- 1General Affairs Office of Shenyang Hongqiao Hospital of Traditional Chinese Medicine, Shenyang, China
- 2Key Laboratory of Cell Biology, Department of Cell Biology, Ministry of Public Health and Key Laboratory of Medical Cell Biology, Ministry of Education, China Medical University, Shenyang, China
The suppressive tumour microenvironment significantly hinders the efficacy of immunotherapy in treating solid tumors. In this context, stromal cells, such as tumour-associated fibroblasts, undergo changes that include an increase in the number and function of immunosuppressive cells. Adenosine, a factor that promotes tumour growth, is produced from ATP breakdown and is markedly elevated in the tumour microenvironment. It acts through specific binding to adenosine receptors, with A2A and A2B adenosine receptor being primary drivers of immunosuppression. This paper presents the roles of various adenosine receptors in different tumour microenvironments. This review focus on the function of adenosine receptors in the stromal cells and non-cellular components of the tumour microenvironment. Additionally, we summarize and discuss recent advances and potential trends in using adenosine receptor antagonists combined with immunotherapy.
1 Introduction
Immunotherapy has become a promising treatment for cancer alongside radiotherapy, achieving significant clinical efficacy. Preclinical studies have demonstrated that immunological approaches, such as immune checkpoint inhibitors (ICIs) and cancer vaccines, can produce effective antitumor responses (1, 2). Additionally, immune checkpoint drugs are currently undergoing clinical trials, yielding encouraging results with reduced adverse reactions. Unfortunately, tumour vaccines are not effective for all tumors, and the complex tumour microenvironment (TME) often renders some solid tumors poorly responsive to ICIs (3). Current research suggests that cytokines secreted by tumour or immune cells within the TME, along with changes in immune cell composition and microenvironmental nutrients, can influence the efficacy of immunotherapy (4, 5). The adenosinergic pathway has long been associated with antitumor immunity (6). Specifically, the use of adenosine receptor antagonists has been demonstrated to enhance the antitumor activity of tumour-infiltration lymphocytes and Natural Killer cells (NK cells) (7, 8). Furthermore, adenosine receptor antagonists can inhibit the immunosuppressive microenvironment, including macrophages infiltration (9). Recent experiments have begun exploring the relationship between adenosine receptors and non-immune components in the TME. Therefore, This artical focuses on summarizing the associations and effects of adenosine receptors with stromal cells and non-cellular components in the TME. A brief overview of recent clinical trials involving adenosine receptor antagonists combined with immunotherapy is also provided. Furthermore, potential combinations to enhance antitumor therapies are considered. This review lays the foundation for studying the relationships and mechanisms between adenosine receptors and TME components, offering insights and strategies for more effective immunotherapy combinations.
2 Distribution and mechanism of adenosine receptors
Adenosine receptors belong to the class A family of retinoid-like G protein-coupled receptors. Four members of the adenosine receptor family have been identified: A1 adenosine receptor (A1AR), A2A adenosine receptor (A2AAR), A2B adenosine receptor (A2BAR), and A3 adenosine receptor (A3AR). These receptors, as the natural endogenous receptors for adenosine are involved in numerous physiological and pathological signaling pathways (10). This section summarize the distribution of adenosine receptors under physiological and pathological conditions based on data from the Human Protein Atlas database and relevant literature.
2.1 Distribution of adenosine receptors under physiological and pathological conditions
Adenosine receptors A2AAR and A2BAR have been approved by the FDA for drug targeting of G protein-coupled receptors. The ADORA2A gene is expressed at the RNA level in various tissues (11, 12), while the A2AAR protein is expressed only in a few tissues, with the highest levels found in the colon, caudate nucleus, and appendix (13, 14). A2AAR protein is also present in the cerebellum, kidney and bone marrow (15). Tissue-specific distribution of A2AAR protein has been observed in endothelial cells of tissues such as the myocardium and kidney. Among immune cells, A2AAR levels are higher in neutrophils and regulatory T cells (Treg cells). Under pathological conditions associated with different tumors, adenosine receptor content varies. In a few cases of ovarian cancer, A2AAR protein expression shows moderate to high positivity in the cytoplasmic and cell membrane. A2AAR protein expression in other cancer tissues is generally negative. The expression of A2BAR protein in various tissues under physiological and tumor conditions has not been fully elucidated. However, tissue-specific distribution has been observed in duct cells, urothelial cells, and keratinocytes related to the urinary system, with high expression levels in immune cells, including bone marrow cells and basophils.
The expression of A1AR protein in various tissues under physiological and tumour conditions is also yet to be fully understood. The ADORA1 gene is highly expressed, mostly in renal carcinoma. Tissue-specific distribution of A1AR protein can be observed in Muller glial cells, oligodendrocyte precursor cells, oligodendrocytes, and excitatory neurons (16–18). The expression level of A1AR protein in immune cells is not high. A3AR is mainly expressed in macrophages in tissues such as the colon, myocardium and lungs. In contrast, A3AR is highly expressed in eosinophils among immune cells (19).
2.2 Mechanisms of adenosine receptors in non-tumour and tumour diseases
This review summarizes the current research status of adenosine receptors and disease, focusing on classical and recent studies. The same adenosine receptor may play different roles in various diseases. A1AR, A2AAR and A2BAR have all been reported to be associated with cardiovascular diseases and diabetic complications, but their roles differ. A1AR is mainly involved in processes such as myocardial hypertrophy, ischemia-reperfusion injury, and various arrhythmias (20, 21). In contrast, A2AAR has been demonstrated to regulate coronary artery dilation (21, 22). Unlike A2AAR, A2BAR is involved in vasoconstrictor effects (21). Furthermore, A1AR is involved in the regulation of gestational hypertension through the HSPA8/β-arrestin1/A1AR axis (23). The potential of A2AAR to treat diseases such as Parkinson’s disease and systemic lupus erythematosus is currently under investigation (24, 25). A2BAR plays a role in schizophrenia and is involved in the regulation of pulmonary fibrosis and alcoholic steatohepatitis (26–28). Compared to other adenosine receptors, A3AR is less well-known and has received less attention in cardiovascular and diabetic diseases. It may, however, be a unique marker and target for inflammatory diseases such as rheumatoid arthritis (29). For more details on specific mechanisms, please refer to Table 1. Compared to the other listed adenosine receptors, the mechanisms of A2AAR in tumors are less studied, primarily related to pathways such as mitochondrial oxidative phosphorylation and ERK (37). For further details, please refer to Table 1.
2.3 Effect of adenosine receptors on components of the TME
The TME is a highly structured ecosystem composed of various immune cells, tumor-associated fibroblasts (CAFs), endothelial cells (ECs), and extracellular matrix (ECM). The composition of these components may vary depending on the specific tissue type and change with tumor development. The TME promotes tumor progression in a manner conducive to tumor growth and survival. A typical TME is suppressive, characterized by a high number of immunosuppressive cells and an acidic pH environment (69, 70). In the previous section, we described the mechanisms of adenosine receptors in tumor cells. This section will detail the targets and modes of action of adenosine receptors in tumor tissues and organs. The immune microenvironment composition of different tumors is complex and diverse, and the effects of adenosine receptors on these environments vary. Table 2 provides examples of this.
3 Mechanisms of adenosine receptors affecting the TME components
3.1 Adenosine receptors and immune cells
It is widely believed that immunosuppression leads to resistance to immunotherapy. CD8+ T cells and NK cells are of crucial in killing tumour cells and are also the cells with reduced capacity in the TME. In addition to the reduced lymphocyte activity caused by cytokines secreted by tumors, animal experiments have shown that inhibiting of adenosine receptors can enhance the antitumor capabilities of CD8+ T cells and NK cells (84, 85). Erika et al. demonstrated in vitro that ADORA2B is a key molecule involved in the CD8+ T cell anti-pancreatic tumour response (71). Simultaneous inhibition of ADORA2B and CD73 may enhance CD8+ T cell-mediated antitumor response in patients with pancreatic ductal adenocarcinoma (PDAC) (71). Similarly, inhibition of A2BAR signaling can restore T cell function and proliferation in the breast cancer microenvironment (87). A2AAR agonists have been demonstrated to inhibit the activity of a range of animal and human immune cells, both under healthy conditions and in the presence of tumors (86, 90). The mechanistic changes occurring within different immune cells when various adenosine receptors are antagonized have not been extensively studied. A more comprehensive understanding of these mechanistic changes will aid in developing more effective target designs and drug combinations. future research is expected to fill this knowledge gap.
3.2 Adenosine receptors and CAFs
CAFs play a critical role in tumour development and progression. They provide nutrients such as glutamine, essential for tumour cells when glutamine is depleted in the TME (91, 92). Changes in adenosine receptors, especially A2BAR, are also associated with CAFs. A2BAR is expressed in fibroblasts (93–95). Studies have found that A2BAR inhibitor PSB1115 can reduce the number of CAFs expressing fibroblast activation protein (FAP) and fibroblast growth factor (FGF-2) in the TME of melanoma mice (81). Additionally, the secretion of the cytokine CXCL12 is also reduced (81). The results suggest that MMP9 expression and contractile function in fibroblasts appear to be fully regulated by A2BAR, with no involvement from A2AAR (96). Furthermore, A2BAR can act as a mediator of adenosin- regulated of TGFβ on fibroblasts (96). Please refer to Figure 1 for specific details.
