- 1Department of Nephrology, First Teaching Hospital of Tianjin University of Traditional Chinese Medicine, National Clinical Research Center for Chinese Medicine Acupuncture and Moxibustion, Tianjin, China
- 2Department of Nephrology, Traditional Chinese Hospital of Xiamen, Xiamen, China
- 3Department of Nephrology, Chongqing City Hospital of Traditional Chinese Medicine, Chongqing, China
Nephrotic syndrome (NS) represents a prevalent syndrome among various chronic kidney disease pathologies and is known for its higher severity and worse prognosis compared with chronic glomerulonephritis. Understanding its pathogenesis and identifying more effective treatment modalities have long been a concern of kidney specialists. With the introduction of the gut–kidney axis concept and the progress in omics technologies, alterations in the gut microbiota have been observed in primary and secondary NS. This link has been extensively researched in conditions such as diabetic nephropathy and immunoglobulin A (IgA) nephropathy. Thus, dysbiosis of the gut microbiota is seen as a crucial contributing factor in NS; however, there is a lack of comprehensive reviews that elucidate the changes in the gut microbiota across different NS conditions and that describe its mechanistic role in the disease. Moreover, serving as an innate regulator of the gut microbiota, traditional Chinese medicine (TCM) has the potential to exert a profound impact on the expression of inflammation-promoting agents, decreasing the levels of endotoxins and uremic toxins. In addition, it strengthens the stability of the intestinal barrier while controlling the metabolic function of the body through its efficient modulation of the gut microbiota. This intricate process yields far-reaching consequences for NS.
1 Introduction
Over the past decade, the prevalence of chronic kidney disease (CKD) has exceeded 9.1%, making kidney disease a major public health burden globally (1). Nephrotic syndrome (NS) is a common manifestation across various CKD pathologies that is characterized by the presence of proteinuria, hypoalbuminemia, hyperlipidemia, and edema. Compared with chronic glomerulonephritis, NS presents with a higher severity and a poorer prognosis. NS can be classified into primary or secondary NS. Primary NS includes minimal change disease (MCD), membranous nephropathy (MN), mesangial proliferative glomerulonephritis (MsPGN), membranoproliferative glomerulonephritis, and focal segmental glomerulosclerosis (FSGS), which are diagnosed primarily through kidney biopsy. Secondary NS is often associated with conditions such as diabetic nephropathy, lupus nephritis (LN), and Henoch–Schönlein purpura nephritis (HSPN). NS is prone to relapses and complications such as infections, thrombosis, cardiovascular diseases, and acute kidney injury (2). However, the exact mechanisms underlying NS remain unclear, and current treatment options are limited. Thus, exploring the pathogenesis of NS and identifying relevant treatment approaches for this disease are crucial for alleviating the societal burden of CKD.
The deepening understanding of diseases and the advent of new technologies have prompted us to explore new perspectives on the pathological mechanisms and treatment methods for this condition. Omics technologies have been widely applied in the exploration of biomarkers and the diagnosis, prognosis, pharmacology, and toxicity assessment of various diseases (3–5), offering novel avenues and strategies for patient identification and continued monitoring. In this context, targeted modulation of the gut microbiota has been suggested as a preventive and therapeutic approach for a variety of diseases; however, its potential remains to be further developed. Since the proposition of the gut–kidney axis, research on the gut microbiota has become a major focus, with extensive studies being done in the field of renal disorders. One study has indicated that the gut microbiota in individuals suffering from kidney diseases experiences significant alterations, including NS (6), and plays a vital role in the onset and progression of diseases. To date, the gut microbiota has been confirmed as a diagnostic and therapeutic target for diabetic kidney disease (DKD) and MN, but there is a lack of comprehensive reviews on the gut microbiota in NS.
Therefore, this article aimed to review the intrinsic connections between common types of NS and the gut microbiota, attempting to identify potential therapeutic directions for clinical treatment. This study also delves into recent studies concerning how traditional Chinese medicine (TCM) can regulate the gut microbiota as a means of treating NS, aiming to provide more insights into the treatment of NS (Figure 1).
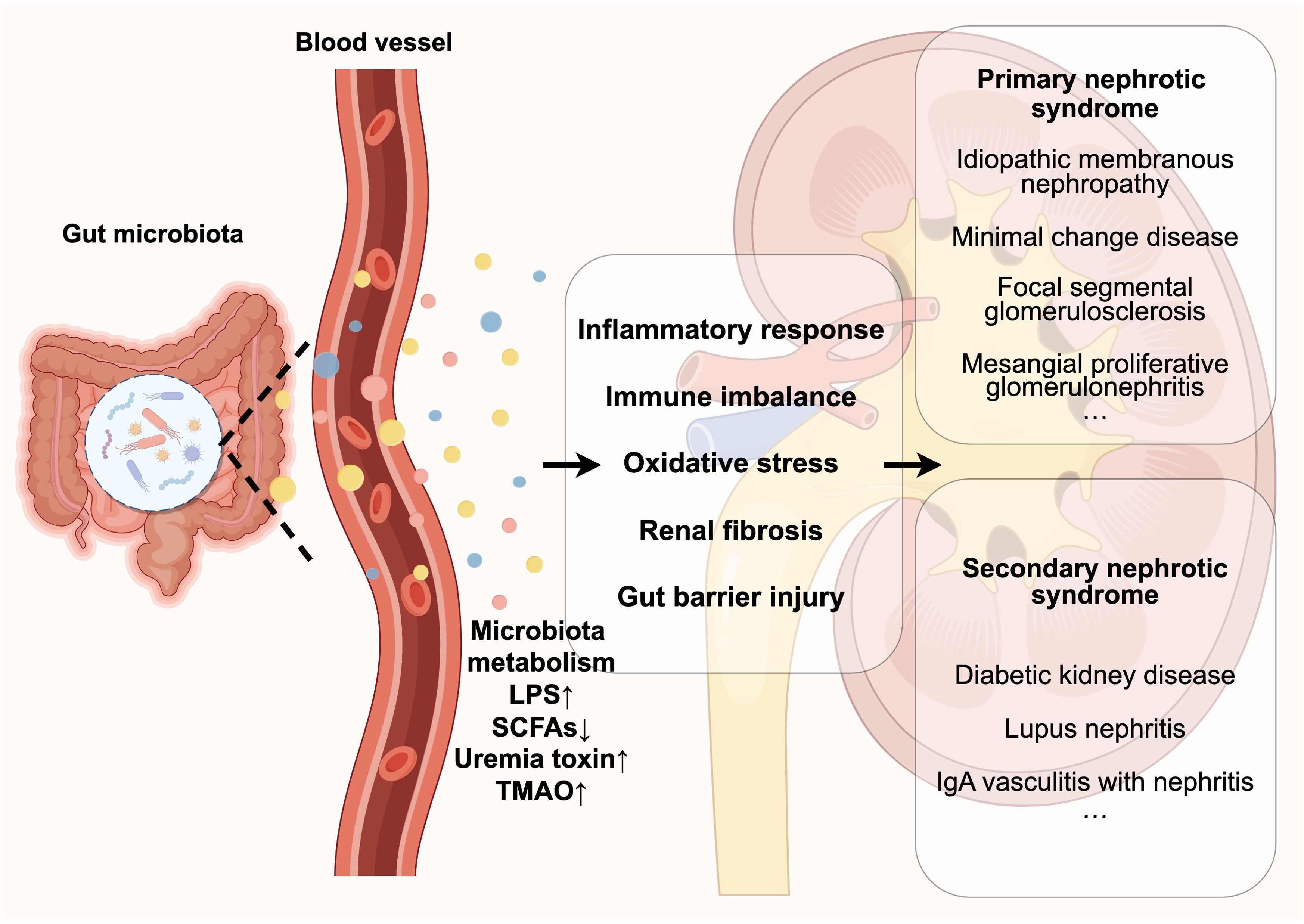
Figure 1. Disturbances in the gut microbiota contribute to the development and progression of nephrotic syndrome by affecting its metabolites (e.g., LPS, SCFAs, etc.), which include triggering a series of pathological reactions such as inflammatory response, immune imbalance, oxidative stress, damage to the gut barrier, and renal fibrosis.
2 Primary nephrotic syndrome and the gut microbiota
2.1 Idiopathic membranous nephropathy
Idiopathic membranous nephropathy (IMN) is a prevalent pathological type of NS in adults that is distinguished by sub-epithelial immune complex deposition, podocyte injury, and diffuse thickening of the glomerular basement membrane. Approximately 30%–50% of patients with IMN will eventually progress to end-stage kidney disease (ESKD) (7). The exact pathogenesis of IMN is yet to be thoroughly deciphered, and to date, it has been mostly considered an antibody-mediated autoimmune disease. The target antigens located on podocytes are recognized by autoantibodies, which then combine with them to form immune complexes and deposit under the basement membrane of podocytes to activate the complement system, thus impairing the function of the glomerular capillary walls and causing podocyte damage and detachment. Consequently, this leads to the increased permeability of the basement membrane and the emergence of large amounts of proteinuria. The immune complexes are composed of various components including immunoglobulin G (IgG) antibodies (mainly IgG4) and the C5b-9 membrane attack complex (8). The identified target antigens include the M-type phospholipase A2 receptor (PLA2R), Nel-like molecule-1, Semaphorin 3B, protocadherin-7, and thrombospondin type 1 domain-containing 7A, all of which bind to IgG subtypes (9).
The gut microbiota can act in a number of ways to impair the body’s immune system. Recent studies on MN and the gut microbiota have shown an increased trend. Studies showed that, compared with the healthy population, patients with IMN have increased levels of Shigella spp., Streptococcus spp., Enterobacter spp., and Enterococcus spp. and decreased abundance of Lachnospira, Lachnospiraceae, and Veillonella (10). Shigella spp. are Gram-negative bacilli that produce lipopolysaccharides (LPS) (11), which activate Toll-like receptor 4 (TLR4) and induce the activation of the nuclear factor kappa B (NF-κB) pathway, leading to the production of pro-inflammatory cytokines such as tumor necrosis factor alpha (TNF-α), interleukin 6 (IL-6), and IL-1 (12, 13), which damage podocytes and the glomerular basement membrane. Likewise, a high abundance of Escherichia coli increases the circulating levels of certain inflammatory factors (14). It is evident that disturbances in the gut microbiota lead to an imbalance in the expression of these cytokines, which is associated with the pathogenesis of IMN. LPS can stimulate secreted PLA2-IIA (sPLA2-IIA) expression (15). In turn, sPLA2-IIA can exert an action on the immune system by hydrolyzing bacterial membranes and modifying the structure of the gut microbiota, thereby participating in the induction of immune phenotypes and promoting inflammatory responses (16), indicating a link between the PLA2R antigen of IMN and the disturbance of the gut microbiota. In an effort to determine whether the gut microbiota is abnormal in IMN, a number of explorations have been undertaken in succession. Zhang et al. (17) found an increase in Clostridium and Aspergillus, but a decline in the abundance of the thick-walled phylum in patients with IMN compared with healthy controls. Furthermore, the gut microbiota showed high potential in the differentiation of IMN from DKD, in which patients with IMN had a relatively increased diversity of bacterial α, such as Peptostreptococcaceae_incertae sedis and Clostridium_sensu_stricto_1, among others (18), which are also capable of producing LPS and invading into the bloodstream through the compromised intestinal barrier, further reactivating the inflammatory pathway and accelerating the renal inflammatory response (19). In addition, a recent large-scale study (20) included fecal specimens from more than 800 patients. Through clinical studies, animal experiments, and fecal bacterial transplantation techniques, it was identified that those suffering from MN exhibited a reduced abundance of gut microbiota compared with those who were healthy and that it was difficult to construct a model of MN in rats that had been cleared of gut microbiota, suggesting that the naturally occurring microflora in the intestinal tract may be imperative for the pathogenesis of MN. On this basis, Ma et al. (21) and Feng et al. (22) identified several bacterial groups with a causative relationship with the occurrence of MN, among which Oscillibacter showed a protective causal relationship in reducing MN occurrence in both studies. Studies have shown that an imbalance between the T helper cell 17 (Th17) and regulatory T cell (Treg) ratio is tightly linked to the pathogenesis of IMN (23). Short-chain fatty acids (SCFAs), which consist of propionate, butyrate, and acetate, are metabolic products of the gut microbiota. SCFAs can exert anti-inflammatory effects by promoting cytokine IL-10 synthesis through binding to specific G-protein-coupled receptors (GPRs), such as GPR43 and GPR109a, in order to induce the production and differentiation of Tregs (24, 25). The study noted that patients with IMN had significantly lower contents of propionic and butyric acids in their feces than the healthy controls (17). Butyrate could enhance the acetylation of the forkhead box protein 3 (Foxp3) promoter and histone H3 in the conserved non-coding region or bind to a free fatty acid receptor to stimulate the proliferation and differentiation of initial CD4+ T cells to Tregs (26, 27). Butyrate and propionate can inhibit the expression of cytokines induced by LPS, such as IL-6 and IL-12/p40, thereby protecting the integrity of podocytes and the glomerular basement membrane (28). It has been found that Clostridia can induce intestinal epithelial cells to produce transforming growth factor beta (TGF-β), providing a favorable environment for the formation of Tregs (29).
