- 1Graduate Program in Tropical Diseases, Botucatu Medical School (FMB), São Paulo State University (UNESP), Botucatu, São Paulo, Brazil
- 2Department of Bioprocess and Biotechnology, School of Agriculture, Agronomic Sciences School, São Paulo State University (UNESP), Botucatu, São Paulo, Brazil
- 3Center for the Study of Venoms and Venomous Animals of UNESP (CEVAP), São Paulo State University (UNESP), Botucatu, São Paulo, Brazil
- 4Minas Gerais Toxicological Information and Assistance Center, João XXIII Hospital, Belo Horizonte, Minas Gerais, Brazil
- 5Graduate Program in Tropical Medicine of the State University of Amazonas, Manaus, Amazonas, Brazil
- 6Department of Clinical Analysis, School of Pharmaceutical Sciences, São Paulo State University (UNESP), Araraquara, São Paulo, Brazil
- 7Center for Translational Science and Development of Biopharmaceuticals FAPESP/CEVAP-UNESP, Botucatu, São Paulo, Brazil
Envenoming resulting from Apis honeybee stings pose a neglected public health concern, with clinical complications ranging from mild local reactions to severe systemic manifestations. This review explores the mechanisms underlying envenoming by honeybee sting, discusses diagnostic approaches, and reviews current pharmacological interventions. This section explores the diverse clinical presentations of honeybee envenoming, including allergic and non-allergic reactions, emphasizing the need for accurate diagnosis to guide appropriate medical management. Mechanistic insights into the honeybee venom’s impact on physiological systems, including the immune and cardiovascular systems, are provided to enhance understanding of the complexities of honeybee sting envenoming. Additionally, the article evaluates emerging diagnostic technologies and therapeutic strategies, providing a critical analysis of their potential contributions to improved patient outcomes. This article aims to provide current knowledge for healthcare professionals to effectively manage honeybee sting envenoming, thereby improving patient care and treatment outcomes.
1 Introduction
Africanized bees have displayed remarkable adaptability in the Americas representing a great public health concern for humans due to their propensity to attack even in mildly provoked situations. They tend to exhibit a high number of bees that attack from unusually far distances from the hive, persistently pursue their targets for extended periods, and release larger volumes of venom compared to other bee species (1–3). Accidents involving Africanized bees can lead to various clinical manifestations, determined by an individual’s sensitivity to the venom and the number of stings. The most common scenario occurs when an individual not sensitized to the venom receives a few stings. In such cases, the symptoms are typically limited to a localized inflammatory reaction, characterized by redness, pain, and local warmth. Often, these symptoms resolve without the need for medical intervention. Another presentation arises when an individual previously sensitized to one or more components of the venom experiences an immediate Gell and Coombs type I hypersensitivity reaction. This is a severe occurrence that can be triggered by just one sting, necessitating urgent medical attention. Manifestations include swelling of the glottis, bronchospasm, and anaphylactic shock. The third form of presentation results from multiple stings, leading to envenoming with a substantial amount of venom in the body. Alongside local signs such as pain, bleeding, bruising, redness, jaundice, and increased blood flow (hyperemia), these instances are frequently reported (4–8). In addition, systemic manifestations like difficulty breathing (dyspnea), neuroparalytic symptoms, intense burning headaches, nausea, vomiting, weakness (asthenia), muscle and joint pain, severe tremors, kidney failure, rhabdomyolysis, and shock (4–8) can occur due to the diverse fractions of the venom. Incidents leading to these symptoms are not uncommon (2, 9).
2 Epidemiology, bee venom, and clinical manifestations
Africanized bees emerged in Brazil during the 1950s when beekeepers introduced African bees (Apis mellifera scutellata) to the country. Renowned for their high productivity and disease resistance (10), these bees accidentally escaped and hybridized with European honey bees (Apis mellifera mellifera), established in Brazil since the early 19th century. The resulting hybrids demonstrated exceptional adaptability to the tropical climate, rapidly spreading throughout the Americas, excluding Canada (9, 11).
The success of Africanized bees in the Americas is attributed to ecological and genetic advantages over native pollinators, including higher reproductive rates, shorter development cycles, increased drone production, swarming frequency, enhanced disease resistance, and less selective nesting site choice (2, 10). A concerning trend is the escalating incidence of honeybee encounters in urban environments. This increase is primarily linked to pesticide use, deforestation, and declining floral resources, exacerbated by the proximity of bee habitats to human settlements (12). Africanized bee stings occur four to ten times more frequently than those of European bees, often involving group attacks (3, 11). Their extended pursuit of threats and increased venom delivery compared to other bee species (3) pose significant public health risks, leading Brazilian health authorities to classify bee-related incidents as a public health surveillance priority (13).
In Brazil, between 2013 and 2023, there were 206.746 reported cases of bee stings, with a notable increase in the year 2023, where 33,317 cases were reported, exceeding the number of snake cases (32,420 cases) leading to 649 direct fatalities and an additional 50 deaths indirectly attributed to bee stings (Figure 1A). As with envenoming by other venomous animals, the number of cases of envenoming by bees varies between Brazilian states (Figures 1B, C), although clinical and epidemiological studies are scarce (7, 14). It is estimated that the lethality rate is about 0.29%, with an annual rate average incidence of 6.89 per 100,000 inhabitants (15), although a temporal increase in reported cases is observed (6, 13, 14, 16, 17). In Amazonas State, the geographical landscape is cited as a contributing factor to the worsening of patients’ conditions (18). This assertion holds merit, considering the geographic obstacles and challenges encountered in route to medical assistance, often leading to fatal outcomes before reaching proper medical care (19). Most reported cases are concentrated in the Northeast, Southeast, and South regions (Figures 1B, C), affecting mainly men (Figure 1D) people of color (Figure 1E). However, the majority of cases are mild (Figure 1F) and progress towards cure (Figure 1G). However, the impact on patient health in moderate and severe cases is unknown. This is substantiated by the high population density and the diminishing presence of natural vegetation on mountains, in landfills, slums, and urban conglomerates, which can serve as habitats for bees. Consequently, these conditions may provoke bee swarms to launch extensive attacks (20, 21).
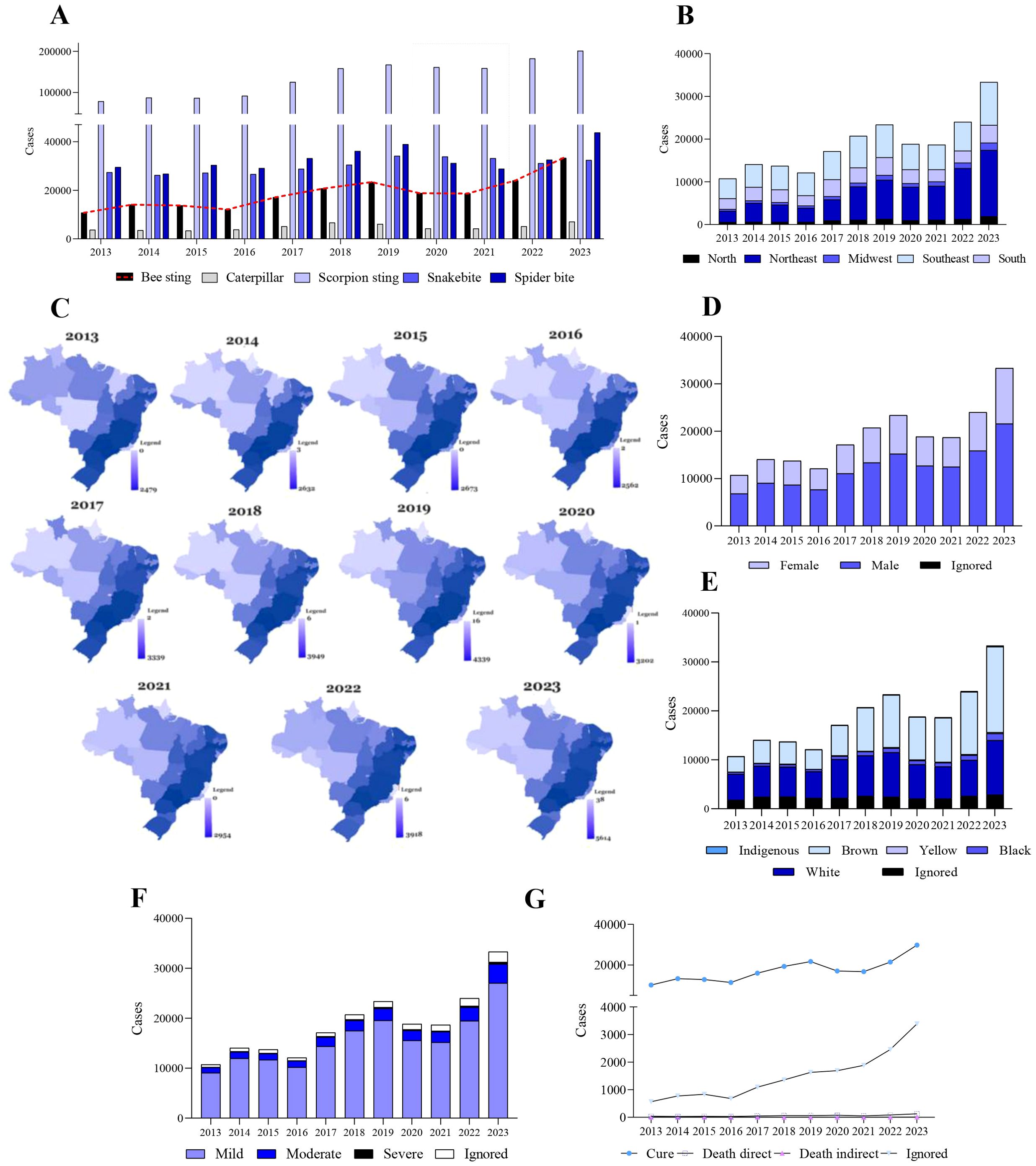
Figure 1. Reported bee accidents and related deaths in Brazil (2013–2023) by state. (A) Bee accidents in Brazil per year. Except for 2020 and 2021, the others showed an increasing trend in cases. This drop refers to the years of the pandemic caused by the Sars-Cov-2 virus. (B) Annual reported bee accidents related to bee stings per region. (C) Distribution of cases of bee accidents according to state. (D) Distribution of cases of accidents caused by bees according to sex. (E) Distribution of cases of accidents caused by bees according to race. (F) Distribution of cases of accidents caused by bees according to clinical classification. (G) Distribution of cases of accidents caused by bees according to outcome. Graphs were produced using GraphPad Prism 9.0 software.
The Apis genus is responsible for most accidents, often resulting in severe outcomes and fatalities (22). Their venom is a complex mixture of proteins, low-molecular-weight peptides, amines, water, and mineral salts. Notably, melittin, phospholipase A2 (PLA2), hyaluronidase, apamin, and mast cell degranulating peptide are highly toxic components (23, 24). Various analytical methods, including electrophoresis, HPLC, HPLC-MS, GC-MS, liquid scintillation counting, ICP-MS, and stripping voltammetry, have been employed to characterize bee venom (25). Size-exclusion chromatography (SEC-HPLC) under isocratic conditions can identify melittin, apamin, and MCDP. However, due to the melittin/apamine ratio (30:1) and the venom’s chemical complexity, reverse-phase chromatography with C18 columns (RP-18) is necessary for comprehensive analysis, enabling the identification of apamin, hyaluronidase, MCDP, melittin, PLA2, procamine, tertiapin, and secapin (26). The abundance of A. mellifera venom components is reflected in the intensity of chromatographic peaks, with melittin being the most prominent, followed by PLA2, apamin, and other constituents (27) (Figure 2).
Immediately following a bee sting, the venom delivery system remains embedded in the skin for approximately 30 seconds, allowing for venom release, with at least 90% injected within the first 20 seconds (28). While removing the stinger is a common initial response, it is unlikely to significantly reduce venom toxicity or quantity absorbed. On average, a bee sting injects 140-150 μg of venom, and the median lethal dose (LD50) is 2.8-3.5 mg/kg of body weight (26, 28). Thus, a non-allergic person weighing 60-70 kg could theoretically experience a 50% risk of fatality from 1,000-1,500 stings. However, fatalities have been reported with as few as 200-500 stings. Various factors influence envenoming severity, including time to medical care, age, weight, sting count, and individual characteristics (e.g., immune status, comorbidities, previous sensitization) (29). Individuals with asthma, allergic rhinitis, or a history of bee sting allergies are at increased risk for severe complications (30).
Typically, the clinical manifestations of bee envenomation can be categorized into local inflammatory reactions, allergic reactions, anaphylactic shock, and systemic toxic reactions (3, 9, 29). Initial symptoms are confined to the sting site, often presenting as pain, swelling, redness, and itching. Allergic reactions, classified as type I hypersensitivity reactions, usually occur within 10 minutes of the sting. These reactions can manifest as systemic urticaria, itching, angioedema, vomiting, or diarrhea. In severe cases, allergic reactions may progress to anaphylactic shock, causing bronchoconstriction (26). Importantly, even individuals without allergies can experience anaphylaxis due to systemic mastocytosis.