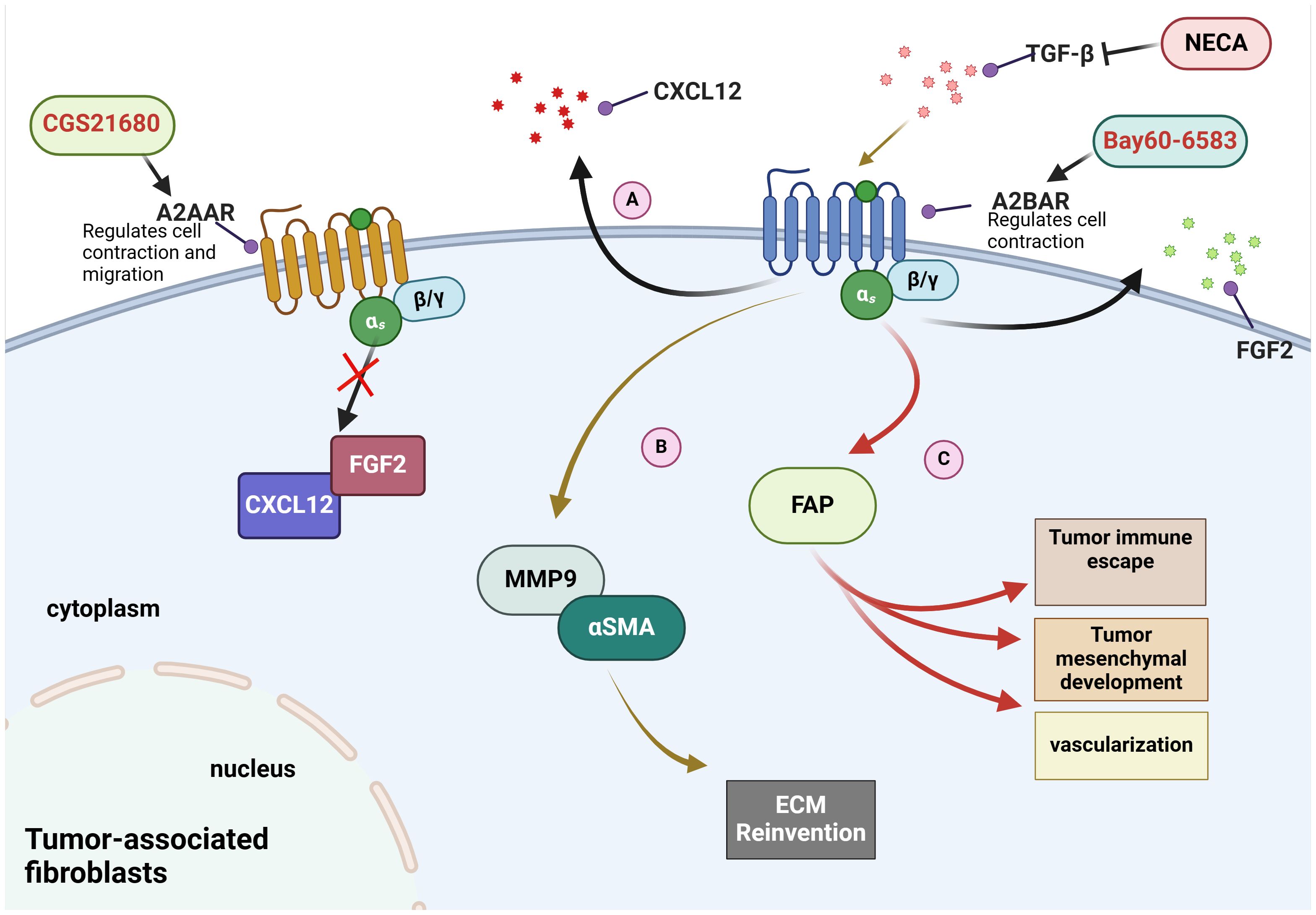
Figure 1 Mechanisms of adenosine receptors on fibroblasts in the tumour microenvironment. (A) Bay60-6583, an agonist of A2BAR, has been demonstrated to promote the secretion of CXCL12 and FGF2. (B) NECA, an adenosine analogue, inhibits the elevation of MMP9 and αSMA induced by TGF-β in A2BAR-dependent manner. (C) A2BAR promotes the expression of FAP to induce immune escape and vascular remodeling.
3.3 Adenosine receptors and tumour ECs
ECs, also known as vascular endothelial cells, typically refer to the single layer of flat epithelial cells located on the inner surface of the heart, blood vessels, and lymphatic vessels. This layer forms the endothelium (97). The TME is often characterized by hypoxia (91). Studies have shown that adenosine levels increase in the hypoxic TME, and A3AR expression is enhanced on glioblastoma stem -like cells (GSCs). A3AR receptors can promote the production of EC markers, including CD144, CD31, and VEGF, thus promoting the differentiation of GSCs into ECs under hypoxic conditions (83). Another study proposed that the A2BAR promoter contains HIF-1α response element that drive receptor expression in hypoxic cells, including ECs and epithelial cells (52, 98). Furthermore, inhibition of A2BAR on ECs can inhibit tumors under hypoxic TME conditions. A2BAR on ECs has been demonstrated to promote angiogenesis (99). In a mouse melanoma model, the use of the A2B receptor agonist Bay60-6583 increased the expression of VEGF-A and vascular density, thereby promoting melanoma growth (80, 99). For further details, please refer to Figure 2.
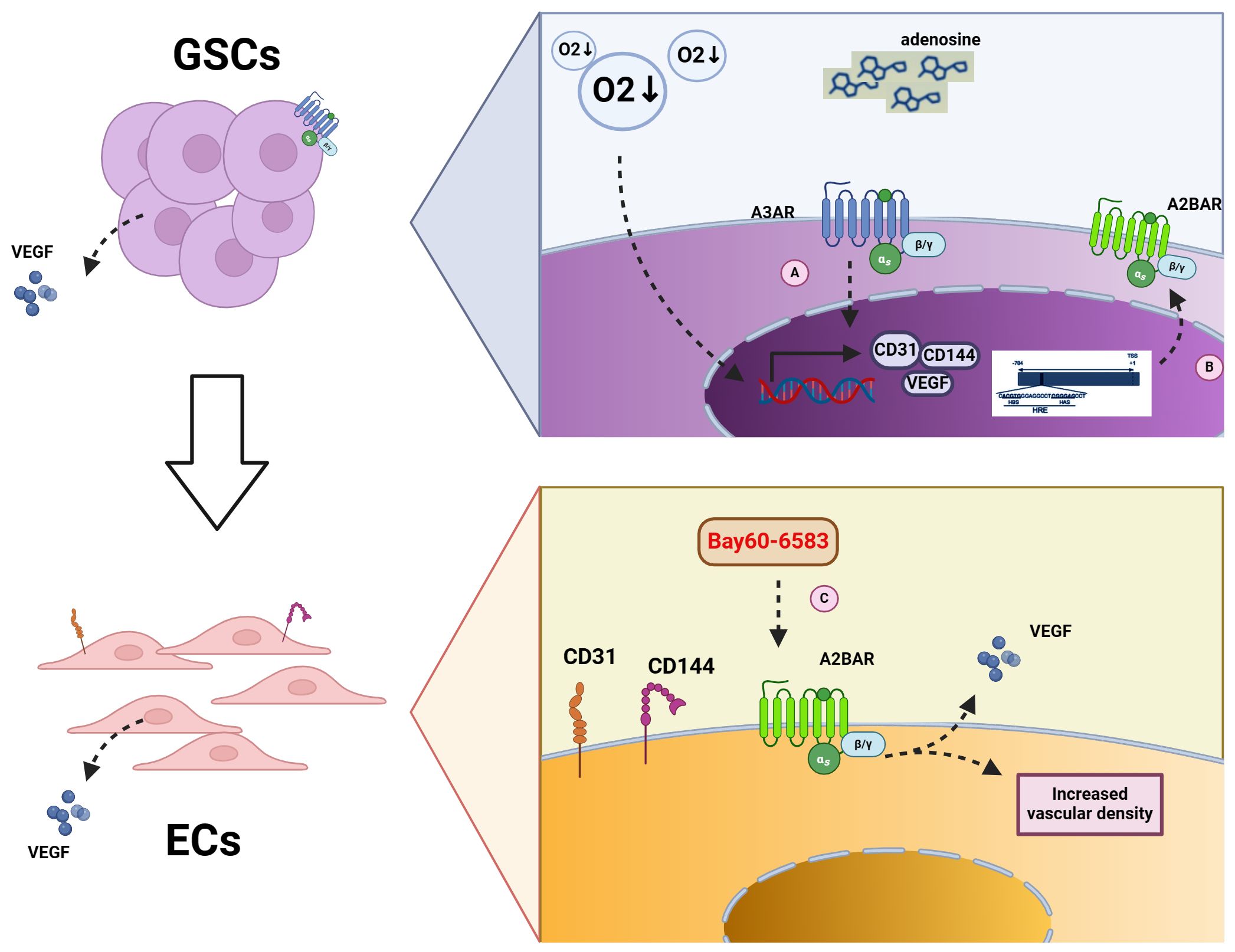
Figure 2 Mechanism of adenosine receptors on GSCs and ECs. (A) Increased A3AR content under hypoxic conditions leads to increased transcription of CD31, CD144, and VEGF in GSCs, contributing to the conversion of GSCs to ECs. (B) HIFα binds to the promoter of A2BAR, thereby increasing A2BAR content under hypoxic conditions. (C) The use of the A2BAR agonist Bay60-6583 results in the increase expression of A2BAR on ECs, which in turn causes the secretion of VEGF and an increase in vascular density.