In summary, the onset and the progression of IMN are highly connected to the gut microbiota and its metabolic metabolites. However, a point of concern is that, due to the complexity of the gut microbiota, the possible etiology of MN cannot be definitively attributed solely to the dysregulation of a singular bacterium (30). Hence, it is imperative to give due attention to the systematic study of the gut microbiota in order to steer clear of any partiality and attain holistic control.
2.2 Minimal change disease
In children and adolescents, MCD is the most frequent cause of NS type. It is characterized by lipid accumulation in the renal tubular epithelial cells and the urine, with extensive podocyte foot process effacement observable under electron microscopy. The underlying pathogenesis of MCD is complex and not fully elucidated yet. Most patients can achieve remission with aggressive treatment, but relapses and steroid dependency are common. Deficiency of Tregs assumes a fundamental position in the emergence and recurrence of MCD (31, 32). Recently, a “second hit” hypothesis has been proposed for MCD, suggesting that an imbalance between the ratio of Tregs and CD80 molecules is closely associated with MCD (33). Moreover, several studies have indicated that the slit-diaphragm molecular component nephrin is associated with the occurrence of MCD (34, 35). Animal experiments have shown that overexpressed CD80 and Neph1 interact through their extracellular domains, disrupting the slit diaphragm (36). LPS induces the loss of foot processes by activating TLR-4 to induce CD80 expression, which ultimately leads to increased glomerular filtration barrier permeability and proteinuria (37, 38).
Jiang et al. (39) analyzed the fecal samples from 45 individuals suffering from MCD. Their findings revealed a noteworthy contrast when compared with those of healthy individuals. Specifically, patients with MCD exhibited a notable increase in the abundance of Bacteroides, while the levels of Faecalibacterium and Prevotella were notably decreased. Analogously, Zhang et al. (40) revealed that untreated MCD patients had a reduced gut microbiota alpha diversity, with decreased abundance of Bacteroides, Faecalibacterium, and Prevotella, with the latter showing the most significant changes and pathogenic bacteria such as Escherichia–Shigella increasing in abundance. After treatment, the composition of the gut microbiota could be partially restored, indicating that the disordered composition of the gut microbiota might be related to the pathogenesis and severity of MCD. Faecalibacterium is one of the bacteria that produce butyrate, and it is possible that the reduction of butyrate could facilitate the entry of inflammatory factors into the bloodstream (41). In conclusion, there is an essential connection between MCD and the gut microbiota; however, the mechanisms linking changes in the gut microbiota to kidney damage are not clear and require further investigation.
2.3 Focal segmental glomerulosclerosis
FSGS is a pathological change characterized by segmental sclerosis of some glomeruli observed under light microscopy, involving progressive structural changes such as podocyte apoptosis, glomerular basement membrane damage, cellular structure changes, and mesangial matrix proliferation. The central aspect of the pathology of FSGS is podocyte injury (42). Primary FSGS can cause rapid and extensive damage to podocytes, with a sudden clinical onset and extensive podocyte lesions observed under electron microscopy, where over 80% of the capillary surface shows foot process effacement (43).
Its pathogenesis is still being explored, and it has been found that the gut microbiota may have a certain role in it. Zha et al. (44) found that the administration of adriamycin (ADR) inhibited the expression of GPR41 in the renal tissue of an ADR-induced FSGS rat model, whereas an increase in SCFAs upregulated the GPR41 receptor expression, reduced the release of TNF-α and IL-1β, and inhibited the excessive proliferation and abnormal apoptosis of glomerular cells. Shi et al. (45) constructed a rat FSGS model through unilateral nephrectomy and caudal vein injection of ADR. Fecal analysis revealed a distinct increase in the populations of Bifidobacterium, Collinsella, and Candida and a notable decrease in the abundance of Granulicatella and Christensenella. Maier et al. (46) induced an adult mouse model of FSGS with podocyte-specific gene Epb41l5 knockout using doxycycline and identified an impaired gut microbiota diversity, i.e., significant increases in Bacteroidetes and Alistipes and decreases in Pseudoflovonifractor and Anaerotignum. Bacteroidetes and Alistipes can produce harmful metabolic products such as ammonia, hydrogen sulfide, cresol, and indole (47, 48). The formation of ammonia increased the intestinal pH value, further promoting gut microbiota dysbiosis and disrupting the tight junctions of the intestinal epithelium, leading to endotoxemia (49). It has been demonstrated that indoxyl sulfate (IS) could activate the mammalian target of rapamycin complex 1 (mTORC1), inducing renal fibrosis and promoting CKD progression through a microbiota-dependent mechanism (50, 51). Injection of ADR induced mice to exhibit mesangial proliferation and segmental glomerulosclerosis, a remarkable increase in the urinary albumin/creatinine ratio, and an obvious modification of the gut microbiota, which was mainly reflected in the enrichment of Odoribacter and the reduction of Turicibacter, Marvinbryantia, and Rikenella (52). Furthermore, it dramatically enhanced the serum inflammatory factors, such as the IL-2 level, in BALB/C mice, which could be attributed to the disorganization of the gut microbiota (52). Zhi et al. (53) treated a patient with FSGS using fecal microbiota transplantation (FMT). After treatment, the kidney function of the patient remained stable and the urinary protein decreased, with no relapse after reducing glucocorticoids. It is evident that the pathogenesis of FSGS is closely associated with the gut microbiota. However, current research on FSGS and the gut microbiota has primarily focused on animal experiments, with limited studies on humans.
2.4 Mesangial proliferative glomerulonephritis
The characteristic pathology of MsPGN manifests as proliferation of glomerular mesangial cells and deposition of the glomerular extracellular matrix (54). The pathogenesis of MsPGN has not been fully elucidated, but is thought to be related to the impaired clearance of immune complexes, dysfunction in immune regulation, and excessive proliferation of mesangial cells (55).
He et al. (6) observed changes in the microbial communities in cases of MsPGN and MN. Compared with the MN group, increases were noted in the populations of Bradyrhizobium, Hyphomicrobium, Bradyrhizobiaceae, Hyphomicrobiaceae, and other taxa. Decreases were observed in Ruminococcaceae, Alistipes, Lachnospira, Tyzzerella, and chloroplast, among others. This research pointed to the underlying change in the gut microbiome that is relevant to MsPGN. Unfortunately, there are only a handful of studies exploring the gut microbiota in MsPGN, which leaves its exact involvement unclear.
3 Secondary nephrotic syndrome and the gut microbiota
3.1 Diabetic kidney disease
Among the complications associated with diabetes mellitus (DM), DKD stands out as a particularly common microvascular complication that accounts for 20.7% of cases (56, 57). This condition can involve various lesions, such as the glomeruli, tubules, and the interstitium, among others (58). In addition, DKD is a leading contributor to end-stage renal disease (ESRD), which comprises approximately 47% of the ESRD cases in the US (59). Clinical signs of DKD include edema, persistent proteinuria, hypertension, and a progressive reduction in the glomerular filtration rate. The development of DKD involves intricate mechanisms, posing a significant risk of progression to ESRD.
Currently, there is a lack of highly efficacious therapies to prevent renal damage, which underscores the necessity of delving into novel treatment modalities and targets. Emerging research increasingly indicates that the gut–kidney axis is pivotal in the progression of DKD (60). Gut microbiota disturbances can occur as a result of a high-sugar and high-fat environment, dietary habits, medication, and gastrointestinal edema. There is a considerable dysbiosis of the gut microbiota in patients with DKD, which is marked by a decline in beneficial bacteria and an increase in detrimental and pathogenic bacteria. The comparison of patients with DKD and healthy individuals revealed a discernible decrease in the diversity and abundance of gut microbiota, with greater levels of Actinobacteria at the phylum level and, at the class level, a more prevalent abundance of Actinobacteria, Bacilli, and Coriobacteriia and lower abundance of Alphaproteobacteria and Clostridia (61). In contrast to DM patients without renal impairment, those with DKD exhibited notably elevated levels of Christensenella, Clostridium-XIVa, and Eisenbergiella, with Eisenbergiella demonstrating a positive correlation with both glomerulosclerosis and thickening of the glomerular basement membrane (62).
Furthermore, it has been found that different stages of DKD exhibit different gut microbial profiles, with Agathobacter potentially serving as a biomarker for distinguishing the early and late stages of DKD (63). A meta-analysis indicated that, compared with healthy controls, patients with DKD had fewer butyrate-producing genera such as Butyricicoccus, Faecalibacterium, Lachnospira, and Roseburia and that, compared with DM, DKD had a significant enrichment of harmful bacteria such as Hungatella, Bilophila, and Escherichia (64). Among these, Hungatella could act on DKD by affecting trimethylamine-N-oxide (TMAO). Clostridium hathewayi was found to produce trimethylamine, the precursor of TMAO (65). Furthermore, C. hathewayi originates from the genus Hungatella. Elevated concentrations of TMAO have been demonstrated to instigate renal impairment by stimulating inflammation, oxidative stress, and fibrosis (66). Moreover, a number of immune-related conditions (67), including rheumatoid arthritis (68), type 2 diabetes (69), inflammatory bowel disease, and colorectal cancer (70), have been linked to Bilophila, a sulfate-reducing bacteria with pro-inflammatory qualities. Escherichia, a potential pathogen, metabolizes dietary tryptophan into precursors of the uremic toxin indole, which further transforms into IS, participating in the process of renal function damage (71). A research indicated a decline in the population of microbiota responsible for the production of butyrate in individuals with DKD, leading to the decreased levels of serum butyrate and total SCFAs (72). SCFAs possess the ability to prevent the activation of the NF-κB pathway, which in turn diminishes the expression of pro-inflammatory cytokines such as TNF-α and IL-6 (73). In addition, under hyperglycemic conditions, SCFAs can exhibit the ability to modulate inflammation in renal tubular cells and podocytes and engage with GPR43 or GPR109A to regulate immunity, consequently lowering the expression of fibrotic and inflammatory genes associated with DKD. This cascade of effects culminates in the mitigation of podocyte injury, as well as mesangial fibrosis, thereby hindering the progression of DKD (74, 75). Studies have demonstrated that the administration of butyrate effectively prevented kidney damage in diabetic rats by enhancing glomerular hypertrophy and mitigating the production of collagen IV and fibronectin (72).
In addition, oxidative stress has emerged as a significant risk factor contributing to the progression of DKD. Sodium butyrate has been revealed to stimulate nuclear factor erythroid 2-related factor 2 (Nrf2) by inhibiting histone deacetylase (HDAC) activity, thus suppressing the renal oxidative stress response and delaying kidney fibrosis (76). Imbalance in the gut microbiota and exposure to a high-glucose environment could weaken the integrity of the intestinal epithelial barrier, allowing bacteria and enterogenic endotoxins to migrate and accelerate kidney damage in diabetic mice (77). By modulating the levels of synaptopodin and claudin-1, butyrate could maintain the integrity of the intestinal barrier (78).