3 Bee venom: molecular basis of physiopathology
A typical bee sting elicits a local inflammatory response characterized by pain, edema, and erythema. Melittin, a primary bee venom allergen, is a potent inducer of acute pain (31). It has been demonstrated that intradermal melittin (5 μg in 50 μL saline) caused severe, 3-minute pain accompanied by local heat and swelling (32–35). Pain intensity and duration correlate with melittin dose, inducing mechanical hyperalgesia at the sting site and heat-thermal hyperalgesia in the surrounding area (34, 35). Animal studies extend these findings to include mechanical and thermal hyperalgesia, edema, and plasma extravasation lasting 72-96 hours (36–39).
Melittin activates the transient receptor potential vanilloid 1 (TRPV1) channel, a nonselective cation channel in peripheral sensory neurons, contributing to pain (40). TRPV1 also mediates pruritus (itching) (41, 42). Histamine, IL-31, IL-4, and cyclooxygenase (COX), lipoxygenase (LOX), and PLA2 pathway products stimulate TRPV1, promoting itching (31, 43). Another mechanism by which melittin induces itching is via serotonin release due to pore formation and mast cell degranulation (31), as well as by increased transcriptional regulation of voltage-gated sodium channels in neurons associated with itch (44, 45). Additionally, melittin enhances nociceptor activity by modulating G protein-coupled receptors (GPCRs), leading to hyperalgesia and allodynia (31).
Another critical effect of venom components, particularly allergens, is hemorrhage. While primarily associated with IgE-mediated anaphylaxis, allergens can also trigger non-IgE-dependent inflammatory responses (46). Bradykinin (BK), whose production increases during envenomation due to melittin’s action, contributes to anaphylactic symptoms. Melittin directly activates PLA2, mimicking BK’s effects on tracheal tone and inducing angioedema (47), leading to airway obstruction, asphyxia, and severe gastrointestinal symptoms resembling acute abdomen (46).
A complex interplay between inflammation and coagulation arises, as plasma kinin formation cascade activation results in factor XII binding, autoactivation, and conversion of prekallikrein to kallikrein. Kallikrein cleaves high-molecular-weight kininogen, releasing vasoactive BK (48). BK subsequently activates and modulates coagulation, particularly factor XII (49). Both BK and BK 1-5 inhibit thrombin-induced platelet aggregation, while thrombin plays a crucial role in coagulation and platelet activation (50, 51). Additionally, BK enhances nitric oxide (NO) production in endothelial cells, inhibiting thrombocyte adhesion through angiotensin II blockade (52)
Interestingly, BK induces prolonged thrombolysis via the bradykinin B2 receptor (B2) and prostacyclin (PGI2) (53, 54). This reveals BK’s potential to activate plasminogen, which can be antagonized by angiotensin-converting enzyme (ACE) inhibitors through amplified tissue plasminogen activator effects (53, 55). ACE inhibition modulates fibrinolytic balance, with angiotensin II-mediated increases in plasminogen activation inhibitor-1 playing a crucial role (56). Consequently, coagulation disorders, including decreased fibrinogen activity and prolonged prothrombin and partial thromboplastin times, have been reported in experimental and clinical bee venom envenomation (57).
In summary, melittin induces BK release and ACE dysfunction, leading to coagulation and fibrinolysis imbalances. Additionally, impaired vascular smooth muscle contractility following envenomation exacerbates hemorrhagic episodes (57, 58). Elevated BK levels contribute to reduced vascular tone through epithelial effects (48, 52), while melittin and PLA2 may further decrease muscle contractility, potentially inducing hemorrhage.
Bee venom PLA2 hydrolyzes phosphatidylcholine, phosphatidylethanolamine, phosphatidylinositol, and phosphatidylserine 2-acyl bonds, disrupting plasma membrane integrity. This process releases lysophospholipids and fatty acids, inducing inflammation and further membrane damage. Notably, purified PLA2 lacks hydrolytic activity against erythrocyte membrane phospholipids, but its combination with melittin synergistically enhances hemolysis beyond that achievable by either component alone (59).
Skeletal and cardiac muscle cell membranes exhibit greater resistance to PLA2, although both crude venom and melittin exert toxic effects on the cardiovascular system. The exact mechanisms underlying these effects and potential contributions from other venom components remain unclear (60). Melittin initially increases, then decreases the spontaneous beating rate of cultured cardiac myocytes, ultimately causing their degeneration (61).
Wistar rats exhibited electrocardiographic (ECG) changes, enzyme alterations, and morphological lesions resembling acute myocardial infarction (AMI) type 8, suggesting a direct toxic effect of the venom on cardiac muscle, primarily attributed to melittin (62, 63). Bee venom induces extensive endothelial damage, collagen degradation, and smooth muscle cell migration within the aorta (64). Melittin and apamin provoke coronary artery vasospasm, facilitating platelet aggregation and thrombosis. At lower concentrations, melittin induces transient relaxation through an endothelium-dependent mechanism involving NO production and activation of smooth muscle charybdotoxin-sensitive K+ channels, but at higher concentrations, it causes contraction. Apamin, while not directly affecting coronary artery contraction or relaxation, inhibits NO and prostanoid production, modulating the coronary artery’s relaxation response to melittin via smooth muscle apamin-sensitive K+ channels (65). Although bee venom triggers endogenous vasodilatory amine release, paradoxical coronary vasoconstriction remains possible, especially in the context of endothelial damage, potentially leading to acute coronary syndrome (66).
Africanized bee venom induces myonecrosis both in vivo and in vitro (67). Specifically, melittin triggers phospholipid breakdown, generating free fatty acids and diacylglycerol in equine and human skeletal muscle primary cultures. At higher concentrations, it also breaks down triglycerides. Additionally, melittin alters calcium (Ca2+) release thresholds in skeletal muscle terminal cisternae fractions (68). Membrane rupture initiates a cascade of intracellular changes, with increased intracellular calcium levels being a critical factor in subsequent cellular dysfunction and death. Melittin and PLA2 synergistically contribute to skeletal muscle cell myonecrosis (69).
Apis mellifera venom contains a protein with a conserved C1q domain (70). C1q activates the classical complement pathway by binding to antibody Fc regions, leading to C1r and C1s activation. Subsequently, C1s cleaves C4, generating pro-inflammatory anaphylatoxins C4a and C4b (71). While C1q is present in A. mellifera venom, recombinant C1q failed to recognize IgE from bee venom allergic patients (70), suggesting a need for further investigation into its role in complement activation during bee stings.
Interestingly, melittin’s hydrophilic head shares similarities with the C1q sequence. Envenomation induces melittin-mediated, antigen-independent IgG and C1q aggregation, triggering the classical pathway and producing anaphylatoxins C3a and C5a (72). C5a rapidly induces physiological responses, including mast cell degranulation, potentially leading to fatal anaphylaxis (73). Bee sting envenomation elicits acute allergic and inflammatory responses. A. mellifera venom and melittin activate the NLRP3 inflammasome, leading to procaspase-1 cleavage and neutrophil recruitment to the sting site. Mast cells play a protective role by degrading and neutralizing A. mellifera toxins post-degranulation (74). Melittin activates the 5-lipoxygenase pathway in neutrophils, releasing arachidonate and inducing neutrophilia (75, 76). Additionally, PLA2 from Apis mellifera lamarckii (Egyptian honeybee) venom hydrolyzes phosphatidylcholine, inhibits platelet aggregation, and impairs blood coagulation by inhibiting the extrinsic pathway (77).
4 Role of oxidative stress, inflammation, and coagulation
Redox homeostasis alterations in venomous animal envenomation victims contribute to secondary or long-term complications, with oxidative stress being a key factor. Bee sting envenomation disrupts hepatic metabolism, elevating plasma alanine transaminase (ALT) and aspartate aminotransferase (AST)levels, indicating hepatotoxicity. Additionally, it induces caspase-1 activation and pro-inflammatory molecule secretion through H2O2 overproduction (78).
One of the most reported clinical complications following bee sting envenomation is ischemic stroke with hemorrhagic transformation. Although the pathophysiology remains unclear, proposed mechanisms involve systemic immune-mediated vasoconstriction and a prothrombotic state, leading to ischemia and subsequent stroke (79), similar to other venomous animal envenomations (80). These events occur through platelet aggregation, coagulation cascade activation via tissue thromboplastin release from damaged tissue phospholipids (81), and rapid declines in platelet count with coagulopathy (82). Combined with hemolysis and endothelial damage, widespread fibrin thrombi formation can lead to vessel occlusion, progressing to hemorrhagic or occlusive transformations.
Apis mellifera venom targets various cells, with hemolysis and rhabdomyolysis being particularly damaging due to oxidative stress, primarily mediated by melittin and PLA2. Hemolysis can occur directly through venom action or indirectly, while rhabdomyolysis is primarily venom-induced but may also involve inflammation, vascular congestion, and edema (83). PLA2-mediated hemolysis results from red blood cell membrane lipid disruption, releasing free hemoglobin (Hb) (83, 84). Free Hb induces macrophage programmed death, shifting macrophage polarization towards a cytotoxic phenotype, exacerbating inflammation and tissue damage (85–87). Additionally, Hb spontaneously oxidizes and reacts with NO, converting hemoglobin to methemoglobin (MtHb).
MtHb is highly pro-oxidant, readily releasing ferric heme, which easily crosses cell membranes and increases oxidative damage. Rhabdomyolysis, another significant contributor to oxidative stress, releases myoglobin, reactive oxygen species (ROS), and uric acid into the bloodstream. Myoglobin undergoes oxidation similar to Hb, releasing free iron and inducing free radical formation. These events can collectively damage the liver and kidneys (83).
Ferroptosis, a ROS-dependent cell death characterized by iron accumulation and lipid peroxidation, may contribute to liver and kidney damage (88). Ferroptosis inducers like erastin or RSL3 increase intracellular iron, generate excessive ROS, and enhance lipid peroxidation through lipoxygenase (ALOX) or EGLN prolyl hydroxylases (PHDs) (88, 89). Hepatocellular death, occurring through apoptosis, necrosis, or pyroptosis, is a common response to various liver diseases (90). Animal studies reveal A. mellifera venom-induced sinusoidal and centrilobular congestion, eosinophilia, cytoplasmic vacuolation, intraparenchymal hemorrhage, centrilobular necrosis, and apoptosis (83). Clinically, bee sting envenomation patients exhibit elevated ALT, AST, and bilirubin levels, consistent with hemolysis, thrombotic microangiopathy, and acute liver injury (91). Hepatic vessel occlusion due to microthrombi formation can also lead to ischemia and necrosis (92). Additionally, venom-induced oxidative stress, evidenced by increased malondialdehyde (MDA) and glutathione (GSH) levels, contributes to liver damage (93).
Elevated MDA and GSH levels indicate lipid peroxidation in liver tissue, a process mediated by ROS generated through various mechanisms, including neutrophil activity (94). This lipid peroxidation serves as a pivot for inflammation, as evidenced by increased TNF-α levels in liver tissue (93). The potential involvement of neutrophil extracellular traps (NETs) in this process warrants further investigation. Nox enzyme-dependent NET formation is well-characterized, involving increased ROS production, neutrophil granule and nuclear membrane disintegration, and the release of neutrophil elastase (NE) and myeloperoxidase (MPO) (95). NE and MPO interact with the neutrophil nucleus, cleaving histones and facilitating chromatin decondensation, ultimately leading to the release of DNA decorated with granular content into the extracellular environment for antigen capture (96, 97). While NET formation has been observed in snakebite envenomation (98), its role in multiple bee sting envenomation remains unexplored.
Oxidative stress induced by bee venom contributes to acute kidney injury. Bee venom and melittin disrupt renal cell redox homeostasis by inhibiting α-MG uptake through a PLA2-oxidative stress-Ca2+ pathway (99). This process involves increased arachidonic acid and lipid peroxide production, along with elevated Ca2+ uptake, suggesting a link between PLA2 activation, Ca2+, and oxidative stress in renal cells (99). Acute kidney injury, primarily affecting proximal tubules, is a common complication of bee sting envenomation (100, 101). Redox imbalance, characterized by lipid peroxidation and membrane protein denaturation, leading to altered membrane fluidity, enzyme function, and ion transport, plays a crucial role in renal failure pathogenesis. The kidney’s high sensitivity to oxidative stress due to its rich polyunsaturated fatty acid content renders it susceptible to tubular necrosis caused by bee venom-induced redox imbalance (102).
Disseminated intravascular coagulation (DIC) with associated thrombocytopenia presents another potential mechanism for kidney injury. Despite its prevalence, effective treatment and management strategies remain limited. Given platelets’ sensitivity to external stimuli, including ROS, their interplay with oxidative stress is plausible, especially considering platelet apoptosis exacerbates oxidative stress induced by the hemorrhagic, hemolytic, and necrotic effects of bee venom components. Numerous case studies have documented initial thrombocytopenia and alterations in hemostatic and renal systems. Platelets play a crucial role in thromboinflammation (80), explaining the pathogenesis of renal changes in bee sting envenomation.
Roodt et al. (83) reported pulmonary congestion, septal enlargement, atelectasis, emphysema, intra-alveolar hemorrhagic foci, and arterial lesions with acute edema in mice following experimental envenomation with Apis mellifera mellifera venom from different regions of Buenos Aires, Argentina. While pulmonary alterations in bee envenomation remain unclear clinically, proposed mechanisms include pro-thrombotic state-induced congestion due to platelet aggregates, fibrin deposition, and erythrocyte accumulation, similar to snake envenomation. Pulmonary edema may result from catecholamine-induced myocarditis, myocardial ischemia due to coronary vasoconstriction, and direct cardiotoxin effects on the myocardium (103–106). Additionally, blood leukocyte mobilization, as observed in other venomous animal envenomations, could contribute to acute lung injury.
