish A, Avramis E, et al. PD-1 blockade expands intratumoral memory T cells. Cancer Immunol Res. (2016) 4:194–203. doi: 10.1158/2326-6066.CIR-15-0210
48. Höpken UE, Droese J, Li JP, Joergensen J, Breitfeld D, Zerwes HG, et al. The chemokine receptor CCR7 controls lymph node-dependent cytotoxic T cell priming in alloimmune responses. Eur J Immunol. (2004) 34:461–70. doi: 10.1002/eji.200324690
Keywords: leukemia, T cell exhaustion, immune checkpoints, tissue resident memory T cells, CD103+CD8+ T cells, PD-1, TIGIT
Citation: Liu L, Lai W, Zhuo X, Chen S, Luo X and Tan H (2024) Higher frequency of peripheral blood CD103+CD8+ T cells with lower levels of PD-1 and TIGIT expression related to favorable outcomes in leukemia patients. Front. Immunol. 15:1437726. doi: 10.3389/fimmu.2024.1437726
Received: 24 May 2024; Accepted: 02 September 2024;
Published: 25 September 2024.
Edited by:
Jiaxiong Tan, Tianjin Medical University Cancer Institute and Hospital, ChinaReviewed by:
Cunte Chen, South China University of Technology, ChinaCopyright © 2024 Liu, Lai, Zhuo, Chen, Luo and Tan. This is an open-access article distributed under the terms of the Creative Commons Attribution License (CC BY). The use, distribution or reproduction in other forums is permitted, provided the original author(s) and the copyright owner(s) are credited and that the original publication in this journal is cited, in accordance with accepted academic practice. No use, distribution or reproduction is permitted which does not comply with these terms.
*Correspondence: Xiaodan Luo, eGRsdW9AZ3pobXUuZWR1LmNu; Huo Tan, dGFuaHVvLjIwMDhAMTYzLmNvbQ==
†These authors have contributed equally to this work and share first authorship
Disclaimer: All claims expressed in this article are solely those of the authors and do not necessarily represent those of their affiliated organizations, or those of the publisher, the editors and the reviewers. Any product that may be evaluated in this article or claim that may be made by its manufacturer is not guaranteed or endorsed by the publisher.
Research integrity at Frontiers
Learn more about the work of our research integrity team to safeguard the quality of each article we publish.