Studies have increasingly found that new diabetes drugs, such as englitazone (79) and saxagliptin/valsartan (80), can exert their effects partly through modulating the gut microbiota. The gut microbiota is essential for the regulation of glucose and lipid metabolism, alleviating inflammatory and host immunity, responding to inflammatory and oxidative stress, and repairing the intestinal barrier, thereby potentially ameliorating kidney function impairment and offering a fresh avenue for the treatment of DKD.
3.2 Lupus nephritis
LN is the most prevalent complication of systemic lupus erythematosus (SLE) and is one of the main causes of death from this condition, with roughly 20% of patients inevitably progressing to ESRD (81). Complement activation, inflammation, and immune complex deposition are involved in the pathophysiology of both acute and chronic renal damage in LN. Research on changes in the gut microbiota and their relationship with autoimmune illnesses has exploded in the last few years, including LN. Compared with healthy adults, patients with LN typically exhibit a reduced alpha diversity in their gut microbiota and a lower ratio of Firmicutes/Bacteroidetes; nonetheless, the salient characteristics may include enrichment of the phylum Proteobacteria, genera Streptococcus and Lactobacillus, and species Ruminococcus gnavus and Lactobacillus reuteri (82).
Specific LN microbial communities may participate in disease progression by affecting the total quantity of T cells in peripheral blood, the inflammatory responses, and the intestinal barrier stability. According to a cohort study, the serum anti-RG strain-restricted antibodies and the lupus disease activity positively correlated with the relative abundance of R. gnavus (83). The study by Zhang et al. (84) established a correlation between the percentage of R. gnavus with the absolute numbers of CD4+ T-cell subgroups and lymphocyte subgroups in peripheral blood. Notably, significant correlations emerged between the ratios of Th1/Th2 and Th17/Tregs. Another study (85) demonstrated increased intestinal permeability in LN mice. After treatment with five Lactobacillus strains, it was found that the damaged intestinal barrier in mice was repaired, with the anti-inflammatory and nephroprotective effects exerted by inhibiting the expression of IL-6, increasing the concentration of IL-10, inhibiting the kidneys from accumulating IgG2a, and by increasing the Treg/Th17 ratio.
The investigation by Valiente et al. (86) revealed that the colonization of NZM2410 mice by segmented filamentous bacteria resulted in a heightened intestinal permeability, along with the amplification of Th17 and lymphoid cells within the lamina propria of the small intestine, and resulted in a more severe case of glomerulonephritis than in mice that were not colonized. The onset of SLE is mostly driven by immunological tolerance rupture and Th17/Treg equilibrium alteration (87). Tregs can not only foster immune tolerance through suppressing autoreactive lymphocytes but can also regulate the production of antinuclear antibodies and the advancement of nephritis in animals with lupus by blocking the development of aberrant B cells (88). A study found that the percentage of Tregs in patients with LN is much lower than that in healthy controls and in non-LN SLE patients (89), and increases in the level of Tregs might be an independent protective factor for the long-term renal prognosis in patients with LN (90).
The inflammatory response is also a major aspect of the gut flora that influences LN. The elevation of the serum inflammatory cytokine IL-6 level correlates with SLE activity (91). By inducing B-cell maturation and differentiation into plasma cells, IL-6 stimulates the generation of autoantibodies. Moreover, during IL-6 stimulation, CD4 T cells might develop into pro-inflammatory Th17 subgroups, which in turn suppress the function of Tregs (92). By downregulating the expression of the pro-inflammatory cytokine IL-6 and upregulating the expression of the anti-inflammatory cytokine IL-10, the gut microbiota can mitigate renal inflammatory responses. It also increases the quantity of Tregs, which can inhibit the formation of aberrant immune complexes and limit renal damage.
3.3 Immunoglobulin A vasculitis with nephritis
Immunoglobulin A vasculitis (IgAV), commonly known as Henoch–Schönlein purpura (HSP), is a type of small vessel vasculitis that mainly affects children and is identified by IgA1 immune deposits. It clinically manifests with involvement of the kidneys, gastrointestinal tract, skin, and joints, with a small proportion affecting the lungs and central nervous system (93). When the kidneys are involved, it leads to Henoch–Schönlein purpura nephritis (HSPN), or IgAV-N, the most severe long-term complication of HSP and a major cause of death (94). Approximately 40% of children with IgAV will go on to develop nephritis (95), and adult patients tend to have more severe renal involvement and poorer prognosis compared with children. The clinical manifestations include hematuria, proteinuria, and nephritic syndrome, and patients with nephritic syndrome present with more severe renal lesions and a higher risk of progressing to ESRD (96).
Studies have suggested that the pathogenesis and characteristics of IgAV-N are extremely similar to those of IgA nephropathy (IgAN), with circulating galactose-deficient IgA1 (Gd-IgA1) immune complexes being a common major pathogenic factor for both conditions (97). IgAN is considered to be related to abnormal mucosal immune responses (98). Comparison with healthy controls has demonstrated that patients with IgAV-N and IgAN have remarkably higher levels of circulating zonulin and Gd-IgA1 (99). Zonulin can regulate the intestinal barrier permeability, and increased levels of zonulin can further enhance circulating autoantibodies and inflammation in the gastrointestinal tract and systemically (100). A study indicated that the pathophysiology of IgAV-N may potentially include gut mucosal immunity (99). IgAV is closely related to respiratory and gastrointestinal infections (101), and individuals with gastrointestinal involvement have a higher risk of developing nephritis (102, 103). The current literature demonstrated that children with IgAV have reduced gut microbiota biodiversity (104, 105) and that enrichment of Aspergillus and Actinobacteria is associated with organ involvement in IgAV (106).
In addition, according to research (107), individuals with IgAV-N had dysbiosis of the gut microbiota, which was primarily marked by an excess of potential pathogens and a decrease in beneficial bacteria. At the genus level, there was a higher relative abundance of Lactobacillus, Shigella, and Streptococcus, but a lower abundance of Prevotella_9, with Streptococcus showing a positive correlation with hypoalbuminemia and hematuria. Studies have shown that an increase in potential pathogens and a decrease in beneficial bacteria are the main features of the dysbiosis of the gut microbiota in patients with IgAV-N. At the genus level, the abundance of Bacteroides was found to be higher, while that of Prevotella was lower. Streptococcus can enter the bloodstream, trigger macrophage activation, activate mucosal immunity, induce systemic and renal inflammation, and accelerate kidney damage (108). Research found that Streptococcus could exacerbate nephritis damage by modulating the chemotaxis of Th22 cells and by stimulating the production of inflammatory cytokines (109). Escherichia–Shigella can increase the levels of LPS in the bloodstream (110), which activates peripheral B lymphocytes, triggering systemic immunity and inflammation (111); moreover, the exotoxin it produces can directly destroy glomerular endothelial cells and tubular epithelial cells (112). In the late stage of CKD, Escherichia–Shigella are highly expressed, possibly participating in renal function damage through the excessive production of IS (113). Prevotella_9 is considered a beneficial bacterium, and its reduction could lead to the decreased production of butyrate (114), which inhibits the generation of pro-inflammatory cytokines and maintenance of the intestinal barrier function (115–117). The gut microbiome and mucosal immunity are closely related. Through both T-cell-dependent and T-cell-independent pathways, dysbiosis of the gut microbiome can also impair the intestinal barrier, cause aberrant immunological activation, increase the antigen burden, and impact the maturation and differentiation of B cells in gut-associated lymphoid tissues. This induces plasma cells to produce large amounts of Gd-IgA1, which forms immune complexes with the autologous antibodies IgG/IgA, depositing under the endothelium, in small vessel walls, and in mesangial areas (98). In this context, they could stimulate the growth of mesangial cells and the extracellular matrix, leading to the migration of neutrophils and T lymphocytes and initiating the complement cascade, which would ultimately result in the release of inflammatory mediators that cause glomerular damage (118, 119).
4 Present situation and advantages of traditional Chinese medicine in the treatment of NS
NS is a clinical syndrome caused by glomerular diseases with multiple etiologies and pathological changes and is often accompanied by complications such as thrombosis and infection, which can eventually progress to ESRD. Clinical treatment mainly includes diuretics, hormones, and immunosuppressants, but the long-term use of hormones and immunosuppressants will increase the risk of infection. Some patients have steroid dependence, frequent recurrence after remission, and steroid resistance (2, 120, 121). Therefore, the rational choice of drug regimen aids in prolonging the remission time and improving the prognosis to a certain extent.
The typical symptom of NS is edema. According to the theory of Chinese medicine, NS is an edema disease. As early as thousands of years ago, “Huangdi Neijing” realized that its induction and progressive factors were due to the imbalance of the lung, spleen, and kidney, leading to abnormal water metabolism. Later generations divided the nature of edema into yin shui and yang shui.
In recent years, there have been more and more studies on the treatment of NS with TCM, and the efficacy of TCM has gradually been recognized. The combination of TCM with conventional treatment can enhance the therapeutic effect. The results of a systematic review that included seven articles involving 487 participants with NS confirmed that Huai Qi Huang granules combined with hormone therapy could reduce the recurrence rate, the incidence of infection in children with NS, and the dose of prednisone, Huai Qi Huang granules could reduce the recurrence rate and the incidence of infection, and improve immune function (122). The “Shulifenxiao” formula can be used as an alternative treatment option for steroid- and immunosuppressant-resistant NS of refractory IMN, which has good safety (123). During the Han Dynasty, Zhongjing Zhang recorded several prescriptions for the treatment of kidney diseases in the book “Shanghan Lun,” such as the Linggui Zhugan (124), Fangji Huangqi (125, 126), and Zhenwu decoctions (127), which are still in use today. The Mahuang Fuzi and Shenzhuo decoction (MFSD) is a compound prescription summarized by Professor Liu Baoli according to the idea of Zhang Zhongjing in the book “Jingui Yaolue.” It consists of six TCMs: Ma Huang (Ephedra sinica Stapf.), Hei Fu Zi (Aconitum carmichaeli Debx.), Zhi Gan Cao (Glycyrrhiza uralensis Fisch.), Gan Jiang (Zingiber officinale Rosc.), Fu Ling [Poria cocos (Schw.) Wolf], and Chao Bai Zhu (Atractylodes macrocephala Koidz.). A multicenter prospective trial included 198 patients with IMN administered MFSD. The study confirmed the effectiveness of MFSD in treating MN. After 24 months, the remission rate was 58.6% (116/198) and the remission rate was 70% (91/130) in the 130 participant subgroups followed up for 36 months (128).
5 TCM treats NS by intervening with the gut microbiota
An unbalanced gut microbiota has been reported in patients with NS, with the gut–kidney axis being important in the development and recurrence of the disease (129, 130). On one hand, this dysbiosis of the gut microbiota, typified by a disequilibrium between pathogenic and probiotic bacteria and a compromised intestinal epithelial barrier, facilitates the bloodstream entrance of uremic toxins, LPS, bacterial endotoxins, and pathogenic bacteria. Furthermore, the gut microbiota interferes with the production of metabolic substances and activates inflammatory signaling pathways and cytokines, thereby triggering immune inflammation and fibrosis processes in nephritis. Probiotic intervention has the potential to counter this imbalance in the gut microbiota (131). Increasing evidence has demonstrated that TCM exhibits unique therapeutic effects in patients with NS (132), acting through multiple levels, targets, and pathways on the pathologic process of NS (133–135). The gut microbiota, as one of the targets of TCM, influences the disease progression through the systemic action of the gut–kidney axis. Current clinical research indicates that TCM affects NS through the following key links by acting on the gut microbiota.