5 Clinical complications in honeybee stings envenoming
A wide array of clinical complications can arise from multiple bee stings (Figure 3). These complications manifest locally or systemically, with variable onset, organ involvement, and overall impact. While frequently reported, the underlying pathogenesis of rarer reactions remains largely undefined (Table 1).
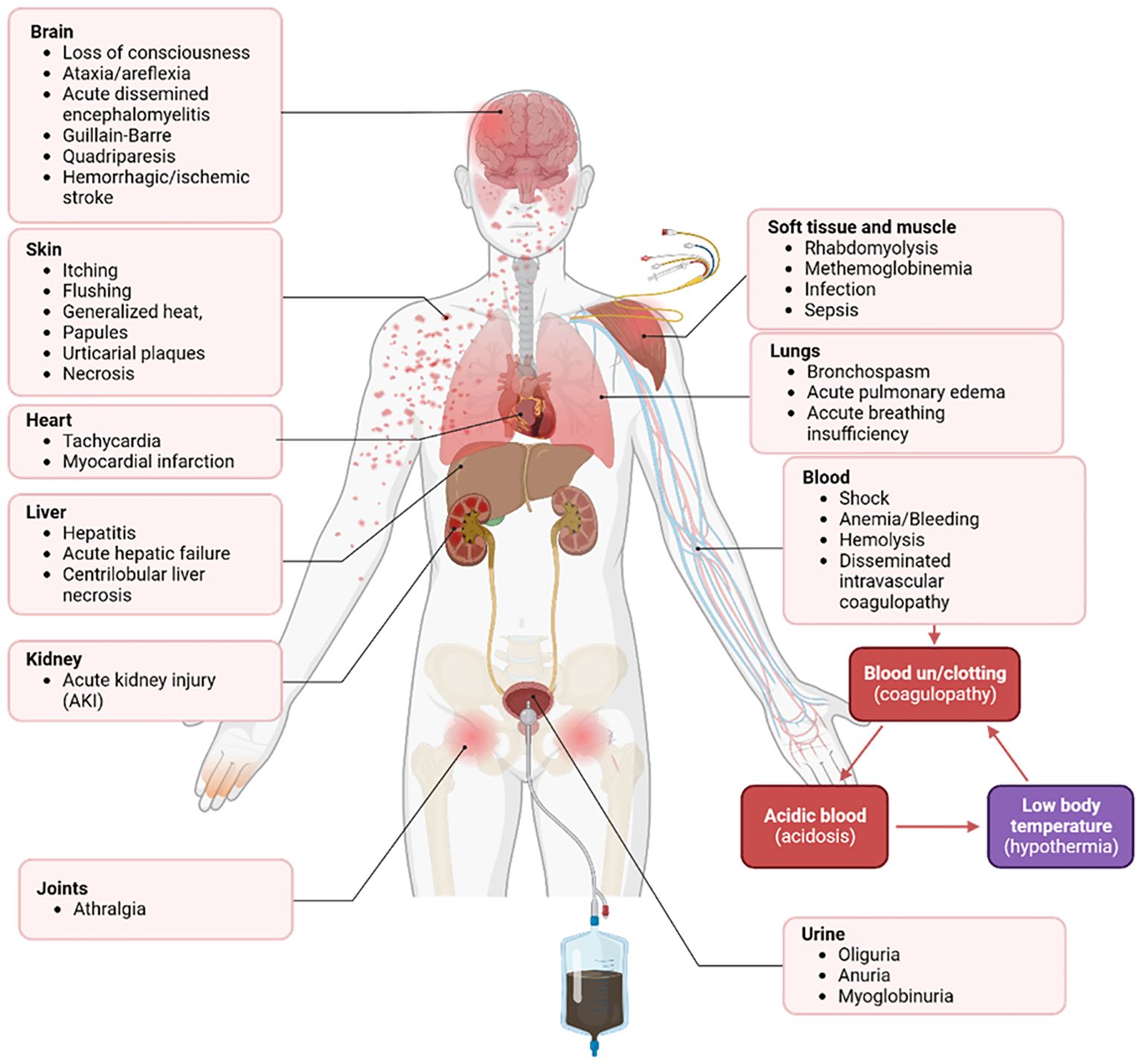
Figure 3. Spectrum of severe systemic effects from bee stings. Multiple bee stings trigger highly severe systemic reactions, exhibiting a broad spectrum of clinical manifestations.
Melittin, phospholipase A2, apamin, and hyaluronidase are the primary toxic components of Apis mellifera bee venom. These substances significantly contribute to the development of clinical complications following multiple bee stings (192). The cardiovascular system is particularly vulnerable, with ischemic events, including acute myocardial infarction and Kounis syndrome (allergy-induced acute coronary syndrome), being common sequelae. Other potential cardiac complications include takotsubo cardiomyopathy, atrial fibrillation, and cardiac damage (193, 194).
Hemorrhage can occur in various locations following a bee sting, including the digestive, nervous, and respiratory systems, potentially leading to gastrointestinal, subarachnoid, or pulmonary hemorrhage, respectively. Additionally, hematological complications such as ischemia, anemia, thrombosis, hemolysis, disseminated intravascular coagulation (DIC), and shock, often culminating in hypovolemia, may arise. While hemolysis occurs in 17-22% of wasp sting cases, DIC is less common but can trigger thromboplastin release and microthrombi formation (192, 194).
Bee stings can induce hepatitis due to liver damage, manifested by elevated transaminases, alkaline phosphatases, and bilirubin levels. However, rhabdomyolysis and cardiac damage can also increase transaminases, complicating liver injury diagnosis. Severe liver damage may progress to liver failure. In rats, melittin has been implicated in liver injury through vasoconstriction and glycogenolysis (194).
Anaphylactic shock, an IgE-mediated immune response causing hypoperfusion and vasodilation, poses a severe risk to individuals with previous bee sting exposure or allergies. This life-threatening condition can lead to organ injury and death. Additionally, mast cell activation syndrome, characterized by excessive mast cell production and inflammatory effects, can trigger multisystem complications, organ failure, and mortality rates exceeding those of anaphylaxis (194).
Rhabdomyolysis, characterized by skeletal muscle breakdown, is frequently associated with bee envenoming, evidenced by elevated creatine phosphokinase (CPK) and bilirubin levels, and can contribute to acute kidney injury (AKI) (18, 192). Hemiparesis, or muscle weakness following ischemic stroke, is another potential muscular complication leading to immobilization or reduced physical activity (195).
Bee sting envenomation can lead to various neurological complications, including stroke (both ischemic and hemorrhagic), behavioral changes, ataxia, areflexia, encephalomyelitis, and Guillain-Barré syndrome. Ischemic and hemorrhagic strokes pose significant risks due to their potentially fatal outcomes. Subarachnoid intracranial hemorrhage often complicates ischemic stroke, undergoing hemorrhagic transformation. While the exact mechanisms remain unclear, two primary theories have been proposed: 1) an immune-mediated systemic reaction causing vasoconstriction and a prothrombotic state leading to ischemia and subsequent stroke; and 2) disseminated intravascular coagulation (DIC) triggered by tissue thromboplastin release, coupled with hemolysis, resulting in vessel occlusion, ischemic stroke, and eventual hemorrhagic transformation (9, 34).
Furthermore, behavioral changes, ataxia, and areflexia typically accompany degeneration or obstruction in specific regions of the brain and cerebellum. In this context, some cases displaying these complications have also experienced strokes, indicating a potential connection (196). It is well-established that the execution of any movement entails the coordinated action of agonist and synergist muscles, which contract to facilitate the movement, while antagonist muscles relax to allow it, and fixator muscles stabilize posture and prevent unintended shifts (196). Hence, considering that bee venom, particularly melittin, induces rhabdomyolysis, it is plausible that the partial loss of motor function in these cases may also be linked to rhabdomyolysis (18, 192).
Rhabdomyolysis, hemolysis, and hypotension can adversely affect the renal system, elevating creatine kinase and bilirubin levels, and ultimately leading to acute kidney injury (AKI) and renal failure. Multiple bee sting envenomation often causes glomerular and peritubular vasoconstriction, potentially resulting in ischemic injury and acute tubular necrosis. The accumulation of myoglobin, Tamm-Horsfall proteins, and uric acid within the tubules contributes to cast formation, further obstructing tubules and inducing ischemia. Anuria and hematuria are additional associated renal complications (192).
Clinical respiratory complications include acute pulmonary edema, pulmonary hemorrhage, and acute respiratory distress syndrome (ARDS). Bee venom’s ability to increase vascular permeability contributes to edema formation in various tissues, including the lungs, trachea, conjunctiva, and subcutaneous areas (194). Alveolar capillary damage can precipitate diffuse alveolar hemorrhage (DAH), potentially progressing to ARDS (197, 198). The pathogenesis of ARDS in bee sting envenomation remains unclear, although melittin and phospholipase A2 are suspected contributors to acute lung injury. Venom-induced inflammation can cause extensive tissue damage, culminating in severe cases of ARDS and multisystemic cardiorespiratory arrest (199, 200).
Ocular complications, though uncommon, encompass a wide range of issues. These include eyelid edema, conjunctival congestion, corneal abrasions, optic neuropathy, ptosis, purulent ocular secretions, conjunctival and episcleral hyperemia, symblepharon, macular retinal striae, ciliary congestion, corneal edema with Descemet’s membrane folding, and even vision loss. Additionally, lens abscess, partial iris atrophy, and cataract formation have been reported. The retained stinger’s direct venom action, coupled with the rapid immune response, primarily causes corneal injury (201, 202).
Clinical complications from bee sting envenomation can be severe, life-threatening, and multi-systemic. These complications, potentially triggered by a single sting, allergies, or anaphylaxis, can impact various bodily systems. Moreover, a high number of bee stings can exacerbate these complications, affecting the cardiovascular, nervous, hematological, or respiratory systems (Table 2).
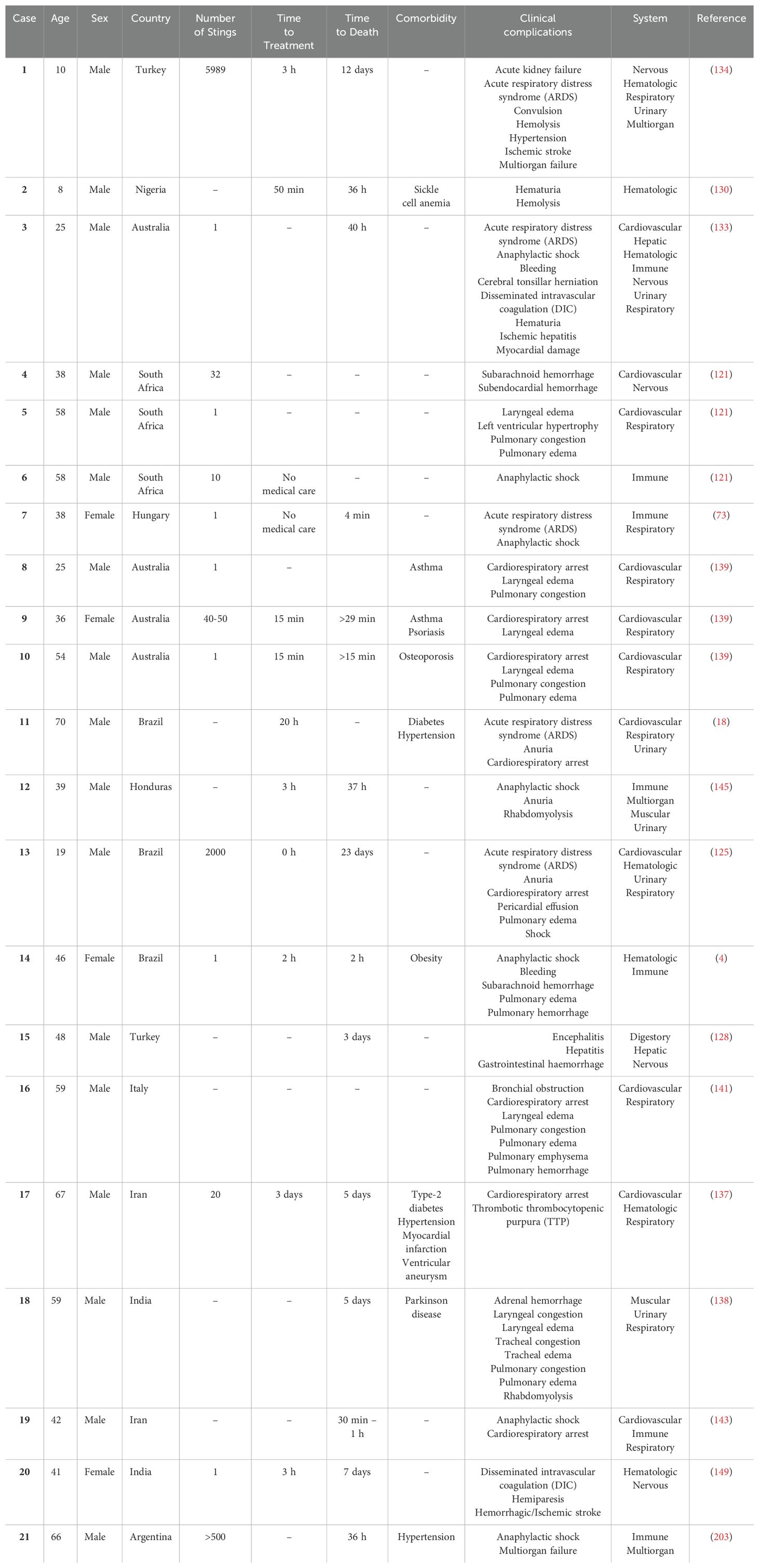
Table 2. Clinical complications associated with honeybee stings envenoming in the entire patient cohort and in patients who died.