3.4 Adenosine receptors and ECM in TME
ECM plays a critical role in tumour invasion and metastasis. ECM consists of many key components, including collagen, laminin, chondroitin sulfate proteoglycans and hyaluronic acid (100–102). The adenosine-mediated increases in laminin invasion and migration depends on both adenosine receptor-dependent and independent pathways (103). Among adenosine receptors, those regulated by extracellular-5’-nucleotidase (ecto-5’-NT)/CD73 are of particular interest in glioma cell adhesion and tumour cell-ECM interactions (104). Moreover, adenosine receptor A2BAR has been identified as involved in ecto-5’-NT/CD73-mediated arterial calcification (ACDC) (105). High levels of A2BAR in the tumour stroma suggest it may play a significant role. Further research is needed to elucidate the specific adenosine receptors involved in ECM and expand these studies.
3.5 Adenosine receptors and mesenchymal stem cells in the TME
Tumors recruit MSCs to the tumour area through the secretion of cytokines, playing a complex role in regulating carcinogenesis and tumourigenesis (106). Subsequently, MSCs can differentiate into more mature MSCs within the tumour area, such as ECs (107). There is little literature on adenosine receptors and MSCs. Studies have shown that elevated adenosine concentrations within tumour tissues can affect the paracrine factors released by MSCs (108). This is related to adenosine receptors, but which specific adenosine receptors are involved remains uncertain and requires further experimental validation.
4 Adenosine receptor antagonists and combination therapies
4.1 Current status of adenosine receptor antagonists development
Current research is developing both single and dual adenosine receptor antagonists. Zhi Li et al. designed and synthesized several novel A2A/A2B AR dual antagonists centered on triazole-pyrimidine-methylbenzonitrile, with satisfactory test results (109, 110). Compared to clinical antagonist AZD4635, some antagonists performed more prominently in vitro immunostimulatory anticancer activity (111). Studies have shown that adenosine receptor antagonists can regulate the immunosuppressive microenvironment, thereby modulating tumour immunity. However, delivering drugs to immune cells remains a challenge. In 2018, it was demonstrated that utilizing CAR-engineered T-cells as active companions, cross-linked multilayered liposome vesicles loaded with A2AAR-specific small-molecule antagonist SCH-58 may be effective. A2AAR-specific small molecule antagonist SCH-58261(SCH) was loaded into 261(SCH) (cMLV) and delivered to tumour-infiltrating T cells deep within the immunosuppressive TME. Adenosine receptor antagonists can be delivered to lymphocytes to exert their effects (112). More precise methods and improvements to existing methods are expected in the future.
4.2 Current status of combining adenosine receptor antagonists with other immunotherapies
treating tumors with immunotherapy involves various techniques, including ICIs, is a complex process, encompassing a range of. These include, adoptive cell transfer therapy (ACT), tumour-specific vaccines, small molecule immunotherapeutics and other drugs targeting the TME (23–27). This section discusses the latest progress in combining adenosine receptor inhibitors with immunotherapy. Table 3 summarizes the combinations of drugs and therapies.
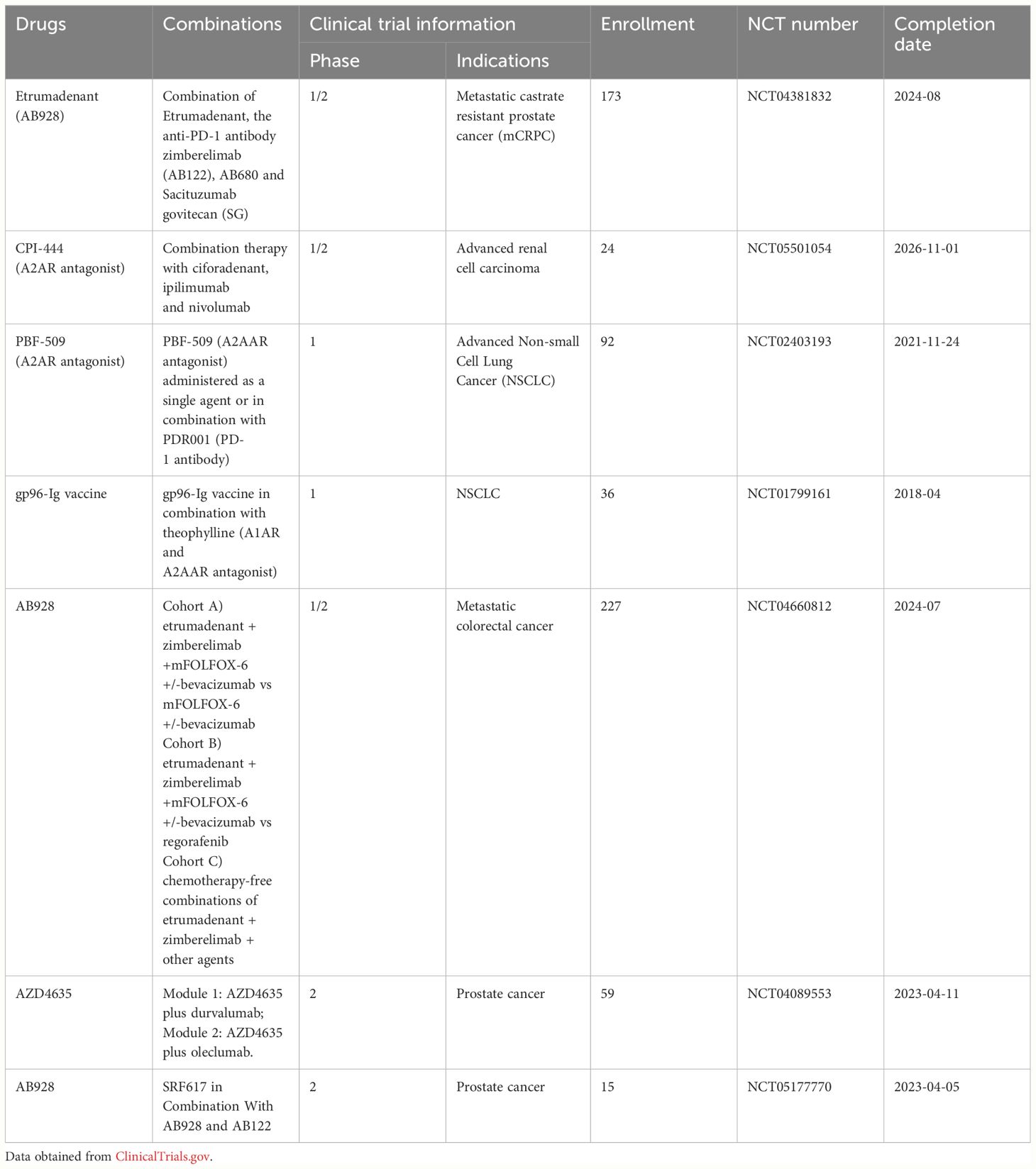
Table 3 Clinical trial listing of adenosine receptor antagonists in combination with tumour immunotherapy.
The use of ICIs has profoundly impacted the field of clinical adjuvant therapy (113, 114). However, some tumors remain unresponsive to ICIs, prompting scientists to explore combining ICIs with other therapeutic drugs to enhance ICIs efficacy. In recent years, adenosine receptor antagonists have been used in tumour immunotherapy as anticancer small molecule drugs. Takao et al. confirmed that elevated A2AAR expression in metastatic renal cell carcinoma is associated with reduced response and survival rates in patients treated with anti-VEGF drugs and anti-PD-1/anti-CTLA4 antibodies (115). The NCT02403193 trial indicated that PBF-509 (A2AAR antagonist) combined with PDR001 (PD-1 Ab) treatment for NSCLC is safer and more tolerable. Furthermore, a current clinical trial is evaluating the efficacy of combining PD-1 inhibitor zimberelimab with adenosine receptor antagonist etrumadenant. This trial is scheduled to end in August 2024. Another ongoing clinical trial investigates the combination of A2AAR antagonist ciforadenant with CTLA4 blocker ipilimumab and PD-1 inhibitor nivolumab in treating advanced renal cell carcinoma.
In addition to antibodies targeting immune checkpoints, numerous small molecule drugs with immunostimulatory effects are available. These drugs include small molecule inhibitors targeting cell growth and tumour metabolism, VEGF and VEGFR inhibitors, cytokine inhibitors, and more (88, 116, 117). Adenosine receptor antagonists are a special class of small molecule immunotherapeutics. Oral small molecule drugs overcome the limitations of antibody drugs, such as high adverse reactions, long half-life, and inconvenient infusion administration, opening a new field for drug discovery (117). Scientists are studying the effects of combining adenosine receptor antagonists with small molecule immunotherapeutics (used alone or in combination with small molecule immunotherapeutics and ICIs). VEGF is a protein that can stimulates angiogenesis, also inhibits antigen presentation, suppresses T-cell killing, and recruits immunosuppressive cells (117). VEGF antibodies, including ranibizumab and bevacizumab, have been shown to synergistically treat tumors with ICIs in vitro and in vivo (117, 118). Currently, a trial named NCT04660812 investigates the effects of combining bevacizumab with etrumadenant and zimberelimab. Adenosine is produced by the catalysis of adenosine monophosphate (AMP) by ectoenzyme CD73, making CD73 another important target for therapeutic intervention. Current drugs targeting CD73 include AB680 and OP5244/ORIC-533, as well as antibody drugs (119–121). Exosomes containing CD73 have been demonstrated to inhibit lymphocyte function and to enhance the efficacy of anti-PD-1 drugs (122). AstraZeneca and colleagues have launched clinical trials to study the efficacy of combining oleclumab (CD73 inhibitor) with AZD4635. Similarly, SRF617, a drug targeting surface molecule CD39, has also entered clinical trials. In contrast, the clinical efficacy of combining adenosine receptor antagonists with small molecule immunotherapeutics (cytokines) for tumour treatment has not yet been confirmed.