5.1 Suppressing the expression of pro-inflammatory cytokines
Inflammation is a crucial contributing factor to kidney damage and fibrosis in renal diseases (136), and suppression of the inflammatory responses is key to stopping the progression of NS. The control of the host’s inflammatory immune system is greatly impacted by the reorganization of the gut microbiome. By increasing the abundance of Akkermansia while simultaneously reducing the abundance of Klebsiella, Sanziguben polysaccharides (SZPs) have demonstrated efficacy in curing disorders in the gut microbiota found in DKD mice. This further alleviates renal inflammation by blocking the TLR4/NF-κB/NLRP3 pathway, effectively lowering the expression levels of TLR4, phosphorylated NF-κB p65, NLRP3, and the interleukins IL-18 and IL-1β (137). Fufang Zhenzhu Tiaozhi (FTZ) capsule, which is composed of Chinese medicinal herbs [i.e., Coptis chinensis Franch., Ligustrum Lucidum W.T. Aiton, Cirsium japonicum DC., Salvia miltiorrhiza Bunge, Panax notoginseng (Burkill) F.H. Chen, Eucommia ulmoides Oliv, Citrus medica L., and Atractylodes macrocephala Koidz.], is a patented Chinese medicine compound preparation developed by Professor Guo Jiao according to the theory of “Tiaogan Qishu Huazhuo.” It has anti-inflammatory effects, improving the glucose and lipid metabolism, intestinal metabolites, and the gut microbiota (138). It is mainly used in the clinical treatment of DKD (139) and non-alcoholic fatty liver (140). FTZ polysaccharides (FTZPs) comprise the main active ingredient. Lan et al. (141) found that FTZPs could ameliorate the gut microbiota in DKD mice and diminish the aberrant proliferation of Weissella, Enterococcus, and Akkermansia, which exhibit a positive link to markers of kidney impairment. In DKD mice, continuous administration of FTZPs could also dramatically lower the expression of the pro-inflammatory cytokines chemokine (C–C motif) ligand 2, IL-6, and intercellular adhesion molecule-1 (ICAM-1). Moreover, transcriptomic analysis showed a reduction in the expression of the kidney genes linked to inflammation. Resveratrol has the characteristics of anti-oxidative stress, anti-inflammation, and anti-apoptosis (142). Similarly, treatment with resveratrol increased the number of taxa in the fecal microbiome of db/db mice, including Bacteroides, Alistipes, and Alloprevotella. Furthermore, it led to a decrease in LPS and inflammatory cytokines, such as TNF-α and IFN-γ, in the serum and intestines of mice. In an effort to corroborate the mechanism of the gut microbiota, Cai et al. (143) found that transplantation of the fecal microbiome community modified by resveratrol brought about a similar alteration in the gut microbiota to that observed in db/db mice following resveratrol intervention. In addition, it was also observed that the mRNA levels of TNF-α, IL-6, IFN-γ, and IL-1β were diminished in the kidneys. Taken together, the gut microbiota could provide a corresponding therapeutic effect by mediating the elimination of inflammation.
5.2 Regulating the stability of the intestinal barrier
Intestinal barrier disruption has been observed to accelerate the progression of kidney disease (144). Nonetheless, stability of the intestinal barrier can successfully reduce inflammation and kidney damage. Lan et al. (141) found that DKD mice exhibited damage to the colonic mucosal barrier and that FTZPs can repair the integrity of the colonic mucosal barrier in DKD mice, showing a dose-dependent increase in the expression of the intestinal epithelial tight junction proteins, e.g., cldn-4, zo-1, E-cadherin, and occludin. The colonic mucosal barrier was dramatically repaired upon a shift in the gut microbiota from FTZP-intervened animals to DKD mice, indicating the crucial role that gut microbiota modulation plays in the control of the intestinal barrier. In addition, analogous effects were observed in another study. Sanziguben Tang, which is composed of four herbs [i.e., Rosa laevigate Michx, Gynostemma pentaphyllum (Thunb.) Makino, Phyllanthus emblica L., and Schisandra chinensis (Turcz.) Baill.], is an empirical prescription for the clinical treatment of DKD. It can reduce the expression of inflammatory factors by inhibiting the activation of NF-κB, thereby protecting kidney tissue (145).
5.3 Reducing the levels of uremic toxins and endotoxins
Excess LPS produced by Gram-negative bacteria can lead to increased intestinal permeability and further damage to the intestinal barrier. Its excess can also translocate into the circulation (146), where it in turn activates the NLRP3 inflammatory vesicles to trigger persistent inflammation (147). SZP can decrease the quantity of Gram-negative bacteria, causing an approximately 1.93-fold reduction in the LPS levels in DKD mice, further reducing renal inflammation and improving kidney function (137). IS, a uremic toxin generated by the gut microbiota fermenting proteins, translocates into the nucleus after binding to the aryl hydrocarbon receptor (AhR), which contributes to kidney inflammation and fibrosis (148). The Tangshen formula (TSF) contains seven TCMs: astragalus [Astragalus membranaceus (Fisch.) Bge.], burning bush [Euonymus alatus (Thunb.) Sieb.], rehmannia (Rehmannia glutinosa Libosch), bitter orange (Citrus aurantium L.), cornus (Cornus officinalis Sieb. Et Zuce), rhubarb (Rheum palmatum L.), and notoginseng [Panax notoginseng (Burk.) F.H. Chen]. It has the effect of replenishing qi and nourishing yin, which promotes blood circulation and dredges collaterals, and is an empirical prescription for the clinical treatment of DKD. A prospective, multicenter, double-blind, randomized controlled trial showed that TSF could reduce the 24-h urinary protein level and increase the glomerular filtration rate in patients with DKD (149). Modern pharmacological research has shown that TSF can regulate the gut microbiota in DKD rats, particularly decreasing hazardous bacteria, and can also reverse the serum IS and LPS levels, thereby ameliorating kidney lesions (150).
5.4 Improving renal fibrosis
FTZPs could significantly reduce the area of fibronectin-positive regions and collagen deposition in the renal interstitium of DKD mice and downregulate the levels of the fibrosis-related pathway proteins such as Fbn2 and Itgb3. Studies involving FMT modified by FTZPs have demonstrated decreases in the glomerular mesangial matrix expansion and fibrosis in mice, indicating a strong connection between the function of the gut microbiota and the anti-fibrotic mechanism of FTZPs (141).
5.5 Regulating metabolic activities
The gut microbiota is involved in the body’s metabolic activities and in modulating illnesses by means of metabolites. For instance, SCFAs can suppress inflammatory responses and oxidative stress and enhance immune tolerance and are essential for the progression of CKD (151). FTZPs could increase the SCFA contents in the cecum of DKD mice and enhance the level of the SCFA transporter Slc22a19, thereby reducing kidney inflammation and damage (143). Moutan Cortex (MC) has the effect of clearing heat and cooling the blood, promoting blood circulation and removing blood stasis, while Moutan Cortex polysaccharide (MC-Pa) is one of the main active ingredients from MC. It can have antioxidant activity, can downregulate the expression of inflammatory factors, and can delay the progression of DKD (152). High doses of MC-Pa could increase the acetate, propionate, and butyrate levels by dynamically modulating the rat gut microbiota, which can help enhance the intestinal barrier function and decrease the pro-inflammatory mediators (153). Bile acids (BAs) transformed by the gut microbiota in the intestines can accumulate in the circulation, causing oxidative stress and inflammatory responses, which are major pathogenic factors of kidney damage and are associated with CKD (154). As a TCM prescription, QiDiTangShen (QDTS) granules have been used in the clinical treatment of DKD for several years. It is based on Zhang Jingyue’s theory of “Zhenyin Jingqi” and combined with clinical experience. It is composed of seven herbs: R. glutinosa, Astragalus propinquus, Euryale ferox, C. officinalis, Whitmania pigra, Rheum officinale, and Hedyotis diffusa. QDTS significantly reduced proteinuria in db/db mice, inhibited autophagy activity, and improved kidney pathological changes (155). A study found that QDTS could regulate Lactobacillus, Bacteroides, and Roseburia related to BA metabolism, reducing the serum BA levels in db/db mice to protect kidney function (156). Magnesium lithospermate B (MLB) could regulate the disordered gut microbiome and inhibit the increase in the total BA levels in mice, affecting BA deconjugation to prevent kidney damage (157). Furthermore, TCM believes that corn silk is a diuretic with diuretic and detumescent effects. It can be used to treat edema, proteinuria, urinary tract infection, and diabetes, and modern pharmacological research believes that it has antioxidant effects and is beneficial for the prevention and treatment of DKD (158). Corn silk polysaccharides (CSPs) have been observed to improve metabolic disorders to limit kidney damage in DKD rats by modifying the structure of the gut microbiota, specifically by enhancing the structure of endogenous metabolites (159).
The pathways linked to CSPs include glycerophospholipid, BA, tyrosine, tryptophan, fatty acid, and phenylalanine metabolism, which show strong correlations with the gut microbiota, such as Firmicutes, Bacteroidota, Lachnospiraceae_NK4A136_group, and Dubosiella. In recent years, the application of TCM in the solid-state fermentation of edible fungi has attracted more and more attention. Astragalus and its preparations have been shown to have a positive effect on the treatment of kidney disease (160). Microbial fermentation of TCM can improve its efficacy through microbial biotransformation. Natural Cordyceps cicadae is widely used in TCM to treat CKD, and Paecilomyces cicadae, as the anamorph stage of C. cicadae, can be used as its substitute. Modern studies have discovered that Radix astragali and P. cicadae-fermented (RPF) can improve the renal structure of DKD and reduce podocyte apoptosis, which is better than A. membranaceus (161). Qing Zhou’s research found that RPF could effectively lower the critical disease parameters, including urine protein, serum creatinine, and blood urea nitrogen, in DKD mice by reversing gut microbiota dysbiosis, in which the eight species of bacteria reversed by RPF correlated significantly with the biochemical indicators and related metabolic parameters in DKD (162). Huangkui capsule is a Chinese patent medicine made from the ethanol extract of the flowers of Abelmoschus manihot. Its pharmacologically bioactive ingredients include rutin, hyperoside, hibifolin, isoquercetin, myricetin, quercetin, and quercetin-3-O-robinobioside, which can be used for the treatment of DKD, CKD, and IgA nephropathy and have the effects of improving renal function and reducing proteinuria (163). Shi et al. (164) found that the alterations in the gut microbiota of DKD mice were related to the changes in the plasma metabolites and that intervention with the Huangkui capsule brought about the restoration of the intestinal microbiota and the amelioration of the metabolite levels in DKD mice, ultimately exerting the effect of delaying the further deterioration of DKD.
6 Discussion
NS can bring about abnormalities in the gut microbiota, which in turn impact the onset and progression of NS. Analysis of the pathogenesis of NS from the perspective of the gut microbiota can offer fresh avenues for clinical treatment and drug development. This holds immense significance for the prevention and cure of NS. Intervention of the gut microbiota can be complementary to NS therapeutic approaches and posits considerable value for clinical research. Current therapies that target the gut microbiome, such as probiotic therapy and FMT, represent encouraging prospects for NS treatment.
TCM has certain advantages in regulating the gut microbiota to treat NS, which is capable of modulating the internal environment to reduce proteinuria and protect kidney function. However, there are many issues and limitations in the process of improving the gut microbiota with TCM. To begin with, numerous clinical trials suffer from poor design, and there is a lack of large-scale and high-quality studies to clarify what specific flora are acting as therapeutic NS agents in TCM. Furthermore, due to the complexity and holistic nature of the gut microbiota, it is difficult to explain the potential mechanisms of treatment through a single flora. This also hinders the discovery of potential signaling pathways and therapeutic targets. All in all, current research on the treatment perspective of the gut microbiota is still at a very early stage of development, with various research aspects yet to mature.
The method of treating NS by improving the gut microbiota with TCM is challenging, but is fully promising. Future research into TCM that aims to regulate the gut microbiota to treat NS should continue to conduct multicenter, large-scale clinical trials in order to discover constructive solutions in practice.