Defining envenomation by sting number remains challenging, as the lethal venom dose is 2.8-3.5 mg/kg body weight (26, 28). Severe complications like disseminated intravascular coagulation, hematuria, ischemic hepatitis, myocardial damage, and death have been reported in adults with fewer than 30, even as few as one sting, particularly in sensitized individuals (Table 2). This highlights the unpredictable nature of bee sting envenomation.
Differentiating the causes and assessing the risks of death linked to either the direct toxic effects of venom or the allergic syndrome triggered by honeybee stings poses a formidable challenge, demanding thorough investigation and vigilant patient monitoring. Consequently, all incidents involving bee stings should be regarded as envenoming, and their significance, even in instances involving only a few stings, should not be underestimated. This recognition was underscored during the Apilic antivenom’s phase I/II clinical trial, wherein a recommendation was made to administer two vials of antivenom to patients with more than five stings. For those with fewer than five stings, the use of apilic antivenom is not advised, except when dictated by medical judgment (5).
6 Prevention of bee stings
Preventing severe systemic reactions to bee stings requires a multi-faceted approach. Clothing choices, such as wearing light-colored, smooth-textured garments, can deter bees. Avoiding scented products and maintaining personal hygiene by wearing clean clothes and showering regularly can also reduce the risk of stings. Minimizing exposure to bee-attracting factors, including flowering plants and food remnants, is crucial (204).
When confronted by a solitary stinging insect, remaining calm and still is crucial to avoid provocation. However, if multiple insects attack, swiftly retreating indoors or to a sheltered area is essential. Submerging in water to escape is ill-advised, as some bee species, like Africanized honeybees, may continue to sting upon resurfacing (205).
Individuals with a history of severe allergic reactions to insect stings should carry an epinephrine auto-injector and wear medical identification. Prompt medical attention is crucial in case of an allergic reaction (206). Integrating these preventive measures into daily routines significantly reduces the risk of severe systemic reactions from bee stings, enhancing safety in various environments.
7 Clinical complications diagnostic
Preventive exams identify diseases in asymptomatic individuals. Bee sting envenomations often present with multiple, late-diagnosed complications. Early diagnosis is crucial for effective management and improved outcomes (Supplementary Table 1). Although warning signs exist, monitoring challenges persist for general clinicians (207).
8 Pharmacological interventions
Current management and treatment protocols for honeybee sting envenomation primarily address analgesia and allergic reactions, with limited guidance on other complications. Consequently, treatment options and associated risks for these complications remain scarce. Given the diverse clinical manifestations of honeybee sting envenomation, adapting treatment protocols from other conditions may be beneficial. It is essential to consider both potential benefits and risks when implementing treatment plans, as some interventions may exacerbate complications or cause adverse effects. A comprehensive understanding of treatment options and their associated risks for various clinical complications is crucial for optimal patient care (Supplementary Table 2).
Symptomatic treatments for severe bee sting envenomations often prove ineffective. To address this, the Center for the Study of Venoms and Venomous Animals, Brazil initiated the development of a bee sting antivenom. Initial efforts focused on venom biochemical characterization to understand its composition. Traditional horse-based antivenom production faced challenges due to high allergic reaction rates and anaphylactic shock in horses. To mitigate these risks, researchers explored various strategies, including cobalt-60 irradiation (208). Irradiation significantly altered the chromatographic profiles of native Apis venom. While irradiated venom combined with IFA or SBA-15 produced similar antibody titers compared to native venom and IFA, these titers were notably lower. Importantly, irradiation reduced venom toxicity while preserving its immunogenicity, and IFA enhanced antibody production (208). However, successful immunization required removing all allergenic components from the venom, leaving only melittin and PLA2 for immunization.
Apilic antivenom demonstrated partial neutralization of venom effects, including hematocrit, vascular permeability, myeloperoxidase activity, edema, plasma CK activity, venom phospholipase and hyaluronidase activity, and cytotoxicity in kidney cell cultures (209, 210). These findings indicate the antivenom’s potential efficacy against Africanized bee (A. mellifera) venom and melittin in vivo and in vitro. Subsequent phase I/II clinical trials were initiated to evaluate the antivenom’s safety and efficacy. Given the absence of established clinical protocols for antivenom evaluation, a new protocol was developed and approved by ethics regulatory (The Brazilian Committee of Ethics in Research – CONEP) and sanitation agencies (Brazilian Health Regulatory Agency – ANVISA). This protocol outlined specific, adjuvant, symptomatic, and complementary treatments, in addition to standard clinical trial guidelines for heterologous antivenoms. It represented the first clinical trial specifically designed to assess the efficacy and safety of an Africanized bee venom antivenom (5).
The Apilic antivenom proved to be safe and demonstrated efficacy in neutralizing various venom effects, including hematocrit, vascular permeability, and enzyme activity, both in vivo and in vitro. These findings highlight the antivenom’s potential for treating Africanized bee (A. mellifera) envenomation. At the time, the pharmacokinetics of Africanized bee venom in humans was reported for the first time. The concentrations of melittin and PLA2 varied between 0.03 ng/mL and 587.35 ng/mL during hospitalization and follow-up, and interestingly, it was possible to observe that the blood concentration of PLA2 and mainly melittin increases again, especially after 10 days hospitalization, but without any clinical symptoms (8)). Mass spectrometry assays, allowed to determine the presence and relative levels of melittin in the participants. It should be noted that these issues were expected, as accidents involving AHB are peculiar and different from all other accidents involving venomous animals described. A phase III clinical trial is essential to confirm these observations, optimize dosing, and fully evaluate the antivenom’s efficacy (8).
The Apilic antivenom production process aligns with established antivenom manufacturing standards (211). However, producing pilot batches compliant with Good Manufacturing Practices (GMP) has presented challenges. Small-scale production for clinical trials is particularly difficult for pharmaceutical industries due to the associated risks and investments. Collaborating with Contract Development and Manufacturing Organizations (CDMOs) could facilitate technology transfer and small-batch production (212).
9 Final remarks
The increasing incidence of bee sting envenomation necessitates a comprehensive understanding of its underlying mechanisms, clinical manifestations, and potential complications to inform effective public health interventions. This review elucidates the intricate effects of bee venom and its associated health consequences. While existing research provides valuable insights into venom composition and its impact, clinical management guidelines for bee sting envenomation remain limited. This review fills a critical knowledge gap by providing a comprehensive overview of the clinical implications of bee venom exposure. Our analysis underscores the complexity of venom-human interactions and the severity of potential outcomes. The development of novel treatment strategies, including an antivenom, is imperative to reduce mortality and long-term complications. However, until such interventions become available, alternative pharmacological approaches must be explored, considering their associated risks.
Author contributions
JC: Conceptualization, Data curation, Methodology, Project administration, Writing – original draft. PR: Data curation, Investigation, Writing – review & editing. AP: Data curation, Investigation, Writing – original draft. BJ: Investigation, Visualization, Writing – review & editing. DA: Investigation, Visualization, Writing – review & editing. FP: Writing – review & editing. AA: Writing – review & editing. FC: Visualization, Writing – original draft, Writing – review & editing. MP: Writing – original draft, Writing – review & editing. RF: Formal analysis, Funding acquisition, Project administration, Visualization, Writing – original draft, Writing – review & editing.
Funding
The author(s) declare financial support was received for the research, authorship, and/or publication of this article. The APC was funded by FAPESP Proc 2021/11936-3 (RF). We are thankful to the Coordination of Superior Level Staff Improvement (CAPES) for the scholarship n° 88887.674376/2022-00 (JC) and 88887.826358/2023-00 (DA), and the Fundação de Amparo à Pesquisa do Estado de São Paulo (FAPESP, São Paulo Research Foundation), scholarships to JC n° 2022/16060-1, to AP n° 2023/09921-3, FAPESP Process 2021/11936-3 and 2023/01554-1 (RF). We also thank the The National Council for Scientific and Technological Development (CNPq) scholarship to MP n° 305778/2023-4 and to AP n° 151190/2023-2, and the Fundação de Amparo à Pesquisa do Estado do Amazonas (FAPEAM), scholarship to FC n° 01.02.016301.01070/2023-13. RF is a CNPq PQ1C fellow researcher 303224/2018-5 and CNPq PQ1D research fellow n° 301608/2022-9. This study was financed in part by the Coordenação de Aperfeiçoamento de Pessoal de Nível Superior - Brasil (CAPES) - Finance Code 001.
Conflict of interest
The authors declare that the research was conducted in the absence of any commercial or financial relationships that could be construed as a potential conflict of interest.
Publisher’s note
All claims expressed in this article are solely those of the authors and do not necessarily represent those of their affiliated organizations, or those of the publisher, the editors and the reviewers. Any product that may be evaluated in this article, or claim that may be made by its manufacturer, is not guaranteed or endorsed by the publisher.