Tumor-specific vaccines utilize antigenic peptides extracted from tumors to activate immune cells (NK cells and T cells) within the TME (121). For example, heat shock protein GP96 (gp96-Ig) can act as a molecular chaperone, binding intracellular cancer embryonic antigens and progenitor antigens and activating T cells to kill tumour cells. Fariba et al. demonstrated that using nanoparticles loaded with siRNA to inhibit A2AAR and PD-1 immune checkpoints can enhance the efficacy of dendritic cell vaccines (123). Clinical trials conducted by Eckhard et al. demonstrated the efficacy of blocking adenosine receptors with theophylline combined with gp96-Ig tumour vaccine. Further research is deeded to determine the efficacy of combining adenosine receptor antagonists with tumour vaccines.
In addition to immunocellular therapy and checkpoint inhibitors, targeting other components of the tumour stroma, such as CAFs, can also help prevent tumors. In an in vitro study of the multitarget combination of the anti-CAFs drug tranilast and anti-tumour drug doxorubicin micelles (DTX-Ms), tranilast alone was less effective in inhibiting tumour growth in vivo. Additionally, it could only be used as an adjuvant drug with antitumor drugs (124). Given that adenosine receptor antagonists act on CAFs, it is hypothesized that combining tranilast and adenosine receptor antagonists may have a synergistic effect on CAFs. FAP protein is a core protein of CAFs, and most drugs targeting FAP in clinical trials are PET drugs. Targeting FAP protein radioligands is the latest development in nuclear medicine (125). OMTX705 is the first ADC targeting FAP. This drug is currently being developed for various gastrointestinal tumors (126). Given that A2BAR can regulate FAP protein in CAFs (81), adenosine receptor antagonists have the potential to promote radiodiagnosis or synergize with OMTX705. now in vivo or in vitro studies have been conducted in this field so far. OMTX705 is currently undergoing Phase I clinical trials (NCT05547321). Moreover, herbal medicines such as epigallocatechin gallate and prunella vulgaris polysaccharide that regulate CAFs may also be associated with various adenosine receptor antagonists in different tumors (127, 128). Further in vivo and in vitro studies are needed to confirm the link between adenosine receptor antagonists and drugs targeting CAFs.
Drugs can also target cytokines in the TME. These cytokines include IL-10, IL-8, and IFN-γ, which are upregulated in the TME (129–131). Adenosine receptor antagonists can act on the TME through these cytokines (77–79). The combination of adenosine receptor antagonists and cytokine drugs is a promising research field. CXCL8 (3–72)K11R/G31P is an antagonist of CXCL8 and has an inhibitory effect on tumour growth in various tumour types (132–134). So far, no in vivo or clinical studies have been conducted on the combination of CXCL8 (3–72)K11R/G31P with adenosine receptors or immunotherapy. An alternative approach is a clinical trial (NCT03400332) evaluating the safety and efficacy of an IL-8 monoclonal antibody (BMS-986253) in combination with the drug Nivolumab, an anti-PD-1 monoclonal antibody. BMS-986253 has been demonstrated to reduce tumour PMN-MDSC and stroma in triple-negative breast cancer. The efficacy of BMS-986253 combined with adenosine receptors or in triple therapy with immune checkpoint therapy remains to be evaluated (135). IFN-γ has complex multifaceted effects in the TME, with its impact on tumour growth depending on the balance between antitumor and protumor effects. Adenosine receptors regulate the secretion of IFN-γ, while IFN-γ acts by binding to adenosine receptors on immune cells such as macrophages (136). Furthermore, no clinical trials have confirmed the synergistic effect of IFN-γ with adenosine receptor antagonists. Additionally, the combined treatment of IFN-γ and adenosine receptors must consider the different microenvironmental conditions of patients, thus requiring extensive experimental validation. The combination of cytokine therapy with adenosine receptor antagonists for tumour treatment is another potential research field in the future.
5 Conclusions and prospects
The role of adenosine has been more widely studied both in vivo and in vitro. Research on adenosine receptors and tumour immunity has primarily focused on the effects of adenosine receptors on immune cells within tumour tissues. However, there is limited research on adenosine receptors and stromal cells or extracellular components in the TME. This review outlines the different roles of adenosine receptors in various TME. The review aims to outline the mechanisms of action between adenosine receptors and stromal cells or extracellular components, laying the groundwork for subsequent development of drugs combining adenosine receptor antagonists with the immune microenvironment. Additionally, this review provides an overview of the current status of combining adenosine receptor antagonist with immunotherapy, focusing on their combination with ICIs, tumour molecular vaccines, and small- molecule immunopharmaceuticals. Furthermore, we present our anticipated vision for the future of combining adenosine receptor antagonists with tumour immune microenvironment therapies (CAFs therapy and cytokine therapy), providing evidence for developing new immunotherapy combinations.
Author contributions
YH: Conceptualization, Data curation, Software, Validation, Visualization, Writing – original draft, Writing – review & editing. CD: Conceptualization, Data curation, Methodology, Validation, Writing – original draft, Writing – review & editing. MH: Conceptualization, Software, Validation, Visualization, Writing – original draft, Writing – review & editing. XW: Data curation, Methodology, Writing – review & editing. GW: Conceptualization, Funding acquisition, Methodology, Supervision, Writing – review & editing.
Funding
The author(s) declare financial support was received for the research, authorship, and/or publication of this article. This work was financially supported in part by grants from the Application Foundation Plan Project of Liaoning Provincial Department of Science and Technology (China) (No. 2023JH2/101300012).
Conflict of interest
The authors declare that the research was conducted in the absence of any commercial or financial relationships that could be construed as a potential conflict of interest.
Publisher’s note
All claims expressed in this article are solely those of the authors and do not necessarily represent those of their affiliated organizations, or those of the publisher, the editors and the reviewers. Any product that may be evaluated in this article, or claim that may be made by its manufacturer, is not guaranteed or endorsed by the publisher.
References
1. Sharma S, Singh N, Turk AA, Wan I, Guttikonda A, Dong JL, et al. Molecular insights into clinical trials for immune checkpoint inhibitors in colorectal cancer: Unravelling challenges and future directions. World J Gastroenterol. (2024) 30:1815–35. doi: 10.3748/wjg.v30.i13.1815
2. Morse MA, Gwin WR 3rd, Mitchell DA. Vaccine therapies for cancer: then and now. Target Oncol. (2021) 16:121–52. doi: 10.1007/s11523-020-00788-w
3. Zhang L, Xue J, Zhang N, Wang Y, Yang X, Wang S, et al. The efficacy and safety of bevacizumab as a salvage therapy for patients with advanced hepatocellular carcinoma targeting immune tolerance. Am J Cancer Res. (2023) 13:3582–90. doi: 10.1172/jci.insight.94296
4. Ying L, Cheng M, Lu Y, Tao Q, Chen X, Shen B, et al. Glutamine metabolism scoring predicts prognosis and therapeutic resistance in hepatocellular carcinoma. Pathol Oncol Res. (2021) 27:1610075. doi: 10.3389/pore.2021.1610075
5. Tay SH, Toh MMX, Thian YL, Vellayappan BA, Fairhurst AM, Chan YH, et al. Cytokine release syndrome in cancer patients receiving immune checkpoint inhibitors: A case series of 25 patients and review of the literature. Front Immunol. (2022) 13:807050. doi: 10.3389/fimmu.2022.807050
6. Huang Y, Gu Z, Fan Y, Zhai G, Zhao X, Sun Q, et al. Inhibition of the adenosinergic pathway: the indispensable part of oncological therapy in the future. Purinergic Signal. (2019) 15:53–67. doi: 10.1007/s11302-018-9641-4
7. Beavis PA, Milenkovski N, Henderson MA, John LB, Allard B, Loi S, et al. Adenosine receptor 2A blockade increases the efficacy of anti-PD-1 through enhanced antitumor T-cell responses. Cancer Immunol Res. (2015) 3:506–17. doi: 10.1158/2326-6066.CIR-14-0211
8. Priebe T, Platsoucas CD, Nelson JA. Adenosine receptors and modulation of natural killer cell activity by purine nucleosides. Cancer Res. (1990) 50:4328–31.
9. Joos G, Jakim J, Kiss B, Szamosi R, Papp T, Felszeghy S, et al. Involvement of adenosine A3 receptors in the chemotactic navigation of macrophages towards apoptotic cells. Immunol Lett. (2017) 183:62–72. doi: 10.1016/j.imlet.2017.02.002
10. Tseng CJ, Kuan CJ, Chu H, Tung CS. Effect of caffeine treatment on plasma renin activity and angiotensin I concentrations in rats on a low sodium diet. Life Sci. (1993) 52:883–90. doi: 10.1016/0024-3205(93)90518-8
11. Wu Z, Xiang Q, Feng L, Wu D, Huang S, Zhang L, et al. Adenosine-ADORA2A promotes ang-induced angiogenesis in intrauterine growth restriction placenta via the stat3/akt pathway. Arterioscler Thromb Vasc Biol. (2023) 43:e190–209. doi: 10.1161/ATVBAHA.122.318948
12. Siokas V, Aloizou AM, Tsouris Z, Liampas I, Liakos P, Calina D, et al. ADORA2A rs5760423 and CYP1A2 rs762551 Polymorphisms as Risk Factors for Parkinson's Disease. J Clin Med. (2021) 10(3):381. doi: 10.3390/jcm10030381
13. Peterfreund RA, MacCollin M, Gusella J, Fink JS. Characterization and expression of the human A2a adenosine receptor gene. J Neurochem. (1996) 66:362–8. doi: 10.1046/j.1471-4159.1996.66010362.x
14. Christofi FL, Zhang H, Yu JG, Guzman J, Xue J, Kim M, et al. Differential gene expression of adenosine A1, A2a, A2b, and A3 receptors in the human enteric nervous system. J Comp Neurol. (2001) 439:46–64. doi: 10.1002/cne.1334
15. Happe HK, Bylund DB, Murrin LC. Alpha-2 adrenergic receptor functional coupling to G proteins in rat brain during postnatal development. J Pharmacol Exp Ther. (1999) 288:1134–42.