Author contributions
JL: Conceptualization, Writing – original draft, Writing – review & editing. YX: Writing – original draft. TS: Investigation, Writing – review & editing. XZ: Investigation, Visualization, Writing – review & editing. HL: Investigation, Writing – original draft. WL: Investigation, Writing – review & editing. HXY: Investigation, Supervision, Writing – review & editing. BY: Supervision, Validation, Writing – review & editing. HTY: Funding acquisition, Supervision, Writing – review & editing.
Funding
The author(s) declare financial support was received for the research, authorship, and/or publication of this article. This work was supported by grants from National Natural Science Foundation of China (NO.82274441), National Key Research and development Program (2023YFC3503502).
Acknowledgments
We wish to sincerely thank the tool of Figdraw (www.figdraw.com) for creating the Figure 1.
Conflict of interest
The authors declare that the research was conducted in the absence of any commercial or financial relationships that could be construed as a potential conflict of interest.
Publisher’s note
All claims expressed in this article are solely those of the authors and do not necessarily represent those of their affiliated organizations, or those of the publisher, the editors and the reviewers. Any product that may be evaluated in this article, or claim that may be made by its manufacturer, is not guaranteed or endorsed by the publisher.
References
1. Bikbov B, Purcell CA, Levey AS, Smith M, Abdoli A, Abebe M. Global, regional, and national burden of chronic kidney disease 1990-2017: a systematic analysis for the Global Burden of Disease Study 2017. Lancet. (2020) 395:709–33. doi: 10.1016/S0140-6736(20)30045-3
2. Frățilă VG, Lupușoru G, Sorohan BM, Obrișcă B, Mocanu V, Lupușoru M. Nephrotic syndrome: from pathophysiology to novel therapeutic approaches. Biomedicines. (2024) 12. doi: 10.3390/biomedicines12030569
3. Cisek K, Krochmal M, Klein J, Mischak H. The application of multi-omics and systems biology to identify therapeutic targets in chronic kidney disease. Nephrol Dial Transpl. (2016) 31:2003–11. doi: 10.1093/ndt/gfv364
4. Yang X. Multitissue multiomics systems biology to dissect complex diseases. Trends Mol Med. (2020) 26:718–28. doi: 10.1016/j.molmed.2020.04.006
5. Joshi A, Rienks M, Theofilatos K, Mayr M. Systems biology in cardiovascular disease: a multiomics approach. Nat Rev Cardiol. (2021) 18:313–30. doi: 10.1038/s41569-020-00477-1
6. He H, Lin M, You L, Chen T, Liang Z, Li D. Gut microbiota profile in adult patients with idiopathic nephrotic syndrome. BioMed Res Int. (2021) 2021:8854969. doi: 10.1155/2021/8854969
7. Beck L, Bomback AS, Choi MJ, Holzman LB, Langford C, Mariani LH. KDOQI US commentary on the 2012 KDIGO clinical practice guideline for glomerulonephritis. Am J Kidney Dis. (2013) 62:403–41. doi: 10.1053/j.ajkd.2013.06.002
8. Ronco P, Debiec H. Pathogenesis of membranous nephropathy: recent advances and future challenges. Nat Rev Nephrol. (2012) 8:203–13. doi: 10.1038/nrneph.2012.35
9. Sethi S. New 'Antigens' in membranous nephropathy. J Am Soc Nephrol. (2021) 32:268–78. doi: 10.1681/ASN.2020071082
10. Dong R, Bai M, Zhao J, Wang D, Ning X, Sun S. A comparative study of the gut microbiota associated with immunoglobulin a nephropathy and membranous nephropathy. Front Cell Infect Microbiol. (2020) 10:557368. doi: 10.3389/fcimb.2020.557368
11. Wexler HM. Bacteroides: the good, the bad, and the nitty-gritty. Clin Microbiol Rev. (2007) 20:593–621. doi: 10.1128/CMR.00008-07
12. Bryant CE, Spring DR, Gangloff M, Gay NJ. The molecular basis of the host response to lipopolysaccharide. Nat Rev Microbiol. (2010) 8:8–14. doi: 10.1038/nrmicro2266
13. Zusso M, Lunardi V, Franceschini D, Pagetta A, Lo R, Stifani S. Ciprofloxacin and levofloxacin attenuate microglia inflammatory response via TLR4/NF-kB pathway. J Neuroinflammation. (2019) 16:148. doi: 10.1186/s12974-019-1538-9
14. Chen SY, Chen CH, Huang YC, Chuang HM, Lo MM, Tsai FJ. Effect of IL-6 C-572G polymorphism on idiopathic membranous nephropathy risk in a Han Chinese population. Ren Fail. (2010) 32:1172–6. doi: 10.3109/0886022X.2010.516857
15. Pruzanski W, Wilmore DW, Suffredini A, Martich GD, Hoffman AG, Browning JL. Hyperphospholipasemia A2 in human volunteers challenged with intravenous endotoxin. Inflammation. (1992) 16:561–70. doi: 10.1007/BF00918980
16. Doré E, Joly-Beauparlant C, Morozumi S, Mathieu A, Lévesque T, Allaeys I. The interaction of secreted phospholipase A2-IIA with the microbiota alters its lipidome and promotes inflammation. JCI Insight. (2022) 7. doi: 10.1172/jci.insight.152638
17. Zhang J, Luo D, Lin Z, Zhou W, Rao J, Li Y. Dysbiosis of gut microbiota in adult idiopathic membranous nephropathy with nephrotic syndrome. Microb Pathog. (2020) 147:104359. doi: 10.1016/j.micpath.2020.104359
18. Yu W, Shang J, Guo R, Zhang F, Zhang W, Zhang Y. The gut microbiome in differential diagnosis of diabetic kidney disease and membranous nephropathy. Ren Fail. (2020) 42:1100–10. doi: 10.1080/0886022X.2020.1837869
19. Ramezani A, Raj DS. The gut microbiome, kidney disease, and targeted interventions. J Am Soc Nephrol. (2014) 25:657–70. doi: 10.1681/ASN.2013080905
20. Shang J, Zhang Y, Guo R, Liu W, Zhang J, Yan G. Gut microbiome analysis can be used as a noninvasive diagnostic tool and plays an essential role in the onset of membranous nephropathy. Adv Sci. (2022) 9:e2201581. doi: 10.1002/advs.202201581
21. Ma Q, Wen X, Xu G. The causal association of specific gut microbiota on the risk of membranous nephropathy: a Mendelian randomization study. Int Urol Nephrol. (2024) 56:2021–30. doi: 10.1007/s11255-023-03926-1
22. Feng Z, Zhang Y, Lai Y, Jia C, Wu F, Chen D. Causal relationship between gut microbiota and kidney diseases: a two-sample Mendelian randomization study. Front Immunol. (2023) 14:1277554. doi: 10.3389/fimmu.2023.1277554
23. Motavalli R, Etemadi J, Soltani-Zangbar MS, Ardalan MR, Kahroba H, Roshangar L. Altered Th17/Treg ratio as a possible mechanism in pathogenesis of idiopathic membranous nephropathy. Cytokine. (2021) 141:155452. doi: 10.1016/j.cyto.2021.155452
24. Smith PM, Howitt MR, Panikov N, Michaud M, Gallini CA, Bohlooly-Y M. The microbial metabolites, short-chain fatty acids, regulate colonic Treg cell homeostasis. Science. (2013) 341:569–73. doi: 10.1126/science.1241165
25. De Rosa V, Procaccini C, Calì G, Pirozzi G, Fontana S, Zappacosta S. A key role of leptin in the control of regulatory T cell proliferation. Immunity. (2007) 26:241–55. doi: 10.1016/j.immuni.2007.01.011
26. Takahashi D, Hoshina N, Kabumoto Y, Maeda Y, Suzuki A, Tanabe H. Microbiota-derived butyrate limits the autoimmune response by promoting the differentiation of follicular regulatory T cells. EBioMedicine. (2020) 58:102913. doi: 10.1016/j.ebiom.2020.102913
27. Furusawa Y, Obata Y, Fukuda S, Endo TA, Nakato G, Takahashi D. Commensal microbe-derived butyrate induces the differentiation of colonic regulatory T cells. Nature. (2013) 504:446–50. doi: 10.1038/nature12721
28. Nastasi C, Candela M, Bonefeld CM, Geisler C, Hansen M, Krejsgaard T. The effect of short-chain fatty acids on human monocyte-derived dendritic cells. Sci Rep. (2015) 5:16148. doi: 10.1038/srep16148
29. Sano T, Huang W, Hall JA, Yang Y, Chen A, Gavzy SJ. An IL-23R/IL-22 circuit regulates epithelial serum amyloid A to promote local effector Th17 responses. Cell. (2015) 163:381–93. doi: 10.1016/j.cell.2015.08.061
30. Zhang Y, Zhao J, Qin Y, Wang Y, Yu Z, Ning X. Specific alterations of gut microbiota in patients with membranous nephropathy: A systematic review and meta-analysis. Front Physiol. (2022) 13:909491. doi: 10.3389/fphys.2022.909491
31. Araya C, Diaz L, Wasserfall C, Atkinson M, Mu W, Johnson R. T regulatory cell function in idiopathic minimal lesion nephrotic syndrome. Pediatr Nephrol. (2009) 24:1691–8. doi: 10.1007/s00467-009-1214-x
32. Boumediene A, Vachin P, Sendeyo K, Oniszczuk J, Zhang SY, Henique C. NEPHRUTIX: A randomized, double-blind, placebo vs Rituximab-controlled trial assessing T-cell subset changes in Minimal Change Nephrotic Syndrome. J Autoimmun. (2018) 88:91–102. doi: 10.1016/j.jaut.2017.10.006
33. Shimada M, Araya C, Rivard C, Ishimoto T, Johnson RJ, Garin EH. Minimal change disease: a "two-hit" podocyte immune disorder? Pediatr Nephrol. (2011) 26:645–9. doi: 10.1007/s00467-010-1676-x
34. van de Lest NA, Zandbergen M, Ijpelaar D, Wolterbeek R, Bruijn JA, Bajema IM. Nephrin loss can be used to predict remission and long-term renal outcome in patients with minimal change disease. Kidney Int Rep. (2018) 3:168–77. doi: 10.1016/j.ekir.2017.09.011
35. Wernerson A, Dunér F, Pettersson E, Widholm SM, Berg U, Ruotsalainen V. Altered ultrastructural distribution of nephrin in minimal change nephrotic syndrome. Nephrol Dial Transpl. (2003) 18:70–6. doi: 10.1093/ndt/18.1.70
36. Khullar B, Balyan R, Oswal N, Jain N, Sharma A, Abdin MZ. Interaction of CD80 with Neph1: a potential mechanism of podocyte injury. Clin Exp Nephrol. (2018) 22:508–16. doi: 10.1007/s10157-017-1489-3
37. Reiser J, von Gersdorff G, Loos M, Oh J, Asanuma K, Giardino L. Induction of B7-1 in podocytes is associated with nephrotic syndrome. J Clin Invest. (2004) 113:1390–7. doi: 10.1172/JCI20402
38. Reiser J, Mundel P. Danger signaling by glomerular podocytes defines a novel function of inducible B7-1 in the pathogenesis of nephrotic syndrome. J Am Soc Nephrol. (2004) 15:2246–8. doi: 10.1097/01.ASN.0000136312.46464.33
39. Jiang Y, Wang T, Yu W, Wu F, Guo R, Li H. Combination of the gut microbiota and clinical indicators as a potential index for differentiating idiopathic membranous nephropathy and minimal change disease. Ren Fail. (2023) 45:2209392. doi: 10.1080/0886022X.2023.2209392