Supplementary material
The Supplementary Material for this article can be found online at: https://www.frontiersin.org/articles/10.3389/fimmu.2024.1437413/full#supplementary-material
References
1. Scott Schneider S, DeGrandi-Hoffman G, Smith DR. T HE A FRICAN H ONEY B EE : factors contributing to a successful biological invasion. Annu Rev Entomol. (2004) 49:351–76. doi: 10.1146/annurev.ento.49.061802.123359
2. Ferreira RS, Almeida RAMB, Barraviera SRCS, Barraviera B. Historical perspective and human consequences of africanized bee stings in the americas. J Toxicol Environ Health Part B. (2012) 15:97–108. doi: 10.1080/10937404.2012.645141
3. Pucca MB, Cerni FA, Oliveira IS, Jenkins TP, Argemí L, Sørensen CV, et al. Bee updated: current knowledge on bee venom and bee envenoming therapy. Front Immunol. (2019) 10:2090. doi: 10.3389/fimmu.2019.02090
4. de Oliveira FA, Guimarães JV, dos Reis MA, Teixeira VdePA. Acidente humano por picadas de abelhas africanizadas. Rev Soc Bras Med Trop. (2000) 33:403–5. doi: 10.1590/S0037-86822000000400012
5. Barbosa AN, Boyer L, Chippaux J-P, Medolago NB, Caramori CA, Paixão AG, et al. A clinical trial protocol to treat massive Africanized honeybee (Apis mellifera) attack with a new apilic antivenom. J Venomous Anim Toxins including Trop Dis. (2017) 23:14. doi: 10.1186/s40409-017-0106-y
6. Diniz AG, Belmino JF, Araújo KA, Vieira AT, Leite Rde S. Epidemiology of honeybee sting cases in the state of ceará, northeastern Brazil. Rev Inst Med Trop Sao Paulo. (2016) 58:40–5. doi: 10.1590/S1678-9946201658040
7. Linard AT, Barros R, Sousa J, Leite R. Epidemiology of bee stings in Campina Grande, Paraíba state, Northeastern Brazil. J Venomous Anim Toxins including Trop Dis. (2014) 20:13. doi: 10.1186/1678-9199-20-13
8. Barbosa AN, Ferreira RS, de Carvalho FCT, Schuelter-Trevisol F, Mendes MB, Mendonça BC, et al. Single-arm, multicenter phase I/II clinical trial for the treatment of envenomings by massive africanized honey bee stings using the unique apilic antivenom. Front Immunol. (2021) 12:653151. doi: 10.3389/fimmu.2021.653151
9. Almeida RAM de B, Olivo TET, Mendes RP, Barraviera SRCS, Souza L do R, Martins JG, et al. Africanized honeybee stings: how to treat them. Rev Soc Bras Med Trop. (2011) 44:755–61. doi: 10.1590/S0037-86822011000600020
10. Scott Schneider S, DeGrandi-Hoffman G, Smith DR. The African honey bee: factors contributing to a successful biological invasion. Annu Rev Entomol. (2004) 49:351–76. doi: 10.1146/annurev.ento.49.061802.123359
11. Harpur BA, Kadri SM, Orsi RO, Whitfield CW, Zayed A. Defense Response in Brazilian Honey Bees (Apis mellifera scutellata × spp.) Is Underpinned by Complex Patterns of Admixture. Genome Biol Evol. (2020) 12:1367–77. doi: 10.1093/gbe/evaa128
12. Zaluski R, Kadri SM, de Souza EA, da Silva VMC, da Silva JRC, Rodrigues-Orsi P, et al. Africanized honeybees in urban areas: a public health concern. Rev Soc Bras Med Trop. (2014) 47:659–62. doi: 10.1590/0037-8682-0254-2013
13. de Oliveira SK, Trevisol DJ, Parma GC, Ferreira Júnior RS, Barbosa AN, Barraviera B, et al. Honey bee envenoming in Santa Catarina, Brazil, 2007 through 2017: an observational, retrospective cohort study. Rev Soc Bras Med Trop. (2019) 52. doi: 10.1590/0037-8682-0418-2018
14. Marques MRV, Araújo KAM, Tavares AV, Vieira AA, Leite RS. Epidemiology of envenomation by Africanized honeybees in the state of Rio Grande do Norte, Northeastern Brazil. Rev Bras Epidemiologia. (2020) 23. doi: 10.1590/1980-549720200005
15. Kono IS, Freire RL, Caldart ET, Rodrigues F de S, Santos JA, Freire LGD, et al. Bee stings in Brazil: Epidemiological aspects in humans. Toxicon. (2021) 201:59–65. doi: 10.1016/j.toxicon.2021.08.014
16. Chippaux J-P. Epidemiology of envenomations by terrestrial venomous animals in Brazil based on case reporting: from obvious facts to contingencies. J Venomous Anim Toxins including Trop Dis. (2015) 21:13. doi: 10.1186/s40409-015-0011-1
17. Costa AG, Chaves BA, Murta FLG, Sachett JAG, Sampaio VS, Silva VC, et al. Hymenoptera stings in Brazil: a neglected health threat in Amazonas State. Rev Soc Bras Med Trop. (2018) 51:80–4. doi: 10.1590/0037-8682-0109-2017
18. Mendonça-da-Silva I, Monteiro WM, Sachett JAG, Barbosa ES, Cordeiro-dos-Santos M, Lacerda MVG, et al. Bee sting envenomation severe cases in Manaus, Brazilian Amazon: clinical characteristics and immune markers of case reports. Rev Soc Bras Med Trop. (2021) 54:e20200319. doi: 10.1590/0037-8682-0319-2021
19. Cristino JS, Salazar GM, MaChado VA, Honorato E, Farias AS, Vissoci JRN, et al. A painful journey to antivenom: The therapeutic itinerary of snakebite patients in the Brazilian Amazon (The QUALISnake Study). PloS Negl Trop Dis. (2021) 15:e0009245. doi: 10.1371/journal.pntd.0009245
20. Baum KA, Tchakerian MD, Thoenes SC, Coulson RN. Africanized honey bees in urban environments: A spatio-temporal analysis. Landsc Urban Plan. (2008) 85:123–32. doi: 10.1016/j.landurbplan.2007.10.005
21. de Toledo VDAA, Toral FLB, de Miranda SB, Shiraishi A, Hashimoto JH, da Silva WR. Ocorrência e coleta de colônias e de enxames de abelhas africanizadas na zona urbana de Maringá, Estado do Paraná, Brasil. Acta Sci. (2006) 28:353–9. doi: 10.4025/actascianimsci.v28i3.53
22. Schmidt JO. Clinical consequences of toxic envenomations by Hymenoptera. Toxicon. (2018) 150:96–104. doi: 10.1016/j.toxicon.2018.05.013
23. Grunwald T, Bockisch B, Spillner E, Ring J, Bredehorst R, Ollert M. Molecular cloning and expression in insect cells of honeybee venom allergen acid phosphatase (Api m 3). J Allergy Clin Immunol. (2006) 117:848–54. doi: 10.1016/j.jaci.2005.12.1331
24. Pereira AFM, Cavalcante JS, Angstmam DG, Almeida C, Soares GS, Pucca MB, et al. Unveiling the pain relief potential: harnessing analgesic peptides from animal venoms. Pharmaceutics. (2023) 15:2766. doi: 10.3390/pharmaceutics15122766
25. El Mehdi I, Falcão SI, Boujraf S, Mustapha H, Campos MG, Vilas-Boas M. Analytical methods for honeybee venom characterization. J Adv Pharm Technol Res. (2022) 13:154–60. doi: 10.4103/japtr.japtr_166_21
26. Fitzgerald KT, Flood AA. Hymenoptera stings. Clin Tech Small Anim Pract. (2006) 21:194–204. doi: 10.1053/j.ctsap.2006.10.002
27. Elena Ionete R, Romina Dinca O, Tamaian R, Irina Geana E. Exploring apis mellifera venom compounds using highly efficient methods. Smart Energy and Sustainable Environment (2013) 16:97–108.
28. Schumacher M, Tveten M, Egen N. Rate and quantity of delivery of venom from honeybee stings. J Allergy Clin Immunol. (1994) 93:831–5. doi: 10.1016/0091-6749(94)90373-5
29. de Toledo LFM, Moore DCBC, Caixeta DMDL, Salú MDS, Farias CVB, Azevedo ZMA. Multiple bee stings, multiple organs involved: a case report. Rev Soc Bras Med Trop. (2018) 51:560–2. doi: 10.1590/0037-8682-0341-2017
30. Ediger D, Terzioglu K, Ozturk RT. Venom allergy, risk factors for systemic reactions and the knowledge levels among Turkish beekeepers. Asia Pac Allergy. (2018) 8:e15. doi: 10.5415/apallergy.2018.8.e15
31. Chen J, Guan S-M, Sun W, Fu H. Melittin, the major pain-producing substance of bee venom. Neurosci Bull. (2016) 32:265–72. doi: 10.1007/s12264-016-0024-y
32. Koyama N, Hirata K, Hori K, Dan K, Yokota T. Computer-assisted infrared thermographic study of axon reflex induced by intradermal melittin. Pain. (2000) 84:133–9. doi: 10.1016/S0304-3959(99)00192-X
33. Koyama N, Hirata K, Hori K, Dan K, Yokota T. Biphasic vasomotor reflex responses of the hand skin following intradermal injection of melittin into the forearm skin. Eur J Pain. (2002) 6:447–53. doi: 10.1016/S1090-3801(02)00029-0
34. Sumikura H, Andersen OK, Drewes AM, Arendt-Nielsen L. A comparison of hyperalgesia and neurogenic inflammation induced by melittin and capsaicin in humans. Neurosci Lett. (2003) 337:147–50. doi: 10.1016/S0304-3940(02)01325-3
35. Sumikura H, Andersen OK, Drewes AM, Arendt-Nielsen L. Secondary heat hyperalgesia induced by melittin in humans. Eur J Pain. (2006) 10:121–1. doi: 10.1016/j.ejpain.2005.01.013
36. Lariviere WR, Melzack R. The bee venom test: a new tonic-pain test. Pain. (1996) 66:271–7. doi: 10.1016/0304-3959(96)03075-8
37. Chen J, Luo C, Li H-L, Chen H-S. Primary hyperalgesia to mechanical and heat stimuli following subcutaneous bee venom injection into the plantar surface of hindpaw in the conscious rat: a comparative study with the formalin test. Pain. (1999) 83:67–76. doi: 10.1016/S0304-3959(99)00075-5
38. Chen H-S, Chen J. Secondary heat, but not mechanical, hyperalgesia induced by subcutaneous injection of bee venom in the conscious rat: effect of systemic MK-801, a non-competitive NMDA receptor antagonist. Eur J Pain. (2000) 4:389–401. doi: 10.1053/eujp.2000.0197
39. Chen J, Chen H-S. Pivotal role of capsaicin-sensitive primary afferents in development of both heat and mechanical hyperalgesia induced by intraplantar bee venom injection. Pain. (2001) 91:367–76. doi: 10.1016/S0304-3959(00)00458-9
40. Chen Y-N, Li K-C, Li Z, Shang G-W, Liu DN, Lu ZM, et al. Effects of bee venom peptidergic components on rat pain-related behaviors and inflammation. Neuroscience. (2006) 138:631–40. doi: 10.1016/j.neuroscience.2005.11.022
41. Tsagareli MG, Nozadze I, Tsiklauri N, Carstens MI, Gurtskaia G, Carstens E. Thermal hyperalgesia and mechanical allodynia elicited by histamine and non-histaminergic itch mediators: respective involvement of TRPV1 and TRPA1. Neuroscience. (2020) 449:35–45. doi: 10.1016/j.neuroscience.2020.09.048
42. Cavalcante J dos S, Nogueira Júnior FA, Bezerra Jorge RJ, Almeida C. Pain modulated by Bothrops snake venoms: Mechanisms of nociceptive signaling and therapeutic perspectives. Toxicon. (2021) 201:105–14. doi: 10.1016/j.toxicon.2021.08.016
43. Oetjen LK, Mack MR, Feng J, Whelan TM, Niu H, Guo CJ, et al. Sensory neurons co-opt classical immune signaling pathways to mediate chronic itch. Cell. (2017) 171:217–228.e13. doi: 10.1016/j.cell.2017.08.006
44. Cevikbas F, Lerner EA. Physiology and pathophysiology of itch. Physiol Rev. (2020) 100:945–82. doi: 10.1152/physrev.00017.2019
45. Yu Y-Q, Zhao Z-Y, Chen X-F, Xie F, Yang Y, Chen J. Activation of tetrodotoxin-resistant sodium channel naV1.9 in rat primary sensory neurons contributes to melittin-induced pain behavior. Neuromolecular Med. (2013) 15:209–17. doi: 10.1007/s12017-012-8211-0
46. Kaplan AP. Kinins, airway obstruction, and anaphylaxis. Anaphylaxis (2010) 95:67–84. doi: 10.1159/000315938
47. Silva A, Amrani Y, Trifilieff A, Landry Y. Involvement of B2 receptors in the bradykinin-induced relaxation of Guinea-pig isolated trachea. Br J Pharmacol. (1995) 114:103–8. doi: 10.1111/j.1476-5381.1995.tb14912.x
48. Joseph K, Kaplan AP. Formation of bradykinin: A major contributor to the innate inflammatory response. Advances in Immunology (2005) 86:159–208. doi: 10.1016/S0065-2776(04)86005-X
49. Schmaier AH. Assembly, activation, and physiologic influence of the plasma kallikrein/kinin system. Int Immunopharmacol. (2008) 8:161–5. doi: 10.1016/j.intimp.2007.08.022
50. Murphey LJ, Malave HA, Petro J, Biaggioni I, Byrne DW, Vaughan DE, et al. Bradykinin and its metabolite bradykinin 1-5 inhibit thrombin-induced platelet aggregation in humans. J Pharmacol Exp Ther. (2006) 318:1287–92. doi: 10.1124/jpet.106.104026
51. Cleary DB, Ehringer WD, Maurer MC. Establishing the inhibitory effects of bradykinin on thrombin. Arch Biochem Biophys. (2003) 410:96–106. doi: 10.1016/S0003-9861(02)00677-X
52. Landmesser U, Drexler H. Effect of angiotensin II type 1 receptor antagonism on endothelial function: role of bradykinin and nitric oxide. J Hypertens. (2006) 24:S39–43. doi: 10.1097/01.hjh.0000220405.38622.23
53. Gryglewski RJ, Uracz W, Chłopicki S, Marcinkiewicz E. Bradykinin as A major endogenous regulator of endothelial function. Pediatr Pathol Mol Med. (2002) 21:279–90. doi: 10.1080/02770930290056514
54. Gryglewski RJ, Święs J, Uracz W, Chłopicki S, Marcinkiewicz E. Mechanisms of angiotensin-converting enzyme inhibitor induced thrombolysis in Wistar rats. Thromb Res. (2003) 110:323–9. doi: 10.1016/j.thromres.2003.08.005
55. Pretorius M, Rosenbaum D, Vaughan DE, Brown NJ. Angiotensin-converting enzyme inhibition increases human vascular tissue-type plasminogen activator release through endogenous bradykinin. Circulation. (2003) 107:579–85. doi: 10.1161/01.CIR.0000046268.59922.A4
56. Felmeden DC, Lip GY. The renin-angiotensin-aldosterone system and fibrinolysis. J Renin-Angiotensin-Aldosterone System. (2000) 1:240–4. doi: 10.3317/jraas.2000.036
57. Prado M, Solano-Trejos G, Lomonte B. Acute physiopathological effects of honeybee (Apis mellifera) envenoming by subcutaneous route in a mouse model. Toxicon. (2010) 56:1007–17. doi: 10.1016/j.toxicon.2010.07.005
58. Sciani JM, Marques-Porto R, Lourenço A, Orsi R de O, Junior RSF, Barraviera B, et al. Identification of a novel melittin isoform from Africanized Apis mellifera venom. Peptides (NY). (2010) 31:1473–9. doi: 10.1016/j.peptides.2010.05.001
59. Vogt W, Patzer P, Lege L, Oldigs H-D, Wille G. Synergism between phospholipase A and various peptides and SH-reagents in causing haemolysis. Naunyn-Schmiedebergs Archiv fur Pharmakologie. (1970) 265:442–54. doi: 10.1007/BF00997079
60. Habermann E. Bee and wasp venoms. Sci (1979). (1972) 177:314–22. doi: 10.1126/science.177.4046.314
61. Okamoto T, Isoda H, Kubota N, Takahata K, Takahashi T, Kishi T, et al. Melittin cardiotoxicity in cultured mouse cardiac myocytes and its correlation with calcium overload. Toxicol Appl Pharmacol. (1995) 133:150–63. doi: 10.1006/taap.1995.1136
62. Ferreira DB, Costa RS, Oliveira JSM, Muccillo G. Cardiac noradrenaline in experimental rat envenomation with africanized bee venom. Exp Toxicologic Pathol. (1994) 45:507–11. doi: 10.1016/S0940-2993(11)80516-6
63. Ferreira DB, Costa RS, De Oliveira JAM, Muccillo G. An infarct-like myocardial lesion experimentally induced in wistar rats with Africanized bee venom. J Pathol. (1995) 177:95–102. doi: 10.1002/path.1711770114
64. Florea A, Crăciun C. Bee (Apis mellifera) Venom Produced Toxic Effects of Higher Amplitude in Rat Thoracic Aorta than in Skeletal Muscle—An Ultrastructural Study. Microscopy Microanalysis. (2012) 18:304–16. doi: 10.1017/S1431927611012876
65. Černe K, Kristan KČ, Budihna MV, Stanovnik L. Mechanisms of changes in coronary arterial tone induced by bee venom toxins. Toxicon. (2010) 56:305–12. doi: 10.1016/j.toxicon.2010.03.010
66. Senthilkumaran S, David SS, Menezes RG, Thirumalaikolundusubramanian P. Acute myocardial infarction triggered by bee sting: An alternative view. Emergency Med Australasia. (2013) 25:615–5. doi: 10.1111/1742-6723.12140
67. Azevedo-Marques MM, Ferreira DB, Costa RS. Rhabdomyonecrosis experimentally induced in Wistar rats by Africanized bee venom. Toxicon. (1992) 30:344–8. doi: 10.1016/0041-0101(92)90875-6
68. Fletcher JE, Tripolitis L, Beech J. Bee venom melittin is a potent toxin for reducing the threshold for calcium-induced calcium release in human and equine skeletal muscle. Life Sci. (1992) 51:1731–8. doi: 10.1016/0024-3205(92)90302-6
69. Ownby CL, Powell JR, Jiang M, Fletcher JE. Melittin and phospholipase A2 from bee (Apis mellifera) venom cause necrosis of murine skeletal muscle in vivo. Toxicon. (1997) 35:67–80. doi: 10.1016/S0041-0101(96)00078-5
70. de Graaf DC, Brunain M, Scharlaken B, Peiren N, Devreese B, Ebo DG, et al. Two novel proteins expressed by the venom glands of Apis mellifera and Nasonia vitripennis share an ancient C1q-like domain. Insect Mol Biol. (2010) 19:1–10. doi: 10.1111/j.1365-2583.2009.00913.x
71. Schartz ND, Tenner AJ. The good, the bad, and the opportunities of the complement system in neurodegenerative disease. J Neuroinflamm. (2020) 17:354. doi: 10.1186/s12974-020-02024-8
72. Efetov KA, Shiriaev NV. Intensification of the interaction of IgG with C1q in the presence of melittin as a possible reason for anaphylaxis under the effect of bee venom. Ukr Biokhim Zh. (1999) 73:49–54.