16. Cherchi F, Pugliese AM, Coppi E. Oligodendrocyte precursor cell maturation: role of adenosine receptors. Neural Regener Res. (2021) 16:1686–92. doi: 10.4103/1673-5374.306058
17. Huang W, Bai S, Zuo X, Tang W, Chen P, Chen X, et al. An adenosine A1R-A2aR imbalance regulates low glucose/hypoxia-induced microglial activation, thereby contributing to oligodendrocyte damage through NF-kappaB and CREB phosphorylation. Int J Mol Med. (2018) 41:3559–69. doi: 10.3892/ijmm.2018.3546
18. Huang ZL, Zhang Z, Qu WM. Roles of adenosine and its receptors in sleep-wake regulation. Int Rev Neurobiol. (2014) 119:349–71. doi: 10.1016/B978-0-12-801022-8.00014-3
19. Knight D, Zheng X, Rocchini C, Jacobson M, Bai T, Walker B. Adenosine A3 receptor stimulation inhibits migration of human eosinophils. J Leukoc Biol. (1997) 62:465–8. doi: 10.1002/jlb.62.4.465
20. Perfilova VN, Muzyko EA, Taran AS, Shevchenko AA, Naumenko LV. Problems and prospects for finding new pharmacological agents among adenosine receptor agonists, antagonists, or their allosteric modulators for the treatment of cardiovascular diseases. BioMed Khim. (2023) 69:353–70. doi: 10.18097/PBMC
21. Nayeem MA, Hanif A, Geldenhuys WJ, Agba S. Crosstalk between adenosine receptors and CYP450-derived oxylipins in the modulation of cardiovascular, including coronary reactive hyperemic response. Pharmacol Ther. (2022) 240:108213. doi: 10.1016/j.pharmthera.2022.108213
22. Eisenstein A, Patterson S, Ravid K. The many faces of the A2b adenosine receptor in cardiovascular and metabolic diseases. J Cell Physiol. (2015) 230:2891–7. doi: 10.1002/jcp.25043
23. Zhang K, Zhang H, Wang F, Gao S, Sun C. HSPA8 is identified as a novel regulator of hypertensive disorders in pregnancy by modulating the beta-arrestin1/A1AR axis. Reprod Sci. (2022) 29:564–77. doi: 10.1007/s43032-021-00719-8
24. Zheng J, Zhang X, Zhen X. Development of adenosine A(2A) receptor antagonists for the treatment of parkinson's disease: A recent update and challenge. ACS Chem Neurosci. (2019) 10:783–91. doi: 10.1021/acschemneuro.8b00313
25. Bortoluzzi A, Vincenzi F, Govoni M, Padovan M, Ravani A, Borea PA, et al. A2A adenosine receptor upregulation correlates with disease activity in patients with systemic lupus erythematosus. Arthritis Res Ther. (2016) 18:192. doi: 10.1186/s13075-016-1089-8
26. Zhao N, Xia G, Cai J, Li Z, Lv XW. Adenosine receptor A2B mediates alcoholic hepatitis by regulating cAMP levels and the NF-KB pathway. Toxicol Lett. (2022) 359:84–95. doi: 10.1016/j.toxlet.2022.01.012
27. Effendi WI, Nagano T. A2B adenosine receptor in idiopathic pulmonary fibrosis: pursuing proper pit stop to interfere with disease progression. Int J Mol Sci. (2023) 24(5):4428. doi: 10.3390/ijms24054428
28. Ma Q, Wang D, Li Y, Yang H, Li Y, Wang J, et al. Activation of A(2B) adenosine receptor protects against demyelination in a mouse model of schizophrenia. Exp Ther Med. (2022) 23:396. doi: 10.3892/etm
29. Fishman P, Cohen S. The A3 adenosine receptor (A3AR): therapeutic target and predictive biological marker in rheumatoid arthritis. Clin Rheumatol. (2016) 35:2359–62. doi: 10.1007/s10067-016-3202-4
30. Tian D, Li J, Zou L, Lin M, Shi X, Hu Y, et al. Adenosine A1 receptor deficiency aggravates extracellular matrix accumulation in diabetic nephropathy through disturbance of peritubular microenvironment. J Diabetes Res. (2021) 2021:5584871. doi: 10.1155/2021/5584871
31. Pak ES, Cha JJ, Cha DR, Kanasaki K, Ha H. Adenosine receptors as emerging therapeutic targets for diabetic kidney disease. Kidney Res Clin Pract. (2022) 41:S74–88. doi: 10.23876/j.krcp.22.011
32. Antonioli L, Fornai M, Blandizzi C, Pacher P, Hasko G. Adenosine signaling and the immune system: When a lot could be too much. Immunol Lett. (2019) 205:9–15. doi: 10.1016/j.imlet.2018.04.006
33. Borea PA, Gessi S, Merighi S, Vincenzi F, Varani K. Pharmacology of adenosine receptors: the state of the art. Physiol Rev. (2018) 98:1591–625. doi: 10.1152/physrev.00049.2017
34. Xu H, Qiu Q, Hu P, Hoxha K, Jang E, O'Reilly M, et al. MSUT2 regulates tau spreading via adenosinergic signaling mediated ASAP1 pathway in neurons. Acta Neuropathol. (2024) 147:55. doi: 10.1007/s00401-024-02703-3
35. Ma H, Li Q, Wang J, Pan J, Su Z, Liu S. Dual inhibition of ornithine decarboxylase and A(1) adenosine receptor efficiently suppresses breast tumor cells. Front Oncol. (2021) 11:636373. doi: 10.3389/fonc.2021.636373
36. Zeynali P, Jazi MS, Asadi J, Jafari SM. A1 adenosine receptor antagonist induces cell apoptosis in KYSE-30 and YM-1 esophageal cancer cell lines. Biomedicine (Taipei). (2023) 13:54–61. doi: 10.37796/2211-8039.1394
37. Daniele S, Zappelli E, Natali L, Martini C, Trincavelli ML. Modulation of A1 and A2B adenosine receptor activity: a new strategy to sensitise glioblastoma stem cells to chemotherapy. Cell Death Dis. (2014) 5:e1539. doi: 10.1038/cddis.2014.487
38. Jacobson KA, Gao ZG, Matricon P, Eddy MT, Carlsson J. Adenosine A(2A) receptor antagonists: from caffeine to selective non-xanthines. Br J Pharmacol. (2022) 179:3496–511. doi: 10.1111/bph.15103
39. Hanif A, Agba SO, Ledent C, Tilley SL, Morisseau C, Nayeem MA. Adenosine A(2A) receptor and vascular response: role of soluble epoxide hydrolase, adenosine A(1) receptor and angiotensin-II. Mol Cell Biochem. (2021) 476:1965–78. doi: 10.1007/s11010-021-04049-w
40. Etique N, Grillier-Vuissoz I, Lecomte J, Flament S. Crosstalk between adenosine receptor (A2A isoform) and ERalpha mediates ethanol action in MCF-7 breast cancer cells. Oncol Rep. (2009) 21:977–81. doi: 10.3892/or_00000311
41. Ibrahim AS, El-Shishtawy MM, Zhang W, Caldwell RB, Liou GI. A((2)A) adenosine receptor (A((2)A)AR) as a therapeutic target in diabetic retinopathy. Am J Pathol. (2011) 178:2136–45. doi: 10.1016/j.ajpath.2011.01.018
42. Liou GI, Auchampach JA, Hillard CJ, Zhu G, Yousufzai B, Mian S, et al. Mediation of cannabidiol anti-inflammation in the retina by equilibrative nucleoside transporter and A2A adenosine receptor. Invest Ophthalmol Vis Sci. (2008) 49:5526–31. doi: 10.1167/iovs.08-2196
43. Gessi S, Bencivenni S, Battistello E, Vincenzi F, Colotta V, Catarzi D, et al. Inhibition of A(2A) adenosine receptor signaling in cancer cells proliferation by the novel antagonist TP455. Front Pharmacol. (2017) 8:888. doi: 10.3389/fphar.2017.00888