40. Zhang Y, Zhou Y, Cui W, Wang Z, Wang X, Wu F. Characterization and diagnostic value of the gut microbial composition in patients with minimal change disease. Front Physiol. (2022) 13:1070569. doi: 10.3389/fphys.2022.1070569
41. Zhou L, Zhang M, Wang Y, Dorfman RG, Liu H, Yu T. Faecalibacterium prausnitzii produces butyrate to maintain Th17/Treg balance and to ameliorate colorectal colitis by inhibiting histone deacetylase 1. Inflamm Bowel Dis. (2018) 24:1926–40. doi: 10.1093/ibd/izy182
42. Campbell KN, Tumlin JA. Protecting podocytes: A key target for therapy of focal segmental glomerulosclerosis. Am J Nephrol. (2018) 47 Suppl 1:14–29. doi: 10.1159/000481634
43. Shabaka A, Tato RA, Fernández-Juárez G. Focal segmental glomerulosclerosis: state-of-the-art and clinical perspective. Nephron. (2020) 144:413–27. doi: 10.1159/000508099
44. Zha Z, Wang X, Wang G, Yin H, Wang H. Synthesis and structural characterization of xylan acetate ester and its antinephritic effects in rats with experimental chronic kidney disease. Int J Biol Macromol. (2023) 240:124413. doi: 10.1016/j.ijbiomac.2023.124413
45. Shi X, Zhang Y, Shi Y, Zhang Q, Duan H, Liu J. Analysis of the alleviating effect of modified Huangqi Chifeng decoction on rats with focal segmental glomerulosclerosis based on gut microbiota and fecal metabolomics. J Appl Microbiol. (2023) 134. doi: 10.1093/jambio/lxad205
46. Maier JI, Rogg M, Helmstädter M, Sammarco A, Walz G, Werner M. A novel model for nephrotic syndrome reveals associated dysbiosis of the gut microbiome and extramedullary hematopoiesis. Cells. (2021) 10. doi: 10.3390/cells10061509
47. Kaur H, Das C, Mande SS. In silico analysis of putrefaction pathways in bacteria and its implication in colorectal cancer. Front Microbiol. (2017) 8:2166. doi: 10.3389/fmicb.2017.02166
48. Parker BJ, Wearsch PA, Veloo A, Rodriguez-Palacios A. The genus alistipes: gut bacteria with emerging implications to inflammation, cancer, and mental health. Front Immunol. (2020) 11:906. doi: 10.3389/fimmu.2020.00906
49. Kanbay M, Onal EM, Afsar B, Dagel T, Yerlikaya A, Covic A. The crosstalk of gut microbiota and chronic kidney disease: role of inflammation, proteinuria, hypertension, and diabetes mellitus. Int Urol Nephrol. (2018) 50:1453–66. doi: 10.1007/s11255-018-1873-2
50. Nakano T, Watanabe H, Imafuku T, Tokumaru K, Fujita I, Arimura N. Indoxyl Sulfate Contributes to mTORC1-Induced Renal Fibrosis via The OAT/NADPH Oxidase/ROS Pathway. Toxins. (2021) 13. doi: 10.3390/toxins13120909
51. Lobel L, Cao YG, Fenn K, Glickman JN, Garrett WS. Diet posttranslationally modifies the mouse gut microbial proteome to modulate renal function. Science. (2020) 369:1518–24. doi: 10.1126/science.abb3763
52. Jiang Q, He X, Zou Y, Ding Y, Li H, Chen H. Altered gut microbiome promotes proteinuria in mice induced by Adriamycin. AMB Express. (2018) 8:31. doi: 10.1186/s13568-018-0558-7
53. Zhi W, Yuan X, Song W, Jin G, Li Y. Fecal microbiota transplantation may represent a good approach for patients with focal segmental glomerulosclerosis: A brief report. J Clin Med. (2022) 11. doi: 10.3390/jcm11226700
54. Floege J, Amann K. Primary glomerulonephritides. Lancet. (2016) 387:2036–48. doi: 10.1016/S0140-6736(16)00272-5
55. Liu B, Lin J, Bai L, Zhou Y, Lu R, Zhang P. Paeoniflorin inhibits mesangial cell proliferation and inflammatory response in rats with mesangial proliferative glomerulonephritis through PI3K/AKT/GSK-3β Pathway. Front Pharmacol. (2019) 10:978. doi: 10.3389/fphar.2019.00978
56. Hung PH, Hsu YC, Chen TH, Lin CL. Recent advances in diabetic kidney diseases: from kidney injury to kidney fibrosis. Int J Mol Sci. (2021) 22. doi: 10.3390/ijms222111857
57. Sabanayagam C, Chee ML, Banu R, Cheng CY, Lim SC, Tai ES. Association of diabetic retinopathy and diabetic kidney disease with all-cause and cardiovascular mortality in a multiethnic Asian population. JAMA Netw Open. (2019) 2:e191540. doi: 10.1001/jamanetworkopen.2019.1540
58. Chandragiri S, Raju SB, Mandarapu SB, Goli R, Nimmagadda S, Uppin M. A clinicopathological study of 267 patients with diabetic kidney disease based on the renal pathology society - 2010 classification system. Indian J Nephrol. (2020) 30:104–9. doi: 10.4103/ijn.IJN_424_17
59. Burrows NR, Koyama A, Pavkov ME. Reported cases of end-stage kidney disease - United States 2000-2019. MMWR Morb Mortal Wkly Rep. (2022) 71:412–5. doi: 10.15585/mmwr.mm7111a3
60. Ni Y, Zheng L, Nan S, Ke L, Fu Z, Jin J. Enterorenal crosstalks in diabetic nephropathy and novel therapeutics targeting the gut microbiota. Acta Biochim Biophys Sin. (2022) 54:1406–20. doi: 10.3724/abbs.2022140
61. Du X, Liu J, Xue Y, Kong X, Lv C, Li Z. Alteration of gut microbial profile in patients with diabetic nephropathy. Endocrine. (2021) 73:71–84. doi: 10.1007/s12020-021-02721-1
62. Lu X, Ma J, Li R. Alterations of gut microbiota in biopsy-proven diabetic nephropathy and a long history of diabetes without kidney damage. Sci Rep. (2023) 13:12150. doi: 10.1038/s41598-023-39444-4
63. Zhang L, Lu QY, Wu H, Cheng YL, Kang J, Xu ZG. The intestinal microbiota composition in early and late stages of diabetic kidney disease. Microbiol Spectr. (2023) 11:e0038223. doi: 10.1128/spectrum.00382-23
64. Han S, Chen M, Cheng P, Zhang Z, Lu Y, Xu Y. A systematic review and meta-analysis of gut microbiota in diabetic kidney disease: Comparisons with diabetes mellitus, non-diabetic kidney disease, and healthy individuals. Front Endocrinol. (2022) 13:1018093. doi: 10.3389/fendo.2022.1018093
65. Genoni A, Christophersen CT, Lo J, Coghlan M, Boyce MC, Bird AR. Long-term Paleolithic diet is associated with lower resistant starch intake, different gut microbiota composition and increased serum TMAO concentrations. Eur J Nutr. (2020) 59:1845–58. doi: 10.1007/s00394-019-02036-y
66. Zixin Y, Lulu C, Xiangchang Z, Qing F, Binjie Z, Chunyang L. TMAO as a potential biomarker and therapeutic target for chronic kidney disease: A review. Front Pharmacol. (2022) 13:929262. doi: 10.3389/fphar.2022.929262
67. Wang T, Sternes PR, Guo XK, Zhao H, Xu C, Xu H. Autoimmune diseases exhibit shared alterations in the gut microbiota. Rheumatology. (2023) 63:856–65. doi: 10.1093/rheumatology/kead364
68. Yun H, Wang X, Wei C, Liu Q, Li X, Li N. Alterations of the intestinal microbiome and metabolome in women with rheumatoid arthritis. Clin Exper Med. (2023) 23:4695–706. doi: 10.1007/s10238-023-01161-7
69. Guan R, Ma N, Liu G, Wu Q, Su S, Wang J. Ethanol extract of propolis regulates type 2 diabetes in mice via metabolism and gut microbiota. J Ethnopharmacol. (2023) 310:116385. doi: 10.1016/j.jep.2023.116385
70. Khan S, Aziz S, Waqas M, Kakar MA, Ahmad S. Targeted vaccine development against Bilophila wadsworthia to curb colon diseases: A multiepitope approach based on reverse vaccinology and computational analysis. Int J Biol Macromol. (2023) 250:126002. doi: 10.1016/j.ijbiomac.2023.126002
71. Takayama K, Maehara S, Tabuchi N, Okamura N. Anthraquinone-containing compound in rhubarb prevents indole production via functional changes in gut microbiota. J Nat Med. (2021) 75:116–28. doi: 10.1007/s11418-020-01459-w
72. Cai K, Ma Y, Cai F, Huang X, Xiao L, Zhong C. Changes of gut microbiota in diabetic nephropathy and its effect on the progression of kidney injury. Endocrine. (2022) 76:294–303. doi: 10.1007/s12020-022-03002-1
73. Tao P, Ji J, Wang Q, Cui M, Cao M, Xu Y. The role and mechanism of gut microbiota-derived short-chain fatty in the prevention and treatment of diabetic kidney disease. Front Immunol. (2022) 13:1080456. doi: 10.3389/fimmu.2022.1080456
74. Li YJ, Chen X, Kwan TK, Loh YW, Singer J, Liu Y. Dietary Fiber Protects against Diabetic Nephropathy through Short-Chain Fatty Acid-Mediated Activation of G Protein-Coupled Receptors GPR43 and GPR109A. J Am Soc Nephrol. (2020) 31:1267–81. doi: 10.1681/asn.2019101029
75. Li YJ, Ma J, Loh YW, Chadban SJ, Wu H. Short-chain fatty acids directly exert anti-inflammatory responses in podocytes and tubular epithelial cells exposed to high glucose. Front Cell Dev Biol. (2023) 11:1182570. doi: 10.3389/fcell.2023.1182570
76. Dong W, Jia Y, Liu X, Zhang H, Li T, Huang W. Sodium butyrate activates NRF2 to ameliorate diabetic nephropathy possibly via inhibition of HDAC. J Endocrinol. (2017) 232:71–83. doi: 10.1530/joe-16-0322
77. Linh HT, Iwata Y, Senda Y, Sakai-Takemori Y, Nakade Y, Oshima M. Intestinal bacterial translocation contributes to diabetic kidney disease. J Am Soc Nephrol. (2022) 33:1105–19. doi: 10.1681/asn.2021060843
78. Singh V, Lee G, Son H, Koh H, Kim ES, Unno T. Butyrate producers, "The Sentinel of Gut": Their intestinal significance with and beyond butyrate, and prospective use as microbial therapeutics. Front Microbiol. (2022) 13:1103836. doi: 10.3389/fmicb.2022.1103836
79. Deng L, Yang Y, Xu G. Empagliflozin ameliorates type 2 diabetes mellitus-related diabetic nephropathy via altering the gut microbiota. Biochim Biophys Acta Mol Cell Biol Lipids. (2022) 1867:159234. doi: 10.1016/j.bbalip.2022.159234
80. Wang P, Guo R, Bai X, Cui W, Zhang Y, Li H. Sacubitril/Valsartan contributes to improving the diabetic kidney disease and regulating the gut microbiota in mice. Front Endocrinol. (2022) 13:1034818. doi: 10.3389/fendo.2022.1034818
81. Jia X, Lu Y, Zheng X, Tang R, Chen W. Targeted therapies for lupus nephritis: Current perspectives and future directions. Chin Med J (Engl). (2024) 137:34–43. doi: 10.1097/cm9.0000000000002959
82. Wang A, Zhao J, Qin Y, Zhang Y, Xing Y, Wang Y. Alterations of the gut microbiota in the lupus nephritis: a systematic review. Ren Fail. (2023) 45:2285877. doi: 10.1080/0886022x.2023.2285877