73. Törő K, Borka K, Kardos M, Kristóf I, Sótonyi P. Expression and function of C5a receptor in a fatal anaphylaxis after honey bee sting*. J Forensic Sci. (2011) 56:526–8. doi: 10.1111/j.1556-4029.2010.01681.x
74. Palm NW, Medzhitov R. Role of the inflammasome in defense against venoms. Proc Natl Acad Sci. (2013) 110:1809–14. doi: 10.1073/pnas.1221476110
75. Nielsen OH, Bouchelouche PN, Berild D. Arachidonic acid and calcium metabolism in rnelittin stimulated neutrophils. Mediators Inflammation. (1992) 1:313–7. doi: 10.1155/S0962935192000462
76. França FO, Benvenuti LA, Fan HW, Dos Santos DR, Hain SH, Picchi-Martins FR, et al. Severe and fatal mass attacks by “killer” bees (Africanized honey bees–Apis mellifera scutellata) in Brazil: clinicopathological studies with measurement of serum venom concentrations. Q J Med. (1994) 87:269–282.
77. Darwish DA, Masoud HMM, Abdel-Monsef MM, Helmy MS, Zidan HA, Ibrahim MA. Phospholipase A2 enzyme from the venom of Egyptian honey bee Apis mellifera lamarckii with anti-platelet aggregation and anti-coagulation activities. J Genet Eng Biotechnol. (2021) 19:10. doi: 10.1186/s43141-020-00112-z
78. Lee K-S, Kim B-Y, Park M-J, Deng Y, Kim J-M, Kim Y-H, et al. Bee venom induces acute inflammation through a H2O2-mediated system that utilizes superoxide dismutase. Toxins (Basel). (2022) 14:558. doi: 10.3390/toxins14080558
79. Guzel M, Akar H, Erenler AK, Baydin A, Kayabas A. Acute ischemic stroke and severe multiorgan dysfunction due to multiple bee stings. Turk J Emerg Med. (2016) 16:126–8. doi: 10.1016/j.tjem.2015.02.002
80. Cavalcante JS, de Almeida DEG, Santos-Filho NA, Sartim MA, de Almeida Baldo A, Brasileiro L, et al. Crosstalk of inflammation and coagulation in bothrops snakebite envenoming: endogenous signaling pathways and pathophysiology. Int J Mol Sci. (2023) 24:11508. doi: 10.3390/ijms241411508
81. Popescu NI, Lupu C, Lupu F. Disseminated intravascular coagulation and its immune mechanisms. Blood. (2022) 139:1973–86. doi: 10.1182/blood.2020007208
82. Zolfagharian H, Mohajeri M, Babaie M. Honey bee venom (Apis mellifera) contains anticoagulation factors and increases the blood-clotting time. J Pharmacopuncture. (2015) 18:7–11. doi: 10.3831/KPI.2015.18.031
83. de Roodt AR, Lanari LC, Lago NR, Bustillo S, Litwin S, Morón-Goñi F, et al. Toxicological study of bee venom (Apis mellifera mellifera) from different regions of the province of Buenos Aires, Argentina. Toxicon. (2020) 188:27–38. doi: 10.1016/j.toxicon.2020.09.014
84. Elieh Ali Komi D, Shafaghat F, Zwiener RD. Immunology of bee venom. Clin Rev Allergy Immunol. (2018) 54:386–96. doi: 10.1007/s12016-017-8597-4
85. Cavalcante JS, Brito IM da C, De Oliveira LA, De Barros LC, Almeida C, Rossini BC, et al. Experimental Bothrops atrox Envenomation: Blood Plasma Proteome Effects after Local Tissue Damage and Perspectives on Thromboinflammation. Toxins (Basel). (2022) 14:613. doi: 10.3390/toxins14090613
86. Cavalcante JS, Borges da Silva WRG, de Oliveira LA, Brito IMC, Muller KS, J. Vidal IS, et al. Blood plasma proteome alteration after local tissue damage induced by Bothrops erythromelas snake venom in mice. J Proteomics. (2022) 269:104742. doi: 10.1016/j.jprot.2022.104742
87. Cavalcante J dos S, de Almeida CAS, Clasen MA, da Silva EL, de Barros LC, Marinho AD, et al. A fingerprint of plasma proteome alteration after local tissue damage induced by Bothrops leucurus snake venom in mice. J Proteomics. (2022) 253:104464. doi: 10.1016/j.jprot.2021.104464
88. Jiang X, Stockwell BR, Conrad M. Ferroptosis: mechanisms, biology and role in disease. Nat Rev Mol Cell Biol. (2021) 22:266–82. doi: 10.1038/s41580-020-00324-8
89. Tang D, Chen X, Kang R, Kroemer G. Ferroptosis: molecular mechanisms and health implications. Cell Res. (2021) 31:107–25. doi: 10.1038/s41422-020-00441-1
90. Conde de la Rosa L, Goicoechea L, Torres S, Garcia-Ruiz C, Fernandez-Checa JC. Role of oxidative stress in liver disorders. Livers. (2022) 2:283–314. doi: 10.3390/livers2040023
91. Witharana RA, Dissanayake A, Karunaratne I, Wijesinghe S. A rare case of micro-angiopathic hemolytic anemia due to envenoming by giant asian honey bee (Apis dorsata). Wilderness Environ Med. (2021) 32:340–3. doi: 10.1016/j.wem.2021.01.008
92. Navaradnam P, Suganthan N, Kumanan T, Sujanitha V, Mayorathan U. Kounis syndrome and multiorgan failure following multiple wasp stings. Cureus. (2021) 13. doi: 10.7759/cureus.14606
93. Senturk A, Dalkiran B, Acikgoz B, Aksu I, Acikgoz O, Kiray M. The effects of bee venom on liver and skeletal muscle in exhaustive swimming rats. Biol Futur. (2022) 73:237–44. doi: 10.1007/s42977-022-00115-6
94. Cichoż-Lach H. Oxidative stress as a crucial factor in liver diseases. World J Gastroenterol. (2014) 20:8082. doi: 10.3748/wjg.v20.i25.8082
95. Ravindran M, Khan MA, Palaniyar N. Neutrophil extracellular trap formation: physiology, pathology, and pharmacology. Biomolecules. (2019) 9:365. doi: 10.3390/biom9080365
96. de Bont CM, Boelens WC, Pruijn GJM. NETosis, complement, and coagulation: a triangular relationship. Cell Mol Immunol. (2019) 16:19–27. doi: 10.1038/s41423-018-0024-0
97. Vorobjeva NV, Chernyak BV. NETosis: molecular mechanisms, role in physiology and pathology. Biochem (Moscow). (2020) 85:1178–90. doi: 10.1134/S0006297920100065
98. Katkar GD, Sundaram MS, NaveenKumar SK, Swethakumar B, Sharma RD, Paul M, et al. NETosis and lack of DNase activity are key factors in Echis carinatus venom-induced tissue destruction. Nat Commun. (2016) 7:11361. doi: 10.1038/ncomms11361
99. Han HJ, Park SH, Lee JH, Yoon BC, Park KM, Mar WC, et al. Involvement of oxidative stress in bee venom-induced inhibition of na+/glucose cotransporter in renal proximal tubule cells. Clin Exp Pharmacol Physiol. (2002) 29:564–8. doi: 10.1046/j.1440-1681.2002.03685.x
100. dos Reis MA, Costa RS, Coimbra TM, Dantas M, Gomes UA. Renal changes induced by envenomation with africanized bee venom in female wistar rats. Kidney Blood Press Res. (1997) 20:271–7. doi: 10.1159/000174157
101. dos Reis MA, Costa RS, Coimbra TM, Teixeira VPA. Acute renal failure in experimental envenomation with africanized bee venom. Ren Fail. (1998) 20:39–51. doi: 10.3109/08860229809045088
102. Gyurászová M, Gurecká R, Bábíčková J, Tóthová Ľ. Oxidative stress in the pathophysiology of kidney disease: implications for noninvasive monitoring and identification of biomarkers. Oxid Med Cell Longev. (2020) 2020:1–11. doi: 10.1155/2020/5478708
103. De Matos IM, Rocha OA, Leite R, Freire-Maia L. Lung oedema induced by Tityus serrulatus scorpion venom in the rat. Comp Biochem Physiol C Pharmacol Toxicol Endocrinol. (1997) 118:143–8. doi: 10.1016/S0742-8413(97)00086-8
104. Freire-Maia L, Almeida HO, Cunha-Melo JR, Azevedo AD, Barroso J. Mechanism of the pulmonary edema induced by intravenous injection of scorpion toxin in the rat. Agents Actions. (1978) 8:113–8. doi: 10.1007/BF01972412
105. Freire-Maia L, de Matos IM. Heparin or a PAF antagonist (BN-52021) prevents the acute pulmonary edema induced by Tityus serrulatus scorpion venom in the rat. Toxicon. (1993) 31:1207–10. doi: 10.1016/0041-0101(93)90137-8
106. Coelho FM, Pessini AC, Coelho AM, Pinho VS, Souza DG, Arantes EC, et al. Platelet activating factor receptors drive CXC chemokine production, neutrophil influx and edema formation in the lungs of mice injected with Tityus serrulatus venom. Toxicon. (2007) 50:420–7. doi: 10.1016/j.toxicon.2007.04.009
107. Sheshala K, Misra KC, Hemanth C, Appasani S. Bee sting to boerhaave's syndrome. Indian J Crit Care Med. (2021) 25:346–8. doi: 10.5005/jp-journals-10071-23770
108. Pirasath S, Senthan V, Seneviratne MH. Kounis syndrome: Acute myocardial infarction following multiple bee stings. SAGE Open Med Case Rep. (2021) 9:2050313X2199920. doi: 10.1177/2050313X21999206
109. Mehdi Z, Gupta M, Garg A, Kaur N. Beyond anaphylaxis : acute myocardial infarction with is- chemic stroke after bee sting a case report. Iranian Journal of Emergency Medicine (2023) 10:3–6. doi: 10.22037/ijem.v10i1.40469
110. Bindu C, Manuprakash S, Srinivasa B. Acute myocardial injury in multiple bee stings - Case report. Int J Med Sci Public Health. (2013) 2:1107. doi: 10.5455/ijmsph.2013.200620132
111. Gopinath B, Kumar G, Nayaka R, Ekka M. Kounis syndrome and atrial fibrillation after bee sting: A case report. J Family Med Prim Care. (2022) 11:7460. doi: 10.4103/jfmpc.jfmpc_901_22
112. França FOS, Benvenuti LA, Fan HW. Severe and fatal mass attacks by ‘killer’ bees (Africanized honey bees—Apis mellifera scutellata) in Brazil: clinicopathological studies with measurement of serum venom concentrations. QJM: Int J Med. (1994) 87:269–282. doi: 10.1093/oxfordjournals.qjmed.a068927
113. Mellyana O, Adelina D, Kusuma J. Acute kidney injury due to multiple bee stings in a 3 years old girl. Med Hospitalia : J Clin Med. (2019) 6:64–70. doi: 10.36408/mhjcm.v6i1.382
114. Tsuruta K, Yokoi K, Yoshioka G, Chen W, Jojima K, Hongo H, et al. Different types of Kounis syndrome caused by different episodes of bee sting anaphylaxis: Misfortunes never come singly. J Cardiol cases. (2022) 26:81–4. doi: 10.1016/j.jccase.2022.03.002
115. E Khoda MM, Islam RN, Rahim MA, Mansur MA. Wcn23-0329 kounis syndrome with fatal outcome: A case report. Kidney Int Rep. (2023) 8:S5. doi: 10.1016/j.ekir.2023.02.011
116. Aytekin G, Çölkesen F, Yıldız E, Arslan Ş, Oltulu P. Kounis syndrome in a patient with secondary mast cell activation syndrome after a bee sting. Ann Med Res. (2020) 1. doi: 10.5455/annalsmedres.2019.10.671
117. Kamalesh TN, Nishanth S, Akanksh MD, Naveen K. Kounis syndrome: is it a heralder or just a mimicker of acute coronary syndrome? J OF Clin AND Diagn Res. (2023) 17. doi: 10.7860/JCDR/2023/60999.18040