44. Gessi S, Merighi S, Sacchetto V, Simioni C, Borea PA. Adenosine receptors and cancer. Biochim Biophys Acta. (2011) 1808:1400–12. doi: 10.1016/j.bbamem.2010.09.020
45. Donoso MV, Lopez R, Miranda R, Briones R, Huidobro-Toro JP. A2B adenosine receptor mediates human chorionic vasoconstriction and signals through arachidonic acid cascade. Am J Physiol Heart Circ Physiol. (2005) 288:H2439–49. doi: 10.1152/ajpheart.00548.2004
46. Bessa-Goncalves M, Braganca B, Martins-Dias E, Vinhas A, Certal M, Rodrigues T, et al. Blockage of the adenosine A(2B) receptor prevents cardiac fibroblasts overgrowth in rats with pulmonary arterial hypertension. Purinergic Signal. (2024) 20:163–79. doi: 10.1007/s11302-023-09952-z
47. He F, Wang F, Xiang H, Ma Y, Lu Q, Xia Y, et al. Activation of adenosine A2B receptor alleviates myocardial ischemia-reperfusion injury by inhibiting endoplasmic reticulum stress and restoring autophagy flux. Arch Biochem Biophys. (2024) 754:109945. doi: 10.1016/j.abb.2024.109945
48. Chandrasekera PC, McIntosh VJ, Cao FX, Lasley RD. Differential effects of adenosine A2a and A2b receptors on cardiac contractility. Am J Physiol Heart Circ Physiol. (2010) 299:H2082–9. doi: 10.1152/ajpheart.00511.2010
49. Eckle T, Krahn T, Grenz A, Kohler D, Mittelbronn M, Ledent C, et al. Cardioprotection by ecto-5'-nucleotidase (CD73) and A2B adenosine receptors. Circulation. (2007) 115:1581–90. doi: 10.1161/CIRCULATIONAHA.106.669697
50. Mendoza-Soto P, Jara C, Torres-Arevalo A, Oyarzun C, Mardones GA, Quezada-Monras C, et al. Pharmacological blockade of the adenosine A(2B) receptor is protective of proteinuria in diabetic rats, through affecting focal adhesion kinase activation and the adhesion dynamics of podocytes. Cells. (2024) 13(10):846. doi: 10.3390/cells13100846
51. Jafari SM, Joshaghani HR, Panjehpour M, Aghaei M. A2B adenosine receptor agonist induces cell cycle arrest and apoptosis in breast cancer stem cells via ERK1/2 phosphorylation. Cell Oncol (Dordr). (2018) 41:61–72. doi: 10.1007/s13402-017-0359-z
52. Cekic C, Sag D, Li Y, Theodorescu D, Strieter RM, Linden J. Adenosine A2B receptor blockade slows growth of bladder and breast tumors. J Immunol. (2012) 188:198–205. doi: 10.4049/jimmunol.1101845
53. Hajiahmadi S, Panjehpour M, Aghaei M, Shabani M. Activation of A2b adenosine receptor regulates ovarian cancer cell growth: involvement of Bax/Bcl-2 and caspase-3. Biochem Cell Biol. (2015) 93:321–9. doi: 10.1139/bcb-2014-0117
54. Erices JI, Niechi I, Uribe-Ojeda A, Toro MLA, Garcia-Romero N, Carrion-Navarro J, et al. The low affinity A2B adenosine receptor enhances migratory and invasive capacity in vitro and angiogenesis in vivo of glioblastoma stem-like cells. Front Oncol. (2022) 12:969993. doi: 10.3389/fonc.2022.969993
55. Giacomelli C, Daniele S, Romei C, Tavanti L, Neri T, Piano I, et al. The A(2B) Adenosine Receptor Modulates the Epithelial- Mesenchymal Transition through the Balance of cAMP/PKA and MAPK/ERK Pathway Activation in Human Epithelial Lung Cells. Front Pharmacol. (2018) 9:54. doi: 10.3389/fphar.2018.00054
56. Molck C, Ryall J, Failla LM, Coates JL, Pascussi JM, Heath JK, et al. The A(2b) adenosine receptor antagonist PSB-603 promotes oxidative phosphorylation and ROS production in colorectal cancer cells via adenosine receptor-independent mechanism. Cancer Lett. (2016) 383:135–43. doi: 10.1016/j.canlet.2016.09.018
57. Barbazan J, Majellaro M, Martinez AL, Brea JM, Sotelo E, Abal M. Identification of A(2B)AR as a potential target in colorectal cancer using novel fluorescent GPCR ligands. BioMed Pharmacother. (2022) 153:113408. doi: 10.1016/j.biopha.2022.113408
58. Singh AK, Mahalingam R, Squillace S, Jacobson KA, Tosh DK, Dharmaraj S, et al. Targeting the A(3) adenosine receptor to prevent and reverse chemotherapy-induced neurotoxicities in mice. Acta Neuropathol Commun. (2022) 10:11. doi: 10.1186/s40478-022-01315-w
59. Durante M, Squillace S, Lauro F, Giancotti LA, Coppi E, Cherchi F, et al. Adenosine A3 agonists reverse neuropathic pain via T cell-mediated production of IL-10. J Clin Invest. (2021) 131(7):e139299. doi: 10.1172/JCI139299
60. Lucarini E, Coppi E, Micheli L, Parisio C, Vona A, Cherchi F, et al. Acute visceral pain relief mediated by A3AR agonists in rats: involvement of N-type voltage-gated calcium channels. Pain. (2020) 161:2179–90. doi: 10.1097/j.pain.0000000000001905
61. Bai H, Zhang Z, Liu L, Wang X, Song X, Gao L. Activation of adenosine A3 receptor attenuates progression of osteoarthritis through inhibiting the NLRP3/caspase-1/GSDMD induced signaling. J Cell Mol Med. (2022) 26:4230–43. doi: 10.1111/jcmm.17438
62. Papp KA, Beyska-Rizova S, Gantcheva ML, Slavcheva Simeonova E, Brezoev P, Celic M, et al. Efficacy and safety of piclidenoson in plaque psoriasis: Results from a randomized phase 3 clinical trial (COMFORT-1). J Eur Acad Dermatol Venereol. (2024) 38:1112–20. doi: 10.1111/jdv.19811
63. Fishman P, Stemmer SM, Bareket-Samish A, Silverman MH, Kerns WD. Targeting the A3 adenosine receptor to treat hepatocellular carcinoma: anti-cancer and hepatoprotective effects. Purinergic Signal. (2023) 19:513–22. doi: 10.1007/s11302-023-09925-2
64. Kotulova J, Lonova K, Kubickova A, Vrbkova J, Kourilova P, Hajduch M, et al. 2−Cl−IB−MECA regulates the proliferative and drug resistance pathways, and facilitates chemosensitivity in pancreatic and liver cancer cell lines. Int J Mol Med. (2022) 49(3):31. doi: 10.3892/ijmm
65. Solanki N, Patel L, Shah S, Patel A, Patel S, Patel M, et al. Pharmacological Study of A3 Adenosine Receptor agonist (AB Meca) in Xenograft Lung Cancer Model in Mice through In Silico and In Vivo Approach: Targeting TNF-alpha. Curr Drug Discovery Technol. (2022) 19:e140122195498. doi: 10.2174/1570163818666210810142022
66. Jafari SM, Panjehpour M, Aghaei M, Joshaghani HR, Enderami SE. A3 adenosine receptor agonist inhibited survival of breast cancer stem cells via GLI-1 and ERK1/2 pathway. J Cell Biochem. (2017) 118:2909–20. doi: 10.1002/jcb.v118.9
67. Fishman P, Bar-Yehuda S, Ardon E, Rath-Wolfson L, Barrer F, Ochaion A, et al. Targeting the A3 adenosine receptor for cancer therapy: inhibition of prostate carcinoma cell growth by A3AR agonist. Anticancer Res. (2003) 23:2077–83.