83. Azzouz D, Omarbekova A, Heguy A, Schwudke D, Gisch N, Rovin BH. Lupus nephritis is linked to disease-activity associated expansions and immunity to a gut commensal. Ann Rheumatol Dis. (2019) 78:947–56. doi: 10.1136/annrheumdis-2018-214856
84. Zhang SX, Wang J, Chen JW, Zhang MX, Zhang YF, Hu FY. The level of peripheral regulatory T cells is linked to changes in gut commensal microflora in patients with systemic lupus erythematosus. Ann Rheumatol Dis. (2021) 80:e177. doi: 10.1136/annrheumdis-2019-216504
85. Mu Q, Zhang H, Liao X, Lin K, Liu H, Edwards MR. Control of lupus nephritis by changes of gut microbiota. Microbiome. (2017) 5:73. doi: 10.1186/s40168-017-0300-8
86. Valiente GR, Munir A, Hart ML, Blough P, Wada TT, Dalan EE. Gut dysbiosis is associated with acceleration of lupus nephritis. Sci Rep. (2022) 12:152. doi: 10.1038/s41598-021-03886-5
87. Hosseini S, Mahmoudi M, Rezaieyazdi Z, Shapouri-Moghaddam A, Hosseinzadeh A, Arab FL. Lupus mice derived mesenchymal stromal cells: Beneficial or detrimental on SLE disease outcome. Int Immunopharmacol. (2024) 126:111306. doi: 10.1016/j.intimp.2023.111306
88. Li Y, Tang D, Yin L, Dai Y. New insights for regulatory T cell in lupus nephritis. Autoimmun Rev. (2022) 21:103134. doi: 10.1016/j.autrev.2022.103134
89. Lerkvaleekul B, Apiwattanakul N, Tangnararatchakit K, Jirapattananon N, Srisala S, Vilaiyuk S. Associations of lymphocyte subpopulations with clinical phenotypes and long-term outcomes in juvenile-onset systemic lupus erythematosus. PloS One. (2022) 17:e0263536. doi: 10.1371/journal.pone.0263536
90. Wang J, Zhu M, Jiao C, Xu X, Xu F, Liang D. Association of regulatory T cells with renal outcomes in patients with proliferative lupus nephritis. Lupus. (2023) 32:1237–44. doi: 10.1177/09612033231201619
91. Nepal D, Gazeley D. Role of IL-6 and IL-6 targeted therapy in systemic lupus erythematosus. Rheumatology. (2023) 62:3804–10. doi: 10.1093/rheumatology/kead416
92. Grebenciucova E, Vanhaerents S. Interleukin 6: at the interface of human health and disease. Front Immunol. (2023) 14:1255533. doi: 10.3389/fimmu.2023.1255533
93. Xu L, Li Y, Wu X. IgA vasculitis update: Epidemiology, pathogenesis, and biomarkers. Front Immunol. (2022) 13:921864. doi: 10.3389/fimmu.2022.921864
94. Williams CEC, Lamond M, Marro J, Chetwynd AJ, Oni L. A narrative review of potential drug treatments for nephritis in children with IgA vasculitis (HSP). Clin Rheumatol. (2023) 42:3189–200. doi: 10.1007/s10067-023-06781-8
95. Ding L, Chen X, Cheng H, Zhang T, Li Z. Advances in IgA glycosylation and its correlation with diseases. Front Chem. (2022) 10:974854. doi: 10.3389/fchem.2022.974854
96. Tan J, Tang Y, Xu Y, Yan S, Xu Y, Tan L. The clinicopathological characteristics of Henoch-Schönlein Purpura nephritis with presentation of nephrotic syndrome. Kidney Blood Pressure Res. (2019) 44:754–64. doi: 10.1159/000501459
97. Pillebout E. IgA vasculitis and IgA nephropathy: same disease? J Clin Med. (2021) 10. doi: 10.3390/jcm10112310
98. He JW, Zhou XJ, Lv JC, Zhang H. Perspectives on how mucosal immune responses, infections and gut microbiome shape IgA nephropathy and future therapies. Theranostics. (2020) 10:11462–78. doi: 10.7150/thno.49778
99. Li Q, Yuan X, Shi S, Liu L, Lv J, Zhu L. Zonulin, as a marker of intestinal permeability, is elevated in IgA nephropathy and IgA vasculitis with nephritis. Clin Kidney J. (2023) 16:184–91. doi: 10.1093/ckj/sfac214
100. Kharrazian D, Herbert M, Lambert J. The relationships between intestinal permeability and target antibodies for a spectrum of autoimmune diseases. Int J Mol Sci. (2023) 24. doi: 10.3390/ijms242216352
101. Wang JJ, Xu Y, Liu FF, Wu Y, Samadli S, Wu YF. Association of the infectious triggers with childhood Henoch-Schonlein purpura in Anhui province, China. J Infect Public Health. (2020) 13:110–7. doi: 10.1016/j.jiph.2019.07.004
102. Li Y, Zhang X, Liu H, Li G, Guan W, Zhang T. Severe gastrointestinal involvement in pediatric IgA vasculitis: a retrospective single-center cohort study in China. Front Pediatr. (2023) 11:1194214. doi: 10.3389/fped.2023.1194214
103. Karadağ ş G, Tanatar A, Sönmez HE, çakmak F, Kıyak A, Yavuz S. The clinical spectrum of Henoch-Schönlein purpura in children: a single-center study. Clin Rheumatol. (2019) 38:1707–14. doi: 10.1007/s10067-019-04460-1
104. Hu X, Fan R, Song W, Qing J, Yan X, Li Y. Landscape of intestinal microbiota in patients with IgA nephropathy, IgA vasculitis and Kawasaki disease. Front Cell Infect Microbiol. (2022) 12:1061629. doi: 10.3389/fcimb.2022.1061629
105. Wang JK, Yan B, Zhao JM, Yuan LP. Effect of gut microbiota from Henoch-Schönlein Purpura patients on acid-sensitive ion channel 3 expression and intestinal motility in germ-free rats. BMC Pediatr. (2021) 21:536. doi: 10.1186/s12887-021-03013-3
106. Li M, Wang X, Lin X, Bian X, Jing R, Frelinger A. Comparison and analysis of gut microbiota in children with IgA vasculitis with different clinical symptoms. Front Pediatr. (2021) 9:800677. doi: 10.3389/fped.2021.800677
107. Tan J, Zhong Z, Tang Y, Qin W. Intestinal dysbiosis featuring abundance of Streptococcus associates with Henoch-Schönlein purpura nephritis (IgA vasculitis with nephritis) in adult. BMC Nephrol. (2022) 23:10. doi: 10.1186/s12882-021-02638-x
108. Boer CG, Radjabzadeh D, Medina-Gomez C, Garmaeva S, Schiphof D, Arp P. Intestinal microbiome composition and its relation to joint pain and inflammation. Nat Commun. (2019) 10:4881. doi: 10.1038/s41467-019-12873-4
109. Xiao C, Xiao P, Li X, Huang G, Li H, Chen Y. Streptococcus may aggravate inflammatory damage in chronic nephritis via the chemotaxis of Th22 cells. Am J Transl Res. (2019) 11:7432–40.
110. Chen YH, Bai J, Wu D, Yu SF, Qiang XL, Bai H. Association between fecal microbiota and generalized anxiety disorder: Severity and early treatment response. J Affect Disord. (2019) 259:56–66. doi: 10.1016/j.jad.2019.08.014
111. Hu X, Du J, Xie Y, Huang Q, Xiao Y, Chen J. Fecal microbiota characteristics of Chinese patients with primary IgA nephropathy: a cross-sectional study. BMC Nephrol. (2020) 21:97. doi: 10.1186/s12882-020-01741-9
112. Hu X, Ouyang S, Xie Y, Gong Z, Du J. Characterizing the gut microbiota in patients with chronic kidney disease. Postgrad Med. (2020) 132:495–505. doi: 10.1080/00325481.2020.1744335
113. Wu IW, Lin CY, Chang LC, Lee CC, Chiu CY, Hsu HJ. Gut microbiota as diagnostic tools for mirroring disease progression and circulating nephrotoxin levels in chronic kidney disease: discovery and validation study. Int J Biol Sci. (2020) 16:420–34. doi: 10.7150/ijbs.37421
114. Zhu Y, He C, Li X, Cai Y, Hu J, Liao Y. Gut microbiota dysbiosis worsens the severity of acute pancreatitis in patients and mice. J Gastroenterol. (2019) 54:347–58. doi: 10.1007/s00535-018-1529-0
115. Xu W, Ishii Y, Rini DM, Yamamoto Y, Suzuki T. Microbial metabolite n-butyrate upregulates intestinal claudin-23 expression through SP1 and AMPK pathways in mouse colon and human intestinal Caco-2 cells. Life Sci. (2023) 329:121952. doi: 10.1016/j.lfs.2023.121952
116. Li Y, Li H, Wang R, Yu Y, Liu X, Tian Z. Protective effect of sodium butyrate on intestinal barrier damage and uric acid reduction in hyperuricemia mice. Biomed Pharmacother. (2023) 161:114568. doi: 10.1016/j.biopha.2023.114568
117. Liu J, Gu QH, Cui Z, Zhao MH, Jia XY. Short-chain fatty acids ameliorate experimental anti-glomerular basement membrane disease. Clin Immunol. (2024) 259:109903. doi: 10.1016/j.clim.2024.109903
118. Hastings MC, Rizk DV, Kiryluk K, Nelson R, Zahr RS, Novak J. IgA vasculitis with nephritis: update of pathogenesis with clinical implications. Pediatr Nephrol. (2022) 37:719–33. doi: 10.1007/s00467-021-04950-y
119. Song Y, Huang X, Yu G, Qiao J, Cheng J, Wu J. Pathogenesis of IgA vasculitis: an up-to-date review. Front Immunol. (2021) 12:771619. doi: 10.3389/fimmu.2021.771619
120. Vivarelli M, Gibson K, Sinha A, Boyer O. Childhood nephrotic syndrome. Lancet. (2023) 402:809–24. doi: 10.1016/s0140-6736(23)01051-6
121. Hahn D, Samuel SM, Willis NS, Craig JC, Hodson EM. Corticosteroid therapy for nephrotic syndrome in children. Cochrane Database Syst Rev. (2024) 8:Cd001533. doi: 10.1002/14651858.CD001533.pub7
122. Zhang L, Chen L. Systematic review of the efficacy and safety of traditional Chinese medicine granules associated with hormone when treating primary nephrotic syndrome in children. Contrast Media Mol Imaging. (2022) 2022:2520367. doi: 10.1155/2022/2520367
123. Cui H, Fu FQ, Liu B, Liu WJ, Liu YN. Herbal medicine "Shulifenxiao" Formula for nephrotic syndrome of refractory idiopathic membranous nephropathy. Front Pharmacol. (2021) 12:675406. doi: 10.3389/fphar.2021.675406
124. Shi L, Deng Y, Luo D, Li L, Kuang X, Qi A. Exploration of the possible mechanisms of Ling Gui Zhu Gan decoction in nephrotic syndrome based on network pharmacology, molecular docking and molecular dynamics simulation. Med (Baltimore). (2023) 102:e34446. doi: 10.1097/md.0000000000034446
125. Zhang WN, Yang L, He SS, Qin XM, Li AP. Metabolomics coupled with integrative pharmacology reveal the protective effect of FangjiHuangqi Decoction against adriamycin-induced rat nephropathy model. J Pharm Biomed Anal. (2019) 174:525–33. doi: 10.1016/j.jpba.2019.05.023
126. Wang Y, Ma Y, Ke Y, Jiang X, Liu J, Xiao Y. Fangji Huangqi decoction ameliorates membranous nephropathy through the upregulation of BNIP3-mediated mitophagy. J Ethnopharmacol. (2024) 324:117734. doi: 10.1016/j.jep.2024.117734
127. Liu Z, Shang Q, Li H, Fang D, Li Z, Huang Y. Exploring the possible mechanism(s) underlying the nephroprotective effect of Zhenwu Decoction in diabetic kidney disease: An integrated analysis. Phytomedicine. (2023) 119:154988. doi: 10.1016/j.phymed.2023.154988