118. Thwe EE, Sudnik P, Dobrovolschi C, Krishnamurthy M. Kounis syndrome: an allergic acute coronary syndrome due to a bee sting. Cureus. (2022) 14. doi: 10.7759/cureus.26395
119. Katsanou K, Tsiafoutis I, Kounis NG. Timeo apis mellifera and dona ferens: bee sting-induced Kounis syndrome. Clin Chem Lab Med (CCLM). (2018) 56:e197–200. doi: 10.1515/cclm-2018-0002
120. Acehan S, Satar S, Gulen M, Yucel C, Segmen MS. Angina and arrhythmia symptoms following multiple bee stings: kounis syndrome. Wilderness Environ Med. (2022) 33:417–21. doi: 10.1016/j.wem.2022.06.003
121. du Toit-Prinsloo L, Morris NK, Meyer P, Saayman G. Deaths from bee stings: a report of three cases from Pretoria, South Africa. Forensic Sci Med Pathol. (2016) 12:81–5. doi: 10.1007/s12024-015-9737-x
122. Le H-Y, Tien ND, Son PN, Viet Hoa LT, Phuong LL, Hai PD. Extracorporeal membrane oxygenation support in refractory anaphylactic shock after bee stings: A case report. Perfusion. (2023) 38:1308–10. doi: 10.1177/02676591221103540
123. Chaudry A. Mobitz type-2 heart block after a bee-sting. Cureus. (2020) 12. doi: 10.7759/cureus.11856
124. Su Z, Hu Z, Wang L, Wang Y, Fang X, Ye P. Visual loss caused by central retinal artery occlusion after bee sting: A case report. Front Med (Lausanne). (2021) 8:707978. doi: 10.3389/fmed.2021.707978
125. de Azevedo RV, de Paiva RB, Ades F, David CM. Síndrome de envenenamento por 2000 picadas de abelhas africanizadas. Relato caso. Rev Bras Ter Intensiva. (2006) 18:99–103. doi: 10.1590/S0103-507X2006000100016
126. Dural İE. Kounis Syndrome with Pericarditis Epistenocardica : a case report. (2022), 1–12. doi: 10.21203/rs.3.rs-1342895/v1
127. Seecheran RV, Ramdin R, Singh S, Seecheran V, Persad S, Peram L, et al. Africanized honey bee sting-induced stress-related cardiomyopathy: A bee or octopus trap. Cureus. (2021) 13. doi: 10.7759/cureus.16681
128. Önder Ö, Aktan AH. Encephalitis and toxic hepatitis caused by bee sting: an unusual case report. Turkish J Intensive Care. (2021) 19:196–9. doi: 10.4274/tybd.galenos.2021.86548
129. Ratnayake GM, Weerathunga PN, Dilrukshi MSA, Amara Witharana EWR, Jayasinghe S. Giant honey bee (Apis dorsata) sting and acute limb ischemia: a case report and review of the literature. BMC Res Notes. (2018) 11:327. doi: 10.1186/s13104-018-3422-6
130. Odinaka K, Achigbu K, Ike I, Iregbu F. Bee sting envenomation resulting in gross haematuria in an eight-year-old Nigerian male with sickle cell anaemia: A case report. Nigerian Med J. (2015) 56:69. doi: 10.4103/0300-1652.149175
131. Akgul MH, Bagırov E. Brachial artery thrombosis following bee sting, case report. Int J Surg Case Rep. (2021) 78:184–6. doi: 10.1016/j.ijscr.2020.11.156
132. Cil M, Leblebisatan G, Leblebisatan S, Barutcu A, Cil MK, Kilinc Y. Deep vein thrombosis after a wild bee sting. J Pediatr Hematol Oncol. (2022) 44:e241–2. doi: 10.1097/MPH.0000000000002072
133. Farhat E, Tegg E, Mohammed S, Grzechnik E, Favaloro EJ. Not as sweet as honey: A rare case of an apparent factor V “inhibitor” in association with bee sting anaphylaxis. Am J Hematol. (2018) 93:965–70. doi: 10.1002/ajh.25121
134. Akyıldız B, Özsoylu S, Öztürk MA, İnci A, Düzlü Ö, Yıldırım A. A fatal case caused by massive honey bee stings. Turkish J Pediatr. (2016) 57:611–4.
135. Rauf A, Vijayan A, Hashitha V, John S, Peringat J. Rhabdomyolysis and acute kidney injury following multiple bee stings in a child: A case report. J Pediatr Crit Care. (2021) 8:252. doi: 10.4103/jpcc.jpcc_38_21
136. Babikir H, Ibrahim N, Altayeb Z, Ahmed A. A rare acute haemorrhagic stroke and severe multiorgan dysfunction following massive honeybee stings. Sudan J Paediatr. (2021) 121:209–14. doi: 10.24911/SJP.106-1598962628
137. Khalighi Z, Azami G, Shafiei E, Sahebi A, Mozafari A. Thrombotic thrombocytopenic purpura following honeybee envenomation: A case report. Int J Med Toxicol Forensic Med. (2020) 10:28794–28794. doi: 10.32598/ijmtfm.v10i2.28794
138. Mukesh R, Rekha JS, Shaha KK, Mylapalli J. Adrenal hemorrhage and rhabdomyolysis following mass bee sting envenomation: An autopsy based case report. J Indian Soc Toxicol. (2020) 16:47–50. doi: 10.31736/2020v16i2/47-50
139. Riches KJ, Gillis D, James RA. An autopsy approach to bee sting-related deaths. Pathology. (2002) 34:257–62. doi: 10.1080/00313020220131327
140. Pujari S, Ranjan R, Verghese S, Manayath G, Narendran V. Case report of central retinal artery occlusion following bee sting injury: A possible link? Oman J Ophthalmol. (2021) 14:112. doi: 10.4103/ojo.ojo_27_21
141. Mondello C, Baldino G, Cianci V, Forzese E, Asmundo A, Ieni A, et al. Postmortem biochemistry and immunohistochemistry in anaphylactic death due to hymenoptera sting: A forensic case report. Int J Environ Res Public Health. (2023) 20:5640. doi: 10.3390/ijerph20095640
142. Bui PV, Haas NL, Herrman NWC, Macias M, Hoch V, Schaeffer W, et al. Epinephrine administered for anaphylaxis unmasking a type 1 brugada pattern on electrocardiogram. J Emerg Med. (2019) 56:444–7. doi: 10.1016/j.jemermed.2018.12.045
143. Fakhar M, Zakariaei Z, Sharifpour A, Soleymani M, Zakariaei A. Fatal outcome following multiple bee stings: A rare case. Clin Case Rep. (2022) 10:e05303. doi: 10.1002/ccr3.5303
144. Contreras Zuniga E, Zuluaga SX, Casas Quiroga IC. Envenenamiento por múltiples picaduras de abejas y choque anafiláctico secundario: descripción de un caso clínico y revisión de la literatura. Acta toxicológica Argent. (2008) 16:27–32.
145. Izaguirre González AI, Martínez-Zepeda ER, Rivas-Godoy AF, Sánchez-Sierra LE, Díaz-Robbio I, Izaguirre González AI, et al. Falla multiorgánica secundaria a múltiples picaduras de abeja (Apis mellifera). Rev la Facultad Medicina (México). (2018) 61:31–7.
146. Lubis M, Puspitasari R, Saragih RA. Multiple bee stings induced multiorgan dysfunction in a 4 year old female. Pediatr Oncall. (2019) 16:19–20. doi: 10.7199/ped.oncall.2019.30
147. Sunny JM, Abrencillo R. Massive bee envenomation treated by therapeutic plasma exchange. J Clin Apher. (2021) 36:654–7. doi: 10.1002/jca.21898
148. Jain J, Banait S, Srivastava A, Lodhe R. Stroke intracerebral multiple infarcts: Rare neurological presentation of honey bee bite. Ann Indian Acad Neurol. (2012) 15:163. doi: 10.4103/0972-2327.95008
149. Gupta A. Acute fatal stroke associated with honeybee sting. J Neurol Neuromedicine. (2019) 5:18–21. doi: 10.29245/2572.942X/2019/1.1262
150. Betten DP, Richardson WH, Tong TC, Clark RF. Massive honey bee envenomation-induced rhabdomyolysis in an adolescent. Pediatrics. (2006) 117:231–5. doi: 10.1542/peds.2005-1075
151. Hiran S, Pande TK, Pani S, Gupta R, Vishwanathan KA. Rhabdomyolysis due to multiple honey bee stings. Postgrad Med J. (1994) 70:937–7. doi: 10.1136/pgmj.70.830.937
152. Seelarathna RMM, Navakumaran M, Kumanan T. Bee stings an unusual cause of severe rhabdomyolysis: A case report and literature review. Jaffna Med J. (2020) 32:42–3. doi: 10.4038/jmj.v32i2.107
153. Constantino K, Pawlukiewicz AJ, Spear L. A case report on rhabdomyolysis after multiple bee stings. Cureus. (2020) 12. doi: 10.7759/cureus.9501
154. Geoffroy S, Fremery A, Lambert Y, Marty C, Elenga N. Case report: acute kidney failure due to massive envenomation of a two-year-old child caused by killer bee stings. Am J Trop Med Hyg. (2021) 105:222–4. doi: 10.4269/ajtmh.20-1276
155. Sathi S, Garg AK, Chand J, Singh MK, Saini VS, Trivedi A. Severe acute kidney injury after multiple honey bee stings. Int J Nephrol Kidney Fail. (2020) 6. doi: 10.16966/2380-5498.195
156. Mahale R, Mehta A, Shankar A, Buddaraju K, John A, Javali M, et al. Isolated posterior circulation stroke following honey-bee sting. Neurol India. (2016) 64:116. doi: 10.4103/0028-3886.178053
157. Saini AG, Sankhyan N, Suthar R, Singhi P. Acute axonal polyneuropathy following honey-bee sting. J Child Neurol. (2014) 29:674–6. doi: 10.1177/0883073813517262
158. Poddar K, Poddar S, Singh A. Acute polyradiculoneuropathy following honey bee sting. Ann Indian Acad Neurol. (2012) 15:137. doi: 10.4103/0972-2327.95000
159. Walter O, Macrine O, George Michael OO. Case Report: An unusual case of bee envenomation presenting with acute kidney injury, cavernous venous thrombosis and multiple episodes of convulsions. Global J Med Clin Case Rep. (2020) 7:103–6. doi: 10.17352/2455-5282.000109
160. Zuluaga C, Lara J, Berrouet MC. Síndrome de Guillain-Barré asociado a accidente apídico : reporte de caso. Medicina Upb. (2021) 40:77–81. doi: 10.18566/medupb.v40n1.a11
161. Ramlackhansingh AF, Seecheran N. Africanised honey bee sting-induced ischaemic stroke. BMJ Case Rep. (2020) 13:e234877. doi: 10.1136/bcr-2020-234877
162. Varuni K, Sivansuthan S, Joseph Piratheepan G, Gajanthan R. A case report on unusual cause of young ischemic Cerebrovascular Accident: a rare complication of honey bee stings. Jaffna Med J. (2018) 30:32–4. doi: 10.4038/jmj.v30i2.25
163. Kabra R, Andhale A, Acharya S, Kumar S, Sawant R. Acute ischemic stroke post honeybee sting: A rare case report. Cureus. (2022) 14. doi: 10.7759/cureus.31851
164. Abhishek BR, Velayutham SS, Jeyaraj KM, Sowmini PR, Kumar MS, Sarvanan SV, et al. Thrombolysis in ischemic stroke after bee sting: A rare scenario. Ann Indian Acad Neurol. (2021) 24:985. doi: 10.4103/aian.AIAN_770_20
165. Rathnayaka N, Ranathunga AN, Rathnayaka B, Kularatne SAM. Massive cerebral infarct following multiple honeybee stings. Ceylon Med J. (2021) 66:151. doi: 10.4038/cmj.v66i3.9494
166. Sancar E, Ararat E, Avci S. Acute ischemic stroke associated with allergic reaction case report. Ann Med Case Rep. (2020) 2:1015.