68. Itzhak I, Bareket-Samish A, Fishman P. Namodenoson inhibits the growth of pancreatic carcinoma via deregulation of the wnt/beta-catenin, NF-kappaB, and RAS signaling pathways. Biomolecules. (2023) 13(11):1584. doi: 10.3390/biom13111584
69. Arner EN, Rathmell JC. Metabolic programming and immune suppression in the tumor microenvironment. Cancer Cell. (2023) 41:421–33. doi: 10.1016/j.ccell.2023.01.009
70. de la Cruz-Lopez KG, Castro-Munoz LJ, Reyes-Hernandez DO, Garcia-Carranca A, Manzo-Merino J. Lactate in the regulation of tumor microenvironment and therapeutic approaches. Front Oncol. (2019) 9:1143. doi: 10.3389/fonc.2019.01143
71. Faraoni EY, Singh K, Chandra V, Le Roux O, Dai Y, Sahin I, et al. CD73-dependent adenosine signaling through adora2b drives immunosuppression in ductal pancreatic cancer. Cancer Res. (2023) 83:1111–27. doi: 10.1158/0008-5472.CAN-22-2553
72. Raskovalova T, Huang X, Sitkovsky M, Zacharia LC, Jackson EK, Gorelik E. Gs protein-coupled adenosine receptor signaling and lytic function of activated NK cells. J Immunol. (2005) 175:4383–91. doi: 10.4049/jimmunol.175.7.4383
73. Bastid J, Regairaz A, Bonnefoy N, Dejou C, Giustiniani J, Laheurte C, et al. Inhibition of CD39 enzymatic function at the surface of tumor cells alleviates their immunosuppressive activity. Cancer Immunol Res. (2015) 3:254–65. doi: 10.1158/2326-6066.CIR-14-0018
74. Remley VA, Linden J, Bauer TW, Dimastromatteo J. Unlocking antitumor immunity with adenosine receptor blockers. Cancer Drug Resist. (2023) 6:748–67. doi: 10.20517/cdr.2023.63
75. Hatfield S, Veszeleiova K, Steingold J, Sethuraman J, Sitkovsky M. Mechanistic justifications of systemic therapeutic oxygenation of tumors to weaken the hypoxia inducible factor 1alpha-mediated immunosuppression. Adv Exp Med Biol. (2019) 1136:113–21. doi: 10.1007/978-3-030-12734-3_8
76. Koscso B, Csoka B, Pacher P, Hasko G. Investigational A(3) adenosine receptor targeting agents. Expert Opin Investig Drugs. (2011) 20:757–68. doi: 10.1517/13543784.2011.573785
77. Torres-Pineda DB, de Lourdes Mora-Garcia M, Garcia-Rocha R, Hernandez-Montes J, Weiss-Steider B, Montesinos-Montesinos JJ, et al. Corrigendum to "Adenosine augments the production of IL-10 in cervical cancer cells through interaction with the A2B adenosine receptor, resulting in protection against the activity of cytotoxic T cells" [Cytokine 130 (2020) 155082]. Cytokine. (2020) 133:155110. doi: 10.1016/j.cyto.2020.155110
78. Merighi S, Simioni C, Gessi S, Varani K, Mirandola P, Tabrizi MA, et al. A(2B) and A(3) adenosine receptors modulate vascular endothelial growth factor and interleukin-8 expression in human melanoma cells treated with etoposide and doxorubicin. Neoplasia. (2009) 11:1064–73. doi: 10.1593/neo.09768
79. Eini H, Frishman V, Yulzari R, Kachko L, Lewis EC, Chaimovitz C, et al. Caffeine promotes anti-tumor immune response during tumor initiation: Involvement of the adenosine A2A receptor. Biochem Pharmacol. (2015) 98:110–8. doi: 10.1016/j.bcp.2015.08.092
80. Sorrentino C, Miele L, Porta A, Pinto A, Morello S. Myeloid-derived suppressor cells contribute to A2B adenosine receptor-induced VEGF production and angiogenesis in a mouse melanoma model. Oncotarget. (2015) 6:27478–89. doi: 10.18632/oncotarget.v6i29
81. Sorrentino C, Miele L, Porta A, Pinto A, Morello S. Activation of the A2B adenosine receptor in B16 melanomas induces CXCL12 expression in FAP-positive tumor stromal cells, enhancing tumor progression. Oncotarget. (2016) 7:64274–88. doi: 10.18632/oncotarget.v7i39
82. Harish A, Hohana G, Fishman P, Arnon O, Bar-Yehuda S. A3 adenosine receptor agonist potentiates natural killer cell activity. Int J Oncol. (2003) 23:1245–9. doi: 10.3892/ijo
83. Rocha R, Torres A, Ojeda K, Uribe D, Rocha D, Erices J, et al. The adenosine A(3) receptor regulates differentiation of glioblastoma stem-like cells to endothelial cells under hypoxia. Int J Mol Sci. (2018) 19(4):1228. doi: 10.3390/ijms19041228
84. Ohta A, Gorelik E, Prasad SJ, Ronchese F, Lukashev D, Wong MK, et al. A2A adenosine receptor protects tumors from antitumor T cells. Proc Natl Acad Sci U S A. (2006) 103:13132–7. doi: 10.1073/pnas.0605251103
85. Kjaergaard J, Hatfield S, Jones G, Ohta A, Sitkovsky M. A(2A) adenosine receptor gene deletion or synthetic A(2A) antagonist liberate tumor-reactive CD8(+) T cells from tumor-induced immunosuppression. J Immunol. (2018) 201:782–91. doi: 10.4049/jimmunol.1700850
86. Young A, Ngiow SF, Gao Y, Patch AM, Barkauskas DS, Messaoudene M, et al. A2AR adenosine signaling suppresses natural killer cell maturation in the tumor microenvironment. Cancer Res. (2018) 78:1003–16. doi: 10.1158/0008-5472.CAN-17-2826
87. Tay AHM, Prieto-Diaz R, Neo S, Tong L, Chen X, Carannante V, et al. A(2B) adenosine receptor antagonists rescue lymphocyte activity in adenosine-producing patient-derived cancer models. J Immunother Cancer. (2022) 10(5):e004592. doi: 10.1136/jitc-2022-004592
88. Aboulkheyr Es H, Bigdeli B, Zhand S, Aref AR, Thiery JP, Warkiani ME. Mesenchymal stem cells induce PD-L1 expression through the secretion of CCL5 in breast cancer cells. J Cell Physiol. (2021) 236:3918–28. doi: 10.1002/jcp.30135
89. Arab S, Kheshtchin N, Ajami M, Ashurpoor M, Safvati A, Namdar A, et al. Increased efficacy of a dendritic cell-based therapeutic cancer vaccine with adenosine receptor antagonist and CD73 inhibitor. Tumour Biol. (2017) 39:1010428317695021. doi: 10.1177/1010428317695021
90. Kuldova M, Svoboda J, Kovaru F, Vannucci L, Kovaru H, Fiserova A. NK cell-mediated cytotoxicity modulation by A(2) adenosine receptor agonist in different mammalian species. Folia Microbiol (Praha). (2009) 54:364–8. doi: 10.1007/s12223-009-0051-4
91. Bai R, Li Y, Jian L, Yang Y, Zhao L, Wei M. The hypoxia-driven crosstalk between tumor and tumor-associated macrophages: mechanisms and clinical treatment strategies. Mol Cancer. (2022) 21:177. doi: 10.1186/s12943-022-01645-2
92. Liu T, Han C, Fang P, Ma Z, Wang X, Chen H, et al. Cancer-associated fibroblast-specific lncRNA LINC01614 enhances glutamine uptake in lung adenocarcinoma. J Hematol Oncol. (2022) 15:141. doi: 10.1186/s13045-022-01359-4
93. Feng W, Song Y, Chen C, Lu ZZ, Zhang Y. Stimulation of adenosine A(2B) receptors induces interleukin-6 secretion in cardiac fibroblasts via the PKC-delta-P38 signaling pathway. Br J Pharmacol. (2010) 159:1598–607. doi: 10.1111/j.1476-5381.2009.00558.x
94. Zhong H, Belardinelli L, Maa T, Zeng D. Synergy between A2B adenosine receptors and hypoxia in activating human lung fibroblasts. Am J Respir Cell Mol Biol. (2005) 32:2–8. doi: 10.1165/rcmb.2004-0103OC
95. Tang J, Jiang X, Zhou Y, Dai Y. Effects of A2BR on the biological behavior of mouse renal fibroblasts during hypoxia. Mol Med Rep. (2015) 11:4397–402. doi: 10.3892/mmr.2015.3320
96. Vasiukov G, Menshikh A, Owens P, Novitskaya T, Hurley P, Blackwell T, et al. Adenosine/TGFbeta axis in regulation of mammary fibroblast functions. PloS One. (2021) 16:e0252424. doi: 10.1371/journal.pone.0252424
97. Kruger-Genge A, Blocki A, Franke RP, Jung F. Vascular endothelial cell biology: an update. Int J Mol Sci. (2019) 20(18):1411. doi: 10.3390/ijms20184411
98. Kong T, Westerman KA, Faigle M, Eltzschig HK, Colgan SP. HIF-dependent induction of adenosine A2B receptor in hypoxia. FASEB J. (2006) 20:2242–50. doi: 10.1096/fj.06-6419com
99. Ryzhov S, Novitskiy SV, Zaynagetdinov R, Goldstein AE, Carbone DP, Biaggioni I, et al. Host A(2B) adenosine receptors promote carcinoma growth. Neoplasia. (2008) 10:987–95. doi: 10.1593/neo.08478