128. Zhang N, Jiang H, Dai H, Huang S, Zhao Q, Zhang N. Efficacy and safety of Mahuang Fuzi and Shenzhuo Decoction for treatment of primary membranous nephropathy: a multicenter prospective trial. Ren Fail. (2024) 46:2320834. doi: 10.1080/0886022x.2024.2320834
129. Kang Y, Feng D, Law HK, Qu W, Wu Y, Zhu GH. Compositional alterations of gut microbiota in children with primary nephrotic syndrome after initial therapy. BMC Nephrol. (2019) 20:434. doi: 10.1186/s12882-019-1615-4
130. Tsuji S, Suruda C, Hashiyada M, Kimata T, Yamanouchi S, Kitao T. Gut microbiota dysbiosis in children with relapsing idiopathic nephrotic syndrome. Am J Nephrol. (2018) 47:164–70. doi: 10.1159/000487557
131. Yamaguchi T, Tsuji S, Akagawa S, Akagawa Y, Kino J, Yamanouchi S. Clinical significance of probiotics for children with idiopathic nephrotic syndrome. Nutrients. (2021) 13. doi: 10.3390/nu13020365
132. Chen Y, Deng Y, Ni Z, Chen N, Chen X, Shi W. Efficacy and safety of traditional chinese medicine (Shenqi particle) for patients with idiopathic membranous nephropathy: a multicenter randomized controlled clinical trial. Am J Kidney Dis. (2013) 62:1068–76. doi: 10.1053/j.ajkd.2013.05.005
133. Feng D, Li XR, Wang ZY, Gu NN, Zhang SX, Li CF. Integrated UPLC-MS and network pharmacology approach to explore the active components and the potential mechanism of Yiqi Huoxue decoction for treating Nephrotic syndrome. Front Pharmacol. (2021) 12:775745. doi: 10.3389/fphar.2021.775745
134. Gao Y, Dai H, Zhang N, Jiang H, Zhang Z, Feng Z. The ameliorative effect of Mahuang Fuzi and Shenzhuo decoction on membranous nephropathy of rodent model is associated with autophagy and Wnt/β-catenin pathway. Front Pharmacol. (2022) 13:820130. doi: 10.3389/fphar.2022.820130
135. Xu B, Yao M, Liu Z, Zhang S, Wang B, Han Y. Metabolomic Analysis of Stephania tetrandra-Astragalus membranaceus Herbal Pair-Improving Nephrotic Syndrome Identifies Activation of IL-13/STAT6 Signaling Pathway. Pharmaceuticals. (2023) 16. doi: 10.3390/ph16010088
136. Qu L, Jiao B. The interplay between immune and metabolic pathways in kidney disease. Cells. (2023) 12. doi: 10.3390/cells12121584
137. Wang F, Liu C, Ren L, Li Y, Yang H, Yu Y. Sanziguben polysaccharides improve diabetic nephropathy in mice by regulating gut microbiota to inhibit the TLR4/NF-κB/NLRP3 signalling pathway. Pharm Biol. (2023) 61:427–36. doi: 10.1080/13880209.2023.2174145
138. Piao S, Zhu Z, Tan S, Zhan H, Rong X, Guo J. An integrated fecal microbiome and metabolome in the aged mice reveal anti-aging effects from the intestines and biochemical mechanism of FuFang zhenshu TiaoZhi(FTZ). Biomed Pharmacother. (2020) 121:109421. doi: 10.1016/j.biopha.2019.109421
139. Yang YQ, Tan HB, Zhang XY, Zhang YZ, Lin QY, Huang MY. The Chinese medicine Fufang Zhenzhu Tiaozhi capsule protects against renal injury and inflammation in mice with diabetic kidney disease. J Ethnopharmacol. (2022) 292:115165. doi: 10.1016/j.jep.2022.115165
140. Lan T, Xu T, Fu Y, Jiang S, Liang X, Yu Z. Fufang Zhenzhu Tiaozhi capsule prevents intestinal inflammation and barrier disruption in mice with non-alcoholic steatohepatitis. Front Endocrinol. (2022) 13:864703. doi: 10.3389/fendo.2022.864703
141. Lan T, Tang T, Li Y, Duan Y, Yuan Q, Liu W. FTZ polysaccharides ameliorate kidney injury in diabetic mice by regulating gut-kidney axis. Phytomedicine. (2023) 118:154935. doi: 10.1016/j.phymed.2023.154935
142. Zhang LX, Li CX, Kakar MU, Khan MS, Wu PF, Amir RM. Resveratrol (RV): A pharmacological review and call for further research. Biomed Pharmacother. (2021) 143:112164. doi: 10.1016/j.biopha.2021.112164
143. Cai TT, Ye XL, Li RR, Chen H, Wang YY, Yong HJ. Resveratrol modulates the gut microbiota and inflammation to protect against diabetic nephropathy in mice. Front Pharmacol. (2020) 11:1249. doi: 10.3389/fphar.2020.01249
144. Meijers B, Farré R, Dejongh S, Vicario M, Evenepoel P. Intestinal barrier function in chronic kidney disease. Toxins. (2018) 10. doi: 10.3390/toxins10070298
145. Zhou K, Zhang J, Liu C, Ou L, Wang F, Yu Y. Sanziguben polysaccharides inhibit diabetic nephropathy through NF-κB-mediated anti-inflammation. Nutr Metab. (2021) 18:81. doi: 10.1186/s12986-021-00601-z
146. Hua Q, Han Y, Zhao H, Zhang H, Yan B, Pei S. Punicalagin alleviates renal injury via the gut-kidney axis in high-fat diet-induced diabetic mice. Food Funct. (2022) 13:867–79. doi: 10.1039/d1fo03343c
147. Niu X, Song H, Xiao X, Yu J, Yu J, Yang Y. Tectoridin alleviates lipopolysaccharide-induced inflammation via inhibiting TLR4-NF-κB/NLRP3 signaling in vivo and in vitro. Immunopharmacol. Immunotoxicol. (2022) 44:641–55. doi: 10.1080/08923973.2022.2073890
148. Wakamatsu T, Yamamoto S, Ito T, Sato Y, Matsuo K, Takahashi Y. Indoxyl Sulfate Promotes Macrophage IL-1β Production by Activating Aryl Hydrocarbon Receptor/NF-κ/MAPK Cascades, but the NLRP3 inflammasome Was Not Activated. Toxins. (2018) 10. doi: 10.3390/toxins10030124
149. Li P, Chen Y, Liu J, Hong J, Deng Y, Yang F. Efficacy and safety of tangshen formula on patients with type 2 diabetic kidney disease: a multicenter double-blinded randomized placebo-controlled trial. PloS One. (2015) 10:e0126027. doi: 10.1371/journal.pone.0126027
150. Zhao T, Zhang H, Yin X, Zhao H, Ma L, Yan M. Tangshen formula modulates gut Microbiota and reduces gut-derived toxins in diabetic nephropathy rats. Biomed Pharmacother. (2020) 129:110325. doi: 10.1016/j.biopha.2020.110325
151. He M, Wei W, Zhang Y, Xiang Z, Peng D, Kasimumali A. Gut microbial metabolites SCFAs and chronic kidney disease. J Transl Med. (2024) 22:172. doi: 10.1186/s12967-024-04974-6
152. Lian Y, Zhu M, Chen J, Yang B, Lv Q, Wang L. Characterization of a novel polysaccharide from Moutan Cortex and its ameliorative effect on AGEs-induced diabetic nephropathy. Int J Biol Macromol. (2021) 176:589–600. doi: 10.1016/j.ijbiomac.2021.02.062
153. Zhang M, Yang L, Zhu M, Yang B, Yang Y, Jia X. Moutan Cortex polysaccharide ameliorates diabetic kidney disease via modulating gut microbiota dynamically in rats. Int J Biol Macromol. (2022) 206:849–60. doi: 10.1016/j.ijbiomac.2022.03.077
154. Liu J, Qu J, Chen H, Ge P, Jiang Y, Xu C. The pathogenesis of renal injury in obstructive jaundice: A review of underlying mechanisms, inducible agents and therapeutic strategies. Pharmacol Res. (2021) 163:105311. doi: 10.1016/j.phrs.2020.105311
155. Wang X, Zhao L, Ajay AK, Jiao B, Zhang X, Wang C. QiDiTangShen granules activate renal nutrient-sensing associated autophagy in db/db mice. Front Physiol. (2019) 10:1224. doi: 10.3389/fphys.2019.01224
156. Wei H, Wang L, An Z, Xie H, Liu W, Du Q. QiDiTangShen granules modulated the gut microbiome composition and improved bile acid profiles in a mouse model of diabetic nephropathy. Biomed Pharmacother. (2021) 133:111061. doi: 10.1016/j.biopha.2020.111061
157. Zhao J, Zhang QL, Shen JH, Wang K, Liu J. Magnesium lithospermate B improves the gut microbiome and bile acid metabolic profiles in a mouse model of diabetic nephropathy. Acta Pharmacol Sin. (2019) 40:507–13. doi: 10.1038/s41401-018-0029-3
158. Wang KJ, Zhao JL. Corn silk (Zea mays L.), a source of natural antioxidants with α-amylase, α-glucosidase, advanced glycation and diabetic nephropathy inhibitory activities. Biomed Pharmacother. (2019) 110:510–7. doi: 10.1016/j.biopha.2018.11.126
159. Dong W, Zhao Y, Li X, Huo J, Wang W. Corn silk polysaccharides attenuate diabetic nephropathy through restoration of the gut microbial ecosystem and metabolic homeostasis. Front Endocrinol. (2023) 14:1232132. doi: 10.3389/fendo.2023.1232132
160. Feng M, Yuan W, Zhang R, Fu P, Wu T. Chinese herbal medicine Huangqi type formulations for nephrotic syndrome. Cochrane Database Syst Rev. (2013) 2013:Cd006335. doi: 10.1002/14651858.CD006335.pub3
161. Yang F, Qu Q, Zhao C, Liu X, Yang P, Li Z. Paecilomyces cicadae-fermented Radix astragali activates podocyte autophagy by attenuating PI3K/AKT/mTOR pathways to protect against diabetic nephropathy in mice. Biomed Pharmacother. (2020) 129:110479. doi: 10.1016/j.biopha.2020.110479
162. Zhou Q, Yang F, Li Z, Qu Q, Zhao C, Liu X. Paecilomyces cicadae-fermented Radix astragali ameliorate diabetic nephropathy in mice by modulating the gut microbiota. J Med Microbiol. (2022) 71. doi: 10.1099/jmm.0.001535
163. Li N, Tang H, Wu L, Ge H, Wang Y, Yu H. Chemical constituents, clinical efficacy and molecular mechanisms of the ethanol extract of Abelmoschus manihot flowers in treatment of kidney diseases. Phytother Res. (2021) 35:198–206. doi: 10.1002/ptr.6818
Keywords: nephrotic syndrome, gut microbiota, pathogenesis, traditional chinese medicine, gut, gut-kidney axis
Citation: Li J, Xu Y, Sun T, Zhang X, Liang H, Lin W, Yu H, Yang B and Yang H (2024) Exploration of the pathogenesis of nephrotic syndrome and traditional Chinese medicine intervention based on gut microbiota. Front. Immunol. 15:1430356. doi: 10.3389/fimmu.2024.1430356
Received: 09 May 2024; Accepted: 18 November 2024;
Published: 09 December 2024.
Edited by:
Xiaoxin Wang, Georgetown University Medical Center, United StatesReviewed by:
Dan-Qian Chen, Northwest University, ChinaXiaoyong Yu, Shaanxi Provincial Hospital of Traditional Chinese Medicine, China
Copyright © 2024 Li, Xu, Sun, Zhang, Liang, Lin, Yu, Yang and Yang. This is an open-access article distributed under the terms of the Creative Commons Attribution License (CC BY). The use, distribution or reproduction in other forums is permitted, provided the original author(s) and the copyright owner(s) are credited and that the original publication in this journal is cited, in accordance with accepted academic practice. No use, distribution or reproduction is permitted which does not comply with these terms.
*Correspondence: Hongtao Yang, dGp0Y21odEAxMjYuY29t
†These authors have contributed equally to this work and share first authorship