167. Ryakitimbo A, Kennedy M, Shao E, Itana ME, Mbwasi R, Kinabo G, et al. Acute kidney injury in a Tanzanian boy following multiple bee stings in resource-limited setting: a case report. Oxf Med Case Rep. (2018) 2018:omy070. doi: 10.1093/omcr/omy070
168. Singer E, Lande L. Critical upper airway edema after a bee sting to the uvula. Wilderness Environ Med. (2022) 33:236–8. doi: 10.1016/j.wem.2022.02.001
169. da Silva GAR, Pires KL, Soares DC de S, Ferreira MR, Ferry FR de A, Motta RN, et al. RRH: envenoming syndrome due to 200 stings from Africanized honeybees. Rev Inst Med Trop Sao Paulo. (2013) 55:61–4. doi: 10.1590/S0036-46652013000100011
170. Mathew A, Chrispal A, David T. Acute myocardial injury and rhabdomyolysis caused by multiple bee stings. J Assoc Physicians India. (2011) 59:518–20.
171. Haniff S, Butler ME, Abou-Jaoude EA, Lenahan ML. An unusual trigger of grover’s disease (GD). Cureus. (2023) 15. doi: 10.7759/cureus.40648
172. Gudiseva H, Uddaraju M, Pradhan S, Das M, Mascarenhas J, Srinivasan M, et al. Ocular manifestations of isolated corneal bee sting injury, management strategies, and clinical outcomes. Indian J Ophthalmol. (2018) 66:262. doi: 10.4103/ijo.IJO_600_17
173. Dau M, Ngu B, Norina T, Jaffar T, Hussein A. Case series of cornea bee sting : different approaches of management. Journal of Biomedical and Clinical Sciences (JBCS) (2019) 4:7–10.
174. Dai XX, Hao JL, Zhou DD, Teng SY, Hui P, Lu CW. A successfully managed case of corneal bee sting: A case report and literature review. Iran Red Crescent Med J. (2019) 21:0–4. doi: 10.5812/ircmj.99335.Case
175. Chilibeck C, Wang N, Murphy C. Making a bee-line for the eye: a limbal sting and retained honey bee. Clin Exp Optom. (2021) 104:538–40. doi: 10.1080/08164622.2021.1878837
176. Duff- Lynes SM, Martin P, Horn EP. Management of bulbar conjunctival injury by honeybee sting: A case report of a retained honeybee stinger. Am J Ophthalmol Case Rep. (2022) 25:101365. doi: 10.1016/j.ajoc.2022.101365
177. Grüb M, Mielke J, Schlote T. Bienenstichverletzung der Hornhaut - eine Kasuistik1. Klin Monbl Augenheilkd. (2001) 218:747–50. doi: 10.1055/s-2001-18669
178. Isawumi MA, Hassan M. Honeybee sting of the sclera: occular features, treatment, outcome and presumed pathogenesis. Pan Afr Med J. (2014) 17. doi: 10.11604/pamj.2014.17.30.3297
179. Bhalerao S, Singh P, Rani P, Rathi V. The sting of a honey bee: An unusual subconjunctival foreign body. Indian J Ophthalmol. (2017) 65:1226. doi: 10.4103/ijo.IJO_533_17
180. Newman AR, Beckman TJ, Meiklejohn BD, Green MD. Migration of retained tarsal bee stinger onto the ocular surface causing superficial keratopathy. Digital J Ophthalmol. (2022) 28:31–3. doi: 10.5693/djo.02.2021.10.001
181. Chen MC, Melnychuk V. Corneal bee sting: improvement in the acute stage in the absence of treatment. Pan Afr Med J. (2020) 37. doi: 10.11604/pamj.2020.37.54.20267
182. Davidorf OA, Ng AE, Davidorf JM. Retained eyelid bee stinger: A case of secondary corneal abrasion. Am J Ophthalmol Case Rep. (2020) 18:100670. doi: 10.1016/j.ajoc.2020.100670
183. Chauhan D. Corneal honey bee sting: endoilluminator-assisted removal of retained stinger. Int Ophthalmol. (2012) 32:285–8. doi: 10.1007/s10792-012-9553-1
184. Dogra M, Narang S, Sood S, Gupta P. Successful management of bee sting induced Aspergillus fumigatus endophthalmitis and scleritis. Indian J Ophthalmol. (2018) 66:461. doi: 10.4103/ijo.IJO_889_17
185. Ahmed M, Lee CS, McMillan B, Jain P, Wiley L, Odom JV, et al. Predicting visual function after an ocular bee sting. Int Ophthalmol. (2019) 39:1621–6. doi: 10.1007/s10792-018-0978-z
186. Tyagi M, Reddy S, Basu S, Pappuru R, Dave V. Endoscopic visualization-assisted corneal bee sting removal. Indian J Ophthalmol. (2021) 69:423. doi: 10.4103/ijo.IJO_1161_20
187. Al Amry M, Al Ghadeer H, Al Gethami AR. Bee sting presumed endophthalmitis: a devastating ocular outcome. Int J Retina Vitreous. (2021) 7:52. doi: 10.1186/s40942-021-00320-w
188. Limaiem R, Chaabouni A, El Maazi A, Mnasri H, Mghaieth F, El Matri L. Lésions oculaires par piqûre d’abeille. À propos d’un cas. J Fr Ophtalmol. (2009) 32:277–9. doi: 10.1016/j.jfo.2009.01.007
189. Rishi E, Rishi P. Intraocular inflammation in a case of bee sting injury. GMS Ophthalmol cases. (2018) 8:Doc02. doi: 10.3205/oc000084
190. del Carmen Zambrano-Infantino R, Piñerúa-Gonsálvez JF, Montaño C, Rodríguez C. Neuritis óptica por picadura de abeja. Investigacion Clinica (Venezuela). (2013) 54:180–5.
191. da Cunha CEX, Ribeiro MVMR, Soares ARG, Castro LR, Jucá JV de O. Uveíte anterior hipertensiva após picada de abelha: um relato de caso. Rev Med (Rio J). (2023) 102. doi: 10.11606/issn.1679-9836.v102i1e-194512
192. Silva GBDJ, Vasconcelos AGJ, Rocha AMT, Vasconcelos VR, Barros JN, Fujishima JS, et al. Acute kidney injury complicating bee stings – a review. Rev Inst Med Trop Sao Paulo. (2017) 59:e25. doi: 10.1590/s1678-9946201759025
193. RodrÍguez-Ruiz C, Puig-Carrión G, Delgado-Nieves A, López-Candales A. Kounis syndrome: A more commonly encountered cause of acute coronary syndrome. Heart Views. (2019) 20:122. doi: 10.4103/HEARTVIEWS.HEARTVIEWS_43_19
194. Ruwanpathirana P, Priyankara D. Clinical manifestations of wasp stings: a case report and a review of literature. Trop Med Health. (2022) 50:82. doi: 10.1186/s41182-022-00475-8
195. Wist S, Clivaz J, Sattelmayer M. Muscle strengthening for hemiparesis after stroke: A meta-analysis. Ann Phys Rehabil Med. (2016) 59:114–24. doi: 10.1016/j.rehab.2016.02.001
196. Pedroso JL, Vale TC, Braga-Neto P, Dutra LA, França MC Jr., Teive HAG, et al. Acute cerebellar ataxia: differential diagnosis and clinical approach. Arq Neuropsiquiatr. (2019) 77:184–93. doi: 10.1590/0004-282x20190020
197. Uzel IF, Arslan M, Demirhan O. Anaphylaxis and diffuse alveolar hemorrhage following bee sting. Respir Case Rep. (2013) 2:10–3. doi: 10.5505/respircase.2013.00710
198. Lara AR, Schwarz MI. Diffuse alveolar hemorrhage. Chest. (2010) 137:1164–71. doi: 10.1378/chest.08-2084
199. Thumtecho S, Suteparuk S, Sitprija V. Pulmonary involvement from animal toxins: the cellular mechanisms. J Venomous Anim Toxins including Trop Dis. (2023) 29:e20230026. doi: 10.1590/1678-9199-jvatitd-2023-0026
200. Cai Z-Y, Xu B-P, Zhang W-H, Peng H-W, Xu Q, Yu H-B, et al. Acute respiratory distress syndrome following multiple wasp stings treated with extracorporeal membrane oxygenation: A case report. World J Clin cases. (2022) 10:11122–7. doi: 10.12998/wjcc.v10.i30.11122
201. Semler-Collery A, Hayek G, Ramadier S, Perone J-M. A case of conjunctival bee sting injury with review of the literature on ocular bee stings. Am J Case Rep. (2019) 20:1284–9. doi: 10.12659/AJCR.917592
202. Çaça I, Ari S, Ülü K, Ayata A. Bee sting of the cornea: A case study and review of the literature. Ann Ophthalmol. (2006) 38:77–80. doi: 10.1385/AO:38:1:77
203. De D, Caso Clínico UN, De R, Contreras Zuniga LLE, Zuluaga SX. Resumen: Envenenamiento por múltiples picaduras de abejas y choque anafiláctico secundario. acta toxicológica argentina (2008) 16:27–32.
205. Greene A, Breisch NL. Avoidance of bee and wasp stings: an entomological perspective. Curr Opin Allergy Clin Immunol. (2005) 5:337–41. doi: 10.1097/01.all.0000173781.58154.53
206. Sicherer SH, Simons FER, Mahr TA, Abramson SL, Dinakar C, Fleisher TA, et al. Epinephrine for first-aid management of anaphylaxis. Pediatrics. (2017) 139. doi: 10.1542/peds.2016-4006
207. Cavalcante J dos S, de Almeida DEG, Moraes MS, Santos SR, Pincinato PM, Riciopo PM, et al. Challenges and opportunities in clinical diagnostic routine of envenomation using blood plasma proteomics. Toxins (Basel). (2023) 15:180. doi: 10.3390/toxins15030180
208. Ferreira Junior RS, Anderlini RP, Pimenta DC, De Oliveira Orsi R, Barraviera B, Sant’Anna OA. New Nanostructured Silica Adjuvant (SBA-15) Employed to Produce Antivenom in Young Sheep Using Crotalus durissus terrificus and Apis mellifera Venoms Detoxified by Cobalt-60. J Toxicol Environ Health A. (2010) 73:926–33. doi: 10.1080/15287391003745069
209. Teixeira-Cruz JM, Monteiro-MaChado M, Cons BL, Martins-Ferreira J, da Cunha LER, Barraviera B, et al. Antagonism of in vitro and in vivo activities of Apis mellifera venom by antiapilic serum. Toxicon. (2016) 117:102–3. doi: 10.1016/j.toxicon.2016.04.003
210. Teixeira-Cruz JM, Strauch MA, Monteiro-MaChado M, Tavares-Henriques MS, de Moraes JA, Ribeiro da Cunha LE, et al. A novel apilic antivenom to treat massive, africanized honeybee attacks: A preclinical study from the lethality to some biochemical and pharmacological activities neutralization. Toxins (Basel). (2021) 13:30. doi: 10.3390/toxins13010030
211. Oliveira Orsi R, Zaluski R, de Barros LC, Barraviera B, Pimenta DC, Ferreira Junior RS. Standardized guidelines for Africanized honeybee venom production needed for development of new apilic antivenom. J Toxicol Environ Health Part B. (2024) 27:73–90. doi: 10.1080/10937404.2023.2300786
Keywords: bee venom, bee sting, clinical envenoming, clinical management, venomous animals, Africanized bee, honeybee
Citation: Cavalcante JS, Riciopo PM, Pereira AFM, Jeronimo BC, Angstmam DG, Pôssas FC, Andrade Filho Ad, Cerni FA, Pucca MB and Ferreira Junior RS (2024) Clinical complications in envenoming by Apis honeybee stings: insights into mechanisms, diagnosis, and pharmacological interventions. Front. Immunol. 15:1437413. doi: 10.3389/fimmu.2024.1437413
Received: 23 May 2024; Accepted: 20 August 2024;
Published: 18 September 2024.
Edited by:
Dale Umetsu, Stanford University, United StatesReviewed by:
Mouzarllem Barros Reis, University of São Paulo, BrazilVanessa de Fátima Borges, Cedars Sinai Medical Center, United States
Copyright © 2024 Cavalcante, Riciopo, Pereira, Jeronimo, Angstmam, Pôssas, Andrade Filho, Cerni, Pucca and Ferreira Junior. This is an open-access article distributed under the terms of the Creative Commons Attribution License (CC BY). The use, distribution or reproduction in other forums is permitted, provided the original author(s) and the copyright owner(s) are credited and that the original publication in this journal is cited, in accordance with accepted academic practice. No use, distribution or reproduction is permitted which does not comply with these terms.
*Correspondence: Rui Seabra Ferreira Junior, cnVpLnNlYWJyYUB1bmVzcC5icg==