100. Walker K, Basehore BM, Goyal A, Zito PM. Hyaluronic Acid. Treasure Island (FL: StatPearls (2024).
101. Bae M, Ngo H, Kang YJ, Lee SJ, Park W, Jo Y, et al. Laminin-augmented decellularized extracellular matrix ameliorating neural differentiation and neuroinflammation in human mini-brains. Small. (2023) 20(23):e2308815. doi: 10.1002/smll.202308815
102. Pinter A, Hevesi Z, Zahola P, Alpar A, Hanics J. Chondroitin sulfate proteoglycan-5 forms perisynaptic matrix assemblies in the adult rat cortex. Cell Signal. (2020) 74:109710. doi: 10.1016/j.cellsig.2020.109710
103. Virtanen SS, Kukkonen-Macchi A, Vainio M, Elima K, Harkonen PL, Jalkanen S, et al. Adenosine inhibits tumor cell invasion via receptor-independent mechanisms. Mol Cancer Res. (2014) 12:1863–74. doi: 10.1158/1541-7786.MCR-14-0302-T
104. Cappellari AR, Vasques GJ, Bavaresco L, Braganhol E, Battastini AM. Involvement of ecto-5'-nucleotidase/CD73 in U138MG glioma cell adhesion. Mol Cell Biochem. (2012) 359:315–22. doi: 10.1007/s11010-011-1025-9
105. Cuevas RA, Wong R, Joolharzadeh P, Moorhead WJ 3rd, Chu CC, Callahan Jt, et al. Ecto-5'-nucleotidase (Nt5e/CD73)-mediated adenosine signaling attenuates TGFbeta-2 induced elastin and cellular contraction. Am J Physiol Cell Physiol. (2023) 324:C327–C38. doi: 10.1152/ajpcell.00054.2022
106. Lan T, Luo M, Wei X. Mesenchymal stem/stromal cells in cancer therapy. J Hematol Oncol. (2021) 14:195. doi: 10.1186/s13045-021-01208-w
107. Janeczek Portalska K, Leferink A, Groen N, Fernandes H, Moroni L, van Blitterswijk C, et al. Endothelial differentiation of mesenchymal stromal cells. PloS One. (2012) 7:e46842. doi: 10.1371/journal.pone.0046842
108. Pietrobono D, Giacomelli C, Marchetti L, Martini C, Trincavelli ML. High adenosine extracellular levels induce glioblastoma aggressive traits modulating the mesenchymal stromal cell secretome. Int J Mol Sci. (2020) 21(20):7706. doi: 10.3390/ijms21207706
109. Li Z, Kou L, Fu X, Xie Z, Xu M, Guo L, et al. Design, synthesis, and biological evaluation of triazole-pyrimidine-methylbenzonitrile derivatives as dual A(2A)/A(2B) adenosine receptor antagonists. J Enzyme Inhib Med Chem. (2022) 37:1514–26. doi: 10.1080/14756366.2022.2077731
110. Reddy GL, Sarma R, Liu S, Huang W, Lei J, Fu J, et al. Design, synthesis and biological evaluation of novel scaffold benzo[4,5]imidazo [1,2-a]pyrazin-1-amine: Towards adenosine A(2A) receptor (A(2A) AR) antagonist. Eur J Med Chem. (2021) 210:113040. doi: 10.1016/j.ejmech.2020.113040
111. Liu S, Ding W, Huang W, Zhang Z, Guo Y, Zhang Q, et al. Discovery of novel benzo[4,5]imidazo[1,2-a]pyrazin-1-amine-3-amide-one derivatives as anticancer human A(2A) adenosine receptor antagonists. J Med Chem. (2022) 65:8933–47. doi: 10.1021/acs.jmedchem.2c00101
112. Siriwon N, Kim YJ, Siegler E, Chen X, Rohrs JA, Liu Y, et al. CAR-T cells surface-engineered with drug-encapsulated nanoparticles can ameliorate intratumoral T-cell hypofunction. Cancer Immunol Res. (2018) 6:812–24. doi: 10.1158/2326-6066.CIR-17-0502
113. Shiravand Y, Khodadadi F, Kashani SMA, Hosseini-Fard SR, Hosseini S, Sadeghirad H, et al. Immune checkpoint inhibitors in cancer therapy. Curr Oncol. (2022) 29:3044–60. doi: 10.3390/curroncol29050247
114. Mamdani H, Matosevic S, Khalid AB, Durm G, Jalal SI. Immunotherapy in lung cancer: current landscape and future directions. Front Immunol. (2022) 13:823618. doi: 10.3389/fimmu.2022.823618
115. Kamai T, Kijima T, Tsuzuki T, Nukui A, Abe H, Arai K, et al. Increased expression of adenosine 2A receptors in metastatic renal cell carcinoma is associated with poorer response to anti-vascular endothelial growth factor agents and anti-PD-1/Anti-CTLA4 antibodies and shorter survival. Cancer Immunol Immunother. (2021) 70:2009–21. doi: 10.1007/s00262-020-02843-x
116. Okikawa S, Morine Y, Saito Y, Yamada S, Tokuda K, Teraoku H, et al. Inhibition of the VEGF signaling pathway attenuates tumor−associated macrophage activity in liver cancer. Oncol Rep. (2022) 47(4):71. doi: 10.3892/or
117. Prange CJ, Sayed NYB, Feng B, Goepfert C, Trujillo DO, Hu X, et al. A redox-responsive prodrug for tumor-targeted glutamine restriction. J Control Release. (2024) 368:251–64. doi: 10.1016/j.jconrel.2024.02.031
118. Fulgenzi CAM, Scheiner B, Korolewicz J, Stikas CV, Gennari A, Vincenzi B, et al. Efficacy and safety of frontline systemic therapy for advanced HCC: A network meta-analysis of landmark phase III trials. JHEP Rep. (2023) 5:100702. doi: 10.1016/j.jhepr.2023.100702
119. Chen Q, Yin H, He J, Xie Y, Wang W, Xu H, et al. Tumor microenvironment responsive CD8(+) T cells and myeloid-derived suppressor cells to trigger CD73 inhibitor AB680-based synergistic therapy for pancreatic cancer. Adv Sci (Weinh). (2023) 10:e2302498. doi: 10.1002/advs.202302498
120. Du X, Moore J, Blank BR, Eksterowicz J, Sutimantanapi D, Yuen N, et al. Orally bioavailable small-molecule CD73 inhibitor (OP-5244) reverses immunosuppression through blockade of adenosine production. J Med Chem. (2020) 63:10433–59. doi: 10.1021/acs.jmedchem.0c01086
121. Ray A, Du T, Wan X, Song Y, Pillai SC, Musa MA, et al. A novel small molecule inhibitor of CD73 triggers immune-mediated multiple myeloma cell death. Blood Cancer J. (2024) 14:58. doi: 10.1038/s41408-024-01019-5
122. Turiello R, Capone M, Morretta E, Monti MC, Madonna G, Azzaro R, et al. Exosomal CD73 from serum of patients with melanoma suppresses lymphocyte functions and is associated with therapy resistance to anti-PD-1 agents. J Immunother Cancer. (2022) 10(7):e004043corr1. doi: 10.1136/jitc-2021-004043
123. Karoon Kiani F, Izadi S, Ansari Dezfouli E, Ebrahimi F, Mohammadi M, Chalajour H, et al. Simultaneous silencing of the A2aR and PD-1 immune checkpoints by siRNA-loaded nanoparticles enhances the immunotherapeutic potential of dendritic cell vaccine in tumor experimental models. Life Sci. (2022) 288:120166. doi: 10.1016/j.lfs.2021.120166
124. Pang N, Li J, Sun A, Yang Z, Cheng S, Qi XR. Prior anti-CAFs break down the CAFs barrier and improve accumulation of docetaxel micelles in tumor. Int J Nanomedicine. (2018) 13:5971–90. doi: 10.2147/IJN
125. van der Heide CD, Dalm SU. Radionuclide imaging and therapy directed towards the tumor microenvironment: a multi-cancer approach for personalized medicine. Eur J Nucl Med Mol Imaging. (2022) 49:4616–41. doi: 10.1007/s00259-022-05870-1
126. Fabre M, Ferrer C, Dominguez-Hormaetxe S, Bockorny B, Murias L, Seifert O, et al. OMTX705, a novel FAP-targeting ADC demonstrates activity in chemotherapy and pembrolizumab-resistant solid tumor models. Clin Cancer Res. (2020) 26:3420–30. doi: 10.1158/1078-0432.CCR-19-2238
127. Hao J, Ding XL, Yang X, Wu XZ. Prunella vulgaris polysaccharide inhibits growth and migration of breast carcinoma-associated fibroblasts by suppressing expression of basic fibroblast growth factor. Chin J Integr Med. (2020) 26:270–6. doi: 10.1007/s11655-016-2587-x
128. Li D, Cao D, Sun Y, Cui Y, Zhang Y, Jiang J, et al. The roles of epigallocatechin gallate in the tumor microenvironment, metabolic reprogramming, and immunotherapy. Front Immunol. (2024) 15:1331641. doi: 10.3389/fimmu.2024.1331641
129. Salkeni MA, Naing A. Interleukin-10 in cancer immunotherapy: from bench to bedside. Trends Cancer. (2023) 9:716–25. doi: 10.1016/j.trecan.2023.05.003
130. Abou Shousha S, Osman EM, Baheeg S, Shahine Y. Anti-IL-8 monoclonal antibodies inhibits the autophagic activity and cancer stem cells maintenance within breast cancer tumor microenvironment. Breast Dis. (2024) 43:37–49. doi: 10.3233/BD-230052
131. Jorgovanovic D, Song M, Wang L, Zhang Y. Roles of IFN-gamma in tumor progression and regression: a review. biomark Res. (2020) 8:49. doi: 10.1186/s40364-020-00228-x
132. Li X, Zhang Y, Walana W, Zhao F, Li F, Luo F. GDC-0941 and CXCL8 (3-72) K11R/G31P combination therapy confers enhanced efficacy against breast cancer. Future Oncol. (2020) 16:911–21. doi: 10.2217/fon-2020-0035
133. Khan MN, Wang B, Wei J, Zhang Y, Li Q, Luan X, et al. CXCR1/2 antagonism with CXCL8/Interleukin-8 analogue CXCL8(3-72)K11R/G31P restricts lung cancer growth by inhibiting tumor cell proliferation and suppressing angiogenesis. Oncotarget. (2015) 6:21315–27. doi: 10.18632/oncotarget.v6i25
134. Liu X, Peng J, Sun W, Yang S, Deng G, Li F, et al. G31P, an antagonist against CXC chemokine receptors 1 and 2, inhibits growth of human prostate cancer cells in nude mice. Tohoku J Exp Med. (2012) 228:147–56. doi: 10.1620/tjem.228.147
135. Dominguez C, McCampbell KK, David JM, Palena C. Neutralization of IL-8 decreases tumor PMN-MDSCs and reduces mesenchymalization of claudin-low triple-negative breast cancer. JCI Insight. (2017) 2(21):e94296. doi: 10.1172/jci.insight.94296
Keywords: tumour microenvironment, adenosine receptor, stromal cell, tumour-associated fibroblasts, immunotherapy
Citation: Han Y, Dong C, Hu M, Wang X and Wang G (2024) Unlocking the adenosine receptor mechanism of the tumour immune microenvironment. Front. Immunol. 15:1434118. doi: 10.3389/fimmu.2024.1434118
Received: 17 May 2024; Accepted: 17 June 2024;
Published: 27 June 2024.
Edited by:
Junjiang Fu, Southwest Medical University, ChinaReviewed by:
Saber Imani, The Affiliated Hospital of Southwest Medical University, ChinaCopyright © 2024 Han, Dong, Hu, Wang and Wang. This is an open-access article distributed under the terms of the Creative Commons Attribution License (CC BY). The use, distribution or reproduction in other forums is permitted, provided the original author(s) and the copyright owner(s) are credited and that the original publication in this journal is cited, in accordance with accepted academic practice. No use, distribution or reproduction is permitted which does not comply with these terms.
*Correspondence: Guiling Wang, Z2xpbmd3YW5nQGNtdS5lZHUuY24=
†These authors have contributed equally to this work